- 1Laboratoire Chrono-Environnement, Université de Franche-Comté, Besançon, France
- 2Dipartimento di Chimica, Università degli Studi di Bari Aldo Moro, Bari, Italy
In Europe, chemical oxygen demand (COD) and biochemical oxygen demand (BOD5) are used as organic indicators for the analytical characterization of laundry water. However, the values of these indicators are derived from one-off analytical measurements at a defined frequency. In addition, there are few data on the temporal variability of COD and BOD5 parameters and on water biodegradability beyond 5 days. In this work, samples of physicochemical treated wastewater were collected for 1 year and their content in COD and BOD5 measured, with BOD monitored over 35 days. The results showed that, for the 24 samples studied, the average and standard deviation values were 835 ± 176 mg/L for COD and 445 ± 114 mg/L for BOD5, indicating a biodegradability index of 2.0 ± 0.4. The interpretation of the data also indicated that the concentrations were close to emission limit value, particularly for BOD5. BOD21 values were particularly high compared to the initial COD, indicating the biodegradability of water. Laundry effluents were also rich in substances very quickly biodegradable, which has been demonstrated by the values of the ratio between BOD7 and BOD5. However, a significant proportion of surfactants is not degraded, as shown by measurements of total organic carbon and anionic surfactant analysis. The results of this study could help the industrial partner involved in our project to guide its choice of the complementary method to better treat wastewater and to reduce its organic load.
1 Introduction
Water contamination with various contaminants including metals, pesticides, fertilizers, surfactants, disinfectants, phenolic substances, dyes, pharmaceuticals, hormones, endocrine disrupters, and other emerging pollutants is a major current problem affecting water quality (Aonghusa and Gray, 2002; Wang et al., 2015; Peña-Guzmán et al., 2019; Patel et al., 2020; Georgin et al., 2023; Warren-Vega et al., 2023). These substances are released into the environment via diverse routes, e.g., agricultural activities, industries, hospitals and individual households, and are found in various environmental compartments despite the decontamination treatments applied to both industrial or urban water sources (Rizzo et al., 2019; Abily et al., 2023). Urban wastewater treatment plants are a main qualitative and quantitative point source of contaminants, notably for contaminants of emerging concern, released to surface water bodies (Rizzo et al., 2019). More than ever, it is necessary to treat upstream industrial pollution as much as possible before it reaches urban treatment plants.
Among anthropic activities, the industrial laundry sector is considered one of the largest users of water, and therefore one of the largest producers of wastewater (Aonghusa and Gray, 2002; Jayanto et al., 2011; Braga and Varesche, 2014; Dąbkowska, 2017; Swartz et al., 2017; Oyedotun and Ally, 2021; Alhinai, 2022; Procházková and Máša, 2022). Indeed, industrial laundries are establishments that treat linen in an industrial way by cleaning it with large quantities of water and cleaning products. Although the wastewater is treated on site and complies with the regulations in force, the discharge water still contains a significant amount of inorganic and organic pollutants. This is why this industrial sector is responsible for discharging oxidizable materials and chemical substances into urban treatment plants in cities where laundries are located, and consequently into the environment (Sheth et al., 2017; Hu et al., 2021; Santiago et al., 2021; Kumar et al., 2022; Singh et al., 2022; Melián et al., 2023; Tomšič et al., 2023). In addition, laundry wastewaters also present high level of turbidity and viscosity, two key parameters used to assess water quality. For example, turbidity in the aquatic environment hinders the passage of light through the water, causing shifts in photosynthetic activity and disrupting the natural balance of flora and fauna (Aonghusa and Gray, 2002; Ho et al., 2021; de; Oliveira Cardoso Nascimento et al., 2021).
To our knowledge, precise data concerning pollution in terms of qualitative and quantitative fluctuations of water discharges from the laundry sector are rarely reported (Braga and Varesche, 2014; Dąbkowska, 2017; Sheth et al., 2017; Alhinai, 2022). In general, to determine the degree of pollution, regulations require the one-off measurement of some water quality indicators (Aguilar-Torrejón et al., 2023), such as pH, temperature, suspended solids, nitrogen compounds, chemical oxygen demand and biochemical oxygen demand, the latter two being the most monitored in the laundry water. These parameters are routine analyses for wastewater and water in France (Journal Officiel de la République Française of 6 October 2017; Annex VII for industrial laundries; Morin-Crini and Crini, 2017).
The chemical oxygen demand (COD) parameter is used to evaluate the pollutant load of a water, by measuring the equivalent amount of oxygen needed to chemically oxidize the compounds present in the water analyzed (Sibil et al., 2014; Morin-Crini and Crini, 2017; Tomšič et al., 2023). COD is the most used and most important indicator of pollution to characterize an industrial effluent. Biochemical oxygen demand (BOD) refers the amount of dissolved oxygen needed to break down organic matter in water. Indeed, it measures the amount of dissolved oxygen that is required for the biochemical oxidation of the organic compounds in 5 days (BOD5) using a standardized protocol (5-day BOD test). The measurement of the COD using a strong oxidizing agent is a straightforward, rapid, and highly precise method commonly employed to assess the extent of water pollution. In contrast, the BOD5 determination is more complicated and time consuming, involving a 5-day incubation period. The BOD5 procedure also requires considerable experience and skill to achieve reproducible results (Sibil et al., 2014; Morin-Crini and Crini, 2017; Tomšič et al., 2023).
It is important to note that the COD measurement encompass both all biodegradable and non-biodegradable oxidizable compounds (Aguilar-Torrejón et al., 2023). Indeed, the amount of oxygen consumed during the oxidation reaction is due not only to organic compounds but also to the inorganic charge that may be oxidized. For this reason, the measurement of COD is generally coupled with that of BOD5 because this quantity allows to evaluate the biodegradable fraction of the carbon compounds present in waters. Finally, the simultaneous measurement of these two parameters is relevant because it makes it possible to evaluate the biochemical degradation of substances in water (Jayanto et al., 2011; Morin-Crini and Crini, 2017; Swartz et al., 2017; Aguilar-Torrejón et al., 2023; Tomšič et al., 2023). The ratio of COD to BOD5 provides a biodegradability index, which gives information on the biodegradability of water, and the source and origin of organic pollution (Ho et al., 2021). In Europe, the water reference value is 2.5 (Directive 91/271/CEE, 21-03-1994; Morin-Crini and Crini, 2017). The higher the value, the worse the biodegradability of the water. In France, the biodegradability index plays an indirect yet significant role in calculating the taxes imposed on manufacturers, aligning with the polluter pays principle. By considering the biodegradability of substances released into the environment, this approach ensures that manufacturers are accountable for the environmental impact of their activities, promoting a fair distribution of the financial responsibility associated with pollution. In wastewater engineering, the value of this biodegradability index is also fundamental and helps guide the choice of the complementary method to better treat wastewater.
In laundries, COD and BOD5 are generated using numerous and diverse commercial formulations containing organic and mineral substances such as surface active agents (surfactants, detergents), builders, disinfectants, stain removers, alkalis, oxidants, bleaching agents, enzymes, softeners, optical brighteners, preservatives, corrosion inhibitors, perfumes, and waterproofing in the industrial process (Nicolaidis and Vyrides, 2014; Sheth et al., 2017; Swartz et al., 2017; Moharir et al., 2020; Kogut et al., 2021; Alhinai, 2022; Lacalamita et al., 2023). Some datasheets of the used products indicate COD values of several hundred grams, without any precise indication of the type of substance (Sumisha et al., 2015; Lutterbeck et al., 2020; Ho et al., 2021; Lacalamita et al., 2023). In addition, the biodegradability of these substances, and therefore of active substances, is rarely indicated.
Currently in France, only the COD and BOD5 parameters are used as organic indicators for the monitoring of organic pollution present in wastewater and treated water. Nevertheless, it is important to note that these values represent specific points in time and are subject to quarterly analysis frequency, as stipulated in the Arrêté Préfectoral (French administrative document) to be complied with by each industry. Furthermore, no data is available on the temporal variability of these two parameters or on the long-term biodegradability of laundry water. Two others relevant parameters for characterizing water, especially within the laundry industry, include the measurement of total organic carbon (TOC) and anionic surfactants (AS). These analytical parameters are crucial in assessing the organic content and surfactant levels, respectively, providing valuable insights into the overall water quality and its compatibility with the specific requirements of the laundry sector. Unlike COD, TOC provides values only referred to the carbon content of organic load in water. Anionic surfactants are often the most used in commercial formulations for their degreasing properties (Morin-Crini and Crini, 2017; Swartz et al., 2017; Collivignarelli et al., 2019; Hu et al., 2021). However, these two parameters are not requested by the French Water Agency or any other regulatory (Morin-Crini and Crini, 2017).
With the progressive implementation of various European directives emanating from the 2000 Water Framework Directive (Directive 2000/60/EC of the European Parliament and of the Council of 23 October 2000) and their transposition into French and Italian laws, the laundry sector is required to implement actions to reduce the flux of certain substances, e.g., surfactants, alkylphenols and metals, and parameters, particularly COD and BOD5, discharged into the environment by 2028 (a decree issued by the French Ministry of Ecology and Solidarity, published in the Journal Officiel de la République Française of 6 October 2017). In addition, a recent French law called anti-waste for a circular economy (AGEC Law–loi anti-gaspillage pour une économie circulaire; decree no. 2022-336 of 10 March 2022) aims to change the production and consumption model in order to preserve natural resources and biodiversity. This law encourages manufacturers to continue their efforts to reduce their environmental impact, notably by reducing COD and BOD5 concentrations. However, before considering further treatments on these two water quality indicators, it is essential, on the one hand, to know quantitatively the concentrations on a dataset larger than the four analyses carried out yearly by the industrial partner of this study to assess their temporal variability and, on the other hand, to have information on the biodegradability of the discharge water on long term.
In this context, we collected 24 discharge water samples from a French industrial laundry over a 12-month period and analyzed their content in COD and BOD5, with the objective of assessing the biodegradability of water and the temporal variability of these water quality indicators. From these measurements, an average biodegradability index was determined. Biodegradability tests over several days (5, 7, 14, 21 and 35 days) were also performed and correlated with TOC and AS measurements. This is the first time such measures have been reported. This work also attempts to answer the following questions related to laundry greywater:
• What is the temporal variability of COD and BOD5 parameters over a year of water sampling?
• Are these parameters sufficient to characterize the organic load of discharged water?
• Is water biodegradation fast?
• Is it possible to quantify the non-biodegradable fraction of water?
2 Experimental
2.1 Industrial site
This study was carried out in collaboration with UNAP, an industrial laundry located in Pontarlier (Doubs, France) and specialized in washing linen mainly from industries, local authorities, restaurants, hotels, hospitals, and retirement homes. The company processes 6,000 to 7,000 kg of laundry per day and uses 120–130 m3 of water per day (Lacalamita et al., 2023). The composition and concentration of the wastewater vary according to the type of linen, the washing cycles, and the used products (Bajpai and Tyagi, 2007; Yu et al., 2008; Zavala and Estrada, 2016; Dąbkowska, 2017; Lacalamita et al., 2023). As a result, different types of wastewaters from industrial washing process are produced and collected in a buffer tank. The wastewater is sent to a physicochemical treatment plant whose the objective is to reduce the quantities of COD and BOD5, the two main concerns of the laundry. For reasons of confidentiality, the wastewater treatment carried out by the industrial partner in the wastewater treatment plant cannot be detailed. Once treated, if water complies with current regulations, it is discharged into the sewage system to join the wastewater treatment plant of the city. Table 1 shows the legal limits for different parameters present in the company’s discharge waters and the frequency of analyses, as defined by their specific regulation, as well as an example of an annual declaration for the administrative authorities.
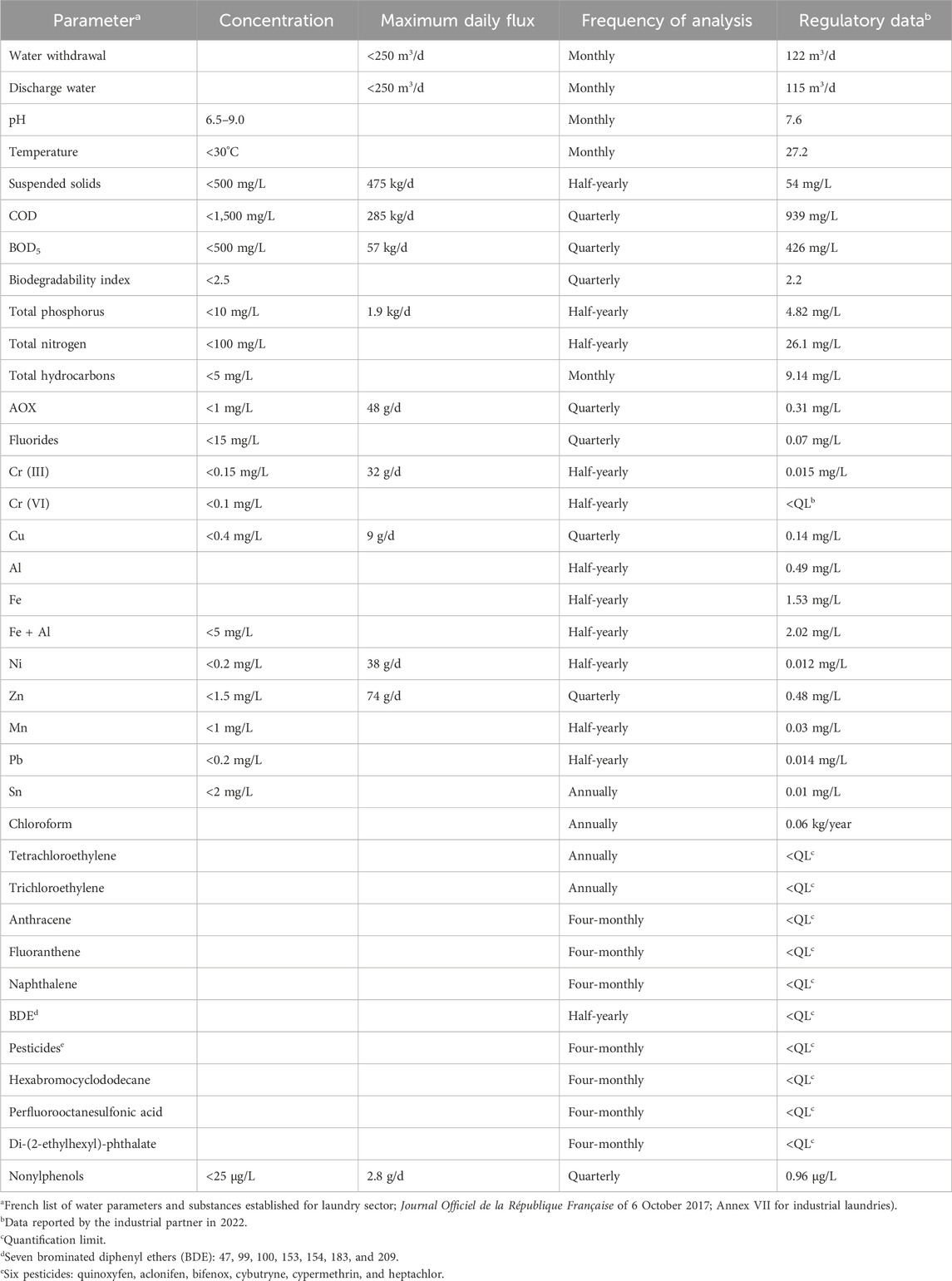
Table 1. Regulatory release limit values expressed in concentration and maximum daily flux for different water quality indicators and substances present in the discharge water, as defined by the regulations for the industrial, frequency of analysis and example of official data declared by the partner of this study.
2.2 Industrial water
The industrial water studied comes from the partner’s physico-chemical wastewater treatment plant and is the same as that sent to the city’s urban treatment plant. This discharge of water is carried out under an agreement that specifies exactly the concentration or flux values not to be exceeded described in Table 1. These values are determined from analytical data from a given analytical frequency. It is important to note that the industrial waters on which we have worked contain practically no nitrogen pollution or phosphate pollution, as we have recently shown (Lacalamita et al., 2023). This indicates that the pollution of laundry water is essentially of carbon origin. Each sample, taken every 15 days, comes from an automatic sampler and is representative of the activity in a whole day in accordance with the regulations. 24 samples were monitored over a 1-year period to determine the COD and BOD5 levels of pollution. These samples, which are turbid, viscous and slightly oily (Figure 1), are the same as those sent by the industrial partner to an accredited laboratory as part of its regulatory self-monitoring. The results were expressed as concentrations (in mg/L) and total flux (in g/d or kg/d), to facilitate comparison with the limits enacted by the law and the data provided by the industrial partner.
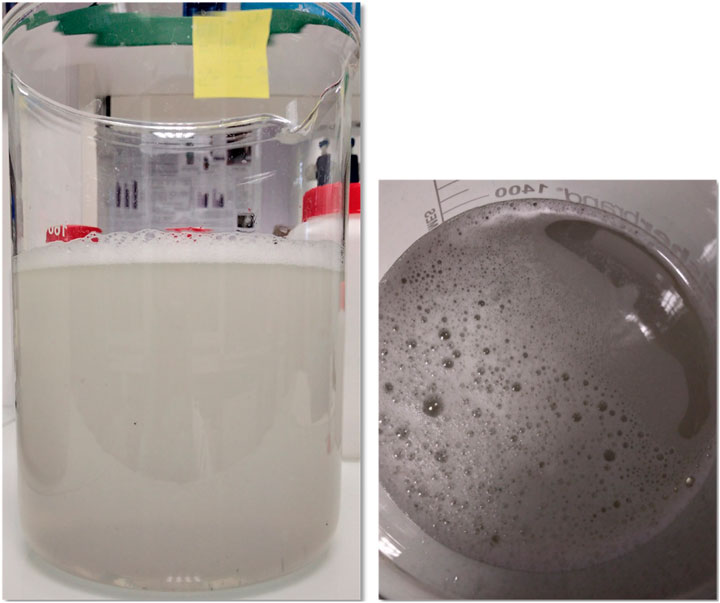
Figure 1. Industrial water sample sent for analysis to an accredited laboratory by the industrial partner. These photographs illustrate the turbid, viscous and foaming nature of waters that are discharged respecting the regulations in force.
2.3 Analytical methods
Analytical methods for determining water parameters for each sample use standardized French protocols (Morin-Crini and Crini, 2017; Lacalamita et al., 2023): COD was assessed following the protocol NF T90-101 (oximetry, internal method), BOD with the respiration method following the standard NF EN ISO 5815-1 (oximetry, internal method), SS by gravimetry (NF EN 872), TOC using oxidation and infrared detection (NF EN 1484), and AS using an internal method.
The determination of the COD is based on the oxidation of the reducing materials contained in the sample by a potassium dichromate solution in an acidic medium and the use of colorimetric measurement (Lacalamita et al., 2023). The detailed protocol is as follows: a fraction of the sample is placed in a digestion tube and 5 mL of a potassium dichromate solution is added, as well as 15 mL of a silver sulphate solution in an acid medium. The tube is then placed in a mineralizer (model FB15006, Fisher Bioblock Scientific Illkirch, France) and heated to 200°C for 2 h following the protocol NF T90-101 (internal method). Once cooled, the excess dichromate is dosed with a titrated solution of Mohr salt in the presence of a colored indicator and the absorbance was measured at 605 nm using a photometer (model COD Vaxio, Aqualytic PCCompact, Dortmund, Germany). The amount of oxygen that was required to neutralize the reducing materials in the sample is then determined to obtain the COD.
The determination of BOD is based on the principle of aerobic degradation of biodegradable organic matter contained in the sample by bacteria (Morin-Crini and Crini, 2017; Lacalamita et al., 2023). A fraction of the sample is placed in contact with bacteria brought by a sewage plant inlet as well as salts and a pH 7.2 buffer, in an incubator in the dark for the desired duration. The incubation temperature is 20°C ± 0.1°C. Thus, the oxygen concentration of the sample is measured at the time 0 (OxiDirect equipment, Aqualytic, Dortmund, Germany). Then, according to the desired incubation period (in our case, 5, 7, 14, 21 and 35 days), the residual oxygen concentration in the sample is measured. The difference then allows access to the amount of oxygen consumed during incubation. A correlation is made to obtain the value of the BODx, x being the duration of incubation. It is crucial to highlight that in France, only 5-day measurements (and more rarely 7-day measurements) are carried out by industrial water users, in accordance with their own specific regulations. In our study, the choice of time values at 5, 7, 14, 21 and 35 days was based on the following considerations:
• BOD5 is the oxidation of pollution that is most rapidly biodegradable. This value with that of the COD allows to calculate the biodegradability index. This index is used by the industrial partner when making its declaration under the polluter pays principle.
• BOD7, which is of course slightly higher or equal to BOD5, provides information on the kinetics of biodegradation of materials. According to the French standard protocol NF EN 1899-1, the values of the BOD7/BOD5 ratio for domestic wastewater and industrial water must be 1.2 and 1, respectively. If this is the case, it is estimated that the effluents are rich in substances very quickly biodegradable.
• After 21 days, the biological oxidation of carbon compounds in a water is almost complete, especially for water of domestic origin. This 21-day period is also necessary for the oxidation of biodegradable nitrogen compounds (this is the case for urban water treatment plants). It allows nitrifying bacteria (which will oxidize ammonia nitrogen into nitrites and then nitrates) to grow despite their slow growth. Oxygen consumption at 21 days (BOD21) is called carbon BOD. If the BOD21 value is less than the COD, it means the presence of a fraction of non-biodegradable organic matter in the analyzed water.
• After 35 days, it is estimated that all biodegradable carbon and nitrogen compounds have been oxidized and assimilated by microorganisms. This duration of 35 days also represents the degradation time of certain hydrocarbon families. Finally, the oxygen consumption corresponding to these two phases (BOD21 and BOD35) is called the ultimate BOD, which corresponds to the non-biodegradable fraction.
TOC analysis is performed by cold chemical oxidation and infrared detection (Analytik Jena, Saint-Aubin, France). The protocol was as follows: a volume of 100 mL of sample is introduced into a tube and acidified with sulfuric acid (pH < 2); prior to analysis, the sample is purged with nitrogen to remove inorganic carbon. The analysis then consists of the chemical oxidation of the sample with sodium persulfate and UV rays. The CO2 produced is detected by the infrared detector and then quantified via an external calibration performed with potassium hydrogen phthalate.
The determination of the anionic surfactant (AS) concentration was as follows: 4 mL of sample is taken and fed into a specific tube containing a standardized dose of chloroform; 500 µL of methylene blue is then added; the tube is manually stirred for 1 min and the analysis is performed by spectrophotometry at a wavelength of 620 nm with a delay of 10 min before performing the reading (reaction time).
The pH of each sample was measured using a portable pH meter (3110 model, WTW, Alès, France). The turbidity was measured in triplicate using a turbidimeter (Aqualytic PCCompact, Dortmund, Germany). The value was measured at 875 nm in formazine nephelometric units (FNU).
3 Results and discussion
3.1 Monitoring of COD and BOD5 parameters
The aim of this study was to quantify the levels of COD and BOD5 present in the discharge waters, the two main concerns for the industrial partner. Figure 2 shows the concentrations of COD and BOD5 (expressed in mg/L) in 24 samples collected during our sampling campaign. The averages and standard deviations obtained from analyzed values, described in Table 2, are 835 ± 176 mg/L for COD and 445 ± 114 mg/L for BOD5. Analysis of median, mean and asymmetric indices showed a positively asymmetrical distribution for COD and BOD5 values, and distributions close to symmetry for the other parameters examined. Collected data also showed a significant variability (Figure 2), which is also confirmed by the high values of the standard deviation. This result indicates a high variability in the water treatment performed by the industrial partner’s wastewater treatment plant for the abatement of COD and BOD5 parameters (Swartz et al., 2017; Lacalamita et al., 2023).
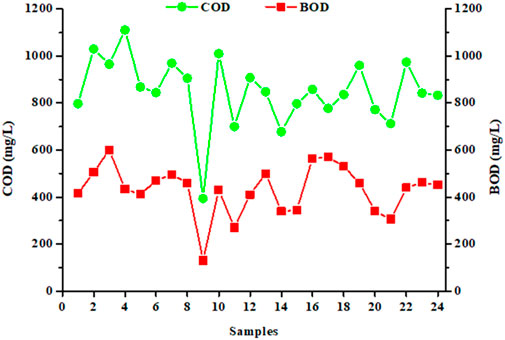
Figure 2. Concentrations of chemical oxygen demand (COD in mg/L) and biochemical oxygen demand (BOD5 in mg/L) in 24 samples collected over a year (regulatory values: less than 1,500 and 500 mg/L for COD and BOD5, respectively; each sample, taken every 15 days, comes from an automatic sampler; each data corresponds to a single measure of the parameter).
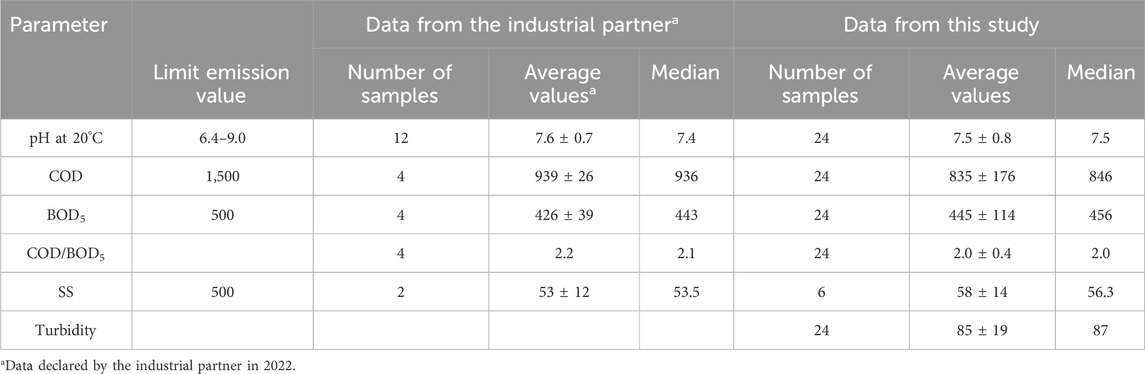
Table 2. Comparison of the average concentrations and standard deviations of the pH, COD, BOD5, SS and turbidity (values expressed in mg/L for COD, BOD5 and SS, and in FTU for turbidity with each median associated) provided by the industrial partner and those collected in this study and the corresponding legal discharge limits (samples characteristic of that day’s activity).
Compared to the averages of the four measurements carried out by the industrial partner, it is found that the values of the COD are significantly different (Table 2) while those of the BOD5 are similar. The COD and BOD5 values reported in this study are similar with those published in the literature (Braga and Varesche, 2014; Alhinai, 2022; Kumar et al., 2022; Procházková and Máša, 2022; Singh et al., 2022). Whatever the sample, the COD analyses comply with the emission limit value that the laundry must respect, namely 1,500 mg/L. It is clear that the physicochemical treatment process used by the industrial for its wastewater treatment ensures an efficient purification, lowering the pollutant load at satisfactory levels for the current regulation limits. pH values are also within the regulatory range (Table 2). However, for BOD5, the values are close to the emission limit value, and, of the 24 samples studied, some exceed the limit value of 500 mg/L (Figure 2). Moreover, the standard deviation values are relatively high, indicating an important variability. This indicates that the physicochemical treatment is highly variable for their removal in terms of performance, resulting in varying COD and BOD5 concentrations (Figure 2; Table 2). Finally, it can also be noted that waters present a significant turbidity with an average of 85 ± 19 FTU, due to the viscous nature of the samples, and therefore to the organic charge.
COD and BOD5 values were used to calculate the biodegradability index, which is the ratio of these two parameters. The value of this index must be equal to or less than 2.5 (Morin-Crini and Crini, 2017). Figure 3 shows the evolution of the values of this index for the 24 water samples studied. The average and standard deviation value obtained over 1 year of sampling is 2.0 ± 0.4, a value compliant with the regulations and similar to that declared by the industrial partner (Table 2). This average value of 2 clearly demonstrates the biodegradability of waters that are sent to the city’s wastewater treatment plant. However, some point values are found to be greater than the value of 2.5, which also confirms a high variability in the water treatment performed by the industrial partner. Similar values for COD, BOD5 and biodegradability index have been published for water from the laundry sector (Braga and Varesche, 2014; Sheth et al., 2017; Alhinai, 2022; Kumar et al., 2022; Procházková and Máša, 2022; Singh et al., 2022; Lacalamita et al., 2023).
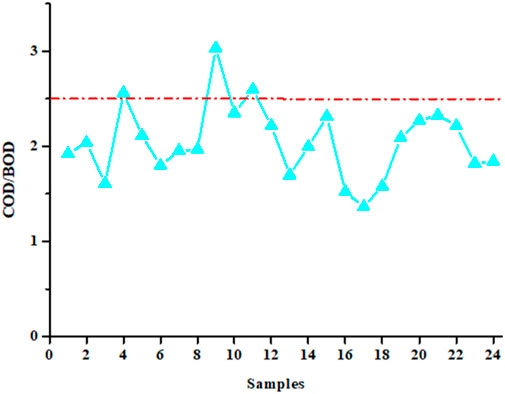
Figure 3. Biodegradability index (COD/BOD ratio) for the 24 samples collected over a year (the dotted red line indicates the regulatory value of 2.5 that should not be exceeded; each sample, collected every 15 days, comes from an automatic sampler).
3.2 Biodegradability of water
Another aim of this study was to assess the biodegradability of water over the long term to understand its organic strength. In general, biochemical oxidation theoretically takes an infinitive time to go to completion because the rate of oxidation is assumed to be proportional to the amount of organic matter remaining. Usually, only 5-day period is used for BOD test. During this period, the oxidation of the carbonaceous organic matter is of the order of 60%–70%, while in a 21-day period, the oxidation is complete at about 95%–99% (Sibil et al., 2014; Morin-Crini and Crini, 2017; Lacalamita et al., 2023; Tomšič et al., 2023).
In this study, we performed BOD measurements not only at 5 days, but also at 7, 14, 21 and 35 days for five randomly selected samples (Figure 4). For each sample and for each time, we also measured the TOC (Figure 4) and AS (Figure 5) parameters. The results showed that the more the incubation time between 5 and 21 days increases, the more the BOD increased. Then from the 21st day, it decreased. Similarly, TOC and AS values decreased from the 5th day to stabilize from the 21st day. After 21 days, TOC values no longer decrease, indicating that biological degradation of carbon compounds is complete. After 35 days, all biodegradable carbon compounds have been oxidized and assimilated by microorganisms.
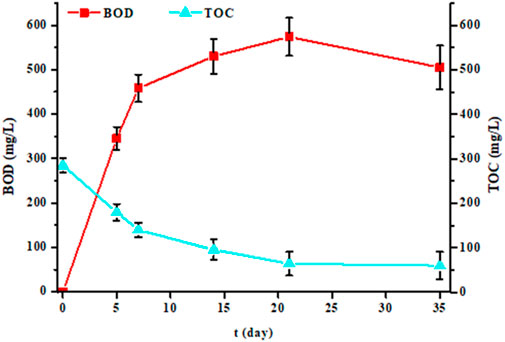
Figure 4. Evolution of biochemical oxygen demand (BOD in mg/L) and total organic carbon (TOC in mg/L) values of five samples measured at 5, 7, 14, 21 and 35 days of experience (each point represents the mean and standard deviation of the five samples).
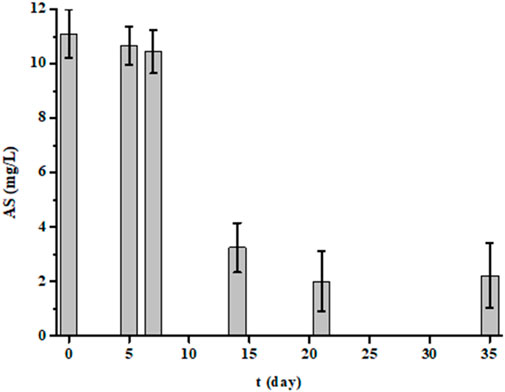
Figure 5. Evolution of anionic detergents (AS in mg/L) values of five samples measured at 5, 7, 14, 21 and 35 days of experience (each bar represents the mean and standard deviation of the five samples).
A pertinent point was to have information on degradation kinetics. For this, we used the values of the BOD at 5 and 7 days. The averages and standard deviations for the five samples were 502 ± 47 mg/L for BOD7 and 402 ± 33 mg/L for BOD5. The ratio value between BOD7 and BOD5 was 1.2, this value being like that of domestic water according to the regulations (French standard NF EN 1899-1). Thus, laundry effluents are rich in substances very quickly biodegradable. This conclusion is also consistent with BOD21 values, which are higher than that of COD. In less than 7 days, much of the organic pollution was eliminated by bacteria since the TOC values significantly decreases (Figure 4). However, the results in Figure 5 also showed that this degradation is not instantaneous for certain substances such as detergents since it takes several days to reach a certain level of decontamination. This is demonstrated by anionic surfactants (AS) measurements over several days. The averages and standard deviations of the 5 waters were 2.4 ± 0.6 mg/L, 3.4 ± 1.5 mg/L, 5.5 ± 1.7 mg/L, 9.7 ± 1.0 mg/L and 10.4 ± 0.8 mg/L after 35, 21, 14, 7 and 5 days of treatment by bacteria, respectively, whereas initially it was 11.4 ± 0.8 mg/L. The AS values were identical after 7 days and only started to decrease after the 14th day. Even after 35 days of measurement, the values were important, indicating that a non-negligible fraction of surfactants is not degraded. If a percentage of elimination is calculated after 35 days of treatment, a value of 79% is obtained. This percentage should be linked to the TOC value. The initial TOC value for the five samples decreased from 276 ± 9 mg/L to 63 ± 30 mg/L after 35 days of treatment, which corresponds to a reduction of 77%. This result demonstrates that some of the water pollutants is not biodegradable (Sheth et al., 2017; Moharir et al., 2020; Hu et al., 2021; Alhinai, 2022; Melián et al., 2023).
4 Discussion
The results described in part 3 provide answers to the following questions:
4.1 What is the temporal variability of COD and BOD5 parameters over a year of water sampling?
For the 24 samples studied in this work, the averages and standard deviations for COD, BOD5 and biodegradability index were 835 ± 176 mg/L, 445 ± 114 mg/L, and 2.0 ± 0.4, respectively. The interpretation of the collected data showed a significant variability, which is confirmed by the high values of the standard deviation. This result indicated a high variability in the water treatment performed by the industrial partner’s wastewater treatment plant for the abatement of COD and BOD5 parameters. This variability in the physicochemical treatment of wastewater can be explained by the variation in the composition and concentration of the water to be treated which is a function of washing cycles, the type of laundry washed, the products used in the industrial cleaning process (Bajpai and Tyagi, 2007; Yu et al., 2008; Zavala and Estrada, 2016; Dąbkowska, 2017; Swartz et al., 2017; Lacalamita et al., 2023). A comparison of our data with those published in the literature showed similar values for COD, BOD5 and biodegradability index (Braga and Varesche, 2014; Sheth et al., 2017; Alhinai, 2022; Kumar et al., 2022; Procházková and Máša, 2022; Singh et al., 2022; Lacalamita et al., 2023). However, it is difficult to compare the results of different studies from laundries that use different linen, washing cycles and products in their industrial processes. The decontamination treatments used by the laundry sector (often confidential and not detailed in the literature) are also different, which can lead to different purification performances. In addition, data on the temporal variability of these parameters are rarer. Braga and Varesche and Lacalamita et al. recently pointed out the high variability of COD and BOD5 parameter values for one sample compared to another (Braga and Varesche, 2014; Lacalamita et al., 2023).
4.2 Are these parameters sufficient to characterize the organic load of discharged water?
Measurements of COD and BOD5 parameters in the wastewater characterization are useful for providing information on the organic load of discharge water from a quantitative point of view, and on its biodegradability. However, other analytical measurements such as TOC and AS need to be supplemented for this type of industrial water. However, in France, continuous or even spot measurement of these two water quality indicators is not mandatory.
4.3 Is water biodegradation fast?
BOD values measured at 5 and 7 days showed that laundry effluents contain substances that are rapidly biodegradable. This result was also confirmed by the TOC values obtained since a large fraction of the organic pollution was eliminated in less than 7 days. However, for some substances, such as surfactants, biodegradation is not instantaneous as it takes several days to reach a certain level of decontamination, which was confirmed by the AS measurements over several days.
4.4 Is it possible to quantify the non-biodegradable fraction of water?
By simultaneously measuring COD, BOD, TOC and AS, it is possible to characterize the biodegradability of the laundry water from a biodegradability point of view. According to the BOD21 and biodegradability index values, much of the water pollution is biodegradable. However, it is necessary to increase the duration of biodegradation tests beyond 21 days. Indeed, even after 35 days of experiments, waters contained yet a non-negligible fraction of surfactants. Swartz et al. noted that the 35-day duration was the time required to degrade a large fraction of hydrocarbon substances, but they were not totally eliminated because of their chemical structure and stability (Swartz et al., 2017). Other studies have also mentioned that some surfactant formulations are difficult to be degrade by bacteria (Sheth et al., 2017; Moharir et al., 2020; Hu et al., 2021; Alhinai, 2022; Melián et al., 2023).
To significantly decrease the COD and BOD5 values, several conventional, e.g., coagulation, carbon adsorption, membrane bioreactor and membrane filtration, and non-conventional, e.g., phycoremediation, biocoagulation, biosorption and advanced oxidation, methods are proposed in the literature, each method having its advantages and disadvantages (Nicolaidis and Vyrides, 2014; Morin-Crini and Crini, 2017; Bering et al., 2018; Pasaribu, 2020; de Oliveira Cardoso Nascimento et al., 2021; Li et al., 2022; Akhere and Chukwuka, 2023; Melián et al., 2023). However, the choice is often difficult for both chemical, technological and financial reasons (Morin-Crini and Crini, 2017). The data of this study could help the industrial partner to guide its choice of the complementary method to better treat its wastewater. Indeed, much of the water pollution is biodegradable, suggesting that on-site biological treatment coupled with the physicochemical process could be considered. In addition, the use of activated carbon could eliminate the refractory organic fraction. These two decontamination processes are being studied not only in terms of their chemical efficacy, but also from an economic point of view, by setting up pilot tests that take into account the chemical variability of the water to be treated, as demonstrated in this work.
5 Conclusion
This study describes in detail for the first-time data on the temporal variability of the chemical oxygen demand (COD) and biochemical oxygen demand (BOD5) parameters and the water biodegradability of the industrial laundry sector over a year of sampling. The results showed that, even if the waters comply with the regulations in force, the concentrations of COD and BOD5 remain high and especially highly variable. Despite this variability, the values of these two water quality indicators are consistent with those of the regulations. According to the BOD21 and biodegradability index values, much of the water pollution is biodegradable. Laundry effluents are also rich in substances very quickly biodegradable, which has been demonstrated by the values of the ratio between BOD7 and BOD5. In less than 7 days, much of the organic pollution was eliminated by bacteria since the TOC values significantly decreases. However, the biodegradability study showed that this degradation is not instantaneous for certain substances such as detergents since it takes several days to reach a certain level of decontamination. This was demonstrated by total organic carbon (TOC) and anionic surfactants (AS) measurements over several days. The AS values were identical after 7 days and only started to decrease after the 14th day. Even after 35 days of measurement, the values of AS were high, indicating that a non-negligible fraction of surfactants is not degraded. It is useful to simultaneously measure not only the COD and BOD parameters but also the TOC and AS. For now, TOC and AS measures are not mandatory from a regulatory point of view in France. Since this study, the industrial partner has decided, on the one hand, to increase the frequency of analysis of the COD and BOD5 parameters, from four annual analyses to one per month, and to carry out a mapping of detergents used in the industrial process and likely to end up in wastewater, and on the other hand, to consider studying treatment techniques to reduce the organic load of water while being economically viable. In this issue, this study could help the industrial partner to guide its choice of the complementary method to better treat wastewater.
Data availability statement
The raw data supporting the conclusion of this article will be made available by the authors, without undue reservation.
Author contributions
DL: Formal Analysis, Investigation, Software, Writing–original draft. CM: Data curation, Formal Analysis, Investigation, Methodology, Writing–review and editing. GC: Conceptualization, Data curation, Funding acquisition, Methodology, Project administration, Resources, Supervision, Validation, Writing–review and editing.
Funding
The authors declare that financial support was received for the research, authorship, and/or publication of this article. This research was funded by the Université de Franche-Comté (Besançon), the Région Bourgogne Franche-Comté, the Fonds européens de développement regional (FEDER), and UNAP (Pontarlier) through the MYDREAU 2022-2024 (2022_ANR PCPT) project, which made it possible to recruit DL as invited Italian engineer.
Acknowledgments
The authors thank the PEA2t analytical platform (Nadia Morin-Crini and Caroline Amiot, Université de Franche-Comté, Besançon) that manages the analytical equipment used in this study, the Qualio Laboratory (Université de Franche-Comté, Besançon) for the help in BOD measurements, and Nadège Collet and Lindsay Strappazzon (UNAP, Pontarlier) for their enthusiasm and their industrial expertise as well as for numerous fruitful discussions. Doctoral student CM thanks the Région Bourgogne Franche-Comté (France) for awarding her a research grant.
Conflict of interest
The authors declare that the research was conducted in the absence of any commercial or financial relationships that could be construed as a potential conflict of interest.
Publisher’s note
All claims expressed in this article are solely those of the authors and do not necessarily represent those of their affiliated organizations, or those of the publisher, the editors and the reviewers. Any product that may be evaluated in this article, or claim that may be made by its manufacturer, is not guaranteed or endorsed by the publisher.
References
Abily, M., Acuña, V., Corominas, L., Rodríguez-Roda, I., and Gernjak, W. (2023). Strategic routes for wastewater treatment plant upgrades to reduce micropollutants in European surface water bodies. J. Clean. Prod. 415, 137867. doi:10.1016/j.jclepro.2023.137867
Aguilar-Torrejón, J. A., Balderas-Hernández, P., Roa-Morales, G., Barrera-Díaz, C. E., Rodríguez-Torres, I., and Torres-Blancas, T. (2023). Relationship, importance, and development of analytical techniques: COD, BOD, and, TOC in water—an overview through time. SN Appl. Sci. 5, 118. doi:10.1007/s42452-023-05318-7
Akhere, M. A., and Chukwuka, H. (2023). Phycoremediation of laundry wastewater using Chlorella vulgaris (Beijerinck). Afr. Sci. 24, 175–182. doi:10.26538/africanscientist.24.2.20230604
Alhinai, A. S. (2022). “Laundry wastewater characterization and treatment for reuse purposes in Oman,” in Towards a sustainable water future: proceedings of OICWE2020. Editors A. Badr, and J. Venables (London: ICE Publishing), 211–219. doi:10.1680/oicwe.65253.211
Aonghusa, C. N., and Gray, N. F. (2002). Laundry detergents as a source of heavy metals in Irish domestic wastewater. J. Environ. Sci. Health A Tox. Hazard. Subst. Environ. Eng. 37, 1–6. doi:10.1081/ese-100108477
Bajpai, D., and Tyagi, V. K. (2007). Laundry detergents: an overview. J. Oleo. Sci. 56, 327–340. doi:10.5650/jos.56.327
Bering, S., Mazur, J., Tarnowski, K., Dąbkowska, N., Janus, M., Mozia, S., et al. (2018). Removal of organic pollutants and surfactants from laundry wastewater in membrane bioreactor (MBR). Des. Water Treat. 134, 281–288. doi:10.5004/dwt.2018.23207
Braga, J. K., and Varesche, M. B. A. (2014). Commercial laundry water characterisation. Am. J. Anal. Chem. 5, 8–16. doi:10.4236/ajac.2014.51002
Collivignarelli, M. C., Miino, M. C., Baldi, M., Manzi, S., Abbà, A., and Bertanza, G. (2019). Removal of non-ionic and anionic surfactants from real laundry wastewater by means of a full-scale treatment system. Process Saf. Environ. Prot. 132, 105–115. doi:10.1016/j.psep.2019.10.022
Dąbkowska, N. (2017). Determination of the quality of industrial laundry raw wastewater. Ecol. Eng. 18, 1–4. doi:10.12912/23920629/76778
de Oliveira Cardoso Nascimento, C., Veit, M. T., Palacio, S. M., and da Cunha Gonçalves, G. (2021). Use of natural coagulants in the removal of color and turbidity from laundry wastewater. Water Air Soil Pollut. 232, 300. doi:10.1007/s11270-021-05253-6
Georgin, J., Meili, L., and Franco, D. S. P. (2023). A review of the application of low-cost adsorbents as an alternative method for biosorption of contaminants present in water. Lat. Am. Dev. Energy Eng. LADEE 4, 1–20. doi:10.17981/ladee.04.02.2023.1
Ho, K. C., Teow, Y. H., Sum, J. Y., Ng, Z. J., and Mohammad, A. W. (2021). Water pathways through the ages: integrated laundry wastewater treatment for pollution prevention. Sci. Total Environ. 760, 143966. doi:10.1016/j.scitotenv.2020.143966
Hu, S., B, Z., and Xu, Y. (2021). Using electric flocculation to treat domestic laundry wastewater with different types of detergents. E3S Web Conf. 61, 04008. doi:10.1051/e3sconf/202126104008
Jayanto, G. D., Widyastuti, M., and Hadi, M. P. (2011). Laundry wastewater characteristics and their relationship with river water quality as an indicator of water pollution. Case study: code Watershed, Yogyakarta. E3S Web Conf. 325, 02011. doi:10.1051/e3sconf/202132502011
Kogut, I., Szwast, M., Hussy, S., Polak, D., Gerhardts, A., and Piątkiewicz, W. (2021). Evaluation of wastewater reuse in commercial laundries: a pilot field study. Des. Water Treat. 214, 39–48. doi:10.5004/dwt.2021.26531
Kumar, S., Mostafazadeh, A. K., Kumar, L. R., Tyagi, R. D., Drogui, P., and Brien, E. (2022). Advancements in laundry wastewater treatment for reuse: a review. J. Environ. Sci. Health Part A Toxic/Hazardous Subst. Environ. Eng. 57, 927–946. doi:10.1080/10934529.2022.2132076
Lacalamita, D., Mongioví, C., and Crini, G. (2023). Chemical substances present in discharge water generated by laundry industry: analytical monitoring. Water Sci. Eng. 16, 324–332. doi:10.1016/j.wse.2023.07.004
Li, J., Dagnew, M., and Ray, M. B. (2022). Effect of coagulation on microfibers in laundry wastewater. Environ. Res. 212, 113401. doi:10.1016/j.envres.2022.113401
Lutterbeck, C. A., Colares, G. S., Dell’Osbel, N., da Silva, F. P., Kist, L. T., and Machado, Ê. L. (2020). Hospital laundry wastewaters: a review on treatment alternatives, life cycle assessment and prognosis scenarios. J. Clean. Prod. 273, 122851. doi:10.1016/j.jclepro.2020.122851
Melián, E. P., Santiago, D. E., Leόn, E., Reboso, J. V., and Herrera-Melián, E. P. (2023). Treatment of laundry wastewater by different processes: optimization and life cycle assessment. J. Environ. Chem. Eng. 11, 109302. doi:10.1016/j.jece.2023.109302
Moharir, S. R., Patni, P. A., Datar, A. V., and Suryawanshi, T. S. (2020). Study of treatment of laundry wastewater. J. Emerg. Technol. Innov. Res. 7, 574–591. http://www.jetir.org/papers/JETIR2009078.pdf.
Morin-Crini, N., and Crini, G. (2017). “Eaux industrielles contaminées,” in Réglementation, paramètres chimiques et biologiques, procédés d’épuration innovants (in French). Editors N. Morin-Crini, and G. Crini (France: Besançon: Presses Universitaires de Franche-Comté). IBSN: 978-2-84867-589-3.
Nicolaidis, C., and Vyrides, I. (2014). Closing the water cycle for industrial laundries: an operational performance and techno-economic evaluation of a full-scale membrane bioreactor system. Resour. Conserv. Recycl. 92, 128–135. doi:10.1016/j.resconrec.2014.09.001
Oyedotun, T. D. T., and Ally, N. (2021). Environmental issues and challenges confronting surface waters in South America: a review. Environ. Challenges 3, 100049. doi:10.1016/j.envc.2021.100049
Pasaribu, M. (2020). Phosphate reduction from the artificial sample of laundry wastewater by using bintaro (Cerbera manghas) fruit shell adsorbent. J. Environ. Eng. Waste Manag. 5, 1–14. doi:10.33021/jenv.v5i1.961
Patel, N., Khan, M. Z. A., Shahane, S., Rai, D., Chauhan, D., Kant, C., et al. (2020). Emerging pollutants in aquatic environ-ment: source, effect, and challenges in biomonitoring and bioremediation - a review. Pollution 6, 99–113. doi:10.22059/poll.2019.285116.646
Peña-Guzmán, C., Ulloa-Sánchez, S., Mora, K., Helena-Bustos, R., Lopez-Barrera, E., Alvarez, J., et al. (2019). Emerging pollutants in the urban water cycle in Latin America: a review of the current literature. J. Environ. Manage. 237, 408–423. doi:10.1016/j.jenvman.2019.02.100
Procházková, M., and Máša, V. (2022). Sustainable wastewater management in industrial laundries. Chem. Eng. Trans. 94, 577–582. doi:10.3303/CET2294096
Rizzo, L., Malato, S., Antakyali, D., Beretsou, V. G., Đolić, M. B., Gernjak, W., et al. (2019). Consolidated vs new advanced treatment methods for the removal of contaminants of emerging concern from urban wastewater. Sci. Total Environ. 655, 986–1008. doi:10.1016/j.scitotenv.2018.11.265
Santiago, D. E., Hernández Rodríguez, M. J., and Pulido-Melián, E. (2021). Laundry wastewater treatment: review and life cycle assessment. J. Environ. Eng. 147, 143966. doi:10.1061/(ASCE)EE.1943-7870.0001902
Sheth, K. N., Patel, M., and Deasi, M. D. (2017). A study on characterization and treatment of laundry effluent. Int. J. Innov. Res. Sci. Technol. 4, 50–55.
Sibil, R., Berkun, M., and Bekiroglu, S. (2014). The comparison of different mathematical methods to determine the BOD parameters, a new developed method and impacts of these parameters variations on the design of WWTPs. Appl. Math. Model. 38, 641–658. doi:10.1016/j.apm.2013.07.013
Singh, N., Randhawa, S., Dutt, I., and Kumar, A. (2022). Characterization of laundry wastewater and its potential applications in garden irrigation and lavatory cleaning: a squat review. AIP Conf. Proc. 2357, 030014. doi:10.1063/5.0086117
Sumisha, A., Arthanareeswaran, G., Lukka Thuyavan, Y., Ismail, A. F., and Chakraborty, S. (2015). Treatment of laundry wastewater using polyethersulfone/polyvinylpyrollidone ultrafiltration membranes. Ecotoxicol. Environ. Saf. 121, 174–179. doi:10.1016/j.ecoenv.2015.04.004
Swartz, C. D., Swanepoel, G., Welz, P. J., Muanda, C., and Bonga, A. (2017). Natsurv 8 – water and wastewater management in the laundry industry. WRC Report N°. TT 703/16. ISBN 978-1-4312-0870-8.
Tomšič, B., Ofentavšek, L., and Fink, R. (2023). Toward sustainable household laundry. Washing quality vs environmental impacts. Int. J. Environ. Health Res. 34, 1011–1022. doi:10.1080/09603123.2023.2194615
Wang, C. Y., Zhou, B., and Huang, B. (2015). A continuing 30-year decline in water quality of Jiaojiang Estuary, China. Water Sci. Eng. 8, 20–29. doi:10.1016/j.wse.2015.01.007
Warren-Vega, W. M., Campos-Rodríguez, A., Zárate-Guzmán, A. I., and Romero-Cano, L. A. (2023). A current review of water pollutants in American continent: trends and perspectives in detection, health risks, and treatment technologies. Int. J. Environ. Res. Public Health 20, 4499. doi:10.3390/ijerph20054499
Yu, Y. X., Zhao, J., and Andrew, E. B. (2008). Development of surfactants and builders in detergent formulations. Chin. J. Chem. Eng. 16, 517–527. doi:10.1016/s1004-9541(08)60115-9
Keywords: laundry industry, discharge waters, COD, BOD, monitoring, biodegradability
Citation: Lacalamita D, Mongioví C and Crini G (2024) Chemical oxygen demand and biochemical oxygen demand analysis of discharge waters from laundry industry: monitoring, temporal variability, and biodegradability. Front. Environ. Sci. 12:1387041. doi: 10.3389/fenvs.2024.1387041
Received: 16 February 2024; Accepted: 22 March 2024;
Published: 08 April 2024.
Edited by:
Spyros Foteinis, Heriot-Watt University, United KingdomReviewed by:
Dunia E. Santiago, University of Las Palmas de Gran Canaria, SpainGianluigi Buttiglieri, Catalan Institute for Water Research, Spain
Copyright © 2024 Lacalamita, Mongioví and Crini. This is an open-access article distributed under the terms of the Creative Commons Attribution License (CC BY). The use, distribution or reproduction in other forums is permitted, provided the original author(s) and the copyright owner(s) are credited and that the original publication in this journal is cited, in accordance with accepted academic practice. No use, distribution or reproduction is permitted which does not comply with these terms.
*Correspondence: Grégorio Crini, gregorio.crini@univ-fcomte.fr
†ORCID: Grégorio Crini, orcid.org/0000-0003-2540-6851