- 1Department of Environmental Geosciences EDGE, Centre for Microbiology and Environmental Systems Science, University of Vienna, Vienna, Austria
- 2Doctoral School in Microbiology and Environmental Science, University of Vienna, Vienna, Austria
- 3Research Platform Plastics in the Environment and Society (PLENTY), University of Vienna, Vienna, Austria
- 4Department of Soil and Water Sciences, Institute of Environmental Sciences, Faculty of Agriculture, Food and Environment, The Hebrew University of Jerusalem, Rehovot, Israel
Introduction: Tire and road wear particles are one of the most abundant types of microplastic entering the environment. The toxicity of tire and road wear particles has been linked to their organic additives and associated transformation products. Tire and road wear particles, and associated tire-derived compounds are introduced to the agricultural environment via atmospheric deposition, irrigation with reclaimed wastewater, and the use of biosolids (treated sewage sludge) as fertilizer. In the agricultural environment, these tire-derived compounds could be taken up by edible plants, leading to human exposure.
Methods: Sixteen tire-derived compounds were measured in twenty-eight commercial leafy vegetable samples from four countries. Based on the results, the estimated daily intake of these tire-derived compounds was calculated due to leafy vegetable consumption based on local diets under a mean and maximum concentration scenario.
Results: In commercial leafy vegetables, six tire-derived compounds were detected: benzothiazole (maximum concentration—238 ng/g dry weight), 2-hydroxybenzothiazole (maximum concentration—665 ng/g dry weight), 1,3-diphenylguanidine (maximum concentration—2.1 ng/g dry weight), N-(1,3-dimethylbutyl)-N′-phenyl-p-phenylenediamine (6PPD, maximum concentration—0.4 ng/g dry weight), N-Isopropyl-N-phenyl-4-phenylenediamine (IPPD, maximum concentration—0.1 ng/g dry weight), and N-phenyl-N-cyclohexyl-p-phenylenediamine (CPPD, maximum concentration—0.3 ng/g dry weight). At least one compound was present in 71% of samples analyzed. The estimated daily intake for 1,3-diphenylguanidine ranged from 0.05 ng/person/day in the mean scenario to 4.0 ng/person/day in the maximum scenario; benzothiazole ranged from 12 to 1,296 ng/person/day; 6PPD ranged from 0.06 to 2.6 ng/person/day; IPPD ranged from 0.04 to 1.1 ng/person/day; CPPD ranged from 0.05 to 2.6 ng/person/day.
Discussion: Statistical analyses did not reveal correlation between known growth conditions and tire-derived compound concentrations in the leafy vegetable samples. The estimated daily intake via leafy vegetable consumption was generally lower than or comparable to the estimated daily intake via other known sources. However, we show that tire-derived compounds are taken up by foodstuff, and exposure might be higher for other produce. Future studies are needed to uncover pathways of tire-derived compounds from road to food, assess the exposure to transformation products, and investigate the biological effects associated with this exposure.
1 Introduction
Abrasion of tires at the road surface generates tire and road wear particles, which represent a major flux of microplastics into the environment. Estimates of tire and road wear particle emissions range widely, from 0.9 to 2.5 kg/capita/year, which corresponds to between 24% and 94% of total microplastic emissions (Mennekes and Nowack, 2022), emphasizing that tire and road wear particle pollution is highly relevant, but still poorly understood. The amount of tire and road wear particles is expected to increase in future years due to the continuous increase in the number of cars on the road. Upon generation at the road surface, 0.1%–10% of tire and road wear particles become airborne (Grigoratos and Martini, 2014), and can potentially be transported over long distances (Kole et al., 2017). The largest fraction of tire and road wear particles (90%–99.9%) accumulates at the road surface and is flushed during road-wash events into roadside soils, receiving water bodies, or sewer systems. Many cities have combined sewer and runoff systems, in which road-wash is collected along with sewage in wastewater treatment systems. Thus, it is expected that biosolids (treated sewage sludge) are contaminated with tire and road wear particles. Biosolids are used in many countries for fertilization, and an estimated 1,400–2,800 tons per year of tire wear particles are deposited on agricultural fields in Germany via this route (Baensch-Baltruschat et al., 2021). Treated wastewater effluent is also used in many countries for irrigation, with the unintended risk of introducing pollutants to agricultural fields (Winpenny et al., 2010; Miller et al., 2016; Gianico et al., 2021).
Tire and road wear particles are formed of material derived both from tires and from the road surface. One of the main concerns associated with tire and road wear particles is the high chemical additive content associated with tire material. Tire and road wear particles have been estimated to contain 5%–10% organic and inorganic additives by weight, added to tires among other functions, as processing aids, antioxidants, and plasticizers (Wagner et al., 2018). These additives and their transformation products, i.e., tire-derived compounds, are not chemically bound within the rubber matrix, and many leach into the environment. Some of the main chemical additives are vulcanization accelerators including benzothiazoles, and several guanidine derivatives such as 1,3-diphenylguanidine (DPG), which comprise 0.5% of tire mass each (Unice et al., 2015), corrosion inhibiting benzotriazoles, the crosslinking agent hexa (methoxymethyl) melamine (HMMM), and p-phenylenediamine compounds (PPDs), which are used as antiozonants, and represent 0.8% of tire mass (Unice et al., 2015). These tire-derived compounds are ubiquitously detected in the environment. Benzothiazole is detected in surface water up to 2,500 μg/L, wastewater up to 49.3 μg/L, sludge up to 50.2 μg/kg, and air up to 32 μg/m3 (Liao et al., 2018). DPG has recently been detected in wastewater effluent at concentrations up to 150 ng/L and surface waters up to 1.9 μg/L (Zhang et al., 2023). HMMM has been detected in wastewater treatment plants at concentrations up to 60.8 μg/L (Alhelou et al., 2019), as well as rivers at concentrations up to 1.6 μg/L (Johannessen et al., 2021a). PPDs and PPD-quinones have been detected in air, water, and soils at sum concentrations of 11.0 pg/m3, 2.3 μg/L, and 776 ng/g, respectively (Cao et al., 2022). Both benzothiazoles and DPG have been associated with toxic effects of tire and road wear particles to fish, albeit above environmentally relevant concentrations (Chibwe et al., 2022). Quinone derivatives of PPDs (PPD-quinones) have been shown to be highly toxic to some aquatic species at environmental concentrations, with the most notable example being 6PPD-quinone (Zoroufchi Benis et al., 2023).
There are multiple pathways by which tire-derived compounds can reach the agricultural environment, but exact pathways and mass fluxes are unknown. The airborne fraction of tire and road wear particles can deposit onto agricultural soils (Brahney et al., 2021), especially where agriculture and major roadways are in close proximity. Reclaimed wastewater containing tire-derived compounds may be applied to agricultural fields for irrigation, mainly in areas of water scarcity. For example, reclaimed wastewater comprises more than 50% of Israel’s agricultural water supply (Miarov et al., 2020). Irrigation with reclaimed wastewater is prohibited in Switzerland (Departement für Umwelt Verkehr Energie und Kommunikation UVEK, 2021), but practiced in several other countries in Europe, particularly in the Mediterranean region. According to a 2008 report, Spain uses 821,920 m3/day and Italy uses 123,288 m3/day (Jimenez and Asano, 2008) of reclaimed wastewater. The use of reclaimed wastewater for irrigation is expected to increase globally over the next years, especially in Europe due to new EU regulation (European Parliament and The Council of the European Union, 2020). Based on the high density of tire and road wear particles (1.5–2.2 g/cm3) relative to water, and reported retention of other types of microplastic particles in wastewater treatment plants, it is assumed that tire and road wear particles are retained in sludge during wastewater treatment (Baensch-Baltruschat et al., 2021). After further treatment, sludge may be used as an alternative fertilizer. Although the presence of contaminants in sludge has led to a ban of its use in agriculture in Switzerland (Admin, 2024), each year 755 kilotons dry mass of sludge is applied to land as biosolids in Spain, and 316 kilotons dry mass in Italy (Collivignarelli et al., 2019). This practice is expected to increase globally due to both the energy demands associated with bioavailable nitrogen production, and global phosphorous scarcity.
Plant uptake of contaminants of emerging concern is well documented in the literature (Wu et al., 2014; Miller et al., 2016; Mordechay et al., 2022). Hydroponic studies have demonstrated plant uptake and translocation from roots to leaves of the tire-derived compounds HMMM (maximum concentration 18.0 μg/g), 6PPD (maximum concentration 0.75 μg/g), 6PPD-quinone (maximum concentration 2.19 μg/g), DPG (maximum concentration 2.29 μg/g), benzothiazole (maximum concentration 1.24 μg/g) (Castan et al., 2023), as well as 2-mercaptobenzothiazole (maximum concentration 1.12 μg/g) (LeFevre et al., 2016). However these experiments were conducted under controlled conditions and at higher concentrations than are expected to occur in the environment. Uptake by plants grown in soils is more complex, involving chemical, physical and biological processes affecting the availability of a compound to the plant. Strong sorption to soil has been demonstrated for several tire-derived compounds, with HMMM displaying a Freundlich sorption coefficient (log Kf) up to 1.19 and DPG up to 2.88 (Tang and Kolodziej, 2022), suggesting that plant uptake of tire-derived compounds may be lower in the soil environment as compared to hydroponic studies. The potential for enhanced solubilization due to the presence of plant root exudates, as well as biotic and abiotic transformations of tire-derived compounds in soil further complicate the extrapolation of results obtained in hydroponic studies to the agricultural environment. In addition to root uptake and translocation, tire and road wear particles may come into direct contact with plant leaves, due to atmospheric deposition, or resuspension from the soil. It has been shown that positively charged microplastics can be taken up through the stomata of lettuce leaves (Wang Y. et al., 2022), so it is plausible that tire and road wear particles themselves are taken up into lettuce leaves. Even if foliar uptake of tire and road wear particles does not occur, the particles may release tire-derived compounds on the leave surfaces, and foliar uptake of these compounds would be possible. Finally, soil particles contaminated with tire-derived compounds may be suspended during irrigation, rainfall, or wind events and attach to leafy vegetables (Pinder and McLeod, 1988). This process could introduce tire-derived compounds which are associated with soil particles to leafy vegetables. Tire-derived compounds with a high affinity to soil would be otherwise unavailable for root uptake.
The increasing body of research demonstrating biological effects of tire-derived compounds (Tian et al., 2021; Chibwe et al., 2022; Zoroufchi Benis et al., 2023), as well as their recent detection in human urine (Asimakopoulos et al., 2013; Li and Kannan 2023) warrant a thorough human exposure assessment. Human exposure to tire-derived compounds via inhalation, dust ingestion, and drinking water have been demonstrated (Wang et al., 2013; Wang et al., 2022b; Cao et al., 2022; Li and Kannan, 2023), however, the concentration of tire-derived compounds in commercial produce and the exposure via dietary intake is unknown. Leaves have been demonstrated to accumulate more organic contaminants than other plant organs (Wu et al., 2014; Ben et al., 2021; Ben Mordechay et al., 2022a), so we hypothesized that leafy vegetables may be contaminated with tire-derived compounds and contribute to the overall human exposure. Due to differences in reclaimed wastewater and biosolids use in different regions of the world, we hypothesized that tire-derived compound contamination of leafy vegetables would depend on national agriculture policy. Therefore, in this study we screened sixteen tire-derived compounds in twenty-eight leafy vegetable samples grown in four countries (Israel, Switzerland, Italy, Spain) in order to estimate human exposure via dietary intake.
2 Materials and methods
2.1 Sample collection and processing
Two sets of leafy vegetable samples, collected from Switzerland and Israel, were analyzed in this study. Samples collected in Switzerland were collected in replicates of three, and analyzed separately. Samples from Israel were collected in replicates of 10–15, and were combined and analyzed as composite samples. In total, fifteen leafy vegetables (grown in Switzerland, Italy, or Spain) were purchased in grocery stores in Switzerland as part of an investigation by the Swiss consumer magazine K-TIPP between February and April 2023. Information regarding use of reclaimed wastewater or biosolids was unavailable for these samples. The leaves were transported frozen to our laboratory, where they were lyophilized. The dry leaves were then homogenized using a blender to obtain representative samples of the entire biomass. A total of thirteen leafy vegetable samples were collected in Israel during 2017, each representing a different commercial field. All samples from Israel were irrigated with reclaimed wastewater. Details regarding the sampling procedure and locations are provided in Ben et al. (2021). The samples were lyophilized and stored frozen (−20°C). The dry samples were shipped frozen to Austria (April 2023) for analysis. Details about individual samples, including the wastewater treatment plant where the reclaimed wastewater was produced are provided in Supplementary Table S1.
Samples from both sample sets were extracted between March and May 2023. Since tire-derived compounds were present at trace levels in leafy vegetable samples, it was necessary to extract a large amount of biomass and then pre-concentrate samples before measurement. To this end, three sub-samples of 1.5 g were extracted from each sample (total biomass extracted: 4.5 g per sample), and then combined and concentrated. Sub-samples were added to 50-mL polypropylene centrifuge tubes (Nunc). Isotopically labelled surrogate standards (0.67 µg of benzothiazole-d4 and 6PPD-quinone-d5) were added to each of the sub-samples. To each tube, 4 g stainless steel beads and 30 mL acetonitrile (LCMS grade, Analytics Shop) were added. Samples were homogenized via bead beating for 3 min (3,000 strokes per min) and centrifuged (17,000 g, 20 min, and 20°C). Twenty mL of supernatant was extracted to a separate glass vial. Then, 4 mL of acetonitrile was re-added to the tube containing the biomass, and extraction was repeated twice following the same procedure, for a total extracted volume of 28 mL per sub-sample. All sub-samples (28 mL) were combined, then filtered (0.2 µm nylon filters) using vacuum filtration. Filtered extract was then pre-concentrated to 5 mL using rotary evaporation (226 mBar, 60°C). Due to precipitation, concentrated samples were re-filtered through 0.2 µm nylon filters before analysis.
2.2 Analysis
Samples were analyzed using ultra performance liquid chromatography coupled with triple quadrupole mass spectrometry (Agilent 6,470), operated in multiple reaction monitoring (MRM) mode. The following compounds were analyzed: benzothiazole (BTZ), 2-hydroxybenzothiazole (2OH-BTZ), 2-aminobenzothiazole (2NH2-BTZ), 2-mercaptobenzothiazole (2SH-BTZ), 5-methyl-1H-benzotriazole (5M-1H-BTR), aniline, 1,3-diphenylguanidine (DPG), hexa (methoxymethyl)melamine (HMMM), N-(1,3-dimethylbutyl)-N’-phenyl-p-phenylenediamine (6PPD), N-isopropyl-N-phenyl-4-phenylenediamine (IPPD), N-phenyl-N’-cyclohexyl-p-phenylenediamine (CPPD), N,N’-diphenyl-p-phenylenediamine (DPPD), N-(1,3-dimethylbutyl)-N’-phenyl-p-phenylenediamine-quinone (6PPDq), N-isopropyl-N-phenyl-4-phenylenediamine-quinone (IPPDq), N-phenyl-N’-cyclohexyl-p-phenylenediamine-quinone (CPPDq), N,N’-diphenyl-p-phenylenediamine-quinone (DPPDq). Molecular structures and physicochemical parameters of all tire-derived compounds are provided in Supplementary Table S2. Chromatographic and MRM parameters are provided in Supplementary Table S3. Raw data were processed using Agilent Quantitative Analysis software, and detailed peak picking settings are provided in Supplementary Section S1.
Samples were additionally analysed using Orbitrap high resolution mass spectrometry (Thermo Scientific Q Exactive). Measurement details are provided in Supplementary Section S2. A suspect screening was applied to search for previously reported tire-derived compounds and transformation products for which we do not have standards (Lefevre et al., 2015a; LeFevre et al., 2015b; Alhelou et al., 2019; Johannessen et al., 2021b; Chibwe et al., 2022; Hu et al., 2022; Seiwert et al., 2022; Wiener and LeFevre, 2022; Fohet et al., 2023; Zhao et al., 2023). Features with an exact mass match to a suspect of Δppm <5 ppm were considered potential transformation products, and the MS2 spectra were manually checked for fragments matching those reported in the literature, those of the respective parent compound, or a good FISH (in-silico fragmentation) annotation, in the case where a structure is known or has been proposed. In addition to the suspect screening, we attempted to apply two non-target data analysis methods to search for transformation products. The first approach used the Expected Compounds feature of Compound Discoverer software, which applies known Phase I and Phase II transformations in-silico to a list of compounds. In this case, we applied common Phase I and Phase II transformations which have been previously reported to occur in plants (Lefevre et al., 2015a; LeFevre et al., 2015b; Wiener and LeFevre, 2022; Sunyer-Caldú et al., 2023) to our sixteen target tire-derived compounds, and then screened acquired data for exact mass matches to these expected compounds. The second approach was to use the Molecular Networking feature of Compound Discoverer, which links compounds based on shared molecular fragments from MS2 spectra. Further details of suspect and non-target analyses are provided in Supplementary Section S3.
2.3 Quality assurance and quality control
Surrogate standards benzothiazole-d4 or 6PPD-quinone-d5 were assigned to each analyte based on similarities in chemical structure or similarities in behavior throughout the extraction procedure. Surrogate standard assignments are provided in Supplementary Table S3. Final measured concentrations were corrected to the recovered amount of surrogate standards. Matrix-matched calibrations were prepared by extracting reference material (i.e., a mixture of those leafy vegetable samples which had been determined to contain no tire-derived compounds in an initial screening run) and spiking it to twelve nominal concentrations from 0.11 to 556 ng/g. Analytical blanks (acetonitrile) were measured after every six samples. Limit of quantification was determined as the lowest concentration within the linear range of the calibration, or the mean plus three times the standard deviation of all analytical blanks. Six method blanks (no plant material) were extracted along with samples. Three compounds (DPG, 2-hydroxybenzothiazole, and benzothiazole) were detected sporadically in method blanks—for these compounds, the mean concentration measured in method blanks was subtracted from the concentrations measured in samples. Recovery tests were performed by spiking dry reference material at nominal concentrations of 1, 10, and 100 ng/g, and extracting as described above. For all tire-derived compounds, limit of quantification (ng/g) and recovery (%) are presented in Supplementary Table S4.
All samples were measured in duplicate, and measurement order was randomized to eliminate bias from instrument carryover. Concentrations in measurement duplicates were averaged. In the case that one duplicate was below the limit of quantification, its value was set to 0. It is important to note that this is a conservative approach to data treatment, and actual concentrations may be slightly higher than what we report. Samples for which one duplicate was below the limit of quantification, or for which variance between measurement duplicates exceeded 30% were annotated (Figure 1).
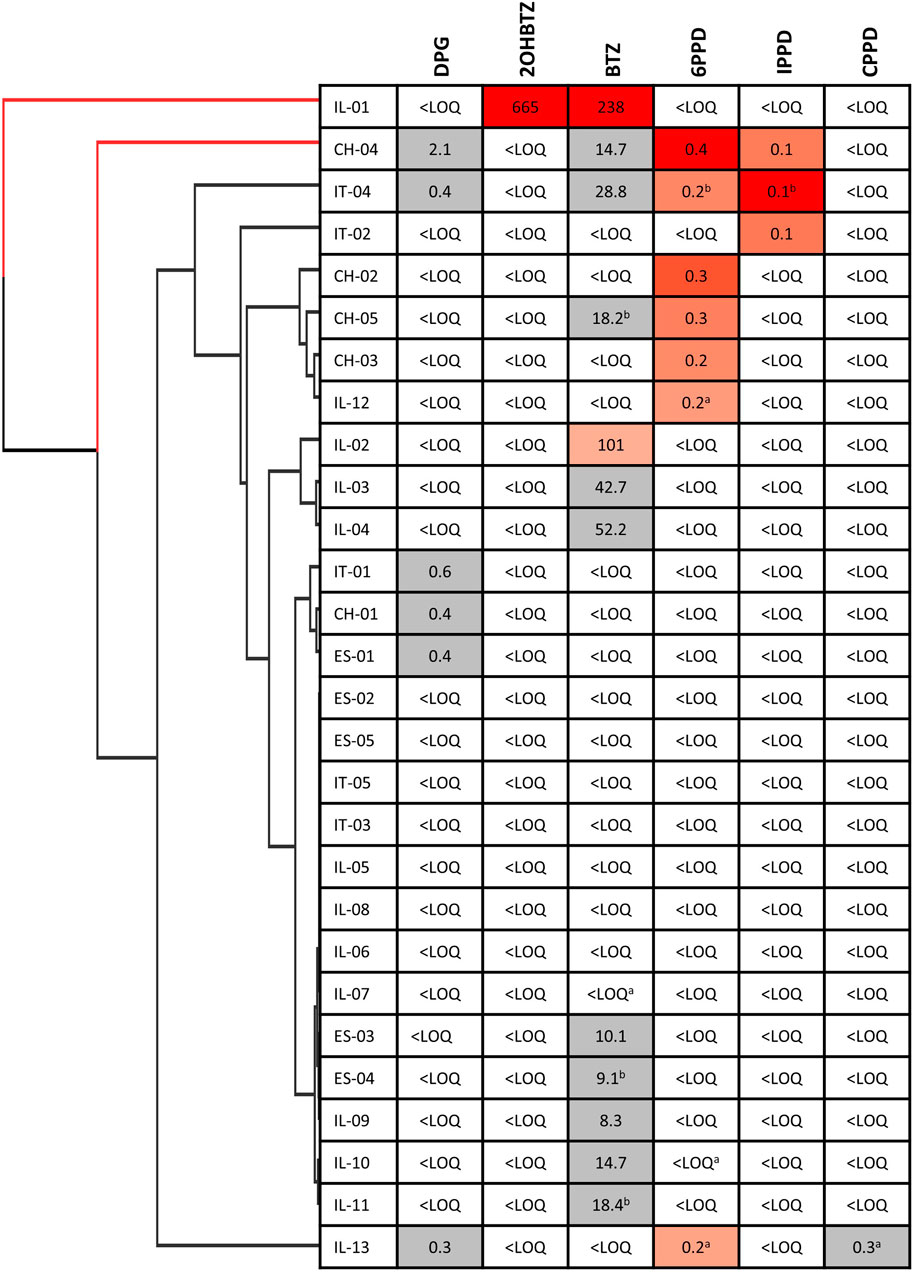
Figure 1. Concentrations of tire-derived compounds (tire-derived compounds) in twenty-eight leafy vegetable samples. Samples are grouped by hierarchical clustering analysis, shown with the dendrogram left of the table. All units are ng/g dry weight. Superscript a indicates that one measurement duplicate was below limit of quantification. Superscript b indicates that variance between measurement duplicates was >30%. Gray color indicates trace detections of tire-derived compounds (detections in a concentration range associated with >40% error, "Supplementary Table S4).
2.4 Calculations and statistical analyses
All data were analyzed in R (Version 4.3.1). Divisive hierarchical clustering analysis (HCA) was applied to all samples. Compound concentrations were first centred and scaled, with concentrations below limit of quantification set to half of the limit of quantification. Dissimilarities between samples were calculated using the Manhattan algorithm (sum of absolute differences) from the cluster R package, and dendrograms were visualized using the factoextra R package. To evaluate individual relationships between specific variables and tire-derived compound contamination, samples were grouped by country of growth, leafy vegetable type, wastewater treatment plant of irrigation water origin, compost application, and growth season. For the last three variables, information was only available for samples from Israel, thus other samples were excluded from these two analyses. Analysis of Variance (ANOVA) was then performed to check for statistically significant variation in either cumulative tire-derived compound concentration or number of tire-derived compounds detected between the different groups. Exposure was estimated for the adult Israeli population based concentrations in samples from Israel, and consumption data from the 2nd Israeli National Health and Nutrition Survey (Ben Mordechay et al., 2022b). Exposure to tire-derived compounds in leafy vegetables was compared with exposure to pharmaceuticals from the same leafy vegetable samples, which was assessed in Ben Mordechay et al., 2022 (Ben Mordechay et al., 2022b). Exposure was estimated for the adult Swiss population based on concentrations in samples purchased in Switzerland. Swiss leafy vegetable consumption data was not readily available, but the EFSA Comprehensive European Food Consumption Database (European Food Safety Authority, 2022) provided consumption data for neighbouring countries Austria, France, and Italy, so Swiss consumption was based on a weighted average of those values. Exposure was calculated with the following equation:
3 Results
Out of the sixteen compounds analyzed, six were detected in at least one leafy vegetable sample: benzothiazole (maximum concentration—238 ng/g), 2-hydroxybenzothiazole (maximum concentration—665 ng/g), DPG (maximum concentration—2.1 ng/g), 6PPD (maximum concentration—0.4 ng/g), IPPD (maximum concentration—0.1 ng/g), CPPD (maximum concentration—0.3 ng/g). The number of tire-derived compounds per sample ranged between 0 (n = 8 samples) and four (n = 2 samples). At least one tire-derived compound was detected above limit of quantification (LOQ) in 71% of the samples. All measured concentrations are presented in Figure 1.
Out of the sixteen tire-derived compounds analyzed, benzothiazole was the most frequently detected (detection frequency of 42.9%). 2-aminobenzothiazole, 2-mercaptobenzothiazole, and 2-hydroxybenzothiazole were not detected (with the exception of 2-hydroxybenzothiazole in CH-04). The only benzotriazole derivative analyzed, 5-methyl-1H-benzotriazole, was not detected. DPG was detected in 21.4% of samples. The detection frequencies of 6PPD, IPPD, and CPPD were 25%, 10.7%, and 3.6%, respectively. DPPD was not detected, nor were the respective quinone derivatives. HMMM and aniline were also not detected.
Divisive hierarchical clustering analysis was performed to identify groups of samples with similar patterns in tire-derived compound concentrations. Most samples clustered together, with the exception of two samples: one lettuce grown in Switzerland (CH-04) contained the highest measured concentrations of DPG (2.1 ng/g dry weight) and 6PPD (0.4 ng/g dry weight), and lettuce grown in Israel (IL-01) contained the highest measured benzothiazole concentration in any sample (238 ng/g dry weight), and the only detection of 2-hydroxybenzothiazole (665 ng/g dry weight), which was also the highest measured concentration of any compound. Plastic factories were located next to lettuce growth sites or distribution centers for both samples, but our data did not allow us to prove that they were a source of emissions. In addition, sample CH-04 was next to a major highway, potentially explaining the high concentrations (further discussion on these two samples is provided in Supplementary Section S4). For these reasons, the two samples were classified as outliers and excluded from further analyses. Analysis of Variance (ANOVA) did not reveal any statistically significant (p < 0.05) relationships between tire-derived compound contamination in leafy vegetables and growth country, crop type, recycled wastewater source, compost application, or growth season (Supplementary Table S5).
With a suspect screening, we tentatively identified two tire-derived compound transformation products which have been previously reported. HMMM TP546 was identified in hydroponically grown lettuce plants exposed to HMMM (Castan et al., 2023), and was proposed to be an amino acid conjugate. Here, HMMM TP546 was detected with a maximum signal in samples CH-05 and ES-02. 6PPD TP194 is a known transformation product of 6PPD which has previously been reported in snow and wastewater treatment plant influent (Seiwert et al., 2022), and was detected with a maximum signal in sample IL-12. According to Schymanski et al. (2014), both compounds were assigned a confidence level of 3, “tentative candidate,” since their exact mass and several MS2 fragments correspond to proposed compounds, but MS2 spectral matching was not thorough enough to merit higher confidence (details Supplementary Section S5). We were unable to identify any further transformation products with non-target analyses, so exposure was assessed based on only the sixteen target tire-derived compounds.
Estimated daily intake (EDI) via leafy vegetable consumption of the detected tire-derived compounds for the Israeli and Swiss adult populations are presented in Table 1. No EDI values are presented for 2-hydroxybenzothiazole, since it was only detected in an outlier sample. Based on mean concentrations measured, and mean leafy vegetable consumption, EDIleafy vegetable ranged from 0.04 ng/person/day of IPPD to 52 ng/person/day of benzothiazole. Under the maximum concentration scenario, EDIleafy vegetable was between one and two orders of magnitude higher for all compounds, and ranged from 1.1 ng/person/day of IPPD to 1,296 ng/person/day of benzothiazole.

Table 1. Leafy vegetable consumption (g/person/day) and estimated daily intake (ng/person/day) via consumption of leafy vegetables of five tire-derived compounds for adult national populations. Both the mean scenario and maximum scenario are shown, (mean/maximum). Note that 2-hydroxybenzothiazole is excluded from estimated daily intake calculations, since it was only detected in one outlier sample.
4 Discussion
The uptake of pharmaceuticals from reclaimed wastewater into edible crops has been well established with both mechanistic (Shenker et al., 2011; Goldstein et al., 2014; Chuang et al., 2019; Kodešová et al., 2019; Li et al., 2019; Li et al., 2022; Zellner et al., 2023) and monitoring studies (Wu et al., 2014; Ben et al., 2021). Several laboratory studies have demonstrated that other classes of compounds, including industrial compounds (Sun et al., 2018; Yu et al., 2021; Gu et al., 2023), plasticizers (Du et al., 2009; Li et al., 2014), and even tire-derived compounds (LeFevre et al., 2016; LeFevre et al., 2017; Castan et al., 2023) can be taken up by plants. However, the occurrence of tire-derived compounds in consumer produce has not previously been established. Here, we report concentrations of sixteen tire-derived compounds in commercial leafy vegetable samples grown in four countries (Switzerland, Italy, Spain, and Israel). The samples collected in Israel have been previously analyzed for 65 pharmaceuticals (Ben et al., 2021), enabling a direct comparison of detection frequency and concentration between tire-derived compounds and pharmaceuticals (Supplementary Table S6). Generally, the samples with the highest pharmaceutical concentrations detected also had the highest tire-derived compound concentrations. Concentrations of individual compounds in the Israeli sample set ranged greatly, both for tire-derived compounds (not detected to 665 ng/g) and pharmaceuticals (not detected to 1,470 ng/g).
Detection frequencies also varied greatly among both tire-derived compounds in the sample set from Israel (0%–38.5%) and pharmaceuticals (0%–100%). This variability is unsurprising since the detection frequencies of both tire-derived compounds and pharmaceuticals in wastewater and surface water bodies also varies highly from compound to compound, due to differences in emissions and environmental stability (Petrie et al., 2013; Seiwert et al., 2020; Johannessen et al., 2021b; Rauert et al., 2022a; Rauert et al., 2022b; Wilkinson et al., 2022). In the agricultural environment, soil sorption, microbial transformation, extent of plant uptake and translocation, and plant metabolism vary greatly for compounds with different chemical structures (Xu et al., 2009; Kodešová et al., 2015; Miller et al., 2016; Kodešová et al., 2019; Li et al., 2019; Li et al., 2022; Ben Mordechay et al., 2023; Muerdter et al., 2023). Benzothiazole was the tire-derived compound with the highest detection frequency of 50% in the samples from Israel, and 42.9% in all samples (Figure 2). Six pharmaceuticals exhibited even greater detection frequencies in leafy vegetables: carbamazepine (100%) and two of its metabolites, epoxide carbamazepine (100%), dihydroxy carbamazepine (85%), lamotrigine (92%), nicotine (85%), and venlafaxine (62%). These compounds were also detected at high frequency in rivers due to their consistent use and emission (Wilkinson et al., 2022). While pharmaceuticals are generally used consistently throughout the year, tire-derived compounds enter wastewater mainly during runoff events, and thus exhibit lower detection frequencies in the environment (Seiwert et al., 2020; Rauert et al., 2022b). Despite inconsistent detections for individual tire-derived compounds, 69% of the samples from Israel contained at least one tire-derived compound, compared with 100% of the same samples which contained at least one pharmaceutical (Ben et al., 2021).
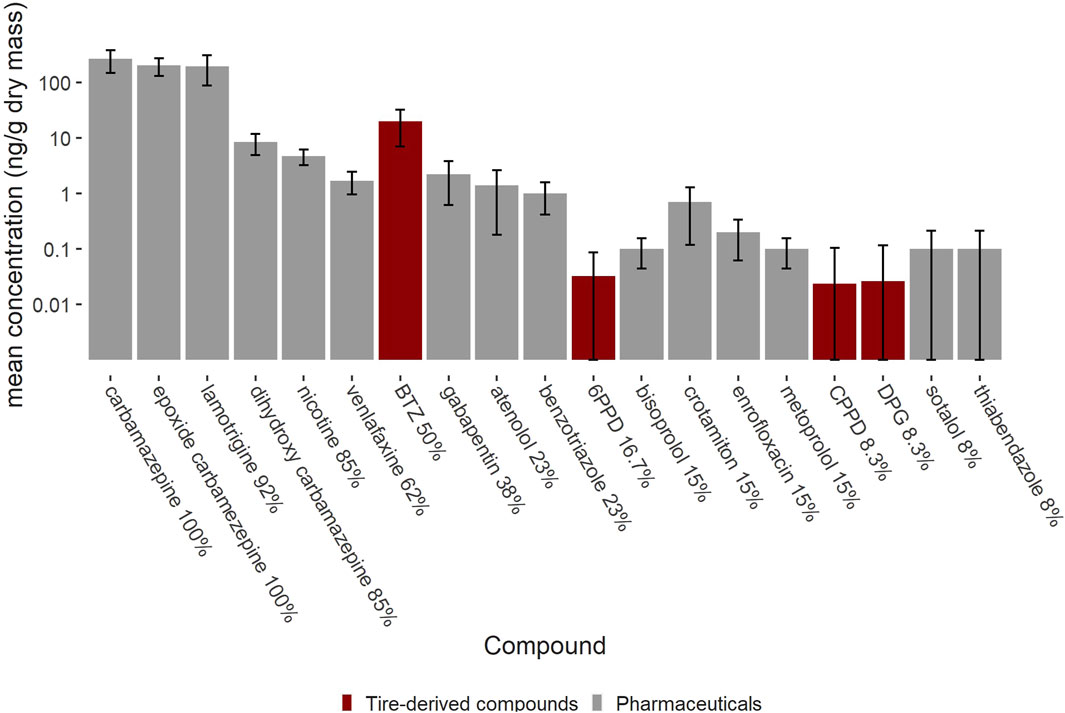
Figure 2. Mean concentrations of pharmaceuticals (gray) and tire-derived compounds (red) measured in leafy vegetable samples from Israel. Standard errors are shown with error bars. Detection frequencies are shown along with the compound names.
Detection frequencies of individual tire-derived compounds could be interpreted based on their physicochemical properties (Supplementary Table S2) and known environmental behavior. It is known that benzothiazole (Castan et al., 2023), as well as 2-mercaptobenzothiazole (LeFevre et al., 2016) can be readily taken up by plants. Unsubstituted benzothiazole was frequently detected (42.9% of samples), while 2-mercaptobenzothiazole, 2-hydroxybenzothaizole, and 2-aminobenzothiazole were not detected. Higher limits of quantification could contribute to the lack of detections for two compounds (75.6 and 55.6 ng/g for 2-mercaptobenzothiazole and 2-hydroxybenzothiazole, respectively), but 2-aminobenzothiazole had a very low limit of quantification (1.1 ng/g). Similarly, 5methyl-1H-benzotriazole was not detected (limit of quantification 2.8 ng/g), although unsubstituted benzotriazole was detected in 23% of the same leafy vegetable samples when they were previously analyzed (Ben et al., 2021). In addition to analytical bias, the differences in detection frequencies could be related to differences in occurrence and concentrations of the compounds in the agricultural environment, differences in physicochemical properties affecting plant uptake, or differences in plant metabolism. Benzothiazoles and benzotriazoles are widely occurring compounds. Benzothiazoles are also used as biocides and food flavorings, and benzothiazole has even been reported to occur naturally in products such as tea leaves (Vitzthum et al., 1975). In fact, benzothiazole has been detected at similar concentrations to those we report in several food products (Flavor and Extract Manufacturers Association, 1997; Liao et al., 2018), so the high detection frequency of benzothiazole compared to the other tire-derived compounds analyzed could simply be a result of its environmental ubiquity.
Soil sorption can reduce the availability of a compound for root uptake. All studied benzothiazoles and 5-methyl-1H-benzotriazole are neutral in the pH range of most soils and are relatively hydrophilic (log KOW of 1.13–2.35, Supplementary Table S2), implying low sorption and high bioavailability to plants for root uptake. 5-methyl-1H-benzotriazole is known to exhibit low soil sorption (Kodešová et al., 2023). The log KOW values of benzothiazoles and benzotriazole are also within the optimal range for root uptake and translocation (Briggs et al., 1982; Chuang et al., 2019). While differential availability for plant uptake may not explain differences in detection frequencies between benzothiazoles and benzotriazoles, it has been reported that the extent of plant uptake and metabolism can be altered by the location and functional group properties of substitutions in benzimidazole and benzotriazoles (Muerdter et al., 2023), which are structurally similar to benzothiazoles. This study showed that Aridopsis plants rapidly and completely depleted unsubstituted benzotriazole from hydroponic medium, while 2-aminobenzotriazole was not depleted, suggesting that substitutions can substantially reduce the uptake of benzotriazoles (Muerdter et al., 2023) and could explain the difference in detection frequency between benzotriazole and 5-methyl-1H-benzotriazole. Furthermore, plant metabolism can occur rapidly, and could contribute to lack of detections. Hydroxy-, primary amino-, and mercapto-groups can be sites of direct conjugation in plants (Schröder and Willey, 2007; Sun et al., 2018), leading to fast transformation kinetics, which could explain the absence of 2-mercaptobenzothiazole, 2-hydroxybenzothiazole and 2-aminobenzothiazole, while benzothiazole was frequently detected. In a comparative metabolomics experiment, after 24 h, a substantial proportion of unsubstituted benzimidazole remained in Arabidopsis plants, while substituted benzimidazoles were further metabolized (Muerdter et al., 2023). This pattern likely holds true for benzothiazoles, which share a very similar base structure with benzimidazoles. Although benzothiazole was rapidly metabolized in lettuce plants in a hydroponic experiment (Castan et al., 2023), it could be more recalcitrant compared to its substituted derivatives in plants grown on the field.
The detection frequencies of the four PPDs analyzed corresponds to usage of the individual compounds. 6PPD (25%), is the most used PPD, followed by IPPD (10.7%), and then the much less used CPPD (3.6%) and DPPD (not detected) (Datta et al., 2007). All four PPDs have detection frequencies >50% in air, soil, and runoff water, with 6PPD dominating the concentration profile in all sample types (Wang et al., 2022b; Cao et al., 2022). Although 6PPD is known to be unstable in aqueous systems (Seiwert et al., 2022), it was detected in 25% of leafy vegetable samples suggesting that it may be more stable in terrestrial environments and/or within plants. On the other hand, it could also suggest the presence of tire and road wear particles on the field, which can act as a long-term source of 6PPD to plants, replenishing losses of 6PPD (Castan et al., 2023). In general, PPDs were present at very low concentrations (<1 ng/g). PPD-quinones were not detected in any samples, likely due to slightly higher limits of quantification (1.1–2.8 ng/g) than their respective PPDs (0.05–0.6 ng/g). PPD-quinones have been reported to occur in air, soil, and runoff water at similar or even higher concentrations to their parent PPDs (Wang et al., 2022b; Cao et al., 2022), however their concentrations in the agricultural environment are unknown. PPDs are relatively hydrophobic (log KOW of 3.28–4.93 [Cao et al., 2022; Hu et al., 2023)]. PPD-quinones are also hydrophobic [log KOW of 2.58–4.30 (14,79)], and could exhibit specific binding at the quinone moiety. This results in a high sorption potential for 6PPD-quinone (Hu et al., 2023; Lokesh et al., 2023), the same is presumably true for other PPD-quinones. Hydrophobic compounds are also known to accumulate in plant roots, as has been previously shown for 6PPD and 6PPD-quinone (Castan et al., 2023), so future work should investigate the occurrence of these tire-derived compounds in root vegetables, such as carrots or potatoes. It is also likely that PPDs and PPD-quinones in the soil environment would sorb to soil organic matter, which could limit the availability for root uptake (Li et al., 2019; Li et al., 2022). However, it is also known that for crops which grow close to the ground, such as leafy vegetables, attachment of soil particles to leaves can introduce hydrophobic contaminants to the crop. This could explain the detection of PPDs in the leafy vegetables despite their hydrophobicity.
HMMM was not detected in any samples, although it was shown to have high uptake into lettuce plants in a hydroponic study (Castan et al., 2023), and had a low limit of quantification of 1.1 ng/g. This could imply that HMMM was not present in the agricultural environment to begin with, or that it was not available for plant uptake due to sorption, or transformation in soil, or that it was rapidly transformed in the plant. Irreversible loss of HMMM was demonstrated in multiple soils (Tang and Kolodziej, 2022), although the mechanism is not currently known. Likewise, DPG was taken up by lettuce under hydroponic conditions (Castan et al., 2023), but demonstrated high sorption in multiple soils due to its positive charge (Tang and Kolodziej, 2022), implying a low availability of DPG for root uptake. In this study, DPG was detected in 21.4% of samples. This observation could suggest that attachment of soil particles to leafy vegetables is a relevant uptake pathway for DPG (Pinder and McLeod, 1988). Aniline was not detected, which could be an artifact of a higher limit of quantification (27.8 ng/g), low occurrence of aniline in the agricultural environment, or plant metabolism (Marco and Novak, 1991).
This study was designed to provide a first overview of tire-derived compound levels in leafy vegetables, not to determine the source of the tire-derived compounds. However, we had assumed that tire-derived compounds are introduced to the agricultural environment predominantly through irrigation with reclaimed wastewater or application of biosolids as fertilizer. We hypothesized that leafy vegetables grown in countries where both practices are prohibited (i.e., Switzerland) would have much lower levels of tire-derived compounds than leafy vegetables from Israel, where all samples were irrigated with reclaimed wastewater. Contrary to our hypothesis, we did not observe any statistical relationships between country of origin, and tire-derived compound levels in the leafy vegetables. Differences in reclaimed wastewater quality originating from different wastewater treatment plants had a large influence on concentrations of pharmaceuticals in edible crops (Ben et al., 2021; Ben Mordechay et al., 2022a) and we had hypothesized that the same would be true for tire-derived compounds, but this trend was not observed (Supplementary Table S5). We also did not observe a statistically significant relationship between tire-derived compound levels and the season in which samples from the Israeli sample set were grown, although we had hypothesized that distinct rainy and dry seasons in Israel would lead to different levels of tire-derived compounds entering the agricultural environment. These observations could be due to our small sample size. Additionally, the flux of tire-derived compounds into wastewater treatment plants is associated with road-wash events, which occur irregularly as compared to the relatively constant emissions of pharmaceuticals. Irregular emissions of tire-derived compounds make it hard to compare reclaimed wastewater quality between different wastewater treatment plants, while for pharmaceuticals a clear trend was observed (Ben et al., 2021; Ben Mordechay et al., 2022a). Overall, the lack of relationship between known growth conditions and tire-derived compound levels in leafy vegetables (Supplementary Table S5) could imply that there are multiple pathways by which tire-derived compounds reach the agricultural environment, and future studies are needed to uncover these pathways in order to guide agriculture policy. In particular, atmospheric deposition of tire and road wear particles may be an equally or more relevant pathway compared to application of biosolids or irrigation with reclaimed wastewater. Atmospheric deposition may be particularly relevant for leafy vegetables which were grown close to major roads. It should be further investigated whether foliar uptake is possible for particles which settle directly on leafy vegetables and whether agricultural policy measures, such as a minimum distance between roads and agricultural areas can reduce the entrance of tire-derived compounds to the agricultural environment. We did observe much higher contamination of two samples grown near plastic factories. Although these data are too limited to draw conclusions, future research should investigate whether plastic factories can act as point sources of tire-derived compounds to the agricultural environment.
Other than two tentatively (confidence level 3) identified transformation products, we were unable to identify transformation products in leafy vegetable samples. This is relatively unsurprising—since tire-derived compounds were generally present in leafy vegetable samples at trace levels, it can be expected that their transformation products were also present at very low concentrations. It is a major analytical challenge to prioritize and identify unknown compounds at such low concentrations with non-target mass spectrometry, particularly when the compounds are present in a complex matrix, such as plant extracts. Often, statistical techniques can be employed to overcome this challenge. For example, ratios of signal between exposed plants and controls (non-exposed plants) are typically used to prioritize potential transformation products. In the case of time-series experiments, trends in signal over time can also be used to identify potential transformation products. However, a survey study is ill-suited for such analyses, since there are neither time series data, nor true controls (leafy vegetable samples which definitely do not contain any tire-derived compounds or transformation products). Our expected compounds analysis screened all samples for the exact masses of products resulting from sixteen tire-derived compounds undergoing all possible permutations of nine Phase I and thirty-two Phase II transformations—this search identified 1,195 potential matches. Without a way to statistically prioritize these potential transformation products, the results of this analysis could not be used.
On the other hand, molecular networking is a more specific approach, since it groups known compounds (tire-derived compounds) with unknown compounds (transformation products) based on similarities in MS2 fragmentation spectra, which imply structural similarities. However, in non-target high resolution mass spectrometry using data-dependent acquisition, MS2 spectra are only collected for compounds exhibiting the highest signals at a given retention time. In this case, it is likely that tire-derived compound transformation products did not fulfill this criterium. Thus, using molecular networks analysis, we did not identify any unknown compounds with meaningful (m/z > 60, minimum 2 related fragments) spectral similarities. This is unsurprising, since endogenous plant molecules are likely to be much more abundant than any tire-derived compound transformation products in leafy vegetable extracts, and thus, data acquired in data-dependent MS2 acquisition mode was poorly suited for molecular network analysis.
These results do not mean that transformation products are not present in consumer produce, or not relevant. On the contrary, it is well established that target monitoring of organic contaminants, including tire-derived compounds, often under-estimates exposure, since a large fraction of organic contaminants in plants are present as transformation products (Lefevre et al., 2015a; LeFevre et al., 2017; Cheng et al., 2021; Wiener and LeFevre, 2022; Castan et al., 2023). To fill this data gap, mechanistic plant metabolomic studies are needed to identify in-plant transformation products of tire-derived compounds. These studies should be followed up with suspect screenings of identified products in real consumer produce to thoroughly assess exposure to tire-derived compounds via produce consumption.
The above discussion highlights the many open questions regarding the sources and fate processes of tire-derived compounds in the agricultural environment. Future research is needed to fully understand the processes which control the flux of tire-derived compounds from the road to food. As future research directions, we suggest: 1) quantification of tire-derived compounds in biosolids and reclaimed wastewater, 2) investigation of sorption and transformation of tire-derived compounds in agricultural soils, and 3) identification of in-plant transformation products of tire-derived compounds, and development of methods capable of measuring these transformation products in real samples.
Based on mean as well as maximum tire-derived compound concentrations, and mean and 95th percentile leafy vegetable consumption, we provide estimated daily intakes via leafy vegetable consumption (EDIleafy vegetable) in Table 1. For most compounds, the EDIleafy vegetable in the maximum scenario exceeds the mean scenario by more than an order of magnitude. This is due to both large differences between the maximum and the mean tire-derived compound concentrations in leafy vegetables, as well as between the 95th percentile and mean leafy vegetable consumption in both the Swiss and Israeli populations. The EDIleafy vegetable based on mean concentrations is generally low compared to other exposure pathways, which are also calculated based on mean environmental concentrations. For example, the EDIleafy vegetable of DPG in the mean case (0.05–0.3 ng/person/day) was lower than via dust ingestion (0.7–60.9 ng/person/day) (Li Z-M. and Kannan, 2023), or drinking water (72.1 ng/person/day) (Zhang et al., 2023), although the exposure from drinking water was estimated based on only one water sample. Benzothiazole EDIleafy vegetable values in the mean scenario (12–52 ng/person/day) are slightly higher than those reported for ∑5 benzothiazole derivatives via inhalation of outdoor air near the workplace (1.4–4.2 ng/person/day) and near the home (3.5–9.1 ng/person/day) (Maceira et al., 2018), and comparable to EDI from drinking water [36.2 ng/person/day (Zhang et al., 2023)]. Total EDI for benzothiazoles has been calculated based on measured concentrations in urine to be 4.8–18.2 μg/person/day (Asimakopoulos et al., 2013), which would suggest that leafy vegetable consumption, inhalation, and drinking water all contribute as only minor sources to total benzothiazole exposure. Potential explanations for this large discrepancy could be that major exposure routes to benzothiazole are still unknown. Another explanation could be that target analyses have underestimated benzothiazole uptake, if benzothiazole is mostly taken up in conjugated (or otherwise transformed) form, and subsequently deconjugated in the body (Cheng et al., 2021). The EDIleafy vegetable of 6PPD and other PPD derivatives via leafy vegetable consumption in the mean scenario (0.04–0.2 ng/person/day) were in the same range as EDI via inhalation of ∑5PPDs (0.01–0.04) (Cao et al., 2022) for general residents, but much lower than for roadside workers (IPPD: 4.2 ng/person/day, 6PPD: 13.3 ng/person/day) (Wang et al., 2022a). EDI of PPDs via roadside soil ingestion (∑5PPDs: 35–63 ng/person/day) (Cao et al., 2022) also much exceeded EDIleafy vegetable. However, for people regularly consuming high quantities of highly contaminated leafy vegetables, exposure to tire-derived compounds will be much higher (EDIleafy vegetable maximum scenario).
EDIleafy vegetable for a given single compound and scenario varies up to nearly ten-fold between different the Israeli and Swiss populations (Table 1), due to differences in both leafy vegetable consumption and tire-derived compound concentrations. Between different compounds, EDIleafy vegetable spans several orders of magnitude—the same is true for EDIleafy vegetable for pharmaceuticals in Israel (Ben Mordechay et al., 2022b). For the Israeli adult population, the highest EDIleafy vegetable of a tire-derived compound was that of benzothiazole (mean scenario 52 ng/person/day), which is comparable to the EDIleafy vegetable of nicotine (40 ng/person/day), caffeine (20 ng/person/day), and sulfamethoxazole (20 ng/person/day), but much lower than EDIleafy vegetable for carbamazepine (870 ng/person/day) or lamotrigine (570 ng/person/day) (Ben Mordechay et al., 2022b). Leafy vegetables tend to accumulate organic contaminants more than other crop types (Wu et al., 2014; Ben et al., 2021), so our data can be used as a starting point for estimating tire-derived compound dietary intake, although the total is likely to be higher since leafy vegetables represent only a fraction of total diet.
Currently, there are limited data regarding effects of tire-derived compounds on human health (Baensch-Baltruschat et al., 2020; Zoroufchi Benis et al., 2023). A no observable effect level of 357 mg/day was proposed for benzothiazole based on a rat study (Abbott and Renwick, 2003), which is substantially above EDIleafy vegetable for the mean and maximum concentration scenario. 6PPD has demonstrated moderate toxicity in various rodent species (OECD, 2004), while 6PPD-quinone demonstrates a species-specific toxicity in fish (Zoroufchi Benis et al., 2023). However, human toxicity of tire-derived compounds has not been systematically evaluated, thus, the risk associated with this exposure cannot be assessed. Future work addressing toxicity associated with ingestion of tire-derived compounds should consider mixture toxicity, since we show that multiple compounds co-occur along with pharmaceuticals in leafy vegetable produce. Additionally, uptake and exposure might be higher in other types of produce. Due to a limited number of samples, and a lack of information about their specific growth conditions, we were unable to implicate specific sources of tire-derived compounds. Future work is needed to quantify the fluxes of tire-derived compounds to the agricultural environment, assess the fate of tire-derived compounds in the agricultural environment, and assess the contribution of transformation products to total tire-derived compound exposure. Future research should also address the biological effects of ingestion of tire-derived compounds.
Data availability statement
The original contributions presented in the study are included in the article/Supplementary Material, further inquiries can be directed to the corresponding author.
Author contributions
AS: Conceptualization, Formal Analysis, Investigation, Methodology, Project administration, Validation, Visualization, Writing–original draft, Writing–review and editing. LH: Investigation, Writing–review and editing. EB: Conceptualization, Resources, Writing–review and editing. BC: Conceptualization, Funding acquisition, Writing–review and editing. THü: Conceptualization, Funding acquisition, Supervision, Writing–review and editing. THo: Conceptualization, Funding acquisition, Resources, Supervision, Writing–review and editing.
Funding
The author(s) declare that financial support was received for the research, authorship, and/or publication of this article. This project was primarily funded by the HUJI-UNIVIE Joint Research Grant 2022. AS work was funded by the research platform PLENTY—Plastics in the Environment and Society, from the University of Vienna. THo acknowledges funding from the Austrian Science Fund, Cluster of Excellence COE7.
Acknowledgments
THo and THu¨ acknowledge co-funding by the European Union, Grant Agreement Number 101059546—TwinSubDyn.
Conflict of interest
The authors declare that the research was conducted in the absence of any commercial or financial relationships that could be construed as a potential conflict of interest.
Publisher’s note
All claims expressed in this article are solely those of the authors and do not necessarily represent those of their affiliated organizations, or those of the publisher, the editors and the reviewers. Any product that may be evaluated in this article, or claim that may be made by its manufacturer, is not guaranteed or endorsed by the publisher.
Supplementary material
The Supplementary Material for this article can be found online at: https://www.frontiersin.org/articles/10.3389/fenvs.2024.1384506/full#supplementary-material
References
Abbott, P. J., and Renwick, A. G. (2003) WHO food additives series: 50 sulfur-containing heterocyclic compounds. Geneva. Available at: https://www.inchem.org/documents/jecfa/jecmono/v50je12.htm#2.3.2.2.
Admin (2024). Ban on the use of sludge as a fertiliser. Available at: https://www.admin.ch/gov/en/start/documentation/media-releases.msg-id-1673.html.
Alhelou, R., Seiwert, B., and Reemtsma, T. (2019). Hexamethoxymethylmelamine – a precursor of persistent and mobile contaminants in municipal wastewater and the water cycle. Water Res. 165, 114973. doi:10.1016/j.watres.2019.114973
Asimakopoulos, A. G., Wang, L., Thomaidis, N. S., and Kannan, K. (2013). Benzotriazoles and benzothiazoles in human urine from several countries: a perspective on occurrence, biotransformation, and human exposure. Environ. Int. 59, 274–281. doi:10.1016/j.envint.2013.06.007
Baensch-Baltruschat, B., Kocher, B., Kochleus, C., Stock, F., and Reifferscheid, G. (2021). Tyre and road wear particles - a calculation of generation, transport and release to water and soil with special regard to German roads. Sci. Total Environ. 752, 141939. doi:10.1016/j.scitotenv.2020.141939
Baensch-Baltruschat, B., Kocher, B., Stock, F., and Reifferscheid, G. (2020). Tyre and road wear particles (TRWP) - a review of generation, properties, emissions, human health risk, ecotoxicity, and fate in the environment. Sci. Total Environ. 733, 137823. doi:10.1016/j.scitotenv.2020.137823
Ben, M. E., Mordehay, V., Tarchitzky, J., and Chefetz, B. (2021). Pharmaceuticals in edible crops irrigated with reclaimed wastewater: evidence from a large survey in Israel. J. Hazard Mater 416, 126184. doi:10.1016/j.jhazmat.2021.126184
Ben Mordechay, E., Mordehay, V., Tarchitzky, J., and Chefetz, B. (2022a). Fate of contaminants of emerging concern in the reclaimed wastewater-soil-plant continuum. Sci. Total Environ. 822, 153574. doi:10.1016/j.scitotenv.2022.153574
Ben Mordechay, E., Shenker, M., Tarchitzky, J., Mordehay, V., Elisar, Y., Maor, Y., et al. (2023). Wastewater-derived contaminants of emerging concern: concentrations in soil solution under simulated irrigation scenarios. Soil Environ. Heal 1, 100036. doi:10.1016/j.seh.2023.100036
Ben Mordechay, E., Sinai, T., Berman, T., Dichtiar, R., Keinan-Boker, L., Tarchitzky, J., et al. (2022b). Wastewater-derived organic contaminants in fresh produce: dietary exposure and human health concerns. Water Res. 223, 118986. doi:10.1016/j.watres.2022.118986
Brahney, J., Mahowald, N., Prank, M., Cornwell, G., Klimont, Z., Matsui, H., et al. (2021). Constraining the atmospheric limb of the plastic cycle. Proc. Natl. Acad. Sci. U. S. A. 118, 20207191188–e2020719210. doi:10.1073/pnas.2020719118
Briggs, G. G., Bromilow, R. H., and Evans, A. A. (1982). Relationships between lipophilicity and root uptake and translocation of non-ionised chemicals by barley. Pestic. Sci. 13, 495–504. doi:10.1002/ps.2780130506
Cao, G., Wang, W., Zhang, J., Wu, P., Zhao, X., Yang, Z., et al. (2022). New evidence of rubber-derived quinones in water, air, and soil. Environ. Sci. Technol. 56, 4142–4150. doi:10.1021/acs.est.1c07376
Castan, S., Sherman, A., Peng, R., Zumstein, M. T., Wanek, W., Hüffer, T., et al. (2023). Uptake, metabolism, and accumulation of tire wear particle-derived compounds in lettuce. Environ. Sci. Technol. 57, 168–178. doi:10.1021/acs.est.2c05660
Cheng, Z., Sun, H., Sidhu, H. S., Sy, N. D., Wang, X., and Gan, J. (2021). Conjugation of di-n-butyl phthalate metabolites in arabidopsis thaliana and potential deconjugation in human microsomes. Environ. Sci. Technol. 55, 2381–2391. doi:10.1021/acs.est.0c07232
Chibwe, L., Parrott, J. L., Shires, K., Khan, H., Clarence, S., Lavalle, C., et al. (2022). A deep dive into the complex chemical mixture and toxicity of tire wear particle leachate in fathead minnow. Environ. Toxicol. Chem. 41, 1144–1153. doi:10.1002/etc.5140
Chuang, Y. H., Liu, C. H., Sallach, J. B., Hammerschmidt, R., Zhang, W., Boyd, S. A., et al. (2019). Mechanistic study on uptake and transport of pharmaceuticals in lettuce from water. Environ. Int. 131, 104976. doi:10.1016/j.envint.2019.104976
Collivignarelli, M. C., Abbà, A., Frattarola, A., Miino, M. C., Padovani, S., Katsoyiannis, I., et al. (2019). Legislation for the reuse of biosolids on agricultural land in Europe: overview. Sustain 11, 6015. doi:10.3390/su11216015
Datta, R. N., Huntink, N. M., Datta, S., and Talma, A. G. (2007). Rubber vulcanizates degradation and stabilization. Rubber Chem. Technol. 80, 436–480. doi:10.5254/1.3548174
Departement für Umwelt Verkehr Energie und Kommunikation (UVEK) (2021) Gereinigtes Abwasser für die Bewässerung nutzen. 217573 FRAGESTUNDE Fr. Available at: https://www.parlament.ch/de/ratsbetrieb/suche-curia-vista/geschaeft?AffairId=20217573.
Du, Q. Z., Fu, X. W., and Xia, H. L. (2009). Uptake of di-(2-ethylhexyl)phthalate from plastic mulch film by vegetable plants. Food Addit. Contam. - Part A Chem. Anal. Control Expo. Risk Assess. 26, 1325–1329. doi:10.1080/02652030903081952
European Food Safety Authority (2022). EFSA comprehensive European food consumption Database. Available at: https://www.efsa.europa.eu/en/data-report/food-consumption-data#the-efsa-comprehensive-european-food-consumption-database.
European Parliament, The Council of the European Union (2020) Regulation on minimum requirements for water reuse. European Union.
Fohet, L., Andanson, J.-M., Charbouillot, T., Malosse, L., Leremboure, M., Delor-Jestin, F., et al. (2023). Time-concentration profiles of tire particle additives and transformation products under natural and artificial aging. Sci. Total Environ. 859, 160150. doi:10.1016/j.scitotenv.2022.160150
Gianico, A., Braguglia, C. M., Gallipoli, A., Montecchio, D., and Mininni, G. (2021). Land application of biosolids in europe: possibilities, con-straints and future perspectives. WaterSwitzerl. 13, 103. doi:10.3390/w13010103
Goldstein, M., Shenker, M., and Chefetz, B. (2014). Insights into the uptake processes of wastewater-borne pharmaceuticals by vegetables. Environ. Sci. Technol. 48, 5593–5600. doi:10.1021/es5008615
Grigoratos, T., and Martini, G. (2014). Non-exhaust traffic related emissions. Brake tyre wear PM. doi:10.2790/21481
Gu, Q., Wen, Y., Wu, H., and Cui, X. (2023). Uptake and translocation of both legacy and emerging per- and polyfluorinated alkyl substances in hydroponic vegetables. Sci. Total Environ. 862, 160684. doi:10.1016/j.scitotenv.2022.160684
Hu, X., Zhao, H., Tian, Z., Peter, K. T., Dodd, M. C., and Kolodziej, E. P. (2023). Chemical characteristics, leaching, and stability of the ubiquitous tire rubber-derived toxicant 6PPD-quinone. Environ. Sci. Process Impacts 25, 901–911. doi:10.1039/d3em00047h
Hu, X., Zhao, H. N., Tian, Z., Peter, K. T., Dodd, M. C., and Kolodziej, E. P. (2022). Transformation product formation upon heterogeneous ozonation of the tire rubber antioxidant 6PPD (N -(1,3-dimethylbutyl)- N ′-phenyl- p -phenylenediamine). Environ. Sci. Technol. Lett. 9, 413–419. doi:10.1021/acs.estlett.2c00187
Jimenez, B., and Asano, T. (2008). Water reclamation and reuse around the world. Water Reuse Int Surv Curr Pract issues needs 14, 3–26.
Johannessen, C., Helm, P., and Metcalfe, C. D. (2021a). Runoff of the tire-wear compound, hexamethoxymethyl-melamine into urban watersheds. Arch. Environ. Contam. Toxicol. 82, 162–170. doi:10.1007/s00244-021-00815-5
Johannessen, C., Helm, P., and Metcalfe, C. D. (2021b). Detection of selected tire wear compounds in urban receiving waters. Environ. Pollut. 287, 117659. doi:10.1016/j.envpol.2021.117659
Kodešová, R., Fedorova, G., Kodeš, V., Kočárek, M., Rieznyk, O., Fér, M., et al. (2023). Assessment of potential mobility of selected micropollutants in agricultural soils of the Czech Republic using their sorption predicted from soil properties. Sci. Total Environ. 865, 161174. doi:10.1016/j.scitotenv.2022.161174
Kodešová, R., Grabic, R., Kočárek, M., Klement, A., Golovko, O., Fér, M., et al. (2015). Pharmaceuticals’ sorptions relative to properties of thirteen different soils. Sci. Total Environ. 511, 435–443. doi:10.1016/j.scitotenv.2014.12.088
Kodešová, R., Klement, A., Golovko, O., Fér, M., Kočárek, M., Nikodem, A., et al. (2019). Soil influences on uptake and transfer of pharmaceuticals from sewage sludge amended soils to spinach. J. Environ. Manage 250, 109407. doi:10.1016/j.jenvman.2019.109407
Kole, P. J., Löhr, A. J., Van Belleghem, FGAJ, and Ragas, A. M. J. (2017). Wear and tear of tyres: a stealthy source of microplastics in the environment. Int. J. Environ. Res. Public Health 14, 1265. doi:10.3390/ijerph14101265
LeFevre, G. H., Lipsky, A., Hyland, K. C., Blaine, A. C., Higgins, C. P., and Luthy, R. G. (2017). Benzotriazole (BT) and BT plant metabolites in crops irrigated with recycled water. Environ. Sci. Water Res. Technol. 3, 213–223. doi:10.1039/C6EW00270F
LeFevre, G. H., Müller, C. E., Li, R. J., Luthy, R. G., and Sattely, E. S. (2015a). Rapid phytotransformation of benzotriazole generates synthetic tryptophan and auxin analogs in arabidopsis. Environ. Sci. Technol. 49, 10959–10968. doi:10.1021/acs.est.5b02749
Lefevre, G. H., Portmann, A. C., Müller, C. E., Sattely, E. S., and Luthy, R. G. (2015b). Plant assimilation kinetics and metabolism of 2-mercaptobenzothiazole tire rubber vulcanizers by arabidopsis. Environ. Sci. Technol. 50, 6762–6771. doi:10.1021/acs.est.5b04716
LeFevre, G. H., Portmann, A. C., Müller, C. E., Sattely, E. S., and Luthy, R. G. (2016). Plant assimilation kinetics and metabolism of 2-mercaptobenzothiazole tire rubber vulcanizers by arabidopsis. Environ. Sci. Technol. 50, 6762–6771. doi:10.1021/acs.est.5b04716
Li, Y., Sallach, J. B., Zhang, W., Boyd, S. A., and Li, H. (2019). Insight into the distribution of pharmaceuticals in soil-water-plant systems. Water Res. 152, 38–46. doi:10.1016/j.watres.2018.12.039
Li, Y., Sallach, J. B., Zhang, W., Boyd, S. A., and Li, H. (2022). Characterization of plant accumulation of pharmaceuticals from soils with their concentration in soil pore water. Environ. Sci. Technol. 56, 9346–9355. doi:10.1021/acs.est.2c00303
Li, Y. W., Cai, Q. Y., Mo, C. H., Zeng, Q. Y., Lü, H., Li, Q. S., et al. (2014). Plant uptake and enhanced dissipation of di(2-ethylhexyl) phthalate (DEHP) in spiked soils by different plant species. Int. J. Phytoremediation 16, 609–620. doi:10.1080/15226514.2013.803021
Li, Z., and Kannan, K. (2023a). Determination of 1,3-diphenylguanidine, 1,3-Di-o-tolylguanidine, and 1, 2, 3-triphenylguanidine in human urine using liquid chromatography-tandem mass spectrometry. Environ. Sci. Technol. 57, 8883–8889. doi:10.1021/acs.est.3c00412
Li, Z.-M., and Kannan, K. (2023b). Occurrence of 1,3-diphenylguanidine, 1,3-di- o -tolylguanidine, and 1,2,3-triphenylguanidine in indoor dust from 11 countries: implications for human exposure. Environ. Sci. Technol. 57, 6129–6138. doi:10.1021/acs.est.3c00836
Liao, C., Kim, U. J., and Kannan, K. (2018). A review of environmental occurrence, fate, exposure, and toxicity of benzothiazoles. Environ. Sci. Technol. 52, 5007–5026. doi:10.1021/acs.est.7b05493
Lokesh, S., Arunthavabalan, S., Hajj, E., Hitti, E., and Yang, Y. (2023). Investigation of 6PPD-quinone in rubberized asphalt concrete mixtures. ACS Environ. Au 3, 336–341. doi:10.1021/acsenvironau.3c00023
Maceira, A., Marcé, R. M., and Borrull, F. (2018). Occurrence of benzothiazole, benzotriazole and benzenesulfonamide derivates in outdoor air particulate matter samples and human exposure assessment. Chemosphere 193, 557–566. doi:10.1016/j.chemosphere.2017.11.073
Marco, G. J., and Novak, R. A. (1991). Natural product interactions during aniline metabolism including their incorporation in biopolymers. J. Agric. Food Chem. 39, 2101–2111. doi:10.1021/jf00012a001
Mennekes, D., and Nowack, B. (2022). Tire wear particle emissions: measurement data where are you? Sci. Total Environ. 830, 154655. doi:10.1016/j.scitotenv.2022.154655
Miarov, O., Tal, A., and Avisar, D. (2020). A critical evaluation of comparative regulatory strategies for monitoring pharmaceuticals in recycled wastewater. J. Environ. Manage 254, 109794. doi:10.1016/j.jenvman.2019.109794
Miller, E. L., Nason, S. L., Karthikeyan, K. G., and Pedersen, J. A. (2016). Root uptake of pharmaceuticals and personal care product ingredients. Environ. Sci. Technol. 50, 525–541. doi:10.1021/acs.est.5b01546
Mordechay, E. B., Sinai, T., Berman, T., Dichtiar, R., Keinan-boker, L., Tarchitzky, J., et al. (2022). Wastewater-derived organic contaminants in fresh produce: dietary exposure and human health concerns. Water Res. 223, 118986. doi:10.1016/j.watres.2022.118986
Muerdter, C. P., Powers, M. M., Webb, D. T., Chowdhury, S., Roach, K. E., and LeFevre, G. H. (2023). Functional group properties and position drive differences in xenobiotic plant uptake rates, but metabolism shares a similar pathway. Environ. Sci. Technol. Lett. 10, 596–603. doi:10.1021/acs.estlett.3c00282
OECD (2004). SIDS Initial assessment report for SIAM 18. N-(1,3-dimethylbutyl)-N’-phenyl-1,4- phenylendiamine (6PPD). SIDS Initial. Assess. Rep., 1–36.
Petrie, B., McAdam, E. J., Scrimshaw, M. D., Lester, J. N., and Cartmell, E. (2013). Fate of drugs during wastewater treatment. Trac. Trends Anal. Chem. 49, 145–159. doi:10.1016/j.trac.2013.05.007
Pinder, J. E., and McLeod, K. W. (1988). Contaminant transport in agroecosystems through retention of soil particles on plant surfaces. J. Environ. Qual. 17, 602–607. doi:10.2134/jeq1988.00472425001700040014x
Rauert, C., Charlton, N., Okoffo, E. D., Stanton, R. S., Agua, A. R., Pirrung, M. C., et al. (2022b). Concentrations of tire additive chemicals and tire road wear particles in an Australian urban tributary. Environ. Sci. Technol. 56, 2421–2431. doi:10.1021/acs.est.1c07451
Rauert, C., Vardy, S., Daniell, B., Charlton, N., and Thomas, K. V. (2022a). Tyre additive chemicals, tyre road wear particles and high production polymers in surface water at 5 urban centres in Queensland, Australia. Sci. Total Environ. 852, 158468. doi:10.1016/j.scitotenv.2022.158468
Schymanski, E. L., Jeon, J., Gulde, R., Fenner, K., Ruff, M., Singer, H. P., et al. (2014). Identifying small molecules via high resolution mass spectrometry: communicating confidence. Environ. Sci. Technol. 48, 2097–2098. doi:10.1021/es5002105
Seiwert, B., Klöckner, P., Wagner, S., and Reemtsma, T. (2020). Source-related smart suspect screening in the aqueous environment: search for tire-derived persistent and mobile trace organic contaminants in surface waters. Anal. Bioanal. Chem. 412, 4909–4919. doi:10.1007/s00216-020-02653-1
Seiwert, B., Nihemaiti, M., Troussier, M., Weyrauch, S., Reemtsma, T., and Seiwert, B. (2022). Abiotic oxidative transformation of 6-PPD and 6-PPD -quinone from tires and occurrence of their products in snow from urban roads and in municipal wastewater. Water Res. 212, 118122. doi:10.1016/j.watres.2022.118122
Shenker, M., Harush, D., Ben-Ari, J., and Chefetz, B. (2011). Uptake of carbamazepine by cucumber plants - a case study related to irrigation with reclaimed wastewater. Chemosphere 82, 905–910. doi:10.1016/j.chemosphere.2010.10.052
Sun, J., Chen, Q., Qian, Z., Zheng, Y., Yu, S., and Zhang, A. (2018). Plant uptake and metabolism of 2,4-dibromophenol in carrot: in vitro enzymatic direct conjugation. J. Agric. Food Chem. 66, 4328–4335. doi:10.1021/acs.jafc.8b00543
Sunyer-Caldú, A., Golovko, O., Kaczmarek, M., Asp, H., Bergstrand, K.-J., Gil-Solsona, R., et al. (2023). Occurrence and fate of contaminants of emerging concern and their transformation products after uptake by pak choi (Brassica rapa subsp. chinensis). Environ. Pollut. 319, 120958. doi:10.1016/j.envpol.2022.120958
Tang, T., and Kolodziej, E. P. (2022). Sorption and desorption of tire rubber and roadway-derived organic contaminants in soils and a representative engineered geomedium. ACS ES T Water 2, 2623–2633. doi:10.1021/acsestwater.2c00380
Tian, Z., Zhao, H., Peter, K. T., Gonzalez, M., Wetzel, J., Wu, C., et al. (2021). A ubiquitous tire rubber–derived chemical induces acute mortality in coho salmon. Science 371, 185–189. doi:10.1126/science.abd6951
Unice, K. M., Bare, J. L., Kreider, M. L., and Panko, J. M. (2015). Experimental methodology for assessing the environmental fate of organic chemicals in polymer matrices using column leaching studies and OECD 308 water/sediment systems: application to tire and road wear particles. Sci. Total Environ. 533, 476–487. doi:10.1016/j.scitotenv.2015.06.053
Vitzthum, O. G., Werkhoff, P., and Hubert, P. (1975). New volatile constituents of black tea aroma. J. Agric. Food Chem. 23, 999–1003. doi:10.1021/jf60201a032
Wagner, S., Hüffer, T., Klöckner, P., Wehrhahn, M., Hofmann, T., and Reemtsma, T. (2018). Tire wear particles in the aquatic environment - a review on generation, analysis, occurrence, fate and effects. Water Res. 139, 83–100. doi:10.1016/j.watres.2018.03.051
Wang, L., Asimakopoulos, A. G., Moon, H. B., Nakata, H., and Kannan, K. (2013). Benzotriazole, benzothiazole, and benzophenone compounds in indoor dust from the United States and East Asian countries. Environ. Sci. Technol. 47, 4752–4759. doi:10.1021/es305000d
Wang, W., Cao, G., Zhang, J., Wu, P., Chen, Y., Chen, Z., et al. (2022b). Beyond substituted p -phenylenediamine antioxidants: prevalence of their quinone derivatives in PM 2.5. Environ. Sci. Technol. 56, 10629–10637. doi:10.1021/acs.est.2c02463
Wang, Y., Xiang, L., Wang, F., Wang, Z., Bian, Y., Gu, C., et al. (2022a). Positively charged microplastics induce strong lettuce stress responses from physiological, transcriptomic, and metabolomic perspectives. Environ. Sci. Technol. 56, 16907–16918. doi:10.1021/acs.est.2c06054
Wiener, E. A., and LeFevre, G. H. (2022). White rot fungi produce novel tire wear compound metabolites and reveal underappreciated amino acid conjugation pathways. Environ. Sci. Technol. Lett. 9, 391–399. doi:10.1021/acs.estlett.2c00114
Wilkinson, J. L., Boxall, A. B. A., Kolpin, D. W., Leung, K. M. Y., Lai, R. W. S., Galbán-Malagón, C., et al. (2022). Pharmaceutical pollution of the world’s rivers. Proc. Natl. Acad. Sci. 119, 21139471199–e2113947210. doi:10.1073/pnas.2113947119
Winpenny, J., Heinz, I., and Koo-Oshima, S. (2010). The wealth of waste: the economics of wastewater use in agriculture. FAO Water Rep., 1–142. Available at: http://www.fao.org/docrep/012/i1629e/i1629e.pdf.
Wu, X., Conkle, J. L., Ernst, F., and Gan, J. (2014). Treated wastewater irrigation: uptake of pharmaceutical and personal care products by common vegetables under field conditions. Environ. Sci. Technol. 48, 11286–11293. doi:10.1021/es502868k
Xu, J., Wu, L., and Chang, A. C. (2009). Degradation and adsorption of selected pharmaceuticals and personal care products (PPCPs) in agricultural soils. Chemosphere 77, 1299–1305. doi:10.1016/j.chemosphere.2009.09.063
Yu, Q., He, A., Shi, D., and Sheng, G. D. (2021). Translocation versus ion trapping in the root uptake of 2,4-dichlorophenol by wheat seedlings. Environ. Sci. Pollut. Res. 28, 56392–56400. doi:10.1007/s11356-021-14627-6
Zellner, L., Klampfl, C. W., and Himmelsbach, M. (2023). Uptake and metabolization of four sartan drugs by eight different plants: targeted and untargeted analyses by mass spectrometry. Electrophoresis, 1–8. doi:10.1002/elps.202300134
Zhang, H. Y., Huang, Z., Liu, Y. H., Hu, L. X., He, L. Y., Liu, Y. S., et al. (2023). Occurrence and risks of 23 tire additives and their transformation products in an urban water system. Environ. Int. 171, 107715. doi:10.1016/j.envint.2022.107715
Zhao, H. N., Hu, X., Tian, Z., Gonzalez, M., Rideout, C. A., Peter, K. T., et al. (2023). Transformation products of tire rubber antioxidant 6PPD in heterogeneous gas-phase ozonation: identification and environmental occurrence. Environ. Sci. Technol. 57, 5621–5632. doi:10.1021/acs.est.2c08690
Keywords: tire wear particles (TWPs), tire additives, contaminant exposure, plant uptake and accumulation, dietary exposure to chemicals, 6PPD and 6PPD-quinone, food monitoring
Citation: Sherman A, Hämmerle LE, Ben Mordechay E, Chefetz B, Hüffer T and Hofmann T (2024) Uptake of tire-derived compounds in leafy vegetables and implications for human dietary exposure. Front. Environ. Sci. 12:1384506. doi: 10.3389/fenvs.2024.1384506
Received: 16 February 2024; Accepted: 30 April 2024;
Published: 28 May 2024.
Edited by:
Oladele Ogunseitan, University of California, Irvine, United StatesReviewed by:
Nicola Montemurro, Spanish National Research Council (CSIC), SpainKilian E. C. Smith, Magdeburg Stendal University of Applied Sciences, Germany
Leif Abrell, University of Arizona, United States
Copyright © 2024 Sherman, Hämmerle, Ben Mordechay, Chefetz, Hüffer and Hofmann. This is an open-access article distributed under the terms of the Creative Commons Attribution License (CC BY). The use, distribution or reproduction in other forums is permitted, provided the original author(s) and the copyright owner(s) are credited and that the original publication in this journal is cited, in accordance with accepted academic practice. No use, distribution or reproduction is permitted which does not comply with these terms.
*Correspondence: Thilo Hofmann, dGhpbG8uaG9mbWFubkB1bml2aWUuYWMuYXQ=