- 1State Key Laboratory of Desert and Oasis Ecology, Xinjiang Institute of Ecology and Geography, Chinese Academy of Sciences, Urumqi, Xinjiang, China
- 2Xinjiang Key Laboratory of Desert Plant Roots Ecology and Vegetation Restoration, Xinjiang Institute of Ecology and Geography, Chinese Academy of Sciences, Urumqi, Xinjiang, China
- 3Cele National Station of Observation and Research for Desert-Grassland Ecosystems, Cele, Xinjiang, China
- 4College of Ecology and Environment, Xinjiang University, Urumqi, Xinjiang, China
- 5University of Chinese Academy of Sciences, Beijing, China
Introduction: Ultraviolet (UV) radiation is believed to play a significant role in accelerating litter decomposition in water-limited ecosystems. Litter traits also influence the decomposition. However, the dominance of litter traits and ultraviolet radiation on litter decomposition in hyper-arid deserts (annual precipitation: potential evaporation < 0.05) with diverse species and seasonal variations remain unclear.
Methods: To address this knowledge gap, we examined the decomposition of three dominant litter species (Karelinia caspia, Alhagi sparsifolia, and Populus euphratica) in the southern edge of the Taklimakan Desert, Northwest China.
Results: Our results revealed that under UV radiation conditions, K. caspia, A. sparsifolia, and P. euphratica experienced mass losses of 45.4%, 39.8%, and 34.9%, respectively, and 20%, 22.2% and 17.4%, respectively under UV filtering treatment. Specifically, the loss rate of carbon and lignin under UV radiation, was 2.5 and 2.2 times higher than under UV filtering treatment, respectively.
Conclusion: UV radiation did not dominate decomposition throughout the year in our study area, and the loss rate of litter traits was significantly higher in summer than in winter under UV radiation. Moreover, this photodegradation is related to the intensity of UV exposure, but not to precipitation or temperature. Surprisingly, species type had no significant effect on litter decomposition. However, when we applied a UV filtering treatment, we observed higher loss rates of nitrogen compared with the ambient treatment, suggesting the involvement of other spectra in the litter decomposition process. Overall, our findings elucidate that UV radiation is a crucial factor that affects litter mass loss. The magnitude of this effect mostly varies with the season rather than the species of litter.
1 Introduction
Decomposition is a critical process in the cycling of carbon and nutrients within ecosystems, involving both biological and abiotic factors (Berg and Laskowski, 2006; Brandt et al., 2009). Previous research highlighted that photodegradation, as a significant abiotic factor, is a key process that controls carbon loss and contributes to litter decomposition in arid areas (Austin and Vivanco, 2006; Gallo et al., 2009; Austin and Ballaré, 2010; Dirks et al., 2010; Smith et al., 2010; Lee et al., 2012; Almagro et al., 2015; Day et al., 2015). Further investigations demonstrated that ultraviolet (UV) radiation can accelerate the decomposition process and alter nutrient dynamics in litter (Henry et al., 2008; Bornman et al., 2015; Predick et al., 2018). Studies have shown that the effect of UV on litter decomposition is limited by rainfall and litter chemistry (Brandt et al., 2007). Moreover, enhanced UV radiation could promote microbial degradation of litter (Duguay and Kilronomos, 2000). Some litter decomposition models have also shown that shortwave solar radiation, particularly UV radiation, can increase the nitrogen pool in surface litter but decrease the carbon content in surface litter and mineral soil to varying degrees (Araujo and Austin, 2020; Berenstecher et al., 2020). These effects further influence soil carbon cycling and storage in drylands under climate change (Parton et al., 2007; Brandt et al., 2010; Smith et al., 2010; Chen et al., 2016; Almagro et al., 2017). However, conflicting findings have been reported, with some studies indicating no net effect of UV radiation on the decomposition rate of surface litter, possibly due to positive photochemical processes counteracting the negative effects on microorganisms (Song et al., 2011; Erdenebileg et al., 2018). Furthermore, certain laboratory control experiments have failed to observe photooxidation or microbial promotion in litter under continuous UV irradiation (Kirschbaum et al., 2011; Lambie et al., 2014). These inconsistencies highlight the gaps surrounding the UV degradation effect of litter in arid areas, emphasizing the need for further research to elucidate litter dynamics in arid and semi-arid ecosystems.
Plant traits have been found to have a significant influence on litter decomposition (Pugnaire and Valladares, 2007). For example, specific leaf area (Day et al., 2018; Rawat et al., 2020) and the nitrogen or lignin content of litter (Austin and Ballaré, 2010) are associated with the UV degradation rate. In dry areas, plant leaves with unique structural features, such as high water conservation capacity and few palisade cells, tend to result in more fragile and easily decomposable plant litter (Cepeda and Whitford, 1987; Cornwell et al., 2008; Vanderbilt et al., 2008). Lignin, being highly sensitive to UV radiation, has been identified as the primary contributor to mass loss in photodegradation (Gallo et al., 2009; Austin and Ballaré, 2010), suggesting that the degradation of lignin may play a crucial role in mass loss during litter decomposition (Brandt et al., 2007; Uselman et al., 2011). In addition, studies have indicated that the initial chemical composition of litter, such as the initial nitrogen concentration, plays a crucial role in net nitrogen fixation and release (Parton et al., 2007), and litter with higher initial water-soluble content tends to exhibit a faster UV degradation rate (Day et al., 2018). However, although plant traits are the main factor that affects UV degradation rate in arid areas (Throop and Archer, 2009), this effect is based on the constant exposure of UV rays (Ma et al., 2017). Variation of the environment (such as sunny to cloudy, seasonal changes) may greatly lead to changes in the duration of UV radiation (Berenstecher et al., 2020), which could also be the cause of the inconsistent conclusions of some litter decomposition studies.
Seasonal variations have been reported to affect litter decomposition rates (Berenstecher et al., 2020; Jiang et al., 2022; Dirks et al., 2010; Ma et al., 2017; Gao et al., 2023). Some studies suggest that light intensity seasonally affects litter decomposition due to stronger solar radiation in summer than in winter (Ma et al., 2017). Strong sunlight in summer accelerates litter decomposition through photodegradation (Austin and Vivanco, 2006; Austin and Ballaré, 2010), and radiation inhibits microbial activity by damaging DNA and inhibiting spore germination (Rohwer and Azam, 2000; Hughes et al., 2003; Smith et al., 2010). However, some studies showed that precipitation and precipitation frequency seasonally affect the UV degradation rate of litter, with the strong effect of photodegradation appearing only during the period of low frequency and amount of precipitation (Huang and Li, 2017b). Wet season precipitation promotes photodegradation by directly leaching litter and indirectly changing microbial communities (Lee et al., 2012). Temperature has also been reported to affect the UV degradation rate of litter at different periods, and this influence usually comes from the combination of photodegradation and thermal degradation (Asperen et al., 2015; Predick et al., 2018). The above studies imply that the climatic factors that dominate UV degradation of litter in arid areas in different seasons are still unclear.
More than 40% of the Earth’s land is currently classified as arid or semi-arid, and this area is projected to expand in the coming decades (Reynolds et al., 2007; Yao et al., 2020). This condition suggests that litter decomposition studies in this area can provide some data support for understanding global carbon turnover (Austin et al., 2011). The Taklimakan Desert, the world’s second-largest shifting desert, is an example of a hyper-arid region. With an average annual precipitation of only 35.1 mm and a distant location from the sea, it experiences a dry climate and has sparse vegetation. With the substantial litter production in this region taken into consideration, coupled with intense UV radiation during summer and limited precipitation, the contribution of litter photodegradation and their seasonal dynamics may be significantly different from that in humid areas (Yang et al., 2020). Therefore, we conducted a field experiment to investigate the effects of photodegradation on litter decomposition under three light conditions in hyper-arid areas. We selected litter leaves from three dominant plant species (Karelinia caspia and Alhagi sparsifolia belong to shrubs, and Populus euphratica belongs to trees) in the Taklimakan Desert, which have a higher exposure rate and larger leaf area compared with other species. The three plants also have great differences in species traits. We proposed two hypotheses for this study: 1) As a result of differences in initial traits, differences in mass loss from UV radiation exist among species in hyper-arid areas; 2) UV is no longer dominant in litter decomposition in winter due to decreased radiation intensity in hyper-arid areas. This study aims to enhance our understanding of the effects of photodegradation on litter decomposition in hyper-arid desert environments.
2 Materials and methods
2.1 Study site
This study was conducted in the experimental area of Cele National Station of Observation and Research for Desert Grassland, Xinjiang Institute of Ecology and Geography, Chinese Academy of Sciences. The experimental site is located on the southern edges of Taklimakan Desert (35°17′55″–39°30′00″N, 80°03′24″–82°10′34″E), which has a typically arid continental climate (Figure 1). The mean annual temperature is 11.9°C, ranging from 41.9°C in summer to −23.9°C in winter. The mean annual precipitation here is only 35.1 mm, and the mean potential annual evaporation is 2,600 mm (annual precipitation: annual evaporation = 0.0135) (Liu et al., 2016). Soils here are classified as aridisols (United States Department of Agriculture USDA, 1999) in the USDA ST system, with poor water holding capacity, severe desertification, and low organic matter content. The area has abundant thermal resources and more sunny days. The annual average sunshine hours are 2,854.2 h (Zhang et al., 2017). The main plant species include desert shrubs and grasses, such as K. caspia, A. sparsifolia, P. euphratica, Tamarix ramosissima, and Calligonum mongolicum, with low vegetation coverage.
2.2 Litter collection and experimental design
Three dominant plant litter leaves of K. caspia, A. sparsifolia, and P. euphratica were collected from September to November 2017. Litter collection baskets were set under vegetation, and the baskets were 50 cm × 50 cm for K. caspia and A. sparsifolia, and 100 cm × 100 cm for P. euphratica. All samples were mixed evenly. The collected samples were dried for 48 h in a 75°C oven after the impurities were removed. Then, 20 × 20 cm nylon mesh bags with a mesh size of 1 mm were used as litter bags, and each bag was precisely filled with 20.0 g litter leaves. Six litter bags were used as a sampling unit for one species, and the size of the unit was 2 × 2 m. The whole experiment included 216 litter bags in total (3 litter species × 3 filter treatments × 6 collection times × 4 replicates).
Bags of three litter species within each treatment were respectively pinned by nail to the sand soil surface in three blocks under the following treatments:
(1) Ambient, where the bags were covered by a fully transparent film (0.05 mm plastic, China) supported by PVC pipes at an inclination angle to prevent precipitation accumulation, a 95% penetration rate of visible light (>280 nm), with data obtained by using a UV photometer (Lambda25, PerkinElmer, USA) and an LI-250A illuminometer (LI-250A, LI-COR, Lincoln, USA). PVC pipes were set 1 m on each side, and 40 cm above the soil surface to avoid potential greenhouse effects (Uselman et al., 2011) and ensure that other climate factors remain consistent for all treatments.
(2) UV radiation filtering, where the bags were covered by a UV-shielding film (0.2 mm Rosco E-Color 226, Finland), 70% of all UV rays (280–400 nm) were blocked, and 10% shading rate of visible light, with data obtained by using a UV photometer (Lambda25, PerkinElmer, USA) and an LI-250A illuminometer (LI-COR, Lincoln, USA), set in the same way with the ambient treatment.
(3) Dark treatments where the samples were covered with a total dark film (0.5 mm polyethylene, top white and bottom black 12 wire, China), 91% shading rate of visible light (>280 nm), with data being obtained by using an LI-250A illuminometer (LI-COR, Lincoln, USA), also set in the same way with the ambient treatment.
The optical properties of the fully transparent film, UV-shielding film, and total dark film in the study are shown in the figure (Supplementary Figure S1). All films were replaced once a month to prevent aging.
The sample plot was checked every 2 weeks. Any sand on the litter bags was removed to ensure normal experimental conditions.
Litter bags were placed in the test plot in January 2018. Four replicates of bags of each plant type/light treatment were collected from April to December 2018. The litter bags were taken back to the laboratory to dry, and the residual sand was removed with a 1 mm mesh sieve. To ensure the rationality of the experimental design, we monitored the seasonal variation of UV irradiance in the study area during the experimental year, which was higher (0.91 MJ m−2 day−1) in summer and lower (0.30 MJ m−2 day−1) in winter (Figure 2A). We also evaluated the precipitation and temperature in the study area from 2016 to 2018. The annual precipitation was 45.4, 92.4 and 53.6 mm, and the average annual temperature was 12.8°C, 12.0°C, and 11.7°C, respectively (Figure 2B).
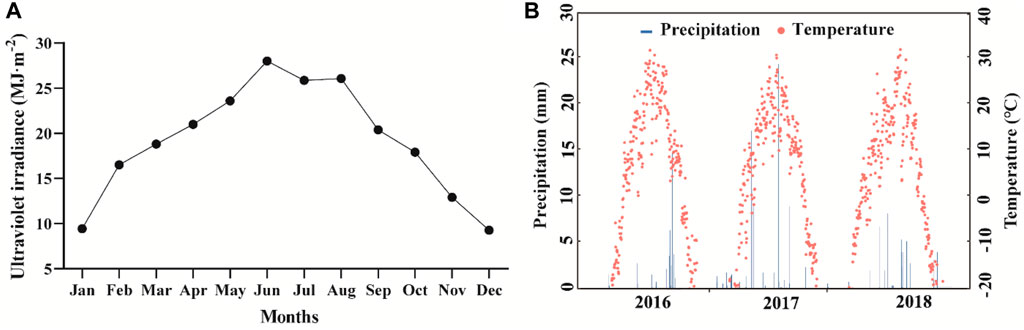
FIGURE 2. Annual variations of UV irradiance in the study site (A) and daily total precipitation and daily mean temperature in the study area from 2016 to 2018 (B).
2.3 Experimental measurement
Climatic data such as temperature, precipitation, and UV irradiance were obtained from a meteorological station located less than 50 m away from the test sample plot. Specific leaf area (surface area: mass [oven dried]) was determined by measuring the projected surface area for each litter type using a leaf area meter (S-102P, Scitek, USA). After fast and fine grinding with an MM400 grinder (RETSCH, Germany), the litter was passed through a 0.2 mm mesh. About 0.2 g of samples were weighed and wrapped in tin foil, and the total carbon content of litter was determined by an elemental analyzer (vario MACRO Cube, Germany) (50 mg phenylalanine calibration). About 0.5 g of the sample was weighed, and 10 mL concentrated sulfuric acids and a piece of catalyst were added to perform the digestion process of the sample. The Kjeldahl apparatus (FOSS, Denmark) was used to determine the nitrogen content of the litter (Ammonium sulfate with purity >99.5% was used to detect the recovery). About 0.5 g of samples were weighed to determine lignin by using a cellulose analyzer (USA Fibertec). The total amount of ash was determined by muffle furnace ashing. Carbonized litter was placed in a 550°C muffle furnace for ashing, which was used to the determine the water-soluble salt content.
2.4 Statistical analysis
The decomposition process of litter is fitted by the Olson exponential decay model (Olson, 1963), and the decomposition constant k is calculated as
where Mao, Mat represents the initial and after decomposition time t litter’s dry weight, respectively. t1 represents a decomposition time of 1 year.
SPSS 20.0 (IBM, USA) for Windows was used to analyze the test data. One-way ANOVA with Tukey correction was used to analyze the difference of initial litter traits on different species; the difference of mass loss rate and decomposition constant k under different illumination conditions and among various species; the difference of carbon loss, nitrogen loss, lignin loss, ash loss, and water-soluble salt loss under different illumination conditions and among various species; and the difference of mass loss, lignin loss, and carbon loss between summer and winter and under different illumination conditions. Two-way ANOVA was used to analyze the influence and interaction between treatments and species on mass loss rate, k, carbon loss, nitrogen loss, lignin loss, ash loss, and water-soluble salt loss. Before the analysis, the Shapiro–Wilk method was used to judge the normality and homogeneity of the variance of the data. The data of water-soluble salt loss rate were converted by log (10) to meet the requirements of residual normality. The test level was set as p = 0.05. Prism (GraphPad, USA) software was used for drawing plots of mass loss, decomposition constant k, carbon loss, lignin loss, and nitrogen loss under different treatments for different species, and plots of mass loss, lignin loss, and carbon loss under different illumination conditions between summer and winter for different species. Relationships among litter loss rate, temperature, precipitation, radiant energy, and geothermal energy under ambient treatment were assessed by Pearson correlations and their corresponding heatmap. A structural equation model (SEM) was established by the piecewiseSEM software package of R software (Lefcheck, 2016; Zhang et al., 2021). SEM was used to tease and quantitatively analyze the chemical components that directly and indirectly affect the mass loss of litters. D-separation test was used to check whether the model omitted important links, and p > 0.05 indicated that the model was approved (Shipley, 2002).
3 Results
3.1 Initial litter traits
Significant differences were found in the initial traits of the three kinds of litter (Table 1). Concentrations of carbon and nitrogen and specific leaf area of A. sparsifolia were significantly higher than those of P. euphratica and K. caspia. The C:N ratios were highest in P. euphratica and lowest in A. sparsifolia. The lignin content of P. euphratica and A. sparsifolia was significantly higher than that of K. caspia. Concentrations of ash and water-soluble salt showed a consistent pattern, with K. caspia, A. sparsifolia, and P. euphratica representing the concentrations in descending order.
3.2 Litter mass loss and decomposition rates
Results showed that the trend of the mass loss rate curve of the three kinds of litter was almost the same (Figure 3). After 1 year of decomposition, the mass loss rates under total solar radiation of K. caspia, A. sparsifolia, and P. euphratica were 45.4%, 39.8%, and 34.9%, respectively, and 20%, 22.2%, and 17.4%, respectively, under UV filtering treatment. Thus, UV radiation increased the mass loss of K. caspia, A. sparsifolia, and P. euphratica by 25.4%, 17.6%, and 17.5%, respectively. The mass loss rate under sunlight condition was significantly higher than that under UV filtering and dark treatments (Table 2, p < 0.05). The decomposition constant k of the three litter species under sunlight condition was significantly higher than that under UV filtering and dark treatment (Figure 4; Table 2, p < 0.05). Litter that received full sunlight had the fastest decomposition rate. However, no significant difference was found between UV filtering and dark treatments (Figure 4; Table 2, p > 0.05), UV radiation played a dominant role in photodegradation.
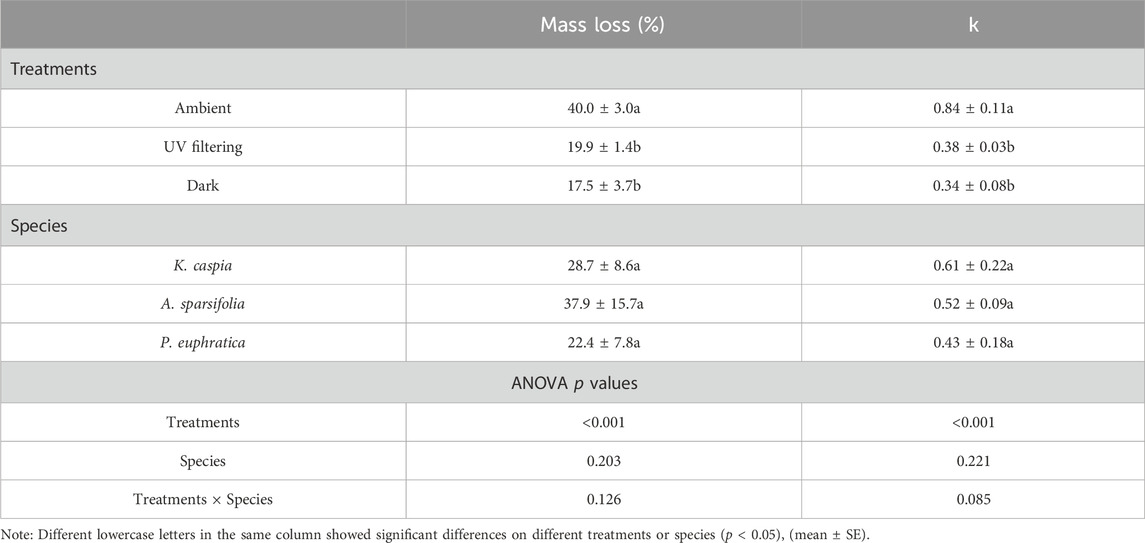
TABLE 2. Analysis of the difference of mass loss rate and k in litter under different illumination conditions and species after 1 year.
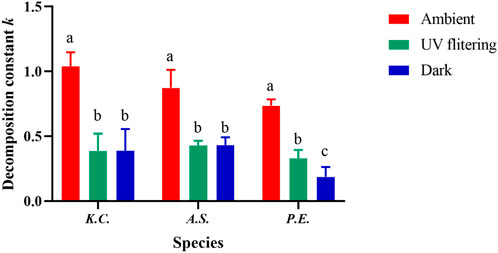
FIGURE 4. Mass decomposition constant k of litter under different illumination conditions (mean ± SE). Different lowercase letters indicate significant differences among treatments (p < 0.05).
In addition, no significant difference was found among three species under the same illumination condition (Table 2, p > 0.05). Similarly, the difference of decomposition constant k among three species did not reach a significant level, and no interaction occurred between treatments and species on mass loss rate and k value (Table 2, p > 0.05).
3.3 Changes in litter traits under different illumination treatments
Illumination treatments significantly affected the litter loss rate of carbon and lignin (Table 3, p < 0.001). The loss rates of all litter traits were highest under full sunlight. Specifically, the loss rate of carbon under full sunlight was 2.5 and 2.4 times higher than under UV filtering and dark treatments, respectively. The loss rate of lignin under full sunlight was 2.2 and 2.1 times higher than under UV filtering and dark treatments, respectively. No significant difference was found in the loss rate of carbon and lignin between UV filtering and dark treatments (Table 3, p > 0.05). The effects of solar radiation on the loss rate of ash and salt were not significant (p > 0.05), but the nitrogen content under UV filtering was significantly higher than that under ambient treatment (Table 3, p < 0.05). Similarly, the carbon and lignin of three litter showed similar results compared with the mass loss process, while the nitrogen loss was very different from the mass loss process (Figures 3, 5).
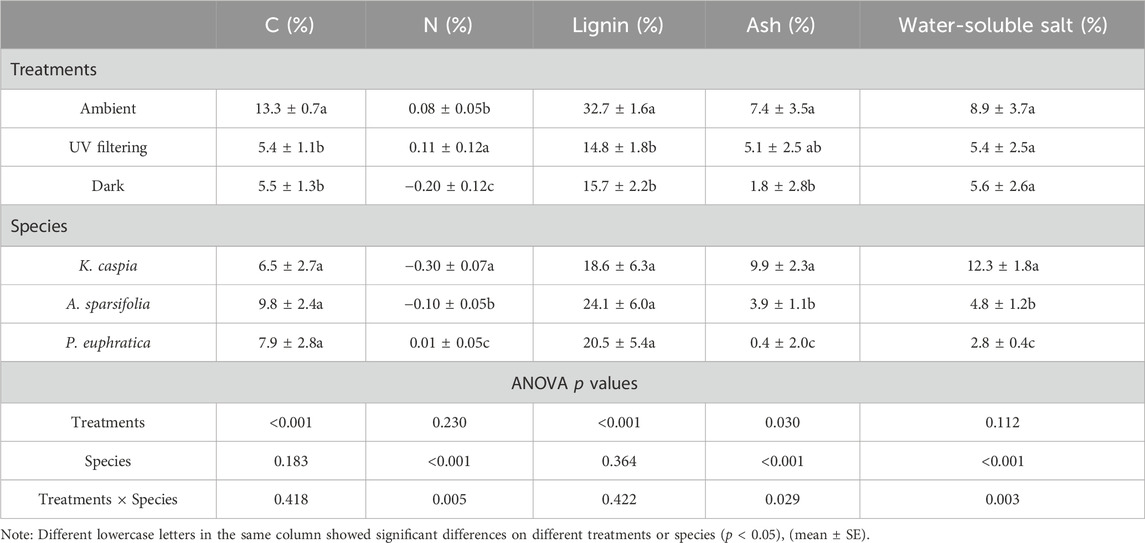
TABLE 3. Analysis of the difference of C (carbon), N (nitrogen), lignin, ash and water-soluble salt fraction loss rate under different illumination conditions and species after 1 year.
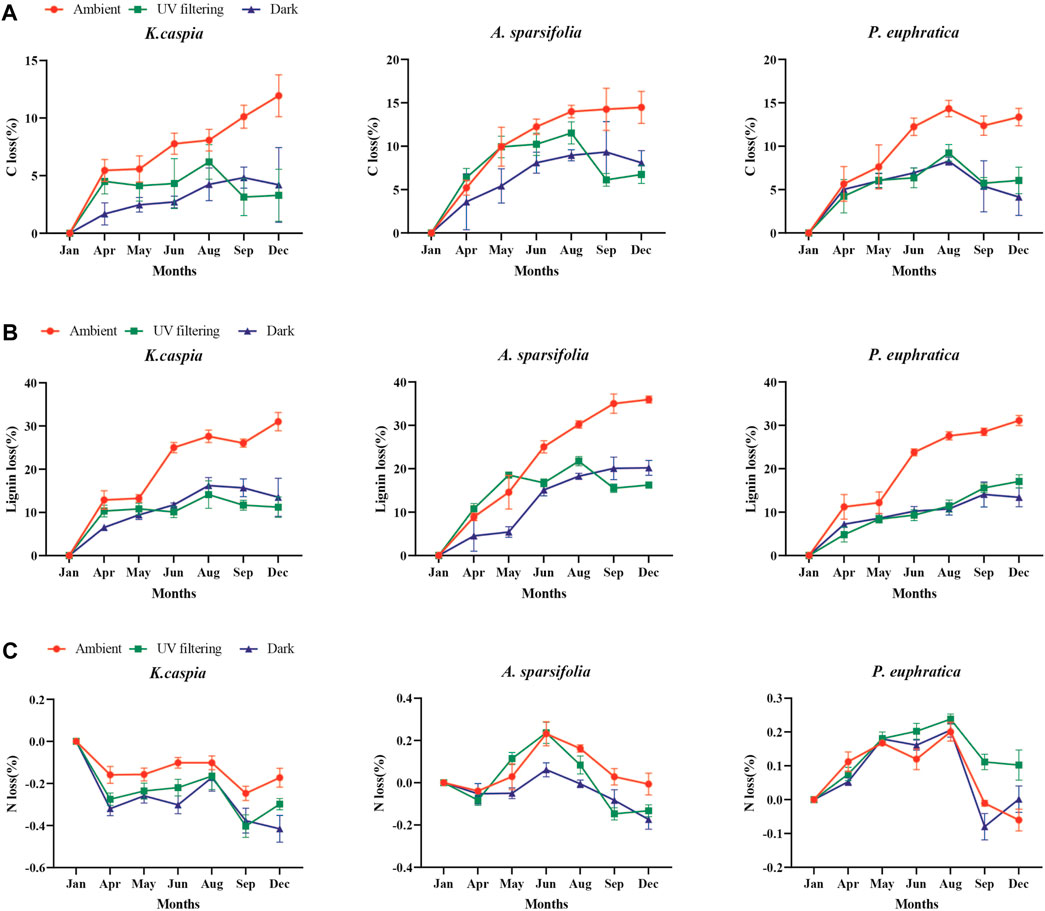
FIGURE 5. C (A), lignin (B), and N (C) loss rate of litter under different illumination conditions (mean ± SE).
Species significantly affected the litter loss rate of nitrogen, ash, and water-soluble salt (Table 3, p < 0.001). The loss rate of ash and water-soluble salt in K. caspia was about 2.5 times than in A. sparsifolia, and more than 4 times than in P. euphratica under the same illumination condition. However they varied significantly between species, which seemed to be related to differences in initial litter traits (Tables 1, 3). In addition, species–treatment interaction was significant only for the loss of nitrogen, ash, and water-soluble salt (Table 3, p < 0.05).
3.4 Seasonal variation on decomposition under different illumination treatments
Under ambient treatment, litter mass loss of all species showed a more significant difference in summer than in winter (p < 0.05). The mass loss of K. caspia, A. sparsifolia and P. euphratica increased (mass losssummer – mass losswinter) by 4.3%, 9.6% and 6.8%, respectively (Figure 6). In addition, the lignin and carbon loss of K. caspia and A. sparsifolia were significantly different between summer and winter (Figures 6A, B, p < 0.05). However, P. euphratica did not show seasonal differences in this regard (Figure 6C, p > 0.05). Most species loss rates did not show seasonal differences under UV filtering treatment (p > 0.05), except for mass loss of A. sparsifolia and carbon loss of P. euphratica (Figures 6B, C, p < 0.05). No seasonal differences in litter loss rates were observed for all species under dark treatment (Figure 6, p > 0.05).
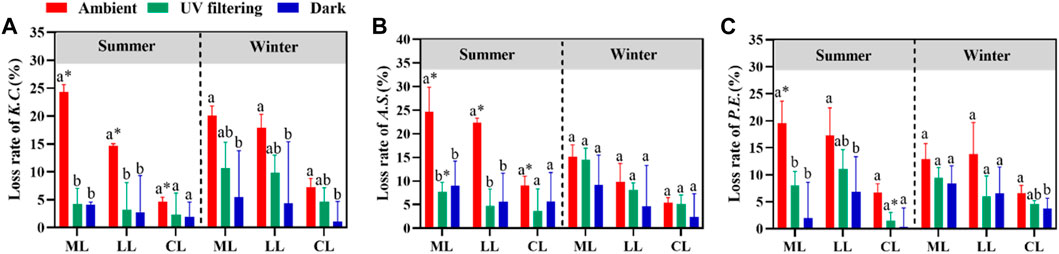
FIGURE 6. Seasonal variation of litter mass, lignin, and carbon loss under different illumination conditions. Different capital letters indicate significant differences among treatments. The asterisk indicates a significant difference between summer and winter (p < 0.05). K.C. in (A) stands for: K. caspia, A.S. in (B): A. sparsifolia, P.E. in (C): P. euphratica, ML, mass loss; LL, lignin loss, CL, carbon loss.
In summer, all species showed significant differences in mass and lignin loss between ambient and shading treatments (p < 0.05). The mass loss of K. caspia, A. sparsifolia and P. euphratica under UV radiation increased (mass lossambient – mass lossUV filtering) by 20.2%, 17.0% and 11.6%, respectively (Figure 6). In contrast, in winter, all species showed little difference between ambient and UV filtering treatments (Figure 6, p > 0.05). The trait loss (including mass loss, lignin loss and carbon loss) of litter was closely and positively correlated with total solar radiation, total UV radiation and optical quantum flux in summer (Figure 7A, mass loss: p < 0.001, lignin loss: p < 0.01, carbon loss: p < 0.05). However, in winter, no significant correlation existed between litter trait loss and climate factors such as temperature, precipitation, and radiation (Figure 7B, p > 0.05).
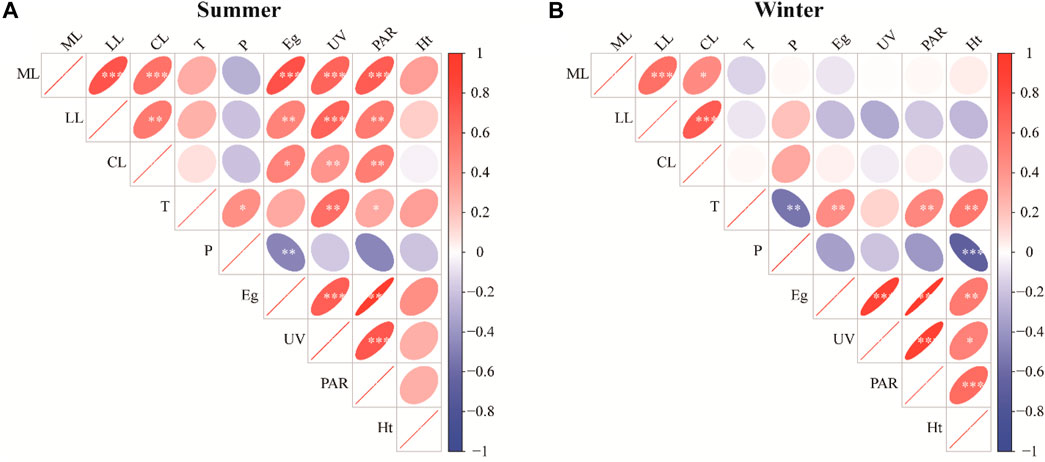
FIGURE 7. Correlations among litter loss rate, temperature, precipitation, radiant energy, and geothermal energy under ambient treatment in summer (A) and winter (B). A red ellipse indicates a positive correlation, and a blue ellipse indicates a negative correlation. The shape and color depth of the ellipse represent the absolute value of the correlation. The flatter the ellipse and the darker the color, the greater the absolute value of the correlation. Asterisks denote significance levels (*p < 0.05, **p < 0.01, ***p < 0.001). ML, mass loss; LL, lignin loss; CL, carbon loss; T, air temperature (℃); P, precipitation (mm); Eg, total solar radiation (W m−1); UV, total UV radiation (W m−2); PAR, optical quantum flux (μmol m−2 s−1); Ht, soil heat flux (W m−2).
3.5 Factors affecting variations in litter mass loss under solar radiation
The key factors that affect the variations in litter mass loss under full sunlight treatment were assessed using SEM (Figure 8A). Under full sunlight treatment, water-soluble salt had direct effects (0.88 and 0.45) on ash and lignin, and indirect effects (0.65) on mass loss. Ash had direct effects (0.31) and indirect effects (−0.18) on mass loss. Lignin had direct effects (0.84) on mass loss. Carbon had direct effects (0.85) on lignin and indirect effects (0.71) on mass loss. Nitrogen had no effect on mass loss. Therefore, the effects of lignin on variations of litter mass loss were the highest in all traits. The standardized effect sizes of the driving factors further clarify the SEM results (Figure 8B).
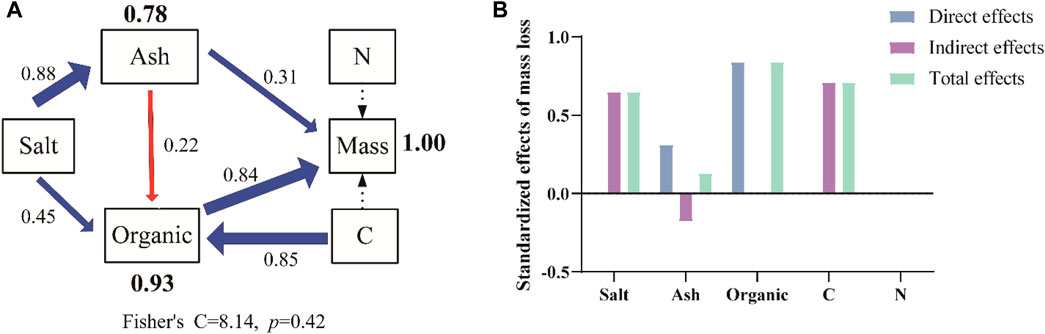
FIGURE 8. Controlling factor analysis of variations in litter mass loss under full sunlight conditions using SEM. Significant regressions and nonsignificant regressions are shown by solid (p < 0.05) and dashed lines, respectively (A). Blue and red arrows denote positive and negative relationships, respectively. The thickness of significant paths was scaled based on the magnitude of the standardized regression coefficient. Standardized effects of driving factors on litter mass loss under full sunlight (B). Salt, water-soluble salt loss; Organic, lignin loss; Ash, ash loss; C, carbon loss; N, nitrogen loss; Mass, mass loss.
4 Discussion
4.1 Litter mass loss and decomposition rate during photodegradation in hyper-arid deserts
Over a period of 1 year, we observed a higher loss of litter quality, ranging from 17.5% to 25.4%, in the ambient treatment compared with the treatment without UV exposure (Figure 3; Table 2). Notably, a significant difference in the impact of UV radiation on litter mass loss was observed starting from June (Figure 3, p < 0.05). When the decomposition time approached 1 year, the mean mass loss rate of litter under UV radiation was 45%, which was similar to other extremely arid deserts (Huang et al., 2017a; Becky et al., 2019). These findings align with the conclusion that photodegradation is the primary controlling factor for above-ground litter decomposition in arid ecosystems (Austin and Vivanco, 2006). The average decomposition rates of litter were 0.88, 0.38, and 0.34 yr−1 under full sunlight, UV filtering, and dark treatment, respectively (Figure 4; Table 2). These rates are higher than those observed for leaf litter in previous studies, which ranged from 0.28 to 0.78 yr−1 (Vanderbilt et al., 2008; Throop and Archer, 2009; Uselman et al., 2011). The difference in decomposition rates might be attributed to the intensity of UV radiation exposure to litter (Berenstecher et al., 2020). In our study area, the daily average UV radiation intensity during summer (630 KJ m−2 day−1) was much higher than that reported in other studies (Vanderbilt et al., 2008; Throop and Archer, 2009; Uselman et al., 2011), which were below 100 KJ m−2 day−1 (Figure 2A).
Surprisingly, in contrast to our first hypothesis that we did not observe any significant differences in final mass loss and decomposition rate (k) among different litter species types, regardless of the treatment (Table 2, p > 0.05). This finding is consistent with a litter decomposition experiment conducted in a semi-arid Mediterranean oak forest, where individual species did not explain the rate of litter decomposition (Sarabi et al., 2021). This result may be attributed to the fact that most of our research objects are shrubby vegetation. Furthermore, the effect of treatment–species interaction did not yield significant differences in final mass loss and decomposition rate (k) in our study area. Overall, our study demonstrated that species type had no significant effect, highlighting the importance of photodegradation in litter decomposition.
4.2 Changes in litter traits under different illumination treatments
Our study demonstrated that different illumination treatments had a significant impact on specific litter trait losses in hyper-arid deserts (Table 3, p < 0.05). Specifically, UV radiation accelerated the loss of carbon and lignin in litter, which is consistent with the observed differences in litter mass loss under varying degrees of photodegradation (Tables 2, 3; Figures 5A, B). This finding aligns with previous studies that showed a preferential degradation of lignin, as a light-absorbing compound, by UV radiation (Meentemeyer, 1978; Day et al., 2007; Austin and Ballaré, 2010). In arid regions, photodegradation accelerates carbon release through direct and indirect mechanisms (Austin and Vivanco, 2006). In hyper-arid areas where plant litter is highly exposed to UV radiation, the photodegradation of lignin may directly contribute to carbon release. Furthermore, photodegradation has the potential to positively influence subsequent biological renewal by breaking down resilient cell wall polymers, such as lignin (Austin and Ballaré, 2010). We did not observe significant differences in the loss rate of carbon and lignin between the UV filtering and dark treatments, which is consistent with the mass loss findings (Table 3; Figures 5A, B, p > 0.05). In addition, no significant differences in carbon and lignin loss were among species nor did significant treatment–species interactions exist (Table 3, p > 0.05). Our results indicate that the litter traits significantly affected by UV radiation in our study area were not influenced by species.
In many ecosystems, traditional indices such as nitrogen have been used to predict litter decomposition rates (Meentemeyer, 1978; Melillo et al., 1982; Cornwell et al., 2008). However, in arid areas, these indices have sometimes been associated with decay in only a few litter types (Throop and Archer, 2009; Day et al., 2015). Similarly, these traditional indices failed to accurately predict litter decomposition in our study region. Interestingly, we found a significant difference in nitrogen loss between the ambient and UV filtering treatments, with the nitrogen loss after UV filtering treatment being significantly higher than that after ambient treatment (Table 3; Figure 5C, p < 0.05). Previous research established a positive correlation between nitrogen release and microbial activity and revealed that UV radiation accelerates nitrogen loss and negatively impacts microbial activity (Parton et al., 2007; Wang et al., 2015; Huang et al., 2017a). As indicated by a comparison of nitrogen loss under different illumination treatments, our results suggest that not only UV radiation but also other light spectrum (such as PAR) may inhibit microbial activity in hyper-arid deserts.
4.3 Effects of seasonal variation on decomposition in hyper-arid deserts
During summer, UV radiation led to a 20.2% increase in the mass loss rate of K. caspia, a 17.0% increase for A. sparsifolia, and an 11.6% increase for P. euphratica. In contrast, no significant difference in litter loss rate was found between treatments with and without UV radiation in winter (Figure 6, p > 0.05). This finding is consistent with our second hypothesis that UV is no longer dominant in litter decomposition in winter. These findings align with similar observations in humid areas where decomposition rates are affected by seasonal variation (Ma et al., 2017). In summer, UV radiation contributed to 9.88% of the mass loss, indicating its significant role in litter decomposition under sufficient sunlight. However, UV radiation did not significantly increase the mass loss rate in autumn and winter (Jiang et al., 2022). In addition, our study revealed that the mass loss during summer was significantly higher than that during winter, with K. caspia, A. sparsifolia, and P. euphratica experiencing increases of 4.3%, 9.6%, and 6.8%, respectively, due to seasonal variation (Figure 6). We observed that the seasonal litter photodegradation rates were significant correlated with UV radiation intensity but not with temperature or precipitation (Figure 7, p < 0.05). Notably, some studies reported higher mass loss of litter in winter than in summer possibly due to abundant rainfall (annual average of 1,100 mm) and the active role of decomposers in carbon turnover in those ecosystems (Berenstecher et al., 2020). In contrast, our study area had an average annual rainfall of only 53.6 mm (Figure 2B), which significantly limited microbial activity. Furthermore, the intensity of UV radiation in summer was higher than in winter (Figure 2A), providing a potential explanation for the higher mass loss observed during summer than in winter.
With regard to lignin loss, we found a significant difference between treatments with and without UV radiation in summer (p < 0.05), while no significant difference was observed in winter (Figure 6, p > 0.05). This finding suggests that lignin was more susceptible to UV radiation degradation in summer. These findings align with the research of Berenstecher et al. in the Patagonia woodlands, where UV radiation can break down lignin into CO2 and small compounds through photodegradation, which is the main cause of carbon loss in this ecosystem during the summer (Berenstecher et al., 2020). Furthermore, experimental results from Austin et al. demonstrated that lignin, as an effective light-absorbing compound, is greatly influenced by light intensity and spectral range (Austin and Ballaré, 2010). In our study area, which is characterized by a hyper-arid desert environment, lignin is particularly susceptible to photodegradation due to the stronger UV radiation in summer (Figure 2A). These findings imply that the mechanism by which radiation influences litter decomposition may vary with the seasons through changes in lignin decay. Therefore, further studies are needed to explore the potential mechanisms involved.
4.4 Lignin affected variations in litter mass loss during photodegradation
Previous studies consistently demonstrated that lignin loss plays a significant role in mass loss during litter decomposition, particularly under the influence of photodegradation (Meentemeyer, 1978; Austin and Ballaré, 2010; Lee et al., 2012; Erdenebileg et al., 2018). These findings are in line with our own results, which indicate that photodegradation, particularly UV degradation, is the primary driver of litter decomposition in hyper-arid areas. We also observed that lignin loss had a significant impact on the final mass loss of litter (Table 3; Figure 8A). In addition, our study revealed that carbon indirectly influenced mass loss by directly affecting lignin content. Furthermore, the loss of water-soluble salts influenced the ash and lignin content, subsequently indirectly affecting the final mass loss. In contrast, nitrogen did not have a direct effect on litter mass loss. The results were similar to the findings in some arid and semi-arid areas, which is that the primary reason for greater mass loss of litter under UV radiation is photodegradation, especially the degradation of lignin (Day et al., 2007; Mendez et al., 2019). Our results provide more specific insights by highlighting the direct influence of lignin (0.84) on litter mass loss in hyper-arid deserts. These detailed findings contribute to a better understanding of decomposition processes and carbon turnover patterns in the region.
5 Conclusion
UV radiation plays an important role in litter decomposition in our study area. Significant differences in litter loss rates were found between ambient and UV filtering treatments. Under UV radiation conditions, K. caspia, A. sparsifolia, and P. euphratica experienced mass losses of 45.4%, 39.8%, and 34.9%, respectively, and 20%, 22.2%, and 17.4%, respectively, under UV filtering treatment. Specifically, the loss rate of carbon and lignin under UV radiation was 2.5 and 2.2 times higher than under UV filtering treatment, respectively. Furthermore, UV radiation did not dominate decomposition throughout the year in our study area, and the loss rate of litter traits was significantly higher in summer than in winter under UV radiation. Moreover, this photodegradation is related to the intensity of UV exposure, but not to precipitation or temperature. Surprisingly, species type had no significant effect on litter decomposition. Notably, our findings indicate that not only UV radiation but also the other light spectrum may inhibit microbial activity in hyper-arid deserts. As a next step in this study, we aim to refine the spectral bands to delve deeper into the process of photodegradation. In conclusion, our results provide insights into the effects of photodegradation on litter mass loss and how the magnitude of this effect mostly varies with the season rather than the species of litter, which are crucial for predicting the response of the carbon cycle to varying radiation conditions in hyper-arid desert ecosystems.
Data availability statement
The original contributions presented in the study are included in the article/Supplementary Material, further inquiries can be directed to the corresponding author.
Author contributions
PW: Conceptualization, Data curation, Investigation, Validation, Writing–original draft, Writing–review and editing. YL: Conceptualization, Validation, Writing–review and editing. BZ: Formal Analysis, Software, Writing–review and editing. LeL: Conceptualization, Investigation, Writing–review and editing. LiL: Data curation, Formal Analysis, Writing–review and editing. XL: Project administration, Resources, Supervision, Validation, Writing–review and editing. QZ: Supervision, Writing–review and editing.
Funding
The author(s) declare that financial support was received for the research, authorship, and/or publication of this article. This research was funded by National Natural Science Foundation, grant number 42171066; West Light Foundation of The Chinese Academy of Sciences, grant number 2019-FPGGRC.
Conflict of interest
The authors declare that the research was conducted in the absence of any commercial or financial relationships that could be construed as a potential conflict of interest.
The author(s) declared that they were an editorial board member of Frontiers, at the time of submission. This had no impact on the peer review process and the final decision.
Publisher’s note
All claims expressed in this article are solely those of the authors and do not necessarily represent those of their affiliated organizations, or those of the publisher, the editors and the reviewers. Any product that may be evaluated in this article, or claim that may be made by its manufacturer, is not guaranteed or endorsed by the publisher.
Supplementary material
The Supplementary Material for this article can be found online at: https://www.frontiersin.org/articles/10.3389/fenvs.2024.1379442/full#supplementary-material
References
Almagro, M., Maestre, F. T., Martínez-López, J., Valencia, E., and Rey, A. (2015). Climate change may reduce litter decomposition while enhancing the contribution of photodegradation in dry perennial Mediterranean grasslands. Soil Biol. Biochem. 90, 214–223. doi:10.1016/j.soilbio.2015.08.006
Almagro, M., Martínez-López, J., Maestre, F. T., and Rey, A. (2017). The contribution of photodegradation to litter decomposition in semiarid Mediterranean grasslands depends on its interaction with local humidity conditions, litter quality and position. Ecosystems 20, 527–542. doi:10.1007/s10021-016-0036-5
Araujo, P. I., and Austin, A. T. (2020). Exotic pine forestation shifts carbon accumulation to litter detritus and wood along a broad precipitation gradient in Patagonia, Argentina. For. Ecol. Manag. 460, 117902. doi:10.1016/j.foreco.2020.117902
Asperen, H., Warneke, T., Sabbatini, S., Nicolini, G., Papale, D., and Notholt, J. (2015). The role of photo- and thermal degradation for CO2 and CO fluxes in an arid ecosystem. Biogeosciences 12, 4161–4174. doi:10.5194/bg-12-4161-2015
Austin, A. T. (2011). Has water limited our imagination for aridland biogeochemistry? Trends Ecol. Evol. 26, 229–235. doi:10.1016/j.tree.2011.02.003
Austin, A. T., and Ballaré, C. L. (2010). Dual role of lignin in plant litter decomposition in terrestrial ecosystems. PNAS 107, 4618–4622. doi:10.1073/pnas.0909396107
Austin, A. T., and Vivanco, L. (2006). Plant litter decomposition in a semi-arid ecosystem controlled by photodegradation. Nature 442, 555–558. doi:10.1038/nature05038
Becky, A. B., Maximilian, P. C., and Sharon, J. H. (2019). Nutrient dynamics during photodegradation of plant litter in the Sonoran Desert. J. Arid Environ. 160, 1–10. doi:10.1016/j.jaridenv.2018.09.004
Berenstecher, P., Vivanco, L., Pérez, L. I., Ballaré, C. L., and Austin, A. T. (2020). Sunlight doubles aboveground carbon loss in a seasonally dry woodland in Patagonia. Curr. Biol. 30, 3243–3251.e3. doi:10.1016/j.cub.2020.06.005
Berg, B., and Laskowski, R. (2006). Litter decomposition: a guide to carbon and nutrient turnover. Advances in Ecological Research Academic Press.
Bornman, J. F., Barnes, P. W., Robinson, S. A., Ballaré, C. L., Flint, S. D., and Caldwell, M. M. (2015). Solar ultraviolet radiation and ozone depletion-driven climate change: effects on terrestrial ecosystems. Photochem. Photobiological Sci. 14, 88–107. doi:10.1039/c4pp90034k
Brandt, L. A., Bohnet, C., and King, J. Y. (2009). Photochemically induced carbon dioxide production as a mechanism for carbon loss from plant litter in arid ecosystems. J. Geophys. Res. Biogeosci 114, G02004. doi:10.1029/2008JG000772
Brandt, L. A., King, J. Y., Hobbie, S. E., Milchunas, D. G., and Sinsabaugh, R. L. (2010). The role of photodegradation in surface litter decomposition across a grassland ecosystem precipitation gradient. Ecosystems 13, 765–781. doi:10.1007/s10021-010-9353-2
Brandt, L. A., King, J. Y., and Milchunas, D. G. (2007). Effects of ultraviolet radiation on litter decomposition depend on precipitation and litter chemistry in a shortgrass steppe ecosystem. Glob. Change Biol. 13, 2193–2205. doi:10.1111/j.1365-2486.2007.01428.x
Cepeda, J. G., and Whitford, W. G. (1987). Litter decomposition patterns in the chihuahuan desert, Nm, USA. Arch. De. Biol. Y Med. Exp. 20, 187.
Chen, M. S., Parton, W. J., Adair, E. C., Asao, S., Hartman, M. D., and Gao, W. (2016). Simulation of the effects of photodecay on long-term litter decay using DayCent. Ecosphere 7, 1–22. doi:10.1002/ecs2.1631
Cornwell, W. K., Cornelissen, J. H. C., Amatangelo, K., Dorrepaal, E., Eviner, V. T., Godoy, O., et al. (2008). Plant species traits are the predominant control on litter decomposition rates within biomes worldwide. Ecol. Lett. 11, 1065–1071. doi:10.1111/j.1461-0248.2008.01219.x
Day, T. A., Bliss, M. S., Tomes, A. R., Ruhland, C. T., and Guénon, R. (2018). Desert leaf litter decay: coupling of microbial respiration,water-soluble fractions and photodegradation. Glob. Change Biol. 11, 5454–5470. doi:10.1111/gcb.14438
Day, T. A., Guénon, R., and Ruhland, C. T. (2015). Photodegradation of plant litter in the Sonoran Desert varies by litter type and age. Soil Biol. Biochem. 89, 109–122. doi:10.1016/j.soilbio.2015.06.029
Day, T. A., Zhang, E. T., and Ruhland, C. T. (2007). Exposure to solar UV-B radiation accelerates mass and lignin loss of Larrea tridentata litter in the Sonoran Desert. Plant Ecol. 193, 185–194. doi:10.1007/s11258-006-9257-6
Dirks, I., Navon, Y., Kanas, D., Dumbur, R., and Grünzweig, J. M. (2010). Atmospheric water vapor as driver of litter decomposition in Mediterranean shrubland and grassland during rainless seasons. Glob. Change Biol. 16, 2799–2812. doi:10.1111/j.1365-2486.2010.02172.x
Duguay, K. J., and Klironomos, J. N. (2000). Direct and indirect effects of enhanced UV-B radiation on the decomposing and competitive abilities of saprobic fungi. Appl. Soil Ecol. 14 (2), 157–164. doi:10.1016/S0929-1393(00)00049-4
Erdenebileg, E., Ye, X., Wang, C., Huang, Z., Liu, G., and Cornelissen, J. H. C. (2018). Positive and negative effects of UV irradiance explain interaction of litter position and UV exposure on litter decomposition and nutrient dynamics in a semi-arid dune ecosystem. Soil Biol. Biochem. 124, 245–254. doi:10.1016/j.soilbio.2018.06.013
Gallo, M. E., Porras-Alfaro, A., Odenbach, K. J., and Sinsabaugh, R. L. (2009). Photoacceleration of plant litter decomposition in an arid environment. Soil Biol. Biochem. 41, 1433–1441. doi:10.1016/j.soilbio.2009.03.025
Gao, T., Song, X. Y., Ren, Y. Z., Liu, H., Meng, Y., and Dong, X. B. (2023). Effects of seasonal changes on the carbon dynamics in mixed coniferous forests. PLOS ONE 18, e0267365. doi:10.1371/journal.pone.0267365
Henry, H. A. L., Brizgys, K., and Field, C. B. (2008). Litter decomposition in a California annual grassland: interactions between photodegradation and litter layer thickness. Ecosystems 11, 545–554. doi:10.1007/s10021-008-9141-4
Huang, G., and Li, Y. (2017b). Photodegradation effects are related to precipitation amount, precipitation frequency and litter traits in a desert ecosystem. Soil Biol. Biochem. 115, 383–392. doi:10.1016/j.soilbio.2017.08.034
Huang, G., Zhao, H. M., and Li, Y. (2017a). Litter decomposition in hyper-arid deserts: photodegradation is still important. Sci. Total Environ. 601-602, 784–792. doi:10.1016/j.scitotenv.2017.05.213
Hughes, K. A., Lawley, B., and Newsham, K. K. (2000). Solar UV-B radiation inhibits the growth of antarctic terrestrial fungi. Applied and Environmental Microbiology 69, 1488–1488. doi:10.1128/AEM.69.3.1488-1491.2003
Jiang, H., Pan, Y., Liang, J., Yang, Y., Chen, Q., Lv, M., et al. (2022). UV radiation doubles microbial degradation of standing litter in a subtropical forest. J. Ecol. 110, 2156–2166. doi:10.1111/1365-2745.13939
Kirschbaum, M. U. F., Lambie, S. M., and Zhou, H. (2011). No UV enhancement of litter decomposition observed on dry samples under controlled laboratory conditions. Soil Biol. Biochem. 43, 1300–1307. doi:10.1016/j.soilbio.2011.03.001
Lambie, S. M., Kirschbaum, M. U. F., and Dando, J. (2014). No photodegradation of litter and humus exposed to UV-B radiation under laboratory conditions: No effect of leaf senescence or drying temperature. Soil Biol. Biochem. 69, 46–53. doi:10.1016/j.soilbio.2013.10.037
Lee, H., Rahn, T., and Throop, H. (2012). An accounting of C-based trace gas release during abiotic plant litter degradation. Glob. Change Biol. 18, 1185–1195. doi:10.1111/j.1365-2486.2011.02579.x
Lefcheck, J. S. (2016). piecewiseSEM: piecewise structural equation modelling in r for ecology, evolution, and systematics. Methods Ecol. Evol. 7, 573–579. doi:10.1111/2041-210X.12512
Liu, B., He, J., Zeng, F., Lei, J., and Arndt, S. K. (2016). Life span and structure of ephemeral root modules of different functional groups from a desert system. New Phytol. 211, 103–112. doi:10.1111/nph.13880
Ma, Z. L., Yang, W. Q., Wu, F. Z., and Tan, B. (2017). Effects of light intensity on litter decomposition in a subtropical region. Ecosphere 8, 1–13. doi:10.1002/ecs2.1770
Meentemeyer, V. (1978). Macroclimate and lignin control of litter decomposition rates. Ecology 59, 465–472. doi:10.2307/1936576
Melillo, J. M., Aber, J. D., and Muratore, J. F. (1982). Nitrogen and lignin control of hardwood leaf litter decomposition dynamics. Ecology 63, 621–626. doi:10.2307/1936780
Mendez, M. S., Martinez, M. L., Araujo, P. I., and Austin, A. T. (2019). Solar radiation exposure accelerates decomposition and biotic activity in surface litter but not soil in a semiarid woodland ecosystem in Patagonia, Argentina. Plant Soil 445, 483–496. doi:10.1007/s11104-019-04325-1
Olson, J. S. (1963). Energy storage and the balance of producers and decomposers in ecological systems. Ecology 44, 322–331. doi:10.2307/1932179
Parton, W., Silver, W. L., Burke, I. C., Grassens, L., Harmon, M. E., Currie, W. S., et al. (2007). Global-Scale similarities in nitrogen release patterns during long-term decomposition. Science 315, 361–364. doi:10.1126/science.1134853
Predick, K. I., Archer, S. R., Aguillon, S. M., Keller, D. A., Throop, H. L., and Barnes, P. W. (2018). UV-B radiation and shrub canopy effects on surface litter decomposition in a shrub-invaded dry grassland. J. Arid Environ. 157, 13–21. doi:10.1016/j.jaridenv.2018.06.007
Rawat, M., Arunachalam, K., Arunachalam, A., Alatalo, J. M., and Pandey, R. (2020). Predicting litter decomposition rate for temperate forest tree species by the relative contribution of green leaf and litter traits in the Indian Himalayas region. Ecol. Indic. 119, 106827. doi:10.1016/j.ecolind.2020.106827
Reynolds, J. F., Smith, D. M. S., Lambin, E. F., Turner, B. L., Mortimore, M., Batterbury, S. P. J., et al. (2007). Global desertification: building a science for dryland development. Science 316, 847–851. doi:10.1126/science.1131634
Rohwer, F., and Azam, F. (2000). Detection of DNA damage in prokaryotes by terminal deoxyribonucleotide transferase-mediated dUTP nick end labeling. Applied and Environmental Microbiology 66, 1001–1006. doi:10.1128/AEM.66.3.1001-1006.2000
Sarabi, S. J., Pilehvar, B., Vajari, K. A., and Waez-Mousavi, S. M. (2021). Effects of tree species diversity on leaf litter decomposition process in semi-arid Mediterranean oak forests. Eur. J. For. Res. 140, 1377–1390. doi:10.1007/s10342-021-01403-x
Shipley, B. (2002). Cause and correlation in biology: a user’s guide to path analysis, structural equations and causal inference. Cambridge University Press Cambridge.
Smith, W. K., Gao, W., Steltzer, H., Wallenstein, M. D., and Tree, R. (2010). Moisture availability influences the effect of ultraviolet-B radiation on leaf litter decomposition. Glob. Change Biol. 16, 484–495. doi:10.1111/j.1365-2486.2009.01973.x
Song, X., Jiang, H., Zhang, H., Peng, C., and Yu, S. (2011). Elevated UV-B radiation did not affect decomposition rates of needles of two coniferous species in subtropical China. Eur. J. Soil Biol. 47, 343–348. doi:10.1016/j.ejsobi.2011.08.008
Throop, H. L., and Archer, S. R. (2009). Resolving the dryland decomposition conundrum: some new perspectives on potential drivers. Prog. Bot. 70, 171–194. doi:10.1007/978-3-540-68421-3_8
United States Department of Agriculture (USDA) (1999). A basic system of soil classification for making and interpreting soil surveys. second ed. U.S. Gov. Print. Office Washington, DC (1999) USDA NRCS Agriculture Handbook. No. 436.
Uselman, S. M., Snyder, K. A., Blank, R. R., and Jones, T. J. (2011). UVB exposure does not accelerate rates of litter decomposition in a semi-arid riparian ecosystem. Soil Biol. Biochem. 43, 1254–1265. doi:10.1016/j.soilbio.2011.02.016
Vanderbilt, K. L., White, C. S., Hopkins, O., and Craig, J. A. (2008). Aboveground decomposition in arid environments: results of a long-term study in central New Mexico. J. Arid Environ. 72, 696–709. doi:10.1016/j.jaridenv.2007.10.010
Wang, J., Liu, L. L., Wang, X., and Chen, Y. W. (2015). The interaction between abiotic photodegradation and microbial decomposition under ultraviolet radiation. Glob. Change Biol. 21, 2095–2104. doi:10.1111/gcb.12812
Yang, F., Huang, J. P., He, Q., Zheng, X. Q., Zhou, C. L., Pan, H. L., et al. (2020). Impact of differences in soil temperature on the desert carbon sink. Geoderma 379, 114636. doi:10.1016/j.geoderma.2020.114636
Yao, J. Y., Liu, H. P., Huang, J. P., Gao, Z. M., Wang, G. Y., Li, D., et al. (2020). Accelerated dryland expansion regulates future variability in dryland gross primary production. Nat. Commun. 11, 1665–1710. doi:10.1038/s41467-020-15515-2
Zhang, B., Tang, G. L., Yin, H., Zhao, S. L., Shareef, M., Liu, B., et al. (2021). Groundwater depths affect phosphorus and potassium resorption but not their utilization in a desert phreatophyte in its hyper-arid environment. Front. Plant Sci. 12, 665168. doi:10.3389/fpls.2021.665168
Keywords: ultraviolet radiation, seasonal variation, decomposition, hyper-arid desert, lignin loss
Citation: Wang P, Liu Y, Zhang B, Li L, Lin L, Li X and Zeng Q (2024) The effect of litter decomposition mostly depends on seasonal variation of ultraviolet radiation rather than species in a hyper-arid desert. Front. Environ. Sci. 12:1379442. doi: 10.3389/fenvs.2024.1379442
Received: 31 January 2024; Accepted: 27 February 2024;
Published: 08 March 2024.
Edited by:
Mukesh K. Gautam, The City University of New York, United StatesReviewed by:
Sanjay Singh, Indian Council of Forestry Research and Education (ICFRE), IndiaLili Dong, Institute of Applied Ecology (CAS), China
Copyright © 2024 Wang, Liu, Zhang, Li, Lin, Li and Zeng. This is an open-access article distributed under the terms of the Creative Commons Attribution License (CC BY). The use, distribution or reproduction in other forums is permitted, provided the original author(s) and the copyright owner(s) are credited and that the original publication in this journal is cited, in accordance with accepted academic practice. No use, distribution or reproduction is permitted which does not comply with these terms.
*Correspondence: Xiangyi Li, lixy@ms.xjb.ac.cn