- 1Shanxi Agricultural University, Jinzhong, China
- 2Hebei Key Laboratory of Agroecological Safety, Hebei University of Environmental Engineering, Qinhuangdao, China
The implementation of unsuitable tillage practices has the potential to disrupt the structure integrity of the ploughed layer, as well as to influence the physical parameters of the soil. The application of a reasonable tillage method has been demonstrated to result in an improvement in the physical quality of the soil. Three autumn tillage practices have been implemented at the Dongyang Experimental Station of Shanxi Agricultural University since 2016: no-tillage with straw mulch (NTS), autumn rotary tillage with straw incorporation (RTS), and autumn plough tillage with straw incorporation (PTS). The impact of autumn tillage practices on soil physical quality in the 0–30 cm profile of spring corn fields was evaluated following the corn harvest in 2018 and 2019. The results showed that compared to the NTS treatment, the application of RTS was found to have decreased significantly by 9.6%–24.2% in soil bulk density, while it increased significantly by 12.8%–34.0% in total porosity and by 43.5%–146.4% in macroporosity at a depth of 5–10 cm. In comparison to the NTS treatment, the adoption of PTS was found to decrease significantly by 10.7%–30.5% soil bulk density, while it increased significantly by 9.9%–42.7% the total porosity and 23.1%–202.8% the macroporosity at a depth of 0–10 cm. Furthermore, the soil microporosity significantly increase of 7.5%–11.1% under the RTS treatment at the 0–5 cm soil depth and 7.7%–11.2% under the PTS treatment at the 10–20 cm soil depth. Soil physical quality index (SQI) significantly increase under the RTS and PTS treatments, with a 41.26% and 57.57% improvement, respectively, in comparison to the NTS treatment. In summary, the adoption of autumn tillage with straw return (RTS and PTS) demonstrated a reduction in soil bulk density, an increase in soil porosity, macroporosity, and a promotion of capillary porosity, and promoted the improvement of soil physical quality on the Eastern Loess Plateau when compared to no-tillage with straw mulch (NTS).
1 Introduction
Land degradation represents a significant global environmental concern that may impede global food productivity. This phenomenon may be exacerbated by unsustainable agricultural intensification (Smith et al., 2016; Prăvălie et al., 2021). The soil quality of farmland on the Loess Plateau has been decline for an extended period of time, largely as a result of the application of farming management practices that are not sustainable (Lu et al., 2022). Soil quality is defined as the capacity to sustain crop production, safeguard the environment and enhance animal and plant health within the soil ecosystem (Seleiman et al., 2018; Ding et al., 2021; Ortiz and Sansinenea, 2022). Soil is defined by a set of physical, chemical and biological parameters. The soil physical quality of soil is reflected in changes to its physical parameters, which can in turn affect its chemical and biological parameters (Oliveira et al., 2019). Furthermore, the soil physical quality of the soil is of paramount importance in determining the sustainability of its utilisation.
Tillage treatment is a traditional method of soil improvement, that can significantly regulate the dynamic balance of water, fertilizer, gas and heat in the soil (Sağlam et al., 2015; Liu et al., 2021). Soil tillage is employed with the objective of creating a fine seedbed, controlling weeds, loosening the soil, and improving infiltration. However, this practice also has the potential to cause soil erosion (Alemayehu et al., 2023). Furthermore, tillage practices exert a significant influence on soil structural stability, crop nutrient uptake and ultimately crop yield (Liu et al., 2021). Soil tillage represents a pivotal variable in the alteration of soil physical quality (Maurya et al., 2020). It is of paramount importance to establish a reasonable tillage method, optimize the structure of the ploughed layer and improve the soil physical parameters in order to halt decline in soil quality observed on the Loess Plateau.
At present, numerous studies have been conducted to evaluate the effects of tillage practices on soil physical quality under straw return conditions. However, the effects on physical properties such as soil bulk weight, soil water content, and soil porosity have been found to vary (Fan et al., 2021). Some studies have indicated that rotary tillage with straw incorporation and plough tillage with straw incorporation can have a significant impact on soil disturbance (Lian et al., 2022), which may result in a reduction in soil bulk density in the 0–10 cm and 0–20 cm layers (Zhao et al., 2022). It is similarly postulated that the topsoil bulk density may be significantly reduced by the implementation of no-tillage with straw mulching and plough tillage with straw incorporation (Hou et al., 2018). In addition, the manner in which soil is cultivated can influence the rate of evaporation, infiltration and other water movements, which in turn affects the content of in soil water (Mulebeke et al., 2013). It is generally accepted that no-tillage with straw mulch can effectively reduce water loss and increase soil water content in the tilled layer compared to rotary tillage with straw incorporation and plough tillage with straw incorporation (Yang et al., 2020; Lin et al., 2022). Some studies have also found that plough tillage with straw incorporation can increase soil water storage compared to rotary tillage with straw incorporation (Pareek et al., 2018; Zhao et al., 2022). In addition, there are some differences in soil disturbance due to different tillage practices, which in turn result in different distribution of soil pores (Xue et al., 2018). Compared to no-tillage with straw mulch, rotary tillage and plough tillage with straw incorporation can significantly increase the porosity of the soil (Yuan et al., 2023). However, some research has found that as the amount of straw incorporated increased, the total soil porosity and soil capillary porosity under the no-tillage straw mulching also gradually increased (Li et al., 2013; Yuan et al., 2022). The differences in soil physical parameters among different tillage practices may be caused by climatic conditions, soil texture, cropping system, tillage period and the amount of straw incorporated.
Autumn tillage practice is a distinctive traditional agricultural management practice of corn production in the Eastern Loess Plateau (Qi et al., 2021). The adoption of autumn tillage practice not only accelerates soil the maturation of the soil, but also breaks weed seed dormancy and stimulates germination (Boström, 1999). However, a review of the literature on the effects of tillage practices on the physical properties of maize field soils in the Loess Plateau revealed that the majority of the soil tillage operations were conducted prior to sowing (Li et al., 2013). A limited number of studies have been conducted on the implementation of post-harvest autumn tillage measures. The majority of these studies have focused on the effect of autumn tillage on crop yield. There is a paucity of academic attention devoted to the impact of autumn tillage practices on soil quality following harvest. The clarification of the impact of autumn tillage practices on soil physical parameters may contribute to improvement of soil quality and the sustainable production of corn in the region (Zhang et al., 2021).
The objective of this study was to analyze the effects of different autumn tillage practices on soil bulk density, soil water content, soil porosity, generalised soil structure index (GSSI) and soil three-phase structure distance (STPSD) of 0–30 cm layers. Additionally, the study aimed to comprehensively evaluate the effects of different autumn tillage practices on the soil physical quality of corn fields using soil quality index (SQI) in the Eastern Loess Plateau. The hypothesiz was that the adoption of autumn tillage would result in improvements in soil bulk density, soil moisture content and soil porosity, leading to a significant enhacement of soil physical properties in corn fields on the Loess Plateau.
2 Materials and methods
2.1 Experimental site
The experiment was conducted at the Dongyang Experimental Demonstration Base of Shanxi Agricultural University (N37°32′44.28″, E112°37′26.78″). The region exhibts a typical temperate continental monsoon climate, with an altitude of 750–850 m, a mean annual temperature of 8°C–12°C, a mean annual precipitation of 450–550 mm, a mean annual evaporation of 1996 mm, an annual sunshine time of 2,650 h, and a frost-free period of 144–170 days. The soil texture is classified as clay loam. The initially soil properties in the 0–40 cm soil layer were presented in Table 1.
2.2 Experimental design
The experimental design employed a single-factor randomized block experimental design since 2016. Three autumn tillage practices were implemented, including no-tillage with straw mulching (NTS), rotary tillage with straw incorporation (RTS) and plough tillage with straw incorporation (PTS). Each practice was performed with three repetitions in a plot area of 150 m2 (5 × 30 m). Following the corn harvest, the crop straw was crushed and covered on the soil surface for all treatments. In generally, autumn tillage was conducted between late October and early November. Rotary tillage was implemented to a depth of 15–20 cm under the RTS treatment. In the PTS treatment, plough tillage was implemented to a depth of 20–25 cm followed by shallow rotary tillage (10–15 cm). In the subsequent year, conventional seeders were employed for sowing, fertilisation and herbicide spraying in both the RTS and PTS treatments. In the autumn, no tillage was employed under the NTS treatment. Instead, a no-tillage seeder was utilised to sow, fertilise and apply herbicide. The corn cultivar employed was Dafeng 30, with the sowing density of 67,500 plantha−1. The corn was planted with uniform row spacing between rows (60 cm) under the RTS and PTS treatments, while the wide-narrow row spacing between rows was 80 + 40 cm were adopted under the NTS treatment. For all treatments, the base fertilisers consisted of 75 kg ha−1 of potassium chloride, 416.7 kg ha−1 of urea and 210 kg ha−1 of diammonium phosphate applied using the seeder. The other farming management practices were the typical of those employed in the region. The precipitation during the growth period of corn was 309.3 mm and 249.6 mm in 2018 and 2019, respectively.
2.3 Measurements
2.3.1 Soil sample collection
Following the harvesting of corn in 2018 and 2019, the soil bulk density of the 0–5 cm, 5–10 cm, 10–20 cm and 20–30 cm layers was quantified utilising the cutting ring method (Xue et al., 2023), while soil water content, soil porosity, GSSI and STPSD were calculated. The cutting ring containing the undisturbed soil sample was transported to the laboratory, where it was promptly weighed. This involved including the fresh soil sample, cutting ring, and the bottom mash lid, and the result was recorded as M1. Subsequently, the cutting ring was positioned on a tray lined with gauze, and a gradual addition of water was initiated. Once the soil had reached saturation, the sample was weighed and recorded as M2. Subsequently, the cutting ring containing the water-saturated undisturbed soil sample was placed in an oven and subjected to drying until a constant weight was achieved at 105°C. Once the cutting ring had cooled, the soil sample was reweighed and recorded as M3. Subsequently, the soil sample was extracted from the cutting ring. The cutting ring and the bottom mash lid were then weighed and recorded as M0. The soil bulk density and soil gravimetric water content were calculated using Eqs 1, 2, respectively.
where, ρb is Soil bulk density (g·cm−3), θg is soil gravimetric water content (%), M0 is the weight of the cutting ring, including the bottom mash lid (g), M1 is the sum of the weight of the cutting ring and fresh soil (g), M3 is the weight of the cutting ring and soil after drying (g), V is the volume of the cutting ring (cm3).
2.3.2 Soil sample collection
The total soil porosity (Pt, %), macroporosity (Pɑ, %) and microporosity (Pc, %) were calculated in accordance with Eqs. 3–5 (Li et al., 2006).
Where, Pd is the particle density, which is generally 2.65 g cm−3 for most mineral soils, ρb is the same as in Eq. 1, θg is the same as in Eq. 2, θg is the soil capillary water content (%), M2 is the weight of the ring cutter and the weight of saturated water absorption (g), M0 and M3 were the same as in Eq. 1.
2.3.3 Generalised soil structure index
The generalised soil structure index (GSSI) and the soil three-phase structure distance (STPSD) were calculated by using Eqs 7, 8, respectively (Liu et al., 2022; Zuo et al., 2022).
Where, XS is the percentage of the soil solid phase (%), XL is the percentage of the soil liquid phase (%), XG is the percentage of soil gaseous phase (%).
2.3.4 Soil physical quality index
The soil physical quality index was calculated by multiplying the assigned weight of the individual soil physical indicator by the indicator score [Eq. (9); Li et al., 2013].
where, SQIp is the soil physical quality index, Wi is the assigned weight of the ith soil physical indicator, Ni is the indicator score of the ith soil physical indicator, n is the number of soil physical indicators.
2.3.5 Statistical analysis
In the present study, Microsoft Excel 2016 software was employed for the sorting and plotting of data. The statistical software package SPSS 16.0 (IBM SPSS Inc., Chicago, United States of America) was employed for variance analysis and multiple comparisons. As additionally, the new multiple range method (Duncan) was utilised to assess the disparity between the various autumn tillage treatments (p ≤ 0.05).
3 Results
3.1 Soil bulk density
The soil bulk density in the 0–30 cm profile under different autumn tillage practices exhibited a range of values between 1.0 and 1.6 g cm−3 (Figure 1). Compared to the NTS treatment, the soil bulk density exhibited a reduction of 10.7%–23.5% at a depth of 0–5 cm under the PTS treatment. The soil bulk density at a depth of 5–10 cm exhibited a reduction of 9.6%–24.2% under the RTS treatment and by 11.1%–30.5% under the PTS treatment, compared to the NTS treatment. However, there was no significant difference between the RTS and PTS treatments. The soil bulk density at the 20–30 cm soil depth under the PTS treatment exhibited a 21.7% decrease in 2018, while it exhibited an10.5% increase in 2019.
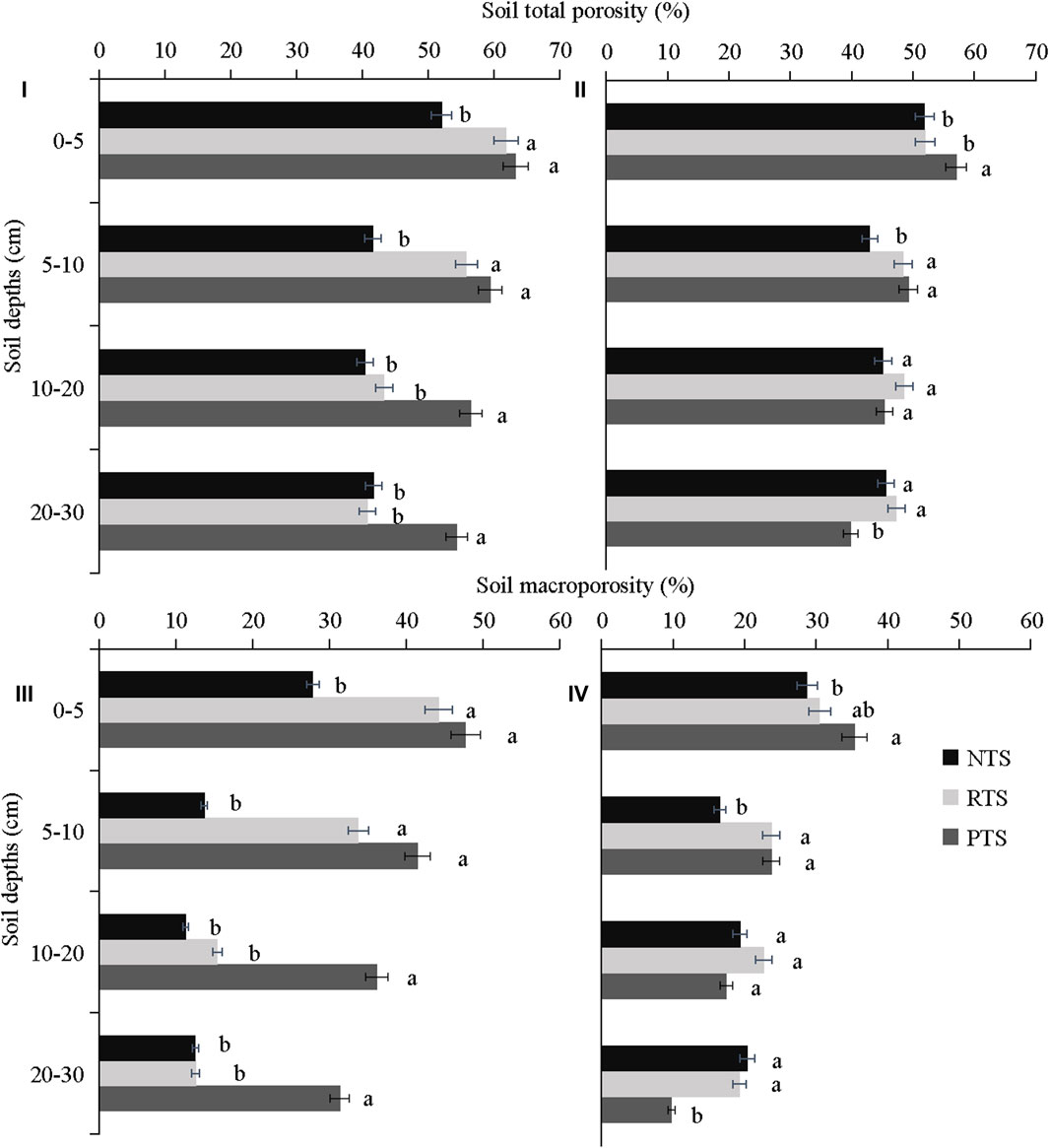
Figure 1. Distribution of soil bulk density under different autumn tillage practices. NTS indicates no-tillage with straw mulching, RTS indicates rotary tillage with straw incorporation, PTS indicates plough tillage with straw incorporation. (I) and (II) with in brackets indicate soil bulk density after corn harvest in 2018 and 2019, respectively. The lowercase letters indicate significant differences between different autumn tillage practices (p ≤ 0.05).
3.2 Soil gravimetric water content
The gravimetric water content of the soil profile between 0 and 30 cm depth, as influenced by different autumn tillage practices, exhibited a range of values between 16.0% and 20.1% (Figure 2). Compared to the RTS treatment, the gravimetric water content of the 0–5 cm layer beneath the PTS treatment exhibited a 7.7% decline 2018, while a 13.0% increase was observed in 2019. Compared to the NTS treatment, the gravimetric water content of the 5–10 cm and 10–20 cm soil layers under the PTS treatment exhibited a decrease of by 7.7% and 4.4%, respectively, in 2018. Conversely, an increase of 8.7% and 8.6%, respectively, was observed in 2019. The gravimetric water content of the 20–30 cm beneath the RTS treatment exhibited a 4.8% decline in 2018, while a 15.0% increase was observed in 2019.
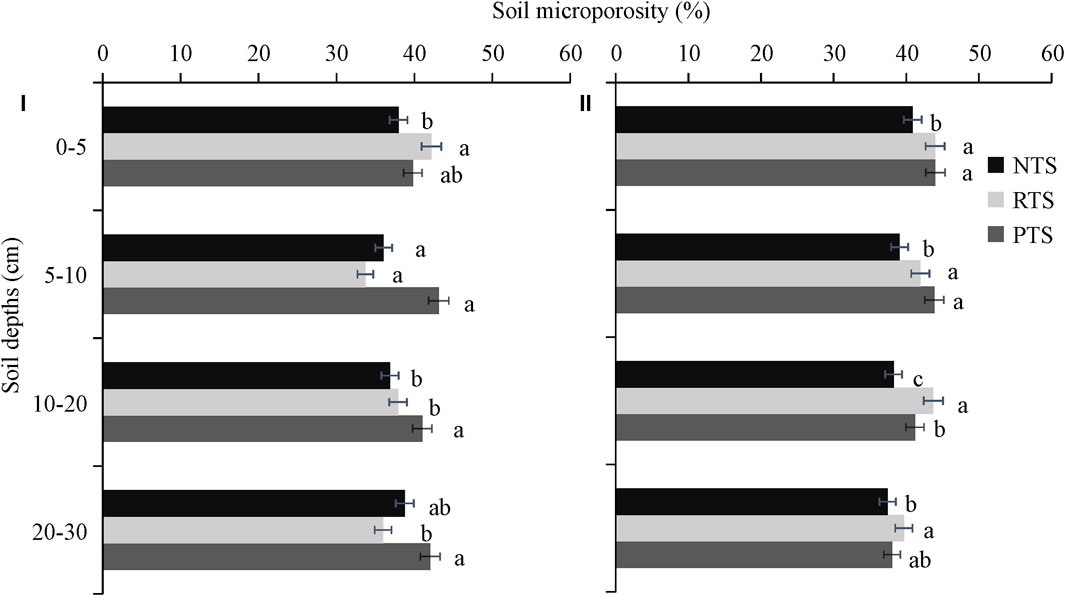
Figure 2. Distribution of soil gravimetric water content under different autumn tillage practices. NTS indicates no-tillage with straw mulch, RTS indicates rotary tillage with straw incorporation, PTS indicates plough tillage with straw incorporation. (I) and (II) with in brackets indicate the gravimetric soil water content after maize harvest in 2018 and 2019, respectively. Lower case letters indicate significant differences between different autumn tillage practices (p ≤ 0.05).
3.3 Soil total porosity and macroporosity
The total porosity of the soil in the 0–30 cm layer under different autumn tillage practices was found to rang from 39.9% to 63.3% (Figures 3–I,3–II). Compared to the NTS treatment, the total porosity of the soil in the 0–5 cm and 5–10 cm layers beneath the PTS treatment exhibited an increase of 9.9%–21.6% and 14.7%–42.7%, respectively. The total porosity of the soil at a depth of 5–10 cm exhibited an increase of 12.8%–34.0% under the RTS treatment compared with the NTS treatment. Compared to the NTS and RTS treatments, the total porosity of the 20–30 cm beneath the PTS treatment significantly increased in 2018, with 30.3% and 33.3% increase, respectively. Conversely, a significant decrease was observed in 2019, with a 12.5% and 15.7% reduction, respectively. Furthermore, the soil macroporosity in the 0–30 cm layer under different autumn tillage practices exhibited a range of value, sfrom 9.8%–47.7% (Figures 3–III,3–IV). Compared to the NTS treatment, The PTS treatment resulted in a significant increase in soil macroporosity, with values ranging from 23.1% to 71.5% in the 0–5 cm and from 43.5% to 202.8% 5–10 cm layers. The soil macroporosity at a depth of 5–10 cm exhibited an increase of 43.6%–146.4% under the RTS treatment compared to the NTS treatment. Compared to the NTS and RTS treatments, the macroporosity of the soil in the 20–30 cm layer beneath the PTS treatment significantly increased in 2018, with values increasing by 150.0% and 149.4%, respectively. Conversely, there was asignificant decrease in 2019, with values decreasing by 52.0% and 49.2%, respectively.
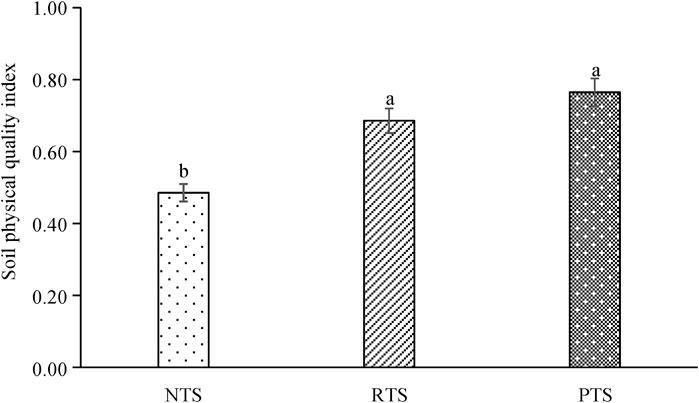
Figure 3. Distribution of soil total porosity and soil macroporosity under different autumn tillage practices. NTS indicates no tillage with straw mulch, RTS indicates rotary tillage with straw incorporation, PTS indicates plough tillage with straw incorporation. (I) and (II) in parentheses indicate soil total soil porosity after maize harvest in 2018 and 2019, respectively, (III) and (IV) in parentheses indicate soil macroporosity after maize harvest in 2018 and 2019, respectively. Lower case letters indicate significant differences between different autumn tillage practices (p ≤ 0.05).
3.4 Soil microporosity
Microporosity in the 0–30 cm profile under different autumn tillage practices ranged from 33.7% to 44.0% (Figure 4). Compared to the NTS treatment, soil microporosity at the 0–5 cm depth increased significantly increased by 7.5%–11.1% under the RTS treatment, while under the PTS treatment it increased significantly by 7.7%–11.2% at the 10–20 cm depth. Compared to the RTS treatment, soil microporosity at the 10–20 cm depth under the PTS treatment increased significantly by 8.2% in 2018, while it decreased significantly by 5.8% in 2019.
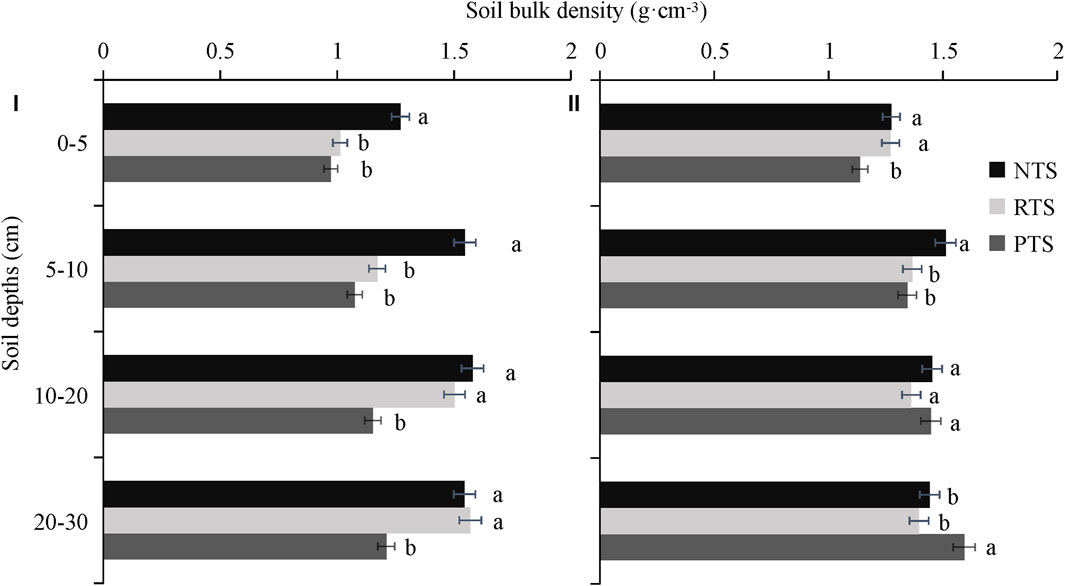
Figure 4. Distribution of soil microporosity under different autumn tillage practices. NTS indicates no tillage with straw mulch, RTS indicates rotary tillage with straw incorporation, and PTS indicates plough tillage with straw incorporation. a and b in parentheses indicate soil microporosity after maize harvest in 2018 and 2019, respectively. Lower case letters indicate significant differences between different autumn tillage practices (p ≤ 0.05).
3.5 Generalised soil structure index and soil three-phase structure distance
Compared with the NTS treatment, the PTS treatment significantly reduced the GSSI at 0–5 cm depth by 5.5%–24.5% (Table 2). Compared to the NTS and RTS treatments, the GSSI at the 20–30 cm depth under the PTS treatment significantly increased in 2018 by 10.2% and 10.8%, respectively, while it significantly decreased in 2019 by 16.8% and 15.8%, respectively. In addition, compared to the NTS treatment, the PTS treatment significantly increased the STPSD at the 0–5 cm depth by 184.4%–675.8%. Compared to the NTS and RTS treatments, the STPSD at the 20–30 cm soil depth under the PTS treatment significantly by 49.1%–49.9% in 2018, while it increased by 195.5%–168.8% in 2019.
3.6 Soil physical quality index
Soil physical quality indexes under the RTS and PTS treatments were significantly greater by 41.26% and 57.57%, respectively, compared with that under the NTS treatment (Figure 5), however, there was no significant difference between the RTS and PTS treatment.
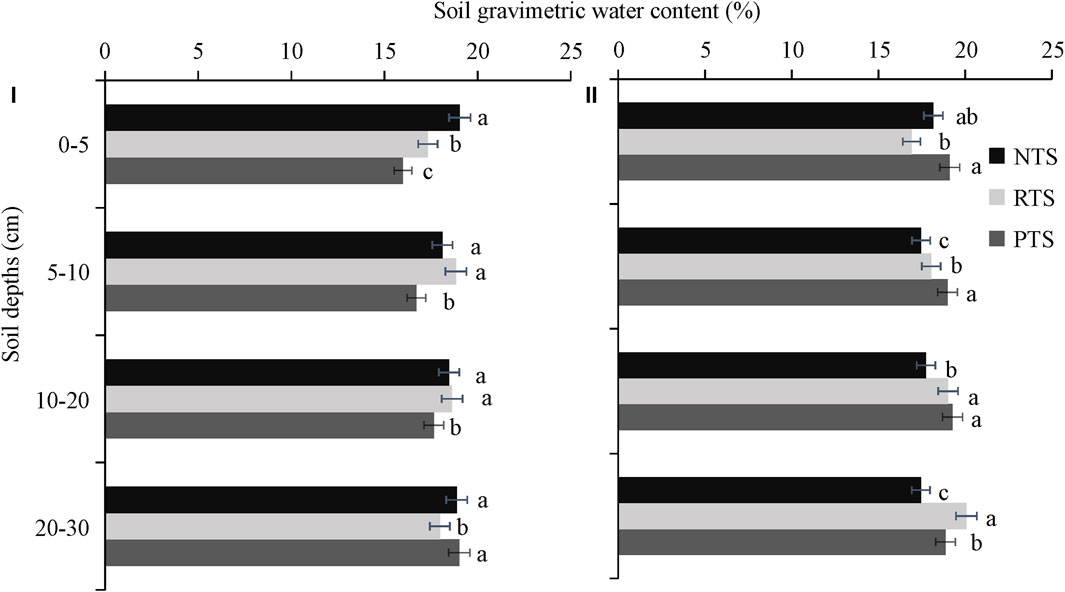
Figure 5. Effect of different autumn tillage practices on the soil physical quality index. NTS indicates no tillage with straw mulch, RTS indicates rotary tillage with straw incorporation, and PTS indicates plough tillage with straw incorporation. The value of the soil physical quality index for each treatment is the mean value between 2018 and 2019. Lower case letters indicate significant differences between different autumn tillage practices (p ≤ 0.05).
4 Discussion
Soil bulk density can affect the quality of soil structure. A lower soil bulk density indicates a higher organic matter content, resulting in a better structure and hydrological conditions (Bondi et al., 2018). This study concluded that, compared to the NTS treatment, the RTS treatment significantly decreased soil bulk density at the 5–10 cm soil depth, while the PTS treatment significantly decreased soil bulk density at the 0–10 cm depth, a similar result was reported by Hou et al. (2012). This may be due to the incorporation of crop straw into the soil during the tillage operations, which promotes the formation of soil aggregates, increases soil aeration, and ultimately improves soil bulk density (Hou et al., 2012; Zhang et al., 2021). Differences between RTS and PTS treatments may be due to differences in tillage depth (Zhang et al., 2018; Zhao et al., 2022). Some studies also concluded that the use of autumn tillage reduced soil bulk density in deeper layers (Fan et al., 2021; Gan et al., 2022). Such different conclusions could be caused by differences in the amount of straw returned, straw return method, trial duration, tillage implements, soil parameters, and climatic conditions during the different trials.
Generally, higher soil porosity indicates that the soil is looser and can hold more air and water, resulting in higher root growth (Zhou et al., 2021). Compared to the NTS treatment, the application of RTS significantly increased soil total porosity and macroporosity at the 5–10 cm depth, while the application of PTS dramatically increased soil total porosity and macroporosity at the 0–10 cm depth in the current study. This may be due to different depths of soil tillage for the different treatments (Xue et al., 2018). It also showed that autumn tillage with straw return can significantly increase soil total soil porosity and aerated porosity at the 5–10 cm depth, in agreement with previous studies (Zhang et al., 2018; Zhao et al., 2022). Zhao et al. (2022) concluded that 16 years of continuous rotary tillage and plough tillage significantly improved the soil porosity of in the 0–20 cm layer compared to no-tillage (Hou et al., 2012). Zhang et al. (2018) showed that continuous implementation of 10-year autumn tillage with straw incorporation in the field significantly increased soil porosity of in the 0–60 cm profile compared to no-tillage with straw return in Shaanxi Province (Hou et al., 2012). In addition, many factors, such as the amount returned of straw returned, the method of straw return method and the duration of the trial can influence the effect of autumn tillage on soil porosity at different depths. The interaction effect of tillage practices and straw return on soil porosity will be investigated in future studies.
Under the ideal conditions of total soil porosity and macroporosity, the higher the soil microporosity, the better the soil structure (Shao et al., 2020). Compared to the NTS treatment, the RTS and PTS treatments significantly increased soil microporosity at depths of 0–5 cm and 10–20 cm depths, respectively. This may be due to the reformation of the soil microporosity structure after soil tillage. However, the difference in the depth and intensity of tillage between the RTS and PTS treatments leads to the difference in soil microporosity distribution. Furthermore, compared to the PTS treatment, the soil microporosity at the 10–20 cm depth under the RTS treatment significantly decreased in 2018, while it significantly increased in 2019, indicating that the effect of autumn tillage with straw return on soil microporosity may be a long-term and slow process (Zang et al., 2021; Wang et al., 2022). We will continue to carry out positioning trials to more rationally assess the effect of autumn tillage with straw return on soil microporosity.
Soil gravimetric water content is an important indicator of soil physical parameters (Shao et al., 2020; Zhao et al., 2022). Compared to the NTS treatment, the RTS and PTS treatments significantly decreased the gravimetric water content in the 5–30 cm soil profile in 2018, whereas they significantly increased it in 2019 (Lai et al., 2023). It indicates that theeffect of autumn straw return on the soil gravimetric water content at the harvest is uncertain (Al-Abed et al., 2003), as it may be affected by the difference in the distribution of rainfall and temperature distribution 2 years, crop water use efficiency and the change in soil infiltration capacity (Maharjan et al., 2018). In future studies, it is necessary to evaluate the effect of autumn tillage with straw return on soil moisture on long time scales based on a site experiment.
The closer the GSSI is to 100, the closer the STPSD is to 0, indicating that the soil structure is closer to the ideal state (Fu et al., 2019). This study found that the trends of GSSI and STPSD in different soil layers were opposite (except for the RTS treatment in 2018) under the same autumn tillage practice, which is consistent results reported by Zuo et al. (Zuo et al., 2022). Compared to the NTS treatment, the GSSI at the 0–5 cm soil depth decreased significantly under the PTS treatment, unlike the STPSD. This may be because the soil temperature under NTS treatment (Huang et al., 2022), which can incresae the activity of soil animals and micro-organisms (Nannipieri et al., 2017), thereby improving soil structure. In addition, there is less soil disturbance under the NTS treatment, which can lead to significant changes in topsoil structure. In addition, there were inconsistent results of GSSI and STPSD in the 5–30 cm soil profile under different treatments, probably due to the short of the autumn tillage experiment treatment, which should be verified by a long-term positioning intensity.
In general, a higher soil quality index indicates better soil quality. In the current study, the use of RTS and PTS significantly increased the SQIp compared to NTS treatment, which is consistent with the results findings of other studies (Zhang et al., 2018; Ling et al., 2022). This may be due to the fact that soil tillage and straw incorporation can increase soil aeration and promote the activity of soil microorganisms, which in turn improves soil physical quality. However, conflicting results have also been reported (Luo et al., 2017; Liu et al., 2022), with significantly higher SQI under the NTS treatment, which may be due to the differences in soil properties and cropping systems between studies. In future studies, it is necessary to integrate the current literature on SQI based on meta-analysis to synthesise the effects of tillage practices on SQI and their influencing factors.
In this study, only some soil physical indicators such as soil bulk density, soil porosity and soil water content were assessed when evaluating the effect of soil tillage on the soil quality index. Physical indicators such as soil compactness (Bondi et al., 2018), soil aggregate stability (Zhou et al., 2021) and soil moisture characteristic curve (Zhang et al., 2021), as well as soil chemical and biological indicators (Fan et al., 2021) were not included, and the results of the study can only explain to a certain extent the effect of soil tillage on soil physical quality to a limited extent and have limitations. Future research will measure more indicators affecting soil quality in drylands to comprehensively assess the impact of tillage on soil quality and provide a basis for the sustainable development of dryland wheat fields (Xue et al., 2023). In addition, the applicability of the study results under different geographical and climatic conditions will be assessed.
There are also some limitations to this research. Soil samples were collected for analysis of soil physical parameters at the time of maize harvest in 2018 and 2019, even when autumn tillage practices were implemented after maize harvest (generally at the end of October). Therefore, the influence of autumn tillage on soil physical parameters was stronger in the winter of that year and in the spring of the following year (Luo et al., 2017). In the future, it will be more important to assess the changes in soil physical parameters from autumn tillage to sowing (Buragienė et al., 2019). Although testing begain in 2016, the duration of testing is relatively short (2–3 years) the sampling time. The potential impact of autumn tillage on soil physical parameters remains uncertain (Zhang et al., 2018; Zhao et al., 2021). Therefore, the impact of autumn tillage practices on soil physical parameters may be limited and long-term research is needed to scientifically assess its effects.
This research mainly analyzed the effect of autumn tillage on soil physical parameters such as soil bulk density, soil water content and soil porosity distribution. Although the above parameters can reflect soil the physical quality of the soil to some extent, there are some shortcomings. In the future, other soil parameters such as soil aggregate structure and stability (Zhou et al., 2021), field water capacity (Bondi et al., 2018), soil cone penetrometer resistance an index of soil compaction (Abu-Hamdeh et al., 1995), and temperature, will be analyzed to provide a comprehensive and scientific assessment of the effects of autumn tillage on soil physical quality (Raghavan et al., 1990). Field water capacity is also an important parameter for assessing the physical quality of the soil and is extremely important for for finding correlations between soil temperature, water and air (Raghavan et al., 1990). Field water capacity is the suspended capillary water, which is determined by soil microporosity. Although this research analyzed the effect of autumn tillage on soil microporosity, it differs from the direct analysis of field water capacity (Shao et al., 2020; Zhang et al., 2021). The soil quality index only selects physical indicators, which to some extent limits the comprehensive assessment of soil properties by autumn tillage + straw treatment. The next study, while refining the physical soil indicators, will add soil chemical and biological indicators will be added to more accurately reflect changes in soil quality and provide theoretical references for soil management (Hermans et al., 2020). In the current study, the focus of our research was to evaluate the effects of different autumn tillage practices on soil physical quality. However, understanding maize growth and development and yield formation is also important for assessing the effects of soil tillage on soil quality (Kovács et al., 2023). In future studies, we will further investigate the relationship between crop yield formation and soil quality.
In the current study, we used a special no-tillage planter with a narrow-wide row planting pattern of 40 cm + 80 cm, which could not be adjusted for the NTS treatments. However, we used the uniform 60 cm row planting pattern for the RTS and NTS treatments, which is widely used by local farmers. The difference between the two planting patterns may have an effect on the physical quality of the soil. In future studies, we will standardise the use of narrow-wide row planting pattern to avoid the influence of other factors such as planting pattern, crop growth on the results. The above limitations will affect the results and the presentation of conclusions, which is of great importance for the scientific and innovative nature of the research. Therefore, in the future, these limitations will be addressed in the future: further tests will be carried out by extending the sampling period and selecting more targeted parameters, thus providing a more scientific assessmeng of the impact of autumn tillage on soil physical quality and providing a more accurate scientific basis for improving soil physical quality through autumn tillage practices in the Eastern Loess Plateau.
5 Conclusion
Compared to the autumn no-tillage with straw mulch (NTS) treatment, the autumn rotary tillage with straw incorporation (RTS) treatment significantly decreased soil bulk density and significantly increased soil total porosity and macroporosity at the 5–10 cm depth, and significantly increased soil microporosity at the 0–5 cm soil depth. The autumn plough tillage with straw incorporation (PTS) treatment significantly reduced soil bulk density while significantly increasing soil total soil porosity and macroporosity at the 0–10 cm depth, and significantly increasing soil microporosity at the 10–20 cm depth. The NTS treatment had a significantly lower soil physical quality index than the RTS and PTS treatments. Overall, the application of RTS and PTS after maize harvest can improve soil physical quality than NTS in the short term more than NTS in the Eastern Loess Plateau.
Data availability statement
The raw data supporting the conclusions of this article will be made available by the authors, without undue reservation.
Author contributions
YF: Data curation, Writing–original draft. YY: Data curation, Writing–original draft. TL: Visualization, Writing–review and editing. WL: Writing–review and editing. XT: Writing–review and editing. GL: Writing–review and editing. NL: Conceptualization, Funding acquisition, Writing–review and editing.
Funding
The author(s) declare that financial support was received for the research, authorship, and/or publication of this article. This research was funded by Fundamental Research Program of Shanxi Province (202303021211096, 202103021224162).
Conflict of interest
The authors declare that the research was conducted in the absence of any commercial or financial relationships that could be construed as a potential conflict of interest.
Publisher’s note
All claims expressed in this article are solely those of the authors and do not necessarily represent those of their affiliated organizations, or those of the publisher, the editors and the reviewers. Any product that may be evaluated in this article, or claim that may be made by its manufacturer, is not guaranteed or endorsed by the publisher.
References
Abu-Hamdeh, N. H., Carpenter, T. G., Wood, R. K., and Holmes, R. G. (1995). Combine tractive devices: effects on soil compaction. SAE Trans. 412–430. doi:10.4271/952159
Al-Abed, N., and Amayreh, J. (2003). Polyacrylamide polymer (PAM) effect on the propagation of the wetting front on a Jordanian soil under trickle irrigation system. Archives Agron. Soil Sci. 49 (3), 289–299. doi:10.1080/0365034031000148309
Alemayehu, A. A., Getu, L. A., Samual, T., Ayalew, B., Addis, H. K., Feyisa, T., et al. (2023). Effects of tillage practices and planting techniques on crop yield and soil properties in northwestern lowlands of Ethiopia. J. Agric. Food Res. 14, 100852. doi:10.1016/j.jafr.2023.100852
Bondi, G., Creamer, R., Ferrari, A., Fenton, O., and Wall, D. (2018). Using machine learning to predict soil bulk density on the basis of visual parameters: tools for in-field and post-field evaluation. Geoderma 318, 137–147. doi:10.1016/j.geoderma.2017.11.035
Boström, U. (1999). Type and time of autumn tillage with and without herbicides at reduced rates in southern Sweden: 1. Yields and weed quantity. Soil Tillage Res. 50 (3-4), 271–281. doi:10.1016/s0167-1987(99)00016-1
Buragienė, S., Šarauskis, E., Romaneckas, K., Adamavičienė, A., Kriaučiūnienė, Z., Avižienytė, D., et al. (2019). Relationship between CO2 emissions and soil properties of differently tilled soils. Sci. Total Environ. 662, 786–795. doi:10.1016/j.scitotenv.2019.01.236
Ding, Z., Kheir, A. M. S., Ali, O. A. M., Hafez, E. M., ElShamey, E. A., Zhou, Z., et al. (2021). A vermicompost and deep tillage system to improve saline-sodic soil quality and wheat productivity. J. Environ. Manag. 277, 111388. doi:10.1016/j.jenvman.2020.111388
Fan, Y., Gao, J., Sun, J., Liu, J., Su, Z., Wang, Z., et al. (2021). Effects of straw returning and potassium fertilizer application on root characteristics and yield of spring maize in China inner Mongolia. Agron. J. 113 (5), 4369–4385. doi:10.1002/agj2.20742
Frouz, J., and Bujalský, L. (2018). Flow of CO2 from soil may not correspond with CO2 concentration in soil. Sci. Rep. 8 (1), 10099. doi:10.1038/s41598-018-28225-z
Fu, Q., Zhao, H., Li, H., Li, T., Hou, R., Liu, D., et al. (2019). Effects of biochar application during different periods on soil structures and water retention in seasonally frozen soil areas. Sci. Total Environ. 694, 133732. doi:10.1016/j.scitotenv.2019.133732
Gan, J., Qiu, C., Han, X., Kwaw-Mensah, D., Chen, X., Yan, J., et al. (2022). Effects of 10 years of the return of corn straw on soil aggregates and the distribution of organic carbon in a Mollisol. Agronomy 12 (10), 2374. doi:10.3390/agronomy12102374
Hermans, S. M., Buckley, H. L., Case, B. S., Curran-Cournane, F., Taylor, M., and Lear, G. (2020). Using soil bacterial communities to predict physico-chemical variables and soil quality. Microbiome 8, 79–13. doi:10.1186/s40168-020-00858-1
Hou, X., Li, R., He, W., Dai, X., Ma, K., and Liang, Y. (2018). Superabsorbent polymers influence soil physical properties and increase potato tuber yield in a dry-farming region. J. Soils Sediments 18, 816–826. doi:10.1007/s11368-017-1818-x
Hou, X., Li, R., Jia, Z., Han, Q., Wang, W., and Yang, B. (2012). Effects of rotational tillage practices on soil properties, winter wheat yields and water-use efficiency in semi-arid areas of north-west China. Field Crops Res. 129, 7–13. doi:10.1016/j.fcr.2011.12.021
Hou, X. Q., Li, R., Jia, Z. K., Han, Q. F., Yang, B. P., and Nie, J. F. (2012). Effects of rotational tillage practices on soil structure, organic carbon concentration and crop yields in semi-arid areas of northwest China. Soil Use Manag. 28 (4), 551–558. doi:10.1111/j.1475-2743.2012.00429.x
Huang, C., Cheng, C., Wang, Z., Zhao, X., Yang, Y., Hu, L., et al. (2022). Straw strip mulching increased soil organic carbon components of a wheat field in dry farming regions of the Loess Plateau. Water 14 (17), 2645. doi:10.3390/w14172645
Kovács, G. P., Simon, B., Balla, I., Bozóki, B., Dekemati, I., Gyuricza, C., et al. (2023). Conservation tillage improves soil quality and crop yield in Hungary. Agronomy 13 (3), 894. doi:10.3390/agronomy13030894
Lai, P., Nabi, F., Chen, H., Zhao, C., Yang, G., Chen, T., et al. (2023). The long-term straw returning to paddy land altered the soil phosphate fractions and composition of microbial communities. Eurasian. Soil Sci. 1–15. doi:10.1134/s1064229322602207
Li, P., Zhang, T., Wang, X., and Yu, D. (2013). Development of biological soil quality indicator system for subtropical China. Soil Tillage Res. 126, 112–118. doi:10.1016/j.still.2012.07.011
Li, Y. Y., and Shao, M. A. (2006). Change of soil physical properties under long-term natural vegetation restoration in the Loess Plateau of China. J. Arid Environ. 64 (1), 77–96. doi:10.1016/j.jaridenv.2005.04.005
Lian, H., Wang, Z., Li, Y., Xu, H., Zhang, H., Gong, X., et al. (2022). Straw strip return increases soil organic carbon sequestration by optimizing organic and humus carbon in aggregates of mollisols in Northeast China. Agronomy 12 (4), 784. doi:10.3390/agronomy12040784
Lin, H., He, J., Li, H., Li, H. W., Wang, Q., Lu, C., et al. (2022). A review of research progress on soil organic cover machinery in China. Agriculture 12 (9), 1311. doi:10.3390/agriculture12091311
Ling, J., Zhou, J., Wu, G., Zhao, D., Wang, Z., Wen, Y., et al. (2022). Deep-injected straw incorporation enhances subsoil quality and wheat productivity. Plant Soil 499, 207–220. doi:10.1007/s11104-022-05660-6
Liu, W., Fu, H., Bao, M., Luo, C., Han, X., Zhang, D., et al. (2022a). Emulsions stabilized by asphaltene-polyacrylamide-soil three-phase components: stabilization mechanism and concentration effects. Sep. Purif. Technol. 302, 122157. doi:10.1016/j.seppur.2022.122157
Liu, X., Cheng, X., Wang, N., Meng, M., Jia, Z., Wang, J., et al. (2021). Effects of vegetation type on soil shear strength in Fengyang Mountain Nature Reserve, China. Forests 12 (4), 490. doi:10.3390/f12040490
Liu, X., Liu, H., Ren, D., Liu, C., Zhang, Y., Wang, S., et al. (2022b). Interlinkages between soil properties and keystone taxa under different tillage practices on the North China Plain. Appl. Soil Ecol. 178, 104551. doi:10.1016/j.apsoil.2022.104551
Liu, Z., Cao, S., Sun, Z., Wang, H., Qu, S., Lei, N., et al. (2021). Tillage effects on soil properties and crop yield after land reclamation. Sci. Rep. 11 (1), 4611. doi:10.1038/s41598-021-84191-z
Lu, A., Tian, P., Mu, X., Zhao, G., Feng, Q., Guo, J., et al. (2022). Fuzzy logic modeling of land degradation in a Loess Plateau watershed China. Remote Sens. 14 (19), 4779. doi:10.3390/rs14194779
Luo, Z., Gan, Y., Niu, Y., Zhang, R., Li, L., Cai, L., et al. (2017). Soil quality indicators and crop yield under long-term tillage systems. Exp. Agric. 53 (4), 497–511. doi:10.1017/s0014479716000521
Maharjan, G. R., Prescher, A. K., Nendel, C., Ewert, F., Mboh, C. M., Gaiser, T., et al. (2018). Approaches to model the impact of tillage implements on soil physical and nutrient properties in different agro-ecosystem models. Soil Tillage Res. 180, 210–221. doi:10.1016/j.still.2018.03.009
Maurya, S., Abraham, J. S., Somasundaram, S., Toteja, R., Gupta, R., and Makhija, S. (2020). Indicators for assessment of soil quality: a mini-review. Environ. Monit. Assess. 192, 604–622. doi:10.1007/s10661-020-08556-z
Mulebeke, R., Kironchi, G., and Tenywa, M. M. (2013). Soil moisture dynamics under different tillage practices in cassava–sorghum based cropping systems in eastern Uganda. Ecohydrol. Hydrobiology 13 (1), 22–30. doi:10.1016/j.ecohyd.2013.02.001
Nannipieri, P., Greco, S., and Ceccanti, B. (2017). Ecological significance of the biological activity in soil. Soil Biochem. 293–356. doi:10.1201/9780203739389-6
Oliveira, F. C. C., Ferreira, G. W. D., Souza, J. L. S., Vieira, M. E. O., and Pedrotti, A. (2019). Soil physical properties and soil organic carbon content in northeast Brazil: long-term tillage systems effects. Sci. Agric. 77. doi:10.1590/1678-992x-2018-0166
Ortiz, A., and Sansinenea, E. (2022). The role of beneficial microorganisms in soil quality and plant health. Sustainability 14 (9), 5358. doi:10.3390/su14095358
Pareek, N. K., Verma, J. K., Godara, S. L., and Kumar, A. (2018). Effect of tillage practices on soil moisture status and performance of Pearl millet cultivars under rainfed conditions. J. Soil Water Conservation 17 (4), 372–377. doi:10.5958/2455-7145.2018.00057.7
Prăvălie, R., Patriche, C., Borrelli, P., Panagos, P., Roșca, B., Dumitraşcu, M., et al. (2021). Arable lands under the pressure of multiple land degradation processes. A global perspective. Environ. Res. 194, 110697. doi:10.1016/j.envres.2020.110697
Qi, Z., Zhang, H., Li, N., Liang, G., Du, T. Q., Li, J. H., et al. (2021). Effects of different autumn tillage practices on soil physical quality of maize field on Loess Plateau of China. Soils 53 (4), 826–832. doi:10.13758/j.cnki.tr.2021.04.021
Raghavan, G. S. V., Alvo, P., and McKyes, E. (1990). Soil compaction in agriculture: a view toward managing the problem. Adv. Soil Sci. 11, 1–36. doi:10.1007/978-1-4612-3322-0_1
Sağlam, M., Selvi, K. C., Dengiz, O., and Gürsoy, F. E. (2015). Efects of diferent tillage managements on soil physical quality in a clayey soil. Environ. Monit. Assess. 187, 1–12. doi:10.1007/s10661-014-4185-8
Seleiman, M. F., and Kheir, A. M. (2018). Saline soil properties, quality and productivity of wheat grown with bagasse ash and thiourea in different climatic zones. Chemosphere 193, 538–546. doi:10.1016/j.chemosphere.2017.11.053
Shao, G., Ai, J., Sun, Q., Hou, L., and Dong, Y. (2020). Soil quality assessment under different forest types in the Mount Tai, central Eastern China. Ecol. Indic. 115, 106439. doi:10.1016/j.ecolind.2020.106439
Smith, P., House, J. I., Bustamante, M., Sobocká, J., Harper, R., Pan, G., et al. (2016). Global change pressures on soils from land use and management. Glob. Change Biol. 22, 1008–1028. doi:10.1111/gcb.13068
Wang, J., Yao, Z., Han, J., Niu, W., and Li, Y. (2022). Different pipe burial depths associated with subsurface drip irrigation significantly affected soil gas emissions. Ann. Appl. Biol. 180 (2), 294–305. doi:10.1111/aab.12736
Xue, J., Ren, A., Li, H., Gao, Z., and Du, T. (2018). Soil physical properties response to tillage practices during summer fallow of dryland winter wheat field on the Loess Plateau. Environ. Sci. Pollut. Res. 25 (2), 1070–1078. doi:10.1007/s11356-017-0684-9
Xue, J. F., Pu, C., Zhao, X., Wei, Y. H., Zhai, Y. L., Zhang, X. Q., et al. (2018). Changes in soil organic carbon fractions in response to different tillage practices under a wheat-maize doublecropping system. Land Degrad. Dev. 29, 1555–1564. doi:10.1002/ldr.2950
Xue, J. F., Qi, Z. W., Chen, J. L., Cui, W. H., Lin, W., and Gao, Z. Q. (2023). Dynamic of soil porosity and water content under tillage during summer fallow in the dryland wheat fields of the Loess Plateau in China. Land 12 (1), 230. doi:10.3390/land12010230
Yang, H., Li, Y., Zhai, S., Fang, C., Liu, J., and Zhang, Q. (2020). Long term ditch-buried straw return affects soil fungal community structure and carbon-degrading enzymatic activities in a rice-wheat rotation system. Appl. Soil Ecol. 155, 103660. doi:10.1016/j.apsoil.2020.103660
Yuan, J., Liang, Y., Zhuo, M., Sadiq, M., Liu, L., Wu, J., et al. (2023). Soil nitrogen and carbon storages and carbon pool management index under sustainable conservation tillage strategy. Front. Ecol. Evol. 10, 1281. doi:10.3389/fevo.2022.1082624
Yuan, X., Ran, C., Gao, D., Zhao, Z., Meng, X., Geng, Y., et al. (2022). Changes in soil characteristics and rice yield under straw returning in saline sodic soils. Soil Sci. Plant Nutr. 68 (5-6), 563–573. doi:10.1080/00380768.2022.2124097
Zhang, L., Wang, J., Fu, G., and Zhao, Y. (2018). Rotary tillage in rotation with plowing tillage improves soil properties and crop yield in a wheat-maize cropping system. PLoS One 13 (6), e0198193. doi:10.1371/journal.pone.0198193
Zhang, Y., Tan, C., Wang, R., Li, J., and Wang, X. (2021). Conservation tillage rotation enhanced soil structure and soil nutrients in long-term dryland agriculture. Eur. J. Agron. 131, 126379. doi:10.1016/j.eja.2021.126379
Zhang, Y., Wang, S., Wang, H., Ning, F., Zhang, Y., Dong, Z., et al. (2018). The effects of rotating conservation tillage with conventional tillage on soil properties and grain yields in winter wheat-spring maize rotations. Agric. For. Meteorology 263, 107–117. doi:10.1016/j.agrformet.2018.08.012
Zhang, Z., Ai, N., Liu, G., Liu, C., and Qiang, F. (2021). Soil quality evaluation of various microtopography types at different restoration modes in the loess area of Northern Shaanxi. Catena 207, 105633. doi:10.1016/j.catena.2021.105633
Zhao, H., Qin, J., Gao, T., Zhang, M., Sun, H., Zhu, S., et al. (2022). Immediate and long-term effects of tillage practices with crop residue on soil water and organic carbon storage changes under a wheat-maize cropping system. Soil Tillage Res. 218, 105309. doi:10.1016/j.still.2021.105309
Zhou, C., Huang, W., Qiu, S., and Liu, Z. (2021). A quantitative study on the amount of water-retaining agent based on adhesive-modified red bed weathered soil. Bull. Eng. Geol. Environ. 80 (4), 3139–3150. doi:10.1007/s10064-021-02113-9
Keywords: conservation tillage, soil physical quality index, soil porosity, generalised soil structure index, soil three-phase structure
Citation: Fan Y, Yuan Y, Li T, Lin W, Tang X, Liang G and Li N (2024) Effects of autumn tillage with straw return on soil physical characteristics of corn fields in the eastern loess plateau. Front. Environ. Sci. 12:1362616. doi: 10.3389/fenvs.2024.1362616
Received: 28 December 2023; Accepted: 17 June 2024;
Published: 07 August 2024.
Edited by:
Lei Liu, Sichuan University, ChinaReviewed by:
Yi Wang, Institute of Earth Environment (CAS), ChinaMahmoud F. Seleiman, King Saud University, Saudi Arabia
Copyright © 2024 Fan, Yuan, Li, Lin, Tang, Liang and Li. This is an open-access article distributed under the terms of the Creative Commons Attribution License (CC BY). The use, distribution or reproduction in other forums is permitted, provided the original author(s) and the copyright owner(s) are credited and that the original publication in this journal is cited, in accordance with accepted academic practice. No use, distribution or reproduction is permitted which does not comply with these terms.
*Correspondence: Nana Li, bGluYW5hMjAwMkAxNjMuY29t
†These authors have contributed equally to this work and share first authorship