- 1Teaching and Research Department of Social and Ecological Civilization, Party School Gansu Committee of C.P.C, Lanzhou, Gansu, China
- 2Teaching and Research Department of Public Administration, Party School of Ningxia Committee of C.P.C, Yinchuan, Ningxia, China
Grazing is a main land use of natural grasslands in the world, which has both positive and negative impact on plant community structure and ecosystem functioning. However, the effects of long-term grazing management on the plant–soil system, in particular above- and belowground community characteristics, are still not well understood in alpine meadow community. In this study, we investigated the vegetation, roots, and soil properties under three management types (16 years of fencing since 2004-2020, moderate grazing and heavy grazing managements) in an alpine meadow on the Tibetan Plateau. The results showed that, compared with moderate grazing meadows, long-term fencing increased plant community cover, above- and belowground biomass, proportion of grass and litter but reduced forbs and soil bulk density, which caused the increases in soil organic carbon, total nitrogen and water content and the decreases in soil pH. However, heavy grazing led to opposite changes in proportion of grass, community biomass and soil physicochemical properties. The maximum of species richness and plant density appeared in moderate grazing meadows, supporting the intermediate disturbance hypothesis, and it can maintain above- and belowground biomass and soil physicochemical properties at medium level. Grazing increased the root: shoot ratio and caused root system shallow, which is consistent with the optimal partitioning hypothesis. Overall, our study suggested that moderate grazing is a more reasonable grazing management for sustainable development in alpine meadows of Tibetan Plateau, fencing could be an effective management strategy for vegetation restoration as well as for nutrient sequestration in degraded grasslands, but long-term fencing dose not benefit for biodiversity maintenance.
1 Introduction
Grasslands occupy about 40% of the world’s land surface, exclusive of Antarctica and Greenland, supporting the livelihoods and food security of almost a billion people (Kemp et al., 2013; Dlamini et al., 2016). However, many of these grasslands suffer degradation because of overused and mismanaged, especially in developing countries (Suttie et al., 2005; Schönbach et al., 2011; Han et al., 2018). Grassland degradation has become a major global concern in recent decades, which would affect not only livestock production and herders’ livelihood, but also vital environmental services, such as biodiversity conservation, soil carbon sequestration and hydrology (Lal et al., 2015). Up to now, about 90% of the grasslands are deemed to be degenerated state in Tibetan Plateau (Harris, 2010). Widespread grassland ecosystems are in urgent need of integrated conservation approaches to combat the land degradation in Tibetan Plateau. In order to prevent grassland deterioration, the Chinese government has implemented a series national key ecological protection engineering in the region during the last decades, such as “Retire Livestock and Restore Grassland” and “Herbage-livestock Balance,” which means that fencing to prevent grazing and moderate grazing management.
Grazing exclusion by fencing is regarded as the most economical and feasible management to increase community coverage, biodiversity and productivity (Gonzales and Clements, 2010; Armitage et al., 2012; Keesstra et al., 2016). Generally, fencing could obviously promote vegetation recovery (Holland and Detling, 1990; Su et al., 2005). However, fencing has different effects due to vegetation types, soil structure, stocking rate, temperature and rainfall (Hafner et al., 2012). For instance, Altesor et al. (2005) found that the above-ground net primary production of moderately grazing was higher than fenced grassland. Squires et al. (2010) also found fencing has negative impacts on biomass and species richness of various grasslands. Fencing cannot restore vegetation in some severely degraded regions because energy flow and material cycle of the ecosystem had been destroyed (Snyman, 2003). For soil nutrients, some research found that grazing exclusion promoted vegetation recovery, improved productivity and thereby increased soil nutrients in degenerated grasslands (Mekuria et al., 2007; Keesstra et al., 2016). However, others reported was unchanged (Pucheta et al., 2004) and even decrease in soil nutrients of grassland (Frank et al., 2002; Hafner et al., 2012). Although grazing exclusion by fencing has been widely adopting in Tibetan Plateau, more factors are needs to consider in order to protect the grassland and to maintain sustainable development, such as exclusion period (Wang et al., 2011). Some studies found that in degraded grassland of Ethiopia, herbage biomass declines or no longer increases after more than 8 years of enclosure, the period of grazing exclusion by fencing should be carefully determined (Yayneshet et al., 2009). And long-term fencing did not significantly improve the productivity of semi-arid desert grassland (Yang et al., 2005). However, we are still not very clear about the effect of long-term fencing on alpine meadow in Tibetan Plateau.
Livestock grazing is one of the most common utilization pattern of land in natural grasslands that influences plant community structure (Klein et al., 2007; Álvarez-Martínez et al., 2016; Su et al., 2017; Tarhouniet al., 2017) and soil physicochemical properties (Wan et al., 2011; Xie et al., 2014; Li et al., 2016). Meanwhile, livestock grazing was considered as one of the key disturbance factors in grassland degradation (Oesterheld and Sala, 1990). Grazing commonly can cause the replacement of palatable plants by unpalatable plants or tall-grasses by short-grasses, and reduce community cover and aboveground biomass (Schönbach et al., 2011). But, on the contrary, grazing improved aboveground biomass in Uruguay grasslands (Altesor et al., 2005). Typically, the response of plant species richness to a grazing gradient showed a hump-shaped variation (Huston and Huston, 1994; Wang and Wesche, 2016). Others, however, species richness reduced with a grazing gradient in meadow and steppe, or grazing has no significant effects on species richness in desert grassland (Gamoun, 2014). The effects of grazing on the root system mainly through altering the biomass and community structure of aboveground to further affect the growth of the belowground root system (Gao et al., 2008), belowground biomass showed complex responses (Frank et al., 2002). Soil is closely correlated with grassland productivity, soil responses to grazing disturbance and environmental changes are slower than plants (Wang and Wesche, 2016). And soil is hard to recover once degraded (Langmaack et al., 2002). Grazing affects soil physicochemical properties through biomass removal and trampling (Dlamini et al., 2016). Reasonable grazing can improve accumulation quantity of plant litter and nutrient cycling (Bardgett et al., 1998). Unreasonable grazing would increase soil bulk density, reduce permeability and aeration, thereby affecting grassland nutrient cycles, causing grassland degradation and losses of soil organic carbon (Dlamini et al., 2016; Yang et al., 2018). These results suggest that the effects of grazing pressure on aboveground and belowground ecosystems are more complex than imagination (Zolda, 2006). Thus, more and more studies have focused on the responses of both aboveground and belowground ecosystems to grazing in order to seek rational management strategies for grasslands (Conant et al., 2001; Bardgett and Wardle, 2003).
The alpine meadows of Tibetan Plateau represent the largest high-altitude pasture on the earth (Hafner et al., 2012; Li et al., 2017), which have not only nourished local people in the past hundreds of years but also have served as water filters and regulators for downstream residents (Dong et al., 2010). Because of the characteristics of high and cold, alpine meadow is more sensitive than other terrestrial ecosystems to management strategy (Klein et al., 2004; Zhao, 2011). In this study, we investigated aboveground and belowground ecological characters in response to 16 years fencing, moderate grazing and heavy grazing in alpine meadows of Tibetan Plateau. Our objective was to assess the effects of the different long-term grazing management strategies in alpine meadow, and help the government to generate a more reasonable grazing management regime for sustainable development in Tibetan Plateau. Specially, the following three questions were addressed: 1) How does grazing management affect alpine meadow community structure and function? 2) How does grazing management affect soil physicochemical characteristics? 3) How does grazing management affect the relationships between above- and belowground ecological characteristics?
Before this research, we propose the following hypothesis: 1) Fencing has significant impacts on aboveground and belowground ecological characters; 2) The highest diversity appeared in the meadows of intermediate grazing disturbance (Connell, 1978); 3) The grazing pressure led to an increase in root: shoot ratio (Chapin et al., 1987; Yang et al., 2010).
2 Materials and methods
2.1 Study area
This study was conducted in alpine meadows at 3,000 m a.s.l. in the northeast margin of the Tibetan Plateau at Zhuaxixiulong pasture (37°12′N, 102°43′E) in Gansu Province, P. R. China (Figure 1). The average daily air temperature is −0.1°C, ranging from −18.3°C in January to 12.7°C in July. The mean annual accumulated temperature is 1380°C. Total mean annual precipitation is 416 mm, which is concentrated in July, August, and September. The region has a typical continental plateau climate characterized with long and cold winter, short and mild summer, and no frost-free period. Plant growth period is 120-140d. The main soil type is alpine meadow soil. Vegetation is dominated by Kobresia humilis, Elymus nutans, Polygonum viviparum, Stipa breviflora, Artemisia smithii, Medicago archiducis-nicolai and Anaphalis lacteal.
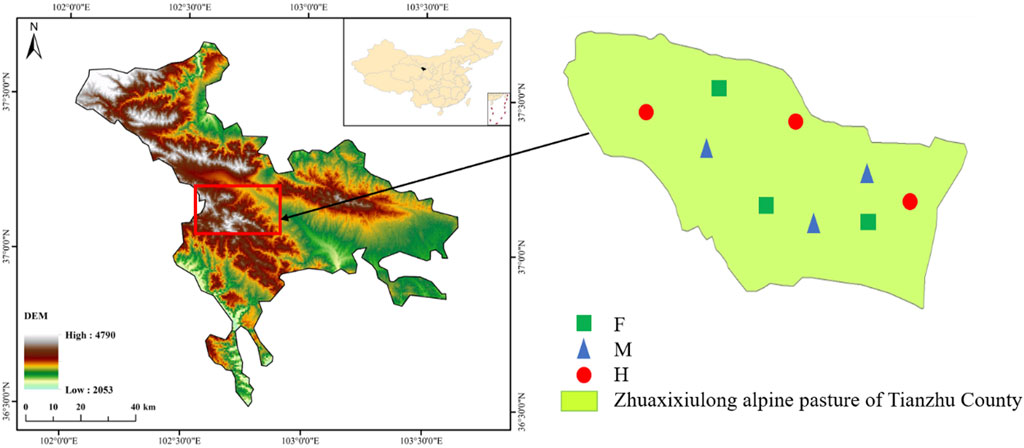
FIGURE 1. The description of the study area. The location of the study area and sampling blocks of the fenced meadows (F), moderate grazing meadows (M) and heavy grazing meadows (H) in Zhuaxixiulong alpine pasture, Tianzhu, Gansu, China. DEM denote Digital Elevation Model (m).
2.2 Experimental design, sampling and measurement
In this study, we compared three types of long-term management meadows: fenced meadows (F), moderate grazing meadows (M) and heavy grazing meadows (H), and all meadows were uniformly before the implementation of managements (Figure 1; Table 1). Each management type had three replicate blocks that was about 1 ha. The distance between the blocks is about 1–2 km. We randomly selected five 1 m × 1 m plots in every block, thus, each management type of meadow had fifteen replicate plots. In total, we surveyed 45 plots in this experiment.
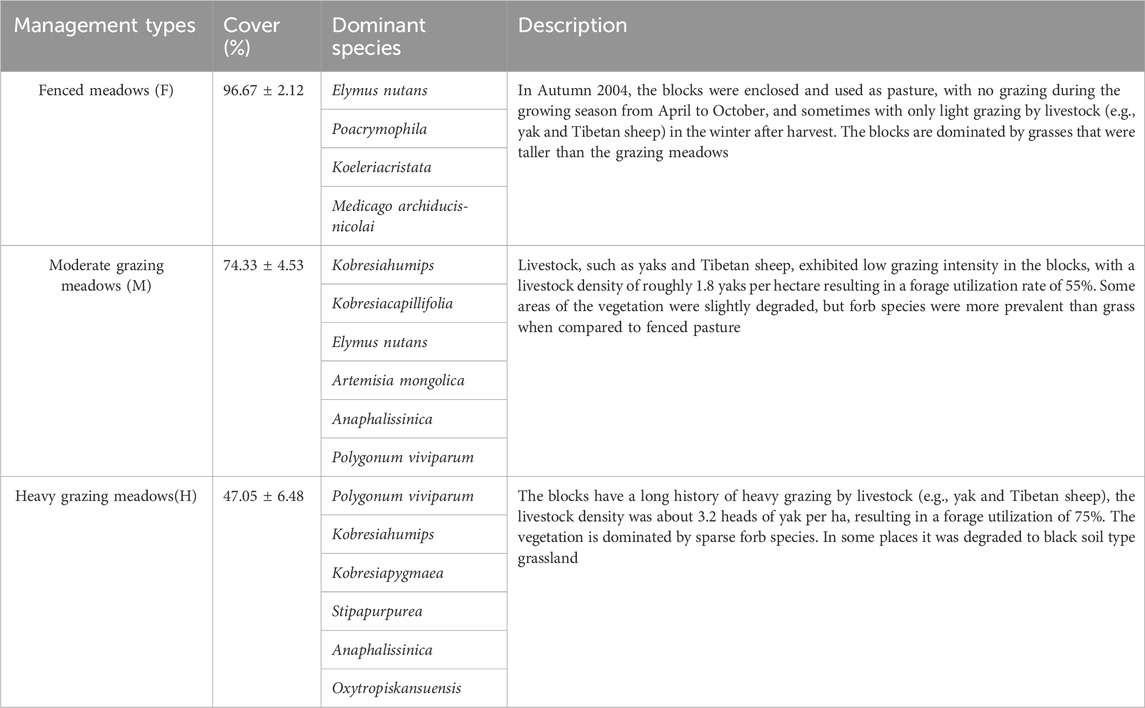
TABLE 1. Description of experimental study design. Characteristics of three grazing management’s meadows in this study. Fenced meadows (F), moderate grazing meadows (M) and heavy grazing meadows (H).
Aboveground plant community were surveyed in Mid-August of 2020. Using a 1 m × 1 m plot, we counted the number of species at the peak of the growing season (defined as species richness). The point-intercept method was used to measure species cover (%). We then measured aboveground parts of all plant individuals and surface litter in the same quadrat size and classified all species into four functional groups: grasses, sedges, forbs, and legumes, based on their functional forms (Niu et al., 2016). Meanwhile, a 10 cm diameter root auger was used to collect soil samples at different depth of 0–10 cm, 10–20 cm, and 20–30 cm for the belowground biomass measurement, and each layer had three repetitions in each plot. The layered soils from each plot were packed into a 2 mm nylon net bag and washed to separate roots and soil (Gao et al., 2008). Aboveground and belowground biomass was oven-dried at 75°C for 72 h and weighed.
We collected soil samples with a 5 cm diameter auger at different depths of 0–10 cm, 10–20 cm, and 20–30 cm were used to analyze soil properties, and each layer had three replications in each plot. All soil samples were air-dried and passed through a 5 mm mesh sieve to remove large roots and plant residues, then passed through a 0.15 mm mesh sieve. Soil pH was determined by a method of soil-water volume ratio of 1:5 (PHS-3C pH acidometer, China). Soil water content before air drying was obtained by the oven-drying method (Soil Science Society of China, Agriculture Chemistry Council, 1983). Soil bulk density at different layers was measured using the soil cores (100 cm3) by cylinders (volumetric ring method). The Kjeldahl method was used to determine soil total nitrogen (Bao, 2000). The percentage of soil organic carbon in the soil samples was measured by the Walkley–Black acid digestion method as described by Nelson and Sommers (1996). In brief, 0.5 g of soil was digested with 5 mL of 1 N K2Cr2O7 and 10 mL of concentrated sulfuric acid at 185°C for 5 min, followed by titration of the digests with standardized FeSO4. All soil sample measurements were replicated three times and operated at the Soil Testing Center at the State Key Laboratory of Grassland Agro-ecosystems, Lanzhou University, China.
2.3 Data analysis
To assess the effects of different long-term grazing managements on above-vegetation and below-ground soil properties, one-way analysis of variance (AVOVA) was performed to test differences in plant community characteristics (species richness, community coverage and density, above- and belowground biomass, litter), and soil proportion (root - shoot ratio, soil bulk density, soil water content, soil organic carbon, total nitrogen, carbon-nitrogen ratio and pH) across each soil depths (0–10 cm, 10–20 cm, and 20–30 cm)) between the fenced, moderate grazing and heavy grazing meadows. All data were expressed as mean ± standard error of mean in 15 plots. Post-hoc comparisons between grazing management types were made using the least significant difference test (LSD) (at p < 0.05). The change in each functional group (grasses, sedges, legumes and forbs) biomass were also analyzed, separately.
Moreover, we tested the correlations between the vegetation (community coverage, species richness, density, aboveground biomass, belowground biomass, root-shoot ratio and litter) and soil properties (soil bulk density, soil water content, soil organic carbon, total nitrogen, carbon-nitrogen ratio and pH) using Pearson correlation tests. All statistical tests and analyses were performed by SPSS 22.0 software (SPSS Inc., Chicago, IL, United States).
In addition, redundancy analysis (RDA) was used to explore the relationship between soil physical and chemical properties and plant community characteristics under three grazing managements. We used CANOCO 5.0 to perform RDA (University of South Bohemia, Ceske Budejovice, Czech Republic).
3 Results
3.1 Species richness and density of plant community
In total, the plant communities in the three grazing managements of 49 species, belonging to 17 families, of which 18.37% were annuals and 81.63% were perennials (Supplementary Table S1). Compared with the moderate grazing meadow, species richness (F = 41.12, p < 0.001) and plant density (F = 9.73, p < 0.01) significant lower in fenced meadows, and significant lower (F = 65.99, p < 0.001; F = 33.77, p < 0.001) in heavy grazing meadows, however, plant community cover increased in fenced plots (F = 179.56, p < 0.001) and decreased in high grazing plots (F = 107.58, p < 0.001) significantly (Table1; Figure 2). The highest of species richness and plant density appeared in moderate grazing meadows was about 28.89 number m-2 and 949.44 individuals m−2. On average, species richness and plant density in fenced meadow decreased by 17.31% and 20.57%, and by 22.71%, 31.73% in heavy grazing meadow. Additionally, community coverage increased by 30.06% in fenced meadow and decreased by 36.77% in heavy grazing meadow compared to moderate grazing meadow (Table 1; Figure 2).
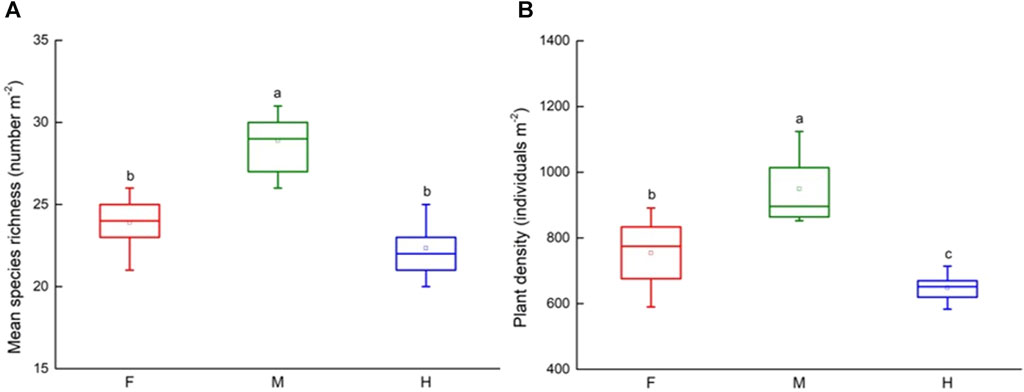
FIGURE 2. Change in species richness and density under grazing management. Variation in (A) species richness and (B) plant density among three grazing managements (F: fenced meadow; M: moderate grazing meadow; H: heavy grazing meadow). Error bars express standard error of the mean (n = 15), different letters indicate significant differences at p < 0.05.
3.2 Above- and belowground biomass and litter
Aboveground biomass differed significantly among three grazing managements (F = 624.19, p < 0.001). The total aboveground biomass of the long-term fenced meadow, moderate grazing meadow and heavy grazing meadow were 698.21 ± 44.44 g m−2, 481.46 ± 33.37 g m−2 and 120.63 ± 24.37 g m−2, respectively (Figure 3A). Compared with moderate grazing areas, fencing significantly increased biomass of grass (F = 273.86, p < 0.001) and sedge (F = 43.59, p < 0.001), but the differences of legumes (F = 0.85, p > 0.05) and forbs (F = 1.67, p > 0.05) biomass were not significant, heavy grazing significantly decreased grasses (F = 287.11, p < 0.001), sedges (F = 198.81, p < 0.001), legumes (F = 58.28, p < 0.001) and forbs (F = 394.30, p < 0.001) biomass. Fencing increased grass, but decreased forbs, for grazing this was reversed (Figure 3A).
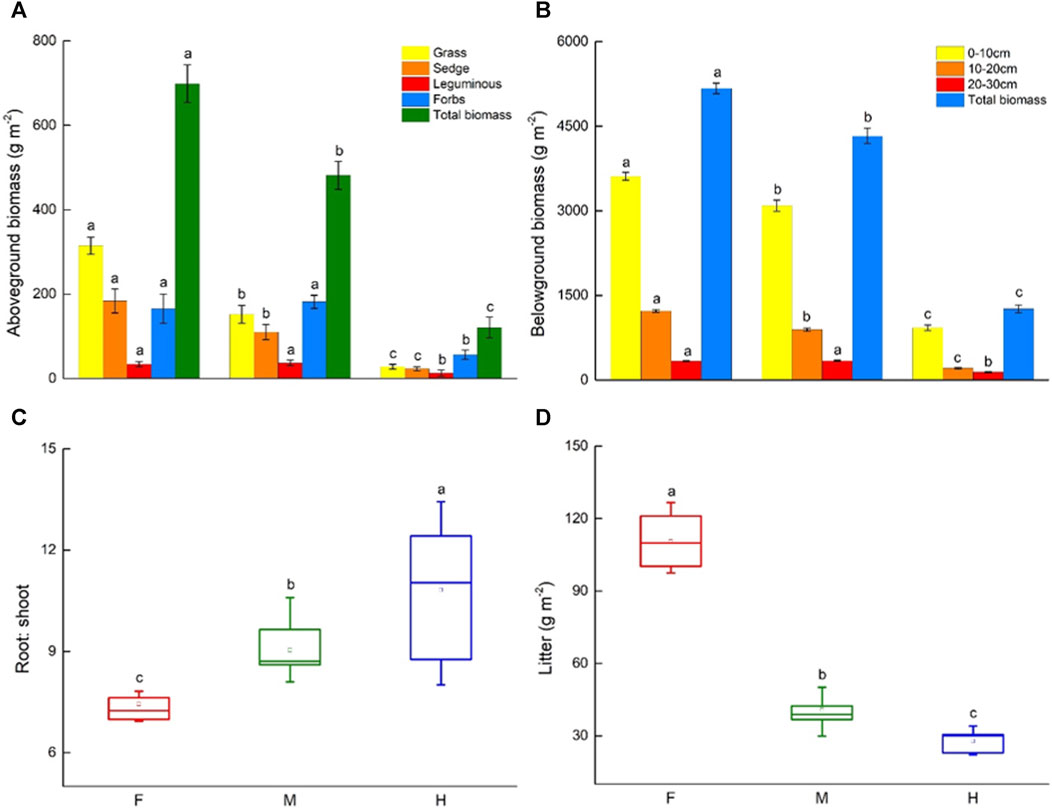
FIGURE 3. Change in biomass, root:shoot and litter under grazing management. (A) Aboveground biomass (four functional groups: grasses, sedges, legumes, forbs, and total aboveground biomass), (B) belowground biomass (in three soil layers: 0–10 cm, 10–20 cm and 20–30 cm, and total belowground biomass in the top 30 cm of soil), (C) root: shoot ratios and (D) litter among three grazing managements (F: fenced meadows; M: moderate grazing meadows; H: heavy grazing meadows). Error bars express standard error of the mean (n = 15), different letters indicate significant differences at p < 0.05.
Long-term grazing management has a significant effect on belowground biomass in 0–30 cm soil profile (F = 3,565.14, p < 0.001). The highest belowground biomass was found in fenced meadow (5170.44 ± 94.06 g m−2), and then in moderate (4326.03 ± 136.39 g m-2), and in heavy grazing (1260.70 ± 67.87 g m−2), respectively (Figure 3B). Compared with moderate grazing meadow, fencing meadow significantly increased belowground biomass both in 0–10 cm (F = 169.06, p < 0.001) and 10–20 cm (F = 763.35, p < 0.001), however, the differences of 20–30 cm soil profile were not significant (F = 2.36, p > 0.05), and heavy grazing significantly decreased belowground biomass of 0–10 cm (F = 3,517.32, p < 0.001), 10–20 cm (F = 4590.31, p < 0.001) and 20–30 cm (F = 2184.33, p < 0.01) soil profile (Figure 3B). Belowground biomass of the 0–10 cm soil profile accounted for about 69.87%, 71.48%, and 74.15% of total belowground biomass of the 0–30 cm in fenced, moderate grazing and heavy grazing meadows, respectively.
In addition, fencing significantly decreased the root: shoot ratios by 17.61% (F = 22.37, p < 0.05) and increased litter by 171.07% (F = 234.64, p < 0.001) compared to moderate grazing. In contrast, heavy grazing significantly increased the root: shoot ratios by 19.82% (F = 7.07, p < 0.05) and decreased litter by 31.86% (F = 19.41, p < 0.01) (Figures 3C, D).
3.3 Soil physicochemical properties
Overall, long-term grazing management had significant impact on soil bulk density, water content, organic carbon, total nitrogen and pH of 0–10 cm (F = 52.95, p < 0.001; F = 30.79, p < 0.001; F = 48.94, p < 0.001; F = 22.06, p < 0.001; F = 16.20, p < 0.001) and 10–20 cm (F = 20.57, p < 0.001; F = 21.96, p < 0.001; F = 31.65, p < 0.001; F = 24.92, p < 0.001; F = 20.41, p < 0.001) soil layers, and no significant impact on soil physicochemical properties of 20–30 cm soil layer (F = 0.06, p > 0.05; F = 1.73, p > 0.05; F = 0.44, p > 0.05; F = 2.39, p > 0.05) except pH (F = 22.25, p < 0.001) (Figure 4). Soil organic carbon and total nitrogen decrease with the depth of the soil increase. However, the differences of carbon-nitrogen ratios were not significant in each soil layer (F = 3.05, p > 0.05; F = 2.01, p > 0.05; F = 0.001, p > 0.05) (Figure 4). Compared with the moderate grazing plots, long-term fencing grazing significantly reduced soil bulk density by 30.18% and 20.0% in 0–10 cm and 10–20 cm layers, respectively, pH by 6.37% and 3.79%, while significantly increased soil water content by 31.37% and 12.16%, organic carbon by 16.51% and 30.67%, total nitrogen by 13.26% and 15.24%. Conversely, heavy grazing significantly increased soil bulk density by 5.66% and 3.64%, increased soil pH by 5.34% and 6.11%, while significantly decreased soil water content by 7.22% and 14.38%, organic carbon by 14.36% and 15.96%, total nitrogen by 7.18% and 9.87%, in soil layers of 0–10 cm and 10–20 cm, respectively (Figure 4).
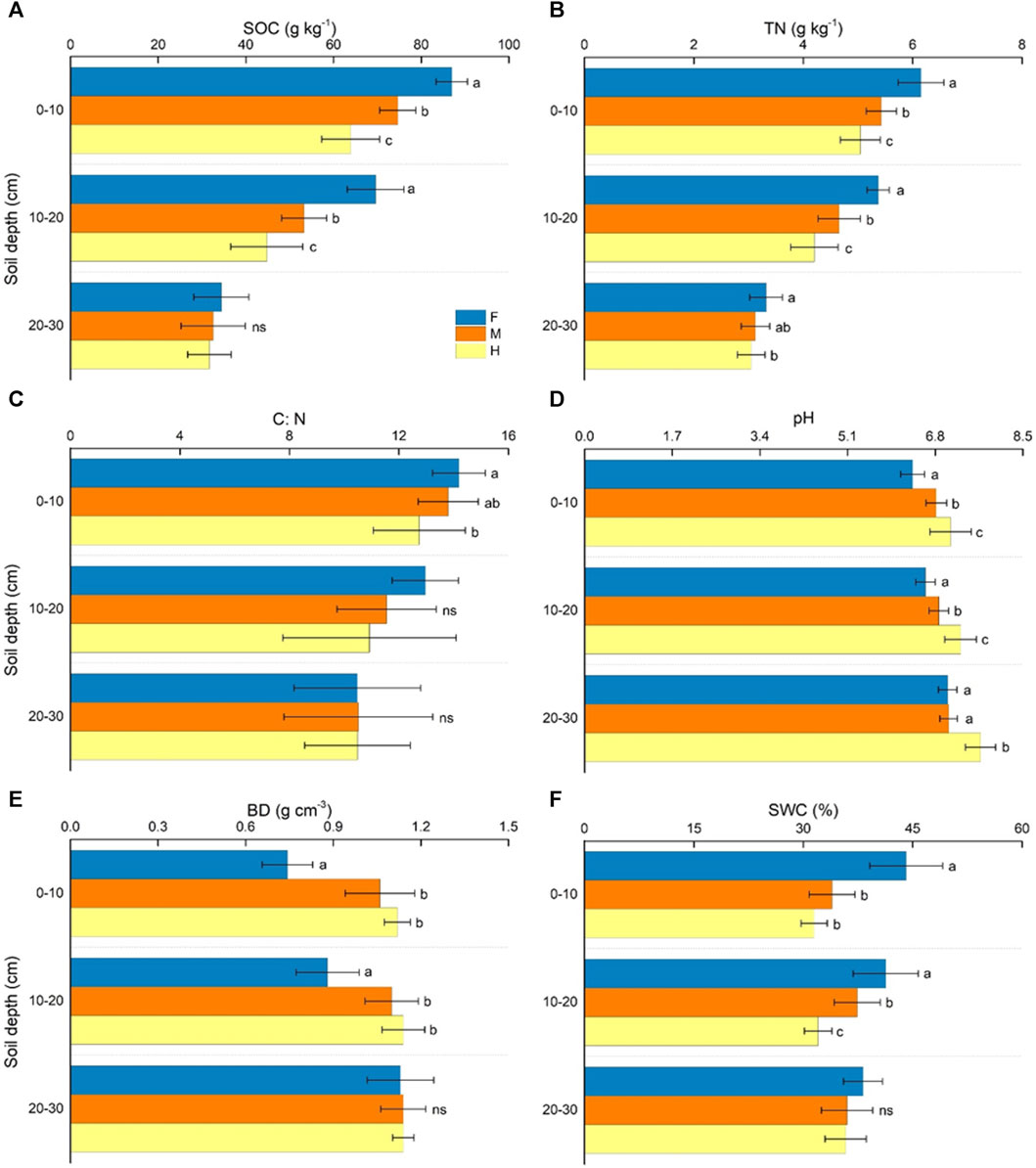
FIGURE 4. Change in soil properties across soil depths. Soil physicochemical properties in three soil layers (0–10 cm, 10–20 cm and 20–30 cm) at the three grazing management types (F: fenced meadows; M: moderate grazing meadows; H; heavy grazing meadows). (A) Soil carbon content (SOC), (B) total nitrogen (TN), (C) carbon: nitrogen ratios (C: N), (D) soil pH, (E) bulk density (BD), (F) soil water content (SWC). Error bars express standard error of the mean (n = 15), different letters indicate significant differences at p < 0.05, ns means no significant difference.
3.4 Relationships between plant community characteristics and soil properties
Axes 1 and Axes 2 in the results of redundancy analysis (RDA) explained 70.85% and 3.10% of the total variations, respectively (Figure 5). In term of Pearson correlation analysis, the results showed that community coverage, above- and belowground biomass, and litter of alpine meadows were significant positively related to soil organic carbon, total nitrogen and water content, and significant negatively related to soil pH and bulk density. There were significant positive correlations among soil organic carbon, total nitrogen and water content. The soil pH and bulk density showed the negative correlations with the soil organic carbon, total nitrogen and water content. Additionally, there were significantly positive correlations among plant community coverage, above- and belowground biomass and litter, but, plant community coverage were not significant related to species richness and plant density. Overall, fencing was positively associated with soil organic carbon, total nitrogen, vegetation coverage, soil water content, above- and belowground biomass; moderate grazing had positive correlation to species richness and plant density; and heavy grazing had positive relationship with soil pH, bulk density and root: shoot ratios (Figure 5; Supplementary Table S2).
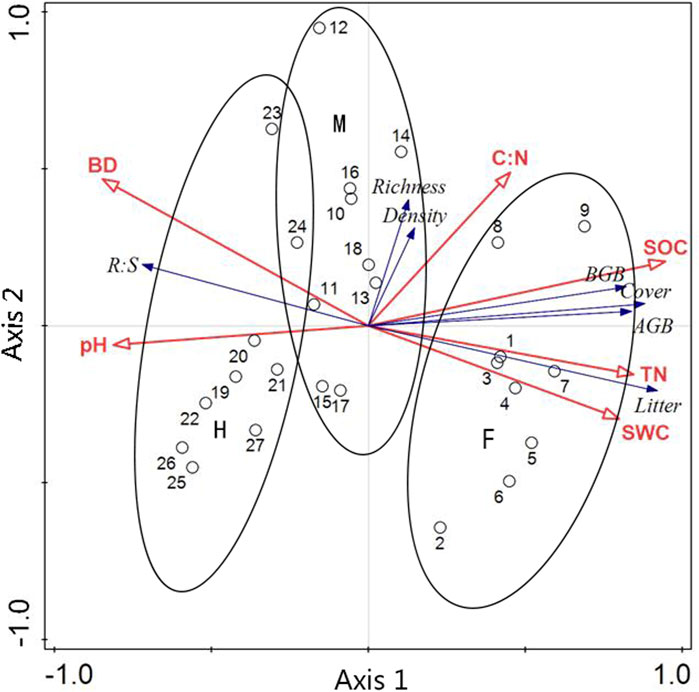
FIGURE 5. The RDA relationship between plant community and soil properties. Redundancy analysis (RDA) of plant community characteristics and soil environmental properties (0–10 cm) in three grazing managements. AGB, aboveground biomass; BGB, belowground biomass; R: S, root: shoot ratios; SOC, soil carbon content; TN, total nitrogen content; C: N, carbon: nitrogen ratios; BD, bulk density; SWC, soil water content. Different numbers represent the different plots in each meadow/treatment. F: fenced meadows; M: moderate grazing meadows; H: heavy grazing meadows.
4 Discussion
4.1 Change in plant community under grazing management
It has been well demonstrated that grazing is a major factor influencing plant community composition and ecosystem functioning in grasslands, such as reduces in plant community cover, above- and belowground biomass (Diaz et al., 2007; Liu et al., 2017). However, there were also other research suggested that the highest community biomass at intermediate grazing condition because of compensatory growth, the interrelation of biomass and grazing intensity is hump-shaped (Patton et al., 2007; Dangal et al., 2016). In this study, long-term fencing caused species composition shifts at community level, increased taller plants with stronger and denser root system (e.g., Elymus dahuricus and Carex capillifolia), which resulted in the highest above- and belowground biomass production. However, we detected a hump-shaped curve for species richness and plant density along the grazing intensity gradient (Figure 2), which was consistent with most previous studies on grazing, generally, low-intensity grazing increases, while high-intensity grazing decreases species richness and plant density (Niedrist et al., 2009; Metera et al., 2010; Niu et al., 2016). These findings fitted well with the hypothesis of intermediate disturbance, which assumes that the highest species diversity occurs at an intermediate level of disturbance (Connell, 1978).
We found an increasing of root: shoot ratios from fenced towards heavily grazed grassland, which is consistent with the previous studies (Pucheta et al., 2004). According to the optimal partitioning hypothesis, plants respond to environmental factors changes by allocating biomass among all organs to acquire water, nutrients and light to achieve the maximum relative growth rate (Chapin et al., 1987; Yang et al., 2010). Generally, plants distribute more biomass to roots in low-nutrient or low-moisture habitats and shift more biomass to shoots in high-nutrient or high-moisture habitats, more belowground biomass will contribute to assimilate nutrient or water at humid sites and dry sites (Yang et al., 2010). Thus, the decreasing of nutrient and moisture induced an increasing of root: shoot ratios from fenced towards heavily grazed meadow in the study. Moreover, the aboveground biomass should be returned to the soils to avoid soil degradation caused by grazing pressure is another reason (Baudron et al., 2014). In addition, we found that long-term heavily intensified grazing increased the proportion of shallow root, which is because the root standing crop is decided by the balance of production and mortality (Phillips et al., 2006; Bai et al., 2015). The impacts of grazing on root biomass could by its effects on root production and mortality, thus, the proportion of shallow root under grazing was higher than that in fenced meadows.
4.2 Change in soil properties under grazing management
Soil properties respond more slowly than plant community properties (cover, diversity, density) and above- and belowground biomass to grazing, thus are usually considered as credible indicators of grazing management (Wang and Wesche, 2016). Our results showed that 16 years of grazing exclusion by fencing positively improved soil organic carbon, total nitrogen and water content, reduced soil pH and bulk density at the 0–10 cm and 10–20 cm soil layer, compared with the moderate grazing, and the results of continuous heavy grazing were opposite. This is because fencing promoted the growth and development of perennial and annual grasses with stronger and denser root systems, which input more carbon into soil and accumulate them, while grazing has decreased this process (Reedera and Schumanb, 2002; Li et al., 2013). Similarly, some researches indicated that fencing can result in a decrease in pH value by accumulating humic acid while the effect of grazing was opposite (Abakumov et al., 2013). And long-term fencing can decrease the outflow of nutrient and energy from soil to plant to livestock, which would lock the abundant nutrients within plants tissues (Harris et al., 2007). Moreover, we found that long-term fencing significantly increased litter layer, which could be another reason to increase soil nutrients. Most litter produced by grasses and accompanied by faster litter decomposition rates in the moister, less dense and more acidic soil of enclosed meadow (Geissen and Guzman, 2006; Pintaldi et al., 2016). In the study, we found that fencing has limited trampling by livestock resulting in obvious decrease in soil bulk density and interception of surface water, however, the effect of heavy grazing exactly contrariwise. Previous studies reported that trampling is a direct effect of livestock grazing on grassland ecosystem, which can cause changes of soil physicochemical properties (Bai et al., 2015). Particularly, heavy intensity grazing led to soil bulk density and sands to increase, and soil nutrients and water contents to reduce (Holt, 1997; Bilotta et al., 2007). Our results also revealed that, soil properties variation in 20–30 cm soil layer were almost insignificant, which maybe because the root distribution was not significant in the soil layer, and most root system was distributed in shallow soil layer which cannot allocate or ingest nutrients in deeper soil (Harris et al., 2007; Bai et al., 2015).
4.3 Mechanistic links between plant community and soil properties under grazing management
Soil is a foundation for the grassland ecosystem. In this study, we found that plant community cover had significantly positive correlation with soil nutrients under grazing management, because the decrease in vegetation cover may speed up soil erosion and increase soil nutrients losses (Wang et al., 2003). Meanwhile, above- and belowground biomass was both significantly positive correlated to soil organic carbon, total nitrogen and water content, and negative correlated with soil pH and bulk density in the study. Fencing can decrease the output of nutrient and energy from soil-vegetation systems to livestock, and which were locked in plant shoot (Harris et al., 2007), then returned to the plant roots (Bardgett and Wardle, 2003; Baudron et al., 2014). Plant root system plays the primary role in regulating the carbon- and nutrient-cycling in the soil. Grazing management changed the aboveground productivity and community compositions to further affect the roots growth (Gao et al., 2008), afterwards, plant root input abundant organic carbon into soil (McCormack et al., 2014), as demonstrated by the positive relation between soil organic carbon and belowground biomass. And the decrease of soil total nitrogen could be due to grazing management, livestock got nutrients across grasslands but release most them in camps (Holst et al., 2007). The reduction of livestock trampling and the increases of underground roots both resulted in improvements of soil texture and bulk density, and increased soil moisture infiltration rate (Bai et al., 2015). Increased bulk density might lead to further loss of nutrients, as shown, the negative correlations between soil bulk density and both soil organic carbon and total nitrogen, especially in humid areas (Bai et al., 2015). The response of soil pH to grazing pressure was positively in most cases, which could be caused by urine deposition (Milchunas and Lauenroth, 1993). In addition, Angers and Caron (1998) indicated that plant roots can improve then on capillary porosity of the soil and promote the formation of water-stable. The study showed that the effects of nitrogen enrichment may be caused by accumulated plant litter (Foster and Gross, 1998). Moreover, besides taller and denser grasss provide shade to forbs and restricts the competition of forbs to light, the thick plant litter was another reason for reduced species richness by inhibiting the establishment of forb seedlings in fenced meadows (Loydi et al., 2013; Borer et al., 2014).
5 Conclusion
In summary, compared with moderate grazing, we conclude that long-term grazing exclusion had a positive effect on vegetation restoration as well as nutrient sequestration and grass but had a negative effect on species richness and forb functional group proportion in alpine meadow ecosystem. However, long-term heavy grazing not only decreased community cover, above- and belowground biomass, grass, litter, soil organic carbon and total nitrogen, but also increased the root-shoot ratio and caused root system move to soil shallow layer. Moderate grazing benefit biodiversity conservation and can maintain biomass and soil physicochemical properties at medium level. Therefore, our study indicated that, regardless of long-term fencing or heavy grazing, both of management types have a negative impact on plant-soil system in alpine meadows. In contrast, moderate grazing should be advocated by government agencies and the restoration ecologists. In the future, we hope that our work will provide an instructive advice for the implementation of local government policies in the alpine region of the Tibetan Plateau.
Data availability statement
The raw data supporting the conclusion of this article will be made available by the authors, without undue reservation.
Author contributions
DW: Conceptualization, Formal Analysis, Funding acquisition, Investigation, Methodology, Writing–original draft. WD: Conceptualization, Formal Analysis, Writing–review and editing.
Funding
The author(s) declare financial support was received for the research, authorship, and/or publication of this article. Soft Science Special Fund of Gansu Province, China, Grant/Award Number: 21CX6ZA052.
Acknowledgments
We thank all the field investigators; and Dr. Zhengwei Ren for their constructive suggestions.
Conflict of interest
The authors declare that the research was conducted in the absence of any commercial or financial relationships that could be construed as a potential conflict of interest.
Publisher’s note
All claims expressed in this article are solely those of the authors and do not necessarily represent those of their affiliated organizations, or those of the publisher, the editors and the reviewers. Any product that may be evaluated in this article, or claim that may be made by its manufacturer, is not guaranteed or endorsed by the publisher.
Supplementary material
The Supplementary Material for this article can be found online at: https://www.frontiersin.org/articles/10.3389/fenvs.2024.1348220/full#supplementary-material
References
Abakumov, E. V., Cajthaml, T., Brus, J., and Frouz, J. (2013). Humus accumulation, humification, and humic acid composition in soils of two post-mining chronosequences after coal mining. J. Soils Sediments 13 (3), 491–500. doi:10.1007/s11368-012-0579-9
Altesor, A., Oesterheld, M., Leoni, E., Lezama, F., and Rodríguez, C. (2005). Effect of grazing on community structure and productivity of a Uruguayan grassland. Plant Ecol. 179 (1), 83–91. doi:10.1007/s11258-004-5800-5
Álvarez-Martínez, J., Gómez-Villar, A., and Lasanta, T. (2016). The use of goats grazing to restore pastures invaded by shrubs and avoid desertification: a preliminary case study in the Spanish Cantabrian Mountains. Land Degrad. Dev. 27, 3–13. doi:10.1002/ldr.2230
Angers, D. A., and Caron, J. (1998). Plant-induced changes in soil structure: processes and feedbacks. Biogeochemistry 42 (1-2), 55–72. doi:10.1023/a:1005944025343
Armitage, H. F., Britton, A. J., van der Wal, R., and Woodin, S. J. (2012). Grazing exclusion and phosphorus addition as potential local management options for the restoration of alpine moss-sedge heath. Biol. Conserv. 153, 17–24. doi:10.1016/j.biocon.2012.04.025
Bai, W., Fang, Y., Zhou, M., Xie, T., Li, L., and Zhang, W. H. (2015). Heavily intensified grazing reduces root production in an Inner Mongolia temperate steppe. Agric. Ecosyst. Environ. 200, 143–150. doi:10.1016/j.agee.2014.11.015
Bardgett, R. D., and Wardle, D. A. (2003). Herbivore-mediated linkages between aboveground and belowground communities. Ecology 84 (9), 2258–2268. doi:10.1890/02-0274
Bardgett, R. D., Wardle, D. A., and Yeates, G. W. (1998). Linking above-ground and below-ground interactions: how plant responses to foliar herbivory influence soil organisms. Soil Biol. Biochem. 30 (14), 1867–1878. doi:10.1016/s0038-0717(98)00069-8
Baudron, F., Jaleta, M., Okitoi, O., and Tegegn, A. (2014). Conservation agriculture in African mixed crop-livestock systems: expanding the niche. Agric. Ecosyst. Environ. 187 (1), 171–182. doi:10.1016/j.agee.2013.08.020
Bilotta, G. S., Brazier, R. E., and Haygarth, P. M. (2007). The impacts of grazing animals on the quality of soils, vegetation, and surface waters in intensively managed grasslands. Adv. Agron. 94, 237–280. doi:10.1016/s0065-2113(06)94006-1
Borer, E. T., Seabloom, E. W., Gruner, D. S., Harpole, W. S., Hillebrand, H., Lind, E. M., et al. (2014). Herbivores and nutrients control grassland plant diversity via light limitation. Nature 508 (7497), 517–520. doi:10.1038/nature13144
Chapin, F. S., Bloom, A. J., Field, C. B., and Waring, R. H. (1987). Plant responses to multiple environmental factors. Bioscience 37 (1), 49–57. doi:10.2307/1310177
Conant, R. T., Paustian, K., and Elliott, E. T. (2001). Grassland management and conversion into grassland: effects on soil carbon. Ecol. Appl. 11 (2), 343–355. doi:10.1890/1051-0761(2001)011[0343:gmacig]2.0.co;2
Connell, J. H. (1978). Diversity in tropical rain forests and coral reefs. Science 199 (4335), 1302–1310. doi:10.1126/science.199.4335.1302
Dangal, S. R., Tian, H., Lu, C., Pan, S., Pederson, N., and Hessl, A. (2016). Synergistic effects of climate change and grazing on net primary production of Mongolian grasslands. Ecosphere 7 (5), e01274. doi:10.1002/ecs2.1274
Diaz, S., Lavorel, S., McIntyre, S. U. E., Falczuk, V., Casanoves, F., Milchunas, D. G., et al. (2007). Plant trait responses to grazing–a global synthesis. Glob. Change Biol. 13 (2), 313–341. doi:10.1111/j.1365-2486.2006.01288.x
Dlamini, P., Chivenge, P., and Chaplot, V. (2016). Overgrazing decreases soil organic carbon stocks the most under dry climates and low soil pH: a meta-analysis shows. Agric. Ecosyst. Environ. 221, 258–269. doi:10.1016/j.agee.2016.01.026
Dong, S. K., Li, J. P., Li, X. Y., Wen, L., Zhu, L., Li, Y. Y., and Wang, Y. L. (2010). Application of design theory for restoring the black beach degraded rangeland at the headwater areas of the Qinghai-Tibetan Plateau. Afr. J. Agric. Res. 5 (25), 3542–3552. doi:10.5897/AJAR10.005
Foster, B. L., and Gross, K. L. (1998). Species richness in a successional grassland: effects of nitrogen enrichment and plant litter. Ecology 79 (8), 2593–2602. doi:10.1890/0012-9658(1998)079[2593:sriasg]2.0.co;2
Frank, D. A., Kuns, M. M., and Guido, D. R. (2002). Consumer control of grassland plant production. Ecology 83, 602–606. doi:10.1890/0012-9658(2002)083[0602:ccogpp]2.0.co;2
Gamoun, M. (2014). Grazing intensity effects on the vegetation in desert rangelands of Southern Tunisia. J. Arid Land 6, 324–333. doi:10.1007/s40333-013-0202-y
Gao, Y. Z., Giese, M., Lin, S., Sattelmacher, B., Zhao, Y., and Brueck, H. (2008). Belowground net primary productivity and biomass allocation of a grassland in Inner Mongolia is affected by grazing intensity. Plant Soil 307 (1-2), 41–50. doi:10.1007/s11104-008-9579-3
Geissen, V., and Guzman, G. M. (2006). Fertility of tropical soils under different land use systems—a case study of soils in Tabasco, Mexico. Appl. Soil Ecol. 31 (1-2), 169–178. doi:10.1016/j.apsoil.2005.02.012
Gonzales, E. K., and Clements, D. R. (2010). Plant community biomass shifts in response to mowing and fencing in invaded oak meadows with non-native grasses and abundant ungulates. Restor. Ecol. 18 (5), 753–761. doi:10.1111/j.1526-100x.2009.00535.x
Hafner, S., Unteregelsbacher, S., Seeber, E., Lena, B., Xu, X., Li, X., et al. (2012). Effect of grazing on carbon stocks and assimilate partitioning in a T ibetan montane pasture revealed by 13 CO2 pulse labeling. Glob. Change Biol. 18 (2), 528–538. doi:10.1111/j.1365-2486.2011.02557.x
Han, D., Wang, G., Xue, B., Liu, T., Yinglan, A., and Xu, X. (2018). Evaluation of semiarid grassland degradation in North China from multiple perspectives. Ecol. Eng. 112, 41–50. doi:10.1016/j.ecoleng.2017.12.011
Harris, R. B. (2010). Rangeland degradation on the Qinghai-Tibetan plateau: a review of the evidence of its magnitude and causes. J. Arid Environ. 74, 1–12. doi:10.1016/j.jaridenv.2009.06.014
Harris, W. N., Moretto, A. S., Distel, R. A., Boutton, T. W., and Boo, R. M. (2007). Fire and grazing in grasslands of the Argentine Caldenal: effects on plant and soil carbon and nitrogen. acta Oecol. 32 (2), 207–214. doi:10.1016/j.actao.2007.05.001
Holland, E. A., and Detling, J. K. (1990). Plant response to herbivory and belowground nitrogen cycling. Ecology 71 (3), 1040–1049. doi:10.2307/1937372
Holst, J., Liu, C., Yao, Z., Brüggemann, N., Zheng, X., Han, X., et al. (2007). Importance of point sources on regional nitrous oxide fluxes in semi-arid steppe of Inner Mongolia, China. Plant soil 296 (1-2), 209–226. doi:10.1007/s11104-007-9311-8
Holt, J. A. (1997). Grazing pressure and soil carbon, microbial biomass and enzyme activities in semi-arid northeastern Australia. Appl. Soil Ecol. 5 (2), 143–149. doi:10.1016/s0929-1393(96)00145-x
Huston, M. A., and Huston, M. A. (1994). Biological diversity: the coexistence of species. United Kingdom: Cambridge University Press.
Keesstra, S., Pereira, P., Novara, A., Brevik, E. C., Azorin-Molina, C., Parras-Alcántara, L., et al. (2016). Effects of soil management techniques on soil water erosion in apricot orchards. Sci. Total Environ. 551, 357–366. doi:10.1016/j.scitotenv.2016.01.182
Kemp, D. R., Guodong, H., Xiangyang, H., Michalk, D. L., Fujiang, H., Jianping, W., et al. (2013). Innovative grassland management systems for environmental and livelihood benefits. Proc. Natl. Acad. Sci. 110 (21), 8369–8374. doi:10.1073/pnas.1208063110
Klein, J. A., Harte, J., and Zhao, X. Q. (2004). Experimental warming causes large and rapid species loss, dampened by simulated grazing, on the Tibetan Plateau. Ecol. Lett. 7 (12), 1170–1179. doi:10.1111/j.1461-0248.2004.00677.x
Klein, J. A., Harte, J., and Zhao, X. Q. (2007). Experimental warming, not grazing, decreases rangeland quality on the Tibetan plateau. Ecol. Appl. 17, 541–557. doi:10.1890/05-0685
Lal, R., Negassa, W., and Lorenz, K. (2015). Carbon sequestration in soil. Curr. Opin. Environ. Sustain. 15, 79–86. doi:10.1016/j.cosust.2015.09.002
Langmaack, M., Schrader, S., Rapp-Bernhardt, U., and Kotzke, K. (2002). Soil structure rehabilitation of arable soil degraded by compaction. Geoderma 105 (1-2), 141–152. doi:10.1016/s0016-7061(01)00097-0
Li, W., Xu, F., Zheng, S., Taube, F., and Bai, Y. (2017). Patterns and thresholds of grazing-induced changes in community structure and ecosystem functioning: species-level responses and the critical role of species traits. J. Appl. Ecol. 54 (3), 963–975. doi:10.1111/1365-2664.12806
Li, W., Zhao, J., Epstein, H. E., Jing, G., Cheng, J., and Du, G. (2016). Community-level trait responses and intra-specific trait variability play important roles in driving community productivity in an alpine meadow on the Tibetan Plateau. J. Plant Ecol. 10 (4), 592–600. doi:10.1093/jpe/rtw069
Li, Y., Dong, S., Wen, L., Wang, X., and Wu, Y. (2013). The effects of fencing on carbon stocks in the degraded alpine grasslands of the Qinghai-Tibetan Plateau. J. Environ. Manag. 128, 393–399. doi:10.1016/j.jenvman.2013.05.058
Liu, J., Wu, J., Su, H., Gao, Z., and Wu, Z. (2017). Effects of grazing exclusion in Xilin Gol grassland differ between regions. Ecol. Eng. 99, 271–281. doi:10.1016/j.ecoleng.2016.11.041
Loydi, A., Eckstein, R. L., Otte, A., and Donath, T. W. (2013). Effects of litter on seedling establishment in natural and semi-natural grasslands: a meta-analysis. J. Ecol. 101 (2), 454–464. doi:10.1111/1365-2745.12033
Mccormack, M. L., Adams, T. S., Smithwick, E. A., and Eissenstat, D. M. (2014). Variability in root production, phenology, and turnover rate among 12 temperate tree species. Ecology 95 (8), 2224–2235. doi:10.1890/13-1942.1
Mekuria, W., Veldkamp, E., Haile, M., Nyssen, J., Muys, B., and Gebrehiwot, K. (2007). Effectiveness of exclosures to restore degraded soils as a result of overgrazing in tigray, Ethiopia. J. Arid Environ. 69 (2), 270–284. doi:10.1016/j.jaridenv.2006.10.009
Metera, E., Sakowski, T., Słoniewski, K., and Romanowicz, B. (2010). Grazing as a tool to maintain biodiversity of grassland-a review. Animal Sci. Pap. Rep. 28 (4), 315–334.
Milchunas, D. G., and Lauenroth, W. K. (1993). Quantitative effects of grazing on vegetation and soils over a global range of environments: ecological archives m063-001. Ecol. Monogr. 63 (4), 327–366. doi:10.2307/2937150
Nelson, D. W., and Sommers, L. E. (1996). Total carbon, organic carbon, and organic matter. Methods soil analysis part 3-chemical methods 1996, 961–1010. doi:10.2136/sssabookser5.3.c34
Niedrist, G., Tasser, E., Lüth, C., Dalla Via, J., and Tappeiner, U. (2009). Plant diversity declines with recent land use changes in European Alps. Plant Ecol. 202 (2), 195–210. doi:10.1007/s11258-008-9487-x
Niu, K., He, J. S., Zhang, S., and Lechowicz, M. J. (2016). Grazing increases functional richness but not functional divergence in Tibetan alpine meadow plant communities. Biodivers. Conservation 25 (12), 2441–2452. doi:10.1007/s10531-015-0960-2
Oesterheld, M., and Sala, O. E. (1990). Effects of grazing on seedling establishment: the role of seed and safe-site availability. J. Veg. Sci. 1 (3), 353–358. doi:10.2307/3235711
Patton, B. D., Dong, X., Nyren, P. E., and Nyren, A. (2007). Effects of grazing intensity, precipitation, and temperature on forage production. Rangel. Ecol. Manag. 60 (6), 656–665. doi:10.2111/07-008r2.1
Phillips, D. L., Johnson, M. G., Tingey, D. T., Storm, M. J., Ball, J. T., and Johnson, D. W. (2006). CO2 and N-fertilization effects on fine-root length, production, and mortality: a 4-year ponderosa pine study. Oecologia 148 (3), 517–525. doi:10.1007/s00442-006-0392-5
Pintaldi, E., D’Amico, M. E., Siniscalco, C., Cremonese, E., Celi, L., Filippa, G., et al. (2016). Hummocks affect soil properties and soil-vegetation relationships in a subalpine grassland (North-Western Italian Alps). Catena 145, 214–226. doi:10.1016/j.catena.2016.06.014
Pucheta, E., Bonamici, I., Cabido, M., and Diaz, S. (2004). Below-ground biomass and productivity of a grazed site and a neighbouring ungrazed exclosure in a grassland in central Argentina. Austral Ecol. 29 (2), 201–208. doi:10.1111/j.1442-9993.2004.01337.x
Reeder, J. D., and Schuman, G. E. (2002). Influence of livestock grazing on C sequestration in semi-arid mixed-grass and short-grass rangelands. Environ. Pollut. 116 (3), 457–463. doi:10.1016/s0269-7491(01)00223-8
Schönbach, P., Wan, H., Gierus, M., Bai, Y., Müller, K., Lin, L., et al. (2011). Grassland responses to grazing: effects of grazing intensity and management system in an Inner Mongolian steppe ecosystem. Plant Soil 340 (1-2), 103–115. doi:10.1007/s11104-010-0366-6
Snyman, H. A. (2003). Revegetation of bare patches in a semi-arid rangeland of South Africa: an evaluation of various techniques. J. Arid Environ. 55 (3), 417–432. doi:10.1016/s0140-1963(02)00286-0
Soil Science Society of China, Agriculture Chemistry Council (1983). General analysis methods of soil agriculture chemistry. Beijing: Science Press.
Squires, V., Hua, L., Zhang, D., and Li, G. (2010). Towards sustainable use of rangelands in North-West China. New York, NY: Springer.
Su, R., Cheng, J., Chen, D., Bai, Y., Jin, H., Chao, L., et al. (2017). Effects of grazing on spatiotemporal variations in community structure and ecosystem function on the grasslands of Inner Mongolia, China. Sci. Rep. 7 (1), 40. doi:10.1038/s41598-017-00105-y
Su, Y. Z., Li, Y. L., Cui, J. Y., and Zhao, W. Z. (2005). Influences of continuous grazing and livestock exclusion on soil properties in a degraded sandy grassland, Inner Mongolia, northern China. Catena 59 (3), 267–278. doi:10.1016/j.catena.2004.09.001
Suttie, J. M., Reynolds, S. G., and Batello, C. (2005). Grasslands of the world. No. 34. Rome, Italy: Food and Agriculture Organisation of the United Nations.
Tarhouni, M., Ben Hmida, W., and Neffati, M. (2017). Long-term changes in plant life forms as a consequence of grazing exclusion under arid climatic conditions. Land Degrad. Dev. 28 (4), 1199–1211. doi:10.1002/ldr.2407
Wan, H., Bai, Y., Schönbach, P., Gierus, M., and Taube, F. (2011). Effects of grazing management system on plant community structure and functioning in a semiarid steppe: scaling from species to community. Plant Soil 340 (1-2), 215–226. doi:10.1007/s11104-010-0661-2
Wang, G., Cheng, G., Shen, Y., and Qian, J. (2003). Influence of land cover changes on the physical and chemical properties of alpine meadow soil. Chin. Sci. Bull. 48 (2), 118–124. doi:10.1007/bf03037014
Wang, S., Wilkes, A., Zhang, Z., Chang, X., Lang, R., Wang, Y., et al. (2011). Management and land use change effects on soil carbon in northern China's grasslands: a synthesis. Agric. Ecosyst. Environ. 142 (3-4), 329–340. doi:10.1016/j.agee.2011.06.002
Wang, Y., and Wesche, K. (2016). Vegetation and soil responses to livestock grazing in Central Asian grasslands: a review of Chinese literature. Biodivers. Conservation 25 (12), 2401–2420. doi:10.1007/s10531-015-1034-1
Xie, Z., Le Roux, X., Wang, C., Gu, Z., An, M., Nan, H., et al. (2014). Identifying response groups of soil nitrifiers and denitrifiers to grazing and associated soil environmental drivers in Tibetan alpine meadows. Soil Biol. Biochem. 77, 89–99. doi:10.1016/j.soilbio.2014.06.024
Yang, X. H., Zhang, K. B., and Hou, R. P. (2005). Impacts of exclusion on vegetative features and aboveground biomass in semi-arid degraded rangeland. Ecol. Environ. 14, 730–734. doi:10.16258/j.cnki.1674-5906.2005.05.024
Yang, Y., Fang, J., Ma, W., Guo, D., and Mohammat, A. (2010). Large-scale pattern of biomass partitioning across China's grasslands. Glob. Ecol. Biogeogr. 19 (2), 268–277. doi:10.1111/j.1466-8238.2009.00502.x
Yang, Z., Zhu, Q., Zhan, W., Xu, Y., Zhu, E., Gao, Y., et al. (2018). The linkage between vegetation and soil nutrients and their variation under different grazing intensities in an alpine meadow on the eastern Qinghai-Tibetan Plateau. Ecol. Eng. 110, 128–136. doi:10.1016/j.ecoleng.2017.11.001
Yayneshet, T., Eik, L. O., and Moe, S. R. (2009). The effects of exclosures in restoring degraded semi-arid vegetation in communal grazing lands in northern Ethiopia. J. Arid Environ. 73 (4-5), 542–549. doi:10.1016/j.jaridenv.2008.12.002
Zhao, X. Q. (2011). Restoration of degraded grassland ecosystem and sustainable management in sanjiangyuan regions. Beijing, China: Science Press, 1–43.
Keywords: grazing management, species richness, plant density, above-and belowground biomass, soil physicochemical properties grazing management, soil physicochemical properties
Citation: Wang D and Ding W (2024) Grazing led to an increase in the root: shoot ratio and a shallow root system in an alpine meadow of the Tibetan plateau. Front. Environ. Sci. 12:1348220. doi: 10.3389/fenvs.2024.1348220
Received: 02 December 2023; Accepted: 23 January 2024;
Published: 12 February 2024.
Edited by:
Chong Jiang, Guangdong Academy of Science (CAS), ChinaReviewed by:
Emmanuel Serrano Ferron, Autonomous University of Barcelona, SpainWanyu Wen, Chinese Academy of Forestry, China
Yixin Wang, Hohai University, China
Copyright © 2024 Wang and Ding. This is an open-access article distributed under the terms of the Creative Commons Attribution License (CC BY). The use, distribution or reproduction in other forums is permitted, provided the original author(s) and the copyright owner(s) are credited and that the original publication in this journal is cited, in accordance with accepted academic practice. No use, distribution or reproduction is permitted which does not comply with these terms.
*Correspondence: Duobin Wang, gswdb@126.com; Wenqiang Ding, dwqjz@126.com