- 1Department of Civil, Architectural and Environmental Engineering, Missouri University of Science and Technology, Rolla, MO, United States
- 2Department of Chemistry, Missouri University of Science and Technology, Rolla, MO, United States
- 3Department of Agriculture and Environmental Sciences and Cooperative Research, Lincoln University of Missouri, Jefferson City, MO, United States
- 4Department of Civil and Environmental Engineering, University of Missouri, Columbia, MO, United States
Accumulation of arsenic (As) in rice grain was reported in many regions of the world, including the United States, which has been a threat to human health. This field research investigated the grain As accumulation and its relationship with the uptake of selenium (Se), molybdenum (Mo), and cadmium (Cd) in soils with and without monosodium methanearsonate (MSMA) amended, as effects of selected rice cultivars and water management. Results indicated that MSMA increased the accumulation of As and Se but decreased Mo for all six cultivars under four irrigation management. MSMA also increased grain-Cd in some cultivars. In no MSMA-amended soil (Native soil), intermittent flooding decreased grain-As by 66%, grain-Se by 21%, and grain-Mo by 63%, but increased grain-Cd by 64% in Zhe 733, a straighthead resistant cultivar, while in MSMA-amended soil, intermittent flooding decreased grain-As by 63% and grain-Mo by 44% but increased grain-Se by 68% and grain-Cd by three times. For all other five cultivars, intermittent flooding generally decreased grain-As and grain-Mo but increased grain-Se and grain-Cd. Zhe 733 cultivar resulted in the lowest grain concentrations of all trace elements in all water treatments. A negative grain As-Se correlation and a positive grain As-Mo correlation were significant but not the As-Cd correlation. This research showed that the uptake of As, Se, Mo, and Cd by rice grain occurred as a complex function of multiple variables, including cultivar type and soil chemistry. As a result, accumulation of As and other trace elements in rice grain may be controlled by selecting appropriate cultivars and adopting appropriate water management practices.
Introduction
Inorganic arsenic (As) has been determined to be a human carcinogen by the United States Environmental Protection Agency (U.S. EPA) (ATSDR, 2007). The contamination of As in rice grain, though primarily composed of less toxic organic As, has also been recognized as carcinogenic (Florea et al., 2005; Williams et al., 2005). Increased As in rice grain could be caused by elevated As levels in soils of traditional cotton growing regions in the south central U.S. (Loebenstein, 1994), because of a century of arsenical herbicide, pesticide, and defoliant applications such as monosodium methanearsonate (MSMA) (ATSDR, 2007). Average total grain-As concentrations of rice grown in the south-central U.S. region was 0.27 μg A g-1 with approximately inorganic As of 0.11 µg g-1 (Williams et al., 2007; Batres-Marquez et al., 2009), which has an oral reference dose of 0.3 μg kg body weight (bw)−1 day-1 for chronic oral exposures (EPA and U. S., 2019). Although the total As in rice is primarily in an organic form, arsenic in subsequent rice food products, such as toddler formula, cereal bars, rice syrup, and energy shots, is primarily in the inorganic form (Meacher et al., 2002; Liang et al., 2010; Jackson et al., 2012; Yüksel et al., 2023). Therefore, the daily consumption of these majorly unregulated rice and rice products could exceed the equivalent of the USEPA’s and World Health Organization’s (WHO) drinking water standard of 10 µg L-1 (EPA, 2001; WHO, 2008), making it imperative that the agricultural practices directly responsible for total As in foods be further studied.
Environmental factors impacting As uptake by rice plants may include soil As content, irrigation management, and genotypes of rice cultivars. Elevated soil As has been found to correspond to higher grain As content (Xie and Huang, 1998; Heikens, 2006; Topaldemir et al., 2023). Straighthead disease is common in rice crops grown with elevated soil-As conditions, where panicle sterility and significant yield loss have been observed (Yan et al., 2005). Grain-As is exacerbated further, where flooded conditions create a reducing environment that can lead to increasing As uptake (Masscheleyn et al., 1991; Xie and Huang, 1998; Somenahally et al., 2011). Plant As uptake also varies among the genotypes of cultivars (Jian et al., 2008; Norton et al., 2009). Thus, cultivar selection could also be useful to reduce grain-As (Pillai et al., 2010).
In addition to As, the accumulation of other trace elements, such as selenium (Se), molybdenum (Mo), and cadmium (Cd) in rice grain could also be of concern. Se is an essential element to human health, and lack of Se could cause cardiovascular disease and cancer (Navarro-Alarcon and Cabrera-Vique, 2008). However, excess Se may also negatively impact human health. USEPA regulates Se in drinking water with a maximum contaminant level (MCL) of 50 µg L-1 (EPA and U. S., 2009) and an oral reference dose of 5 μg kg bw−1 day-1 (EPA and U. S., 2019). By understanding Se transport mechanisms, rice Se concentrations could be regulated, as necessary, to minimize Se biofortification practices and provide optimal dosage for human consumption (Zhu et al., 2009; Premarathna et al., 2012). Mikkelsen et al. (Mikkelsen et al., 1989) showed that flooded condition could reduce Se uptake, while Premarathna et al. (Premarathna et al., 2012) indicated that irrigation management may impact total grain Se in the order: field capacity > submerged > submerged/drained.
Mo is also an essential trace element for humans, but also has a negative effect at high exposure, which is listed on the USEPA’s drinking water Contaminant Candidate List 3 (CCL3) (EPA and U. S., 2009). The USEPA oral reference dose is 5 μg Mo kg bw−1 day-1 (EPA and U. S., 2019). Mo has been found to regulate calcium (Ca), magnesium (Mg) and copper (Cu) metabolisms in humans, where the greatest risk of Mo toxicity occurs in humans with Cu deficiencies (Antoine et al., 2012). Dunn and Dunn (Dunn and Dunn, 2012) discovered that increasing yield of rice grain (i.e., decreasing in total grain-As) resulted in a decrease in grain-Mo, suggesting a positive As-Mo correlation. Mo toxicity may be a factor for crops grown in poorly drained soils, where the tolerance varied with cultivar (Rout and Das, 2002).
In recent years, the accumulation of Cd in rice has been reported in many areas, especially China (Liu et al., 2003; Arao et al., 2009). Unlike As, Se, and Mo, which are mostly in anionic form, Cd is a cation form. The Cd bioaccumulation in rice may be greater than some other essential elements (Liu et al., 2003). Further, Cheng et al. (Cheng et al., 2006) showed a positive As-Cd correlation in rice roots and straw. Negative health impacts are known for Cd, including kidney dysfunction (Jakubowski et al., 2002). The USEPA oral reference dose is 1 μg Cd kg bw−1 day-1 (EPA and U. S., 2019). The Joint Food and Agriculture Organization/World Health Organization Expert Committee (JECFA) has set the Cd provisional tolerable weekly intake (PTWI) at 7 μg kg bw−1 wk-1 (FAO/WHO, 2003).
Previously, most studies on the uptake of trace elements by rice focused on individual elements. In this research, it was hypothesized that the uptake of multiple elements of concern is correlated. The objectives of this research were to provide an understanding of i) the impact of soil chemistry, such as MSMA amendment and water management (intermittent flooding vs continuous flooding), on total grain concentrations of selected trace elements of six cultivars, and ii) correlations among trace elements of concern in rice grain. The findings from this research would help rice growers adopt appropriate management practices that control the uptake of As and selected trace elements by rice grain.
Materials and methods
Field experiment
The field study was conducted at the USDA-ARS Dale Bumpers National Rice Research Center near Stuttgart, Arkansas in 2009. The soil on the site is a Dewitt silt-loam soil (fine, smectitic, thermic Typic Albaqualf). The experiment was arranged in a split-split plot design with four replications per treatment, in which MSMA-amended soils were main plots and water management as sub-plots. The main plots included MSMA-amended and no MSMA treatments. The MSMA-amended plots were established since 1980s for the straighthead resistance study (Yan et al., 2005), where MSMA was sprayed and incorporated into soil at a rate of 6.7 kg MSMA ha-1 prior to planting, as described by Yan et al. (Yan et al., 2005). The Native plot was located nearby where no MSMA was applied. Topsoil (0–20 cm) were collected from both MSMA-amended and Native plots before rice planting for soil characterization (Hua et al., 2011). Each main plot was divided into four completely randomized sub-plots for water treatments. The water treatment included four water management practices: one intermittent flooding and three continuous flooding. Intermittent flooding conditions were maintained by initially flooding the soil, and then allowing soil to dry until small, surface cracks were evident. At this point, the plots were irrigated up to 10 cm water depth with a nearby reservoir water (pH ∼7.0, <2 μg A L-1). Continuous floodings were conditions under which plots were drained at 10, 20, or 35 days after the heading date (50% panicle emergence from flag leaf). Each sub-plot contained six-row (1.8 m by 1.5 m) blocks in a completely randomized arrangement with four replications per cultivar (Pillai et al., 2010). Six rice cultivars were selected in this study: Cocodrie, Rondo and Wells from the south-central U.S.; Zhe 733 and GP-2 from China; and Spalcik from Russian Federation (Yan et al., 2008; Yan and McClung, 2010). These six cultivars included early, mid- and late-maturity and varied with the resistance or tolerance for As-induced straighthead disease (resistant, moderate, and susceptible) (Yan et al., 2008; Yan and McClung, 2010). This field study concurred with development of the practical solution to minimize grain-As accumulation in field conditions and primarily focused on Zhe 733 cultivar, since it has been identified as an As tolerant cultivar and widely studied for As-induced straighthead disease (Yan et al., 2005; Yan et al., 2008) and grain-As uptake (Yan et al., 2005; Pillai et al., 2010; Hua et al., 2011).
At maturity, rice grains from each plot were harvested, dried at 65°C to approximately 12% of moisture content, and stored at room temperature for digestion and then chemical analysis. Prior to digestion, grain samples were de-hulled using a ceramic mortar and pestle and oven dried at 65°C for 48 h and stored in a desiccator. 127.
Chemical analysis
A Multiwave 3,000 microwave digester (Anton Paar, Austria) was used for grain and soil sample digestion. In particular, EPA Method 3,052 was followed to digest rice grain samples, and slightly modified EPA Method 3051A was used to digest soil samples for the determination of the total concentrations of trace elements. For rice grain digestion, 0.5 g rice grain was placed in the acid cleaned Rotor 48 (MF50) digestion vessels and added with 8.0 mL optima grade nitric acid (HNO3) and 0.3 mL trace metal hydrogen peroxide (H2O2) (Fisher Scientific Co., Boston, U.S.). The digestion program consisted of a 10-min ramp to 100°C with a 10-min hold, followed by a 10-min ramp to 140°C, with a 15-min hold, then a 10-min ramp to 170°C, with a 10-min hold before cooling down.
For soil digestion, 0.5 g soil samples were placed in acid cleaned Rotor 8 (XF100) digestion vessels and digested with 9.0 mL trace metal grade HNO3 and 3.0 mL trace metal grade hydrochloric acid (HCl). The digestion program consisted of a 10-min ramp to 1100 W, with a 20-min hold before cooling down. All digested samples were allowed to cool down to <50°C before venting. Digested samples were rinsed from digestion vessels to acid-cleaned 50 mL conical tubes, and then diluted to 20 mL using Millipore water (18.2 MΩ-cm).
Standard reference materials (NIST, Gaithersburg, MD) for rice grain (SRM1568a) and soil (SRM2711a Montana II) were included in respective digestions for quality assurance and quality control (QA/QC). Additional digestion QA/QC included one sample blank, one sample duplicate, and one sample spike per 10 samples for rice and soil digestion. Spike concentrations were analyzed at an estimated two times of average sample concentrations.
Total As, Se, Mo, and Cd concentrations in digested grain samples and extractable Se, Mo, and Cd concentration in soil samples were determined using inductively coupled mass spectrometry (ICP-MS) (PerkinElmer Elan DRC-e, MDS Sciex, Concord, Ontario, Canada). Extractable As from soil was determined using graphite furnace atomic adsorption spectroscopy (GFAA) (PerkinElmer AAnalystTM 600, Shelton, CT, United States). Digested grain and soil samples were diluted using 10% and 5% HNO3 prior to chemical analysis, respectively. Sample method detection limits (MDLs) for all tested elements were determined for GFAA and ICP-MS, according to EPA Method 200.9 and 200.8, respectively. Estimated soil MDLs for As, Se, Mo, and Cd were 0.10, 0.08, 0.02, and 0.02 mg kg-1, respectively, while rice grain MDLs for As, Se, Mo, and Cd were 0.004, 0.031, 0.003, and 0.003 mg kg-1, respectively. Sample concentrations greater than 2–3 times MDL were required. QA/QC was validated if the following criteria were met: (a) relative difference of a duplicate sample less than 20%; (b) spike recovery between 85% and 115%; and (c) the accuracy of a calibration standard within 10% per 10 samples analyzed.
Statistical analysis
The analysis of variance (ANOVA) procedure that was appropriate for the split-split-plot design was accrued out using SAS 9.2 to estimate the impact of experimental variables on trace element uptake. The experimental variables (factors) were soil-As (Soil), irrigation management (Water), and rice cultivar (Cultivar), with Soil as the main-plot factor, Water as the sub-plot factor and Cultivar as the sub-sub-plot factor. ANOVA allows interaction terms representing the combined impact of different factors on element uptake to be tested, whereby a significant interaction term signifies that the impact of the changing levels of one factor is influenced by the level of the other factor(s). Least square means with Tukey’s adjustment were used to contrast means of specific Soil-Water combinations. Tukey’s means adjustment allowed experiment-wise error to be kept at the selected rate, α = 0.05 (Montgomery, 2009). Pearson’s partial correlations between grain-As and grain-Se, grain-Mo, and grain-Cd were also estimated.
Results and discussion
Soil characterization
Characterization and major physiochemical properties of two soils, Native and MSMA-amended soils, were presented in Hua et al. (Hua et al., 2011) and Yan et al. (Yan et al., 2008). The average As content in Native soil was 5.9 ± 0.25 mg kg-1 while MSMA-amended soil 19.5 ± 0.36 mg kg-1. Furthermore, Native soil contained 0.230 ± 0.004 mg Se kg-1, 0.38 ± 0.04 mg Mo kg-1, and 0.060 ± 0.003 mg Cd kg-1 Cd, while MSMA-amended soil contained 0.53 ± 0.09 mg Se kg-1, 0.63 ± 0.24 mg Mo kg-1, and 0.060 ± 0.006 mg Cd kg-1.
Effect of soil As level
For each type of soil, total concentrations of the four trace elements analyzed were found not significantly different among three continuous flooding treatments (drain at 10, 20, and 35 days after heading), but MSMA amendment resulted in significantly higher grain-As and lower grain-Mo, as indicated in Table 1. Thus, the average value of three continuous flooding treatments was used when compared with that from the intermittent flooding treatment.
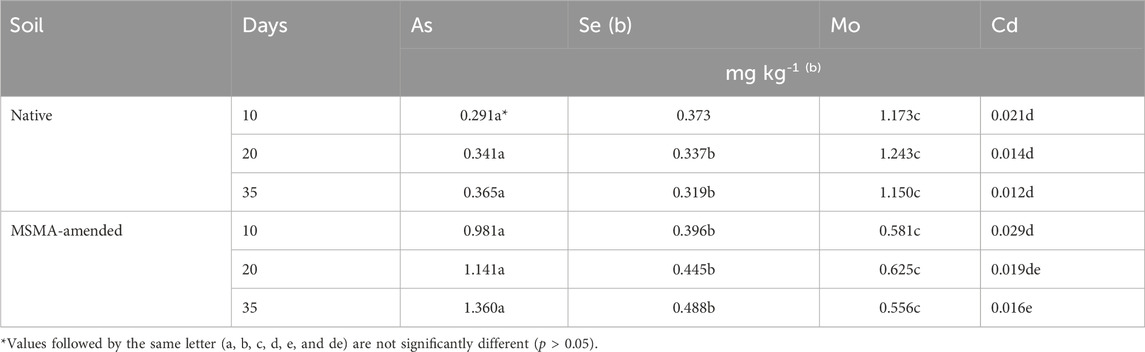
Table 1. Total grain trace element concentrations for rice grown under three continuous flooding conditions (drained 10, 20, and 35 days after heading, respectively).
Elevated grain-As concentrations were reported in low-As soils under both continuous and intermittent flooding conditions, and the presence of trace MSMA quantities could strongly affect grain-As (Pillai, 2009). Yan et al. (Yan et al., 2005) showed increased grain-As and reduced grain yield due to MSMA-induced straighthead susceptibility under continuous flooding conditions.
The effect of soil-As on average grain concentrations of As, Se, Mo, and Cd under continuous and intermittent flooding for the As-tolerant Zhe 733 was presented in Figure 1. Data can be compared with current global As standards of the United Kingdom Food Statutory General Limit (1.0 mg kg-1 fresh weight in food) (Kingdom et al., 1959). Currently, there is no food standards established for As, Se, Mo, and Cd in United States. Zhe 733 cultivar grown under continuous flooding and MSMA-amendments significantly increased grain-As from 0.30 to 0.60 mg kg-1 (103%), grain-Se from 0.24 to 0.31 mg kg-1 (30%), grain-Cd from 0.014 to 0.016 mg kg-1 (18%), and decreased grain-Mo from 1.15 to 0.64 mg kg-1 (45%) (Figure 1). Under intermittent flooding, MSMA-amendments increased As from 0.10 to 0.22 mg kg-1 (115%), Se from 0.19 to 0.52 mg kg-1 (175%), Cd from 0.023 to 0.065 mg kg-1 (183%), and decreased Mo from 0.42 to 0.36 mg kg-1 (−13%). Thus, MSMA amendment resulted in the same trend on trace element concentrations in both irrigation managements, with a greater impact by intermittent flooding. The highest grain-As levels from MSMA-amended and continuous flooding were considerably less than the United Kingdom Food Statutory General Limit (Kingdom et al., 1959). However, rice consumption at the average U.S. consumer diet of ≥61.2 g rice grain day-1 (Batres-Marquez et al., 2009) and the average Asian-American diet of 400 g rice grain day-1 (Navarro-Alarcon and Cabrera-Vique, 2008) may result in an ingestion of approximately 0.52 and 3.4 μg A kg-1 day-1, respectively, which is substantially greater than the regulated intake amount of 0.29 μg A kg-1 day-1 through drinking water (EPA, 2001). The highest grain- Cd levels of 0.065 mg kg-1 were found in MSMA-amended intermittent flooding conditions. Consuming such rice grain for the average U.S. consumer and the Asian-American may intake 0.4 and 2.6 μg kg-1 wk-1, which is less than the JEFCA Cd standard (FAO/WHO, 2003). Furthermore, increased soil-As level not only increased grain-As, but also impacted the uptake of Se, Mo, and Cd, especially under intermittent flooding conditions.
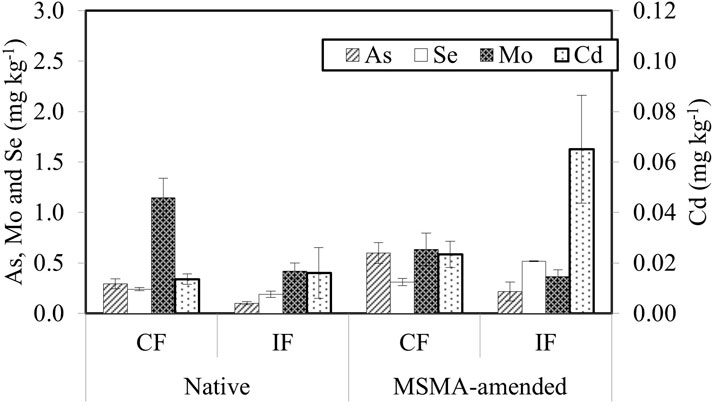
Figure 1. Average grain trace element concentrations of Zhe 733 grown in two soil treatments and two water managements.
A general trend of soil-As on average grain trace element concentrations of other five cultivars under continuous and intermittent flooding was illustrated in Figure 2. For the five cultivars under continuous flooding, MSMA amendment increased As from 0.33 to 1.16 mg kg-1 (252%), Se from 0.34 to 0.44 mg kg-1 (29%), Cd from 0.016 to 0.021 mg kg-1 (31%), and decreased Mo from 1.19 to 0.59 mg kg-1 (50%). Under intermittent flooding, MSMA amendment increased grain-As from 0.26 to 0.38 mg kg-1 (46%), Se from 0.41 to 0.70 mg kg-1 (71%), decreased Mo from 0.67 to 0.50 mg kg-1 (25%) and Cd from 0.057 to 0.050 mg kg-1 (12%). Overall, increasing soil-As increased levels of grain-As and grain-Se, but decreased grain-Mo level for both irrigation management practices. However, MSMA amendment slightly increased grain-Cd under continuous flooding but slightly decreased grain-Cd under intermittent flooding.
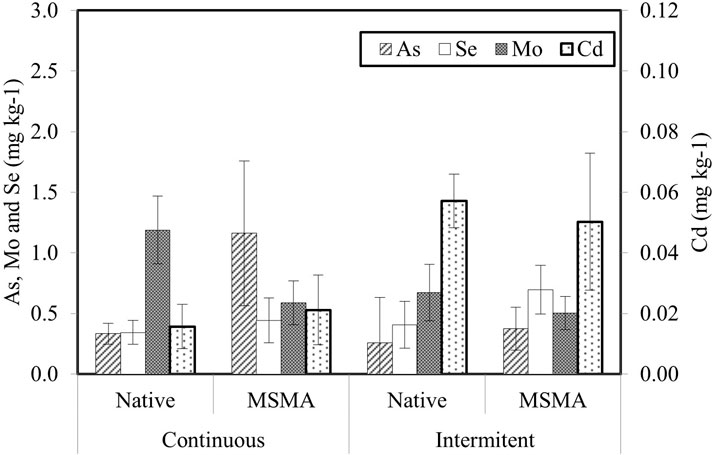
Figure 2. Average grain trace element concentrations of five cultivars grown in two soil treatments and two water managements.
Zhe 733 cultivar had been widely used as a resistant check cultivar for As-induced straighthead disease studies (Yan et al., 2005; Yan et al., 2008; Pillai, 2009; Pillai et al., 2010; Hua et al., 2011; Somenahally et al., 2011). Comparing with five other cultivars, Zhe 733 had much less grain-As concentrations in all of the irrigation-MSMA treatments: 9% less in Native-continuous, 48% less in MSMA-continuous, 62% less in Native-intermittent, and 42% less in MSMA-intermittent (Figures 1, 2). Data suggested that straighthead resistance be associated with less As uptake.
Effect of water management
Also shown in Figure 1, in compared with the continuous flooding, Zhe 733 cultivar in Native soil under intermittent flooding significantly decreased grain-As from 0.30 to 0.10 mg kg-1 (66%), grain-Se from 0.24 to 0.19 mg kg-1 (21%), grain-Mo from 1.15 to 0.42 mg kg-1 (63%) and increased grain-Cd from 0.014 to 0.023 mg kg-1 (64%). The other five cultivars grown in same soil under intermittent flooding slightly decreased grain-As from 0.33 to 0.26 mg kg-1, slightly increased grain-Se from 0.34 to 0.41 mg kg-1, significantly decreased grain-Mo from 1.19 to 0.67 mg kg-1, and significantly increased grain-Cd from 0.016 to 0.057 mg kg-1 (Figure 2).
Soil redox potential had a large impact on the fate and transport of As, due to the presence of insoluble FeIII (hydr)oxides and, consequently, the formation of AsV-FeIII complexes in aerobic systems (Charlet et al., 2011; Wang et al., 2011). Soil redox measurements in intermittent and continuous flooding plots (339 ± 84 vs 160 ± 52 mV) supports the above analysis because intermittent flooding condition provided a more oxidized condition facilitating the formation of AsV-FeIII complexes, decreasing As solubility and subsequent plant uptake (Masscheleyn et al., 1991). In addition, under more oxidized conditions, As is likely in the less mobile AsV form (Cullen and Reimer, 1989).
For Zhe 733, intermittent flooding also significantly decreased grain-Se. However, the overall trend of the other five cultivars indicated a slight increase in grain-Se under intermittent flooding, confirming the results from Mikkelsen et al. (Mikkelsen et al., 1989). This further suggested that the Se uptake mechanism by rice was more of a function of cultivar. Therefore, cultivar selection based on minimizing grain-As may also have implications on other elements. Similar to grain-As, overall grain-Mo significantly decreased as a result of intermittent flooding. The primary uptake mechanism for Mo and As under aerobic conditions occurs via the phosphate pathway (Balistrieri et al., 1994). Thus, it is evident that the oxidized soil environment would regulate and reduce Mo bioavailability by the formation of immobile iron (hydr)oxide complexes, resulting in lower mobility and thus plant uptake (Moore and Patrick, 1991). Overall grain-Cd concentrations significantly increased under the intermittent flooding. Due to the oxidized conditions associated with intermittent flooding, insoluble CdS may have converted to the more soluble CdSO4, resulting in a greater Cd availability for rice uptake (Ito and Iimura, 1976).
The significant impact of cultivars on the soil As and water management interaction in both Native and MSMA-amended soils was presented in Table 2. In compared to other cultivars, Zhe 733 grown in Native soil under intermittent flooding had the most significant decrease in grain-As. Among the six cultivars in Native soil under both continuous and intermittent flooding, cultivar selection had at least one significant impact on the uptake of selected elements. Results from this research could provide evidence that cultivar selection alone would significantly affect grain trace element concentration, varying with irrigation management practice.
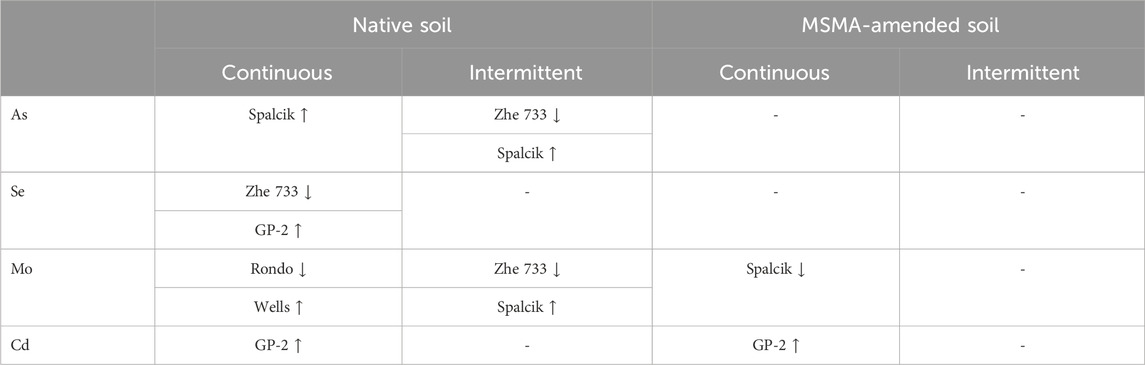
Table 2. Effects of cultivar on total grain trace element concentration of As, Se, Mo, and Cd under soil As and water management interactions.
Data in Figure 1 also showed the effect of water management on grain concentrations of As, Se, Mo, and Cd for Zhe 733 under MSMA-amended soil. In MSMA-amended soil and compared to continuous flooding, intermittent flooding significantly decreased grain-As from 0.60 to 0.22 mg kg-1 (63%) and grain-Mo from 0.64 to 0.36 mg kg-1 (44%), and significantly increased grain-Se from 0.31 to 0.52 mg kg-1 (68%) and grain-Cd from 0.016 to 0.065 mg kg-1 (3.1 times). Therefore, water management resulted in a similar effect on As, Mo, and Cd uptake in both Native and MSMA-amended soil, however, the effect on grain-Se was the opposite. For other five cultivars in MSMA-amended soil and compared to continuous flooding, intermittent flooding significantly decreased grain-As from 1.16 to 0.38 mg kg-1 and grain-Mo from 0.59 to 0.50 mg kg-1, and significantly increased grain-Se from 0.44 to 0.70 mg kg-1 and grain-Cd from 0.021 to 0.050 mg kg-1 (Figure 2). As compared to Native soil, intermittent flooding resulted in a greater decrease in grain-As for MSMA-amended soil. The grain-As concentrations determined in MSMA-amended soil were slightly different from those reported (Williams et al., 2007; Pillai et al., 2010), which was likely due to the cultivar variation used in this study.
The increase in grain-Se as a result of intermittent flooding in MSMA-amended soil in the current research confirms Mikkelsen et al. (Mikkelsen et al., 1989) and constant with Native soil results. Se is most prevalent in the mobile selenate form under aerobic conditions, therefore causing the increase in grain-Se in intermittent flooding (Mikkelsen et al., 1989). The increase in grain-Cd as a result of intermittent flooding discovered in the current research in MSMA-amended soil is consistent with Arao et al. (Arao et al., 2009). This increase in Cd concentrations of rice grain due to aerobic treatments makes it difficult to simultaneously decrease human exposure to both As and Cd elements based on irrigation management.
Among the six cultivars in MSMA-amended soil under continuous or intermittent flooding, cultivar selection had a significant impact on grain-Mo and grain-Cd (Table 2). Compared to other cultivars, GP-2 had the most significant increase in grain-Cd under continuous flooding and MSMA-amended soil, validating that cultivar can significantly alter grain element accumulation varying with water management. This is most likely due to cultivar specific uptake mechanisms. Though the cultivar impact on grain-As, Se, Mo, and Cd in MSMA-amended soil under intermittent flooding was determined to be negligible, Zhe 733 cultivar minimized grain-As in MSMA-amended soil, consistent with findings by Hua et al. (Hua et al., 2011). Overall, this result verified previous findings that cultivar selection is a useful practice to control trace element uptake in rice grain (Jian et al., 2008; Zhang et al., 2008; Norton et al., 2009).
Correlations among trace elements
Correlations among trace elements in all six cultivars grown from both Native and MSMA-amended soils were developed. The impacts of grain As uptake on Se, Mo, and Cd concentrations for Zhe 733 cultivar were presented Figure 3. Grain-As and grain-Se showed a positive correlation in Native soil, but a negative relationship in MSMA-amended soil. The correlation between grain-As and grain-Mo was positive in both soils. However, grain-As was negatively corelated with grain-Cd in both soils. Measurements contradicted the findings by Cheng et al. (Cheng et al., 2006) who reported a positive As-Cd correlation in rice root and straw.
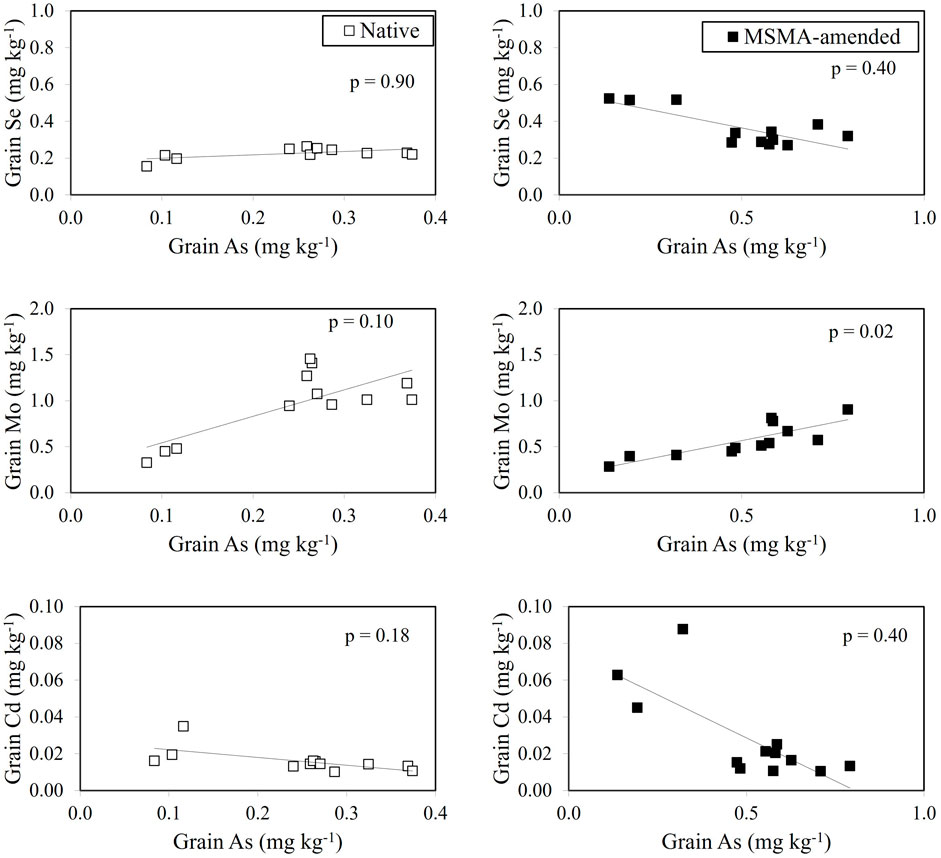
Figure 3. Trace element correlations for Zhe 733 cultivar in both Native (left) and MSMA-amended soils (right).
The correlations of grain-As with Se, Mo, and Cd for other five cultivars indicated an overall negative trend between grain-As and grain-Se, but with a weak correlation. Similar to Zhe 733 cultivar, a strong positive grain As-Mo trends were found for Cocodrie, Rondo, and Wells cultivars. Dunn and Dunn (Dunn and Dunn, 2012) discovered that plant Mo was negatively correlated with the straighthead score, suggesting that grain Mo decreased with increasing the straighthead score and total grain-As, which contradicted to the overall positive grain As-Mo trend found in this study. A negative grain As-Mo correlation was also reported by Norton et al. (Norton et al., 2012). Strong neutral As-Cd trends were discovered for Cocodrie, Spalcik, Zhe 733 cultivars grown in MSMA-amended soil, and GP-2 in Native soil.
Analysis of variance
ANOVA was performed on all cultivars to determine the significance of main effects and the corresponding interactions. The significant levels of main effects and interactions were shown in Table 3. The soil × water interaction for Zhe 733 was significant for Mo only, indicating that the impact of soil-As on grain-Mo was influenced by water management. Soil-As level significantly affected As and Se content in grain, confirming the increase in grain-As and grain- Se in MSMA-amended soil as compared to that in Native soil. For all six cultivars, the interaction of cultivar × soil × water was significant for grain-As and Se, and the interaction of cultivar × soil was significant for grain-Mo. Further analysis by Tukey’s means adjustment indicated that the occurrence of multiple significant interactions for As, Se, Mo, and Cd uptake by the six cultivars was a complex function rather than a function of a single element.
This field study demonstrated that the uptake of trace elements by rice was strongly impacted by soil chemistry, such as element contents or availability and redox conditions in soil. In addition, the correlations among grain trace elements revealed that these elements interacted with each other during the uptake process. Therefore, the uptake of an element could also be indirectly impacted by another element. The cultivar selection was also important for controlling the accumulation of trace elements. Conclusions based on single element uptake may not be appropriate for another element, even though they are in the same group. This research provided evidence that rice grain accumulation of trace elements may be selectively controlled by management practices during rice cultivation.
Conclusion
The impact of MSMA amendment and irrigation management on the accumulation of As, Se, Mo, and Cd in rice grains was assessed using six different rice cultivars. The findings demonstrated that the addition of MSMA might lead to elevated levels of As, Se, and Cd in the grain, while simultaneously reducing the amount of Mo accumulation. Intermittent flooding resulted in a reduction in grain-As and grain-Mo, while dramatically increasing grain-Se and grain-Cd. The Zhe 733 cultivar, which is resistant to straighthead disease, exhibited lower grain-As compared to other five cultivars across all treatments. A negative correlation between grain-Se and As concentrations was found in the MSMA amended soil. Additionally, a positive correlation between grain-As and Mo was found in the same soil. The accumulation of selected elements in grains was significantly influenced by soil chemistry and the specific rice cultivar. By selecting the straighthead-resistant Zhe 733 cultivar and implementing intermittent flooding, it is possible to greatly decrease the levels of As, Se, and Mo in the grain and help mitigate the health risks associated with the As buildup in the grain.
Data availability statement
The raw data supporting the conclusion of this article will be made available by the authors, without undue reservation.
Author contributions
EF: Formal Analysis, Investigation, Data curation, Writing–original draft. JW: Conceptualization, Funding acquisition, Investigation, Project administration, Supervision, Writing–original draft. HS: Methodology, Writing–review and editing. JY: Conceptualization, Funding acquisition, Investigation, Project administration, Resources, Supervision, Validation, Writing–review and editing. BH: Investigation, Writing–review and editing. BD: Writing–review and editing.
Funding
The author(s) declare financial support was received for the research, authorship, and/or publication of this article. This study was supported by USDA-NIFA. This research was funded by the USDA-NIFA (Award No. 2008-38814-04727) to Missouri University of Science and Technology (Missouri S&T) through Lincoln University of Missouri. The authors gratefully acknowledge supports from Dr. Wengui Yan and Ms. Tiffany Sookaserm at USDA Dale Bumpers National Rice Research Center in Stuttgart, Arkansas, for sample collection and preparation, and Ms. Brandi Clark and Dr. Joel Burket at Missouri S&T for assistance with analytical method development and facility support, respectively.
Conflict of interest
The authors declare that the research was conducted in the absence of any commercial or financial relationships that could be construed as a potential conflict of interest.
Publisher’s note
All claims expressed in this article are solely those of the authors and do not necessarily represent those of their affiliated organizations, or those of the publisher, the editors and the reviewers. Any product that may be evaluated in this article, or claim that may be made by its manufacturer, is not guaranteed or endorsed by the publisher.
References
Antoine, J. M. R., Hoo Fung, L. A., Grant, C. N., Dennis, H. T., and Lalor, G. C. (2012). Dietary intake of minerals and trace elements in rice on the Jamaican market. J. Food Compos. Analysis 26 (1-2), 111–121. doi:10.1016/j.jfca.2012.01.003
Arao, T., Kawasaki, A., Baba, K., Mori, S., and Matsumoto, S. (2009). Effects of water management on cadmium and arsenic accumulation and dimethylarsinic acid concentrations in Japanese rice. Environ. Sci. Technol. 43 (24), 9361–9367. doi:10.1021/es9022738
ATSDR (2007). “Toxicological profile for arsenic,” in Usdhhs (Atlanta, Georgia: Public Health Service Agency for Toxic Substances and Disease Registry).
Balistrieri, L. S., Murray, J. W., and Paul, B. (1994). The geochemical cycling of trace elements in a biogenic meromictic lake. Geochimica Cosmochimica Acta 58 (19), 3993–4008. doi:10.1016/0016-7037(94)90262-3
Batres-Marquez, S. P., Jensen, H. H., and Upton, J. (2009). Rice consumption in the United States: recent evidence from food consumption surveys. J. Am. Dietetic Assoc. 109 (10), 1719–1727. doi:10.1016/j.jada.2009.07.010
Charlet, L., Morin, G., Rose, J., Wang, Y., Auffan, M., Burnol, A., et al. (2011). Reactivity at (nano)particle-water interfaces, redox processes, and arsenic transport in the environment. Comptes Rendus Geosci. 343 (2-3), 123–139. doi:10.1016/j.crte.2010.11.005
Cheng, W. D., Zhang, G. P., Yao, H. G., Wu, W., and Xu, M. (2006). Genotypic and environmental variation in cadmium, chromium, arsenic, nickel, and lead concentrations in rice grains. J. Zhejiang Univ. Sci. B 7 (7), 565–571. doi:10.1631/jzus.2006.b0565
Cullen, W. R., and Reimer, K. J. (1989). Arsenic speciation in the environment. Chem. Rev. 89 (4), 713–764. doi:10.1021/cr00094a002
Dunn, B. W., and Dunn, T. S. (2012). Influence of soil type on severity of straighthead in rice. Commun. Soil Sci. Plant Analysis 43 (12), 1705–1719. doi:10.1080/00103624.2012.681742
EPA (2001). National primary drinking water regulations; arsenic and clarifications to compliance and new source contaminants monitoring. Available at: https://www.federalregister.gov/documents/2001/01/22/01-1668/national-primary-drinking-water-regulations-arsenic-and-clarifications-to-compliance-and-new-source.
EPA, U. S. (2009). Drinking water contaminant candidate list 3. Available at: https://www.federalregister.gov/documents/2009/10/08/E9-24287/drinking-water-contaminant-candidate-list-3-final.
EPA, U. S. (2019). Integrated risk information system. Available at: http://www.epa.gov/IRIS/.
Florea, A.-M., Yamoah, E. N., and Dopp, E. (2005). Intracellular calcium disturbances induced by arsenic and its methylated derivatives in relation to genomic damage and apoptosis induction. Environ. Health Perspect. 113 (6), 659–664. doi:10.1289/ehp.7634
Heikens, A. (2006). “Arsenic contamination of irrigation water, soil and crops in Bangladesh: risk implications for sustainable agriculture and food safety in Asia,” in Food and agriculture organization of the united nations, 46.
Hua, B., Yan, W., Wang, J., Deng, B., and Yang, J. (2011). Arsenic accumulation in rice grains: effects of cultivars and water management practices. Environ. Eng. Sci. 28 (8), 591–596. doi:10.1089/ees.2010.0481
Ito, H., and Iimura, K. (1976). The absorption and translocation of cadmium in rice plants and its influence on their growth, in comparison with zinc: studies on heavy metal pollution of soils (Part 1). Bull. Hokuriku Natl. Agriculuture Exp. Stn. 19, 71–139.
Jackson, B. P., Taylor, V. F., Karagas, M. R., Punshon, T., and Cottingham, K. L. (2012). Arsenic, organic foods, and Brown rice syrup. Environ. Health Perspect. 120 (5), 623–626. doi:10.1289/ehp.1104619
Jakubowski, M., Trzcinka-Ochocka, M., Hałatek, T., Raźniewska, G., and Szymczak, W. (2002). Integrated indexes of occupational exposure as predictors of kidney dysfunction. Int. J. Occup. Med. Environ. Health 15 (4), 393–399.
Jian, F. M., Yamaji, N., Mitani, N., Xu, X. Y., Su, Y. H., McGrath, S. P., et al. (2008). Transporters of arsenite in rice and their role in arsenic accumulation in rice grain. Proc. Natl. Acad. Sci. U. S. A. 105 (29), 9931–9935. doi:10.1073/pnas.0802361105
Kingdom, U. (1959). “Arsenic in food regulations,” in 831, the minister of agriculture, F. A. F. Editor T. M. o. Health (London: Her Majesty's Stationary Office).
Liang, F., Li, Y., Zhang, G., Tan, M., Lin, J., Liu, W., et al. (2010). Total and speciated arsenic levels in rice from China. Food Addit. Contam. - Part A Chem. Analysis, Control, Expo. Risk Assess. 27 (6), 810–816. doi:10.1080/19440041003636661
Liu, J., Li, K., Xu, J., Liang, J., Lu, X., Yang, J., et al. (2003). Interaction of Cd and five mineral nutrients for uptake and accumulation in different rice cultivars and genotypes. Field Crops Res. 83 (3), 271–281. doi:10.1016/s0378-4290(03)00077-7
Loebenstein, J. R. (1994). The materials flow of arsenic in the United States. Bureau Mines Inf. Circular 9382.
Masscheleyn, P. H., Delaune, R. D., and Patrick Jr, W. H. (1991). Effect of redox potential and pH on arsenic speciation and solubility in a contaminated soil. Environ. Sci. Technol. 25 (8), 1414–1419. doi:10.1021/es00020a008
Meacher, D. M., Menzel, D. B., Dillencourt, M. D., Bic, L. F., Schoof, R. A., Yost, L. J., et al. (2002). Estimation of multimedia inorganic arsenic intake in the U.S. population. Hum. Ecol. Risk Assess. 8 (7), 1697–1721. doi:10.1080/20028091057565
Mikkelsen, R. L., Mikkelsen, D. S., and Abshahi, A. (1989). Effects of soil flooding on selenium transformations and accumulation by rice. Soil Sci. Soc. Am. J. 53 (1), 122–127. doi:10.2136/sssaj1989.03615995005300010023x
Montgomery, D. C. (2009) Design and analysis of experiments. Hoboken, New Jersey, United States: John Wiley and Sons.
Moore, P. A., and Patrick, Jr. W. H. (1991). Aluminium, boron and molybdenum availability and uptake by rice in acid sulfate soils. Plant Soil 136, 171–181. doi:10.1007/bf02150048
Navarro-Alarcon, M., and Cabrera-Vique, C. (2008). Selenium in food and the human body: a review. Sci. Total Environ. 400 (1-3), 115–141. doi:10.1016/j.scitotenv.2008.06.024
Norton, G. J., Duan, G., Dasgupta, T., Islam, M. R., Lei, M., Zhu, Y., et al. (2009). Environmental and genetic control of arsenic accumulation and speciation in rice grain: comparing a range of common cultivars grown in contaminated sites across Bangladesh, China, and India. Environ. Sci. Technol. 43 (21), 8381–8386. doi:10.1021/es901844q
Norton, G. J., Duan, G. L., Lei, M., Zhu, Y. G., Meharg, A. A., and Price, A. H. (2012). Identification of quantitative trait loci for rice grain element composition on an arsenic impacted soil: influence of flowering time on genetic loci. Ann. Appl. Biol. 161 (1), 46–56. doi:10.1111/j.1744-7348.2012.00549.x
Pillai, T. R. (2009) Variability of grain arsenic concentration and speciation in rice (Oryza sative). College Station, TX: Texas A&M University.
Pillai, T. R., Yan, W., Agrama, H. A., James, W. D., Ibrahim, A. M. H., McClung, A. M., et al. (2010). Total grain-arsenic and arsenic-species concentrations in diverse rice cultivars under flooded conditions. Crop Sci. 50 (5), 2065–2075. doi:10.2135/cropsci2009.10.0568
Premarathna, L., McLaughlin, M. J., Kirby, J. K., Hettiarachchi, G. M., Stacey, S., and Chittleborough, D. J. (2012). Selenate-enriched urea granules are a highly effective fertilizer for selenium biofortification of paddy rice grain. J. Agric. Food Chem. 60 (23), 6037–6044. doi:10.1021/jf3005788
Rout, G. R., and Das, P. (2002). Rapid hydroponic screening for molybdenum tolerance in rice through morphological and biochemical analysis. Rostl. Vyroba 48 (11), 505–512. doi:10.17221/4404-pse
Somenahally, A. C., Hollister, E. B., Yan, W., Gentry, T. J., and Loeppert, R. H. (2011). Water management impacts on arsenic speciation and iron-reducing bacteria in contrasting rice-rhizosphere compartments. Environ. Sci. Technol. 45 (19), 8328–8335. doi:10.1021/es2012403
Topaldemir, H., Tas, B., Yüksel, B., and Ustaoglu, F. (2023). Potentially hazardous elements in sediments and Ceratophyllum demersum: an ecotoxicological risk assessment in Miliç Wetland, Samsun, Türkiye. Environ. Sci. Pollut. Res. 30, 26397–26416. doi:10.1007/s11356-022-23937-2
Wang, Y., Morin, G., Ona-Nguema, G., Juillot, F., Calas, G., and Brown, G. E. (2011). Distinctive arsenic(V) trapping modes by magnetite nanoparticles induced by different sorption processes. Environ. Sci. Technol. 45 (17), 7258–7266. doi:10.1021/es200299f
WHO (2008). “Guidelines for drinking-water quality,” in Incorporating the first and second addenda (Geneva: World Health Organization).
Williams, P. N., Price, A. H., Raab, A., Hossain, S. A., Feldmann, J., and Meharg, A. A. (2005). Variation in arsenic speciation and concentration in paddy rice related to dietary exposure. Environ. Sci. Technol. 39 (15), 5531–5540. doi:10.1021/es0502324
Williams, P. N., Raab, A., Feldmann, J., and Meharg, A. A. (2007). Market basket survey shows elevated levels of as in South Central U.S. processed rice compared to California: consequences for human dietary exposure. Environ. Sci. Technol. 406 (7), 2178–2183. doi:10.1021/es061489k
Xie, Z. M., and Huang, C. Y. (1998). Control of arsenic toxicity in rice plants grown on an arsenic-polluted paddy soil. Commun. Soil Sci. Plant Analysis 29 (15-16), 2471–2477. doi:10.1080/00103629809370125
Yan, W., Agrama, H. A., Slaton, N. A., and Gibbons, J. W. (2008). Soil and plant minerals associated with rice straighthead disorder induced by arsenic. Agron. J. 100 (6), 1655–1661. doi:10.2134/agronj2008.0108
Yan, W., Dilday, R. H., Tai, T. H., Gibbons, J. W., McNew, R. W., and Rutger, J. N. (2005). Differential response of rice germplasm to straighthead induced by arsenic. Crop Sci. 45 (4), 1223–1228. doi:10.2135/cropsci2004.0348
Yan, W., and McClung, A. M. (2010). ‘Rondo’, a long-grain indica rice with resistances to multiple diseases. J. Plant Registrations 4 (2), 131–136. doi:10.3198/jpr2009.07.0404crc
Yüksel, B., Ustaoğlu, F., Yazman, M. M., Şeker, M. E., and Öncü, T. (2023). Exposure to potentially toxic elements through ingestion of canned non-alcoholic drinks sold in Istanbul, Türkiye: a health risk assessment study. Food Compos. Analysis 121, 105361. doi:10.1016/j.jfca.2023.105361
Zhang, J., Zhu, Y. G., Cheng, W. D., Qian, Q., and Duan, G. L. (2008). Mapping quantitative trait loci associated with arsenic accumulation in rice (Oryza sativa). New Phytol. 177 (2), 350–355. doi:10.1111/j.1469-8137.2007.02267.x
Keywords: rice cultivar, arsenic, trace elements, accumulation, water management
Citation: Farrow EM, Wang J, Shi H, Yang J, Hua B and Deng B (2024) Selected trace element uptake by rice grain as affected by soil arsenic, water management and cultivar -a field investigation. Front. Environ. Sci. 12:1347330. doi: 10.3389/fenvs.2024.1347330
Received: 30 November 2023; Accepted: 08 April 2024;
Published: 16 May 2024.
Edited by:
Qi Liao, Central South University, ChinaReviewed by:
Arup Giri, Baba Mastnath University, IndiaMariusz Gusiatin, University of Warmia and Mazury in Olsztyn, Poland
Copyright © 2024 Farrow, Wang, Shi, Yang, Hua and Deng. This is an open-access article distributed under the terms of the Creative Commons Attribution License (CC BY). The use, distribution or reproduction in other forums is permitted, provided the original author(s) and the copyright owner(s) are credited and that the original publication in this journal is cited, in accordance with accepted academic practice. No use, distribution or reproduction is permitted which does not comply with these terms.
*Correspondence: Jianmin Wang, wangjia@mst.edu; John Yang, yangj@lincolnu.edu