- Ecodynamics Group, Department of Physical, Earth and Environmental Sciences, University of Siena, Siena, Italy
Introduction: Hemp is a crop cultivated in Europe since ancient times, with a variety of purposes and products. Despite being known for its positive environmental effects on ecosystems, the impacts of hemp-based food products have not been sufficiently investigated yet. This paper contributed to deepen the knowledge of the hemp industry by focusing on the potential environmental impact of the cultivation phase (under three different agronomic practices in Italy: organic outdoor and conventional outdoor, and indoor) and the production of selected hemp-based goods (seed oil and flour for food purposes and flowers for therapeutic uses).
Methods: The impact was quantified utilizing the life cycle assessment within different impact categories, such as carbon footprint (CF), eutrophication (EP), acidification (AP), and water footprint (WF). For a carbon offset assessment, the carbon storage capability (i.e., the carbon fixed in crop residues left in the field) of hemp was also investigated through the guidelines provided by the Intergovernmental Panel on Climate Change (IPCC).
Results and Discussion: The cultivation phase contributed to a CF that ranged from 1.2 (organic outdoor) to 374 (indoor) kg per kg of grains (conventional outdoor). These results were in line with the literature. Sensitivity scenarios based on hotspot analysis were also presented for CF mitigation for each kind of cultivation. On the other hand, the ability of hemp to sequester carbon in the soil due to crop residues left in the field (i.e., carbon storage) was evaluated (−2.7 kg CO2 (ha year)−1), showing that the CF was fully compensated (−0.27 kg CO2 (ha year)−1 for conventional outdoor and −1.07 kg CO2 (ha year)−1 for organic outdoor). Regarding hemp-based products, only dried flowers showed a negative balance (−0.99 kg CO2 per kg dry flower), while hemp oil and flour reported 31.79 kg CO2 per kg flour) when carbon storage was accounted. The results support the idea that the production chain can be sustainable and carbon-neutral only when all the different parts of the plant (flowers, seeds, fibers, leaves, and all residues) were used to manufacture durable goods according to the framework of the circular economy.
1 Introduction
Hemp (Cannabis sativa L.) is an annual dicotyledonous angiosperm plant belonging to the order Rosales, suborder Rosidae, and family Cannabaceae (The Angiosperm Phylogeny Group, 1998; Adesina et al., 2020). Hemp is a versatile plant, and it easily adapts to different climatic conditions. It is used today in several agricultural and industrial sectors, such as textiles manufacturing, bio-composite materials, papermaking, construction field, biofuels, personal care, and cosmetics (Salentijn et al., 2015; Campiglia et al., 2017). Hemp is also grown for its therapeutic uses and for food production (i.e., seeds). The seeds are the edible parts of Cannabis sativa L. and contain a large amount of macro and micro nutrients, such as proteins, unsaturated fatty acids, dietary fibers, and minerals, making them a good fortifying component in food production (Teterycz et al., 2021). Furthermore, hemp oil shows a growing marketable potential, and hemp flour, a by-product of oil processing, is added in many protein-rich foods and animal feeds (Yano and Fu, 2023). Hemp is an excellent break crop that can improve the soil structure due to its extensive root system (Amaducci et al., 2008); moreover, it also reduces weed pressure and enhances the yield of the subsequent crop (Bocsa et al., 1998; Amaducci et al., 2015; Campiglia et al., 2020). Additionally, hemp shows the ability of absorbing and accumulating heavy metals, such as cadmium, nickel, chromium, lead, mercury, cobalt, and arsenic in contaminated soils (Citterio et al., 2005; Gryndler et al., 2008; Ćaćić et al., 2019). Industrial hemp can be utilized for phytoremediation of heavy metal-polluted soil, while the resulting contaminated biomass can be used as an energy source (Todde et al., 2022). Finally, hemp contributes to the provision of the ecosystem’s services by supporting pollination. Late-season crop flowering provides bee communities with supplementary nutritional resources during the months of floral scarcity (i.e., late summer and the beginning of autumn in Italy) (Dowling et al., 2021), thus sustaining pollination and biodiversity richness, with benefits for the other crops in the agroecosystems and the surrounding natural systems (Journals and Dalio, 2014; Flicker et al., 2020).
Hemp has been cultivated since ancient times in many parts of Europe, and among all the possible applications, its use in the production of textile was prevalent for many centuries (Mercuri et al., 2002; Allegret et al., 2013; Skoglund et al., 2013). However, during the 20th century, the increasing use of cotton and synthetic fibers (Allegret et al., 2013) and the rising cost of labor (Campiglia et al., 2020) led to a decline in hemp cultivation. Moreover, the cultivation of hemp was forbidden in many countries due to the delta-9-tetrahydrocannabinol (Δ9-THC) content, i.e., the main psychoactive constituent of Cannabis and one of at least 113 total cannabinoids identified in the plant. Nowadays, in several EU countries, hemp with less than 0.3% or 0.2% Δ9-THC does not fall within the drug regulation laws, thus increasing the interest in this crop (Faux et al., 2013; Farinon et al., 2022). Finally, when considering the low content of total Δ9-THC, hemp-based food products do not represent any risk to human and animal health (Kladar et al., 2021).
Since 2017, the demand for hemp-based food has grown by 500% (Sorrentino, 2021), causing the intensification of agricultural practices and a substantial increase in the consumption of resources along the supply chain (Amaducci et al., 2015; Sawler et al., 2015; Petit et al., 2020). In the European community, hemp cultivation is included in the European Green Deal objectives because of its contribution to increasing the carbon storage capacity of the agricultural system, breaking of the diseases cycle, preventing soil erosion, and enhancing biodiversity by reducing the use of pesticides (European Commission, 2023).
Although some information regarding the evaluation of the environmental impact of hemp fiber production can be found in the literature, the environmental assessment under different agronomic conditions and for hemp-derived food products (e.g., seeds, oil, and flour) are not sufficiently investigated. Zampori et al. (2013) provided a “from cradle to gate” life cycle assessment (LCA) of thermal insulators from hemp material, emphasizing the greenhouse gas (GHG) emissions along the overall supply chain and the carbon dioxide (CO2) uptake by the plant biomass. Heidari et al. (2019) assessed the environmental impact of innovative bio-based materials (such as hemp shiv) for construction, while Andrianandraina et al. (2015) developed a methodological approach to assess the influence of the parameters of elementary processes in the foreground system of an LCA study and utilized hemp-based insulation materials as a case study. Concerning hemp fiber, Patyk and Reinhardt (1998) conducted a preliminary life cycle analysis of hemp products, including the cultivation, harvest, and pressing of oil for biofuel production, decorticating, steam pressure digestion of fiber, and textile production. Van Der Werf (2004) compared the environmental impact of agricultural practices for different crops including hemp in France. González-García et al. (2010) analyzed the impact associated with the production of hemp and flax fibers for paper pulp. Campiglia et al. (2020) evaluated the environmental impacts of different agronomic practices for hemp seed, focusing on three agricultural variables: the genotypes, plant density, and nitrogen content in fertilizers.
The goal of this paper is to contribute to the ongoing research on the sustainability aspects of the hemp industry from the point of view of circular economy. This objective is achieved by carrying out an environmental impact assessment of the cultivation processes of hemp in Italy, according to a “from cradle to farm gate” life cycle approach. The quantified impact categories are carbon footprint (CF), eutrophication (EP), acidification (AP), and water footprint (WF). CF is selected as a reference indicator, focusing on the CF offset (i.e., the distance from the carbon neutral condition) due to the temporary carbon storage in crop residues left in the field to mineralize the soil.
Three different agronomic methods are examined, i.e., conventional (outdoor and indoor) and organic (outdoor). For each of these, primary data are collected, and all emissions, both direct and indirect, are evaluated. This contributes to the completeness and reliability of the results. Furthermore, the ability of hemp to sequester carbon in the soil (i.e., the contribution by crop residues left in the field) is also accounted for. The balance emission vs. storage reveals the position of hemp in the carbon neutrality scale. In addition, the environmental impacts of the manufacturing and packing processes for hemp-based products (seed oil, flour, and dried flowers) are assessed.
Finally, after hotspot identification, some management practices are proposed and analyzed in terms of impact reduction. Such measures include the utilization and market of all parts of the plant, thus reducing waste and promoting a circular and more sustainable production model (Scrucca et al., 2020; Kaur and Kander, 2023).
2 Materials and methods
2.1 Case studies
Three different case studies are used as proxies for the assessment of the environmental impact of the hemp industry. They differ for agronomic methods (i.e., both conventional outdoor and indoor and organic outdoor) and commercial purposes (i.e., flowers for therapeutics uses and grains for hemp-based food products). All the case studies are located in Italy. A brief description of the hemp life cycles with their specific characteristics, management, operational phases, and outputs is provided below. All the system boundaries are “from cradle to farm gate” (i.e., from the resource’s extraction to the packaged product leaving the farm), while the temporal boundaries are 1 year of agricultural activity.
Conventional outdoor (Figure 1): a medium-sized farm located in Siena (43°18′13.3″ N and 11°22′57.2″ E, Tuscany, central Italy). The final marketable products are seed oil and flour. The cultivar is Finola, a variety that has been bred specifically to produce grains and sometimes fiber and oilseed for food items (Jasinskas et al., 2020). Cultivation takes place outdoor with conventional management, i.e., using fertilizers and without irrigation, due to the low water requirements of hemp. Sowing is carried out in May, and the biomass with ripe grains is harvested in September. Once collected, the biomass (also containing fibers) is deliberately left in the cropland, while the grains are dried and processed to obtain food products. Hempseed oil is obtained by cold pressing the grains, while hemp flour is obtained by grinding the leftovers of hemp oil production. Hempseed oil is packed in 250-mL glass bottles. A plastic film is used for the packing of 1 kg of flour.
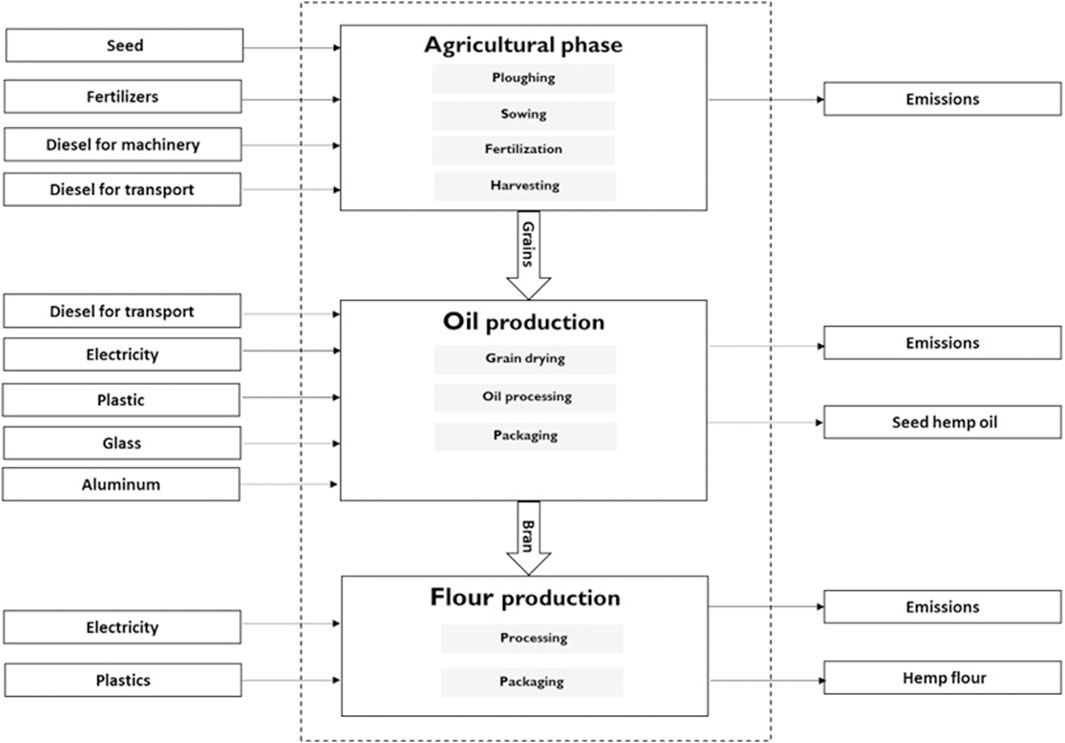
Figure 1. Flowchart of conventional outdoor hemp crops from cultivation to products processing and packaging (Tuscany, Italy).
Organic outdoor (Figure 2): a small farm located in Sovicille (43°15′56.2″ N and 11°14′15.7″ E, Tuscany, central Italy) produces C. sativa for therapeutic uses. Hemp cultivation (cultivar Carmagnola) happens outdoor without irrigation and with extremely limited use of fertilizers. The sowing takes place in May, and the fresh flowers are harvested manually in September using specific scissors. The unused parts of the plant (i.e., biomass) are left in the fields. Fresh flowers are dehydrated naturally and packed in 1-kg plastic buckets.
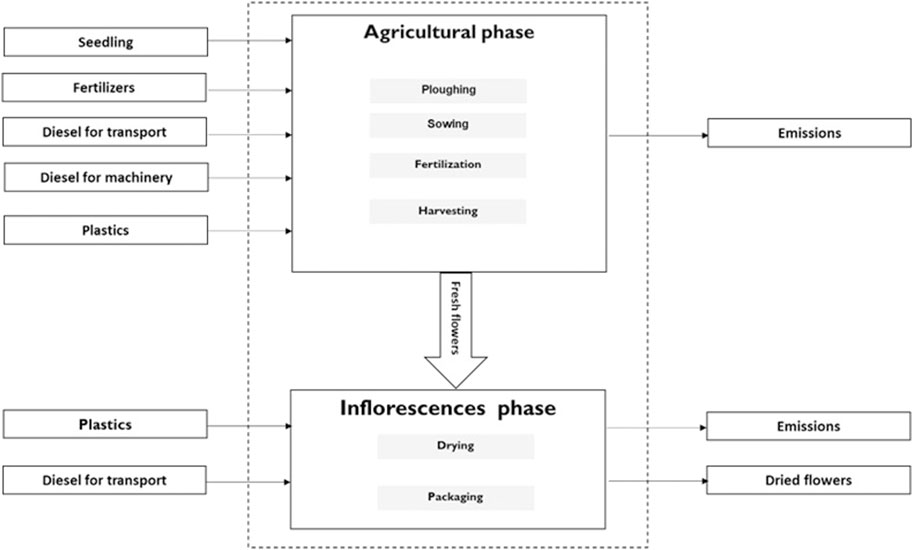
Figure 2. Flowchart of organic outdoor hemp crops from cultivation to dried flowers and packaging (Tuscany, Italy).
Indoor (Figure 3): a farm in Eboli (40°37′01″ N, 15°03′23″ E, Campania, Southern Italy) that produces C. sativa in greenhouses for therapeutic uses. Hemp production (cultivar Carmagnola) takes place indoor, quarterly of a year, and requires a lot of resources and energy to recreate the natural external microclimatic conditions. Prolonged exposure to LED light (up to 18 h a day) increases the photosynthetic capacity and the possibility of achieving excellent vegetative development. At the end of the growing period, the light hours are reduced to 12 h to recreate the autumn conditions and induce the flowering of the plant. Fresh flowers are harvested manually, and the residual biomass is placed in home composters outside the greenhouse. Once harvested, the fresh flowers are dried naturally and packaged in small aluminum boxes (5 g).
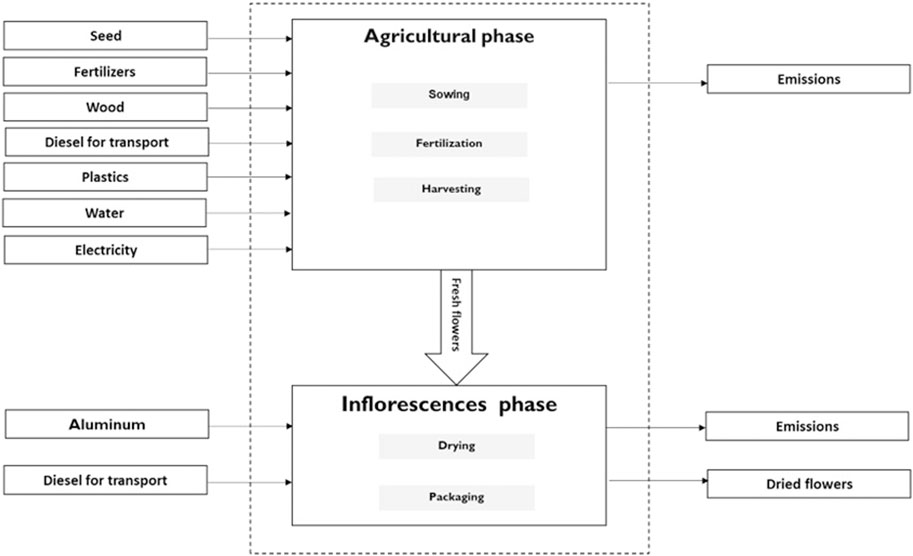
Figure 3. Flowchart of indoor hemp crops from cultivation in pots to dried flowers and packaging (Campania, Italy).
2.2 Data collection and processing
Most of the data about the hemp life cycle were primary, i.e., directly collected from farmers, with the best accuracy, with a bottom-up approach. Data collection referred to: 2018 (conventional outdoor), 2020 (organic outdoor), and 2019 (indoor). The LCA included both direct and indirect GHG emissions due to the upstream processes of obtaining materials, fuels, and all the products used by farmers during 1 year of production (Niccolucci et al., 2021). The calculation was carried out using SimaPro 9.0.0.49 software (Ecoinvent, 2020), Ecoinvent 3.6 database, and by selecting the CML-IA method. The identified impact categories were carbon footprint (CF), eutrophication (EP), acidification (AP), and water footprint (WF).
The following assumptions and approximations made were the following: 1) machinery, equipment, and infrastructures were included in the general cut-off, which ranged from 1% to 5% (Palacios-Munoz et al., 2019). 2) According to the information provided by the owner of the indoor cultivation, agricultural tools (irrigation pipes, wooden poles, and plastic pots) used in the greenhouse were replaced every 3 years (i.e., their lifetime). 3) Diesel consumption for transportation was estimated based on the weight of the carried materials and the traveled distance. 4) Direct emissions deriving from the use of fossil fuels for transport, agricultural machinery, and other devices, as well as due to the fertilizers use and the crop residues left on the field or composted, were included in the calculation by applying the equations framework and emissions factors proposed by the Intergovernmental Panel on Climate Change (IPCC) (IPCC, 2006; IPCC, 2019) and the European Environmental Agency (EMEP/EEA, 2019).
Finally, according to ISO 14044:2006/Amd 2:2020 (ISO, 2020), a mass allocation was adopted to take into account the transformation of hemp seeds into oil (20%) and flour (80%). The inventory was organized in three main phases: 1) agricultural, 2) crop transformation, and 3) product packaging. Two different functional units have been used depending upon the different research question: 1) 1 ha of cultivated land per year; 2) 1 kg of hemp products (i.e., fresh flowers or grains) and relative marketable products (i.e., dried flowers, seed oil, and flour).
The IPCC framework (IPCC, 2006; IPCC, 2019) was adopted as it is a standardized methodology that is valid and replicable at an international level. Carbon footprint offset (CFOFFSET, i.e., the net annual carbon balance) is quantified by subtracting the annual storage (quantified as CO2 STORAGE, i.e., the annual CO2 stock in cropland soil, as in the case of conventional and organic outdoor) from the anthropogenic GHG emissions (quantified as CFTOT and expressed in tons of equivalent carbon dioxide annually emitted, CO2eq, due to the agronomic practices and product processing) (see Eqs 1–3).
The variation in carbon stock in soil was calculated with Equation 1 (IPCC, 2006), which considers the reference carbon storage in 0–30 cm of soil depth
The carbon stock over time will occur primarily during the first 20 years, following the management field practices. After that, the rates will tend toward a new steady-state level, with little or no change occurring unless further changes in management conditions occur (IPCC, 2006).
3 Results and discussion
3.1 Hemp cultivation
The life cycle inventory (LCI) is elaborated as a quantification of all relevant flows coming from (i.e., energy and raw material) and directed to (i.e., direct emissions) the environment, which are needed to support the overall hemp life cycle. In Table 1 the LCI results are presented focusing on the respective agricultural phase. Although this phase is common for all kinds of hemp-based products (i.e., grains and flowers), the crop transformation and packaging phases depend on the type of the products. The inventory is organized in two different functional units, depending on the addressed research purpose. The first FU is 1 ha of cultivated field, and it provides a local perspective for discussing those impacts that produce emissions in the field. Furthermore, this FU is chosen to be used as a reference in comparison with other similar case studies found in the literature. The second FU is 1 kg of products (grains or flowers), and it has a regional and global relevance and is more convenient when comparing agronomic practices.
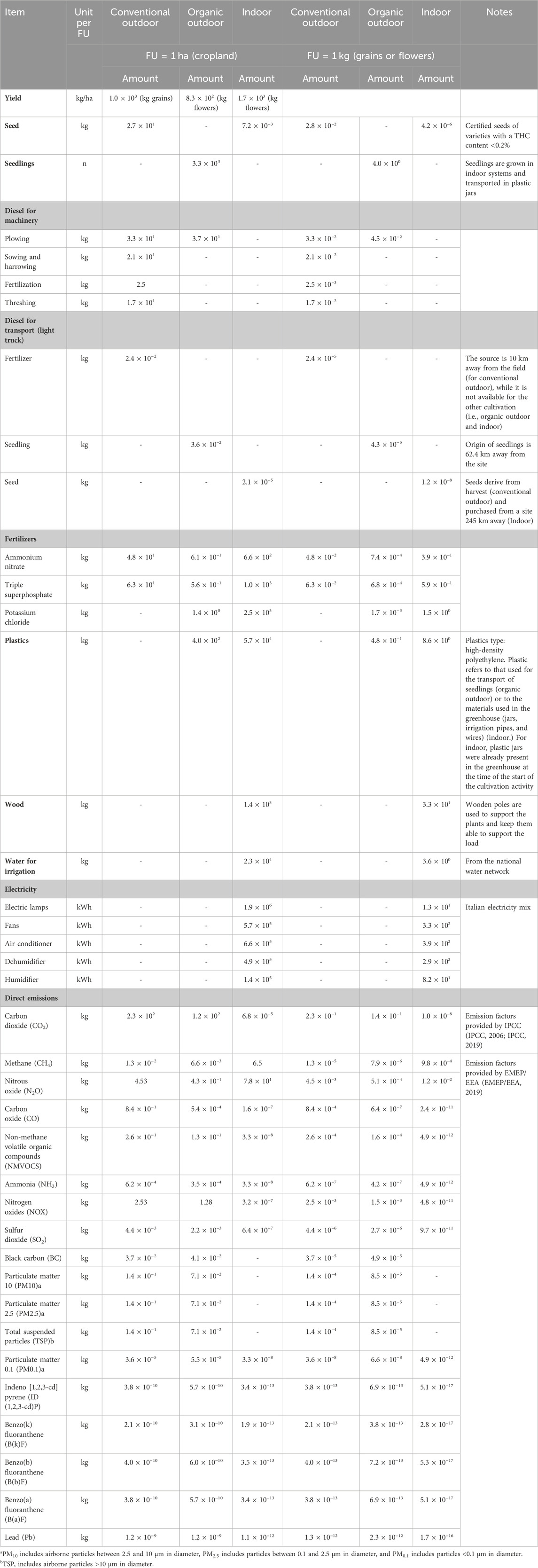
Table 1. LCI of the hemp cultivation phase within the three agronomic practices (conventional outdoor, organic outdoor, and indoor) and two functional units (1 ha of cultivated field and 1 kg of grains for conventional outdoor or of flowers for organic outdoor and indoor).
The most relevant LCA environmental impact results for the three case studies according to the two functional units are reported in Table 2.
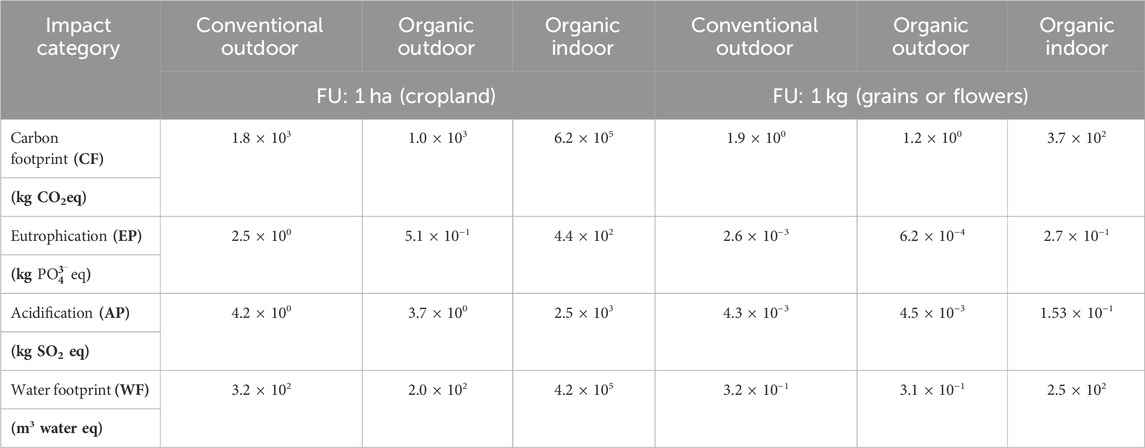
Table 2. Environmental impacts due to hemp production in the three agronomic practices. Results are reported for both the two functional units: 1 ha of cultivated field and 1 kg of grains (conventional outdoor) or flowers (organic outdoor and indoor).
Considering the mass unit as FU, the organic practice shows the lowest impact, while the indoor practice shows the highest, within all the evaluated impact categories. In the case of outdoor practices, the CF is 1.2 kg CO2eq for organic and 1.9 kg CO2eq for conventional practices, with a variation of −37%. The indoor practice is two orders of magnitude larger (3.7 × 102 kg CO2eq), and this is essentially due to the intensive use of fertilizers and, above all, the large electricity requirements. Furthermore, indoor production occurs four times per year.
The CFs show the following different compositions (Figure 4):
• Conventional outdoor practice is dominated by direct emissions to air (66%) due to residual crops, with a moderate contribution from fertilizer production (29%).
• Organic outdoor practice is characterized by a substantial contribution from plastic jars for seedlings (81%), with a marginal contribution from residual crops as direct emissions (16%).
• Conventional indoor practice is predominantly caused by the energy requirements for the operational and maintenance activities of the greenhouses.
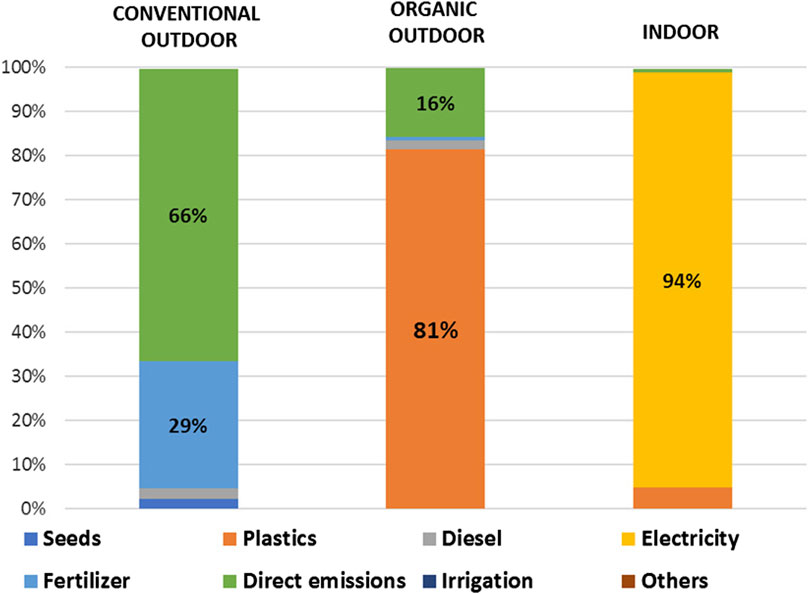
Figure 4. Carbon footprint (CF) composition for the considered agronomic practices (conventional outdoor, organic outdoor, and indoor).
In this study, the emissions are accounted, and the contribution of direct emissions is included for those selected processes from database that do not include them. Direct emissions accounting represents an important added value for this kind of study. Direct contributions from crop residues left in the field or composted, fertilizers, and fossil fuel consumption are separately accounted according to the IPCC framework (IPCC, 2006; IPCC, 2019) and EMEP/EEA (EMEP/EEA, 2019) (see Table 1). This contribution is especially relevant for agricultural products with intensive management, as is also confirmed in this case.
The WF results, calculated as the water scarcity index, show that the water use intensity for indoor practice (250 m3 water eq) is around three orders of magnitude larger than that of outdoor cultivation (0.3 m3 water eq). All the selected impact categories explored (EP, AP, and WF) show a similar percentage composition to those of CF.
The results from FU = 1ha are compared with the available recent literature (see Table 3). This was possible only for conventional outdoor practice. In this study, the CF is in line with those shown in the literature for different European countries. Data variability increases when only the outlier is included, but our value still scores among the lowest (Figure 5). The literature (Van Der Werf, 2004; González-García et al., 2010; Andrianandraina et al., 2015) confirms that the main contributors to CF are the production and use of diesel and fertilizers in addition to crop residues (generally neglected). The differences could be, for example, due to the country of origin, system boundaries, the (partial or total) inclusion of direct emissions, the evaluation methods (i.e., endpoint and middle point), weather conditions, and the prevalent management practices (i.e., the choice of cultivar variety, the rate of fertilization, plant density, and the type of production system). The EP and AP results confirm that conventional outdoor practice has the lowest impact like for CF, even if the variability is low (Figure 5).
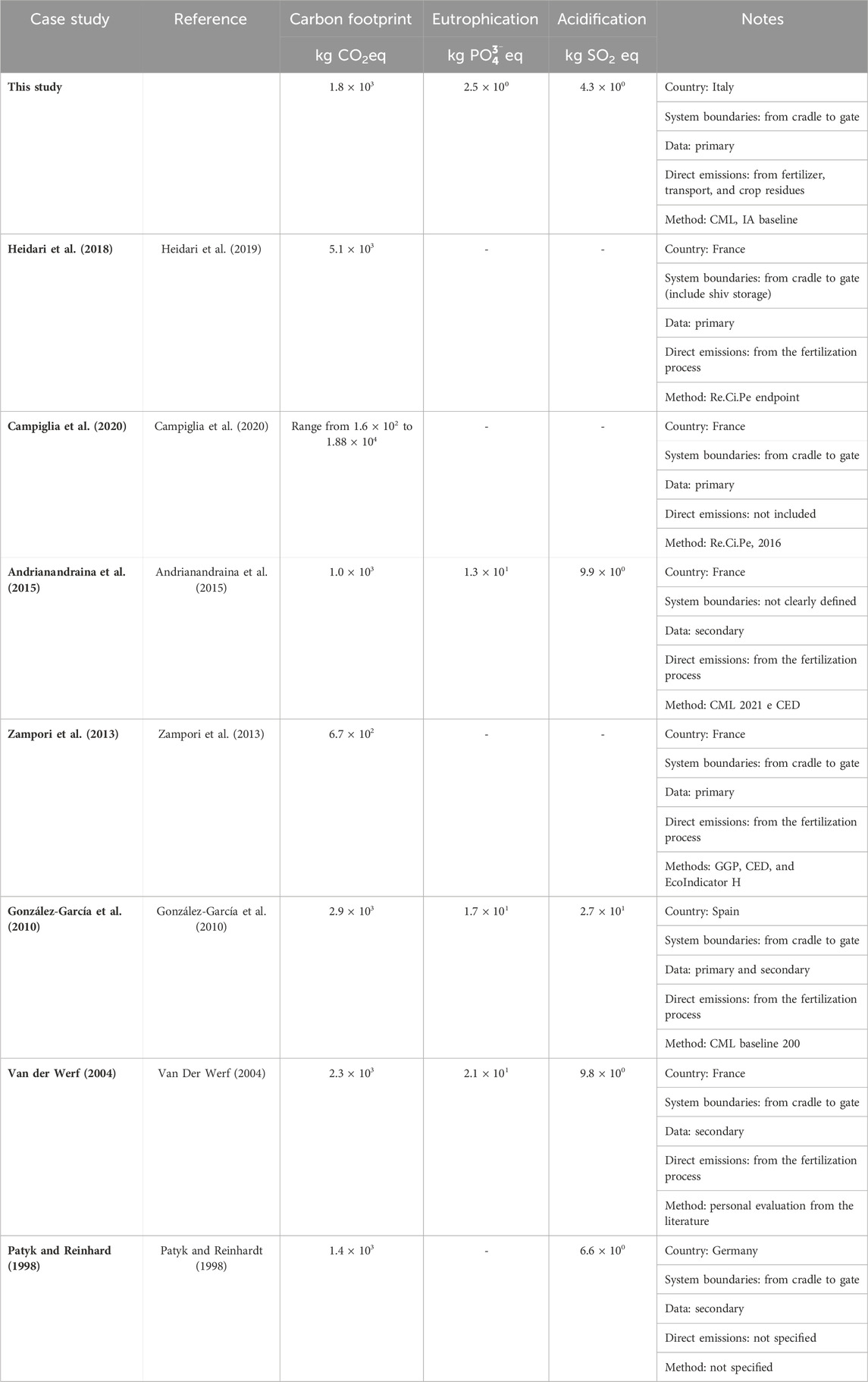
Table 3. Comparison of environmental impacts of the hemp cultivation phase according to conventional outdoor practice (FU 1 ha of cropland) with the existing literature.
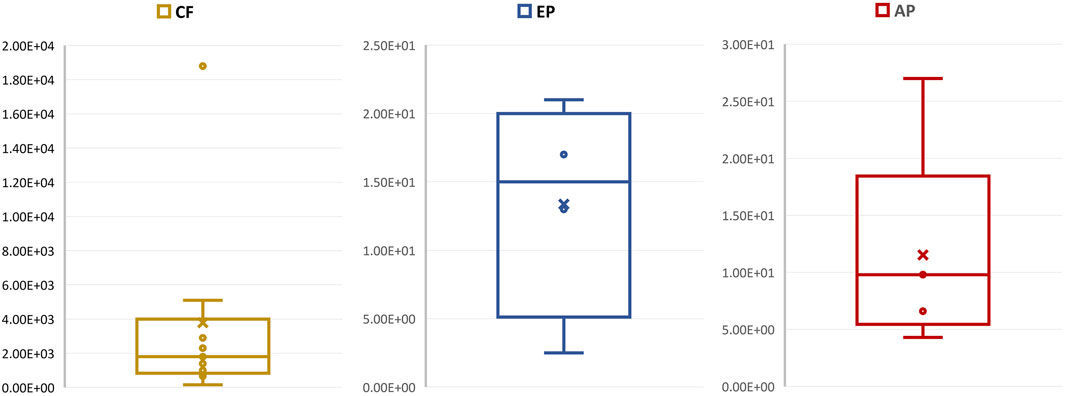
Figure 5. Data variability between this study and other research found in the literature. CF is expressed in kgCO2eq, EP in
For the three agronomic practices, various mitigation scenarios are proposed to promote a more efficient use of natural resources and are discussed in terms of CF management (Table 4).
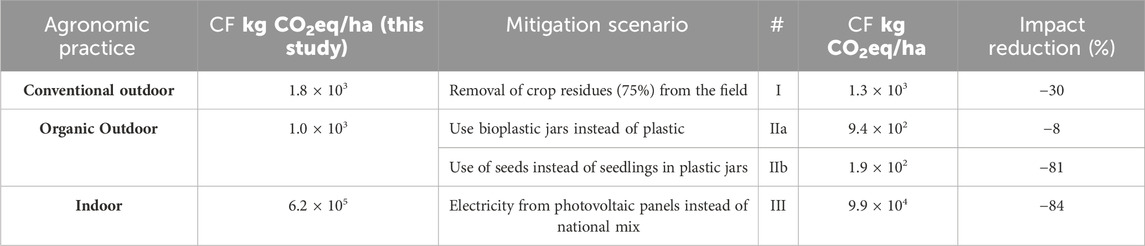
Table 4. Mitigation scenarios for carbon footprint management for each agronomic practice. Results are expressed per kg CO2eq/ha of cropland.
In conventional outdoor practice, crop residues are a hotspot of the system, and as such, it is important to focus preliminarily on their role. Crop residues left in the field (approximately 15 t of dry biomass (ha year)−1) have a natural mulching function, protecting the soil from the disruptive effects of rain, wind, and sun. Their presence on the surface of hardened soils increases the soil fertility, reducing the susceptibility to surface compaction. Another important function of crop residues is the supply of organic matter, following their degradation, with the release of nutritive elements, and the stimulation of biological processes by microorganisms. In this regard, it would be interesting to understand and quantify the ability of residual hemp biomass in reducing the use of fertilizer resulting from this practice. The CO2 stored annually in the soil due to the crop residues left in the field and the CO2eq net emissions due to agronomic practices are evaluated. The yearly carbon stock in soil (with more than 30% of hemp residues) is estimated in −2.07 t CO2 ha-1 because tillage practices are carried out for both conventional outdoor practice, in which hemp seeds are strewn in the field, and organic outdoor practice, in which the installation of seedlings is planned. The carbon stored over a period of 20 years of cultivation is −121 t CO2 ha−1. When considering the conventional and organic outdoor practices, the total CFs of the agricultural phase (1.8 and 1.0 t CO2eq ha−1, respectively) are fully compensated by the biomass fraction stored annually in the ground (−0.27 and −1.07 t CO2eq ha−1, respectively). The largest energy and environmental impacts of hemp cultivation are due to the production and use of the fertilizers and pesticides, contributing to most of the CFs, and are consistent with other studies (Pervaiz and Sain, 2003; Scrucca et al., 2020). Therefore, the practice of leaving crop residues in the field could lead to impact mitigation.
Since hemp is a fibrous plant, crop residues can also be harvested and transformed into consumer products such as textiles and building materials. In this sense, the removal of residual biomass from the field, for example, 75% (scenario I in Table 4), produces a significant reduction in gross carbon emissions (−30%) due to the cultivation phase. Furthermore, according to Zampori et al. (2013), the fraction of crop residues collected in the field would lead to the manufacture of 4.4 × 102 insulation panels composed by hemp (85%) and polyester (15%) fibers. The manufacture of all these panels emits 2.0 × 103 kg CO2eq, accounting for 4.4 kg CO2eq (panel)−1. However, a hemp-based insulating panel impacts 5 times less in terms of CF when compared with a traditional cork one and 10 times less with respect to an expanded clay one (Asdrubali et al., 2015; Essaghouri et al., 2023). The carbon stocked in each hemp-based insulating panel is −8.7 t CO2, representing a semi-permanent storage throughout their life (≥50 years). The CF offset shows a net negative value (−4.2 t CO2 per panels), confirming the carbon neutral condition of this production chain, as claimed by other studies (Ingrao et al., 2015; Scrucca et al., 2020; Liu et al., 2023). Based on these estimations, 1 kg of dried hemp biomass contains 1.7 t CO2 (Struik et al., 2000), and each insulating panel is composed of 5.1 kg of fiber.
For organic outdoor practice, two scenarios are assumed: the use of starch-based bioplastic instead of plastic jars (scenario IIa) for seedlings or the use of seed instead of seedlings (scenario IIb). CF shows a moderate decrease (8%) for starch-based bioplastic and an important reduction (−81%) for seeds.
In the indoor agricultural practice, because of the large contribution of electricity on the overall impact, an agri-voltaic scenario is proposed, i.e., a 100% renewable source (i.e., photovoltaic panels) instead of the current Italian energy mix (almost 40% renewable) (scenario III). To cover the annual energy consumption (3.7 × 106 kWh), the greenhouse would use the electricity produced by 1.1 × 103 photovoltaic panels of 3 kW (taking up approximately 2 ha under the hypothesis to develop a local energy community comprising all the farmers of the area).
3.2 Hemp-based products
A separate inventory for hemp-based products is elaborated, including the processing and packaging phases.
Regarding flour and oil processing (Table 5), two inputs are accounted: diesel (used during the transport of hemp seeds from the field to the processing plant) and electricity (used during the transformation processes). Regarding the packaging, only the raw materials are accounted, for while their transportation is not considered because they are purchased in the same place where the processing and bottling of the final oil product take place.
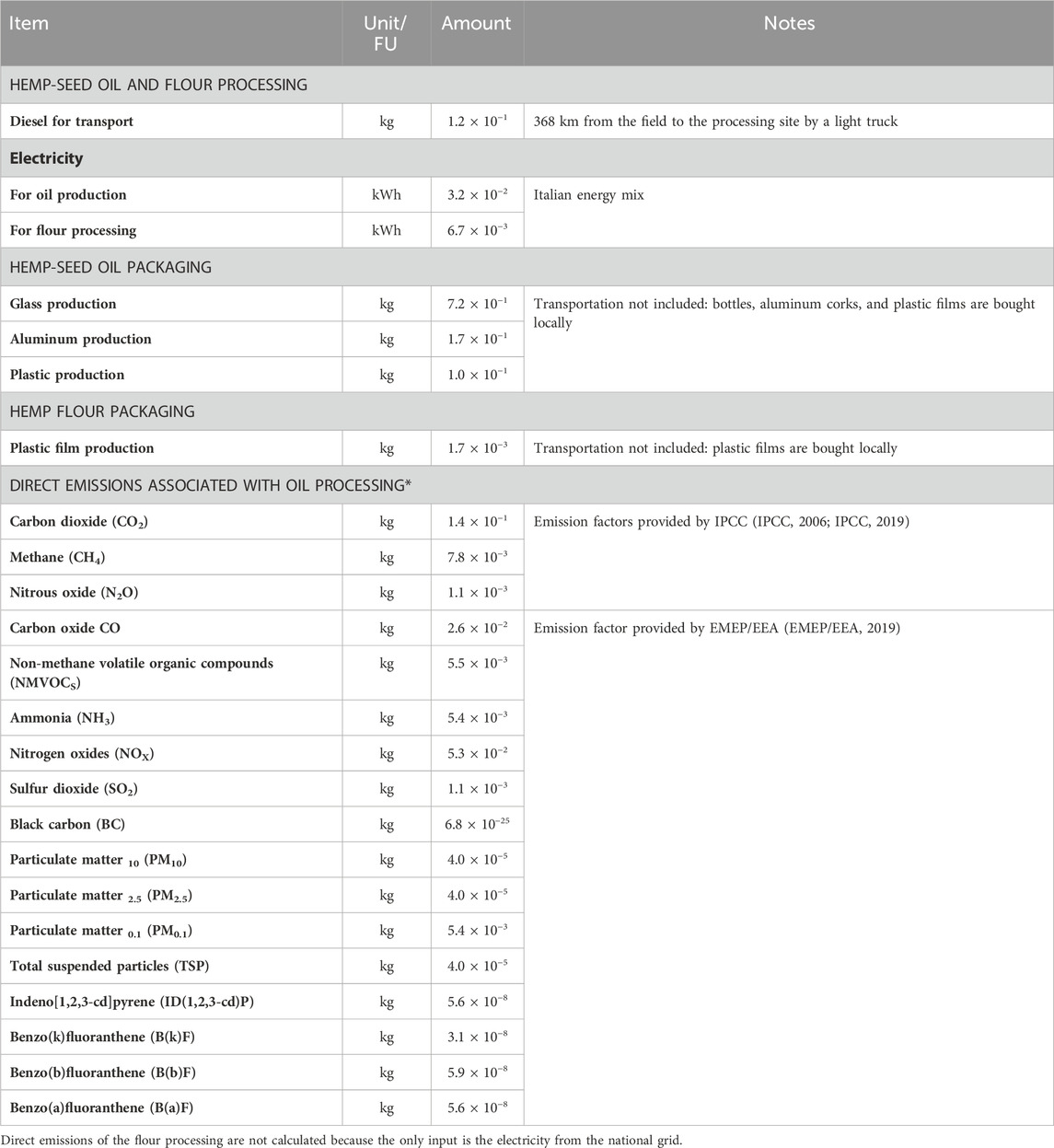
Table 5. Data inventory associated with processing and packaging of oil and flour from conventional outdoor practice. Functional unit: 1 kg of oil and flour.
The dried flowers are packaged using different materials depending on the practice used (i.e., organic outdoor or indoor) (Table 6).
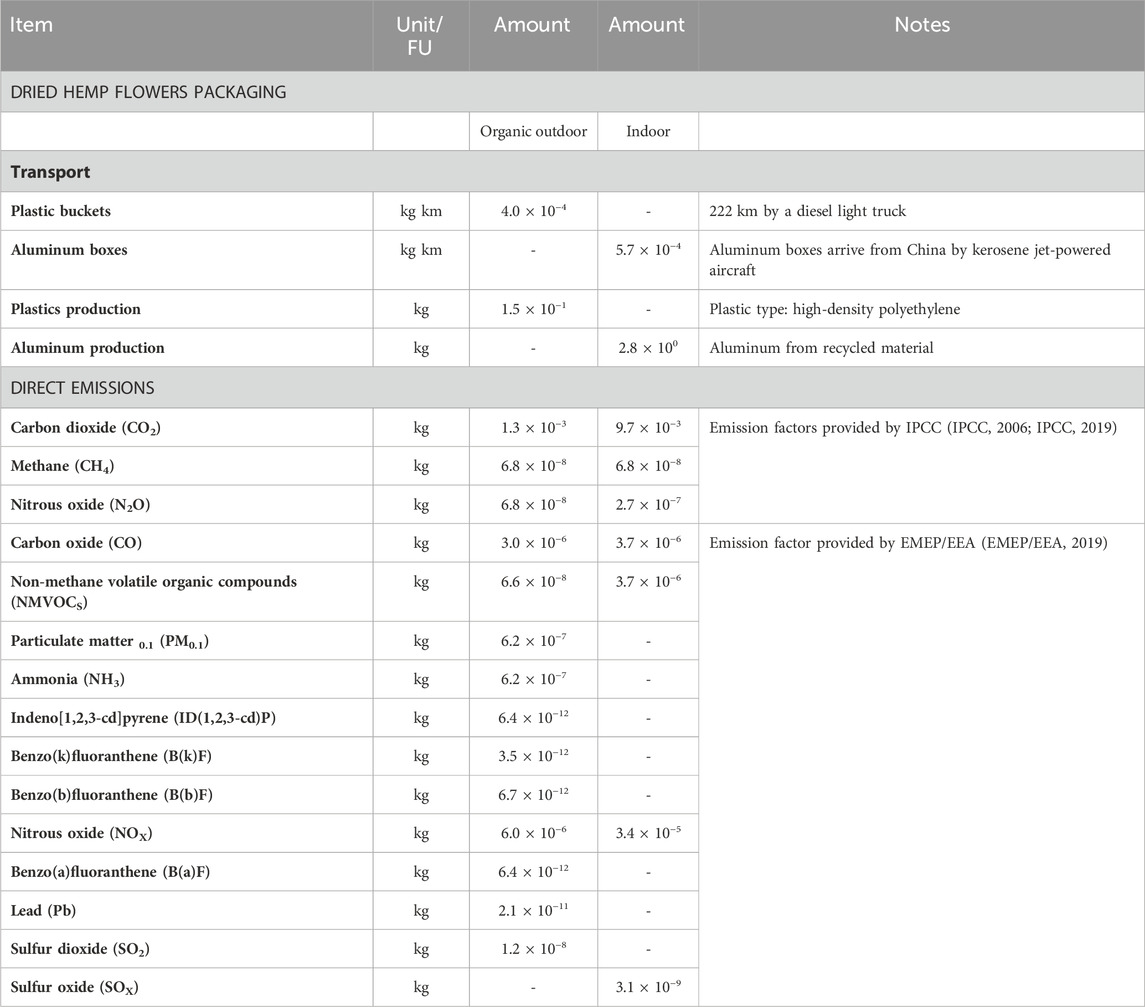
Table 6. Data inventory associated with the processing and packaging of dried flowers. Functional unit: 1 kg of dried flowers.
The environmental impacts of the production of 1 kg of hemp-seed oil and 1 kg of flour are shown in Table 7.
The CF is 26 kgCO2eq kg-1 for oil, while it is 33 kgCO2eq kg−1 for flour. Due to the lack of specific literature reports, other kinds of flour and oil are used for comparison (Table 8). The CF of hemp-based food products is one order of magnitude higher than the others. This could be due to a very low yield of hemp seed (1 t ha−1) with respect to winter wheat (yield 9.7 Mt ha−1) and winter rye (8.5 Mt ha−1) (Baldini et al., 2019; Riedesel et al., 2022). In addition, the transformation and processing of hemp oil has a low yield (1 L requires 5 kg of seeds) when compared with other kinds of oil (Rapa et al., 2019). The choice of the packaging format (250 mL bottles) is an important aspect, causing a relevant variation in the total impact. Hemp generally requires low inputs demand but also has a low oil yield (Bernas et al., 2021).
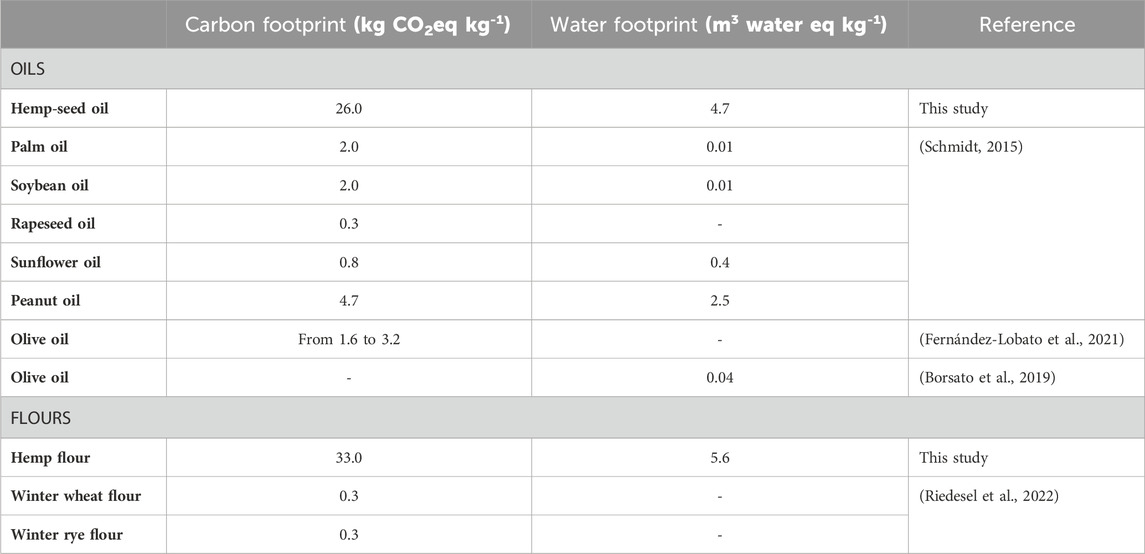
Table 8. Environmental impacts from hemp oil anf flour production, compared with the existing literature referring to other kinds of oils and flours.
The CF composition of hemp-based food products is dominated by the agricultural phase (90%), with a lower contribution from packaging (10%).
The WF shows the highest value when compared to the literature due to the low yield for both hemp oil and flour.
Regarding flowers, the impacts of the indoor production are slightly larger than that of organic outdoor (Table 9), and our results are a thousand times smaller than the literature. Summers et al. (2021) analyzed the energy and materials required to grow hemp indoors and quantified the corresponding greenhouse gas (GHG) emissions using LCA for a cradle-to-gate system boundary. The analysis was performed across the United States, and the resulting life cycle GHG emissions range from 2.2 × 103 to 5.1 × 103 kg CO2eq per kg of dried flowers, depending on the location. Mills (2012) estimated that when performed indoor, the production of 1 kg of dried flowers is associated with 4.6 × 103 kg of carbon dioxide emissions into the atmosphere.
The difference between the CF results in our study and in the literature can be justified by considering the following points:
• System boundary: the literature studies include the transport of workers and those of huge quantities of hemp flowers to the warehouses and the redistribution over long distances before the final sale.
• Data source: our study mainly used primary data, while the literature papers are based mostly on secondary (based on public-domain sources) data.
• Kind of indoor production: the case from the United States is much more energy intensive than those in Italy. Production takes place five times a year (four in our case) to obtain high yields (5,000 kg ha-1 year−1). A larger amount of electricity is used and is combined with natural gas to ensure suitable conditions in greenhouses. CO2 is injected to increase foliage growth, and due to the large scale of production, electricity is also used during the drying process (in our case, drying occurs under natural conditions).
Regarding the packaging of the flowers, the impact categories comparison shows that for the packaging of 1 kg of dried flowers, the use of plastic boxes has a slightly higher impact than the use of recycled aluminum boxes.
Moreover, in the case of dried flowers, as for hemp oil and flour, the CF composition is mainly due to the hemp cultivation (about 90%), with a lower contribution of the packaging (10%).
Table 10 shows the CF offset of dried flowers from organic outdoor practice and hemp-based food products from conventional outdoor practice because in these cases, the crop residues are left in the soil, creating a temporary carbon storage. In the indoor condition, on the other hand, biomass residues are composted and then exit the system boundary. The temporary storage is represented by the annual rate of carbon contained in crop residues, which is stabilized in the soil during continuous cultivation cycles (20 years). This value is quantified considering the cropland surface needed to obtain 1 kg of product. The production of 1 kg of dried flowers, cultivated organically outdoor, naturally processed, and packaged in plastic buckets, is carbon-neutral (−0.99 kg CO2) when the temporary carbon storage in soil is included. On the other hand, 1 kg of hemp-seed oil and flour have annual CFs higher than the rate of carbon storage in soil due to the more impactful processes to obtain hemp-based food products (23.41 kg CO2 and 31.79 kg CO2, respectively).
When properly arranged, carbon storage represents a useful tool for developing mitigation strategies and guidelines for supporting the consumers’ choice. It is opportune to keep in mind that sequestration should be guaranteed over a long period (≥100 years) and not just for 1 year. In many cases, the soil carbon storage could be lost if changes in cropland management or climate effects lead to lower organic matter inputs or increased microbial activity (Paul et al., 2023).
4 Conclusion
The hemp life cycle is quite articulated and complex and provides a number of positive ecosystem services like, among others, carbon uptake and storage, pollination, fertility, heavy-metal absorption, and biodiversity. Furthermore, virtually, every part of the hemp plant has a potential application and can be used to manufacture a variety of marketable products like food items, construction materials, pharmaceuticals, and textiles. Due to the growing importance of hemp in recent years, this paper assesses its environmental potential as a carbon storage plant and the relevance of its production chain.
Three different case studies, representing the Italian industry, are analyzed and compared. An environmental impact profile is then defined through life cycle assessment and a set of mainly primary data.
Considering the mass unit as FU, the organic cultivation practice registers the lowest impact, while the indoor practice shows the highest impact in all the evaluated impact categories (CF, AP, EP, and WF).
Furthermore, carbon footprint offset is evaluated by comparing the carbon footprint (i.e., the direct and indirect emissions in agricultural and transformation phases) with the temporary carbon storage in soil (i.e., the stock due to crop residues in the field when practiced). The cultivation phase provides a CF that ranks from 1.2 (organic outdoor) to 374 (indoor) kg CO2eq per kg of flowers or 1.9 kg CO2eq per kg of grains (conventional outdoor). The ability of hemp to sequester carbon in the soil due to crop residues left in the field is evaluated as −2.7 kg CO2 (ha year)−1; this value effectively neutralizes the CF of the agricultural phase for both conventional and organic outdoor practices in the first year.
Dried flowers show a negative balance (−0.99 kg CO2 per kg dry flower) only when carbon storage due to crop residues in soil is included. Emissions from hemp oil and flour are not compensated, reporting positive values (23.41 kg CO2 per kg oil and 31.79 kg CO2 per kg flour).
Under a perspective of circular economy, a scenario based on the use of hemp biomass for insulating panels allows the appreciation of the advantage of fixing carbon in durable goods. As such, the hemp industry can be considered a clear example of a fully circular and sustainable production chain.
Data availability statement
The original contributions presented in the study are included in the article/Supplementary material; further inquiries can be directed to the corresponding author.
Author contributions
MMK: data curation, formal analysis, investigation, methodology, software, visualization, and writing—original draft. MM: conceptualization, data curation, formal analysis, investigation, methodology, writing—original draft, and writing–review and editing. EN: data curation, software, and writing–original draft. NM: conceptualization, supervision, and writing–review and editing. VN: conceptualization, data curation, formal analysis, investigation, methodology, supervision, visualization, writing–original draft, and writing–review and editing.
Funding
The author(s) declare that financial support was received for the research, authorship, and/or publication of this article. This study is carried out within the Agritech National Research Center and received funding from the European Union Next-Generation EU (PIANO NAZIONALE DI RIPRESA E RESILIENZA (PNRR)—MISSIONE 4 COMPONENTE 2, INVESTIMENTO 1.4—D.D. 1032 17/06/2022, CN00000022). This manuscript reflects only the authors’ views and opinions, and neither the European Union nor the European Commission can be considered responsible for them. The publication was made possible thanks to the specific contribution of the University of Siena for the support of Open Access and funds from the Department of Physical Sciences, Earth and Environment.
Acknowledgments
The authors thank Gaia Esposito and Cosimo Montefrancesco for their precious support and the three anonymous farmers for providing data and all the precious information.
Conflict of interest
The authors declare that the research was conducted in the absence of any commercial or financial relationships that could be construed as a potential conflict of interest.
Publisher’s note
All claims expressed in this article are solely those of the authors and do not necessarily represent those of their affiliated organizations, or those of the publisher, the editors, and the reviewers. Any product that may be evaluated in this article, or claim that may be made by its manufacturer, is not guaranteed or endorsed by the publisher.
References
Adesina, I., Bhowmik, A., Sharma, H., and Shahbazi, A. (2020). A review on the current state of knowledge of growing conditions, agronomic soil health practices and utilities of hemp in the United States. Agriculture 10 (4), 129. doi:10.3390/agriculture10040129
Allegret, S. (2013). “The history of hemp,” in Hemp: industrial production and uses. Editors P. Bouloc, S. Allegret, and L. Arnaud (UK: CABI), 4–26. Available at: http://www.cabidigitallibrary.org/doi/10.1079/9781845937935.0004.
Amaducci, S., Colauzzi, M., Bellocchi, G., and Venturi, G. (2008). Modelling post-emergent hemp phenology (Cannabis sativa L.): theory and evaluation. Eur. J. Agron. 28 (2), 90–102. doi:10.1016/j.eja.2007.05.006
Amaducci, S., Scordia, D., Liu, F. H., Zhang, Q., Guo, H., Testa, G., et al. (2015). Key cultivation techniques for hemp in Europe and China. Industrial Crops Prod. 68, 2–16. doi:10.1016/j.indcrop.2014.06.041
Andrianandraina, V. A., Senga Kiessé, T., Cazacliu, B., Idir, R., and Van Der Werf, H. M. G. (2015). Sensitivity analysis of environmental process modeling in a life cycle context: a case study of hemp crop production. J Industrial Ecol. 19 (6), 978–993. doi:10.1111/jiec.12228
Asdrubali, F., D’Alessandro, F., and Schiavoni, S. (2015). A review of unconventional sustainable building insulation materials. Sustain. Mater. Technol. 4, 1–17. doi:10.1016/j.susmat.2015.05.002
Baldini, M., Zuliani, F., Cattivello, C., and Barbiani, G. (2019). Nuove indicazioni per la coltivazione della canapa in Friuli Venezia Giulia. Available at: https://www.ersa.fvg.it/export/sites/ersa/aziende/in-formazione/notiziario/allegati/2019/1/2_Nuove-coltivazioni-della-canapa.pdf.
Bernas, J., Bernasová, T., Nedbal, V., and Neugschwandtner, R. W. (2021). Agricultural LCA for food oil of winter rapeseed, sunflower, and hemp, based on Czech standard cultivation practices. Agronomy 11 (11), 2301. doi:10.3390/agronomy11112301
Bocsa, I., Bosca, I., and Karus, M. (1998). The cultivation of hemp: botany, varieties, cultivation and harvesting. Available at: https://www.semanticscholar.org/paper/The-Cultivation-of-Hemp%3A-Botany%2C-Varieties%2C-and-Bocsa-Bosca/05d7ce569a509e6be4ac2c4b88d2d3e311f93486.
Borsato, E., Giubilato, E., Zabeo, A., Lamastra, L., Criscione, P., Tarolli, P., et al. (2019). Comparison of water-focused life cycle assessment and water footprint assessment: the case of an Italian wine. Sci. Total Environ. 666, 1220–1231. doi:10.1016/j.scitotenv.2019.02.331
Ćaćić, M., Perčin, A., Zgorelec, Ž., and Kisić, I. (2019). Evaluation of heavy metals accumulation potential of hemp (Cannabis sativa L.). J. Cent. Eur. Agric. 20 (2), 700–711. doi:10.5513/jcea01/20.2.2201
Campiglia, E., Gobbi, L., Marucci, A., Rapa, M., Ruggieri, R., and Vinci, G. (2020). Hemp seed production: environmental impacts of cannabis sativa L. Agronomic practices by life cycle assessment (LCA) and carbon footprint methodologies. Sustainability 12 (16), 6570. doi:10.3390/su12166570
Campiglia, E., Radicetti, E., and Mancinelli, R. (2017). Plant density and nitrogen fertilization affect agronomic performance of industrial hemp (Cannabis sativa L.) in Mediterranean environment. Industrial Crops Prod. 100, 246–254. doi:10.1016/j.indcrop.2017.02.022
Citterio, S., Prato, N., Fumagalli, P., Aina, R., Massa, N., Santagostino, A., et al. (2005). The arbuscular mycorrhizal fungus Glomus mosseae induces growth and metal accumulation changes in Cannabis sativa L. Chemosphere 59 (1), 21–29. doi:10.1016/j.chemosphere.2004.10.009
Dowling, C. A., Melzer, R., and Schilling, S. (2021). Timing is everything: the genetics of flowering time in Cannabis sativa. Biochem. 43 (3), 34–38. doi:10.1042/bio_2021_138
Ecoinvent (2020). Swiss centre for life-cycle inventories. Ecoinvent database - ecoinvent. Available at: https://ecoinvent.org/the-ecoinvent-database/.
EMEP/EEA (2019). EMEP/EEA air pollutant emission inventory guidebook 2019 — European Environment Agency. Available at: https://www.eea.europa.eu/publications/emep-eea-guidebook-2019.
Essaghouri, L., Mao, R., and Li, X. (2023). Environmental benefits of using hempcrete walls in residential construction: an LCA-based comparative case study in Morocco. Environ. Impact Assess. Rev. 100, 107085. doi:10.1016/j.eiar.2023.107085
European Commission (2023). Hemp. Available at: https://agriculture.ec.europa.eu/farming/crop-productions-and-plant-based-products/hemp_en.
Farinon, B., Molinari, R., Costantini, L., and Merendino, N. (2022). “Biotechnological transformation of hempseed in the food industry,” in Cannabis/hemp for sustainable agriculture and materials. Editors D. C. Agrawal, R. Kumar, and M. Dhanasekaran (Singapore: Springer), 163–202. Available at: https://link.springer.com/10.1007/978-981-16-8778-5_7.
Faux, A. M., Draye, X., Lambert, R., d’Andrimont, R., Raulier, P., and Bertin, P. (2013). The relationship of stem and seed yields to flowering phenology and sex expression in monoecious hemp (Cannabis sativa L.). Eur. J. Agron. 47, 11–22. doi:10.1016/j.eja.2013.01.006
Fernández-Lobato, L., García-Ruiz, R., Jurado, F., and Vera, D. (2021). Life cycle assessment, C footprint and carbon balance of virgin olive oils production from traditional and intensive olive groves in southern Spain. J. Environ. Manag. 293, 112951. doi:10.1016/j.jenvman.2021.112951
Flicker, N. R., Poveda, K., and Grab, H. (2020). The bee community of cannabis sativa and corresponding effects of landscape composition. Environ. Entomol. 49 (1), 197–202. doi:10.1093/ee/nvz141
González-García, S., Hospido, A., Feijoo, G., and Moreira, M. T. (2010). Life cycle assessment of raw materials for non-wood pulp mills: hemp and flax. Resour. Conservation Recycl. 54 (11), 923–930. doi:10.1016/j.resconrec.2010.01.011
Gryndler, M., Sudová, R., Püschel, D., Rydlová, J., Janoušková, M., and Vosátka, M. (2008). Cultivation of high-biomass crops on coal mine spoil banks: can microbial inoculation compensate for high doses of organic matter? Bioresour. Technol. 99 (14), 6391–6399. doi:10.1016/j.biortech.2007.11.059
Heidari, M. D., Lawrence, M., Blanchet, P., and Amor, B. (2019). Regionalised life cycle assessment of bio-based materials in construction; the case of hemp shiv treated with sol-gel coatings. Materials 12 (18), 2987. doi:10.3390/ma12182987
Ingrao, C., Lo Giudice, A., Bacenetti, J., Tricase, C., Dotelli, G., Fiala, M., et al. (2015). Energy and environmental assessment of industrial hemp for building applications: a review. Renew. Sustain. Energy Rev. 51, 29–42. doi:10.1016/j.rser.2015.06.002
IPCC (2006). 2006 IPCC guidelines for national greenhouse gas inventories — IPCC. Available at: https://www.ipcc.ch/report/2006-ipcc-guidelines-for-national-greenhouse-gas-inventories/.
IPCC (2019). 2019 refinement to the 2006 IPCC guidelines for national greenhouse gas inventories — IPCC. Available at: https://www.ipcc.ch/report/2019-refinement-to-the-2006-ipcc-guidelines-for-national-greenhouse-gas-inventories/.
ISO (2020). International Standard published. ISO 14044:2006/Amd 2:2020 Environmental management Life cycle assessment Requirements and guidelines. Available at: https://www.iso.org/standard/76122.html.
Jasinskas, A., Streikus, D., and Vonžodas, T. (2020). Fibrous hemp (Felina 32, USO 31, Finola) and fibrous nettle processing and usage of pressed biofuel for energy purposes. Renew. Energy 149, 11–21. doi:10.1016/j.renene.2019.12.007
Journals, I., and Dalio, Dr. J. S. (2014). Cannabis sativa-an important subsistence pollen source for Apis mellifera. Available at: http://figshare.com/articles/Cannabis_sativa_An_Important_Subsistence_Pollen_Source_for_Apis_mellifera_/1134447.
Kaur, G., and Kander, R. (2023). The sustainability of industrial hemp: a literature review of its economic, environmental, and social sustainability. Sustainability 15 (8), 6457. doi:10.3390/su15086457
Kladar, N., Čonić, B. S., Božin, B., and Torović, L. (2021). European hemp-based food products – health concerning cannabinoids exposure assessment. Food control., 129, 108233. doi:10.1016/j.foodcont.2021.108233
Liu, J., Liu, S., Zhu, L., Sun, L., Zhang, Y., Li, X., et al. (2023). Carbon neutrality potential of textile products made from plant-derived fibers. Sustainability 15 (9), 7070. doi:10.3390/su15097070
Mercuri, A. M., Accorsi, C. A., and Mazzanti, M. B. (2002). The long history of Cannabis and its cultivation by the Romans in central Italy, shown by pollen records from Lago Albano and Lago di Nemi. Veg. Hist. Archaeobotany 11 (4), 263–276. doi:10.1007/s003340200039
Mills, E. (2012). The carbon footprint of indoor Cannabis production. Energy Policy 46, 58–67. doi:10.1016/j.enpol.2012.03.023
Niccolucci, V., Marchi, M., Neri, E., Pulselli, R. M., Bastianoni, S., and Marchettini, N. (2021). Insights into nitrogen footprint accounting for products and application to an organic pig farm. Ecol. Indic. 133, 108411. doi:10.1016/j.ecolind.2021.108411
Palacios-Munoz, B., Peuportier, B., Gracia-Villa, L., and López-Mesa, B. (2019). Sustainability assessment of refurbishment vs. new constructions by means of LCA and durability-based estimations of buildings lifespans: a new approach. Build. Environ. 160, 106203. doi:10.1016/j.buildenv.2019.106203
Patyk, A., and Reinhardt, G. A. (1998). Life cycle assessment of hemp products. Life Cycle Assess. hemp Prod., 39–44.
Paul, C., Bartkowski, B., Dönmez, C., Don, A., Mayer, S., Steffens, M., et al. (2023). Carbon farming: are soil carbon certificates a suitable tool for climate change mitigation? J. Environ. Manag. 330, 117142. doi:10.1016/j.jenvman.2022.117142
Pervaiz, M., and Sain, M. M. (2003). Carbon storage potential in natural fiber composites. Resour. Conservation Recycl. 39 (4), 325–340. doi:10.1016/s0921-3449(02)00173-8
Petit, J., Salentijn, E. M. J., Paulo, M. J., Thouminot, C., Van Dinter, B. J., Magagnini, G., et al. (2020). Genetic variability of morphological, flowering, and biomass quality traits in hemp (cannabis sativa L.). Front. Plant Sci. 11, 102. doi:10.3389/fpls.2020.00102
Rapa, M., Ciano, S., Rocchi, A., D’Ascenzo, F., Ruggieri, R., and Vinci, G. (2019). Hempseed oil quality parameters: optimization of sustainable methods by miniaturization. Sustainability 11 (11), 3104. doi:10.3390/su11113104
Riedesel, L., Laidig, F., Hadasch, S., Rentel, D., Hackauf, B., Piepho, H. P., et al. (2022). Breeding progress reduces carbon footprints of wheat and rye. J. Clean. Prod. 377, 134326. doi:10.1016/j.jclepro.2022.134326
Salentijn, E. M. J., Zhang, Q., Amaducci, S., Yang, M., and Trindade, L. M. (2015). New developments in fiber hemp (Cannabis sativa L.) breeding. Industrial Crops Prod. 68, 32–41. doi:10.1016/j.indcrop.2014.08.011
Sawler, J., Stout, J. M., Gardner, K. M., Hudson, D., Vidmar, J., Butler, L., et al. (2015). The genetic structure of marijuana and hemp. PLoS ONE 10 (8), e0133292. doi:10.1371/journal.pone.0133292
Schmidt, J. H. (2015). Life cycle assessment of five vegetable oils. J. Clean. Prod. 87, 130–138. doi:10.1016/j.jclepro.2014.10.011
Scrucca, F., Ingrao, C., Maalouf, C., Moussa, T., Polidori, G., Messineo, A., et al. (2020). Energy and carbon footprint assessment of production of hemp hurds for application in buildings. Environ. Impact Assess. Rev. 84, 106417. doi:10.1016/j.eiar.2020.106417
Skoglund, G., Nockert, M., and Holst, B. (2013). Viking and early middle ages northern scandinavian textiles proven to be made with hemp. Sci. Rep. 3 (1), 2686. doi:10.1038/srep02686
Sorrentino, G. (2021). Introduction to emerging industrial applications of cannabis (Cannabis sativa L.). Rend. Fis. Acc. Lincei 32 (2), 233–243. doi:10.1007/s12210-021-00979-1
Struik, P. C., Amaducci, S., Bullard, M. J., Stutterheim, N. C., Venturi, G., and Cromack, H. T. H. (2000). Agronomy of fibre hemp (Cannabis sativa L.) in Europe. Industrial Crops Prod. 11 (2–3), 107–118. doi:10.1016/s0926-6690(99)00048-5
Summers, H. M., Sproul, E., and Quinn, J. C. (2021). The greenhouse gas emissions of indoor cannabis production in the United States. Nat. Sustain 4 (7), 644–650. doi:10.1038/s41893-021-00691-w
Teterycz, D., Sobota, A., Przygodzka, D., and Łysakowska, P. (2021). Hemp seed (Cannabis sativa L.) enriched pasta: physicochemical properties and quality evaluation. PLoS ONE 16 (3), e0248790. doi:10.1371/journal.pone.0248790
The Angiosperm Phylogeny Group (1998). An ordinal classification for the families of flowering plants. Ann. Mo. Botanical Gard. 85 (4), 531–553.
Todde, G., Carboni, G., Marras, S., Caria, M., and Sirca, C. (2022). Industrial hemp (Cannabis sativa L.) for phytoremediation: energy and environmental life cycle assessment of using contaminated biomass as an energy resource. Sustain. Energy Technol. Assessments 52, 102081. doi:10.1016/j.seta.2022.102081
Van Der Werf, H. M. G. (2004). Life Cycle Analysis of field production of fibre hemp, the effect of production practices on environmental impacts. Euphytica 140 (1–2), 13–23. doi:10.1007/s10681-004-4750-2
Yano, H., and Fu, W. (2023). Hemp: a sustainable plant with high industrial value in food processing. Foods 12 (3), 651. doi:10.3390/foods12030651
Keywords: life cycle assessment, agrifood, hemp (Cannabis sativa L.), environmental impact, carbon footprint, carbon storage
Citation: Meffo Kemda M, Marchi M, Neri E, Marchettini N and Niccolucci V (2024) Environmental impact assessment of hemp cultivation and its seed-based food products. Front. Environ. Sci. 12:1342330. doi: 10.3389/fenvs.2024.1342330
Received: 21 November 2023; Accepted: 15 April 2024;
Published: 10 May 2024.
Edited by:
Chenxi Li, Xi’an University of Architecture and Technology, ChinaReviewed by:
Martí Rufí-Salís, 2.-0 LCA Consultants, SpainAlexander N. Ignatov, Peoples’ Friendship University of Russia, Russia
Joseph Onyango Gweyi, Kenyatta University, Kenya
Copyright © 2024 Meffo Kemda, Marchi, Neri, Marchettini and Niccolucci. This is an open-access article distributed under the terms of the Creative Commons Attribution License (CC BY). The use, distribution or reproduction in other forums is permitted, provided the original author(s) and the copyright owner(s) are credited and that the original publication in this journal is cited, in accordance with accepted academic practice. No use, distribution or reproduction is permitted which does not comply with these terms.
*Correspondence: Nadia Marchettini, bmFkaWEubWFyY2hldHRpbmlAdW5pc2kuaXQ=