- 1Department of Applied and Landscape Ecology, Faculty of AgriScience, Mendel University in Brno, Brno, Czechia
- 2Department of Agrochemistry, Soil Science, Microbiology and Plant Nutrition, Faculty of AgriScience, Mendel University in Brno, Brno, Czechia
- 3Department of Agricultural, Food and Environmental Engineering, Faculty of AgriScience, Mendel University in Brno, Brno, Czechia
- 4Department of Chemistry and Biochemisty, Faculty of AgriScience, Mendel University in Brno, Brno, Czechia
- 5Department of Landscape Planning, Faculty of Horticulture, Mendel University in Brno, Brno, Czechia
The Hranice hypogenic karst region includes urban, spa and agricultural areas and industry complexes that affect water quality in the region. Emerging organic contaminants (EOCs), especially pesticides and pharmaceuticals, are released into the complex aquatic system. These substances and their metabolites can affect aquatic and human life, as well as the regional development of the wider area traditionally associated with the spa. In this study, we conducted preliminary screening for pesticides and pharmaceuticals at 33 sampling sites and across different location types, including surface water, drainage water, a shallow well, groundwater and thermal karst water. Sampling occurred between February 2022 and June 2023. The results generally confirm that current land use is causing pollution in the karst system. The monitored substances were present in all water types, and in most cases the concentrations of pesticides were lower than those of their metabolites. Chloridazon desphenyl (DESPH) is the most widespread pesticide in surface, ground and hypogenic waters. Its concentrations in surface waters were 5.7 ng·L−1–2,230 ng·L−1, in groundwaters were 11.3 ng·L−1–1,490 ng·L−1 and in karst hypogenic waters 5.4 ng·L−1–378 ng·L−1. Diclofenac was the most widespread substance from the pharmaceutical group. Its concentrations ranged from 5.6 ng·L−1–549 ng·L−1 in surface waters, 8.4 ng·L−1–112 ng·L−1 in groundwaters and 5.1 ng·L−1–47.4 ng·L−1 in karst hypogenic waters. Directly in the karst hypogenic waters, the following EOCs were repeatedly detected: atrazine and its metabolites, simazin, metazachlor ethane sulfonic acid (ESA), metolachlor ESA, alachlor ESA, chloridazon DESPH, diclofenac, ibuprofen, azithromycin, bisphenol A and diethyltoluamide (DEET). The initial research hypothesis is that hydrothermal karst waters are of sub-recent age and deep circulation and, unlike surface and groundwaters, are not contaminated by recent pollutants. A certain component of these waters is therefore a shallow and shorter circulation.
1 Introduction
Karstic aquifers supply 25% of all drinking water in the world, but they are highly vulnerable to contamination, and their preservation is constantly threatened in developing countries (Wilkinson, et al., 2022).
In densely populated and environmentally sensitive areas, the vulnerability of groundwater is in and, more recently, there is concern regarding contamination by pesticides or pharmaceuticals (including their metabolites) and their further spatial distribution question (Wachniew, et al., 2016; Douglas, et al., 2018). The risk of contamination in karst areas can be considered one of the most significant. The results of previous studies explicitly conducted in karst areas have confirmed the considerable flow rate of distribution of substances in the water-soil-geological system (Huang, et al., 2021).
Pesticides and pharmaceuticals belong to a group of chemical compounds called emerging organic contaminants (EOCs) (García, et al., 2020). EOCs are substances potentially harmful to the environment (Chopra and Kumar 2020; Mali, et al., 2023). Many EOCs can accumulate in aquifers through a process known as leaching, which involves their movement into deeper soil layers and eventually into groundwater sources such as aquifers (Zemann, et al., 2016; Pérez-Lucas, et al., 2019). The accumulation of pesticides in aquifers poses serious environmental and health risks, as these water sources are used for drinking water worldwide. Severe effects on human health resulting from significantly prolonged exposure and accumulation are possible (Zhang, et al., 2006; Bhandari, et al., 2020; Perera-Rios, et al., 2022; Ruomeng, et al., 2023). EOCs are widely used and can accumulate in the environment through various sources. The predominant entry points of EOCs into the environment are via wastewater discharge, agricultural and aquacultural practices, and industry and hospital inputs (Alderton, et al., 2021). The transportation of microorganic pollutants to aquifers is determined by their characteristics and subsurface conditions, such as hydrogeological factors, groundwater residence time, redox processes, soil type, and chemical properties. In addition, other processes, such as ion exchange, adsorption or desorption onto minerals and organic matter, biodegradation and chemical transformations, may control their migration into aquifer systems (Stoppiello, et al., 2020). Many global studies and research papers have revealed the findings pesticides and their metabolites in surface and groundwaters, including karst waters and drinking water sources (Eurostat, 2018; Ferrando and Matamoros, 2020; Baran, et al., 2021; Syafrudin, et al., 2021; Troldborg, et al., 2022).
Treating water contaminated by new artificial chemical compounds, mainly from pharmaceutical and personal care products, is a significant concern (Metcalfe, et al., 2011). Pharmaceutical findings are prevalent in surface waters (Bexfield, et al., 2019; Chopra and Kumar 2020) and also identified in karst aquifers (Morasch, 2013; Dodgen, et al., 2017).
The European Water Framework Directive 2000/60/EC (European Council, 2000) aims to elevate all surface waters to “good” status, involving a range of biological, hydrological and chemical metrics. An Environmental Quality Standard (EQS) is a chemical metric used to assess status. Chemicals selected for EQS derivation are deemed to present a Europe-wide risk, and these EQSs are applied across all European Union countries. The EQSs represent legally binding maximum surface water concentrations, and drive regulatory action to reduce the concentration of chemicals in surface waters (Leverett, et al., 2021).
The occurrence of pesticide residues is repeatedly confirmed in agricultural soils (Karasali, et al., 2016; Hvězdová, et al., 2018; Silva, et al., 2019; Geissen, et al., 2021; Bakanov, et al., 2023; Riedo, et al., 2023), but no limits have yet been set.
This study aims to determine the vulnerability of the hypogene karst study area to pesticide leaching and potential accumulation. We employed advanced analytical methods, supported by geological and topological knowledge, to evaluate the transfer of emerging organic pollutants into hypogene karstic waters.
1.1 Study area
The study of the Hranice karst region (Figure 1) focuses on a distinct type of landscape, namely, hypogene hydrothermal karst. This karstic region is extensively populated and utilised. Its carbonated mineral waters have been used for their healing properties since the 16th century, making the spa in Teplice nad Bečvou one of the oldest in Moravia. The area’s potential is highly diversified and is influenced by various interests—agriculture, surface mining, nature conservation, recreation, spa, industry and housing (Oppeltová, et al., 2022).
The territory is between the Český massif’s geological units and the Outer Western Carpathians. The interaction between the Bohemian Massif and the Western Carpathians has significantly influenced the tectonic structure and present-day form of the Hranice Karst. Carbonate (limestone) rocks of the Devonian and Lower Carboniferous ages emerge in an area of approximately 5 × 3 km in the eastern part of the Czech Republic (N 49 31′, E 17 ° 45′). Paleozoic carbonates are partially overlain by younger sediments belonging to the Neogene of the Carpathian foreland, consisting mainly of clays and sandstones (Šráček, et al., 2019). The Quaternary cover consists of reference soil groups (RSG) rendzic leptosols, arenosols and regosols on the karst bedrock; on agricultural land, RSG luvisols or retisols and cambisols dominate (IUSS Working Group WRB, 2015).
Regarding limestone, 30 caves are known in the karst area, the largest of which are the Zbrašov aragonite caves (ZAC) with unique their hydrothermal decoration and gas lakes with CO2. Hranice Abyss (HA) is also located here and is the deepest freshwater flooded abyss in the world. It is widely accepted that the formation of the abyss and the surrounding mineral springs in the karst area is associated with the influx of juvenile CO2 from deeper regions of the geosphere (Špaček, et al., 2015). The abyss is filled with mineral water with a high level of CO2 (Šráček, et al., 2019), and there are springs of hypogenic mineral water to the depth of 30 m, 40 m, and 60 m.
There are also lakes of warm mineral water covered by a layer of gaseous CO2 in the Zbrašov aragonite caves. The infiltration areas of the waters from which the mineral waters are created have not yet been identified. However, it appears that the mineral waters result from the mixing of older and younger waters and that some of the waters infiltrate underground at slightly higher elevations (Vysoká, et al., 2019; Šráček, et al., 2019).
Mean annual air temperature and precipitation within the study area are 8.5°C and 677 mm, respectively (for 1961–2021). The Bečva River and its tributaries drain the whole study site. In the Teplice nad Bečvou municipality, the gauge is managed by the Czech Hydrometeorological Institute (CHMI) with a contributing basin area of 1275.32 km2. According to the CHMI, the average annual flow of the Bečva River in Hranice is 14.8 m3·s−1, and the average water level is 0.89 m.
2 Materials and methods
2.1 Sampling sites
The screening aimed to determine whether and to what extent deep karst mineral waters (i.e., outflows of warm mineral waters) are contaminated with emerging organic contaminants (EOCs). Monitoring focused on EOCs in the area of interest, the Hranice Abyss, has never been conducted.
Samples were collected at locations in the Hranice Abyss where warm mineral waters emerge, presumed to be predominantly of hypogenic origin. Locations of watercourses and shallow subsurface waters, including drainage waters and shallow wells, were sampled. Those were suspected of contamination by agricultural and other anthropogenic EOCs. Deep groundwater was sampled through boreholes, including spa wells, to verify the distribution of EOCs. Collaborating with divers, mineral water samples were obtained from caves and warm outflows in the Hranice Abyss at 30, 40, and 60 m depths. Waters in the Zbrašov Aragonite Caves lakes were also included in this study.
Total 32 locations (Figure 2) were selected for processing in the article, which was divided into three groups: “surface water” (S: 13 locations), “groundwater” (G: 8 locations) and “thermal karst water” (TKW: 11 locations) (Table 1).
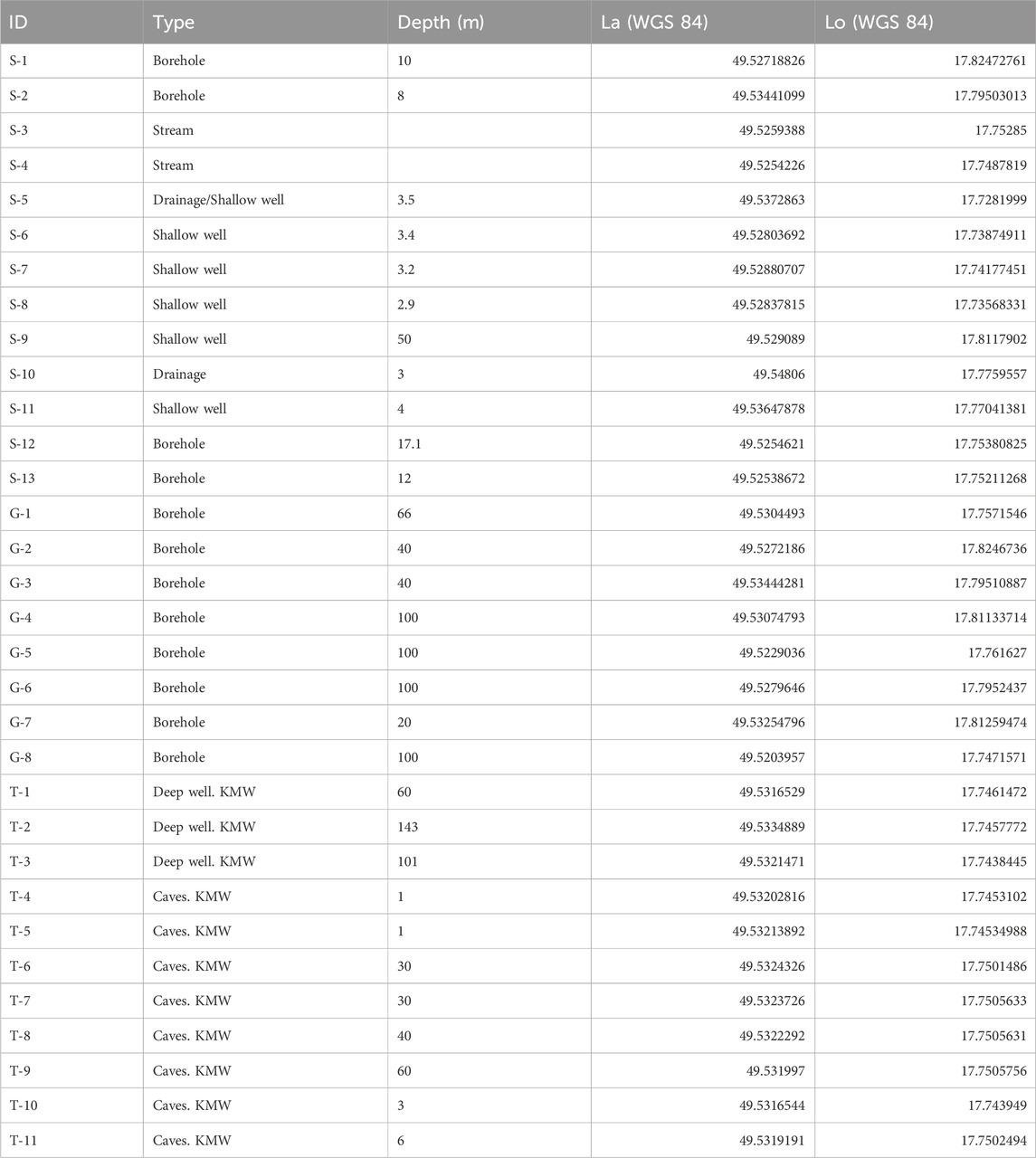
TABLE 1. List of the sampling sites including depth and geographical location (WGS 84) (KMW - karst mineral water).
From February 2022 to June 2023, water monitoring focused on observing selected emerging EOCs—mainly pesticides and their metabolites, pharmaceuticals and their metabolites—was carried out in the Hranice Karst (Figure 2). Sampling was conducted seasonally, depending on hydrometeorological conditions. Due to drying up of some resources, it was not possible to sample regularly. Due to the specific conditions and dependence on other subjects and circumstances (divers, high CO2 concentration in caves), water sampling from the abyss and caves was not carried out regularly and took place at different times than the sampling of surface, drainage waters and of boreholes and balneological wells. Seasonal changes in EOCs concentrations were not part of this research. During the monitored period from February 2022 to June 2023, 14 monitor campaigns were carried out. In total 44 surface, 30 groundwater and 45 TKW samples were taken.
2.2 EOCs quantification
The samples were collected in 100 ml glass screw-cap vials. An ultra-high-performance liquid chromatography system (Agilent 1290 Infinity, Agilent Technologies, Inc., California) coupled with a tandem mass spectrometer (Triple Quadrupole 6495C, Agilent Technologies, Inc., California), was used to determine the selected pesticides, pharmaceuticals, and their metabolites in aqueous matrices. An enriched sample was also prepared to verify yield efficiency for each sample. Measurements were carried out in dynamic multiple reaction monitoring (dMRM) mode. The analytes were identified based on their quantification ions, qualifying ions (if applicable), and retention times. Prior to analysis, samples were enriched with internal deuterated standards, if available. Corresponding internal standards in concentration 100 µg·ml−1, diluted in acetone, acetonitrile or methanol, were used. Their list, including their description, is presented in Supplementary Table S1. The analysis was conducted using a direct injection method, where 100 μl of the not-filtered sample was directly introduced into the column. Chromatograpic analysis was performed using, 0.002% formic acid and 0.2 mM NH4F gradient (gradient parameters in Supplementary Table S2). The column utilized for this study was an Agilent Zorbax Eclipse Plus C18, with dimensions of 2.1 mm × 100 mm and a particle size of 1.8 μm. The quality of analyses was ensured by regular optimization of the working parameters of the mass detector before each measurement—Autotune, Checktune. The quality was additionally monitored, by continuous control of the yield of analytes in enriched samples and in an enriched blank. The method is validated and accredited according to ČSN, EN, (2018).
The ability of chemical compounds to contaminate groundwater and the leaching potential of pesticides was evaluated according to Groundwater Ubiquity Score (GUS). GUS is a technique to predict the ability of chemical compounds to contaminate groundwater and it is also used for assessing leaching potential of pesticides (Wauchope, et al., 1992) (Table 2). GUS is an experimentally calculated value by Gustafson (1989) that relates pesticide half-life and sorption potential.
European Union legislation—the Water Framework Directive 2000/60/EC, the related Drinking Water Directive European Union, (2020) and the Groundwater Directive (European Comission, 2006b)—require that the concentration of individual pesticides in groundwater must not exceed 0.1 µg·L−1, and the total concentration of all pesticides must be below 0.5 µg·L−1 “Total” means the sum of all individual pesticides detected and quantified in the monitoring procedure, including their relevant metabolites, degradation and reaction products (European Comission, 2006a). These values must not be exceeded in drinking water and are recommended as a groundwater quality standards (European Comission, 2006a).
3 Results
The data obtained within this study revealed contaminants, such as pesticides, pesticide metabolites, and pharmaceuticals, in the waters of the Hranice Karst. Moreover, bisphenol A (BPA) and DEET were also occasionally found. Pesticides or their metabolites banned in the EU were found in all types of waters (Tables 3–5). Box plot of all EOCs in the groups surface water (S), groundwater (G), and thermal karst waters (T) in ng·L−1 was created (Figure 3). Box plot of all EOCs parent compounds and EOCs metabolites in S, G and T groups shows Figure 4. The sum of the contents of the pesticide parent compound and their metabolite shows Figure 5 and the sum of the contents of the pharmaceutical parent compound and their metabolite shows Figure 6. The chart values represent the sums of EOCs substances measured during individual samplings at all monitored locations. Upper whisker: max value of the dataset; lower whisker: min value of the dataset; the box boundaries from top to bottom are as follows: top: 75th percentile; first bar: median; bottom: 25th percentile. The chart shows the cross-arithmetic mean; stars: outliers.
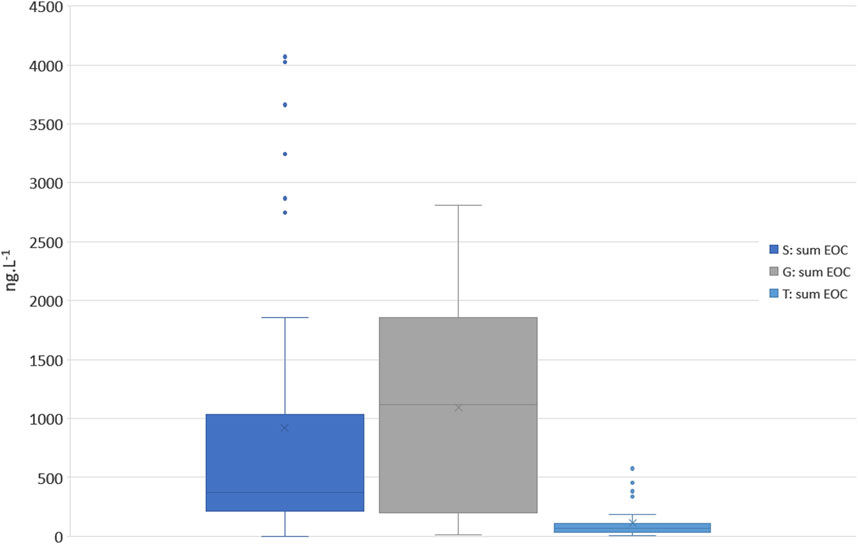
FIGURE 3. Box plot of all EOCs in the groups surface water (S), groundwater (G), and thermal karst waters (T).
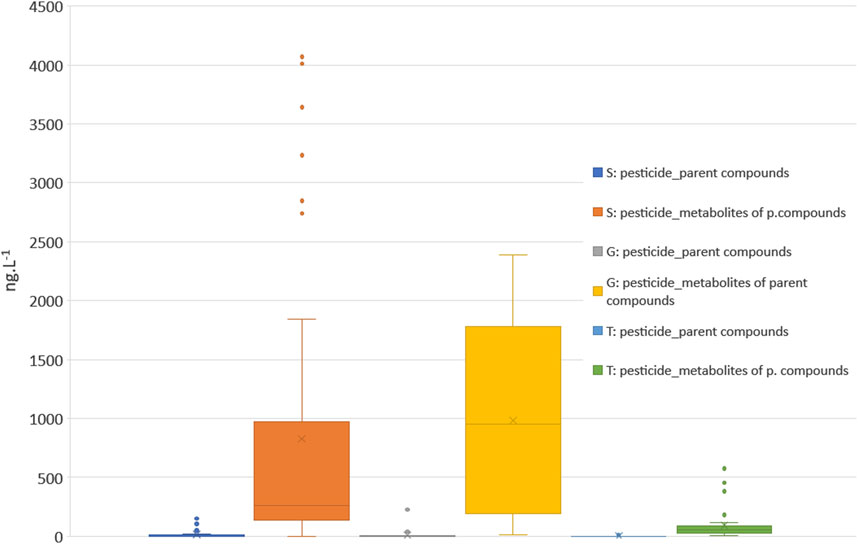
FIGURE 5. Sum of the contents of the pesticide parent compound and their metabolite in S, G, and T groups.
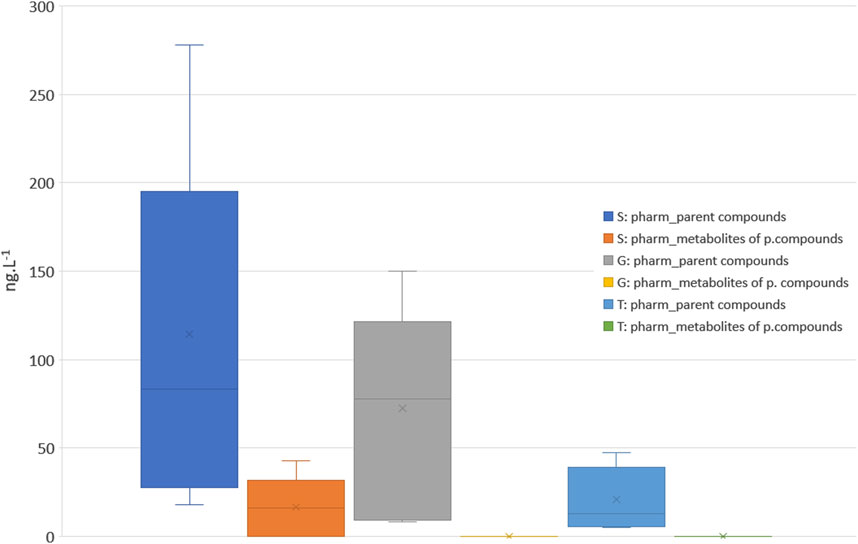
FIGURE 6. Sum of the contents of the pharmaceutical parent compound and their metabolite shows in S, G, and T groups.
3.1 Surface waters
This category includes 13 sites encompassing surface and shallow subsurface waters, including drainage waters and shallow wells (Table 1). These are locations where there is a high assumption that EOCs enter the aquatic environment (Figures 3–6). As for the number of contaminants found, the Bečva River ranks first. The highest concentrations often reached the order of µg·L−1 (Figures 3–5). As expected for the chloroacetate and pyridazone groups, higher levels of metabolites than parent substances were consistently detected (Table 3). In none of the cases were the maximum values of primary substances or metabolites associated with a watercourse but with drainages or shallow wells of about 3 m in depth, many of which serve as local drinking water sources.
The highest total of all primary pesticide substances in a single collection (152.6 ng·L−1) was found at the shallow well S-6. The highest pesticide metabolites content (4070.2 ng·L−1) was detected at shallow well S-10 (Supplementary Table S3, Figure 5).
For triazine pesticides, the maximum value of primary substances was found at S-6 (142 ng·L−1 atrazine) and metabolites at S-7 (105 ng·L−1 desethylatrazine). For chloroacetate pesticides of primary substances, the maximum was at S-9 (25.5 ng·L−1 dimethenamid) and metabolites at S-11 (1,400 ng·L−1 metazachlor ESA). Primary substances of pyridazol pesticides and their metabolites had their maximum in drainage S-10 (14.3 ng·L−1 chloridazon and 2,230 ng·L−1 chloridazon-DESPH) (Table 3).
For pharmaceuticals, the maximum values of non-steroidal anti-inflammatory drugs (NSAIDs) were in well S-8 (976 ng·L−1); antibiotics (ATB) and antidepressants (ATDe) were in the Bečva River and S-3 (79.7 and 62.6 ng·L−1) (Table 3).
In S-3 and S-2, Bisphenol A (BPA) was also analysed (54.1 ng·L−1; 144 ng·L−1), and in well S-13, DEET was found (141 ng·L−1) (Table 3).
3.2 Groundwater
In this category, eight sites comprising only boreholes (Table 1) with varying depths from 20 m (G-7) to 100 m (G-4, G-5, G-6, and G-8) were included. These are, therefore, sites where further transport of EOC substances from the “S” locations into the hydrogeological system can be assumed. In all monitored groundwater locations, findings of pesticide metabolites exceeded the parent substances, both in the number of substances found as well as the concentrations (Table 4).
The highest total of all primary pesticide substances in a single collection (226.3 ng·L−1) was detected at G-8. The highest total pesticide metabolites in a single collection (2,390.7 ng·L−1) was at G-1 (Figure 5).
From the urea pesticides, Chlortoluron was detected in G-8 (43.3 ng·L−1) (Table 4).
Most substances belonging to triazine pesticides were found in boreholes G-6 and G-8. In these two boreholes, metabolites and parent substances of triazine pesticides were found to be the only ones in the category of groundwater. For triazine pesticides, the maximum value of primary substances was found in G-8 (28.5 ng·L−1 prometryn). In terms of individual metabolite findings, the maximum values were again in borehole G-8, atrazine-2-OH (241 ng·L−1) and terbuthylazine-2-OH (532 ng·L−1) (Table 4). Triazine pesticide metabolites were also detected by repeated findings in borehole G-1 (9.8–39 ng·L−1). In borehole G-8, triazole pesticide—tetraconazole was detected (142 ng·L−1). (Supplementary Table S3).
Chloroacetamide pesticides are represented here only by metabolites; the parent substances were not found in ground waters. The highest concentration of metabolite was found at borehole G-1 (1,050 ng·L−1 metazachlor ESA) (Table 4).
In the pyridazone group, both parent substances and metabolites were found. The highest value of the parent substances was found at borehole G-6 (33.5 ng·L−1 chloridazon); the highest value of metabolites was found at borehole G-4 (1,490 ng·L−1) (Table 4).
No substances from the strobilurin group were found here.
For pharmaceuticals, the maximum values of NSAIDs were in borehole G-4 (150 ng·L−1 ibuprofen); antibiotics were found only in borehole G-6 (115 ng·L−1 azithromycin) and in borehole G-7 (25.6 ng·L-1 sulfamethoxazole). No ATDe were detected at any groundwater sites (Table 4).
BPA was repeatedly found in boreholes G-6 and G-4 at concentrations up to 268 ng·L−1 and 655 ng·L−1. DEET was detected in borehole G-6 (114 ng·L−1) (Table 4).
3.3 Thermal karst waters
This category included 11 sites encompassing thermal springs of mineral waters, cave lakes and outputs in abysses (Table 5). Therefore, these are locations where the accumulation of EOC substances from areas “S” and “G” was assumed, or they should be areas minimally influenced anthropogenically.
The highest sum of all primary pesticide substances within a single collection (20.6 ng·L−1) was found at T-10. The highest sum of all pesticide metabolites within one collection (576.1 ng·L−1) was at T-11 (Figure 5).
In TKW, trace amounts of parent substances and metabolites of diazine and triazine pesticides were occasionally found. Specifically, these were atrazine and its metabolites, as well as simazine. The maximum value of parent substances of diazine and triazine pesticides was in T-10 (16.2 ng·L−1 atrazine), and the maximum concentration of metabolites was also in T-10 (18.5 ng·L−1 desethylatrazine) (Table 5).
As for chloroacetate pesticides in TKW, only metabolite findings were present, with no parent substances of this group found. The highest amount of metabolites was detected in the balneological well T-1, specifically metazachlor ESA (63 ng·L−1) and metolachlor ESA (123 ng·L−1) (Table 5).
The pyridazone group is also represented only by metabolites. The maximum metabolite value was in T-11 (378 ng·L−1 chloridazon-DESPH) (Table 5).
Trace amounts of pharmaceuticals were found at most TKW sites during the monitoring period (Supplementary Table S3). In most cases, these were NSAIDs, with the highest value at the balneological well T-3 (47.4 ng·L−1 Diclofenac). The only finding of ATB was T-7 (73.8 ng·L−1). Pharmaceuticals in the ATDe group were not detected at any TKW sites (Table 5).
BPA was found at TKW sites only in T-9 (242 ng·L−1). DEET was detected in HA at T-6 (132 ng·L−1) (Table 5).
4 Discussion
From the above results, variable inputs of primary substances and metabolites into the system are evident. The results indicate that the highest concentrations of pesticides, their metabolites, and pharmaceuticals (reaching up to µg·L−1) were identified in surface waters group. These sites encompasses surface and shallow subsurface waters, including drainage waters and shallow wells. Group surface waters represents areas where pollution from both non-point and point sources enters the hydrological system. These sites are typically situated in intensively cultivated agricultural landscapes, where pesticides and industrial fertilisers are substantially used. In addition, the influence of human habitation in these areas might contribute to potential contamination through wastewater discharge. Contaminants enter the hydrological system mainly via surface runoff infiltration, leaching through soil layers and from wastewater since none of the sewage treatment plants in the area of interest have technology for removal. The degree of pesticide contamination in water is affected by the properties of the pesticide (e.g., solubility), characteristics of the soil (e.g., organic matter content within the soil, soil pH or content of clay and coarse particles) (Park, et al., 2020), site conditions and the application and management of pesticides (Syafrudin, et al., 2021). Shallow groundwater is easily contaminated with pesticides, and their distribution is linked to land use and crop growth (Andrade and Stigter, 2009). Land management practices, such as irrigation and application, together with intensive rainfall events, can increase a pesticide’s mobility (Menchen, et al., 2017).
Elevated concentrations of EOCs, also in the µg·L−1 range, have been detected in groundwater (Figure 4), specifically in wells ranging from 10 s to 100 m deep. These deep wells contained pesticide metabolites, indicative of contamination originating from agricultural activities, and pharmaceuticals and BPA, both likely derived from wastewater contamination. Given the permeable substrate in these areas, characterised by locally permeable sandy soils and underlying limestone, the EOCs can disperse from these deep groundwater sources. The sandy soils and limestone substrate facilitate the migration and dispersion of these contaminants in the groundwater and allow deep karst mineral waters contamination.
The occurrence of organic micropollutants in the groundwater, such as pesticides, has attracted global attention because of the potential negative effects on human health (Luo, et al., 2014). The threshold value of 0.1 μg·L−1 (European Comission, 2006b) was repeatedly exceeded in all monitored groundwater samples, with some contaminants surpassing this limit up to tenfold. The sole exception is well G-7, which did not exceed this threshold throughout the observation period. The geological profile of the borehole G-7 is composed of fluvial gravels and sandy loam sediments with high permeability down to a depth of 6.8 m; below that, to a depth of 20.0 m, it consists of clays and claystones with low permeability. In addition, the limit of 0.5 μg·L−1 was surpassed in wells G-1, G-3, G-4, G-6, and G-8 (Supplementary Table S3).
The geological profile of well G-1 at depths of 0–18 m consists of alternating highly permeable and impermeable rocks, specifically Neogene sands and sandstones, or possibly clays. It comprises tectonically fractured and karstified Palaeozoic limestones at 18–66 m depth. Both rock types generally exhibit high permeability, so the studied substances may be transported from greater distances or villages opposite the water flow. The flow here is considered to be conformal with the direction of the river’s current. The geological profile of well G-3 up to a depth of 6.8 m consists of fluvial gravels and sandy loam sediments of high permeability and up to 40 m by weathered and karstified limestones. Well G-4 is formed up to a depth of 4.8 m by sandy and clayey fluvial gravels in the profile. From a depth of 4.8 m onwards, it consists of limestones that show intense weathering, karstification and the presence of cave spaces. The geological profile of well G-6 up to a depth of 8.6 m is made of fluvial gravels and sandy loam sediments of high permeability. At depths of 8.6–28.5 m, there are clays and claystones of minimal permeability. Beneath these clays, karstified Paleozoic limestones have been confirmed down to a depth of 100 m. The geological profile of well G-8 is led by Paleozoic limestones, which exhibit high permeability. The interaction between land use and water quality is essential worldwide, as agriculture has been proven to exert considerable pressure on the quality of groundwater and surface waters due to leaching and erosion processes (Kronvang, et al., 2020).
In wells G-6 and G-4, BPA was repeatedly detected, with concentrations reaching up to 655 ng·L−1 in G-4 and 268 ng·L−1 in G-6 (Table 4).
Ibuprofen tends to be detected in various environmental samples: wastewater, sludge, soil, surface water and groundwater. In Europe, groundwater concentrations of ibuprofen occur between 3 ng·L−1 and 395 ng·L−1 (Chopra and Kumar 2020). As part of the groundwater quality monitoring, wells located within the built-up areas of the villages Černotín and Teplice nad Bečvou (S-6, S-7, S-8, and S-11) were also included; contamination by pharmaceuticals (diclofenac, ibuprofen) as well as metabolites of pesticides in concentrations reaching up to 1 μg·L−1, was detected. The source of this contamination is likely wastewater leaks from sewer systems (or sewage treatment plants with infiltration, but these should not be present) in family homes built above the sampled sites. Once in the groundwater, these pollutants attenuate very slowly and can persist for long periods because of unfavourable redox and degradation conditions (e.g., anaerobic conditions and limited microbial activity) (Sorensen, et al., 2015; Sorensen et al., 2015; Dueñas-Moreno, et al., 2022). Dueñas-Moreno, et al. (2022) pointed out the high-risk BPA represents for human health once introduced into the groundwater.
Negligible to trace concentrations of EOCs (sum of all found substances), on the order of tens to hundreds of ng·L−1, were detected at the TKW sites (Figure 3), which were presumed to be predominantly of hypogenic origin and hence were not expected to contain EOC-type substances. The threshold values of 0.1 μg·L−1 and 0.5 μg·L−1 were exceeded at TKW sites only in the case of T-11, located precisely at the Hranice Abyss and in T-6. From the TKW group, significant findings of EOCs were observed in the balneological wells T-1 and T-3 (Table 3, Supplementary Table S3). These findings included trace amounts of diclofenac, ibuprofen, metazachlor ESA, metolachlor ESA, and chloridazon-DESPH. These monitoring results refute the initial hypothesis that deep hydrothermal karst waters are ancient and uncontaminated.
Among all the monitored pesticides and their metabolites within the scope of the Hranice Karst monitoring (refer to our table), metazachlor ESA and metolachlor ESA were among the substances with the highest GUS indices (Table 2), with values of 6.18 and 7.22, respectively. GUS is a technique to predict the ability of chemical compounds to contaminate groundwater and is also used for assessing the leaching potential of pesticides. GUS is an experimentally calculated value that relates pesticide half-life and sorption potential (from laboratory data). The monitoring results demonstrated that, within the karst system, EOCs with elevated GUS indices can contaminate wells at depths of 60 m and 101 m. When utilising pesticides, particularly within karst terrains and on permeable soils, there should be thorough monitoring of the propensity of chemical compounds to contaminate groundwater. Subsequently, application strategies should be adjusted based on these observations to mitigate contamination risks.
The GUS may be used to rank pesticides for their potential to move toward groundwater (Gustafson, 1989). Pesticides with a short half-life, low water solubility and high sorption potential may have less potential to move through soil. Pesticides with a long half-life, high water solubility and low sorption potential possess a more significant potential to move through soil (Wauchope, et al., 1992).
Chloridazone is an herbicide widely used in agriculture to control beets and corn. The primary chloridazon metabolites are desphenyl-chloridazon (DPC) and methyl-desphenyl-chloridazon (Me-DPC), which can be a threat to surface and groundwater resources (Vidal, et al., 2022). Chloridazon inhibits the photosynthesis process in annual broad-leaf weeds and has been widely used in the past several decades because it was considered relatively harmless (Cioni and Maines, 2010; Jakiene, et al., 2015). Due to the polarity and solubility of metabolites, they are regarded as mobile compounds which cause surface water and groundwater pollution.
Chloridazon-DESPH also possesses a notably high GUS index of 5.64, indicating a significant potential for movement toward groundwater. Furthermore, it exhibits a high Screening Concentration In Ground Water (SCI-GROW) index of 9.25 μg·L−1, signifying a pronounced likelihood of appearing in groundwater due to its propensity for leaching and mobility in the soil–water environment. These indices collectively suggest a need for careful management and monitoring of chloridazon-DESPH to prevent groundwater contamination.
SCI-GROW is a screening model which the Office of Pesticide Programs in the US Environmental Protection Agency frequently uses to estimate pesticide concentrations in vulnerable groundwater. The model provides an exposure value to determine the potential risk to the environment and human health from drinking water contaminated with the pesticide. The SCI-GROW estimate is based on the environmental fate properties of the pesticide (aerobic soil degradation half-life and linear adsorption coefficient normalised for soil organic carbon content), the maximum application rate, and existing data from small-scale prospective groundwater monitoring studies at sites with sandy soils and shallow groundwater (USEPA, 2016).
In the lakes within the Zbrašov Aragonite Caves (T-10, T-11), trace amounts of atrazine and its metabolites have been confirmed (Table 5). In the slopes above the caves, intensively farmed fields are located to the south and west. The land use of this area has not changed significantly over the long term. Atrazine is a triazine herbicide extensively used against various dicotyledonous weeds, but it has been banned in the European Union since 2005 due to its environmental and health implications. Despite the ban, the persistence and mobility of atrazine in the environment can result in its continued detection in various water bodies, reflecting its historical use and long-term environmental persistence. These findings highlight the necessity for ongoing monitoring and assessment of water quality within the cave environment to understand the environmental distribution and fate of banned or restricted substances. Although atrazine use was banned in France in 2003, both atrazine and its metabolite desethylatrazine were detected in French groundwater years after its removal from the market (Baran, et al., 2008). The GUS leaching potential index of atrazine is 2.57 (moderate potential for movement toward groundwater), and the GUS of desethylatrazine is 3.24 (high potential for movement toward groundwater). Atrazine has a high potential to contaminate groundwater, especially in environments with porous soil profiles (de Albuquerque, et al., 2020), as confirmed by the research results in the Hranice Karst. Many studies are published yearly on atrazine’s presence in the environment, especially for its metabolites (Kolpin, et al., 1998; Fava, et al., 2007; Iker, et al., 2010; Jablonowski, et al., 2011) modelled atrazine concentrations in shallow groundwater (concentrations ranged from <0.004 to 4.7 μg·L−1) and found that atrazine concentrations are controlled mainly by the history of atrazine use in relation to the groundwater age.
Despite banned application, triazines and acetochlor are still detected (Baran, et al., 2021). Furthermore, in the lakes within the Zbrašov Aragonite Caves, trace amounts of chloridazon-DESPH, chloridazon-DESPH-ME, alachlor ESA, and the pharmaceutical diclofenac have been detected (Supplementary Table S3). Although intensively farmed fields are located on the slopes above the caves, pharmaceuticals must be attributed to historical infiltration of wastewater or leaks from sewage systems in the past. Recently, a modern sewage system was built in the village. The detection of these compounds, even in trace amounts, indicates the presence of anthropogenic contaminants within the karstic aquifer, necessitating continued vigilance and monitoring to assess the environmental health and potential risks associated with these residues.
EOCs were also detected in the deep waters of the Hranice Abyss (T-6, T-7, T-8, T-9, and T-11). Noteworthy are the findings of BPA (242 ng·L−1 in T-9), DEET (132 ng·L−1 in T-6), chloroacetanilide pesticides and their metabolites (including alachlor ESA, metolachlor oxanilic acid (OA), metolachlor ESA, metazachlor OA, and metazachlor ESA), as well as compounds from the pyridazone group (chloridazon-DESPH, chloridazon-DESPH-ME) (Table 5).
The highest concentrations of the pollutants were identified in well T-11 (Table 5). Given the lower water conductivity values’ decreased concentrations of bicarbonates (HCO3), calcium (Ca), and magnesium (Mg) compared to the other inputs (T-9 and T-6), it can be inferred that the water in T-11 is not purely mineral water. Instead, a mixing process appears to have occurred, involving surface waters (which are likely contaminated) blending with the deeper, mineral-rich waters in this location. This mixing introduced pollutants into the deeper water strata, which warrants careful and continuous monitoring to understand the extent and implications of such contamination for the water quality in the area adjacent to Hranice Abyss.
The detection of BPA indicates contamination from wastewater sources. Dueñas-Moreno, et al. (2022) reported that BPA has been globally detected in groundwater reservoirs and landfills. Sewage sludge and wastewater are the primary BPA sources of groundwater pollution. BPA pollution is caused by anthropogenic activities, including manufacturing processes, the distribution, use, consumption, treatment and disposal of devices and products containing phthalates and BPA, resulting in long-term transport from the environment to groundwater reservoirs. Their physicochemical properties condition the transport and fate of phthalates and BPA, the type of pollution sources, the type of matrix (air, water, and soil) and the environmental conditions (pH, temperature, and pressure) (Tran, et al., 2022).
The continued detection of alachlor ESA in both caves and the abyss (Supplementary Table S3), despite its ban since 2007, raises significant considerations. Despite their prohibited use, many pesticides and their metabolites are still found in water and other areas (Jablonowski, et al., 2011; Baran, et al., 2021; Kruć-Fijałkowska, et al., 2022). The water in the specified areas may exhibit slow movement or circulation dynamics, allowing contaminants introduced before the ban to remain in situ for extended periods. Slow-moving or stagnant water bodies can act as reservoirs for these compounds, releasing them gradually (Zhu, et al., 2017). The unique environmental conditions in these areas might hinder the degradation process of alachlor and its metabolites. The environmental persistence of certain compounds can be extended under conditions that are not conducive to their breakdown, resulting in prolonged detectability (Mouvet, et al., 1997). Although alachlor has been banned, illegal or unregulated use might still be occurring, or sources of contamination might not have been identified or mitigated. This continued input can maintain the presence of alachlor and its metabolites in the environment. The persistence of alachlor ESA and its metabolites in the water demands further investigation to understand the dynamics of water movement, the degradation pathways of these compounds under local environmental conditions and potential unidentified sources of contamination.
Trace amounts of the NSAID diclofenac and the antibiotic azithromycin were detected in the waters of the Hranice Abyss. Notably, diclofenac was found at its highest concentration of 47.4 ng·L−1 in T-3. Azithromycin was detected in T-7 (73.8 ng·L−1) (Table 5).
The presence of pharmaceuticals like diclofenac and azithromycin in aquatic environments is often attributed to the incomplete metabolism of these drugs in humans and animals and the insufficient removal of these compounds during wastewater treatment processes (Jones, et al., 2022). Once released into the environment, these pharmaceuticals can persist and be transported through water bodies, leading to their detection in remote and deep-water locations like the Hranice Abyss.
Active pharmaceutical ingredients are emitted into the natural environment during manufacture, use and disposal. There is evidence that environmental exposure to pharmaceuticals has deleterious effects on the health of ecosystems and humans (e.g., by selecting antibiotic-resistant bacteria, feminising fish and increasing the susceptibility of fish to predation) (Kidd, et al., 2007; Brodin, et al., 2013; Wellington, et al., 2013; Horký, et al., 2021).
Diclofenac has thus been detected in trace concentrations in the balneological wells within the ZAC and in the Hranice Abyss (Supplementary Table S3). The limit recommended by European Commission in surface water is 5.4 ng·L−1 resp. 230 ng·L−1 to protect birds from diclofenac secondary poisoning through the food chain (Peters, et al., 2022). The limit of 230 ng·L−1 was exceeded at two of our monitored sites. In S-8 and S-9, the maximum concentration of diclofenac was 549 ng·L−1 and 231 ng·L−1 (Table 3, Supplementary Table S3). The less strict limit of 5.4 ng·L−1 was exceeded in group S (S-3, S-6, S-9, and S-10), group G (G-1, G-2, G-4, G-5, and G-6) and group T (T-1, T-3, T-4, T-9, and T-10) (Supplementary Table S3).
The correlation between land use and concentrations of EOCs (specifically pharmaceuticals) was also confirmed by other studies (Schäfer, et al., 2016; Chen, et al., 2023). In particular, there is a pattern of positive correlation between contaminant concentrations and land use characterised by cropland and impermeable surfaces (when considering horizontal distribution) and a negative correlation between concentrations and areas covered with vegetation.
5 Conclusion
The monitoring of the Hranice Hypogenic Karst showed the presence of EOCs in all types of waters, including trace concentrations in deep TKW and balneological wells. The detected EOCs were represented by pesticides, pharmaceuticals and their metabolites; BPA and DEET were occasionally found. Thus, the results refute the original hypothesis that deep hydrothermal karst waters are ancient and uncontaminated. The excessive permeability of the karst system leads to an enhanced vulnerability for retention and spread of contaminants. The results generally confirm that current land use is associated with pollution in the karst system.
In groundwater and the Zbrašov Aragonite Caves lakes, trace findings of atrazine and its metabolites were confirmed. In the warm HA springs and Zbrašov Aragonite Caves lakes, trace concentrations of alachlor metabolites were found. In both cases, these are substances whose application has been banned in the European Union for more than 10 years. The unique environmental conditions in these areas might hinder the degradation processes of these substances, and slow-moving or stagnant water bodies can thus act as reservoirs for these compounds.
When using pesticides, especially in karst locations and on permeable soils, one should monitor the ability of chemical compounds to contaminate groundwater. In agricultural practice, this means monitoring the values of the GUS index of the substances used and adapting one’s application to these values. This karst area is a highly vulnerable system to anthropogenic activities and it is recommended to be monitored beyond legal requirements. In the future, more extensive sampling of source and ground waters through concerted campaigns or deployed samplers and higher resolution weather and land use measures would bring more certainty to understanding contamination of karst aquifers and their environmental, drinking water and mineral water risks.
Data availability statement
The original contributions presented in the study are included in the article/Supplementary Material, further inquiries can be directed to the corresponding author.
Author contributions
PO: Data curation, Investigation, Methodology, Supervision, Writing–original draft, Writing–review and editing, Conceptualization, Resources. VV: Conceptualization, Data curation, Formal Analysis, Methodology, Writing–review and editing. MG: Conceptualization, Data curation, Funding acquisition, Methodology, Supervision, Validation, Writing–original draft, Writing–review and editing, Investigation. PC: Data curation, Writing–review and editing. OU: Data curation, Visualization, Writing–review and editing, Resources. JS: Funding acquisition, Project administration, Visualization, Writing–review and editing. HV: Writing–review and editing. KK: Writing–review and editing. RK: Visualization, Writing–review and editing. JŠ: Writing–review and editing, Project administration.
Funding
The author(s) declare financial support was received for the research, authorship, and/or publication of this article. This research was funded by the Gregor Johann Mendel Grant Agency of the Mendel University in Brno (Czechia), within the project Landscape in Whole and Landscape in Detail - an Interdisciplinary Research of the Hranice Karst.
Acknowledgments
The authors greatly appreciate Michal Guba, Martin Strnad, Miroslav Lukáš, Petr Hřebejk, Jan Musil, Martin Prachař and other cave divers, members of the Czech Speleological Society 7–02 Hranický Karst, Czech Republic, for underwater sampling, as well as the staff of the Cave Authority of the Czechia, Zbrašov Aragonite Caves headquarters. We thank Veronika Matušková for help with field sampling and laboratory measurements. We thank Povodí Morava s. p. for allowing access to the sampling sites.
Conflict of interest
The authors declare that the research was conducted in the absence of any commercial or financial relationships that could be construed as a potential conflict of interest.
Publisher’s note
All claims expressed in this article are solely those of the authors and do not necessarily represent those of their affiliated organizations, or those of the publisher, the editors and the reviewers. Any product that may be evaluated in this article, or claim that may be made by its manufacturer, is not guaranteed or endorsed by the publisher.
Supplementary material
The Supplementary Material for this article can be found online at: https://www.frontiersin.org/articles/10.3389/fenvs.2024.1339818/full#supplementary-material
References
Alderton, I., Palmer, B. R., Heinemann, J. A., Pattis, I., Weaver, L., Gutiérrez-Ginés, M. J., et al. (2021). The role of emerging organic contaminants in the development of antimicrobial resistance. Emerg. Contam. 7, 160–171. doi:10.1016/j.emcon.2021.07.001
Andrade, A., and Stigter, T. (2009). Multi-method assessment of nitrate and pesticide contamination in shallow alluvial groundwater as a function of hydrogeological setting and land use. Agric. Water Manag. 96, 1751–1765. doi:10.1016/j.agwat.2009.07.014
Bakanov, N., Honert, C., Eichler, L., Lehmann, G. U., Schulz, R., and Brühl, C. A. (2023). A new sample preparation approach for the analysis of 98 current-use pesticides in soil and herbaceous vegetation using hplc-ms/ms in combination with an acetonitrile-based extraction. Chemosphere 331, 138840. doi:10.1016/j.chemosphere.2023.138840
Baran, N., Lepiller, M., and Mouvet, C. (2008). Agricultural diffuse pollution in a chalk aquifer (trois fontaines, France): influence of pesticide properties and hydrodynamic constraints. J. Hydrology 358, 56–69. doi:10.1016/j.jhydrol.2008.05.031
Baran, N., Surdyk, N., and Auterives, C. (2021). Pesticides in groundwater at a national scale (France): impact of regulations, molecular properties, uses, hydrogeology and climatic conditions. Sci. Total Environ. 791, 148137. doi:10.1016/j.scitotenv.2021.148137
Bexfield, L. M., Toccalino, P. L., Belitz, K., Foreman, W. T., and Furlong, E. T. (2019). Hormones and pharmaceuticals in groundwater used as a source of drinking water across the United States. Environ. Sci. Technol. 53, 2950–2960. doi:10.1021/acs.est.8b05592
Bhandari, G., Atreya, K., Scheepers, P. T., and Geissen, V. (2020). Concentration and distribution of pesticide residues in soil: non-dietary human health risk assessment. Non-dietary Hum. health risk Assess. Chemosphere 253, 126594. doi:10.1016/j.chemosphere.2020.126594
Brodin, T., Fick, J., Jonsson, M., and Klaminder, J. (2013). Dilute concentrations of a psychiatric drug alter behavior of fish from natural populations. Science 339, 814–815. doi:10.1126/science.1226850
Chen, M., Hong, Y., Jin, X., Guo, C., Zhao, X., Liu, N., et al. (2023). Ranking the risks of eighty pharmaceuticals in surface water of a megacity: a multilevel optimization strategy. Sci. Total Environ. 878, 163184. doi:10.1016/j.scitotenv.2023.163184
Chopra, S., and Kumar, D. (2020). Ibuprofen as an emerging organic contaminant in environment, distribution and remediation. Heliyon 6, e04087. doi:10.1016/j.heliyon.2020.e04087
Cioni, F., and Maines, G. (2010). Weed control in sugarbeet. Sugar Tech. 12, 243–255. doi:10.1007/s12355-010-0036-2
ČSN, EN (2018). ISO/IEC 17025: posuzování shody–Všeobecné požadavky na způsobilost zkušebních a kalibračních laboratoří. Praha: ČNI.
de Albuquerque, F. P., de Oliveira, J. L., Moschini-Carlos, V., and Fraceto, L. F. (2020). An overview of the potential impacts of atrazine in aquatic environments: perspectives for tailored solutions based on nanotechnology. Sci. Total Environ. 700, 134868. doi:10.1016/j.scitotenv.2019.134868
Dodgen, L., Kelly, W., Panno, S., Taylor, S., Armstrong, D., Wiles, K., et al. (2017). Characterizing pharmaceutical, personal care product, and hormone contamination in a karst aquifer of southwestern Illinois, USA, using water quality and stream flow parameters. Sci. total Environ. 578, 281–289. doi:10.1016/j.scitotenv.2016.10.103
Douglas, S. H., Dixon, B., and Griffin, D. (2018). Assessing the abilities of intrinsic and specific vulnerability models to indicate groundwater vulnerability to groups of similar pesticides. A Comp. study Phys. Geogr. 39, 487–505. doi:10.1080/02723646.2017.1406300
Dueñas-Moreno, J., Mora, A., Cervantes-Avilés, P., and Mahlknecht, J. (2022). Groundwater contamination pathways of phthalates and bisphenol a: origin, characteristics, transport, and fate-a review. Environ. Int. 170, 107550. doi:10.1016/j.envint.2022.107550
European Comission (2006a). Directive 2006/118/ec of the european parliament and of the council. https://eur-lex.europa.eu/LexUriServ/LexUriServ.do?uri=OJ:L:2006:372:0019:0031-EN:PDF.
European Comission (2006b). Directive 2006/118/ec on the protection of groundwater against pollution and deterioration. Available at: https://eur-lex.europa.eu/LexUriServ/LexUriServ.do?uri=OJ:L:2006:372:0019:0031.EN:PDF.
European Council (2000). Directive 2000/60/ec of the european parliament and of the council of 23 october 2000 establishing a framework for community action in the field of water policy. Available at: https://eur-lex.europa.eu/eli/dir/2000/60/oj.
European Union (2020). Directive 2020/2184 of the European Parliament and of the Council of 16 December 2020 on the quality of water intended for human consumption. Available at: https://eur-lex.europa.eu/legal-content/EN/TXT/?uri=CELEX:32020L2184.
Eurostat (2018). Archive:Agri-environmental indicator - pesticide pollution of water. Available at: https://ec.europa.eu/eurostat/statistics-explained/index.php?title=Archive:Agri-environmental_indicator_-_pesticide_pollution_of_water.
Fava, L., Orrù, M. A., Scardala, S., and Funari, E. (2007). Leaching potential of carbamates and their metabolites and comparison with triazines. Microchem. J. 86, 204–208. doi:10.1016/j.microc.2007.03.003
Ferrando, L., and Matamoros, V. (2020). Attenuation of nitrates, antibiotics and pesticides from groundwater using immobilised microalgae-based systems. Sci. Total Environ. 703, 134740. doi:10.1016/j.scitotenv.2019.134740
García, J., García-Galán, M. J., Day, J. W., Boopathy, R., White, J. R., Wallace, S., et al. (2020). A review of emerging organic contaminants (eocs), antibiotic resistant bacteria (arb), and antibiotic resistance genes (args) in the environment: increasing removal with wetlands and reducing environmental impacts. Bioresour. Technol. 307, 123228. doi:10.1016/j.biortech.2020.123228
Geissen, V., Silva, V., Lwanga, E. H., Beriot, N., Oostindie, K., Bin, Z., et al. (2021). Cocktails of pesticide residues in conventional and organic farming systems in europe–legacy of the past and turning point for the future. Environ. Pollut. 278, 116827. doi:10.1016/j.envpol.2021.116827
Gustafson, D. I. (1989). Groundwater ubiquity score: a simple method for assessing pesticide leachability. Environ. Toxicol. Chem. 8, 339–357. doi:10.1002/etc.5620080411
Horký, P., Grabic, R., Grabicová, K., Brooks, B. W., Douda, K., Slavík, O., et al. (2021). Methamphetamine pollution elicits addiction in wild fish. fish J. Exp. Biol. 224, jeb242145. doi:10.1242/jeb.242145
Huang, H., Liu, H., Xiong, S., Zeng, F., Bu, J., Zhang, B., et al. (2021). Rapid transport of organochlorine pesticides (ocps) in multimedia environment from karst area. Sci. Total Environ. 775, 145698. doi:10.1016/j.scitotenv.2021.145698
Hvězdová, M., Kosubová, P., Košíková, M., Scherr, K. E., Šimek, Z., Brodský, L., et al. (2018). Currently and recently used pesticides in central european arable soils. Sci. Total Environ. 613, 361–370. doi:10.1016/j.scitotenv.2017.09.049
Iker, B. C., Kambesis, P., Oehrle, S. A., Groves, C., and Barton, H. A. (2010). Microbial atrazine breakdown in a karst groundwater system and its effect on ecosystem energetics. J. Environ. Qual. 39, 509–518. doi:10.2134/jeq2009.0048
IUSS Working Group WRB (2015). World Reference Base for Soil Resources 2014, update 2015. International soil classification system for naming soils and creating legends for soil maps. World Soil Resources Reports No. 106. Rome: FAO.
Jablonowski, N. D., Schäffer, A., and Burauel, P. (2011). Still present after all these years: persistence plus potential toxicity raise questions about the use of atrazine. Environ. Sci. Pollut. Res. 18, 328–331. doi:10.1007/s11356-010-0431-y
Jakiene, E., Spruogis, V., Dautarte, A., Romaneckas, K., and Avizienyte, D. (2015). The bio-organic nano fertilizer improves sugar beet photosynthesis process and productivity. Zemdirbyste 102, 141–146. doi:10.13080/z-a.2015.102.018
Jones, E. R., Bierkens, M. F., Wanders, N., Sutanudjaja, E. H., van Beek, L. P., and van Vliet, M. T. (2022). Current wastewater treatment targets are insufficient to protect surface water quality. Earth Environ. 3, 221. doi:10.1038/s43247-022-00554-y
Karasali, H., Marousopoulou, A., and Machera, K. (2016). Pesticide residue concentration in soil following conventional and low-input crop management in a mediterranean agro-ecosystem, in central Greece. Sci. Total Environ. 541, 130–142. doi:10.1016/j.scitotenv.2015.09.016
Kidd, K. A., Blanchfield, P. J., Mills, K. H., Palace, V. P., Evans, R. E., Lazorchak, J. M., et al. (2007). Collapse of a fish population after exposure to a synthetic estrogen. Proc. Natl. Acad. Sci. 104, 8897–8901. doi:10.1073/pnas.0609568104
Kolpin, D. W., Barbash, J. E., and Gilliom, R. J. (1998). Occurrence of pesticides in shallow groundwater of the United States: initial results from the national water-quality assessment program. Environ. Sci. Technol. 32, 558–566. doi:10.1021/es970412g
Kronvang, B., Wendland, F., Kovar, K., and Fraters, D. (2020). Land use and water quality. Encycl. hydrological Sci. 2020. doi:10.3390/w12092412
Kruć-Fijałkowska, R., Dragon, K., Drożdżyński, D., and Górski, J. (2022). Seasonal variation of pesticides in surface water and drinking water wells in the annual cycle in western Poland, and potential health risk assessment. Sci. Rep. 12, 3317. doi:10.1038/s41598-022-07385-z
Leverett, D., Merrington, G., Crane, M., Ryan, J., and Wilson, I. (2021). Environmental quality standards for diclofenac derived under the European Water Framework Directive: 1. Aquatic organisms. Aquat. Org. Environ. Sci. Eur. 33, 133. doi:10.1186/s12302-021-00574-z
Luo, Y., Guo, W., Ngo, H. H., Nghiem, L. D., Hai, F. I., Zhang, J., et al. (2014). A review on the occurrence of micropollutants in the aquatic environment and their fate and removal during wastewater treatment. Sci. total Environ. 473, 619–641. doi:10.1016/j.scitotenv.2013.12.065
Mali, H., Shah, C., Raghunandan, B., Prajapati, A. S., Patel, D. H., Trivedi, U., et al. (2023). Organophosphate pesticides an emerging environmental contaminant: pollution, toxicity, bioremediation progress, and remaining challenges. J. Environ. Sci. 127, 234–250. doi:10.1016/j.jes.2022.04.023
Menchen, A., Heras, J. D., and Alday, J. J. G. (2017). Pesticide contamination in groundwater bodies in the júcar river European Union pilot basin (se Spain). Environ. Monit. Assess. 189, 146–218. doi:10.1007/s10661-017-5827-4
Metcalfe, C. D., Beddows, P. A., Bouchot, G. G., Metcalfe, T. L., Li, H., and Van Lavieren, H. (2011). Contaminants in the coastal karst aquifer system along the Caribbean coast of the Yucatan Peninsula, Mexico. Mex. Environ. Pollut. 159, 991–997. doi:10.1016/j.envpol.2010.11.031
Morasch, B. (2013). Occurrence and dynamics of micropollutants in a karst aquifer. Environ. Pollut. 173, 133–137. doi:10.1016/j.envpol.2012.10.014
Mouvet, C., Jeannot, R., Riolland, H., and Maciag, C. (1997). Stability of isoproturon, bentazone, terbuthylazine and alachlor in natural groundwater, surface water and soil water samples stored under laboratory conditions. Chemosphere 35, 1083–1097. doi:10.1016/s0045-6535(97)00174-4
Oppeltová, P., Vavrouchová, H., Sedláček, J., Geršl, M., Ulrich, O., Vlček, V., et al. (2022). Spatial conflicts management in hranice karst with emphasis on nature protection and tourist management. Křtiny, Czech Republic: Mendel University Press.
Pérez-Lucas, G., Vela, N., El Aatik, A., and Navarro, S. (2019). Environmental risk of groundwater pollution by pesticide leaching through the soil profile. Pesticides-use misuse their impact Environ. 2019, 1–28. doi:10.5772/intechopen.82418
Park, W.-P., Chang, K.-M., Hyun, H.-N., Boo, K.-H., and Koo, B.-J. (2020). Sorption and leaching characteristics of pesticides in volcanic ash soils of jeju island. korea Appl. Biol. Chem. 63, 1–13. doi:10.1186/s13765-020-00555-5
Perera-Rios, J., Ruiz-Suarez, E., Bastidas-Bastidas, P. J., May-Euán, F., Uicab-Pool, G., Leyva-Morales, J. B., et al. (2022). Agricultural pesticide residues in water from a karstic aquifer in yucatan, Mexico, pose a risk to children’s health. Int. J. Environ. Health Res. 32, 2218–2232. doi:10.1080/09603123.2021.1950652
Peters, A., Crane, M., Merrington, G., and Ryan, J. (2022). Environmental quality standards for diclofenac derived under the european water framework directive: 2. Avian Second. poisoning Environ. Sci. Eur. 34, 1–16. doi:10.1186/s12302-022-00601-7
Riedo, J., Wächter, D., Gubler, A., Wettstein, F. E., Meuli, R. G., and Bucheli, T. D. (2023). Pesticide residues in agricultural soils in light of their on-farm application history. Environ. Pollut. 331, 121892. doi:10.1016/j.envpol.2023.121892
Ruomeng, B., Meihao, O., Siru, Z., Shichen, G., Yixian, Z., Junhong, C., et al. (2023). Degradation strategies of pesticide residue: from chemicals to synthetic biology. Synthetic Syst. Biotechnol. 8, 302–313. doi:10.1016/j.synbio.2023.03.005
Schäfer, R. B., Kühn, B., Malaj, E., König, A., and Gergs, R. (2016). Contribution of organic toxicants to multiple stress in river ecosystems. Freshw. Biol. 61, 2116–2128. doi:10.1111/fwb.12811
Silva, V., Mol, H. G., Zomer, P., Tienstra, M., Ritsema, C. J., and Geissen, V. (2019). Pesticide residues in european agricultural soils–a hidden reality unfolded. Sci. Total Environ. 653, 1532–1545. doi:10.1016/j.scitotenv.2018.10.441
Sorensen, J., Lapworth, D., Nkhuwa, D., Stuart, M., Gooddy, D., Bell, R., et al. (2015). Emerging contaminants in urban groundwater sources in africa. Water Res. 72, 51–63. doi:10.1016/j.watres.2014.08.002
Špaček, P., Bábek, O., Štěpančíková, P., Švancara, J., Pazdírková, J., and Sedláček, J. (2015). The nysa-morava zone: an active tectonic domain with late cenozoic sedimentary grabens in the western carpathians’ foreland (ne bohemian massif). Int. J. Earth Sci. 104, 963–990. doi:10.1007/s00531-014-1121-7
Šráček, O., Geršl, M., Faimon, J., and Bábek, O. (2019). The geochemistry and origin of fluids in the carbonate structure of the Hranice Karst with the world's deepest flooded cave of the Hranicka Abyss, Czech Republic. czech Repub. Appl. Geochem. 100, 203–212. doi:10.1016/j.apgeochem.2018.11.013
Stoppiello, M. G., Lofrano, G., Carotenuto, M., Viccione, G., Guarnaccia, C., and Cascini, L. (2020). A comparative assessment of analytical fate and transport models of organic contaminants in unsaturated soils. Sustainability 12, 2949. doi:10.3390/su12072949
Syafrudin, M., Kristanti, R. A., Yuniarto, A., Hadibarata, T., Rhee, J., Al-Onazi, W. A., et al. (2021). Pesticides in drinking water—a review. Int. J. Environ. Res. public health 18, 468. doi:10.3390/ijerph18020468
Tran, H. T., Lin, C., Bui, X.-T., Nguyen, M. K., Cao, N. D. T., Mukhtar, H., et al. (2022). Phthalates in the environment: characteristics, fate and transport, and advanced wastewater treatment technologies. Bioresour. Technol. 344, 126249. doi:10.1016/j.biortech.2021.126249
Troldborg, M., Gagkas, Z., Vinten, A., Lilly, A., and Glendell, M. (2022). Probabilistic modelling of the inherent field-level pesticide pollution risk in a small drinking water catchment using spatial bayesian belief networks. Hydrology Earth Syst. Sci. 26, 1261–1293. doi:10.5194/hess-26-1261-2022
USEPA (2016). Sci-grow description. Washington, DC: United States Environmental Protection Agency. Available at: https://archive.epa.gov/epa/pesticide-science-and-assessing-pesticide-risks/sci-grow-description.html.
Vidal, J., Báez, M. E., Calzadilla, W., Aranda, M., and Salazar, R. (2022). Removal of chloridazon and its metabolites from soil and soil washing water by electrochemical processes. Electrochimica Acta 425, 140682. doi:10.1016/j.electacta.2022.140682
Vysoká, H., Bruthans, J., Falteisek, L., Žák, K., Rukavičková, L., Holeček, J., et al. (2019). Hydrogeology of the deepest underwater cave in the world: Hranice Abyss, Czechia. Journal 27, 2325–2345. doi:10.1007/s10040-019-01999-w
Wachniew, P., Zurek, A. J., Stumpp, C., Gemitzi, A., Gargini, A., Filippini, M., et al. (2016). Toward operational methods for the assessment of intrinsic groundwater vulnerability: a review. A Rev. Crit. Rev. Environ. Sci. Technol. 46, 827–884. doi:10.1080/10643389.2016.1160816
Wauchope, R. D., Buttler, T., Hornsby, A., Augustijn-Beckers, P., and Burt, J. (1992). The scs/ars/ces pesticide properties database for environmental decision-making. Rev. Environ. Contam. Toxicol. 123, 1–155. doi:10.1007/978-1-4612-2862-2_1
Wellington, E. M., Boxall, A. B., Cross, P., Feil, E. J., Gaze, W. H., Hawkey, P. M., et al. (2013). The role of the natural environment in the emergence of antibiotic resistance in gram-negative bacteria. Lancet Infect. Dis. 13, 155–165. doi:10.1016/s1473-3099(12)70317-1
Wilkinson, J. L., Boxall, A. B., Kolpin, D. W., Leung, K. M., Lai, R. W., Galbán-Malagón, C., et al. (2022). Pharmaceutical pollution of the world’s rivers. Proc. Natl. Acad. Sci. 119, e2113947119. doi:10.1073/pnas.2113947119
Zemann, M., Majewsky, M., and Wolf, L. (2016). Accumulation of pharmaceuticals in groundwater under arid climate conditions–results from unsaturated column experiments. Chemosphere 154, 463–471. doi:10.1016/j.chemosphere.2016.03.136
Zhang, H., Luo, Y., Zhao, Q., Wong, M. H., and Zhang, G. (2006). Residues of organochlorine pesticides in Hong Kong soils. Chemosphere 63, 633–641. doi:10.1016/j.chemosphere.2005.08.006
Keywords: pesticide residues, pharmaceuticals, field sampling, contamination control, Karst aquifers, groundwater
Citation: Oppeltová P, Vlček V, Geršl M, Chaloupský P, Ulrich O, Sedláček J, Vavrouchová H, Kohoutková K, Klepárník R and Šimečková J (2024) Occurrence and path pollution of emerging organic contaminants in mineral water of Hranice hypogenic Karst. Front. Environ. Sci. 12:1339818. doi: 10.3389/fenvs.2024.1339818
Received: 16 November 2023; Accepted: 18 January 2024;
Published: 05 February 2024.
Edited by:
Abdul Qadeer, Chinese Research Academy of Environmental Sciences, ChinaReviewed by:
Zeljko Jaksic, Rudjer Boskovic Institute, CroatiaAndrés Perez Parada, Universidad de la República, Uruguay
Copyright © 2024 Oppeltová, Vlček, Geršl, Chaloupský, Ulrich, Sedláček, Vavrouchová, Kohoutková, Klepárník and Šimečková. This is an open-access article distributed under the terms of the Creative Commons Attribution License (CC BY). The use, distribution or reproduction in other forums is permitted, provided the original author(s) and the copyright owner(s) are credited and that the original publication in this journal is cited, in accordance with accepted academic practice. No use, distribution or reproduction is permitted which does not comply with these terms.
*Correspondence: Petra Oppeltová, oppeltova@mendelu.cz