- 1Department of Community Medicine, KMU-Institute of Medical Sciences, Kohat, Khyber Pakhtunkhwa, Pakistan
- 2Department of Community Medicine, Nowshera Medical College, Nowshera, Khyber Pakhtunkhwa, Pakistan
- 3Department of Earth and Environmental Sciences, Bahria University, Islamabad, Pakistan
- 4Department of Biological, Geological and Environmental Sciences, Alma Mater Studiorum University of Bologna, Bologna, Italy
- 5School of Environmental Studies, China University of Geosciences, Wuhan, China
- 6Department of Environmental Sciences, Kohat University of Science and Technology, Kohat, Pakistan
- 7Department of Zoology, College of Science, King Saud University, Riyadh, Saudi Arabia
- 8Imperial College London, London, United Kingdom
Groundwater quality was investigated in three urban semi-arid multi-industrial metropolitan areas i.e. (Hayatabad Industrial Estate, Peshawar (HIEP), Gadoon Industrial Estate, Swabi (GIES) and Hattar Industrial Estate, Haripur (HIEH). The main aim of the study was to determine their physicochemical parameters, potentially harmful elements (PHEs) concentration, pollution sources and public health risks in semi-arid multi-industrial metropolitan areas of Khyber Pakhtunkhwa (KPK), Pakistan. The physicochemical parameters (pH, TDS and EC), PHEs (Cd, Zn, Pb, Cr and Ni) concentrations and Mg in drinking water sources were found within the permissible limits, except Fe, which exceeded the World Health Organization (WHO) acceptable limit. Among PHEs, Zn had the highest contribution rates of 69.6%, 58.2% and 67.64% in HIEP, GIES and HIEH, respectively, while Cd showed the lowest contribution rates (3.15%, 1.98% and 2.06%) for HIEP, GIES and HIEH respectively. Principal component analysis (PCA) showed significant correlations between parameters, with contributions of industrial effluents and wastewater discharge (46.81%), mixed sources (34.05%) and geo-genic sources (19.14%) for drinking water in the study area. The carcinogenic risk (CR) for Cd, Cr, Ni and Pb, were found within the acceptable threshold value of 1 × 10−4. This study suggests that urban groundwater should also be regularly monitored for PHEs contamination as over-extraction, industrialization and informal E-waste recycling events surges the public health risks globally, facing related environmental contamination difficulties of the urban groundwater.
Highlights
• The presence of potential harmful elements (Cd, Zn, Pb, Cr, and Ni) in wastewater of three industrial estates and in local ground water were assessed
• The quantity of harmful elements in ground water were compared with WHO standards
• The antagonistic and synergistic effects of the mentioned toxic elements on local ground water were summarized
• Recommendations were suggested for the mitigation of the toxic effects of the potential harmful elements
Introduction
Contamination by PHEs has increased due to unplanned urbanization and rapid industrialization, leading to adverse health risks. The industrial revolution is responsible for severe water contamination problems due to the discharge of potentially toxic chemicals into the receiving water bodies (Hafeez et al., 2020). Generally, PHEs originate from different heavy manufacturing industries including metallurgy, electroplating, civil construction, paint, and ceramic ware factories, and are regularly discharged into the aquatic environment through industrial wastewater (Chandra et al., 2017; Jordanova et al., 2018; Ullah et al., 2019; Hoang et al., 2021; Roy et al., 2021; Xiao et al., 2021). Wastewater discharge from industrial activities containing PHEs (Pb, Cr, Cd, Cu and Zn) heavily deteriorates the drinking water quality (Sajjad et al., 2017; Yin et al., 2018; Singh and Chandra, 2019; Kumar et al., 2020; Ghani et al., 2022a; Ghani et al., 2022b), and causes severe environmental impacts due to its bioaccumulation and high toxicity (Jia et al., 2020; Xia et al., 2020; Zhang R. et al., 2020; Lv et al., 2021). Industrial development has resulted in the discharge of PHEs into water resources and the surroundings (Islam et al., 2015; Sanad et al., 2021). Currently, metal smelting and other industrial activities are major sources of PHEs worldwide (Qu et al., 2020; Sharma et al., 2020). The paper manufacturing industries including writing paper industries and hardboard waste discharge also contribute to the release of various PHEs, mainly including Fe, Mg, Cd, Zn, Cu, Cr, Ni, and Pb (Singh and Chandra, 2019). Other manmade sources of PHEs include intensive agriculture practices, smelting and mining, sewage sludge, landfills and municipal solid waste (Nawab et al., 2018a; Nawab et al., 2018b; Nawab et al., 2019). Natural sources also contribute to the release of PHEs which include cadmium (Cd), chromium (Cr), nickel (Ni) lead (Pb), and zinc (Zn) to the environment from the weathering of old rocks in addition to volcanic eruption (Nawab et al., 2017; Nawab et al., 2018a; Liu et al., 2020). These hazardous PHEs enter aquatic systems due to industrial operational activities (Mahmoud et al., 2016). According to the US Environmental Protection Agency (US-EPA), PHEs like Cd, Cr, Pb, Ni and Zn are among the chemicals considered to be high priority pollutants due to their high toxicity (EPA, 2014). Thus, it is important to determine and mitigate the potential risk of PHEs released from intensive industrial activities.
PHEs have been extensively studied as inorganic contaminants due to their toxicity, persistent nature, and bioaccumulation in the food chain, thereby imposing high human health risk worldwide (Machado et al., 2017; Baken et al., 2018; Saleem et al., 2019; Ayejoto and Egbueri, 2023; Boum-Nkot et al., 2023). PHEs such as Cd, and Pb are among the hazardous chemicals having strongly toxic characteristics (Souza et al., 2017). These PHEs enter the human body through the exposure routes of water and food ingestion, inhalation, and dermal contact with surface water (Tayebi and Sobhanardakani, 2020). Pb poisoning may disrupt the central nervous system and cause disorders of the kidneys and gastrointestinal system (Palin et al., 2016), and Cd causes emphysema, endocrine andoxidative damage, anemia, and hypertension (Jain et al., 2015; Zhang et al., 2019). These lethal PHEs are listed as adverse environmental endocrine disruptors by the US-EPA, due to their disruptive endocrine-like properties (Kim et al., 2015). Source identification and risk assessment of PHEs in aquatic environments have been well-documented for industrial estates worldwide, but the relationship between pollution sources and health risks in drinking water sources is still unclear (Men et al., 2020). Furthermore, it has been reported that in Pakistan, industrial workers were incidentally visible to dermal uptake of toxic PHEs due to the absence of irrigation safety procedures (Muhammad et al., 2021). The high health risks posed by long-term exposure to PHEs in drinking water sources cannot be ignored. Hence, it is necessary to quantify the pollution sources of PHEs in less-studied industrial estates and identify potential health risks posed to the residents in the study area.
In the past, limited studies related to the health risks posed by PHEs in drinking groundwater sources of industrial estates has been conducted in developing countries such as in Pakistan. To fill this research gap, a comprehensive scientific study of further research is still needed to investigate the PHEs contamination in groundwater and reduces its potential sources to minimize the health risks in industrial regions via different exposure pathways (ingestion and dermal contact) of drinking water. This research could provide a deep understanding of scientific support to control PHEs contamination in the context of improving healthy and stabilized urban environments worldwide. The main objective of this study was to assess the pollution levels of PHEs and their associated health risks in the urban industrial estates of Hayatabad Industrial Estate, Peshawar (HIEP), Gadoon Industrial Estate, Swabi (GIES), and Hattar Industrial Estate, Haripur (HIEH). The objectives of the study were i) to conduct physiochemical analysis and assess the PHE concentrations (Cd, Zn, Pb, Cr, and Ni) in industrial estates drinking water sources, ii) to determine the contamination degree of drinking water and the pollution sources of PHEs, and iii) to perform health risk assessment (carcinogenic and non-carcinogenic) via different exposure routes for local inhabitant adults and children.
Methods and materials
Study area description
The HIEP is in the northwest region of Peshawar on the main Jamrud Road in Khyber Pakhtunkhwa (KPK) province, Pakistan (Figure 1). HIEP comprises of 372 large installed factories, and 246 are currently working at full scale (Sarhad development authority, 2016). The allocated main water drain passes through the industrial area and carries the effluents produced by the HIEP in Peshawar. The effluents are drained away into the main rivers close to the factories (Jan et al., 2010), which causes the water quality of River Kabul to be deteriorated by industrial wastewater and affects the quality of water used for fish farming and irrigation purposes (Inam ullah and Alam, 2014). Gadoon Industrial Estate Swabi (GIES) is situated in the Swabi district area of KPK province, at 72032’ 45” E and 3305’20” N at 328 m altitude above sea level. The GIES has about 310 operational industrial units. The urban industrial entities in GIES usually discharge wastewater into the region without any proper treatment. The industrial estate wastewater has been used for irrigation purposes in adjacent agricultural fields, due to the limited water supply (Muhammad et al., 2021).
The HIEH is in Haripur district of KPK province, at 72°51′8 E and 33°51′71 N, as shown in Figure 1. The HIEH has a total of 215 industrial functional units, 69 units still under construction and 91 that are closed. Wastewater, solid wastes, and atmospheric air pollutants are generated in functional industrial units, which are thereby dumped and discharged into the surrounding environment without any proper treatment (Khan et al., 2016). The local population in the HIEP, GIES, and HIEH is dependent on the use of groundwater and surface water resources. Therefore, monitoring of drinking water resources is mandatory to control pollution (Hussain et al., 2008).
Geology and hydrogeology of the study area
Peshawar city is surrounded by high mountains in the southeast, southwest and in the west, creating a complex of metamorphic and sedimentary rocks that are undulated and distorted. The study area is surrounded by alluvial deposits, eroded from the adjacent mountains. The west and southwest terrain is covered by sand and cemented gravels in Peshawar, and water percolates through these sediments to recharge the groundwater. The land surrounding the mountains in the study area’s west, and south-west is porous and significantly affects the recharge to the ground.
Based on their hydrogeological characteristics and borehole drilling research, shallow and deep aquifers have been identified in Peshawar District and have been defined as two hydrogeological units (UPU, 2014). The depth of the groundwater table (aquifer’s depth) in the district and industrial estate’s regions ranged from 210 m to 515 m (Khan and Ali, 2019). The industrial estates (HIEP, GIES, and HIEH) have natural surface drains and industrial effluents and wastewater, release to nearby surface water bodies and resulting to reach the groundwater. At the Dingi village, all of the natural drains carrying hazardous substances from various industries like adjacent HEI effluents enter the “Jari Kass” nullah. The main sources for groundwater recharge near HIEP are the Rivers Kabul and Bara, while the main source for groundwater recharge near GIES, and HIEH is Tarbela Dam. However, the process is also aided by irrigation canals, streams, and precipitation. The primary rivers of Haripur near HIEH are the Dor and Haro Rivers, which are refilled by snowmelt, rainfall and finally reach the groundwater system.
Drinking water sampling
A total of (n = 90) water samples were collected from groundwater in polythene bottles in August 2020. The water samples were collected from the industrial estates of HIEP (n = 30), GIES (n = 30) and HIEH (n = 30). The geological coordinates of sampling locations of drinking water samples in HIEP, GIES, and HIEH are presented in Supplementary Table S1. During sampling, water was permitted to run for a while before the sampling collection from targeted points, of specific regions in the industrial estates. The polyethylene bottles were first rinsed with distilled water and after drying it, water samples were collected in these. Prior testing for the toxic metals, few drops of acid (HNO3, 0.5% v/v) were added to these bottles. The collected water samples were filtered using Whatman filter paper (0.45 μm). Samples were carefully sealed, labeled and preserved following the procedures adopted by Muhammad et al. (2021).
Sample analysis
The concentrations and physicochemical parameters and six selected PHEs in the drinking water samples of all industrial estates were analyzed by standard methods. The original water samples of the industrial estates were directly used for the analysis of physicochemical parameters. pH was determined utilizing a digital pH meter (Model C93, Turnhout, Belgium), total dissolved solids (TDS) by a TDS meter (Model S518877), and electrical conductivity (EC) was measured using a conductivity meter (Model HI98303). Standard solutions of corresponding metals were arranged by diluting standard solutions of 1000 mg/L (FlukaKamica, Buchs, Switzerland). The samples were analyzed for PHEs including cadmium (Cd), chromium (Cr), magnesium (Mg), lead (Pb), zinc (Zn), nickel (Ni) and iron (Fe) by graphite furnace atomic absorption spectrophotometry (PerkinElmer, United States of America, ASS-PEA- 700) in the Centralized Resources Laboratory of Chemistry, in Kohat University of Science and Technology, at Kohat Pakistan, using standard procedures adopted by Nawab et al. (2016).
Quality assurance and quality control
For quality control of analysis of PHEs, atomic absorption spectrometry (AAS) was calibrated with a certified standard solution (1,000 mg L−1) of deionized water (DI) from Fluka Kamica (Buchs, Switzerland). Sample analysis was done for PHEs analysis in triplicates with normal and optimum conditions of AAS with the error (>0.999). Recovery and reproducibility of all the findings were detected at high assurance levels of 93 + 7 and 91 + 6. Overall, mean results were found and used for the data analysis. Grade analytical reagents (Merck, Germany) with the analytical grade of spectroscopic purity (99.9%) were used to ensure overall quality of the data. Clean glassware and new plastic items were used and thoroughly washed with solution (10% HNO3) of DI water and then dried in the oven. During analysis, 5 seconds of delay time was used during integration in AAS. The reagent blanks samples, and certified standards solution were used in different concentrations. Calibration curves of multielement standard solution were prepared after a dilution to μg/L levels.
Contamination factor
The point of PHE contamination can be determined for polluted and background sites as defined by Hakanson (1980), which can be calculated as;
Where Cm represents the concentration of PHEs in water samplesand Cb is the background PHE level. The observed CF values show the contamination level of water samples, such as the CF > 6: high level of contamination; 3 ≤ CF ≤ 6: significant high contamination; 1 ≤ CF ≤ 3: moderate contamination, while CF < 1: low contamination (Abdullah et al., 2011).
Pollution load index
The Pollution Load Index (PLI) specifies the whole pollution level of PHEs in water bodies. PLI was evaluated for each industrial estate site (Muhammad et al., 2011) by Eq. 2:
Where n indicates the number of PHEs, and CF shows the contamination factor. The pollution level of the studied industrial areas has been classified based on the background level to the high polluted samples on the basis of the observed PLI values. A PLI value > 1 indicates pollution, while a PLI value < 1 shows no or zero pollution (Ranjan et al., 2016).
Water quality index
The water quality index (WQI) method used to determine water quality of the industrial estates. It can be used to reflect the overall pollution level of water bodies by evaluating the degree of PHE pollution (WHO, 2017). WQI can be calculated by the following Eq. 3, followed by Ghani et al. (2022a):
Where n is the number of total parameters and WPI can be calculated to combine the contamination load of all contaminants with the observed PLI value. The WPI classifies the water contamination into four different classes, with WPI values (<0.5) representing excellent water quality; WPI (0.5–0.75) directs good water quality; WPI (0.75 and 1) specifies moderately contaminated water; and WPI (>1) shows highly contaminated water (Hossain and Patra, 2020).
Principal components analysis multiple linear regression (PCA−MLR)
PCA−MLR is a multivariate statistical technique that can be used for PHEs source identification (Huang et al., 2021). It can be used to extract the important factors from various parameters in big datasets (Rashid et al., 2021), and can be also used to reduces and convert the datasets into simple information by providing a fundamental composition within the whole data. In the present study, PCA−MLR was applied to extract the significant factors, with the obtained eigenvalues (>1) and the total variance (>75%) (Wu et al., 2014). PCA−MLR can be computed and expressed by the following Eq. 4:
Where y signifies the dependent variable, x1 … xm represents the independent variables. b0 … bm indicates the coefficients regression and E shows the random error (Wang et al., 2020).
Correlation analysis
The correlation analysis was applied to find the relationship among parameters on the basis of Pearson’s correlation coefficient (Wu et al., 2014). Pearson’s correlation coefficient (r) can be calculated by the given Eq. 5.
Where r is the correlation coefficient of the two variables (x and y). The correlation coefficient (r) values are generally found in the range from -1 to 1. r > 0 indicates a significant positive correlation and r < 0 shows a negative correlation between the variables. While r = 0 signifies no correlation among the variables. Overall, the results of the correlation analysis show low, moderate, and high correlation among variables.
Health risk assessment
The health risk assessment model was applied to measure the carcinogenic as well as non-carcinogenic effects on humans contact with PHEs, developed by the US-EPA (Zhang et al., 2021). The present study divided the population into two different age groups (adults and children) for accurate assessment of potential risks by considering the variation in vulnerability rates.
Exposure assessment
The population (adults and children) can be exposed to toxic PHEs by three exposure corridors, comprising inhalation, dermal contact and through ingestion (USEPA, 1997). The average daily intake (DMI) was calculated for ingestion and dermal contact, using Eqs 6, 7:
where DMI is daily metal intake (μg/L); C is PHE concentration; EF indicates exposure frequency (350 days/year for both the children and adults, ED indicates the exposure duration (6 years for children and 30 years for adults), IngR indicates the ingestion rate for adults (100 mg/day) as well as children (200 mg/day), BW is the average body weight (16.2 kg for children and 61.8 kg for adults), ATnc is for the average time for non-carcinogens (ED×365 days for both the adults and children), SL indicates the skin adherence factor (0.2 mg/cm2 for children and 0.07 mg/cm2 for adults), SA is exposed skin area (2800 cm2 for children and 5700 cm2 for adults), and ABS is dermal absorption factor (0.001 (non-carcinogen) and 0.01 (carcinogen)) (USDOE, 2011).
Risk characterization
The non-carcinogenic risks (Non-CR) were identified for each PHE by calculating the hazard quotients (HQ) (USEPA, 2007) with the following Eq. 8:
The hazard index (HI) was applied for the whole assessment of non-carcinogenic risk posed by PHEs via different exposure pathways. HI was determined from Eq. 9 (Xiao et al., 2020);
Both the HI and HQ were used to calculate the non-carcinogenic risk (Gu et al., 2016). HQ < 1 show no adverse health risk, while HQ > 1 indicates adverse health consequences for the human population. Likewise, HI ≤ 1 indicated as no significant risk of non-carcinogenic effects. HI > 1 was defined as showing the occurrence of non-carcinogenic affects, with the potential effects increasing by the increasing of HI values.
Carcinogenic risk (CR) denotes the absolute likelihood of an individual having cancer disease by adverse exposure to hazardous pollutants in a lifetime, which can be calculated by Eq. 10 (Kumar et al., 2019; Ghani et al., 2022b, 2022c). In CR calculation, CSF is the cancer slope factor of the selected PHEs.
Where CSF and reference dose (RfD) values of exposure pathways for non-carcinogenic risk were used as the guidelines suggested by the US Environmental Protection Agency (USEPA, 2010; USEPA, 2013), as shown in Supplementary Table S2. The acceptable CR level for regulatory purposes in the proposed guideline falls between 1 × 10–6 and 1 × 10–4 (Gu et al., 2016).
Statistical analysis
The obtained experimental information data was analyzed statistically utilizing Statistix 10 (Analytical software, TN, United States). Origin Lab (version 2018) was used to plot graphs and pollution source parameters were studied using IBM SPSS (version 20). Arc-GIS 10.6 software was used for the study area location map.
Results and discussion
Physiochemical parameters and PHE concentrations in drinking water sources
The descriptive statistical data of physicochemical parameters, PHE concentrations, and contribution rates for the freshwater sources in industrial estates are presented in Table 1 and Figure 2. Drinking water sources have neutral pH values varying from 6.40 to 7.10 with an average of 6.68. Similarly, the pH values in the present study were slightly alkaline and ranged from 6.60 to 7.60 and 6.70 to 7.40 with average values of 7.10 and 7.06 for GIES and HIEH, respectively. These pH values depict the neutral to alkaline nature of the drinking water sources (Talib et al., 2019).
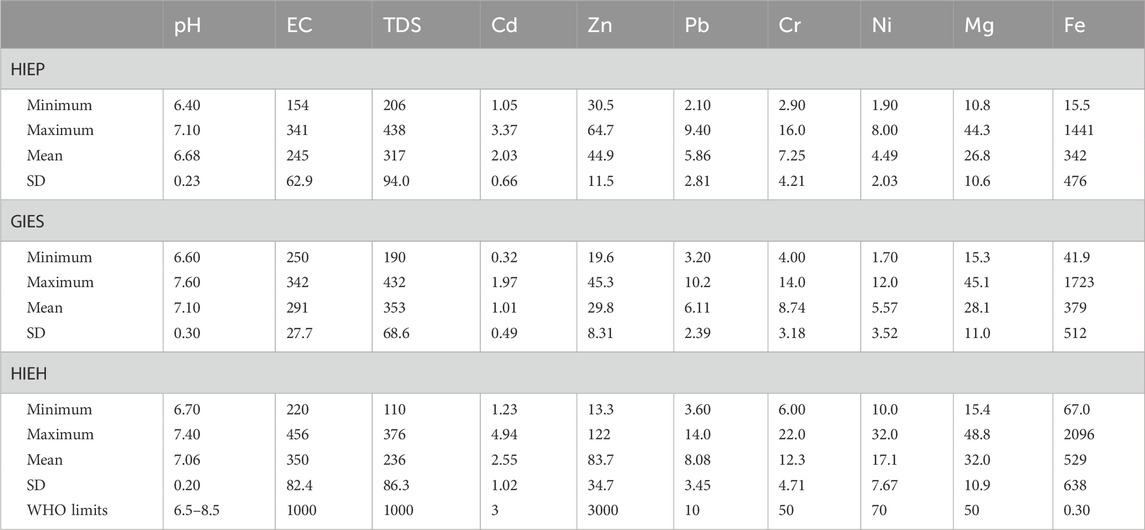
TABLE 1. Descriptive statistics of selected parameters in drinking water sources of HIEP, GIES, and HIEH.
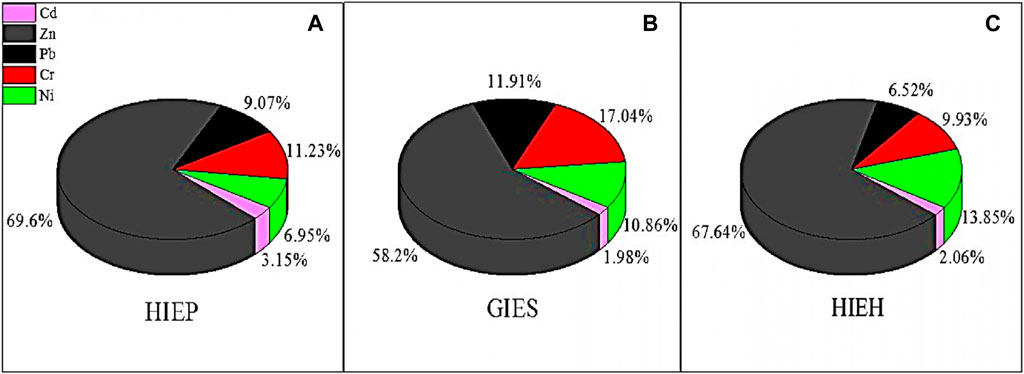
FIGURE 2. Contribution (percentage) of PHEs in drinking water sources of industrial estates (A) HIEP, (B) GIES, and (C) HIEH.
The EC had low mean values of 245, 291, and 350 μS/cm, while TDS concentrations were also observed to have low mean values of 317, 353, and 236 mg/L the drinking water sources of HIEP, GIES, and HIEH, respectively. The values of EC and TDS are directly proportional to each other (Liu and Liptak, 1999). Slight variations in the EC, TDS and pH could be attributed to water-rock interaction under the influence of anthropogenic activities, thereby leading to the solubilization of salts and minerals (Ravikumar et al., 2011). The parameters pH, EC, and TDS concentration were found to be within the drinking water quality guidelines of WHO (2011), PAK-EPA, US limits, and Canadian limits (Supplementary Table S3).
PHEs had low to moderate concentrations in all drinking water sources of industrial estates compared with previous studies, as shown in Table 2. For instance, Zn had high concentrations ranged from (13.3–122 μg/L) with a mean value of (83.7 μg/L) in HIEH, while Cd showed the lowest concentration and ranged from 1.05 to 3.37 μg/L with mean value of 2.03 μg/L in HIEP. The Cd mean concentration was found to be higher than in a previous study of steel industries in Yazd city, Iran (Mousavian et al., 2017), and Uttar Pradesh, India (Sharma et al., 2020). Among PHEs, Zn had the highest contribution rates of 69.6%, 58.2%, and 67.64%, while Cd showed the lowest contribution rates (3.15%, 1.98% and 2.06%) for HIEP, GIES and HIEH, respectively as shown in Figures 2A–C. Zn showed the highest concentrations among the PHEs in all industrial estates, followed by Pb and Cr, which could be possibly have resulted from the high degree of industrialization and other anthropogenic inputs like the deposition of particles of aerosol produced by traffic emission in addition to industrial processes (Mico et al., 2006).
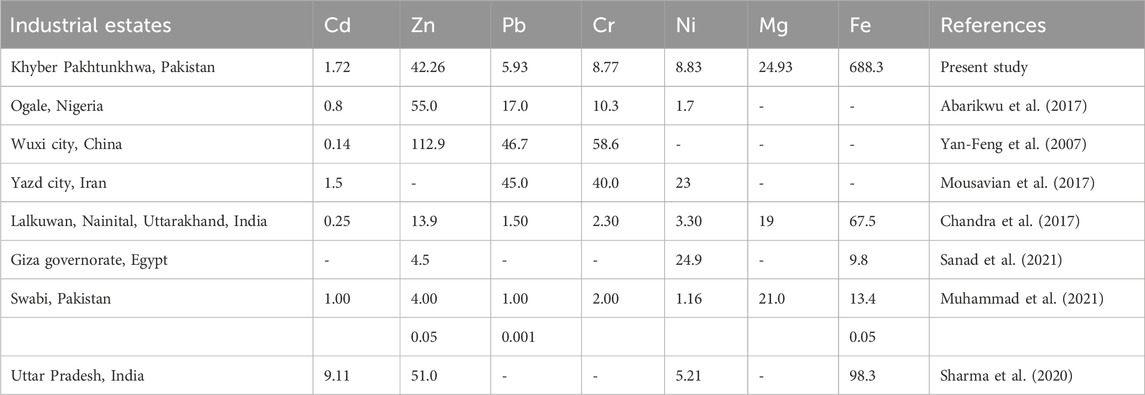
TABLE 2. PHEs mean concentration of industrial estates reported in different regions around the world.
However, all PHEs concentrations (Cd, Cr, Ni, Pb and Zn) as well as the Mg mean concentrations were found to be within the drinking water quality guidelines, although Fe exceeded the permissible WHO limit (2011) and the other different organizations. Fe had the most elevated mean concentrations of (342, 379, and 529) mg/L in HIEP, GIES, and HIEH, respectively, which were above the WHO, US and Canadian guideline value. High Fe concentrations were also found in the effluent discharge from the industrial zones of Hayatabad, Peshawar, which were substantially higher than the other PHEs in the studied area (Amin et al., 2014), and also higher than those in the Lalkuwan, Nainital, and Uttarakhand regions in India (Chandra et al., 2017) (see Table 2). Despite the importance of Fe to the body, elevated Fe of >200 ppm in drinking water may cause serious problems such as damage to the liver, heart, pancreas, and bone marrow. Water-soluble binary compounds of Fe including FeSO4, and FeCl2 may cause toxic effects at 200 mg/L (Xiaoshuai et al., 2009). Mg showed moderate mean concentrations of (26.8, 28.1, and 32.0) mg/L in HIEP, GIES, and HIEH respectively. Elevated Mg concentrations can be found in natural water bodies. Consumption of drinking water with high Mg concentrations results in harmful laxative effects to humans, while its deficiency may alter the structural and functional systems (Hem, 1985). In the present study, Zn, Fe and Mg were found to be high compared with the other parameters, and these metals are toxic beyond certain permissible levels (Metcalf Eddy Inc, 2003). These contaminants could likely originate from industrial effluents, combined with wastewater produced from various industries including iron and steel industries, organic chemical industries, mines, and food industries (Mahmood et al., 2019). During the last few decades, PHE pollution has drastically increased due to rapid industrialization, leading to adverse health risk in the environment.
The concentrations of PHEs in water sources confirmed the substantial influence of anthropogenic activities in industrial estates. For instance, the highest PHE concentrations were found near the studied urbanized areas, demonstrating the anthropogenic sources of the pollution (Kshetriya et al., 2021). Based on the concentration levels, PHEs were low to moderately distributed in all industrial estates, demonstrating low pollution levels in drinking water sources in comparison with studies conducted in Ogale, Nigeria by Abarikwu et al. (2017), and Wuxi city, China (Yan-Feng et al., 2007), as shown in Table 2. Our results were also found to be varied with the previous studies of PHEs concentration in different industrial regions around the world. For instance, PHEs contents were relatively low than the previous studies of Charsadda district of industrial zone in KPK province, Pakistan (Khan et al., 2013), Andhra Pradesh of industrial region, Hyderabad India (Krishna and Mohan, 2014), and in contrast, were higher than the past studies of PHEs in industrial area of Lagos, Nigeria (Ukan et al., 2019), and in similar study of Hattar industrial estate of Haripur Pakistan (Afzal et al., 2018), as shown in Supplementary Table S4.
The mean concentrations of PHEs were observed to follow the descending order of Zn > Cr > Pb > Ni > Cd for all industrial estates. Comparatively, high mean concentrations of all PHEs with high contribution (Figure 2) were generally found in the HIEH, followed by GIES, and HIEP of the study area. The increase in PHEs of the HIEH area could be associated to the discharge of industrial effluents, surface wastewater runoff into the water sources, and continuous waste release to the environment (Abdullah et al., 2016). Furthermore, the PHEs were moderate to high in drinking water of industrial estates with below permissible limits but the long-term exposure to these PHEs may disrupt immune, nervous, and endocrine systems (Li et al., 2014), and also lead to cancer or disability with adverse effects in both adults and children (Wu et al., 2018). Other health problems can occur like stomach and heart diseases, anorexia, and hypertension (Qian et al., 2020). lung disease, fertility, bone fracture, renal failure, and hormonal problem (Yuanan et al., 2020). For instance, long-term exposure of PHEs like Cr could pose health problems, such as deformation of kidney and immune system, damaging of skin and liver, respiratory problem and can also affect the fishes due to accumulation, and Pb exposure can cause potential toxicity in children (Pandey et al., 2010; Khan et al., 2011), and diverse health problems via ingestion (Xiao et al., 2013). To understand and to cope with this kind of threat, it is important to evaluate the groundwater quality by means of PHEs distribution and contamination in polluted industrial areas and their associated health risks of the local population.
Degree of PHE contamination in industrial estates
The CF results for all the calculated parameters for the evaluation of PLI are summarized in Table 3. Fe had the highest CF mean values (6.03, 6.69, 9.33) for HIEP, HIEH, and GIES, respectively, followed by Mg (1.27, 1.33, and 1.52), while the lowest mean CF values were observed for Pb, Ni, and Cd. The degree of contamination was low with respect to PHE concentrations in the drinking water sources of the industrial estates. However, the CF values were slightly high for HIEH, indicating a low contamination level in the drinking water sources.

TABLE 3. Contamination factor (CF), pollution load index (PLI, and water pollution index (WPI) values of selected parameters in industrial estates.
PLI and WPI were calculated for every sample to assess the degree of contamination in drinking water utilizing parameters of water quality (n = 10), as presented in Table 3. The PLI mean values were below (<1) for HIEP and GIES, thus demonstrating the low pollution level of the drinking water sources with respect to the parameters’ concentration levels. HIEH showed a high PLI mean value (1.13) for the parameters compared to HIEP and GIES, indicating the presence of water pollution in HIEH. This could be related to industrial effluent discharge into the surface water bodies and groundwater (Afzal et al., 2018). Similarly, the WPI values were also found to be (<1) for HIEP and HIES, thereby indicating excellent water quality, while HIEH had WPI of 1.12, showing highly polluted water. Based on the results, it can be emphasized that the water sources (tube wells, dug wells and bore wells) are not safe for the purpose of drinking for the local inhabitants of HIEH. However, the high concentrations of Mg, and Fe and their high CF values in all industrial estates could be associated with the use of high iron and steel contents in industrial operational units, resulting in discharge of wastewater without any proper treatment (Muhammad et al., 2021).
Parameter source identification
PCA-MLR generally reduces and extracts a dataset into a small number of loading factors by examining the substantial relationships between the observed variables (Orani et al., 2019). PCA-MLR can be used to classify and indicate the possible pollution sources of PHEs. The total variance percentages of (PC1, PC2, PC3, and PC4) with eigenvalues (>1) are presented in Table 4.
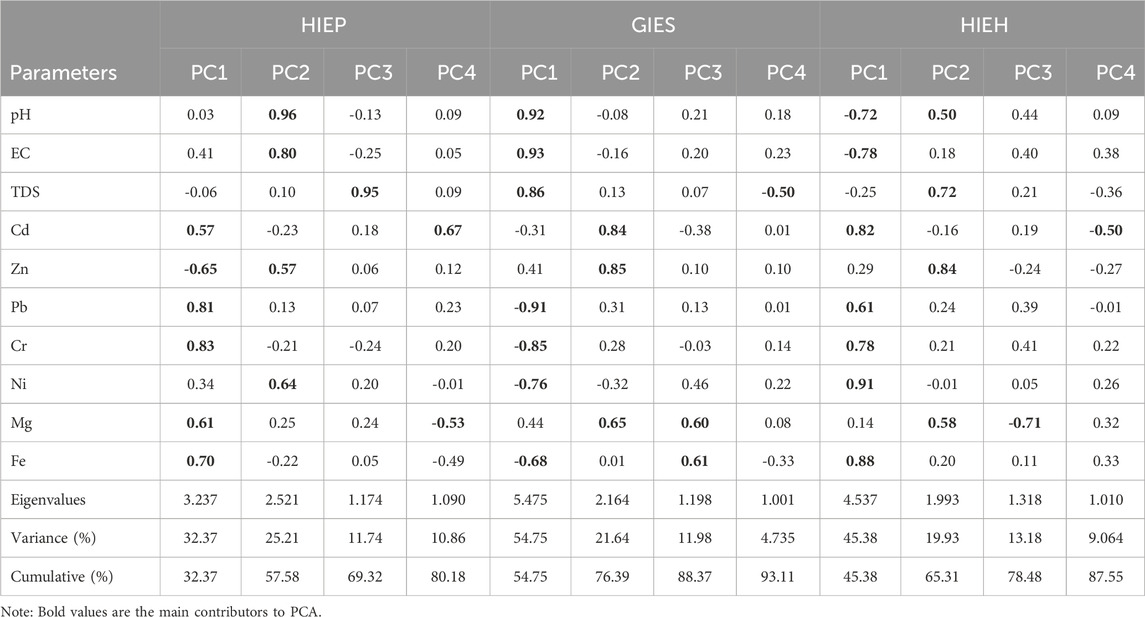
TABLE 4. Principal component analysis results of parameters of industrial estates (HIEP, GIES, AND HIEH) regions.
The first component of PC1 showed 32.37, 54.75, and 45.38% variability with eigenvalues of 3.23, 5.47, and 4.53. The predominant contributors to PC1 showed moderate and strong loadings. PC1 indicates the strength of PHEs in all industrial estates, suggesting that these contaminants could mainly originate from the anthropogenic sources of agriculture runoff, domestic and industrial waste (Abdullah et al., 2016). Strong factor loadings of Zn, Cr, Ni, Cu, and Pb in the water sources may be credited to the discharge of anthropogenic sources of industrial wastewater, mining activities, in addition to agrochemical use in the regions (Ullah et al., 2019). The second component (PC2) described 25.21, 21.64, and 19.93% of variability with eigenvalues of 2.52, 2.16, and 1.99. Thus, PC1 and PC2 showed the contributions of anthropogenic sources to drinking water in the study area. The third component (PC3) explained 11.74, 11.98, and 13.18% of variability with eigenvalues of 1.17, 1.19, and 1.31.
The fourth component (PC4) explained 10.86, 4.735, and 9.064% of variability with eigenvalues of 1.02, 1.00, and 1.02 for HIEP, GIES, and HIEH, respectively. The moderate and high loadings of TDS, Mg, Fe, Cd, and Pb in PC3 and PC4 of HIEP, GIES, and HIEH suggest the contribution of mixed sources of anthropogenic and geo-genic origins, including weathering of igneous and ultramafic rocks and erosion in the study area (Ahmad et al., 2020). Thus anthropogenic sources of industrial wastewater discharge, traffic emission and other domestic waste, and natural geo-genic sources include erosion and weathering of igneous and mafic-ultramafic rocks contributed to the origins of selected parameters in the PCA results of the study area (Figure 3). The results of PCA were found to be in agreement with previous studies of PHEs in water sources conducted by Nawab et al. (2021), and Ahmad et al. (2020). In industrial estates regions, the occurrence and dispersion of PHEs like Cd could be associated to high fossil fuel combustion, smelting of metals, and waste incineration process. Furthermore, the PHEs may also accumulate to the environment by traffic vehicular emissions, tire wears and disposal of municipal solid waste are primarily contributing factors in the study area. For instance, few studies have shown an increase in PHEs contamination due to high traffic emissions and fossil fuel combustion in the study area of industrial estates.
Pearson correlation analysis of parameters
Pearson correlation analysis is a useful method which provides important variable relationship information by classifying the contaminant sources (Guo et al., 2015). This analysis indicates low, moderate and strong correlations among variables in the drinking water sources of the study area. A correlation coefficient value < 0.5 is weak, 0.5 to 0.75 moderate, and >0.75 specifies strong correlation (Nawab et al., 2021). Significant correlations between selected pairs of parameters were obtained for all industrial estates, as shown in Table 5. The correlation of observed parameters were further supported by the results of PCA in the groundwater of all industrial estates. The positive correlation values showed that variables of groundwater samples are greatly dependent on one another. The positive correlated values also suggest that parameters show a strong influence on each other, while inverse correlated values exhibit that groundwater parameters have no effect on each other.
Positive and moderate-strong correlations were found between pH-EC (0.80), EC-Ni (0.54), Cd-Pb (0.52), Zn-Cr (-0.62), Pb-Cr (0.60), pH-Fe (-0.52), and Cr-Fe (0.69) in HIEP. Furthermore, positive, and moderate-strong correlations were found between pH-EC (0.96), pH-TDS (0.71), EC-TDS (0.67), Cd-Cr (0.54), Pb-Cr (0.76), Pb-Ni (0.65), Cr-Ni (0.53), TDS-Mg (0.54), Zn-Mg (0.72), and Pb-Fe (0.50) in GIES. On the contrary, negative correlations were noted between pH-Pb (-0.84), pH-Cd (-0.69), pH-Ni (-0.58), EC-Pb (-0.86), EC-Cr (-0.79), EC-Ni (-0.53), TDS-Pb (-0.75), TDS-Cr (-0.73), TDS-Ni (-0.73), TDS-Mg (-0.56). These negative loading factors show that the water quality of the industrial estates is not significantly affected by the variables. Furthermore, positive and negative correlations were found between pH-EC (0.83), pH-TDS (0.54), pH-Cd (-0.63), pH-Ni (0.63), EC-Cd (-0.72), EC-Ni (-0.62), Cd-Pb (0.63), Cd-Cr (0.53), Cd-Ni (0.59), Zn-Mg (0.67), Cd-Cr (0.90), Cd-Fe (0.50), Cr-Ni (0.80), Cr-Fe (0.79), and Ni-Fe (0.85) in HIEH. High PHEs concentrations and these moderate to strong correlations coefficients (r > 0.50) in the groundwater of industrial estates suggest the contribution of high anthropogenic and natural sources. The PHEs dispersion could be attributed to important contributions from multiple manmade sources like industrial effluents discharge, improper waste disposal and poor sanitation, agricultural practices, and decomposition of organic products in the study area (Howladar, 2017). The significant positive correlation between physiochemical and PHEs suggests the same origin of various sources in the groundwater aquifers that primarily contribute to contaminate drinking water sources of industrial estates. The results of this correlation analysis could be used to identify the interrelationship among PHEs, their contamination and their potential sources for understanding the dynamics of PHE distribution in future research, especially in the groundwater.
Health risk assessment of PHEs
Non-carcinogenic risk assessment
The obtained results for the daily metal intake and non-carcinogenic risk of selected PHEs (Cd, Zn, Pb, Cr and Ni) are shown in Supplementary Table S5 and Table 6. A low mean HQ dermal value of 4.55E-09 was observed for adults in HIEP, while a high mean HQ ingestion value of 1.03E-05 was observed for children in HIEH. The contributions of non-carcinogenic risk to children were high in comparison with adults for all industrial estates. The dermal route has low contribution, based on mean HQ values lower than those for ingestion.
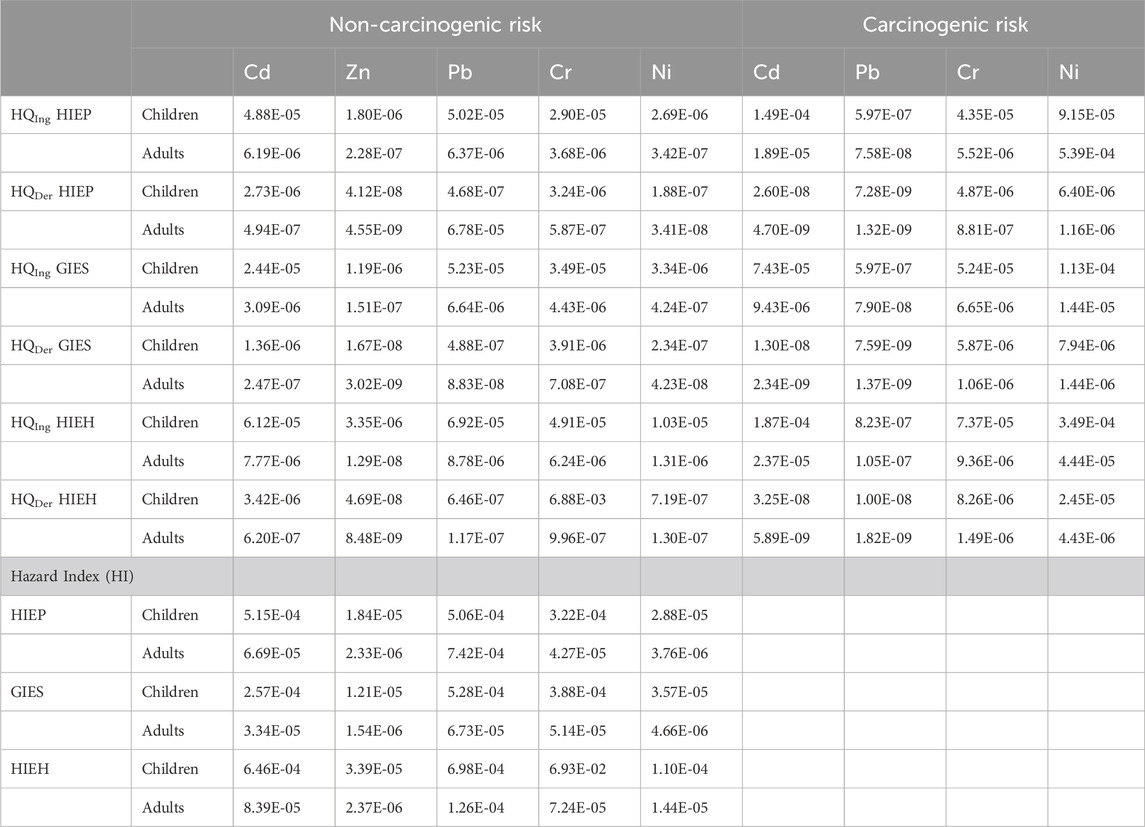
TABLE 6. Non-carcinogenic and carcinogenic risk of selected PHEs via ingestion, inhalation, and dermal for HIEP, GIES, and HIEH.
The low and high mean HI values for adults and children calculated were 4.66E-06 and 6.93E-02 for GIES and HIEH, respectively. The HQs and HIs of these five PHEs for children were found to be higher as compared to adults (Table 6). The ingestion route made the highest contribution (66.67% and 57.48%), followed by the dermal route (33.33% and 42.52%) to HI for children and adults, respectively as shown in Figures 4A, B. The total HI values of Cr, Pb and Cr for children exceeded (>1) the acceptable limit, indicating non-carcinogenic risk to children exposed for all drinking water sources of HIEP, GIES and HIEH. The concentrations of PHEs were low, although the high HQ and HI values indicate that children were found greatly susceptible to unwanted bad effects from PHEs in the drinking water of the industrial estates (especially HIEH). This exposure could cause alterations in the social patterns of affected children, e.g., recurrent, and weird pica manners, sucking of fingers and hands, in addition to high rate of respiration (Zhang W. et al., 2020).
Carcinogenic risk
Table 6 and Figure 5 represent the obtained results for carcinogenic risks (CR) of selected PHEs are presented in. Cd, Cr, Pb and Ni are categorized as cancer causing agents metals for children as well as adults via dermal and ingestion pathways (USEPA, 2020). PHEs carcinogenic effect differs according to their exposure routes. Like non-carcinogenic risk, the ingestion CR values were greatly higher as compared the dermal results (Table 6), indicating ingestion as the primary exposure pathway for PHEs. In addition, a lower CR was observed for each PHE for adults than for children. The low and high mean CR values of adults and children were 7.90E-08 and 1.13E-04 for HQDer-GIES and HQIng-GIES respectively.
Cd, Pb, Cr, and Ni had low mean CR values for HIEP, GIES, and HIEH, and were found to be within the acceptable threshold value of 1 × 10–4, recommended by USEPA (2011). As a result, PHEs were generally found to be within the acceptable threshold and did not cause cancer risk (Mukherjee et al., 2020). Among PHEs, Pb had the lowest contribution to CR mean values for HIEP, GIES, and HIEH (see Figure 5), indicating low carcinogenic risk to the local children and adult inhabitants. The average CR for both the children and adults were found to be within the guideline threshold values, based on the availability of cancer slope factor (CSF) values of the selected toxic PHEs. The results revealed that PHEs in industrial estates have no noteworthy harmful effects on human health. However, children are comparatively more sensitive and vulnerable to exposure of PHEs via different exposure routes than adults. Hence, the long-term exposure of PHEs could be properly monitored to investigate the environmental impacts and health problems via ingestion of drinking groundwater or dermal contact. The industrial operations need to be monitored and reduce contamination of wastewater by proper treatment, before discharge into the drinking water sources and aquatic ecosystem, in order to protect the health of residents in the industrial estate regions (especially in HIEH) of the KPK, Pakistan. There should be a treatment facility installed at each industrial unit such that the industrial wastewater is treated for PHEs before discharge to the outside and protect human health. The potential health risks posed by PHEs contamination due to the groundwater of industrial estates cannot be ignored, and special consideration needs to be taken to mitigate PHEs contamination. Furthermore, the potential sources and adverse health risks of PHEs could provide a significant contribution for further research of groundwater in industrial estates and urban environments. The findings of the present study also proposed that effective and advanced refinement systems should be particularly introduced to provide clean drinking water in the study area, especially in industrial estates of Pakistan. Further research studies on monitoring, implementation, and dynamics of PHEs in industrial estates regions should be necessary to undertake the long-term associated health risks.
Conclusion
In the present study, comparative assessments of the physiochemical parameters and PHE concentrations in the drinking water sources of three industrial estates were examined. It is concluded that all physiochemical and PHE concentrations were found to be within the acceptable limits, although Fe exceeded the WHO, US, and Canadian permissible limits. The contamination degrees of all parameters showed low to moderate level of contamination for all industrial estates, except Fe and Mg. According to PLI and WPI results, freshwater sources had low pollution levels, indicating excellent water quality for HIEP and GIES, while HIEH had high PHEs contamination, showing bad water quality compared to the other industrial estates. Pearson’s correlations and PCA results showed that anthropogenic sources of industrial effluents, wastewater discharge, and geogenic origins influenced the physiochemical parameters and PHE concentrations in drinking water sources. PHEs had low contamination levels in all industrial estates, and thus posed low non-carcinogenic and carcinogenic risks to the local inhabitants in the study area. In conclusion, PHEs were generally found to be within the acceptable threshold values and did not cause cancer risk. Non-etheless, it is strongly recommended that continuous monitoring of the operational activities of industries as well as mitigation of the over-exploitation of environmental resources are necessary to implement especially in the KPK, Pakistan. Most importantly, there should be strict enforcement of laws regarding the discharge of industrial wastewater containing PHEs, and their concentrations in wastewater should be within permissible limits specified in NEQs. The limitations in assessment of PHEs with risk assessment, still remain on a broad scale in industrial estates regions and urban environments. However, this study would help decision-makers to improve long-term strategic plans in development for groundwater resource in all industrial estates of Pakistan. Moreover, it is recommended to remarkably abate the pollution sources of multiple pathways of PHEs with proper treatment and management in industrialized and urban environments, following the standard WHO and PAK-EPA guidelines.
Data availability statement
The original contributions presented in the study are included in the article/Supplementary Material, further inquiries can be directed to the corresponding author.
Author contributions
MI: Formal Analysis, Methodology, Writing–original draft, Writing–review and editing. MK: Formal Analysis, Methodology, Writing–original draft, Writing–review and editing. SK: Conceptualization, Validation, Writing–review and editing. JG: Formal Analysis, Software, Data curation, Writing–review and editing. ZU: Formal Analysis, Software, Data curation, Writing–review and editing. JN: Formal Analysis, Methodology, Writing–original draft, Writing–review and editing. AA: Conceptualization, Funding acquisition, Validation, Writing–review and editing. MA: Conceptualization, Funding acquisition, Validation, Writing–review and editing. SA: Conceptualization, Funding acquisition, Validation, Writing–review and editing.
Funding
The author(s) declare financial support was received for the research, authorship, and/or publication of this article. This project was financially supported and funded by Researchers Supporting Project (No. RSP2024R191), King Saud University, Riyadh, Saudi Arabia.
Conflict of interest
The authors declare that the research was conducted in the absence of any commercial or financial relationships that could be construed as a potential conflict of interest.
Publisher’s note
All claims expressed in this article are solely those of the authors and do not necessarily represent those of their affiliated organizations, or those of the publisher, the editors and the reviewers. Any product that may be evaluated in this article, or claim that may be made by its manufacturer, is not guaranteed or endorsed by the publisher.
Supplementary material
The Supplementary Material for this article can be found online at: https://www.frontiersin.org/articles/10.3389/fenvs.2024.1332965/full#supplementary-material
References
Abarikwu, S. O., Essien, E. B., Iyede, O. O., John, K., and Mgbudom-Okah, C. (2017). Biomarkers of oxidative stress and health risk assessment of heavy metal contaminated aquatic and terrestrial organisms by oil extraction industry in Ogale, Nigeria. Chemosphere 185, 412–422. doi:10.1016/j.chemosphere.2017.07.024
Abdullah, M. Z., Manap, N. R. A., Saat, A., and Hamzah, Z. (2016). Assessment of surface water metal pollution based on pollution load index (pli) supported by multivariate statistical analysis. https://www.researchgate.net/publication/299597229.
Abdullah, M. Z., Saat, A., and Hamzah, Z. (2011). Optimization of energy dispersive X-ray fluorescence spectrometerto analyze heavy metals in moss samples. Sci. Publ. 4 (3), 355–362. doi:10.3844/ajeassp.2011.355.362
Afzal, M. S., Ashraf, A., and Nabeel, M. (2018). Characterization of industrial effluents and groundwater of Hattar industrial estate. Haripur. Adv. Agric. Environ. Sci. Open Access (AAEOA) 1 (2), 70–77. doi:10.30881/aaeoa.00013
Ahmad, K., Muhammad, S., Ali, W., Jadoon, I. A., and Rasool, A. (2020). Occurrence, source identification and potential risk evaluation of heavy metals in sediments of the Hunza River and its tributaries, Gilgit-Baltistan. Environ. Technol. Innov. 18, 100700. doi:10.1016/j.eti.2020.100700
Amin, N., Ayaz, M., Alam, S., and Gul, S. (2014). Heavy metals contamination through industrial effluent to irrigation water in GadoonAmazai (Swabi) and Hayatabad (Peshawar) Pakistan. J. Sci. Res. 6 (1), 111–124. doi:10.3329/jsr.v6i1.16336
Ayejoto, D. A., and Egbueri, J. C. (2023). Human health risk assessment of nitrate and heavy metals in urban groundwater in Southeast Nigeria. Acta Ecol. Sin. in press. doi:10.1016/j.chnaes.2023.06.008
Baken, K. A., Sjerps, R. M. A., Schriks, M., and van Wezel, A. P. (2018). Toxicological risk assessment and prioritization of drinking water relevant contaminants of emerging concern. Environ. Int. 118, 293–303. doi:10.1016/j.envint.2018.05.006
Boum-Nkot, S. N., Nlend, B., Komba, D., Ndondo, G. N., Bello, M., Fongoh, E., et al. (2023). Hydrochemistry and assessment of heavy metals groundwater contamination in an industrialized city of sub-Saharan Africa (Douala, Cameroon). Implication on human health. HydroResearch 6, 52–64. doi:10.1016/j.hydres.2023.01.003
Canadian or BC Health Act Safe Drinking Water Regulation BC Reg 230/92, & Sch 120, 2001. Task force of the Canadian council or resource and environment ministers guidelines for Canadian drinking water quality, 1996.
Chandra, R., Yadav, S., and Yadav, S. (2017). Phytoextraction potential of heavy metals by native wetland plants growing on chlorolign in containing sludge of pulp and paper industry. Ecol. Eng. 98, 134–145. doi:10.1016/j.ecoleng.2016.10.017
EPA (2014). Toxic and priority pollutants under the clean water act. https://www.epa.gov/eg/toxic-and-priority-pollutants-under-clean-water-act#priority.
Ghani, J., Nawab, J., Faiq, M. E., Ullah, S., Alam, A., Ahmad, I., et al. (2022b). Multi-geostatistical analyses of the spatial distribution and source apportionment of potentially toxic elements in urban children's park soils in Pakistan: a risk assessment study. Environ. Pollut. 311, 119961. doi:10.1016/j.envpol.2022.119961
Ghani, J., Nawab, J., Khan, S., Khan, M. A., Ahmad, I., Ali, H. M., et al. (2022c). Organic amendments minimize the migration of potentially toxic elements in soil–plant system in degraded agricultural lands. Biomass Convers. Biorefin. doi:10.1007/s13399-022-02816-3
Ghani, J., Ullah, Z., Nawab, J., Iqbal, J., Waqas, M., Ali, A., et al. (2022a). Hydrogeochemical characterization, and suitability assessment of drinking groundwater: application of geostatistical approach and geographic information system. Front. Environ. Sc. 10, 874464. doi:10.3389/fenvs.2022.874464
Gu, Y. G., Gao, Y. P., and Lin, Q. (2016). Contamination, bioaccessibility and human health risk of heavy metals in exposed-lawn soils from 28 urban parks in southern China's largest city, Guangzhou. Guangzhou. Appl. Geochem. 67, 52–58. doi:10.1016/j.apgeochem.2016.02.004
Guo, W., Huo, S., Xi, B., Zhang, J., and Wu, F. (2015). Heavy metal contamination in sediments from typical lakes in the five geographic regions of China: distribution, bioavailability and risk. Ecol. Eng. 81, 243–255. doi:10.1016/j.ecoleng.2015.04.047
Hafeez, A., Shamair, Z., Shezad, N., Javed, F., Fazal, T., urRehman, S., et al. (2020). Solar powered decentralized water systems: a cleaner solution of the industrial wastewater treatment and clean drinking water supply challenges. J. Clean. Product. 289, 125717. doi:10.1016/j.jclepro.2020.125717
Hakanson, L. (1980). An ecological risk index for aquatic pollution control. A sedimentological approach. Water Res. 14 (8), 975–1001. doi:10.1016/0043-1354(80)90143-8
Hem, J. D. (1985). Study and interpretation of the chemical characteristics of natural water. Department of the Interior, US Geological Survey, USGS Publishing Network.
Hoang, H. G., Chiang, C. F., Lin, C., Wu, C. Y., Lee, C. W., Cheruiyot, N. K., et al. (2021). Human health risk simulation and assessment of heavy metal contamination in a river affected by industrial activities. Environ. Pollut. 285, 117414. doi:10.1016/j.envpol.2021.117414
Hossain, M., and Patra, P. K. (2020). Water pollution index–A new integrated approach to rank water quality. Eco. Indic. 117, 106668. doi:10.1016/j.ecolind.2020.106668
Howladar, M. (2017). An assessment of surface water chemistry with its possible sources of pollution around the Barapukuria Thermal Power Plant Impacted Area, Dinajpur, Bangladesh. Groundw. Sust. Dev. 5, 38–48. doi:10.1016/j.gsd.2017.03.004
Huang, J., Wu, Y., Sun, J., Li, X., Geng, X., Zhao, M., et al. (2021). Health risk assessment of heavy metal (loid) s in park soils of the largest megacity in China by using Monte Carlo simulation coupled with Positive matrix factorization model. J. Hazard Mater. 415, 125629. doi:10.1016/j.jhazmat.2021.125629
Hussain, K., Shahazad, A., and Zia-ul-Hussnain, S. (2008). An ethnobotanical survey of important wild medicinal plants of Hattar district Haripur, Pakistan. Ethnobot. Leafl. 12, 29–35.
Inam Ullah, E., and Alam, A. (2014). Assessment of drinking water quality in Peshawar, Pakistan. Bulg. J. Agri. Sci. 20 (3), 595–600.
Islam, M. S., Ahmed, M. K., Habibullah-Al-Mamun, M., and Masunaga, S. (2015). Assessment of trace metals in fish species of urban rivers in Bangladesh and health implications. Environ. Toxic. Pharm. 39, 347–357. doi:10.1016/j.etap.2014.12.009
Jain, N., Johnson, T. A., Kumar, A., Mishra, S. V., and Gupta, N. (2015). Biosorption of Cd(II) on jatropha fruit coat and seed coat. Environ. Monit. As. 187, 411. doi:10.1007/s10661-015-4658-4
Jan, F. A., Ishaq, M., Khan, S., Ihsanullah, I., Ahmad, I., and Shakirullah, M. (2010). A comparative study of human health risks via consumption of food crops grown on wastewater irrigated soil (Peshawar) and relatively clean water irrigated soil (lower Dir). J. Hazard. Mater. 179 (1-3), 612–621. doi:10.1016/j.jhazmat.2010.03.047
Jia, X., Fu, T., Hu, B., Shi, Z., and Zhu, Y. (2020). Identification of the potential risk areas for soil heavy metal pollution based on the source-sink theory. J. Hazard. Mater. 393, 122424. doi:10.1016/j.jhazmat.2020.122424
Jordanova, M., Hristovski-Musa, S., Violeta, M., Katerina, B., Suzana, R., and Melovski, D. K. L. (2018). Accumulation of heavy metals in some organs in Barbeland chub from CrnDrim River in the Republic of Macedonia. Bull. Environ. Contam. Toxicol. 3, 392–397. doi:10.1007/s00128-018-2409-2
Khan, A., and Ali, M. (2019). Impact of built environment on groundwater depletion in Peshawar, Pakistan. J. Himal. Earth Sci. 52, 1–86.
Khan, M. A., Khan, M. A., Rahman, Z., Khan, A., and Khan, S. (2016). Impacts of industrial effluents on ground water quality of Hattar Industrial Estate and its associated health risks. J. Sci. Technol. 40, 9–22.
Khan, S., Shahnaz, M., Jehan, N., Rehman, S., Shah, M. T., and Din, I. (2013). Drinking water quality and human health risk in Charsadda district, Pakistan. J. Clean. Prod. 60, 93–101. doi:10.1016/j.jclepro.2012.02.016
Khan, S., Uddin, Z., and Ihsanullah, Z. A. (2011). Levels of selected heavy metals in drinking water of Peshawar City. Int. J. Sci. Nat. 2 (3), 648–652.
Kim, Y., Joo, H., Her, N., Yoon, Y., Sohn, J., Kim, S., et al. (2015). Simultaneously photocatalytic treatment of hexavalent chromium (Cr (VI)) and endocrine disrupting compounds (EDCs) using rotating reactor under solar irradiation. J. Hazard. Mater. 288, 124–133. doi:10.1016/j.jhazmat.2015.02.021
Krishna, A. K., and Mohan, K. R. (2014). Risk assessment of heavy metals and their source distribution in waters of a contaminated industrial site. Environ. Sci. Pollut. Res. 21 (5), 3653–3669. doi:10.1007/s11356-013-2359-5
Krishna, A. K., Satyanarayanan, M., and Govil, P. K. (2009). Assessment of heavy metal pollution in water using multivariate statistical techniques in an industrial area: a case study from Patancheru, Medak District, Andhra Pradesh, India. J. Hazard. Mater. 167 (1-3), 366–373. doi:10.1016/j.jhazmat.2008.12.131
Kshetriya, D., Warjri, C. D., Chakrabarty, T. K., and Ghosh, S. (2021). Assessment of heavy metals in some natural water bodies in Meghalaya, India. Environ. Nanotech. Monit. Manag. 16, 100512. doi:10.1016/j.enmm.2021.100512
Kumar, S. B., Padhi, R. K., Mohanty, A. K., and Satpathy, K. K. (2020). Distribution andecological- and health-risk assessment of heavy metals in the seawater of thesoutheast coast of India. Mar. Pollut. Bull. 161, 111712. doi:10.1016/j.marpolbul.2020.111712
Kumar, V., Parihar, R. D., Sharma, A., Bakshi, P., Sidhu, G. P. S., Bali, A. S., et al. (2019). Global evaluation of heavy metal content in surface water bodies: a meta-analysisusing heavy metal pollution indices and multivariate statistical analyses. Chemosphere 236, 124364–124377. doi:10.1016/j.chemosphere.2019.124364
Li, Z., Ma, Z., van der Kuijp, T. J., Yuan, Z., and Huang, L. (2014). A review of soil heavy metal pollution from mines in China: pollution and health risk assessment. Sci. Total Environ. 468, 843–853. doi:10.1016/j.scitotenv.2013.08.090
Liu, D. H. F., and Liptak, B. G. (1999). Environmental engineers’ handbook on CD-ROM. Second edied. Lewis Publishers.
Liu, M., Xu, Y., Nawab, J., Rahman, Z., Khan, S., Idress, M., et al. (2020). Contamination features, geo-accumulation, enrichments and human health risks of toxic heavy metal (loids) from fish consumption collected along Swat river, Pakistan. Environ. Technol. Innov. 17, 100554. doi:10.1016/j.eti.2019.100554
Lv, J., Hu, R., Wang, N., Zhu, L., Zhang, X., Yuan, X., et al. (2021). Distribution and movement of heavy metals in sediments around the coastal areas under the influence of multiple factors: a case study from the junction of the Bohai Sea and the Yellow Sea. Chemosphere 278, 130352. doi:10.1016/j.chemosphere.2021.130352
Machado, K. S., Al Ferreira, P. A., Rizzi, J., Figueira, R., and Froehner, S. (2017). Spatial and temporal variation of heavy metals contamination in recent sediments from Barigui river basin, South Brazil. Environ. Pollut. Clim. Change 108, 1. doi:10.4172/2573-458x.1000108
Mahmood, Q., Shaheen, S., Bilal, M., Tariq, M., Zeb, B. S., Ullah, Z., et al. (2019). Chemical pollutants from an industrial estate in Pakistan: a threat to environmental sustainability. Appl. Water Sci. 9 (3), 47–49. doi:10.1007/s13201-019-0920-1
Mahmoud, M. E., Abdou, A. E. H., and Ahmed, S. B. (2016). Conversion of waste styrofoam into engineered adsorbents for efficient removal of cadmium, lead and mercury from water. ACS Sust. Chem. Eng. 4, 819–827. doi:10.1021/acssuschemeng.5b01149
Men, C., Liu, R., Xu, L., Wang, Q., Guo, L., Miao, Y., et al. (2020). Source-specific ecological risk analysis and critical source identification of heavy metals in road dust in Beijing, China. J. Hazard. Mater. 388, 121763. doi:10.1016/j.jhazmat.2019.121763
Metcalf, Eddy, Inc. (2003). Wastewater engineering treatment and reuse. 4th edn. New York: McGraw-Hill.
Mico, C., Recatala, L., Peris, M., and Sanchez, J. (2006). Assessing heavy metal sources in agricultural soils of an European Mediterranean area by multivariate analysis. Chemosphere 65, 863–872. doi:10.1016/j.chemosphere.2006.03.016
Mousavian, N. A., Mansouri, N., and Nezhadkurki, F. (2017). Estimation of heavy metal exposure in workplace and health risk exposure assessment in steel industries in Iran. Measurement 102, 286–290. doi:10.1016/j.measurement.2017.02.015
Muhammad, N., Nafees, M., Ge, L., Khan, M. H., Bilal, M., Chan, W. P., et al. (2021). Assessment of industrial wastewater for potentially toxic elements, human health (dermal) risks, and pollution sources: a case study of Gadoon Amazai industrial estate, Swabi, Pakistan. J. Hazard. Mater. 419, 126450. doi:10.1016/j.jhazmat.2021.126450
Muhammad, S., Shah, M. T., and Khan, S. (2011). Heavy metal concentrations in soil and wild plants growing around Pb-Zn sulfide terrain in the kohistan region, northern Pakistan. Microchem. J. 99 (1), 67–75. doi:10.1016/j.microc.2011.03.012
Mukherjee, I., Singh, U. K., Singh, R. P., Anshumali Kumari, D., Jha, P. K., Mehta, P., et al. (2020). Characterization of heavy metal pollution in an anthropogenically and geologically influenced semi-arid region of east India and assessment of ecological and human health risks. Sci. Total Environ. 705, 135801. doi:10.1016/j.scitotenv.2019.135801
Nawab, J., Din, Z. U., Ahmad, R., Khan, S., Zafar, M. I., Faisal, S., et al. (2021). Occurrence, distribution, and pollution indices of potentially toxic elements within the bed sediments of the riverine system in Pakistan. Environ. Sci. Pollut. Res. 28, 54986–55002. doi:10.1007/s11356-021-14783-9
Nawab, J., Ghani, J., Khan, S., and Xiaoping, W. (2018b). Minimizing the risk to human health due to the ingestion of arsenic and toxic metals in vegetables by the application of biochar, farmyard manure and peat moss. J. Environ. Manag. 214, 172–183. doi:10.1016/j.jenvman.2018.02.093
Nawab, J., Khan, N., Ahmed, R., Khan, S., Ghani, J., Rahman, Z., et al. (2019). Influence of different organic geo-sorbents on Spinaciaoleracea grown in chromite mine-degraded soil: a greenhouse study. J. Soils Sediment. 19 (5), 2417–2432. doi:10.1007/s11368-019-02260-3
Nawab, J., Khan, S., Ali, S., Sher, H., Rahman, Z., Khan, K., et al. (2016). Health risk assessment of heavy metals and bacterial contamination in drinking water sources: a case study of Malakand Agency, Pakistan. Environ. Monit. As. 188 (5), 286. doi:10.1007/s10661-016-5296-1
Nawab, J., Khan, S., Khan, M. A., Sher, H., Rehamn, U. U., Ali, S., et al. (2017). Potentially toxic metals and biological contamination in drinking water sources in chromite mining-impacted areas of Pakistan: a comparative study. Expos. Health 9, 275–287. doi:10.1007/s12403-016-0240-8
Nawab, J., Khan, S., and Xiaoping, W. (2018a). Ecological and health risk assessment of potentially toxic elements in the major rivers of Pakistan: general population vs. fishermen. Chemosphere 202, 154–164. doi:10.1016/j.chemosphere.2018.03.082
Orani, A. M., Vassileva, E., Renac, C., Schmidt, S., Angelidis, M. O., Rozmaric, M., et al. (2019). First assessment on trace elements in sediment cores from Namibian coast and pollution sources evaluation. Sci. Total Environ. 669, 668–682. doi:10.1016/j.scitotenv.2019.03.059
Pak-EPA (2008). Pakistan environmental protection agency. Pakistan: National Environmental Quality Standards, Ministry of Environment. http://www.pakepa.org/neqs.html (Accessed May 10, 10).
Palin, D., Rufato, K. B., Linde, G. A., Colauto, N. B., Caetano, J., Alberton, O., et al. (2016). Evaluation of Pb (II) biosorption utilizing sugarcane bagasse colonized by Basidiomycetes. Environ. Monit. As. 188, 279. doi:10.1007/s10661-016-5257-8
Pandey, J., Shubhashish, K., and Pandey, R. (2010). Heavy metal contamination of Ganga river at Varanasi in relation to atmospheric deposition. Trop. Ecol. 51 (2), 365–373.
Perveen, I., Raza, M. A., Sehar, S., Naz, I., Young, B., and Ahmed, S. (2017). Heavy metal contamination in water, soil, and milk of the industrial area adjacent to Swan River, Islamabad, Pakistan. Hum. Ecol. Risk Assess. Int. J. 23 (7), 1564–1572. doi:10.1080/10807039.2017.1321956
Qian, Y., Cheng, C., Feng, H., Hong, Z., Zhu, Q., Kolenčík, M., et al. (2020). Assessment of metal mobility in sediment, commercial fish accumulation and impact on human health risk in a large shallow plateau lake in southwest of China. Ecotoxicol. Environ. Saf. 194, 110346. doi:10.1016/j.ecoenv.2020.110346
Qu, S., Wu, W., Nel, W., and Ji, J. (2020). The behavior of metals/metalloids during natural weathering: a systematic study of the mono-lithological watersheds in the upper Pearl River Basin, China. Sci. Total Environ. 708, 134572. doi:10.1016/j.scitotenv.2019.134572
Ranjan, R. K., Sinha, A. K., Gupta, D., Sappal, S. M., Kumar, A., and Ramanathan, A. L. (2016). Sedimentary geochemistry of kabartal wetland, begusarai, Bihar, India. J. Appl. Geochem. 18 (4), 414–429.
Rashid, A., Ayub, M., Javed, A., Khan, S., Gao, X., Li, C., et al. (2021). Potentially harmful metals, and health risk evaluation in groundwater of Mardan, Pakistan: application of geostatistical approach and geographic information system. Geosci. Front. 12 (3), 101128. doi:10.1016/j.gsf.2020.12.009
Ravikumar, P., Somashekar, R. K., and Angami, M. (2011). Hydrochemistry and evaluation of groundwater suitability for irrigation and drinking purposes in the Markandeya River basin, Belgaum District, Karnataka State, India. Environ. Monit. As. 173 (1), 459–487. doi:10.1007/s10661-010-1399-2
Roy, D., Neogi, S., and De, S. (2021). Adsorptive removal of heavy metals from battery industry effluent using MOF incorporated polymeric beads: a combined experimental and modeling approach. J. Hazard. Mater. 403, 123624. doi:10.1016/j.jhazmat.2020.123624
Sajjad, M., Khan, S., Baig, A. S., Munir, S., Naz, A., Khan, A., et al. (2017). Removal of potentially toxic elements from aqueous solutions and industrial wastewater using activated carbon. J. Water Sci. Technol. 75 (11), 2571–2579. doi:10.2166/wst.2017.130
Saleem, M., Iqbal, J., and Shah, M. H. (2019). Seasonal variations, risk assessment and multivariate analysis of trace metals in the freshwater reservoirs of Pakistan. Chemosphere 216, 715–724. doi:10.1016/j.chemosphere2018.10.173
Sanad, S. A., Moniem, S. M. A., Abdel-Latif, M. L., Hossein, H. A., and El-Mahllawy, M. S. (2021). Sustainable management of basalt in clay brick industry after its application in heavy metals removal. J. Mater. Res. Technol. 10, 1493–1502. doi:10.1016/j.jmrt.2020.12.070
Sharma, P., Tripathi, S., and Chandra, R. (2020). Phytoremediation potential of heavy metal accumulator plants for waste management in the pulp and paper industry. Heliyon 6 (7), e04559. doi:10.1016/j.heliyon.2020.e04559
Singh, A. K., and Chandra, R. (2019). Pollutants released from the pulp paper industry: aquatictoxicity and their health hazards. Aquat. Toxicol. 88, 214–219. doi:10.1016/j.aquatox.2019.04.007
Souza, I. P. A. F., Cazetta, A. L., Pezoti, O., and Almeida, V. C. (2017). Preparation of biosorbentsfrom the Jatoba (Hymenaeacourbaril) fruit shell for removal of Pb (II) and Cd (II) from aqueous solution. Environ. Monit. As. 189, 632. doi:10.1007/s10661-017-6330-7
Talib, M. A., Tang, Z., Shahab, A., Siddique, J., Faheem, M., and Fatima, M. (2019). Hydrogeochemical characterization and suitability assessment of groundwater: a case study in Central Sindh, Pakistan. Int. J. Environ. Res. Pub. He 16 (5), 886. doi:10.3390/ijerph16050886
Tayebi, L., and Sobhanardakani, S. (2020). Analysis of heavy metal contents and non-carcinogenic health risk assessment through consumption of Tilapia fish (Oreochromisniloticus). Pollution 6, 59–67. doi:10.22059/POLL.2019.284500.639
Ukah, B. U., Egbueri, J. C., Unigwe, C. O., and Ubido, O. E. (2019). Extent of heavy metals pollution and health risk assessment of groundwater in a densely populated industrial area, Lagos, Nigeria. Int. J. Energy Water Resour. 3, 291–303. doi:10.1007/s42108-019-00039-3
Ullah, R., Muhammad, S., and Jadoon, I. A. (2019). Potentially harmful elements contamination inwater and sediment: evaluation for risk assessment and provenance in the northern Sulaiman fold belt, Baluchistan, Pakistan. Microchem. J. 147, 1155–1162. doi:10.1016/j.microc.2019.04.053
Urban Policy Unit (UPU) (2014). Master plan Vol.1 water supply, treatment and distribution. Planning and Development Department Government of Khyber Pakhtunkhwa. http://urbanpolicyunit.gkp.pk/wp-content/uploads/2018/02/Water-Sanitation-Peshawar-Report.pdf.
USDOE (2011). The risk assessment information system (RAIS). Washington, DC: U.S Department of Energy’s Oak Ridge Operations Office (ORO).
USEPA (1997). Exposure factors handbook, Vol. 1. Washington: General Factors. U.S, Environmental Protection Agency, Office of Research and Development.
USEPA (2007). Guidance for evaluating the oral bioavailability of metals in soils for use in human health risk assessment. OSWER 928.7e80. Available on-line at http://www.epa.gov/superfund/health/contaminants/bioavailability/guidance.htm.
USEPA (2010). Region 9, regional screening levels. Available on-line at http://www.epa.gov/region9/superfund/prg/index.html.
USEPA (2011). Screening levels (RSL) for chemical contaminants at superfund sites. Washington, DC: U.S. Environmental Protection Agency.
USEPA (2013). Region IX, regional screening levels (formerly PRGs). Available on-line at http://www.epa.gov/region9/superfund/prg/index.html.
USEPA (2020). Available at: https://www.epa.gov/iris/ (Accessed August 2, 2020).
Velea, T., Gherghe, L., Predica, V., and Krebs, R. (2009). Heavy metal contamination in the vicinity of an industrial area near Bucharest. Environ. Sci. Pollut. Res. 16, 27–32. doi:10.1007/s11356-008-0073-5
Wang, D., Wu, J., Wang, Y., and Ji, Y. (2020). Finding high-quality groundwater resources to reduce the hydatidosis incidence in the Shiqu County of Sichuan Province, China: analysis, assessment, and management. Expos. Health 12 (2), 307–322. doi:10.1007/s12403-019-00314-y
WHO, 2011. Guidelines for drinking-water quality, fourth ed. In: Recommendations, vol. 1, World Health Organization, Geneva, Switzerland, Fourth ed. .
WHO, 2017. Guidelines for drinking-water quality: first Addendum to the fourth edition. Geneva, Switzerland: World Health Organization.
Wu, J., Li, P., Qian, H., Duan, Z., and Zhang, X. (2014). Using correlation and multivariate statistical analysis to identify hydrogeochemical processes affecting the major ion chemistry of waters: a case study in Laoheba phosphorite mine in Sichuan, China. China Arab. J. Geosci. 7 (10), 3973–3982. doi:10.1007/s12517-013-1057-4
Wu, W., Wu, P., Yang, F., Sun, D. L., Zhang, D. X., and Zhou, Y. K. (2018). Assessment of heavy metal pollution and human health risks in urban soils around an electronics manufacturing facility. Sci. Total Environ. 630, 53–61. doi:10.1016/j.scitotenv.2018.02.183
Xia, P., Ma, L., Sun, R., Yang, Y., Tang, X., Yan, D., et al. (2020). Evaluation of potential ecological risk, possible sources and controlling factors of heavy metals in surface sediment of Caohai Wetland, China. Sci. Total Environ. 740, 140231. doi:10.1016/j.scitotenv.2020.140231
Xiao, G., Wang, Z., Qiu, Z., Li, Y., Qi, J., Liu, W., et al. (2013). Occurrence and infection risk of waterborne pathogens in Wanzhou watershed of the three Gorges reservoir. China J. Environ. Sci. 25 (9), 1913–1921. doi:10.1016/S1001-0742(12)60241-1
Xiao, H., Shahab, A., Xi, B., Chang, Q., You, S., Li, J., et al. (2021). Heavy metal pollution, ecological risk, spatial distribution, and source identification in sediments of the Lijiang River, China. Environ. Pollut. 269, 116189. https://doi.org/10.1016/j.envpol.2020.116189.
Xiao, X., Zhang, J., Wang, H., Han, X., Ma, J., Ma, Y., et al. (2020). Distribution and health risk assessment of potentially toxic elements in soils around coal industrial areas: a global meta-analysis. Sci. Total Environ. 713, 135292. doi:10.1016/j.scitotenv.2019.135292
Xiaoshuai, H., Huoyan, W., Jianmin, Z., Chengling, M., Changwen, D., and Xiaoqin, C. (2009). Risk assessment of potentially toxic element pollution in soils and rice (Oryza sativa) in a typical area of the Yangtze River Delta. Environ. Pollut. 157, 2542–2549. doi:10.1016/j.envpol.2009.03.002
Yan-Feng, Z. H. A. O., Xue-Zheng, S. H. I., Huang, B., Dong-Sheng, Y. U., Hong-Jie, W. A. N. G., Wei-Xia, S. U. N., et al. (2007). Spatial distribution of heavy metals in agricultural soils of an industry-based peri-urban area in Wuxi, China. Pedosphere 17 (1), 44–51. doi:10.1016/s1002-0160(07)60006-x
Yin, R., Guo, Z., Hu, L., Liu, W., Hurley, J. P., Lepak, R. F., et al. (2018). Mercury inputs to Chinese marginal seas: impact of industrialization and development of China. J. Geophys. Res. Oceans 123, 5599–5611. doi:10.1029/2017jc013691
Yuanan, H., He, K., Sun, Z., Chen, G., and Cheng, H. (2020). Quantitative source apportionment of heavy metal (loid) s in the agricultural soils of an industrializing region and associated model uncertainty. J. Hazard. Mater. 391, 122244. doi:10.1016/j.jhazmat.2020.122244
Zhang, H., Zhang, F., Song, J., Tan, M. L., and Johnson, V. C. (2021). Pollutant source, ecological and human health risks assessment of heavy metals in soils from coal mining areas in Xinjiang, China. Environ. Res. 202, 111702. doi:10.1016/j.envres.2021.111702
Zhang, R., Tao, C., Zhang, Y., Hou, Y., and Chang, Q. (2020). Health risk assessment of heavy metals in agricultural soils and identification of main influencing factors in a typical industrial park in northwest China. Chemosphere 252, 126591. doi:10.1016/j.chemosphere2020.126591
Zhang, W., Liu, M., and C, L. (2020). Soil heavy metal contamination assessment in the Hun-Taizi River watershed, China. Sci. Rep. 10, 8730. doi:10.1038/s41598-020-65809-0
Keywords: industrial wastewater, potentially harmful elements, drinking water, health risk, mitigation measures
Citation: Ishtiaq M, Khan MJ, Khan SA, Ghani J, Ullah Z, Nawab J, Alrefaei AF, Almutairi MH and Alharbi SN (2024) Potentially harmful elements and health risk assessment in groundwater of urban industrial areas. Front. Environ. Sci. 12:1332965. doi: 10.3389/fenvs.2024.1332965
Received: 03 November 2023; Accepted: 24 January 2024;
Published: 20 February 2024.
Edited by:
Arbind Kumar Patel, Jawaharlal Nehru University, IndiaReviewed by:
Bulgariu Laura, Gheorghe Asachi Technical University of Iași, RomaniaWenbing Tan, Chinese Research Academy of Environmental Sciences, China
Copyright © 2024 Ishtiaq, Khan, Khan, Ghani, Ullah, Nawab, Alrefaei, Almutairi and Alharbi. This is an open-access article distributed under the terms of the Creative Commons Attribution License (CC BY). The use, distribution or reproduction in other forums is permitted, provided the original author(s) and the copyright owner(s) are credited and that the original publication in this journal is cited, in accordance with accepted academic practice. No use, distribution or reproduction is permitted which does not comply with these terms.
*Correspondence: Javed Nawab, amF2ZWRuYXdhYjExQHlhaG9vLmNvbQ==