- IHCantabria—Instituto de Hidráulica Ambiental de la Universidad de Cantabria, Santander, Spain
Mountain streams harbor unique biodiversity and provide essential ecosystem services to human societies. Yet, these ecosystems face numerous threats, such as the construction of dams and land use changes, leading to rapid habitat degradation, water pollution, and biodiversity loss. In this study, we assess the effect of irrigation dams on mountain riverine biota using traditional biotic indices and trait-based approaches. We selected diatom and macroinvertebrate communities surveyed between 2015 and 2017 in mountain streams located in different regions in northern Spain (Cantabrian Cordillera, Iberian System, and Pyrenees) under natural and altered flow conditions (i.e., downstream of irrigation dams). Hydrological and biological changes related to the presence of dams, the mountain range, and the interaction between these two factors were identified. Summer flows, frequency of high flow events, and minimum annual flows timing were significantly affected by irrigation dams, independently of the region. Winter flows, the magnitude of high flow extremes, and the number of flow rises and falls varied significantly with the dam-mountain range interaction. The frequency and duration of flow pulses depended on the mountain range only. In the Cantabrian Cordillera, a region with larger reservoirs (>150 hm3), impacted sites showed a marked inversion of the seasonal flow patterns (i.e., increased summer flows but reduced winter flows). In the other mountain ranges, reservoirs had smaller storage volumes and multiple purposes, causing significant flow change frequency variations. Diatom traits, taxonomic richness, diversity, and IPS score varied with dam presence and mountain ranges, while macroinvertebrate traits and biotic indices responded weakly. These findings suggest that diatom communities might be more sensitive to hydrological alteration, while macroinvertebrates might be more influenced by space-related factors, such as biogeography and dispersal, overriding dam-related impacts. Furthermore, dam-related changes in ecosystems may depend not only on the presence of dams and their characteristics (e.g., reservoir size and operation), but also on local conditions and biogeography. Our findings emphasize that, when using pre-existing biomonitoring datasets, although some dam-related patterns emerge (e.g., with diatoms), other patterns may be constrained by the datasets’ low spatio-temporal coverage and taxonomic resolution, highlighting the need of well-structured study designs.
1 Introduction
Mountain streams provide a unique set of habitat conditions in the river network (i.e., fast water velocities and low temperatures) and harbor an important portion of the global biodiversity (Rahbek et al., 2019). Their high runoff from rainfall and the delayed releases of water stored in the form of snow or lake reserves characterize mountains as “water towers,” providing lowlands with relatively constant essential water resources and ecosystem services (Viviroli and Weingartner, 2008; Immerzeel et al., 2020). Population dependence on mountain water runoff is expected to grow steadily, reaching 1.5 billion people critically relying on these water resources by 2050 (Viviroli et al., 2020). At the same time, due to the narrow niches of endemic species, mountain environments are extremely vulnerable to global change. As elevation can amplify global warming effects (Grabherr et al., 2001; Christmann and Oliveras, 2020), even small environmental changes can cause significant ecological impacts, such as the reductions of glaciers and the ice-melt inputs into mountain rivers (Fell et al., 2017). Thus, mountain rivers might experience more rapid climate change effects than their lowland counterparts (Pepin et al., 2015).
An extensive number of mountain rivers have been affected by dams and other water infrastructures, e.g., in the Pyrenees (López-Moreno et al., 2008), in the Alps (Spitale et al., 2015), in the Colorado Front Range (Wohl, 2006), in the Andean Amazon region (Anderson et al., 2018), and in the High Mountain Asia (Li et al., 2022). Apart from the potential gravitational energy, which is extensively exploited for hydropower production, mountain dams store and build up water reserves during seasons of heavy snow melt to assure lowland irrigation and domestic water uses during low flow periods, as well as supply water to mountain lakes used for tourism and fishing (Marnezy, 2008; Consoli et al., 2021). Irrigation dams tend to homogenize flow dynamics (i.e., reducing peak flows and increasing minimum flows; Moyle and Mount, 2007), reduce or increase the downstream water temperature (depending on the residence time, size of reservoir, release depth, and timing of releases; Lessard and Hayes, 2003; Prats et al., 2010), as well as alter the nutrient and sediment fluxes (Olden and Naiman, 2010; Maavara et al., 2020), and limit the habitat connectivity (Grill et al., 2019). It leads to pervasive effects on riverine biodiversity and functioning, e.g., increases in planktonic diatoms (Goldenberg-Vilar et al., 2021), reduction of macroinvertebrate richness (Kennedy and Turner, 2011) or of salmon populations downstream of dams (Moore et al., 2011), among others. Therefore, identifying, monitoring, and managing these impacts on mountain riverine ecosystems while securing human water needs for socioeconomic development has become a major challenge for water resource managers, especially in highly vulnerable regions (e.g., wetlands and mountains; Pang et al., 2018).
Diatoms and macroinvertebrates have been widely used as environmental biomonitoring indicators due to their easy sampling, widespread occurrence, diversity, and reliable indication of anthropogenic impacts (Bonada et al., 2006; Resh, 2008; Pompeu et al., 2023) and are among the biological quality elements recommended to monitor and assess water bodies’ ecological state within the European Water Framework Directive (European Commission, 2000). Taxonomy-based biotic indices are extensively employed to measure ecological responses to nutrient enrichment and organic pollution; however, the effects of flow modification have been more rarely addressed (Birk et al., 2012). In addition, the taxonomic-based metrics may be subject to significant biogeographic and evolutionary constraints (Soininen et al., 2016), reducing their broad geographic applicability (Dolédec et al., 1999). Thus, species traits (i.e., organism’s biological and ecological characteristics) have been increasingly used in ecological research (e.g., White et al., 2017; Brown et al., 2019; Wu et al., 2019b) to assess stressor effects in lotic ecosystems (Statzner and Bêche, 2010) and to develop mechanistic trait-stressor links valid across broad geographical scales (Culp et al., 2011).
In this context, the data originally collected for water quality assessment (e.g., European Water Framework Directive (WFD) surveys), including biological communities and physico-chemical habitat conditions, could be potentially used to assess other eco-hydromorphological relationships in rivers, such as the ecological effects of dams (Vaughan and Ormerod, 2010; Krajenbrink et al., 2019). To do so, an efficient monitoring network is essential (Arrighi et al., 2021), since the actual effects of hydrological alteration may be underestimated with an inadequate spatial (e.g., lack of gauges immediately downstream of dams; Peñas et al., 2016) or temporal coverage (e.g., biological sampling not covering inter and intra-annual hydrological variability; Munné and Prat, 2011).
In this study, our main objective was to assess the effects of dams primarily used for irrigation (although they might include other minor uses such as water supply and hydropower) on the river flow regime, water quality characteristics, and riverine biota (i.e., diatom and macroinvertebrate communities) of mountain rivers using biological datasets originally collected within the EU WFD. We selected streams under natural and regulated flow regimes (i.e., downstream of irrigation dams) and, in order to minimize the effects of geographical distance on generating differences among riverine communities (Pompeu et al., 2022a), our stream selection was clustered around three mountain ranges in northern Spain: the Cantabrian Cordillera, the Iberian System, and the Pyrenees.
We hypothesized that 1) the presence of dams would be linked to hydrological and water physico-chemical alterations, such as changes in the seasonal flow patterns, homogenization of flow dynamics, and reduction of nutrients downstream of dams. As pointed out by Peñas and Barquín (2019), the altered flow patterns caused by dams in Spanish rivers depend, in part, on local factors, e.g., geographical context and the pre-dam river characteristics). Therefore, we expect that 2) other hydrological and physico-chemical changes, such as changes in flow dynamics associated to snow melt and altitude and water quality parameters caused by different geological characteristics, would be linked to the local conditions present in each mountain range (i.e., the “mountain factor”). 3) Taxonomic biotic indices (e.g., richness, diversity, water quality sensitive indices), which are commonly used in diatom and macroinvertebrate bioassessment worldwide, would also significantly respond to the presence of dams. We expect a decrease in taxonomic richness and diversity downstream of dams, and specific indices, such as the Specific Polluosensitivity Index (IPS) for diatoms, the Iberian Biomonitoring Working Party (IBMWP) and Lotic invertebrate Index for Flow Evaluation (LIFE index) for macroinvertebrates, would likely show negative responses to flow regulation. These trends have been observed previously in Spanish rivers (Ladrera and Prat, 2013; Quevedo et al., 2018; Mellado-Díaz et al., 2019; Pompeu et al., 2022b), even though these specific indices have not been designed for the assessment of the impacts of dams but to evaluate the effects of organic pollution (e.g., IPS and IBMWP). 4) Trait-based approaches, however, would provide clearer a insight into the effects of dams on biological communities compared to biotic indices based on taxonomy and designed to respond mainly to organic pollution. Unlike taxonomy, traits are consistent across broad spatial scales and provide mechanistic linkages of biotic responses to stressors (Menezes et al., 2010; Culp et al., 2011). Thus, we expect to detect significant changes, such as changes in diatom ecological guilds or macroinvertebrate feeding groups associated to dam-related impacts.
2 Materials and methods
2.1 Study area and site selection
Our study area covered streams located in three mountain ranges in northern Spain: the Cantabrian Cordillera, the Iberian System, and the Pyrenees (Figure 1A). These mountains constitute the natural geographical limits of the Ebro catchment (>85.000 km2) and present a significant number of structures to attend water and energy demands (e.g., dams and reservoirs for hydroelectric power generation, irrigation, urban water supply, and recreation; López-Moreno et al., 2008; García de Jalón et al., 2019), making them an interesting case study. Most dams in the Pyrenees, for example, were constructed between the 1950s and the 1980s to supply water demands in the Mediterranean region (i.e., irrigation and hydropower production; López-Moreno et al., 2008). In the Cantabrian Cordillera, besides several small hydroelectric power plants, important reservoirs (e.g., Ebro, Aguilar de Campoo, Riaño) supply water for agricultural and urban uses. Reservoirs in the Iberian System, in general, have smaller capacities in relation to the other mountain ranges and provide water for irrigation, urban uses, and hydropower generation.
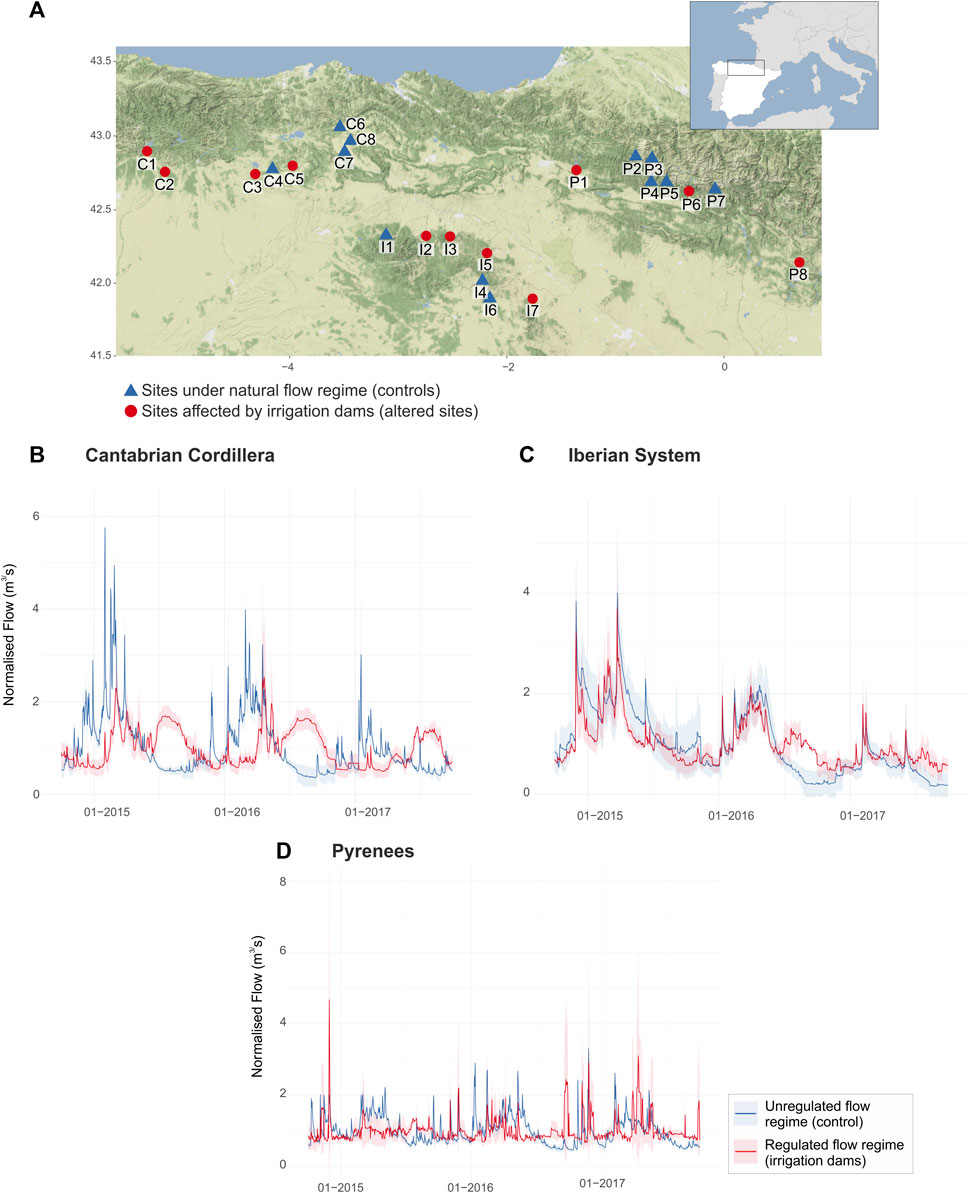
Figure 1. (A) Location of the 23 studied sites: controls (n = 12) and sites downstream of irrigation dams (n = 11). Mean normalized daily discharge between 2015 and 2017 in controls and sites influenced by irrigation dams in (B) the Cantabrian Cordillera, (C) the Iberian System, and (D) the Pyrenees. Shaded areas represent 1SD confidence intervals. See also Supplementary Table S1 for information on sampling sites, reservoirs’ capacity, and purpose. Map tiles by Stamen Design, under CC BY 3.0 Data by OpenStreetMap, under ODbL.
Our sampling site selection was restricted to sites complying with the following requirements: 1) river reaches classified as “good” or “very good” ecological status according to the River Basin Management Plans 2015-2021 (BOE, 2015) within the European WFD; 2) river reaches with available hydrological and water physico-chemical data; and 3) at least one survey on biological communities (diatoms or macroinvertebrates) between June and October 2015, 2016, or 2017. These criteria allowed us to reduce the environmental variability and the effect of other anthropogenic stressors, such as water pollution and nutrient enrichment associated with human activities (e.g., extensive urban or agricultural land uses), while ensuring a sufficient spatial coverage. In total, we selected 23 sampling sites (Figure 1A; Table 1), from which 11 were located downstream of dams, mostly with multiple uses (e.g., hydroelectric power generation, irrigation, recreation), but primarily used for irrigation (i.e., altered sites, see Supplementary Table S1) and 12 were under minimally disturbed conditions (i.e., control sites).
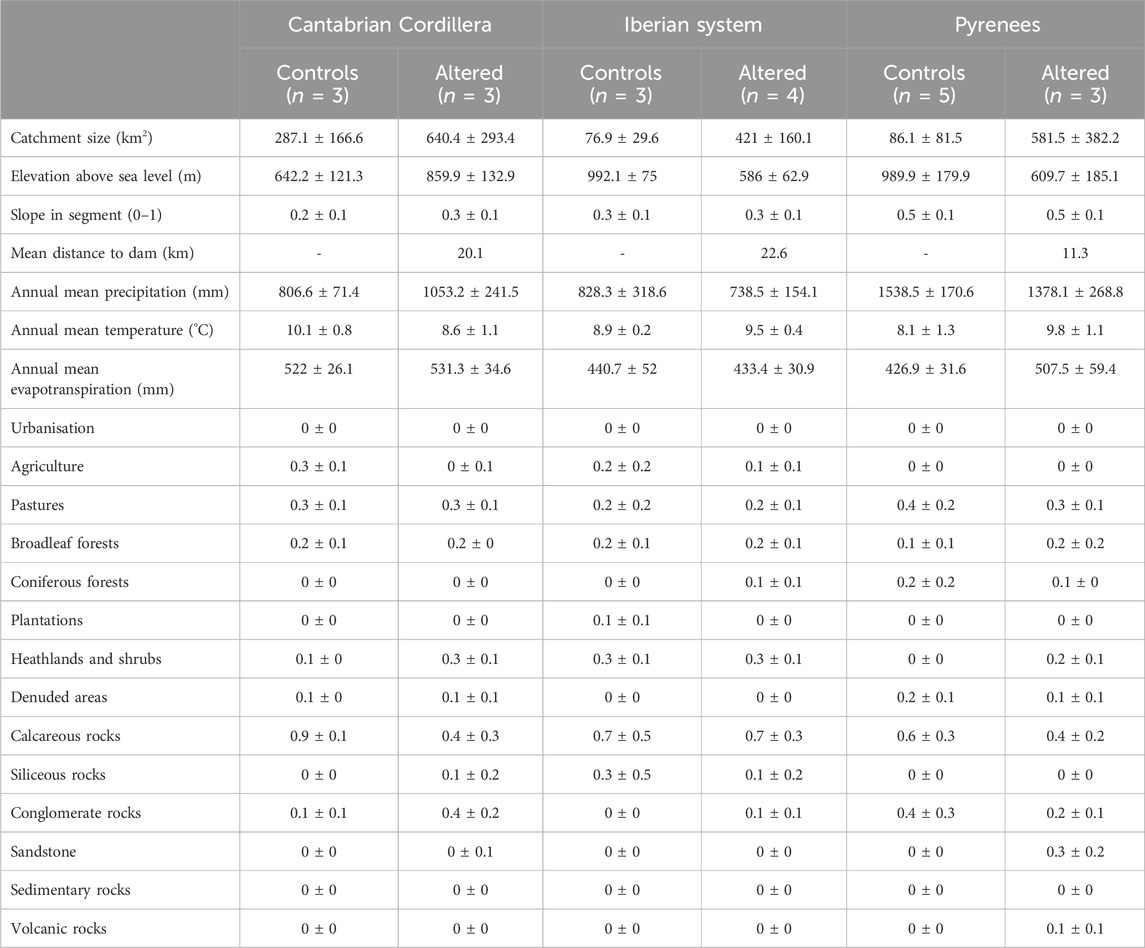
Table 1. Catchment-scale environmental variables in controls and sites affected by dams in each region describing topography, climate, the fractions occupied by land use and land cover, and lithology. Values refer to the catchment mean ± sd upstream of the sampling site.
River gauge stations provided by the Centre for Hydrological Studies (CEDEX, Ministry for Ecological Transition, Spain) located nearby each sampling site recorded the daily river flow discharge. The normalized daily flow series of the three hydrological years (2015–2017), which presented relatively heterogeneous climatic characteristics (2015 marked by extremely warm and dry conditions, 2016 warm and wet, and 2017 warm and very dry; Agencia Estatal de Meteorología, 2023) was then used to generate 35 hydrological indices (HIs; Supplementary Table S2). These HIs characterized the five flow regime components (magnitude, frequency, duration, timing, and rate of change; Olden and Poff, 2003).
2.2 Biological surveys and taxonomic-based biotic indices
Diatom and macroinvertebrate communities were surveyed annually by regional water agencies as part of the water bodies’ ecological status assessment within the European WFD. This biological information was then compiled by the Spanish Ministry for the Ecological Transition in the national database NABIA (BOE, 2015). We selected streams surveyed between June and October, during the low flows (when the streams are wadeable), in 2015, 2016, and 2017 to reduce the seasonal flow variation. In some of these sites, surveys occurred for three consecutive years and/or covered both biological communities. In combination with the biological sampling, the NABIA database provided water temperature (TWATER, °C), pH, dissolved oxygen (DO, mg.L−1), electrical conductivity (µS.cm−1), nitrite (NO2−; mg N.L−1), nitrate (NO3−; mg N.L−1), ammonium (NH4+; mg N.L−1), and phosphate (PO43-; mg P.L−1) concentrations that were collected and determined using standard protocols (UNE-EN ISO 15681-2:2005 for phosphates, UNE-EN ISO 13395:1997 for nitrite and nitrates, UNE-EN ISO 11732:2005 for ammonium).
Diatom communities were surveyed following UNE-EN13946:2014 (CEN, 2014b). Five to ten cobbles were randomly selected from the benthos (circa 100 cm2 of exposed surface area) and the upper part of the substratum was scrubbed with a dishwasher brush. The material was decanted in a sample bottle and preserved using formaldehyde. From each sample, 400 diatom valves were identified in laboratory using a microscope (1000 × magnification) at the lowest feasible taxonomic level, according to the standard procedure UNE-EN 14407:2014 (CEN, 2014a). In total, we gather results from 56 sampling events of diatom communities collected in the 21 sampling sites (see Supplementary Table S1).
Benthic macroinvertebrate surveys followed the standard procedure UNE-EN 16150:2012 (CEN, 2012). The sampling was distributed among the different habitat types present along a 100-m reach. Using a kick hand net (0.1 m2 and 0.5 mm mesh size), community samples were collected and preserved in 96% ethanol. The identification in the laboratory was mainly at the family level, except for Hydracarina, Nematoda, Ostracoda, Copepoda, and Oligochaeta. In total, we gather results from 51 sampling events of macroinvertebrate communities collected in the 21 sampling sites (see Supplementary Table S1).
We eliminated rare taxa (those representing less than 2% of the total sampled individuals when considering the total number of occurrences; Lavoie et al., 2009). In sites surveyed over multiple years, the abundance of taxa was averaged to obtain a site-specific assemblage structure and to reduce interannual variation, following Paavola et al. (2003), resulting in 21 averaged communities for diatoms and 21 for macroinvertebrates. Even though this approach might lead to a simplification of the ecological complexity and the temporal dynamics, as well as the comparison of sites with different sampling efforts (i.e., sampled in less than 3 years), it allowed us to focus on the effect of the presence of dams rather than on the annual climatic and environmental variability. Likewise, for each site-specific assemblage, the average physico-chemical characteristics were calculated.
In each averaged biological community, taxa richness and Shannon-Wiener diversity index were computed using the R package vegan (Oksanen et al., 2019). Additionally, for diatom communities, we computed the Specific Polluosensitivity Index (IPS; CEMAGREF, 1982; Ministerio de Agricultura, Alimentación y Medio Ambiente, 2013a). For macroinvertebrates, the Iberian Average Score per Taxon (IASPT) and the Lotic invertebrate Index for Flow Evaluation (LIFE; Extence et al., 1999) were computed using the R package biomonitoR (Laini et al., 2019).
Diatom species were assigned to ecological guilds, life forms, and cell size (biovolume; see Supplementary Table S3), following Passy (2007) and Rimet and Bouchez (2012). In macroinvertebrate samples, taxa were classified into 11 biological traits for European macroinvertebrate genera (Tachet et al., 2010; Schmidt-Kloiber and Hering, 2015). The traits (Supplementary Table S4) described the organisms’ life history, morphology, food source, feeding habits, respiration, and reproduction, among other characteristics using a fuzzy coding approach. Since most of the taxa were identified at the family level but traits are reported at the genus level, each trait affinity was averaged and re-scaled to this lower taxonomic resolution using affinities of all genera computed within a family. Although the use of family-level identification can result in a loss of ecological information, previous studies (Dolédec et al., 2000; Gayraud et al., 2003; García-Roger et al., 2014) reported minor impacts on the functional description of macroinvertebrate communities.
2.3 Data analysis
We used a two-way analysis of variance (ANOVA; factors: dam/no dam and mountain range) to test (a) the general effects of the presence of irrigation dams (control vs. altered sites), (b) the effect of the mountain range (Cantabrian Cordillera, Iberian System, and Pyrenees), i.e., the influence of local factors, and (c) the interactions between the presence of dams and the mountain range to assess the effects of irrigation dams within each mountain range. Independent analyses were done for the HIs encompassing the three hydrological years (2015, 2016, and 2017), water physicochemical characteristics, and biotic indices. The tests were calculated with type III sums of squares due to the unequal sample sizes in control and altered sites among mountain ranges (Quinn and Keough, 2002). The homogeneity of variances was checked using Levene’s tests and variables were log-transformed when needed. Following the significant two-way ANOVA tests, post hoc pairwise t-tests with Bonferroni correction were used to identify significant differences (p < 0.05) among factors.
Spearman’s rank correlations were also used to identify statistically significant relationships (p < 0.05) among diatom and macroinvertebrate biotic indices, HIs, and physico-chemical characteristics. In line with the two-way ANOVA analyses, the HIs used in the Spearman’s rank correlations were also computed based on the 2015–2017 hydrological years.
The relationships between organisms’ traits and environmental descriptors were investigated with the RLQ and fourth-corner analyses using the R packages ade4 (Dray and Dufour, 2007) and adespatial (Dray et al., 2020). The RLQ analysis (Dolédec et al., 1996), an exploratory ordination method, combines three tables: table R (n × m) containing the measurement of m environmental variables (i.e., HIs encompassing the three hydrological years and physico-chemical variables) at n sites; table L (n × p) with the abundance of p taxa (i.e., diatom and macroinvertebrate taxa) at n sites; and table Q (p × s) describing s traits (i.e., diatom and macroinvertebrate traits) related to the same p taxa. The method generates a fourth matrix representing the cross-variance structure explained by environmental variables and functional traits, providing a broad overview of how they are associated (Brown et al., 2014; Thioulouse et al., 2018). The overall significance of the trait-environment relationship was then assessed with 9999 permutations using a two-step procedure (Dray et al., 2014), which simultaneously test the permutation of sites (testing the null hypothesis that the species distribution is randomly attributed to sites, irrespective of the site characteristics) and species (testing the null hypothesis that species are distributed irrespective of their traits) while controlling the type I error (Ter Braak et al., 2012). Further, the fourth-corner analysis was used to identify significant individual trait-environment relationships. This method computes a matrix (s × m) with one-to-one trait-environment correlations (Dray et al., 2014).
Prior to the analyses, physico-chemical variables were standardized (zero mean and unit standard deviation) to reduce the effects of different measurement units and to improve normality (Quinn and Keough, 2002). The statistical significance level for the ANOVA tests, Spearman’s rank correlation, and RLQ and fourth corner analyses was set to p ≤ 0.05. All the analyses were performed in the R environment (R Core Team, 2020).
3 Results
3.1 Dam effects on HIs and physico-chemical variables
The two-way ANOVA tests associated with the presence of dams only (D factor in Figure 2) identified significant changes uniquely due to this factor in the summer (+M7,8,9), winter (-M12), and spring flows (-M3), the frequency of high flow events (-FRE3), and the timing of minimum flows (-JMin). These findings indicated that irrigation dams produced seasonal flow inversions (i.e., increased summer flows but reduced spring and winter flows) and less frequent high flow events independently of the mountain range, i.e., the geographical region.
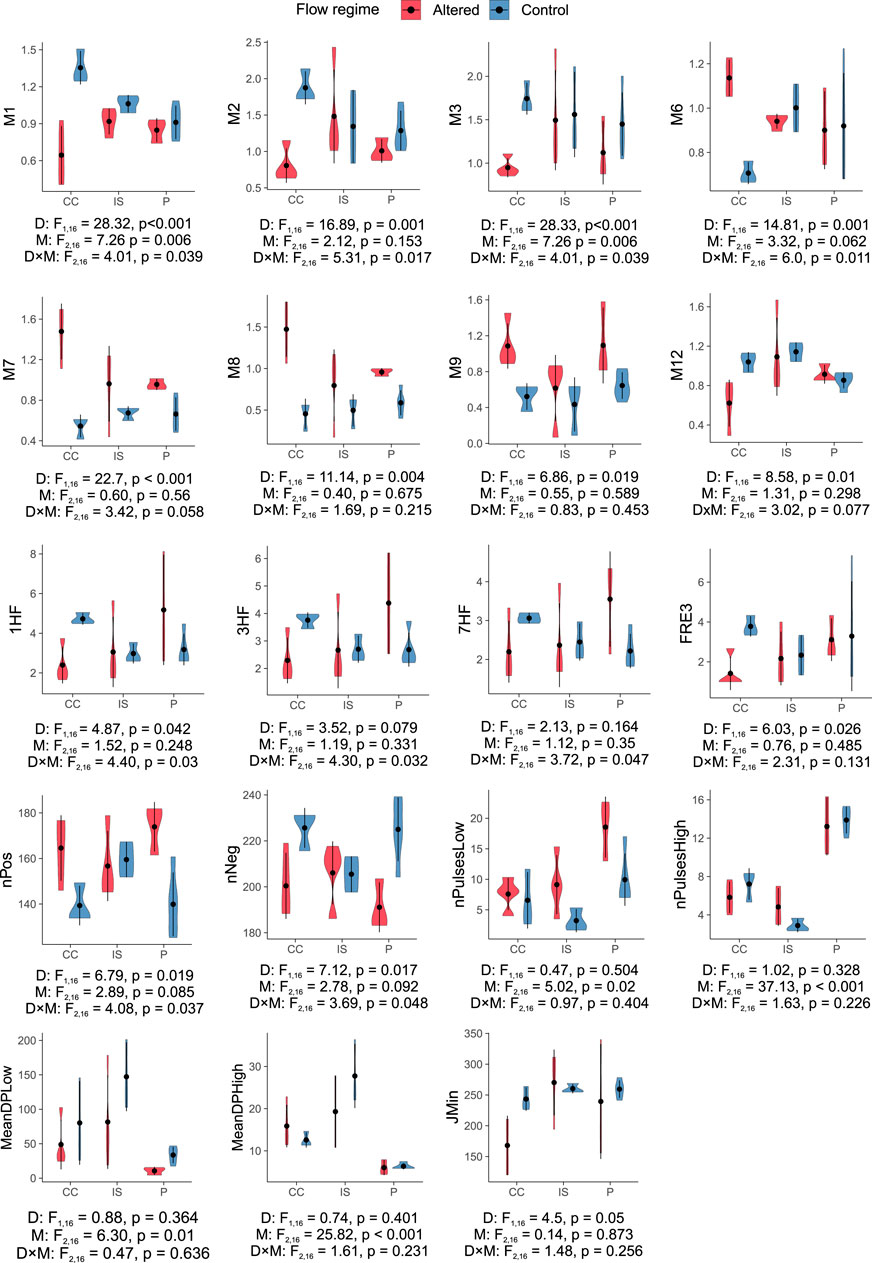
Figure 2. Violin plots representing the distribution of hydrological indices in the Cantabrian Cordillera (CC), Iberian System (IS), and Pyrenees (P) among control (blue) and altered (red) sites. The two-way ANOVA tests are shown: “D” refers to the ANOVA test with flow regime (dam presence) as a factor, “M” to mountain range as a factor, and “D × M” as the interaction between these factors. Only hydrological indices with significant differences are shown (see all variables in Supplementary Figure S1). Vertical bars represent the standard deviation.
In turn, when considering the mountain factor alone (M factor in Figure 2), the number of high and low flow pulses within a year (nPulsesHigh, nPulsesLow) and their duration (MeanDPHigh, MeanDPLow) showed significant changes. In the Pyrenees, the post hoc tests showed that the high and low flow pulses were significantly more frequent than in the Iberian System (p < 0.04), but shorter in duration in comparison to other regions (p < 0.001 for MeanDPHigh in all cases; p = 0.006 for MeanDPLow in relation to the Iberian System). These findings indicated that the frequency and duration of flow pulses varied among mountain ranges independently of the presence of dams.
The interaction term that allowed us to analyze the effect of irrigation dams based on the comparison of control and altered sites within each mountain range (D × M in Figure 2), showed significant differences in the winter (M1,2) and early summer flows (M6), the magnitude of short-term high flow extremes (1, 3, 7HF), and the number of days with increasing and decreasing flows (nPos, nNeg). The post hoc tests revealed that most of the significant differences were found between control and altered sites in the Cantabrian Cordillera (p at least <0.015 for M1, M2, M6) and in the Pyrenees (p at least <0.045 for nPos and nNeg).
In contrast to hydrology, physico-chemical characteristics did not seem to be significantly influenced by the presence of dams or their geographical location (Figure 3). Nitrate was the only variable that presented a marginally significant reduction downstream of dams (p = 0.052), independently of the mountain range (D factor in Figure 3).
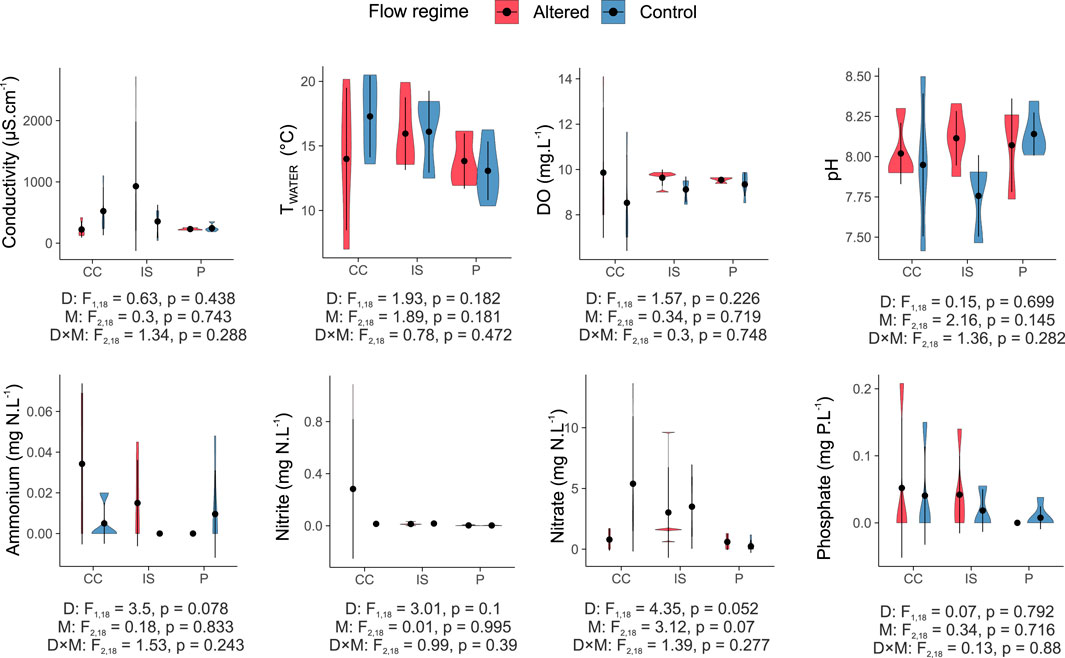
Figure 3. Violin plots representing the distribution of physico-chemical variables in the Cantabrian Cordillera (CC), Iberian System (IS), and Pyrenees (P) among control (blue) and altered (red) sites. The two-way ANOVA tests are shown: “D” refers to the ANOVA test with flow regime (dam presence) as a factor, “M” to mountain range as a factor, and “D × M” as the interaction between these factors. Vertical bars represent the standard deviation.
3.2 Biological communities and the effects of dams on biotic indices
We retained 55 diatom species for the analyses (see Supplementary Table S5 for the taxa list). The most ubiquitous species were Achnanthidium pyrenaicum and Achnanthidium minutissimum. Control sites also showed higher mean abundances of Gomphonema angustivalva and Achnanthidium subatomus, while altered sites presented higher abundances of Achnanthidium lineare and Cocconeis euglypta.
Taxonomic-based biological indices, in contrast to hydrological indices, did not show significant differences linked to dam or mountain range effects alone. However, the dam-mountain range interaction seemed to significantly influence the diatom taxonomic richness, Shannon-Wiener diversity index, and the IPS score (Figure 4A). The effect of dams on biological communities varied among regions, with streams in the Iberian System (IS in Figure 4A) showing clearer changes than the other two mountain ranges. In this regard, diatom richness and diversity increased, while the IPS score decreased in altered sites in comparison to controls; despite this, the post hoc pairwise t-tests with Bonferroni correction did not detect significant differences. The Spearman’s rank correlations (Supplementary Figure S2A) indicated that, in general, richness and Shannon-Wiener diversity were significantly linked to summer flows and water temperature.
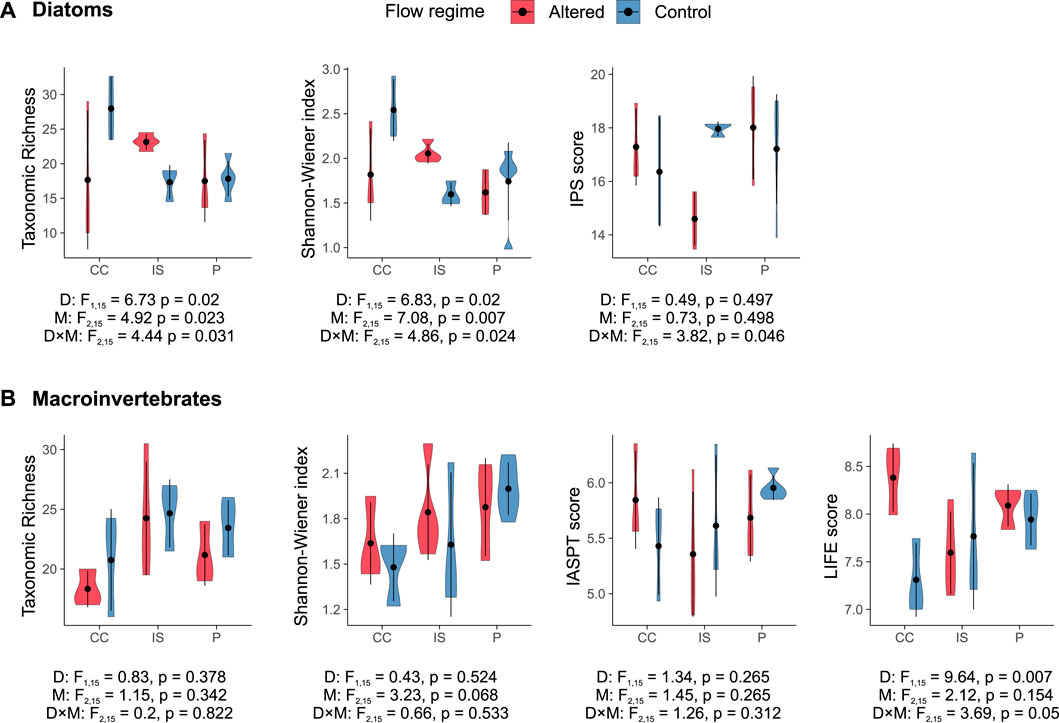
Figure 4. Violin plots representing the distribution of taxonomic biotic indices in (A) diatom and (B) macroinvertebrate communities in the Cantabrian Cordillera (CC), Iberian System (IS), and Pyrenees (P) among control (blue) and altered (red) sites. The two-way ANOVA tests are shown: “D” refers to the ANOVA test with flow regime (dam presence) as a factor, “M” to mountain range as a factor, and “D × M” as the interaction between these factors. Vertical bars represent the standard deviation.
In macroinvertebrate communities, a total of 43 taxa were retained for the analyses (Supplementary Table S6). Baetidae, Chironomidae, and Gammaridae were the most ubiquitous families. Control sites showed higher mean abundances of Chironomidae, Heptageniidae, and Leuctridae, while Simuliidae, Hydrobiidae, and Elmidae were more abundant in impacted sites.
The LIFE score (Figure 4B) was the only macroinvertebrate biotic index that presented significant changes associated with dams and the dam-mountain range interaction, although the post hoc pairwise t-tests with Bonferroni correction did not detect significant differences between groups. On average, altered sites in the Cantabrian Cordillera scored higher than control sites, while in the other mountain ranges, scores in control and altered sites were similar. The Spearman’s rank correlation (Supplementary Figure S2B) indicated that the LIFE score was positively correlated to summer flows and the magnitude of low flow extremes but negatively correlated to winter flows and the frequency of high flow events.
3.3 Trait-based responses to dams in diatom communities
The overall RLQ permutation test on diatom communities showed a marginally significant trait-environment relationship (permutation across sites p = 0.05, across species p = 0.064). The first two axes of the RLQ analysis explained 85.7% of the cross-variance between diatom traits and HIs and physico-chemical conditions (Figure 5).
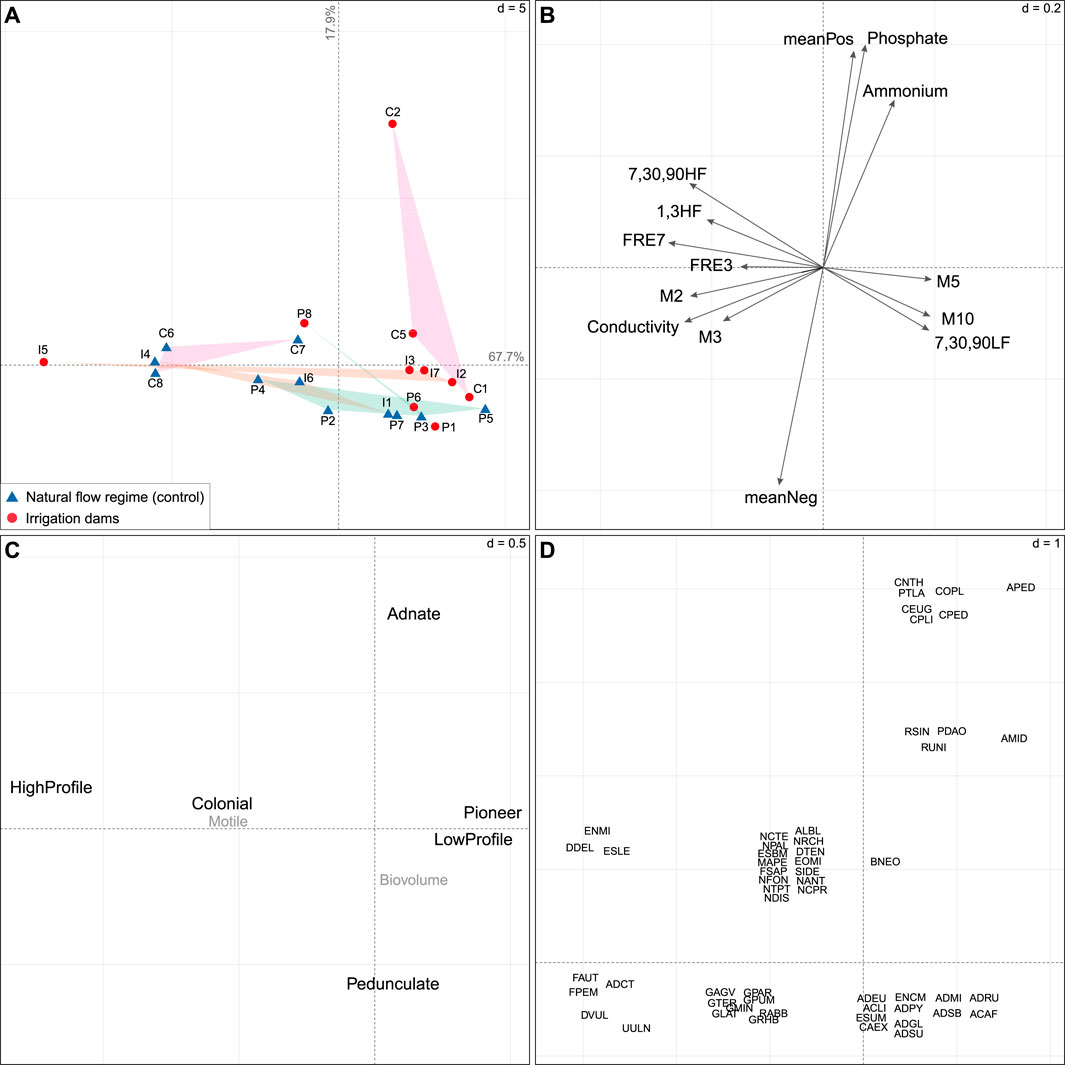
Figure 5. Ordinations showing the first two RLQ axes on diatom communities. (A) Sampling site scores classified according to the presence (red circles) or absence (blue triangles) of dams and geographical regions: Cantabrian Mountain Range (C; in pink), Iberian System (I; orange), and Pyrenees (P; green). (B) Coefficients for HIs (see Supplementary Table S2 for codes) and physico-chemical variables with strong links to traits (see fourth corner analysis in Supplementary Figure S3). (C) Coefficients for traits (see Supplementary Table S3 for codes). Traits with no significant relationships (see fourth-corner analysis, Supplementary Figure S3) are represented in grey. (D) Coefficients for species (see Supplementary Table S5 for codes). The “d” values give the grid size for scale comparison across the graphs.
Along the first axis (67.7% of the total variance, Figure 5), the Cantabrian Cordillera and Iberian System controls were concentrated on the negative side (left). Sites I5 and P8, which were affected by the multipurpose reservoirs Enciso and Escales, respectively (see Supplementary Table S1), were also clustered with controls. They were linked to more frequent and stronger high flow events (+FRE3,7, +1-90HF), higher February (+M2) and March (+M3) flows, and higher water conductivity. These conditions favored high profile and colonial diatoms while reducing the low profile guild. In contrast, altered sites in the Cantabrian Cordillera and most of the altered sites in the Iberian System were linked to higher mean magnitudes of spring (+M5) and autumn (+M10) flows and long-term low flow extremes (+7,30,90LF), favoring pioneer diatoms and reducing the high profile guild.
A further 17.9% of the total variance was explained by the second RLQ axis (Figure 5). The higher phosphate and ammonium concentrations, as well as the stronger rates of flow change (+meanPos, -meanNeg), observed especially in the C2 site, favored adnate diatoms. In turn, pedunculate diatoms were linked to less strong flow change rates (+meanNeg) and lower nutrient concentrations.
3.4 Trait-based responses to dams in macroinvertebrate communities
The overall RLQ permutation test revealed a non-significant macroinvertebrate trait-environment relationship (permutation across sites p = 0.318, across species p = 0.47). The first two RLQ axes accounted for 70.3% of the total variance (Figure 6).
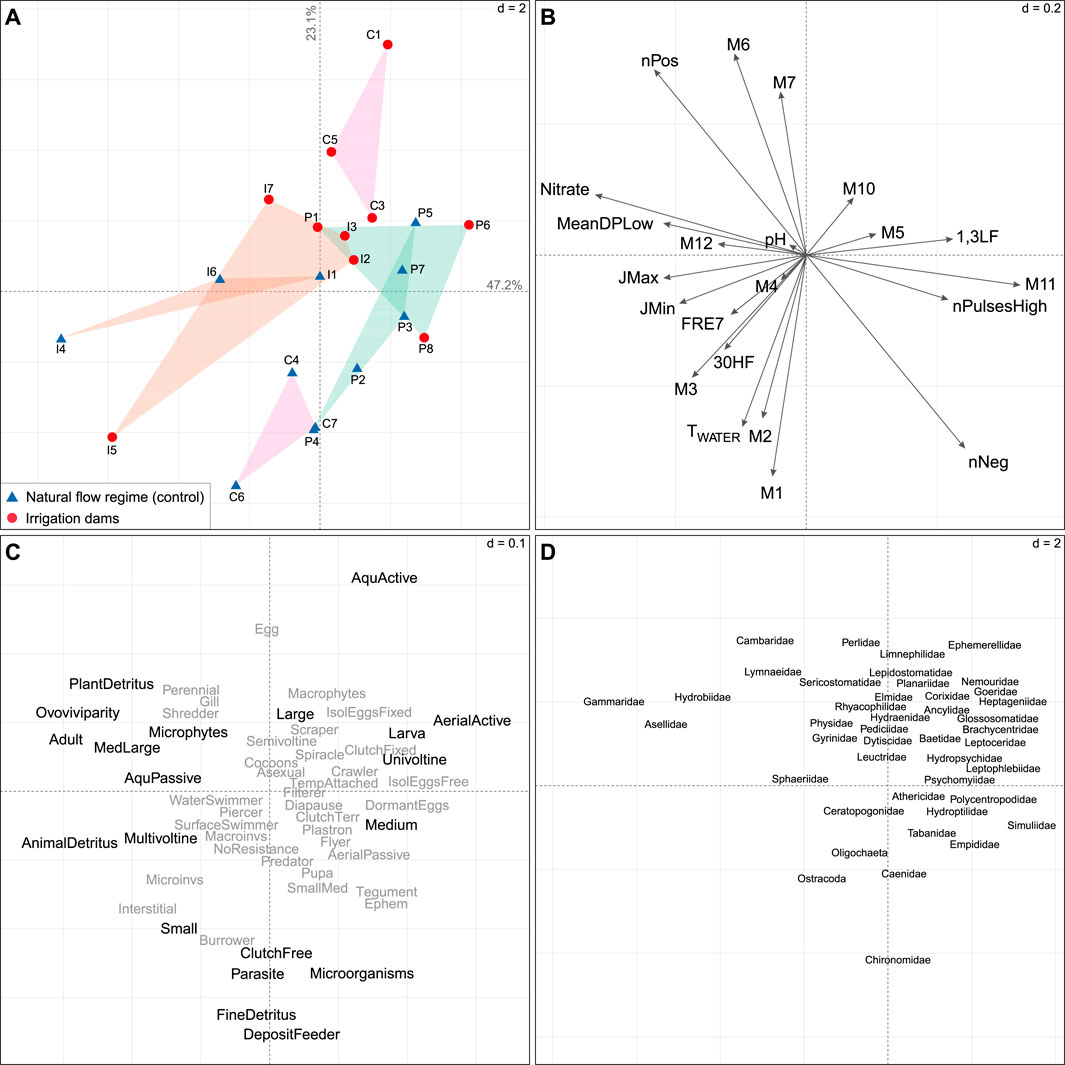
Figure 6. Ordinations showing the first two RLQ axes on macroinvertebrate communities. (A) Sampling site scores classified according to the presence (red circles) or absence (blue triangles) of dams and geographical regions: Cantabrian Mountain Range (C; in pink), Iberian System (I; orange), and Pyrenees (P; green). (B) Coefficients for HIs (see Supplementary Table S2 for codes) and physico-chemical variables with strong links to traits (see fourth corner analysis in Supplementary Figure S4). (C) Coefficients for traits (see Supplementary Table S7 for codes). Traits with no significant relationships (see fourth-corner analysis, Supplementary Figure S4) are represented in grey. (D) Coefficients for species (see Supplementary Table S9). The “d” values give the grid size for scale comparison across the graphs.
Along a gradient of flow regulation, control sites were mainly concentrated in the bottom part of the ordination, except for site I5, which was affected by the reservoir Enciso. The Cantabrian Cordillera and Iberian System controls were mostly clustered in the bottom-left quadrant. These sites were associated with increased frequency (+FRE7) and magnitude of high flow events (+30HF), winter (+M1,2) and spring (+M3,4) flows, later timing of minimum and maximum annual extremes (+JMin, +JMax), and higher water temperatures (+TWATER). These conditions favored macroinvertebrates with small body sizes (<1cm, Small, e.g., Hydroptilidae), more than one reproductive cycle per year (Multivoltine, e.g., Sphaeriidae), deposit feeders, and fine detritus (FineDetritus, e.g., Ostracoda, Caenidae) and animal detritus (AnimalDetritus; e.g., Lymnaeidae) as food sources.
In turn, altered sites were mostly concentrated in the top-right quadrant (except for control sites P5 and P7). They were associated with increased spring (+M5), summer (+M6,7), and autumn (+M10) flows and increased magnitude of short-term low flow extremes (+1,3LF). These conditions favored macroinvertebrates with larger body sizes (>4 cm, Large, e.g., Pediciidae), one potential reproductive cycle per year (Univoltine), larval aquatic stages (Larva, e.g., Perlidae), aquatic (AquActive, e.g., Ephemerellidae) and aerial active (AerialActive, e.g., Heptageniidae) dispersions.
Macroinvertebrate communities in the Cantabrian Cordillera sites showed a more pronounced difference among control and altered sites than the other mountain ranges. Along the second axis (23.1% of the total variance, Figure 6), the Cantabrian Cordillera impacted sites, which were linked to higher summer flows (+M6,7), showed to favor taxa with larger body sizes and larval aquatic stage. In contrast, control sites were associated with increased winter flows (+M1,2) and higher water temperatures, and favored small-sized macroinvertebrates and detritus feeders.
Nutrient concentration and flow change gradients were observed along the first axis (47.2% of the total variance, Figure 6). Iberian System sites were more concentrated on the left side with increased nitrate concentration and longer low flow pulses, favoring macroinvertebrates with medium to large body sizes (MedLarge, e.g., Rhyacophilidae) and adult aquatic stage (Adult, e.g., Hydrobiidae, Gammaridae). In contrast, lower nitrate concentrations and more frequent and shorter flow pulses (+nPulsesHigh, -MeanDPLow) were more associated with Pyrenees’ sites, favoring macroinvertebrates with medium body sizes (Medium, e.g., Heptageniidae), aquatic larvae, and aerial active dispersion.
4 Discussion
In this study, we assessed the effects of irrigation dams on the riverine flow regime, water quality, and diatom and macroinvertebrate communities of mountain streams. Summer flows (e.g., magnitude of July, August, and September flows), frequency of high flow events, and the timing of minimum annual flows showed to be significantly affected by the presence of dams, independently of the local environmental conditions, as hypothesized. Water physico-chemical variables, however, did not show to be significantly influenced by the presence of dams. Our second hypothesis was also partially supported. Flow pulse patterns showed to be significantly dependent on the mountain conditions (i.e., mountain range factor), while the magnitude of winter flows and high flow extremes and the rate of flow change were influenced by both factors. Water physico-chemical variables did not exhibit significant changes in response to the mountain conditions.
Diatom communities presented significant responses to dam impacts in terms of biotic indices and traits across the three mountain ranges, partially supporting Hypothesis 3. Macroinvertebrate communities, in contrast, showed less evident responses to geographical and dam-induced changes when analyzing both biotic indices and functional traits. In general, traits showed to be useful in understanding dam-related effects on biological communities, in contrast to biotic indices, as expected in Hypothesis 4.
4.1 Abiotic changes associated with dams
In our study, the magnitude of seasonal flows, the frequency of high flow events, and the timing of minimum annual flows, in general, varied significantly with the presence of dams. Although the hydrological period analyzed was relatively short (3 years), we observed significant alterations across the three mountain ranges. The magnitude of the alteration (and in some cases the direction, e.g., M6) varied between the three regions, with particularly relevant hydrological alterations in the Cantabrian Cordillera. Winter flows, high flow extremes, and the number of flow rises and falls were shown to be significantly affected by the interaction between flow regulation and mountain range factors. Moreover, changes in other HIs such as flow pulse changes were related exclusively to the mountain range factor (i.e., affected by local conditions only). These results indicate that there is an influence of the regional characteristics (e.g., climate, LULC, geology) besides dam-induced changes. This also agrees with Peñas and Barquín (2019), who observed a geographical dependence of the patterns of altered river flow regimes on the river’s pre-dam characteristics.
As it has been briefly discussed above, dams located in the Cantabrian Cordillera displayed the most pronounced inversion of the seasonal flow regime compared to the other mountain ranges. This inversion results from the retention of winter flow peaks and the release of impounded water during summer to address seasonal water demands, as also reported in previous studies (Belmar et al., 2013; García de Jalón et al., 2019). The relatively large capacity of these reservoirs (e.g., Riaño, Ebro, Juan Benet, >150 hm3 of storage volume; see Supplementary Table S1) contributed to the pronounced seasonal flow inversions. Conversely, in the Pyrenees and the Iberian System, no significant changes in HIs associated to seasonal patterns were detected. The substantially lower storage volumes of these reservoirs may not be the only responsible for these results. Other dam uses (e.g., Bubal, Lanuza, and Escales are also used for flood control and hydropower production; López Moreno et al., 2016) might also contribute to the observed differences. Accordingly, we found that HIs associated with hydropower, such as nPos and nNeg, were significantly altered in the Pyrenees, in agreement with previous studies (Almeida et al., 2020; Chalise et al., 2021; Pompeu et al., 2022b).
Independently of the dam presence, the streams in the Pyrenees exhibited shorter and more frequent high and low flow pulses in comparison to the Iberian System. This can be associated to the higher altitudes, slope, and increased precipitation found in Pyrenean streams. In addition, altered sites in both Iberian System and the Pyrenees showed a slight reduction in the spring flows (see Supplementary Figure S1), which could be related to the retained spring snowmelt flows occurring in these mountain areas (Dott et al., 2022). These results highlight the importance of the regional mountain conditions and the dam characteristics in the hydrological responses to flow regulation.
The physico-chemical variables did not show significant changes associated to irrigation dams or the mountain range. Although water temperature and nutrient concentration have been reported to be strongly impacted by dams (Olden and Naiman, 2010; Chandesris et al., 2019; Maavara et al., 2020), in our case, the available physico-chemical data (spot measurements collected along with the annual biological surveys) might be insufficient to show these effects. For instance, attending to our criteria for sampling site selection (i.e., sites in “good” ecological status), we limited the range of nutrient concentrations, which certainly contributed to the low ability to detect significant differences in nutrient-related variables. Moreover, the long distances between sampling sites and the dams (>20 km downstream of dams in some cases), might buffer the dam-related effects on the temperature. A study conducted by Zaidel et al. (2021) reported persistent changes in water temperature for an average of only 1.3 km downstream of dams. Therefore, a continuous monitoring strategy in closer proximity to dams would be needed to better understand the effects of irrigation dams on physico-chemical variables (Vilmin et al., 2018).
4.2 Changes in taxonomic biotic indices associated with dams and mountain ranges
The diatom biotic indices showed significant changes to the flow regulation-mountain range interaction, suggesting that the dam-induced effects on diatom communities may be dependent on the mountain range. In the Iberian System, slightly higher taxonomic richness and diversity were detected in regulated sites, in agreement with previous studies (Smolar-Žvanut and Mikoš, 2014; Krajenbrink et al., 2019). It suggests that diatom communities might benefit from more stable flows in regulated sites, as these conditions favor the development of biofilm algae (Biggs et al., 1998; Biggs and Smith, 2002). The IPS score, which was originally developed to target organic pollution (CEMAGREF, 1982) but also widely used in riverine bioassessment in Spain within the WFD (BOE, 2015), showed a reduction in flow-regulated sites in the Iberian System only. Since the index did not respond significantly to HIs in our study, it could be linked to local environmental factors (e.g., higher altitudes and higher siliceous geology in controls in comparison to altered sites in the Iberian System) or other water quality characteristics not covered in our study. These findings can be linked to the influence of local habitat characteristics and spatial-related factors (e.g., dispersal and biogeography) on the ecosystems besides the presence of dams. Previous studies reported this interactive effect of river hydrological variation, local, and spatial factors on the diatom and macroinvertebrate communities (Karaouzas et al., 2019; Laini et al., 2019; Pompeu et al., 2022a; Krajenbrink et al., 2022).
The macroinvertebrates’ taxonomic richness and the Shannon-Wiener diversity did not show significant responses to flow regulation. Although numerous studies indicate that dam construction decrease macroinvertebrate richness (Wu et al., 2019a), these biotic indices could be weakly correlated to hydromorphological alterations, as observed in our study (see Spearman’s rank correlation in Supplementary Figure S2) and elsewhere (Feld et al., 2014). Thus, taxonomic replacement associated with hydrological alteration may not cause important changes in biotic indices based on the whole community (i.e., richness and diversity), as different species can replace those lost due to hydrological impacts (Feld et al., 2014). Both IBMWP and IASPT macroinvertebrate scores, which are commonly used in the WFD river health assessment, did not show significant responses to flow regulation, contradicting previous research conducted on Spanish rivers (Quevedo et al., 2018; Mellado-Díaz et al., 2019). Click or tap here to enter text.These findings could be attributed to the fact that IBMWP and IASPT are based on macroinvertebrate families’ sensitivity to organic pollution and were originally designed to target water quality rather than other stressors (Friberg et al., 2011). Hence, our findings support the argument that these indices should only be used to assess the impacts for which they were designed while they are prone to fail when assessing the effects of other stressors (e.g., flow regulation by dams).
Contrary to expected, the LIFE index, a UK-based index designed to evaluate hydromorphological stress (Extence et al., 1999), failed to detect impacts downstream of irrigation dams. Moreover, altered sites in the Cantabrian Cordillera scored higher than controls, in contrast to the findings reported by White et al. (2017). This divergence could be attributed to the study design and the distances between the dam and the sampling location. Mellado-Díaz et al. (2019) reported an increase in the LIFE score immediately downstream dams (between 0 and 4 km downstream), then they showing a recovery across the downstream gradient (4–20 km). Pompeu et al. (2022b), following an optimized and statistically robust control-impact design, highlighted clear biological and ecological responses downstream irrigation dams. Local factors other than hydrology (e.g., water quality, channel morphology, and anthropogenic alteration of riverine habitats), which were not fully addressed in our study might also have a significant impact on the macroinvertebrate community structure and thus, on the recorded LIFE scores (Worrall et al., 2014; Laini et al., 2018; Laini et al., 2022).Therefore, long-term biological sampling schemes following optimal control-impact designs are critical to develop biological indices able detect changes in macroinvertebrate communities associated with natural gradients and specific stressors, such as irrigation dams (Vaughan and Ormerod, 2012).
4.3 Changes in trait-based communities associated with dams and mountain ranges
As hypothesized, the trait-based biological responses varied with the different flow regime alteration patterns and the regional control. In the Cantabrian Cordillera, communities showed a clear segregation between control and impacted streams, which can be linked to the marked hydrological alteration identified in this region (i.e., seasonal flow inversion, with increased summer flows and lower winter flows) and the larger size and regulation capacity of these reservoirs. In contrast, in the Iberian System and the Pyrenees, the community shifts associated to seasonal flow inversions were less evident. This was especially obvious in some specific altered sites (e.g., I5 and P8), where impacted communities were not different from controls. In these sites, we argue that the reduced influence of dams on the biological communities might be associated to the smaller reservoir sizes, and the significant sampling site-dam distances (exceeding 20 km, with tributaries joining the main stem; see Supplementary Table S1) would act as a buffer, mitigating dam-related hydrological and biological impacts. Moreover, the multiple-purpose character of these dams (used for irrigation, urban water supply, and hydropower generation) may cause a less clear-cut hydrological alteration (e.g., changes in the flow rise frequency). These findings highlight the importance of thoroughly planning appropriate biomonitoring study designs when investigating the effects on riverine communities. Factors such as the distances between the dams (or other stressors) and the sampling sites, the similarities between impacted and unimpacted sites, the geographical location or the sampling period and consistency along large periods, among others, must be carefully considered.
The diatom trait-based analysis indicated that control sites, in general, favored the high profile guild and colonial life forms, which are both traits linked to low flow disturbances (Passy, 2007). Dam-impacted sites, in turn, favored pioneer and low profile diatoms, i.e., traits resistant to flow disturbances (Passy, 2007; Tornés et al., 2015). Our results contrast with Goldenberg-Vilar et al. (2021), who reported that resistant traits (e.g., small-sized, non-colonial diatoms) in unregulated streams were replaced by colonial life forms, large-sized, and loosely attached diatoms in sites affected by dams in northern Spain. These divergences can be attributed to the different sampling periods and the effect of environmental variability present in our dataset. The study conducted by Goldenberg-Vilar et al. (2021) sampled biological communities in 2017 using a more straightforward study design, which was specifically planned to investigate the effects of dams on diatom communities.
The increased flow rates of change may also have contributed to the significant diatom community shifts observed in our study, which favored adnate life forms and reduced pedunculate ones. Adnate life forms adhere to the substrate and are highly resistant to repeated scour disturbances (Tornés et al., 2015). However, we highlight that diatom responses to flow regime components may be confounded by the interactions between dam-related effects and environmental variability (Wagenhoff et al., 2017; Krajenbrink et al., 2019), e.g., differences in the altitude, land use and land cover, and geology among mountain ranges. Therefore, reducing the environmental variability would contribute to better assess dam-related impacts.
In contrast to diatoms, macroinvertebrates did not show a globally significant relationship between trait composition and the hydrological and physico-chemical variables, although the impacted sites in the Cantabrian Cordillera streams were clearly segregated from controls. This result highlights the potential use of diatom traits to assess the dam-related impacts over relatively broad spatial scales. Nevertheless, the individual significant trait-hydrology relationships identified in the macroinvertebrate analyses might be useful to understand the mechanistic effects of dam-related impacts. Traits better adapted to the dynamic flow conditions (e.g., small body sizes, multiple reproductive cycles per year) in unregulated mountain streams, especially in the Cantabrian Cordillera, were replaced by univoltine taxa, larger body sizes, larval aquatic stages, and active dispersion, as also reported in previous studies (Bonada and Dolédec, 2018; Piano et al., 2020).
4.4 Study limitations
This study relies on data originally collected for the water bodies’ ecological status assessment within the European WFD. Post hoc analyses of these existing datasets may support hydroecological research and help identifying some general patterns at large spatial scales (e.g., Krajenbrink et al., 2019; Feeley et al., 2020; Kelly-Quinn et al., 2020); however, several inherent challenges arise when using data surveyed for other purposes (Vaughan and Ormerod, 2010).
One key issue potentially obscuring the emergence of more clear patterns is the high degree of data heterogeneity in WFD datasets. In this regard, adhering to rigorous criteria for sites selection allowed us to obtain statistically meaningful results and conclusions, but also revealed several barriers establishing a robust study design. These barriers include, among others, the difficulty in finding comparable rivers within and between different regions (i.e., mountain ranges), the distance to the dam, the temporal extent (e.g., surveys carried out in years with different hydrological and environmental legacies), the limited spatial coverage due to the lack of essential data in many sites (e.g., relatively few sampling sites paired to flow gauges), the variations in sampling efforts among sites, and the simplification of the ecological complexity when averaging the biological communities sampled in different years.
Additionally, the lack of relevant variables measured in the field (e.g., hydromorphological conditions, sediments or longer-term water quality) and longer river flow discharge series (>10 years) to characterize long-term hydrological patterns may also have contributed to the uncertainties observed in this study. All these issues should be specifically addressed in the design of future works aiming at improving our understanding on the effects of different types of dams on riverine biological communities. In this sense, previous studies have demonstrated the suitability of WFD datasets to understand the main environmental factors controlling biological communities at large spatial scales (Feeley et al., 2020; Sturbois et al., 2021; Pompeu et al., 2022a; Heß et al., 2023; Pompeu et al., 2023). In contrast, their suitability to test hypotheses dealing with the effect of specific stressors (i.e., dams) in more localized areas (i.e., local and regional studies) could be more uncertain and controversial, as highlighted in this study. In this case, to discern between the effect of the dams and the natural variability of the riverine ecosystem, more explicit and meticulous study designs are necessary (England et al., 2021; Pompeu et al., 2022b). Long-term monitoring strategies should be also considered to capture the temporal dynamics of biotic communities and overcome the ecological complexity under variable environmental conditions (Jackson and Füreder, 2006; England et al., 2021).
In addition, as required by the lotic bioassessment protocols in Spain (e.g., IBMWP index; Alba-Tercedor et al., 2002; Ministerio de Agricultura, Alimentación y Medio Ambiente, 2013b), macroinvertebrate communities were mainly identified at the family level. Although family-level identification is reported to be cost-effective and suitable for bioassessment (Gayraud et al., 2003; Heino and Soininen, 2007), the use of finer taxonomic resolution may be necessary to detect subtle impacts in macroinvertebrate communities (Chessman et al., 2007). Likewise, taxonomic resolution may cause significant differences in LIFE scores (Monk et al., 2012) and in the detection of the key environmental variables controlling community patterns (Jiang et al., 2013).
We also point out that the RLQ permutation tests failed to indicate a significant overall trait-environment association in macroinvertebrate communities. These findings indicate that macroinvertebrate taxa distribution was independent of the environmental characteristics (i.e., hydrology and physico-chemistry) or functional traits. Even though all selected sites were located in mountain areas, the environmental and hydrological heterogeneity among regions (e.g., variations in altitude, climate, geology, and hydrological characteristics) may produce substantial trait-based differences among communities at the regional scale (Bonada et al., 2007; Pompeu et al., 2022a; 2022b) and substantially increase the noise in the analyses. Previous research has also reported that controlled settings produced more significant outcomes since the effect of confounding variables is reduced and a direct investigation of the trait-environment response is allowed (Brown et al., 2019). Thus, future research should focus on the reduction of hydrological and environmental variability. Methods such as control-impact study design, grouping dams with similar characteristics (McManamay et al., 2016), and hydrological classification (Arthington et al., 2006) would reduce the noise arising from the inherent environment variability and, therefore, enable a more precise analysis of dam-related impacts (Pompeu et al., 2022b).
4.5 Implications for biomonitoring and conservation efforts
In contrast to taxonomic biotic indices, trait-based analyses allowed establishing mechanistic linkages of biotic functional responses to hydrological and physico-chemical conditions (Culp et al., 2011), such as changes in the diatom life forms and biovolume along the dam impact gradient, which has been reported elsewhere (Murphy et al., 2017; Troia and McManamay, 2019). In combination with HIs, traits can provide a template to create and test large-scale hypotheses regarding flow-ecology responses (Poff and Allan, 1995; McManamay et al., 2015). Although research in this field is growing (Wu et al., 2019b; Goldenberg-Vilar et al., 2021; Larsen et al., 2021; Pompeu et al., 2022b), due to the lack of long-term discharge data and paired biological samples, linkages between the flow regime and biological communities are usually not fully explored. Therefore, setting biomonitoring networks in which communities are sampled more frequently (e.g., covering seasonal variability), over a long and meaningful time frame (e.g., at least 10 years), and with adequate spatial coverage (e.g., relatively close to dams and with paired river gauging) would be beneficial for monitoring and tackling dam impacts on river ecosystems, as well as for identifying future research priorities.
Furthermore, exploring the responses of different organisms to dam-related impacts is essential to select meaningful biomonitoring metrics (Poff and Zimmerman, 2010). For instance, we demonstrated that diatom communities showed significant responses to dam-related changes, whereas the influence on macroinvertebrate communities was not so clear. The differences between diatoms and macroinvertebrates in terms of body size, life-history strategies, and dispersal capacity may have contributed to their responses to local environmental characteristics and stressors (Grenouillet et al., 2008; Shurin et al., 2009; De Bie et al., 2012). Diatoms have good dispersal abilities and fast population growth rates and, therefore, are considered good bioindicators to track environmental change over large spatial scales (Bennett et al., 2010). Macroinvertebrates, in contrast, are weaker dispersers in comparison to diatoms, thus spatial-related processes may have a greater influence on their community structure than environmental control (Heino, 2013; Padial et al., 2014).
In conclusion, our findings highlighted the main hydrological alterations associated to irrigation dams in mountain rivers and their effects on riverine biota, which varied among biotic groups, mountain ranges, and dams’ operational rules and impoundment capacity. Trait-based analyses allowed us to capture more complex changes in the communities (e.g., life form, body sizes, life history strategies) along the dam impact gradient than the taxonomic approaches. Diatoms were shown to better respond to the presence of dams than macroinvertebrates, which could be linked to their lower spatial dependency. These results contribute to the development of efficient biomonitoring designs and strategies (e.g., selection of suitable biotic groups, location of control and altered sites at adequate spatial scales) and the definition of flow-ecology relationships (e.g., ecological responses to key HIs) as part of riverine conservation strategies (e.g., ELOHA environmental flow framework; Poff et al., 2010).
Data availability statement
The data that support the findings of this study are available from the Spanish Ministry for the Ecological Transition and were used under license for this study. The datasets are available upon reasonable request with the permission of the national authority.
Ethics statement
The manuscript presents research on animals that do not require ethical approval for their study.
Author contributions
CRP: Data curation, Formal Analysis, Investigation, Methodology, Software, Visualization, Writing–original draft, Writing–review and editing. FP: Conceptualization, Funding acquisition, Investigation, Methodology, Project administration, Supervision, Writing–review and editing. JB: Conceptualization, Funding acquisition, Investigation, Methodology, Project administration, Resources, Supervision, Writing–review and editing.
Funding
The author(s) declare that financial support was received for the research, authorship, and/or publication of this article. This project received funding from the European Union’s Horizon 2020 Research and Innovation Programme under Marie Skłodowska-Curie Grant Agreement No. 765553 and from the WATERLANDS Project PID 2019-107085RB-I00, which has been funded by MCIN/AEI/10.13039/501100011033 and by the European Regional Development Fund “ERDF.” This publication is also part of the I + D + I project RIFFLE PID 2020-114427RJ-I00 funded by MCIN/AEI/10.13039/501100011033.
Acknowledgments
We would like to thank the Spanish Ministry for the Ecological Transition for providing the biological monitoring data.
Conflict of interest
The authors declare that the research was conducted in the absence of any commercial or financial relationships that could be construed as a potential conflict of interest.
The author(s) declared that they were an editorial board member of Frontiers, at the time of submission. This had no impact on the peer review process and the final decision.
Publisher’s note
All claims expressed in this article are solely those of the authors and do not necessarily represent those of their affiliated organizations, or those of the publisher, the editors and the reviewers. Any product that may be evaluated in this article, or claim that may be made by its manufacturer, is not guaranteed or endorsed by the publisher.
Supplementary material
The Supplementary Material for this article can be found online at: https://www.frontiersin.org/articles/10.3389/fenvs.2024.1332268/full#supplementary-material
References
Agencia Estatal de Meteorología (2023). Resúmenes climatológicos anuales. España. Available at: https://www.aemet.es/es/serviciosclimaticos/vigilancia_clima/resumenes?w=0&datos=2 (Accessed December 27, 2023).
Alba-Tercedor, J., Jáimez, P., Álvarez, M., Avilés, J., Bonada, N., Casas, J., et al. (2002). Caracterización del estado ecológico de ríos mediterráneos ibéricos mediante el índice IBMWP (antes BMWP’). Limnetica 21, 175–185. doi:10.23818/limn.21.24
Almeida, R. M., Hamilton, S. K., Rosi, E. J., Barros, N., Doria, C. R. C., Flecker, A. S., et al. (2020). Hydropeaking operations of two run-of-river mega-dams alter downstream hydrology of the largest Amazon tributary. Front. Environ. Sci. 8. doi:10.3389/fenvs.2020.00120
Anderson, E. P., Jenkins, C. N., Heilpern, S., Maldonado-Ocampo, J. A., Carvajal-Vallejos, F. M., Encalada, A. C., et al. (2018). Fragmentation of Andes-to-Amazon connectivity by hydropower dams. Sci. Adv. 4, eaao1642. doi:10.1126/sciadv.aao1642
Arrighi, C., Bonamini, I., Simoncini, C., Bartalesi, S., and Castelli, F. (2021). WFD ecological quality indicators are poorly correlated with water levels in river catchments in Tuscany (Italy). Hydrology 8, 185–189. doi:10.3390/hydrology8040185
Arthington, A. H., Bunn, S. E., Poff, N. L. R., and Naiman, R. J. (2006). The challenge of providing environmental flow rules to sustain river ecosystems. Ecol. Appl. 16, 1311–1318. doi:10.1890/1051-0761(2006)016[1311:tcopef]2.0.co;2
Belmar, O., Bruno, D., Martínez-Capel, F., Barquín, J., and Velasco, J. (2013). Effects of flow regime alteration on fluvial habitats and riparian quality in a semiarid Mediterranean basin. Ecol. Indic. 30, 52–64. doi:10.1016/j.ecolind.2013.01.042
Bennett, J. R., Cumming, B. F., Ginn, B. K., and Smol, J. P. (2010). Broad-scale environmental response and niche conservatism in lacustrine diatom communities. Glob. Ecol. Biogeogr. 19, 724–732. doi:10.1111/j.1466-8238.2010.00548.x
Biggs, B. J. F., and Smith, R. A. (2002). Taxonomic richness of stream benthic algae: effects of flood disturbance and nutrients. Limnol. Oceanogr. 47, 1175–1186. doi:10.4319/lo.2002.47.4.1175
Biggs, B. J. F., Stevenson, R. J., and Lowe, R. L. (1998). A habitat matrix conceptual model for stream periphyton. Arch. Hydrobiol. 143, 21–56. doi:10.1127/archiv-hydrobiol/143/1998/21
Birk, S., Bonne, W., Borja, A., Brucet, S., Courrat, A., Poikane, S., et al. (2012). Three hundred ways to assess Europe’s surface waters: an almost complete overview of biological methods to implement the Water Framework Directive. Ecol. Indic. 18, 31–41. doi:10.1016/j.ecolind.2011.10.009
BOE (2015). Real Decreto 817/2015, de 11 de septiembre, por el que se establecen los criterios de seguimiento y evaluación del estado de las aguas superficiales y las normas de calidad ambiental. MAPAMA. 80582–80677.
Bonada, N., and Dolédec, S. (2018). Does the Tachet trait database report voltinism variability of aquatic insects between Mediterranean and Scandinavian regions? Aquat. Sci. 80, 7–11. doi:10.1007/s00027-017-0554-z
Bonada, N., Dolédec, S., and Statzner, B. (2007). Taxonomic and biological trait differences of stream macroinvertebrate communities between mediterranean and temperate regions: implications for future climatic scenarios. Glob. Chang. Biol. 13, 1658–1671. doi:10.1111/j.1365-2486.2007.01375.x
Bonada, N., Prat, N., Resh, V. H., and Statzner, B. (2006). Developments in aquatic insect biomonitoring: a comparative analysis of recent approaches. Annu. Rev. Entomol. 51, 495–523. doi:10.1146/annurev.ento.51.110104.151124
Brown, A. M., Warton, D. I., Andrew, N. R., Binns, M., Cassis, G., and Gibb, H. (2014). The fourth-corner solution - using predictive models to understand how species traits interact with the environment. Methods Ecol. Evol. 5, 344–352. doi:10.1111/2041-210X.12163
Brown, L. E., Aspray, K. L., Ledger, M. E., Mainstone, C., Palmer, S. M., Wilkes, M., et al. (2019). Sediment deposition from eroding peatlands alters headwater invertebrate biodiversity. Glob. Chang. Biol. 25, 602–619. doi:10.1111/gcb.14516
CEN (2012). UNE- EN 16150:2012. Water quality - guidance on pro-rata multi-habitat sampling of benthic macro-invertebrates from wadeable rivers.
CEN (2014a). UNE-EN 14407:2014. Water quality - guidance for the identification and enumeration of benthic diatom samples from rivers and lakes.
CEN (2014b). UNE-EN13946:2014. Water quality - guidance for the routine sampling and preparation of benthic diatoms from rivers and lakes.
Chalise, D. R., Sankarasubramanian, A., and Ruhi, A. (2021). Dams and climate interact to alter river flow regimes across the United States. Earths Future 9. doi:10.1029/2020EF001816
Chandesris, A., Van Looy, K., Diamond, J. S., and Souchon, Y. (2019). Small dams alter thermal regimes of downstream water. Hydrol. Earth Syst. Sci. 23, 4509–4525. doi:10.5194/hess-23-4509-2019
Chessman, B., Williams, S., and Besley, C. (2007). Bioassessment of streams with macroinvertebrates: effect of sampled habitat and taxonomic resolution. J. North Am. Benthol. Soc. 26, 546–565. doi:10.1899/06-074.1
Christmann, T., and Oliveras, I. (2020). Nature of alpine ecosystems in tropical mountains of south America. Encycl. World’s Biomes 1, 282–291. doi:10.1016/b978-0-12-409548-9.12481-9
Consoli, G., Lepori, F., Robinson, C. T., and Bruder, A. (2021). Predicting macroinvertebrate responses to water abstraction in alpine streams. Water (Basel) 13, 2121. doi:10.3390/w13152121
Culp, J. M., Armanini, D. G., Dunbar, M. J., Orlofske, J. M., Poff, N. L., Pollard, A. I., et al. (2011). Incorporating traits in aquatic biomonitoring to enhance causal diagnosis and prediction. Integr. Environ. Assess. Manag. 7, 187–197. doi:10.1002/ieam.128
De Bie, T., De Meester, L., Brendonck, L., Martens, K., Goddeeris, B., Ercken, D., et al. (2012). Body size and dispersal mode as key traits determining metacommunity structure of aquatic organisms. Ecol. Lett. 15, 740–747. doi:10.1111/j.1461-0248.2012.01794.x
Dolédec, S., Chessel, D., ter Braak, C. J. F., and Champely, S. (1996). Matching species traits to environmental variables: a new three-table ordination method. Environ. Ecol. Stat. 3, 143–166. doi:10.1007/BF02427859
Dolédec, S., Olivier, J. M., and Statzner, B. (2000). Accurate description of the abundance of taxa and their biological traits in stream invertebrate communities: effects of taxonomic and spatial resolution. Arch. Hydrobiol. 148, 25–43. doi:10.1127/archiv-hydrobiol/148/2000/25
Dolédec, S., Statzner, B., and Bournard, M. (1999). Species traits for future biomonitoring across ecoregions: patterns along a human-impacted river. Freshw. Biol. 42, 737–758. doi:10.1046/j.1365-2427.1999.00509.x
Dott, C., Bombaci, S., Kasprak, A., and Korb, J. (2022). A tale of two rivers: dam-induced hydrologic drought on the lower Dolores River and its impact on tamarisk establishment. Ecohydrology 15, 1–13. doi:10.1002/eco.2429
Dray, S., Bauman, D., Blanchet, G., Borcard, D., Clappe, S., Guenard, G., et al. (2020). Adespatial: multivariate multiscale spatial analysis.
Dray, S., Choler, P., Dolédec, S., Peres-Neto, P. R., Thuiller, W., Pavoine, S., et al. (2014). Combining the fourth-corner and the RLQ methods for assessing trait responses to environmental variation. Ecology 95, 14–21. doi:10.1890/13-0196.1
Dray, S., and Dufour, A. B. (2007). The ade4 package: implementing the duality diagram for ecologists. J. Stat. Softw. 22, 1–20. doi:10.18637/jss.v022.i04
England, J., Angelopoulos, N., Cooksley, S., Dodd, J., Gill, A., Gilvear, D., et al. (2021). Best practices for monitoring and assessing the ecological response to river restoration. WaterSwitzerl. 13, 3352. doi:10.3390/w13233352
European Commission (2000). Directive 2000/60/EC of the European Parliament and of the Council of 23 October 2000 establishing a framework for Community action in the field of water policy. Official J. Eur. Parliam. L327, 1–82.
Extence, C. A., Balbi, D. M., and Chadd, R. P. (1999). River flow indexing using British benthic macroinvertebrates: a framework for setting hydroecological objectives. Regul. Rivers Res. Manag. 15, 545–574. doi:10.1002/(sici)1099-1646(199911/12)15:6<545::aid-rrr561>3.0.co;2-w
Feeley, H. B., Bradley, C., Free, G., Kennedy, B., Little, R., McDonnell, N., et al. (2020). A national macroinvertebrate dataset collected for the biomonitoring of Ireland’s river network, 2007–2018. Sci. Data 7, 280–289. doi:10.1038/s41597-020-00618-8
Feld, C. K., de Bello, F., and Dolédec, S. (2014). Biodiversity of traits and species both show weak responses to hydromorphological alteration in lowland river macroinvertebrates. Freshw. Biol. 59, 233–248. doi:10.1111/fwb.12260
Fell, S. C., Carrivick, J. L., and Brown, L. E. (2017). The multitrophic effects of climate change and glacier retreat in mountain rivers. Bioscience 67, 897–911. doi:10.1093/biosci/bix107
Friberg, N., Bonada, N., Bradley, D. C., Dunbar, M. J., Edwards, F. K., Grey, J., et al. (2011). Biomonitoring of human impacts in freshwater ecosystems: the good, the bad and the ugly. Adv. Ecol. Res. 44, 1–68. doi:10.1016/B978-0-12-374794-5.00001-8
García de Jalón, S., González del Tánago, M., and García de Jalón, D. (2019). A new approach for assessing natural patterns of flow variability and hydrological alterations: the case of the Spanish rivers. J. Environ. Manage 233, 200–210. doi:10.1016/j.jenvman.2018.12.049
García-Roger, E. M., Sánchez-Montoya, M., Cid, N., Erba, S., Karaouzas, I., Verkaik, I., et al. (2014). Spatial scale effects on taxonomic and biological trait diversity of aquatic macroinvertebrates in Mediterranean streams. Fundam. Appl. Limnol. 183, 89–105. doi:10.1127/1863-9135/2013/0429
Gayraud, S., Statzner, B., Bady, P., Haybachp, A., Scholl, F., Usseglio-Polatera, P., et al. (2003). Invertebrate traits for the biomonitoring of large European rivers: an initial assessment of alternative metrics. Freshw. Biol. 48, 2045–2064. doi:10.1046/j.1365-2427.2003.01139.x
Goldenberg-Vilar, A., Delgado, C., Peñas, F. J., and Barquín, J. (2021). The effect of altered flow regimes on aquatic primary producer communities: diatoms and macrophytes. Ecohydrology 15. 0–3. doi:10.1002/eco.2353
Grabherr, G., Gottfried, M., and Pauli, H. (2001). High Mountain environment as indicator of global change. 331–345. doi:10.1007/0-306-48051-4_32
Grenouillet, G., Brosse, S., Tudesque, L., Lek, S., Baraillé, Y., and Loot, G. (2008). Concordance among stream assemblages and spatial autocorrelation along a fragmented gradient. Divers Distrib. 14, 592–603. doi:10.1111/j.1472-4642.2007.00443.x
Grill, G., Lehner, B., Thieme, M., Geenen, B., Tickner, D., Antonelli, F., et al. (2019). Mapping the world’s free-flowing rivers. Nature 569, 215–221. doi:10.1038/s41586-019-1111-9
Heino, J. (2013). Does dispersal ability affect the relative importance of environmental control and spatial structuring of littoral macroinvertebrate communities? Oecologia 171, 971–980. doi:10.1007/s00442-012-2451-4
Heino, J., and Soininen, J. (2007). Are higher taxa adequate surrogates for species-level assemblage patterns and species richness in stream organisms? Biol. Conserv. 137, 78–89. doi:10.1016/j.biocon.2007.01.017
Heß, S., Hof, D., Oetken, M., and Sundermann, A. (2023). Effects of multiple stressors on benthic invertebrates using Water Framework Directive monitoring data. Sci. Total Environ. 878, 162952. doi:10.1016/j.scitotenv.2023.162952
Immerzeel, W. W., Lutz, A. F., Andrade, M., Bahl, A., Biemans, H., Bolch, T., et al. (2020). Importance and vulnerability of the world’s water towers. Nature 577, 364–369. doi:10.1038/s41586-019-1822-y
Jackson, J. K., and Füreder, L. (2006). Long-term studies of freshwater macroinvertebrates: a review of the frequency, duration and ecological significance. Freshw. Biol. 51, 591–603. doi:10.1111/j.1365-2427.2006.01503.x
Jiang, X., Xiong, J., Song, Z., Morse, J. C., Jones, F. C., and Xie, Z. (2013). Is coarse taxonomy sufficient for detecting macroinvertebrate patterns in floodplain lakes? Ecol. Indic. 27, 48–55. doi:10.1016/j.ecolind.2012.11.015
Karaouzas, I., Theodoropoulos, C., Vourka, A., Gritzalis, K., and Skoulikidis, N. T. (2019). Stream invertebrate communities are primarily shaped by hydrological factors and ultimately fine-tuned by local habitat conditions. Sci. Total Environ. 665, 290–299. doi:10.1016/j.scitotenv.2019.02.134
Kelly-Quinn, M., Feeley, H., and Bradley, C. (2020). Status of freshwater invertebrate biodiversity in Ireland’s rivers – time to take stock. Biol. Environ. 120B, 65–82. doi:10.3318/bioe.2020.09
Kennedy, T. L., and Turner, T. F. (2011). River channelization reduces nutrient flow and macroinvertebrate diversity at the aquatic terrestrial transition zone. Ecosphere 2, art35–13. doi:10.1890/ES11-00047.1
Krajenbrink, H. J., Acreman, M., Dunbar, M. J., Greenway, L., Hannah, D. M., Laizé, C. L. R., et al. (2019). Diatoms as indicators of the effects of river impoundment at multiple spatial scales. PeerJ 2019, e8092. doi:10.7717/peerj.8092
Krajenbrink, H. J., White, J. C., Dunbar, M. J., and Wood, P. J. (2022). Macroinvertebrate and diatom community responses to thermal alterations below water supply reservoirs. River Res. Appl. 38, 595–612. doi:10.1002/rra.3922
Ladrera, R., and Prat, N. (2013). Changes in macroinvertebrate community and biotic indices associated with streamflow regulation and wastewater inputs in Sierra Cebollera Natural Park (La Rioja, Northern Spain). Limnetica 32, 353–372. doi:10.23818/limn.32.27
Laini, A., Bolpagni, R., Cancellario, T., Guareschi, S., Racchetti, E., and Viaroli, P. (2018). Testing the response of macroinvertebrate communities and biomonitoring indices under multiple stressors in a lowland regulated river. Ecol. Indic. 90, 47–53. doi:10.1016/j.ecolind.2018.02.051
Laini, A., Burgazzi, G., Chadd, R., England, J., Tziortzis, I., Ventrucci, M., et al. (2022). Using invertebrate functional traits to improve flow variability assessment within European rivers. Sci. Total Environ. 832, 155047. doi:10.1016/j.scitotenv.2022.155047
Laini, A., Viaroli, P., Bolpagni, R., Cancellario, T., Racchetti, E., and Guareschi, S. (2019). Taxonomic and functional responses of benthic macroinvertebrate communities to hydrological and water quality variations in a heavily regulated river. Water (Basel) 11, 1478. doi:10.3390/w11071478
Larsen, S., Majone, B., Zulian, P., Stella, E., Bellin, A., Bruno, M. C., et al. (2021). Combining hydrologic simulations and stream-network models to reveal flow-ecology relationships in a large alpine catchment. Water Resour. Res. 57. doi:10.1029/2020WR028496
Lavoie, I., Dillon, P. J., and Campeau, S. (2009). The effect of excluding diatom taxa and reducing taxonomic resolution on multivariate analyses and stream bioassessment. Ecol. Indic. 9, 213–225. doi:10.1016/j.ecolind.2008.04.003
Lessard, J. L., and Hayes, D. B. (2003). Effects of elevated water temperature on fish and macroinvertebrate communities below small dams. River Res. Appl. 19, 721–732. doi:10.1002/rra.713
Li, D., Lu, X., Walling, D. E., Zhang, T., Steiner, J. F., Wasson, R. J., et al. (2022). High Mountain Asia hydropower systems threatened by climate-driven landscape instability. Nat. Geosci. 15, 520–530. doi:10.1038/s41561-022-00953-y
López Moreno, J. I., Beguería, S., and García-Ruiz, J. M. (2016). Efecto de los embalses en el control de las avenidas en el Pirineo Central Español. Geographicalia 57, 57. doi:10.26754/ojs_geoph/geoph.2003441351
López-Moreno, J. I., Beniston, M., and García-Ruiz, J. M. (2008). Environmental change and water management in the Pyrenees: facts and future perspectives for Mediterranean mountains. Glob. Planet Change 61, 300–312. doi:10.1016/j.gloplacha.2007.10.004
Maavara, T., Chen, Q., Van Meter, K., Brown, L. E., Zhang, J., Ni, J., et al. (2020). River dam impacts on biogeochemical cycling. Nat. Rev. Earth Environ. 1, 103–116. doi:10.1038/s43017-019-0019-0
Marnezy, A. (2008). Alpine dams: from hydroelectric power to artificial snow. Rev. Geogr. Alp., 103–112. doi:10.4000/rga.430
McManamay, R. A., Bevelhimer, M. S., and Frimpong, E. A. (2015). Associations among hydrologic classifications and fish traits to support environmental flow standards. Ecohydrology 8, 460–479. doi:10.1002/eco.1517
McManamay, R. A., Oigbokie, C. O., Kao, S.-C., and Bevelhimer, M. S. (2016). Classification of US hydropower dams by their modes of operation. River Res. Appl. 32, 1450–1468. doi:10.1002/rra.3004
Mellado-Díaz, A., Sánchez-González, J. R., Guareschi, S., Magdaleno, F., and Toro Velasco, M. (2019). Exploring longitudinal trends and recovery gradients in macroinvertebrate communities and biomonitoring tools along regulated rivers. Sci. Total Environ. 695, 133774. doi:10.1016/j.scitotenv.2019.133774
Menezes, S., Baird, D. J., and Soares, A. M. V. M. (2010). Beyond taxonomy: a review of macroinvertebrate trait-based community descriptors as tools for freshwater biomonitoring. J. Appl. Ecol. 47, 711–719. doi:10.1111/j.1365-2664.2010.01819.x
Ministerio de Agricultura, Alimentación y Medio Ambiente (2013b). Protocolo de muestreo y laboratorio de fauna bentónica de invertebrados en ríos vadeables.
Ministerio de Agricultura, Alimentación y Medio Ambiente (2013a). Protocolo de cálculo del Índice de Polusensibilidad Específica.
Monk, W. A., Wood, P. J., Hannah, D. M., Extence, C. A., Chadd, R. P., and Dunbar, M. J. (2012). How does macroinvertebrate taxonomic resolution influence ecohydrological relationships in riverine ecosystems. Ecohydrology 5, 36–45. doi:10.1002/eco.192
Moore, J. W., Hayes, S. A., Duffy, W., Gallagher, S., Michel, C. J., and Wright, D. (2011). Nutrient fluxes and the recent collapse of coastal California salmon populations. Can. J. Fish. Aquatic Sci. 68, 1161–1170. doi:10.1139/f2011-054
Moyle, P. B., and Mount, J. F. (2007). Homogenous rivers, homogenous faunas. Proc. Natl. Acad. Sci. U. S. A. 104, 5711–5712. doi:10.1073/pnas.0701457104
Munné, A., and Prat, N. (2011). Effects of Mediterranean climate annual variability on stream biological quality assessment using macroinvertebrate communities. Ecol. Indic. 11, 651–662. doi:10.1016/j.ecolind.2010.09.004
Murphy, J. F., Jones, J. I., Arnold, A., Duerdoth, C. P., Pretty, J. L., Naden, P. S., et al. (2017). Can macroinvertebrate biological traits indicate fine-grained sediment conditions in streams? River Res. Appl. 33, 1606–1617. doi:10.1002/rra.3194
Oksanen, J., Blanchet, F. G., Friendly, M., Kindt, R., Legendre, P., Dan McGlinn, P., et al. (2019). Vegan: community ecology package.
Olden, J. D., and Naiman, R. J. (2010). Incorporating thermal regimes into environmental flows assessments: modifying dam operations to restore freshwater ecosystem integrity. Freshw. Biol. 55, 86–107. doi:10.1111/j.1365-2427.2009.02179.x
Olden, J. D., and Poff, N. L. (2003). Redundancy and the choice of hydrologic indices for characterizing streamflow regimes. River Res. Appl. 19, 101–121. doi:10.1002/rra.700
Paavola, R., Muotka, T., Virtanen, R., Heino, J., and Kreivi, P. (2003). Are biological classifications of headwater streams concordant across multiple taxonomic groups? Freshw. Biol. 48, 1912–1923. doi:10.1046/j.1365-2427.2003.01131.x
Padial, A. A., Ceschin, F., Declerck, S. A. J., De Meester, L., Bonecker, C. C., Lansac-Tôha, F. A., et al. (2014). Dispersal ability determines the role of environmental, spatial and temporal drivers of metacommunity structure. PLoS One 9, e111227–e111228. doi:10.1371/journal.pone.0111227
Pang, A., Li, C., Sun, T., Yang, W., and Yang, Z. (2018). Trade-off analysis to determine environmental flows in a highly regulated watershed. Sci. Rep. 8, 14130–14211. doi:10.1038/s41598-018-32126-6
Passy, S. I. (2007). Diatom ecological guilds display distinct and predictable behavior along nutrient and disturbance gradients in running waters. Aquat. Bot. 86, 171–178. doi:10.1016/j.aquabot.2006.09.018
Peñas, F. J., and Barquín, J. (2019). Assessment of large-scale patterns of hydrological alteration caused by dams. J. Hydrol. (Amst) 572, 706–718. doi:10.1016/j.jhydrol.2019.03.056
Peñas, F. J., Barquín, J., and Álvarez, C. (2016). Assessing hydrologic alteration: Evaluation of different alternatives according to data availability. Ecol. Indic. 60, 470–482. doi:10.1016/j.ecolind.2015.07.021
Pepin, N., Bradley, R. S., Diaz, H. F., Baraer, M., Caceres, E. B., Forsythe, N., et al. (2015). Elevation-dependent warming in mountain regions of the world. Nat. Clim. Chang. 5, 424–430. doi:10.1038/nclimate2563
Piano, E., Doretto, A., Mammola, S., Falasco, E., Fenoglio, S., and Bona, F. (2020). Taxonomic and functional homogenisation of macroinvertebrate communities in recently intermittent Alpine watercourses. Freshw. Biol. 65, 2096–2107. doi:10.1111/fwb.13605
Poff, N. L., and Allan, J. D. (1995). Functional organization of stream fish assemblages in relation to hydrological variability. Ecology 76, 606–627. doi:10.2307/1941217
Poff, N. L., Richter, B. D., Arthington, A. H., Bunn, S. E., Naiman, R. J., Kendy, E., et al. (2010). The ecological limits of hydrologic alteration (ELOHA): a new framework for developing regional environmental flow standards. Freshw. Biol. 55, 147–170. doi:10.1111/j.1365-2427.2009.02204.x
Poff, N. L., and Zimmerman, J. K. H. (2010). Ecological responses to altered flow regimes: a literature review to inform the science and management of environmental flows. Freshw. Biol. 55, 194–205. doi:10.1111/j.1365-2427.2009.02272.x
Pompeu, C. R., Peñas, F. J., and Barquín, J. (2022a). Large-scale spatial patterns of riverine communities: niche versus geographical distance. Biodivers. Conserv. 32, 589–607. doi:10.1007/s10531-022-02514-6
Pompeu, C. R., Peñas, F. J., Belmar, O., and Barquín, J. (2023). Large-scale factors controlling biological communities in the Iberian Peninsula: an insight into global change effects on river ecosystems. Aquat. Sci. 85, 95. doi:10.1007/s00027-023-00995-3
Pompeu, C. R., Peñas, F. J., Goldenberg-Vilar, A., Álvarez-Cabria, M., and Barquín, J. (2022b). Assessing the effects of irrigation and hydropower dams on river communities using taxonomic and multiple trait-based approaches. Ecol. Indic. 145, 109662. doi:10.1016/j.ecolind.2022.109662
Prats, J., Armengol, J., Marcé, R., Sánchez-Juny, M., and Dolz, J. (2010). “Dams and reservoirs in the lower Ebro river and its effects on the River thermal cycle,” 77–95. doi:10.1007/698_2010_68
Quevedo, L., Ibáñez, C., Caiola, N., Cid, N., and Hampel, H. (2018). Impact of a reservoir system on benthic macroinvertebrate and diatom communities of a large Mediterranean river (Lower Ebro river, Catalonia, Spain). Limnetica 37, 209–228. doi:10.23818/limn.37.18
Quinn, G. P., and Keough, M. J. (2002). Experimental design and data analysis for biologists. Cambridge: Cambridge University Press.
Rahbek, C., Borregaard, M. K., Colwell, R. K., Dalsgaard, B., Holt, B. G., Morueta-Holme, N., et al. (2019). Humboldt’s enigma: what causes global patterns of mountain biodiversity? Science 365, 1108–1113. doi:10.1126/science.aax0149
Resh, V. H. (2008). Which group is best? Attributes of different biological assemblages used in freshwater biomonitoring programs. Environ. Monit. Assess. 138, 131–138. doi:10.1007/s10661-007-9749-4
Rimet, F., and Bouchez, A. (2012). Life-forms, cell-sizes and ecological guilds of diatoms in European rivers. Knowl. Manag. Aquat. Ecosyst. 1, 01. doi:10.1051/kmae/2012018
Schmidt-Kloiber, A., and Hering, D. (2015). www.freshwaterecology.info – an online tool that unifies, standardises and codifies more than 20,000 European freshwater organisms and their ecological preferences. Ecol. Indic. 53, 271–282. doi:10.1016/j.ecolind.2015.02.007
Shurin, J. B., Cottenie, K., and Hillebrand, H. (2009). Spatial autocorrelation and dispersal limitation in freshwater organisms. Oecologia 159, 151–159. doi:10.1007/s00442-008-1174-z
Smolar-Žvanut, N., and Mikoš, M. (2014). The impact of flow regulation by hydropower dams on the periphyton community in the Soča River, Slovenia. Hydrological Sci. J. 59, 1032–1045. doi:10.1080/02626667.2013.834339
Soininen, J., Jamoneau, A., Rosebery, J., and Passy, S. I. (2016). Global patterns and drivers of species and trait composition in diatoms. Glob. Ecol. Biogeogr. 25, 940–950. doi:10.1111/geb.12452
Spitale, D., Angeli, N., Lencioni, V., Tolotti, M., and Cantonati, M. (2015). Comparison between natural and impacted Alpine lakes six years after hydropower exploitation has ceased. Biol. Bratisl. 70, 1597–1605. doi:10.1515/biolog-2015-0185
Statzner, B., and Bêche, L. A. (2010). Can biological invertebrate traits resolve effects of multiple stressors on running water ecosystems? Freshw. Biol. 55, 80–119. doi:10.1111/j.1365-2427.2009.02369.x
Sturbois, A., Cormy, G., Le Moal, A., Schaal, G., Broudin, C., Thiébaut, E., et al. (2021). Using ecological trajectories to track long-term taxonomic and functional changes in benthic shallow soft-bottom communities (Bay of Saint-Brieuc, English Channel). Aquat. Conserv. 31, 3013–3033. doi:10.1002/aqc.3704
Tachet, H., Richoux, P., Bournard, M., and Usseglio-Polatera, P. (2010). Invertébrés d’Eau Douce - systématique, Biologie, Écologie. Paris: CNRS.
Ter Braak, C. J. F., Cormont, A., and Dray, S. (2012). Improved testing of species traits–environment relationships in the fourth-corner problem. Ecology 93, 1525–1526. doi:10.1890/12-0126.1
Thioulouse, J., Dufour, A. B., Jombart, T., Dray, S., Siberchicot, A., and Pavoine, S. (2018). Multivariate analysis of ecological data with ade4. New York: Springer-Verlag. doi:10.1007/978-1-4939-8850-1
Tornés, E., Acuña, V., Dahm, C. N., and Sabater, S. (2015). Flood disturbance effects on benthic diatom assemblage structure in a semiarid river network. J. Phycol. 51, 133–143. doi:10.1111/jpy.12260
Troia, M. J., and McManamay, R. A. (2019). Biogeographic classification of streams using fish community– and trait–environment relationships. Divers Distrib. 26, 108–125. doi:10.1111/ddi.13001
Vaughan, I. P., and Ormerod, S. J. (2010). Linking ecological and hydromorphological data: approaches, challenges and future prospects for riverine science. Aquat. Conserv. 20, 125–130. doi:10.1002/aqc.1104
Vaughan, I. P., and Ormerod, S. J. (2012). Large-scale, long-term trends in British river macroinvertebrates. Glob. Chang. Biol. 18, 2184–2194. doi:10.1111/j.1365-2486.2012.02662.x
Vilmin, L., Flipo, N., Escoffier, N., and Groleau, A. (2018). Estimation of the water quality of a large urbanized river as defined by the European WFD: what is the optimal sampling frequency? Environ. Sci. Pollut. Res. 25, 23485–23501. doi:10.1007/s11356-016-7109-z
Viviroli, D., Kummu, M., Meybeck, M., Kallio, M., and Wada, Y. (2020). Increasing dependence of lowland populations on mountain water resources. Nat. Sustain 3, 917–928. doi:10.1038/s41893-020-0559-9
Viviroli, D., and Weingartner, R. (2008). “Water towers”—a global view of the hydrological importance of mountains. Adv. Glob. Change Res. 31, 15–20. doi:10.1007/978-1-4020-6748-8_2
Wagenhoff, A., Liess, A., Pastor, A., Clapcott, J. E., Goodwin, E. O., and Young, R. G. (2017). Thresholds in ecosystem structural and functional responses to agricultural stressors can inform limit setting in streams. Freshw. Sci. 36, 178–194. doi:10.1086/690233
White, J. C., Hannah, D. M., House, A., Beatson, S. J. V., Martin, A., and Wood, P. J. (2017). Macroinvertebrate responses to flow and stream temperature variability across regulated and non-regulated rivers. Ecohydrology 10, 1–21. doi:10.1002/eco.1773
Wohl, E. (2006). Human impacts to mountain streams. Geomorphology 79, 217–248. doi:10.1016/j.geomorph.2006.06.020
Worrall, T. P., Dunbar, M. J., Extence, C. A., Laizé, C. L. R., Monk, W. A., and Wood, P. J. (2014). The identification of hydrological indices for the characterization of macroinvertebrate community response to flow regime variability. Hydrological Sci. J. 59, 645–658. doi:10.1080/02626667.2013.825722
Wu, H., Chen, J., Xu, J., Zeng, G., Sang, L., Liu, Q., et al. (2019a). Effects of dam construction on biodiversity: a review. J. Clean. Prod. 221, 480–489. doi:10.1016/j.jclepro.2019.03.001
Wu, N., Thodsen, H., Andersen, H. E., Tornbjerg, H., Baattrup-Pedersen, A., and Riis, T. (2019b). Flow regimes filter species traits of benthic diatom communities and modify the functional features of lowland streams - a nationwide scale study. Sci. Total Environ. 651, 357–366. doi:10.1016/j.scitotenv.2018.09.210
Keywords: freshwater biota, flow regime, irrigation dams, river regulation, diatoms, benthic macroinvertebrates
Citation: Rocha Pompeu C, Peñas FJ and Barquín J (2024) Effects of irrigation dams on riverine biota in mountain streams. Front. Environ. Sci. 12:1332268. doi: 10.3389/fenvs.2024.1332268
Received: 02 November 2023; Accepted: 01 March 2024;
Published: 27 March 2024.
Edited by:
Alan Steinman, Annis Water Resources Institute, United StatesReviewed by:
Jordi-René Mor, National Research Council (CNR), ItalyXianfu Zhao, Ministry of Water Resources and Chinese Academy of Sciences, China
Copyright © 2024 Rocha Pompeu, Peñas and Barquín. This is an open-access article distributed under the terms of the Creative Commons Attribution License (CC BY). The use, distribution or reproduction in other forums is permitted, provided the original author(s) and the copyright owner(s) are credited and that the original publication in this journal is cited, in accordance with accepted academic practice. No use, distribution or reproduction is permitted which does not comply with these terms.
*Correspondence: Cássia Rocha Pompeu, cassia.rocha@alumnos.unican.es