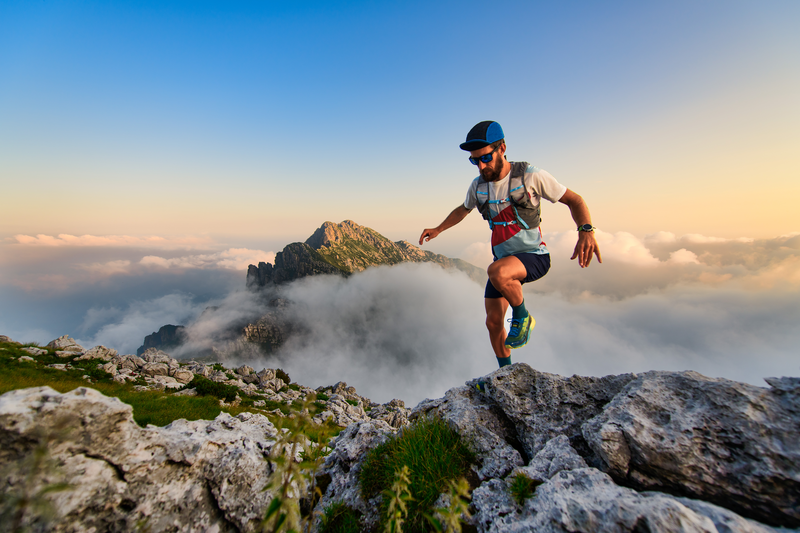
95% of researchers rate our articles as excellent or good
Learn more about the work of our research integrity team to safeguard the quality of each article we publish.
Find out more
ORIGINAL RESEARCH article
Front. Environ. Sci. , 24 October 2024
Sec. Toxicology, Pollution and the Environment
Volume 12 - 2024 | https://doi.org/10.3389/fenvs.2024.1326517
This article is part of the Research Topic Atmospheric Aerosol and Pollution: Characterization Techniques and Source Identification View all 7 articles
Introduction: Indoor air pollution occurs in a microenvironment such as residential homes, closed working places, or industries in which people spend a large part of their time. Cooking or baking food in traditional or open three-stone cookstoves by using firewood is the cause for kitchen-related smoke indoor air pollution and deforestation. Modifying traditional firewood cookstoves could reduce the emissions of indoor air pollutants. The objective of the present study was to determine the effect of firewood cookstove types on indoor air pollution, emission reduction, and deforestation in selected rural houses of Bure, Ameya, and Dalocha woredas (districts) of Ethiopia.
Methods: Indoor air pollutants such as carbonmonoxide (CO), carbon dioxide (CO2), sulfurdioxide (SO2), and particulate matters (PMs) were measured in three replicates for 3 min. within an average cooking time of 1 h using an automatic gas sensor. The amount of wood was separately measured by using graduated weight balance (0.1 gm).
Results and discussion: The cooking place was mostly the same room as the sleeping place without a separate kitchen. The improved cookstoves were installed without a chimney (smokeoutlet) and with wider spaces left for a firewood inlet. In improved Injera baking cookstoves, the mean emission of CO was 1004.80 mg/m3, 33.00 mg/m3, and 53.85 mg/m3 in Bure, Ameya, and Dalocha woredas, respectively, which were lower than the emissions from open cookstoves. The mean concentration of PM2.5 in open cookstoves in Bure, Ameya, and Dalocha woredaswas 124.50 mg/m3, 0.53 mg/m3, and 0.04 mg/m3, respectively, which are higher than the emission from improved stoves. In Bure woreda households, the CO exposure was above 26 times the permissible limit of WHO standards. Biomass consumption was positively correlated with the moisture content of wood (p < 0.05). Improved cookstoves reduced indoor air pollution by 13%–81% and carbon emission and deforestation by 20%–41% when compared with the open three-stone cookstoves. Indoor air pollutants are risks to health and cause climate change problems. Therefore, awareness should be created on the health effects of firewood smoke indoor air pollution, ventilation of cooking places, and installation of chimneys with improved cookstoves.
Indoor air pollution (IAP) occurs in a microenvironment such as residential homes, closed working places, or industries in which people spend a large part of their time. Indoor air pollutions are caused by exposure to pollutants generated outdoors that penetrate the indoor environment and also by pollutants produced indoors, for example, as a result of space heating, cooking, and other indoor activities or emitted from products used indoors (WHO, 2010). Human beings are exposed to air pollutants both outdoors and indoors in the absence of a continuous supply of free and acceptable-quality air, approximately 10–20 m3 per day. While air pollution occurs in an outdoor urban environment due to vehicle transport, industrial emission, waste disposal, rural areas also face smoke air pollutants emitted from burning solid fuel in open stoves (Theuri, 2009). Since people generally spend 80%–90% of their time indoors, the pollution in indoor air is more severe than that outdoors, where there is a high rate of indoor emission such as firewood smoke (Huang et al., 2022; Yang et al., 2021). Indoor air pollution in residential houses can be caused by the smoke due to the incomplete combustion of solid biomass fuels, such as charcoal, wood, crop residues, and animal dung inside rooms without a chimney (smoke outlet from the cooking area) (WHO, 2011; Huboyo et al., 2014). The combustion of solid biomass fuels in unventilated and narrow homes emits harmful smoke as IAP that causes serious injuries from scalding, burns, and poisoning and creates over 65,000 premature deaths and more than 3.1 million disability-adjusted life–years per year (Sanbata et al., 2014; WHO, 2018). Therefore, kitchen-related indoor air pollution is a major environmental and health hazard (WHO, 2018). The World Bank reported that indoor air pollution is one of the most critical environmental problems in developing countries (Carter, 1998; Mac, 2009). Annually, 0.5 million deaths were estimated in the world from outdoor pollution, while 2.2–4.0 million deaths were estimated from indoor air pollution (GACCS, 2014). The emissions contain many of the toxins found in tobacco smoke caused by incomplete combustion (Smith et al., 2000; Alemayehu, 2011) including coarse, fine, and ultrafine particulate matter (PM); carbon monoxide (CO); oxides of nitrogen and sulfur; methane (CH4); fluorinated gases; tropospheric ozone; soot; black carbon; volatile organic compounds; aromatic hydrocarbons; and polycyclic aromatic hydrocarbons (PAHs) such as benzo[a]pyrene, formaldehyde, bio-aerosols, oxygenated organics, hydrocarbons, chlorinated organics, transition metals, free radicals, and butadiene that pose health hazards (Smith et al., 2000; Naeher et al., 2007; Alemayehu, 2011; Lin et al., 2016; WHO, 2016). The particulate matter component of indoor pollutant smoke is classified according to its size, with inhalable material <2.5 μm and <10 μm in aerodynamic diameter referred to as PM2.5 and PM10, respectively. Although both PM10 and PM2.5 cause serious health impacts, particles in air with 2.5 μm of diameter or smaller (PM2.5) are better predictors of health effects than particles up to 10 μm in diameter (PM10) (Wang et al., 2023a). Generally, smoke from wood burning contains 17 substances designated as priority pollutants by USEPA (2002) because of their toxicity observed in animal studies, up to 14 carcinogenic compounds, 6 cilia-toxic and mucus-coagulating agents, and 4 co-carcinogenic or cancer-promoting agents (Smith and Liu, 1994). These gases and particulate matters with short life span contribute to climate change (USDOE, 2011). The use of improved cookstoves with chimneys can reduce indoor air pollution by 70% (Perez-Padilla et al., 2010) and wastage of wood by up to 54% of the wood when compared with three-point open-fire cookstoves (Kedir et al., 2019; Adane et al., 2021; Phillip et al., 2023). Since solid fuels are cheaper than gas and electricity, they are used more frequently in poor urban and rural households and are probably the main source of indoor air pollution (Schwela, 1997). The number of people in the family who are more exposed to indoor air pollution is greater in the developing countries because the indoor pollutants account for 76% of the global particulate matter-related indoor air pollution (Smith et al., 2005).
The lack of ventilation in homes that use biomass fuels and the poor design of stoves that do not have chimneys to expel smoke out of the living area exacerbate the adverse health effects of indoor air pollution generated by products of incomplete combustion (Naeher et al., 2007; Lin et al., 2016; Du et al., 2017). The amount of indoor air pollutants also varies with the type of the fuel used and among biomass fuels. According to Smith et al. (2005), among the biomass energy sources, compared with liquid petroleum gas (LPG), the highest amount of particulate matter is emitted by crop residues, and the lowest from biogas. Dung emits the greatest amount of carbon monoxide.
The air pollutant gases emitted during fuel combustion, such as CO, carbon dioxide (CO2), sulfur dioxide (SO2), and PM, are harmful because of their characteristics. CO is a colorless, odorless, tasteless, and poisonous gas created due to the incomplete combustion of carbonaceous fuels such as wood, petrol, coal, natural gas, propane, gasoline, and kerosene. Other potential CO sources are cloth dryers (gas), water heaters, furnaces and boilers, fireplaces, gas stoves, cars, grills, generators, and power tools. It is only slightly soluble in water, blood serum, and plasma. Hazardous concentrations of CO can accumulate indoors and cause illness before the realization of being poisoned. Symptoms of CO poisoning are very similar to those of flu, which may cause us to ignore its early signs including headache, breathlessness with mild exercise, dizziness, fatigue, and nausea. The symptoms may grow to confusion and irritability, impaired judgment and loss of coordination, and unconsciousness. All exposed people can become sick and die from CO poisoning when exposed to very high levels. However, CO poisoning can be prevented by installing a CO detector in homes and going outside whenever the symptoms or signs are felt (DoH, 2017).
CO2 is a colorless and odorless gas. It is produced both naturally and through human activities, such as burning gasoline, coal, oil, and wood. The outdoor concentration of CO2 can vary from 629.86 to 719.84 mg/m3 or higher. The indoor CO2 level depends on the number of people present, the duration of time for which an area is occupied, the amount of outdoor fresh air entering the area, the size of the room, and presence of contaminants in the indoor air. The indoor CO2 concentration varies from several hundred mg/m3 to over 1,000 mg/m3 (https://www.health.state.mn.us/communities/environment/air/toxins/co2.html).
SO2 is another colorless gas with a sharp odor and is non-combustible, toxic, and readily soluble in water. Sulfur dioxide is produced by fuel combustion (especially high-sulfur coal); electric utilities and industrial processes; and natural sources such as volcanoes (WHO, 2018).
PMs are a generic class of contaminants that contain solid or liquid particles suspended in the air independently of the composition (Bell and Samet, 2005). The particles are derived from various sources including traffic, biomass burning, waste incineration, and industrial processes (Schwarze et al., 2006). These airborne particles vary in size and composition, including gaseous pollutants, sulfate and nitrate ions, condensed acid, soil dust, soot, ash, mold, and pollen (Kajekar, 2007). The aerodynamic properties of particles determine how the particles are transported and removed from the air, their deposition in the respiratory system, and their chemical composition and source (Tasic et al., 2006). PMs are formed through chemical reactions; fuel combustion (e.g., burning coal, wood, and diesel); industrial processes; agriculture (plowing and field burning); and unpaved roads.
The most commonly used fuel is solid biomass that accounted for approximately 86.00% of the total energy supply of Ethiopia in 2020 (MoWE, 2023) because only 48.30% of the people had access to electricity (WB, 2019). In 2013, the total biomass consumption of Ethiopia was 131 million tons, of which wood, charcoal, crop residue, and dung accounted for 68%, 3.20%, 14.10%, and 14.80%, respectively, of the total consumption (Geissler et al., 2013).
In Ethiopia, the excessive use of firewood in open cookstoves has caused deforestation (Maddox, 2006) because of the low combustion efficiency. The cutting of trees without reforestation has resulted in habitat damage, biodiversity loss, aridity, and degradation (Gessesse, and Carl, 2008). Deforestation in Ethiopia has been leading to the extinction of plant and animal species, changes in climatic conditions, desertification, and displacement of indigenous people. The deforestation rate in Ethiopia in 2005–2010 was 141,000 ha per year. The burning of forest land releases tons of CO2, which contributes to global warming. Deforestation of tropical areas creates one-third of the total anthropogenic carbon dioxide emissions (Tsegaye, 2007). Deforestation reduces soil cohesion, which results in erosion, flooding, and landslides. Burning of dung as a fuel instead of using it as a soil conditioner has led to a reduction of 550,000 tons of grain production per year (FAO, 2019).
Hence, understanding the emission levels of smoke (indoor pollutant) allows us to propose solutions that reduce indoor air pollution, such as improved stoves, modifying cooking rooms, and using the appropriate type of fuel. Emission measurements for household cookstoves have been conducted since the early 1990s; however, there are few field emission measurements from the cookstoves used. Therefore, less attention was given to the research and development of indoor air pollution. Most of the emission measurements are laboratory-based and generalized over large geographical regions, although over 16.68 million fuel-saving biomass stoves were disseminated throughout Ethiopia in the past 10 years (2010/11–2020/21) (MoWE, 2023). Accordingly, the present study was conducted in areas where improved cookstoves were widely distributed in order to determine the emission levels, and deforestation reduced with use of improved stoves compared to three-stone open cookstoves in Bure, Ameya, and Dalocha woredas (districts) of Ethiopia.
The materials used were the GPS, weight balance, moisture meter, questionnaire, and automatic and portable gas sensor emission meters. Moreover, local guides who know the locality very well were hired to show different houses with open and improved cookstoves.
The study was conducted in Bure (Amhara Region), Ameya (Oromia Region), and Dalocha woredas (Southern Nations, Nationalities, and Peoples’ Region, SNNPR) (Figure 1). The geophysical condition shows that the annual temperature ranges from 12°C to 32°C, and annual precipitation ranges from 550 to 1,750 mm (Table 1). The stoves used were Mirt and open three-stone cookstoves in Bure and Ameya woredas and improved Gonzie and open three-stone cookstoves in the Dalocha woreda. Bure is located in Northern Ethiopia at 700–2,750 m and Ameya in Central Ethiopia at 1,946–2,026 m above sea level (Table 1).
The improved cookstoves, i.e., Mirt and improved Gonzie, have specific materials and dimensions (GIZ ECO, 2014). The Mirt stove is produced with a mortar using a mixture of scoria (red ash) or pumice or river sand with cement. It serves for more than 5 years. The Mirt stove has six parts that are joined together, four parts fit to make a cylindrical-shaped enclosure and two other parts joined one on top of the other and are fitted with the cylindrical enclosure from behind (Figure 2). The other two parts serve as a smoke outlet within the cooking places and rest of the cooking pot. The improved Gonzie stove is made from clay. It has four parts, which form its basic circular enclosure when assembled together (GIZ ECO, 2014). The major change shown in improved stoves is closing the circular area forming the combustion chamber, where plates are placed at the top. Open three-stone stoves are made from stone or mud, and they are placed in circular points in such a way that the cooking plate is placed on top of each, and the three points are placed at equal open space (Figure 2). The size or diameter of the combustion chamber of improved stoves is determined by the size of the plate and is usually 50–60 cm (Kedir et al., 2019), but the size or diameter of the traditional open three-stone stoves depends on the diameter of the cooking plate and has no standard size of the combustion chamber, and it is open.
Figure 2. Mirt stove (top left); improved Gonzie stove (top right); and open three-stone (point) stoves (bottom).
The portable air-quality monitor used was the 500 Series Portable Air Quality Monitor. It is used for real-time measurements of a variety of common air pollutants. Sensors are housed in a separate, easy-to-change cartridge, allowing the owner to choose which gasses and how many gasses to monitor. Swappable sensor heads measuring up to 16 different pollutants including ammonia, carbon dioxide, carbon monoxide, chlorine, formaldehyde, hydrogen, hydrogen sulfide, methane, nitrogen dioxide, non-methane hydrocarbons, ozone, perchloroethylene, and sulfur can be purchased. The 500 Series enables accurate measurement to 0.1-mg real-time surveying of common outdoor and indoor pollutants in an ultra-portable device. It is suitable for use on a range of projects, including wide-area air quality surveys, personal exposure monitoring, and short-term fixed monitoring. The 500 Series monitor from Aeroqual is a feature-rich portable monitor (Figure 3) with the ability to accurately measure multiple target gases at different concentrations in indoor and outdoor applications. It is produced in New Zealand with built-in data-logging and a long life with a fast-charging lithium battery.
The studied households were using firewood, crop residue, dung, and charcoal as solid biomass energy sources. However, the most common biomass energy source used by all households for cooking in improved cookstoves was firewood. The rural houses were relatively crowded because of the poor condition of the family. There was no separate kitchen for cooking in most of the households surveyed. The cooking area was either in the same room as the living or sleeping place or connected with it and partitioned by temporary walls. As shown in Figure 4, the cooking and residential places were located in the same single room. The use of the same single room aggravates indoor air pollution due to smoke. It was observed that the installation of improved stoves was not appropriate because of the lack of a smoke outlet or chimney and wider spaces left for the firewood inlet. In most houses, all members of the family were exposed to indoor air pollution because there was no separate kitchen. Since there was no properly designed smoke outlet or chimney, the roof and wall of the houses were dark and accumulated soot.
In the present study, both qualitative and quantitative data were collected by interview and measurement. Households that use both open three-stone firewood cookstoves and closed improved cookstoves (Mirt and improved Gonzie) were selected on a nearly average size of a 1.80-m-long, 1.90-m-wide, and 2.10-m-high cooking room or kitchen. The cooking rooms had one 50 cm by 50 cm window but without a smoke outlet from the cooking place. In Ameya and Bure woredas, 100 households that were using Mirt baking stoves were purposively selected for comparison with the amount of wood used in traditional open three-stone cookstoves. In the case of the Dalocha woreda, 100 households that have improved Gonzie stoves were also purposively selected for comparison with traditional open three-stone cookstoves. The moisture content was measured using a portable moisture meter for wood stored for a maximum of 2 weeks under a shaded dry place, and the weight of firewood consumed per baking and/or cooking session in households was measured by trained enumerators by using a graduated weight balance (0.1 gm). The emissions of selected indoor air pollutants were measured three times (replicated) on different days for 3 min, at 10-min intervals, within 1-h cooking time in May 2021. The instrument used for emission was placed at a distance of 1.3 m from the cookstove (Figures 3, 5). The gas sensor instrument was fully charged before the measurement (Padhi et al., 2010).
Qualitative data were reported and summarized. The amount of firewood consumed by a household on a daily basis was calculated using an Excel software application developed by Shell Foundation. Here, daily refers to sessional firewood consumption used during cooking. The analysis of statistical mean variance, standard errors and correlations on wood consumption, wood moisture content, and amount of emission was used to know whether there are significant differences in the consumption of firewood when using the two different cookstove types. SPSS statistical software for Windows (version 20.0 SPSS Inc., Chicago, IL, United States) was used for data analysis.
The deforestation caused due to use of open firewood cookstoves was compared with that caused by use of improved stoves using the woody biomass stock density of 85.50 tons/ha (Moges et al., 2010) (Equation 1). The average household cooking or baking time using an injera was twice per week and 104 times per year.
where Defo (ha) is the deforestation in hectare/year; the amount of wood used is air-dried wood, commonly used during cooking and baking.
The annual carbon emission at the household level was determined by assuming half of the biomass as carbon and the rate of conversion of carbon to carbon dioxide emission of 3.67 (Equation 2).
On average, the amount of wood consumed ranged from 6.80 to 31.40 kg and 3.60 to 21.20 kg per session in open three-stone cookstoves and Mirt cookstoves, respectively. The highest wood consumption was observed in the Bure woreda, i.e., 8.20–13.10 kg, with the lowest number of persons of family per household, 4.7 persons, when compared with the other woredas. The least wood consumption was observed in Mirt stoves of Ameya, i.e., 2.20–14.00 kg per session (Table 2). The moisture content of the wood ranged from 8.60% to 8.90% in Bure, from 7.00% to 7.50% in Ameya, and from 7.30% to 7.60% in Dalocha. Eucalyptus tree species was the most common firewood species in Bure and Ameya woredas, and Carissa edulis was the most common in the Dalocha woreda (Table 2).
The highest annual deforestation was caused by use of firewood in open cookstoves, ranging from 119.20 to 159.35 m2 per year. The rate of deforestation was reduced by using improved cookstoves, ranging from 20% in Dalocha to 37% in Bure and 41% in Ameya. The complete combustion of firewood at the household level converted the biomass carbon to CO2e, which ranged from 3.74 to 5.00 tCO2e in open cookstoves and from 2.33 to 3.13 tCO2e in improved cookstoves (Table 2).
Visual observation during baking with an injera revealed that the emission of gas and particulate matter as indoor air pollutants made it difficult to enter the baking room or cooking place. There were different products of incomplete combustion that emit gases such as CO. In improved injera baking stoves, the mean emission of CO in the Bure woreda was 1,004.8 mg/m3, that in Ameya was 33 mg/m3, and that in Dalocha was 53.85 mg/m3. The mean concentration of PM2.5 in open three-stone stoves in the Bure woreda was 124.50 mg/m3, in Ameya 0.53 mg/m3, and in the Dalocha woreda 0.04 mg/m3 (Table 3). The difference in the amount of indoor air pollutant emissions was attributed to the amount of wood used. As shown in Table 2, in the Ameya woreda, improved stoves used the least amount of wood, and the emission level, as shown in Table 3, was also the lowest.
In the observed 1 h cooking/baking time, there was a concentration change among the indoor air pollutants. In improved injera baking stoves, the minimum concentration of carbon monoxide in the Bure woreda was 969 mg/m3 and the maximum was 1,035 mg/m3; in Ameya, the minimum was 22.00 mg/m3 and the maximum was 45.00 mg/m3; and in Dalocha, the minimum was 11.00 mg/m3 and the maximum was 98.00 mg/m3. The minimum and maximum concentrations of PM2.5 in open three-stone stoves in the Bure woreda were 121.00 mg/m3 and 128.00 mg/m3; in Ameya 0.33 mg/m3 and 0.80 mg/m3; and in Dalocha 0.005 mg/m3 and 0.097 mg/m3, respectively (Figure 6).
The surveyed households qualitatively expressed that there were eye and breathing problems, but did not know the cause of the problem and had no knowledge of indoor air pollution. The responses from the interviewed professionals also revealed that knowledge about indoor air pollution available at the institutional level was not addressed to the rural households. They also stated that air pollutants such as particulate matter can contribute to climate change. Particulate matter stays in the atmosphere for only a few days or weeks; reducing these emissions can help reduce climate impacts in the near term. Some particles warm the atmosphere (e.g., black carbon); others create cooling (e.g., sulfates and nitrates). However, there exists much uncertainty about interactions between air quality and climate change and the relative impacts of different modes of development and climate change on pollutants. The indoor air pollution created reduces the adaptive capacity of rural people to climate change. The deforestation caused by the excess use of firewood also reduces mitigation of climate change. However, further study is needed for understanding the interrelation of indoor air pollution and climate change.
Carbon monoxide and carbon dioxide emissions were positively correlated with PM2.5 and PM10 at both p < 0.05 and p < 0.001 levels (Table 4). The particulate matter emissions were also positively correlated with the amount of wood consumed at p < 0.05; as the amount of wood used increased, the amount of particulate matter also increased (Table 4). The moisture content of wood was significantly and positively correlated with all the gases recorded, except with SO2 and PM2.5 (p < 0.05).
Interviews with energy experts in Ethiopia revealed that indoor air pollution was given less attention in research and development, and most of the study results considered laboratory-level cookstove emission. Regulatory frameworks on indoor air pollution are very weak, and most of the people have poor knowledge regarding the health effects and health risks of indoor air pollution. Despite efforts to incorporate the WHO’s air quality guidelines into the Ethiopian national legislation, there were no monitoring and practical implementation in the current health offices in rural household cooking. There were no explicit policies regarding indoor air pollution. Households were using their cookstoves in any arbitrary place, such as in the same place as the residing or sleeping place and in outdoor or a separate kitchen. In the administrative structure, the consideration of indoor air pollution and cookstoves at the institutional level frequently changed, and its position was either with health sectors, energy sectors, or environmental sectors. As a result, few protective measures taken toward indoor air pollution in Ethiopia. The installation of a chimney that expels smoke outside the cooking area and placing oneself away from the fire points were less practiced. The efforts in introducing improved cookstoves to reduce indoor pollution were hampered by the lack of chimneys that expel smoke outside the cooking areas and because of the poor technical capacity and poor economic condition of households.
There are climate change mitigation policies that make buildings increasingly airtight to increase energy efficiency. However, building airtightness increases the concentrations of air pollutants such as fine particulate matter (PM2.5) and carbon monoxide. Then, properly ventilated systems, such as mechanical ventilation systems with heat recovery and air filtration, are possible solutions but not practically used in Ethiopia. Therefore, policies should promote long-term energy-efficient building interventions, accompanied by proper ventilation and shading, and the removal of indoor air pollution sources by building chimneys and separate kitchens for cooking food using improved cookstoves.
Indoor air pollution is created by air pollutants from indoor sources and from outdoor sources that enter houses. Outdoor air pollution in rural areas is negligible owing to the absence of industrial activities and car transport. In the present study, the average size of the cooking room or kitchen was 1.80 m long, 1.90 m wide, and 2.10m high. The cooking rooms had one 50 cm by 50 cm window but without a smoke outlet from cooking areas. Indoor pollution in rural area households, therefore, was attributed mostly to indoor cooking. The solid biomasses were a cheap source of energy, which causes indoor pollution, for poor households, as stated in Schwela (1997). Du et al. (2017) and Lin et al. (2016) emphasized the health impacts of firewood cookstoves because of the higher amount of emissions of elemental carbon, PM2.5, organic carbon, and PAHs during firewood combustion, which were even greater than that of fossil coal. In this study, the emission was measured in the dry season, and according to Haung et al. (2022), the emission could be twice greater in the rainy season due to the higher moisture content of firewood.
In use of the improved stoves of Mirt and Gonzie, higher emissions of indoor pollutant gases were observed in all the studied woredas of Bure, Ameya, and Dalocha. However, the amount of emission associated with the type of firewood plant species used such as Eucalyptus and Carissa edulis was not studied. Other studies stated that Eucalyptus and Acacia produce approximately 25 times more small particles than LPG. Combustion of pine, oak, and Eucalyptus firewood emits particles that range from 0.10 to 0.30 µm (Kleeman et al., 1999). Softwoods generally release more emissions than hardwoods during combustion in fireplaces. The intensity of indoor air pollution is affected by the extent of human exposure, fuel moisture, burning rate, ventilation, and cooking behavior. PM2.5 emissions were predominant in the burning of forest biomass, which are hazardous to health. Similarly, the PM levels observed in this study were higher than the levels recommended by the WHO (Table 3), which cause different health problems such as blood pressure (Wang. et al., 2023b). Solid biomass fuels require large volumes and mass relative to the energy delivered, and they produce a high level of combustion emissions (Zhao et al., 2020). For household energy sources, the energy density ladder in decreasing order is electricity > gas > kerosene > wood > crop residues > dung, showing biomass as the least energy-dense component (Smith et al., 1994) but higher emitter. Combustion of compacted biomass resulted in lower particulate emission factors. Indoor air carbon monoxide levels caused by faulty or unvented combustion appliances in poorly ventilated rooms are usually higher than those in outdoor air. In kitchens, short peak concentrations may exceed 115 mg/m3 (100 ppm). Smoke from tobacco in dwellings, offices, vehicles, and restaurants can increase the 8-h average carbon monoxide concentration by up to 23–46 mg/m3 (20–40 ppm) (Washington D.C, 1991). The indoor air pollution is often exacerbated in homes using biomass fuel and poorly designed stoves, which do not have fireplaces or hoods to expel the smoke outside the dwelling area. The combustion efficiency of biomass is also very low, resulting in relatively high levels of incomplete combustion products. Improved stoves and well-dried biomass fuel that can be burned cleanly can be used in order to produce carbon dioxide and water instead of incomplete combustion products. Therefore, modifying different aspects of current traditional fuel stoves and energy use behaviors could reduce the emission of indoor air pollutants better than using of stoves that use liquid fuel, gas, or electricity (Fullerton et al., 2008).
The indoor pollutants in smoke such as carbon monoxide, carbon dioxide, sulfur dioxide, and particulate matter emitted during cooking or heating are hazardous substances because their amount was above the levels recommended by WHO (2006) and WHO (2023) (Table 3), which lead to a broad range of health problems and may even be fatal, as stated by Smith and Liu (1994). According to WHO (2006) and WHO (2023), the 1-h concentration of CO, SO2, PM2.5, and PM10 emission levels is 40 mg/m3, 35 μg/m3, 25 μg/m3, and 50 μg/m3, respectively. Concentrations above these limits, as shown in the current study, are dangerous to human health. In Adama City of Central Ethiopia, which was similar to the current study area, household air pollution attributable to mortality was estimated to be 50% due to acute lower-respiratory infections (ALRIs), 50% due to chronic obstructive pulmonary disease (COPD), and 50% due to lung cancer (Balidemaj et al., 2021). Although it was believed that women and children who are exposed to high particulate matter concentrations when cooking indoors are more vulnerable to the health impacts of indoor air pollution (Abera et al., 2021), staying indoors for longer hours during the 2020 COVID-19 pandemic revealed the occurrence of increased indoor air pollution in all groups of rural household residents (Du and Wang, 2020).
In the current study, the households did not emphasize on smoke indoor pollution, which unknowingly causes health effects such as eye problems and respiratory diseases, which is in line with the documented information in the study by Levesque et al (2001); WHO (2010); and Washington D.C, (1991) about the impacts of CO in reducing the availability of O2 in the blood system. The levels of carbon monoxide emitted in all cooking places of the current study areas were higher than the WHO standards, except in the Ameya woreda (Table 3) where the improved stoves caused lower emission and open three-stone stoves showed the maximum tolerable limit of approximately 35–40 mg/m3 (WHO, 2023). The CO exposure of Bure woreda households was above 26 times the permissible limit of 1-h average WHO standards (Table 3), which is very dangerous for the residents. Therefore, awareness creation on the health effects of the fire smoke indoor air pollution and remedial measures like ventilation of the cooking area and installation of improve stoves with a chimney is highly important, as confirmed by Adane et al. (2021) and Phillip et al. (2023).
According to USEPA (2002), the threshold level of daily average concentration of PM10 should be less than 150 μg/m3 and the annual average should be less than 50 μg/m3 for human beings. However, in many parts of developing countries, the indoor concentration of PM10 often exceeds 2,000 μg/m3 (Regalado et al., 2006). The present study showed a similarly higher amount. Particulate matter can easily bind to toxic metals or carcinogenic compounds, and it is deposited deep in the alveolar lung tissue for prolonged time periods (Badamassi et al., 2017). PM2.5 is hazardous to health as it comprises organic matter (30%–60%), nitrates and sulfates (25%–30%), elemental carbon (5%), and metals (1%) (USEPA, 1995). The households in the Bure district exceeded the WHO guidelines for 24-h mean particulate matter levels set as 50 μg/m3 for PM10 and 25 μgm−3 for PM2.5 (WHO, 2006). The other districts showed normal conditions of particulate matter, which could be because of the type of woody species used for firewood, which requires further study (Table 3). Carbon dioxide emission in the cooking place of the study areas was 35–562 times higher than the permissible limit of the 1-h average stated by WHO (2006). Therefore, the cooking areas of all the studied woredas were at high risk for CO2 indoor pollution. This also requires institutional attention both from the energy sector in supplying clean energy and from the housing sector in creating better ventilation and appropriate cooking places. The ventilation of rooms needs to keep carbon dioxide concentrations below 1,800 mg/m3 to create acceptable indoor air quality conditions. When people are exposed to levels of CO2 above 8,998 mg/m3 for hours, it could cause headache, dizziness, nausea, and other symptoms. Concentrations of CO2 of approximately 71,984 mg/m3 are immediately dangerous to life and health because they replace oxygen in the blood stream (https://www.health.state.mn.us/communities/environment/air/toxins/co2.html). In stationary combustion units, such as household firewood combustion in open stoves for food cooking, carbon dioxide is the major greenhouse gas (Gillen water, 2005) that has indoor air pollution and climate change effects. The emission level of SO2 in the present study was lower than the WHO standard (Table 3), which could be due to the low amount of sulfur contained in wood (WHO, 1997). Therefore, SO2 emission was not a health risk in the cooking places of the studied woredas (WHO, 2018). The wood moisture content was highly correlated with the emission levels. In developing countries, such as Nepal and China, where traditional methods such as open cooking fire stoves and other open devices are used, the total suspended particulate matter from wood smoke range from 2.7 to 25 mg/m3 (WHO, 2016). The emission levels of most of the gases were significantly different (p < 0.05) depending on the amount of moisture content of the wood, with higher moisture content indicating higher emission (Table 4). Indoor air pollution affects climate change and vice versa (West et al., 2013). Outdoor environmental changes such as temperature increase indoor air pollution (Abera et al., 2021). The indoor pollutants also create short-term atmospheric composition change (USDOE, 2011). The combustion of fossil fuel is estimated to result in tens of thousands of avoidable deaths due to air pollution by 2030 (Marais and Wiedinmyer, 2016) and accelerate climate change. Therefore, investing in an appropriate type of firewood and other renewable energy resources with improved stoves rather than relying on the combustion of fossil fuels is essential for the health of the African population (Marais et al., 2019).
The use of firewood aggravates the rate of deforestation and is attributed to negative impacts on soil, water, and livelihood, including loss of agricultural productivity (FAO, 2019). The highest firewood consumption observed in the Bure woreda has a potential of deforesting 99.74 m2 in Mirt cookstoves and 159.35 m2 in open cookstoves per household per year, which emitted approximately 3.13 tCO2e and 5.0 tCO2e, respectively, in complete combustion (Table 2). It was known that introducing efficient stoves reduces forest degradation by saving approximately 0.9 tons of biomass/year/household and saves woody biomass from being cut and burned to increase carbon sequestration to 2.1 tons/year/household. In Ethiopia, the dissemination of improved stoves to 20 million households was proposed to reduce the emission by 35 MtCO2e in 2030 (CRGE, 2011). Parker et al. (2015) reported that the emissions from traditional open stoves account for approximately 2% of global greenhouse gas emissions contributing to global warming.
Most of Ethiopian legislations of air pollution focus on industries. The pollutions caused by domestic biomass burning were given low law enforcement and lack monitoring (Joss et al., 2017). However, in developed countries like America and Europe, real-time monitoring that facilitates modeling of indoor air pollution was practiced by using a predetermined policy environment (Wang. et al., 2023a). Therefore, the food cooking sector requires additional policy attention in rural Ethiopia.
The impact of smoke emission and associated indoor air pollution increases when baking or cooking was done inside the main dwelling unit using open cookstoves. Houses that use a higher amount of wood during cooking or baking faced a greater emission of indoor air pollutants. The highest rate of deforestation, i.e., 159.35 m2/year, and the highest firewood combustion emission of 5 tCO2e per household were observed in the open cookstoves of Bure woreda. In most of the households surveyed, there was no separate kitchen for cooking. The particulate matter emissions were correlated with the amount of wood consumption at p < 0.05. The narrow size of the rural house cooking area and the lack of sufficient ventilation revealed that indoor cooking was the main source of indoor air pollution in households in rural areas. Other studies emphasized the health impacts of firewood cookstoves because of the higher amount of emission of elemental carbon, PM2.5, organic carbon, and PAHs during firewood combustion, which were even greater than the fossil fuel. The emission of PM2.5 in open three-stone cookstoves in the Bure woreda was 124.5 mg/m3; in Ameya 0.528 mg/m3; and in Dalocha 0.038 mg/m3, which is dangerous for the health of the household inhabitants, especially for the common rural cooking women and the accompanying children. In particular, in Bure woreda households, CO exposure was above 26 times the permissible limit of the 1-h average WHO standards. The PM in the current study was higher than the recommended levels of the WHO, which causes different health problems such as blood pressure. Modifying different aspects of current traditional fuel stoves and energy use behaviors could reduce the emission of indoor air pollutants better than using stoves that use liquid fuel, gas, or electricity. According to the WHO, the 1-h concentration of CO, SO2, PM2.5, and PM10 emission levels is 40 mg/m3, 35 μg/m3, 25 μg/m3, and 50 μg/m3, respectively. Concentrations above these limits, as shown in the current study, are dangerous to human health. The households in the Bure district exceeded the WHO guidelines in 24-h mean particulate matter levels set as 50 μg/m3 for PM10 and 25 μg/m3 for PM2.5. The minimum particulate matters studied were PM2.5, which indicates the presence of ultrafine particles (less than 1 µ), and therefore, further study should be conducted on the extent of ultrafine particles in the studied areas. Carbon dioxide emission in the cooking place of the study areas was 35–562 times higher than the permissible limit of WHO standards, which result in health conditions such as headache, dizziness, and nausea. This also requires institutional attention both from the energy sector in supplying clean energy and from the housing sector in creating better ventilation and appropriate cooking places.
The health effects induced by exposure to indoor pollutants were given less attention by the local people of the current study area, although the negative impacts are known at the institutional level. The households surveyed were not taking care of their health associated with the problems of indoor air pollution in the areas of cooking and the type of cookstoves used.
The risks of exposure to indoor air pollutants and its health effects were very high in houses that use firewood for cooking food. Indoor air pollution is a great problem in all rural areas and some urban areas of Ethiopia. The role of improved stoves in the reduction of indoor air pollution was not observed in the current study because of the inappropriate installation of a wide opening left for the firewood inlet and the lack of a smoke outlet and chimney from the cooking place. Although improved stoves deliver some climate benefits and save wood consumption, they do not protect human health adequately. There was also a lack of information about the health impacts of indoor pollution on the studied Ameya, Bure, and Dalocha woredas households of Ethiopia. Therefore, remedial measures are highly important in order to reduce the heath-damaging effects of smoke indoor air pollution in rural house settings of Ethiopia, and further empirical study should be conducted in hospitals and health centers about the impacts of indoor air pollution. There should be appropriate policies and institutions that deal with the cookstoves, solid biomass fuel utilization, and indoor air pollution in Ethiopia.
The original contributions presented in the study are included in the article/Supplementary Material; further inquiries can be directed to the corresponding author.
Ethical review and approval were not required for the study on human participants in accordance with the local legislation and institutional requirements. Written informed consent from the patients/ participants or patients/participants’ legal guardian/next of kin was not required to participate in this study in accordance with the national legislation and the institutional requirements.
MF: conceptualization, data curation, formal analysis, investigation, methodology, and writing–original draft. MM: writing review and editing. BH: writing review and editing.
The author(s) declare financial support was received for the research, authorship, and/or publication of this article. The study was financially supported by the Ethiopian Environment and Forest Research Institute.
The authors declare that the research was conducted in the absence of any commercial or financial relationships that could be construed as a potential conflict of interest.
All claims expressed in this article are solely those of the authors and do not necessarily represent those of their affiliated organizations, or those of the publisher, the editors, and the reviewers. Any product that may be evaluated in this article, or claim that may be made by its manufacturer, is not guaranteed or endorsed by the publisher.
Abera, A., Friberg, J., Isaxon, C., Jerrett, M., Malmqvist, E., Sjöström, C., et al. (2021). Air quality in africa: public health implications. Annu. Rev. Public Health 42 (1), 193–210. doi:10.1146/annurev-publhealth-100119-113802
Adane, M. M., Alene, G. D., and Mereta, S. T. (2021). Biomass-fuelled improved cookstove intervention to prevent household air pollution in Northwest Ethiopia: a cluster randomized controlled trial. Environ. Health Prev. Med. 26, 1. doi:10.1186/s12199-020-00923-z
Alemayehu, E. Y. (2011). Rural housing skill transfer and the milestones for scaling-up: the case of sustainable rural dwelling units (SRDU) in Ethiopia. ATDF J. 8 (3/4), 24–28.
Badamassi, A., Xu, D., and Leyla, B. (2017). The impact of residential combustion emissions on health expenditures: empirical evidence from Sub-Saharan Africa. Atmosphere 8 (9), 157. doi:10.3390/atmos8090157
Balidemaj, F., Isaxon, C., Abera, A., and Malmqvist, E. (2021). Indoor air pollution exposure of women in adama, Ethiopia, and assessment of disease burden attributable to risk factor. Int. J. Environ. Res. Public Health 18, 9859. doi:10.3390/ijerph18189859
Bell, M. L., and Samet, J. M. (2005). in Enviromental health: from global to local. Editor H. Frumkin (San Francisco, CA: Jossey-Bass).
CRGE (2011). “Ethiopia’s climate-resilient green economy, green economy strategy: the path to sustainable development,” in Environmental protection authority. Addis Ababa, Ethiopia: Government of Ethiopia, 188p.
DoH (Department of Health) (2017). Carbon monoxide preventing carbon monoxide poisoning in your home. Indoor Air Unit. Available at: www.health.state.mn.us.4/2017ID#56687.
Du, W., Shen, G., Chen, Y., Zhu, X., Zhuo, S., Zhong, Q., et al. (2017). Comparison of air pollutant emissions and household air quality in rural homes using improved wood and coal stoves. Atmos. Environ. 166, 215–223. doi:10.1016/j.atmosenv.2017.07.029
Du, W., and Wang, G. (2020). Indoor air pollution was non negligible during COVID-19 lockdown. Aerosol Air Qual. Res. 20, 1851–1855. doi:10.4209/aaqr.2020.06.0281
FAO (2019). National gender profile of agriculture and rural livelihoods – Ethiopia. Rome, Italy: Country Gender Assessment Series. Available at: http://www.fao.org/3/ca3224en/ca3224en.pdf.
Fullerton, D. G., Bruce, N., and Gordon, S. B. (2008). Indoor air pollution from biomass fuel smoke is a major health concern in the developing world. Trans. R. Soc. Trop. Med. Hyg. 102 (9), 843–851. doi:10.1016/j.trstmh.2008.05.028
GACCS (Global Alliance for Clean Cook Stoves) (2014). Clean cook stoves and fuels: a catalog of carbon offset projects and advisory service providers. 2nd edition.84p
Geissler, S., Hagauer, D., Horst, A., Krause, M., and Sutcliffe, P. (2013). Biomass energy strategy for Ethiopia. Addis Ababa, Ethiopia: Ministry of Water and Energy, 92.
Gessesse, D., and Carl, C. (2008). Forest decline and its causes in the South-Central Rift Valley of Ethiopia: human impact over a one hundred year perspective. Ambio 37 (4), 263–271.
Huang, Y., Wang, J. Z., Chen, Y., Chen, L., Chen, Y. C., Du, W., et al. (2022). Household PM2.5 pollution in rural Chinese homes: levels, dynamic characteristics and seasonal variations. Sci. Total Environ. 817, 153085. doi:10.1016/j.scitotenv.2022.153085
Huboyo, H. S., Tohno, S., Lestari, P., Mizohata, A., and Okumura, M. (2014). Characteristics of indoor air pollution in rural mountainous and rural coastal communities in Indonesia. Atmos. Environ. 82, 343–350. doi:10.1016/j.atmosenv.2013.10.044
Joss, M. K., Eeftens, M., Gintowt, E., Kappeler, R., and Künzli, N. (2017). Time to harmonize national ambient air quality standards. Int. J. Public Health 62, 453–462. doi:10.1007/s00038-017-0952-y
Kajekar, R. (2007). Environmental factors and developmental outcomes in the lung. Pharmacol. Ther. 114, 129–145. doi:10.1016/j.pharmthera.2007.01.011
Kedir, M. F., Bekele, T., and Feleke, S. (2019). Problems of Mirt. and potentials of improved Gonzie and traditional open cook stoves in biomass consumption and end use emission in rural wooden houses of Southern Ethiopia. Sci. Afr. 3, e00064. doi:10.1016/j.sciaf.2019.e00064
Kleeman, M. J., Schauer, J. J., and Cass, G. R. (1999). Size and composition distribution of fine particulate matter emitted from wood burning, meat charbroiling and cigarettes. Environ. Sci. Technol. 33, 3516–3523. doi:10.1021/es981277q
Levesque, B., Allaire, S., Gauvin, D., Koutrakis, P., Gingras, S., Rhainds, M., et al. (2001). Wood-burning appliances and indoor air quality. Sci. Total Environ. 281, 47–62.
Lin, N., Chen, Y., Du, W., Shen, G., Zhu, X., Huang, T., et al. (2016). Inhalation exposure and risk of polycyclic aromatic hydrocarbons (PAHs) among the rural population adopting wood gasifier stoves compared to different fuel-stove users. Atmos. Environ. 147, 485–491. doi:10.1016/j.atmosenv.2016.10.033
Mac (2009). Indoor air pollution create problems for rural areas. Available at: http://www.lifeofearth.org.
Marais, E. A., Silvern, R. F., Vodonos, A., Dupin, E., Bockarie, A. S., Mickley, L. J., et al. (2019). Air quality and health impact of future fossil fuel use for electricity generation and transport in africa. Environ. Sci. and Technol. 53 (22), 13524–13534. doi:10.1021/acs.est.9b04958
Marais, E. A., and Wiedinmyer, C. (2016). Air quality impact of diffuse and inefficient combustion emissions in africa (DICE-Africa). Environ. Sci. and Technol. 50 (19), 10739–10745. doi:10.1021/acs.est.6b02602
Moges, Y., Eshetu, Z., and Nune, S. (2010). Ethiopian forest resources: current status and future management options in view of access to carbon finances can be. Available at: http://wwwnorwayorget/PageFiles/628168/EthiopiaForestResourcesCurrentStatusandFutureManagementOptionsECRN-UNDP2010pdf.
MoWE (2023). Draft energy policy of Ethiopia. Addis Ababa, Ethiopia: MoWE Ministry of Water and Energy.
Naeher, L. P., Brauer, M., Lipsett, M., Zelikoff, J. T., Simpson, C. D., Koenig, J. Q., et al. (2007). Woodsmoke health effects: a review. Inhal. Toxicol. 19 (1), 67–106. doi:10.1080/08958370600985875
Padhi, B. K., Padhy, P. K., Sahu, L., Jain, V. K., and Ghosh, R. (2010). Assessment of intra-Urban variability in indoor air quality and its impact on children’s health. Air Qual. Atmos. Health 3, 149–158. doi:10.1007/s11869-010-0063-x
Parker, R., Vázquez-Rowe, I., and Tyedmers, P. (2015). Fuel performance and carbon footprint of the global purse seine tuna fleet. J. Clean. Prod. 103, 517–524. doi:10.1016/j.jclepro.2014.05.017
Perez-Padilla, R., Schilmann, A., and Riojas-Rodriguez, H. (2010). Respiratory health effects of indoor air pollution. Int. J. Tuberc. Lung Dis. 14 (9), 1079–1086.
Phillip, E., Langevin, J., Davis, M., Kumar, N., Walsh, A., Jumbe, V., et al. (2023). Improved cookstoves to reduce household air pollution exposure in sub-Saharan Africa: a scoping review of intervention studies. PLoS ONE 18 (4), e0284908. doi:10.1371/journal.pone.0284908
Regalado, J., Perez-Padilla, R., Sansores, R., Ramirez, P. J. I., Brauer, M., Pare, P., et al. (2006). The effect of biomass burning on respiratory symptoms and lung function in rural Mexican women. Am. J. Resp. Crit. Care Med. 174901905.
Sanbata, H., Asfaw, A., and Kumie, A. (2014). Indoor air pollution in slum neighbourhoods of Addis Ababa, Ethiopia. Atmos. Environ. 89, 230–234. doi:10.1016/j.atmosenv.2014.01.003
Schwarze, P. E., Ovrevik, J., Lag, M., Refsnes, M., Nafstad, P., Hetland, R. B., et al. (2006). Particulate matter properties and health effects: consistency of epidemiological and toxicological studies. Hum. Exp. Toxicol. 25, 559–579. doi:10.1177/096032706072520
Smith, K. R., Apte, M. G., Yuqing, M., Wongsekiarttirat, W., and Kulkarni, A. (1994). Air pollution and the energy ladder in Asian cities. Energy 19, 587–600. doi:10.1016/0360-5442(94)90054-x
Smith, K. R., and Liu, Y. (1994). “Indoor air pollution in developing countries,” in Epidemiology of lung cancer. Editor J. M. Samet (New York, NY: Marcel Dekker).
Smith, K. R., Rogers, J., and Cowlin, S. 2005. Households and ill-health in developing countries: what improvements can be brought by LP gas? Paris, France: world LP gas association.
Smith, K. R., Samet, J. M., Romieu, I., and Bruce, N. (2000). Indoor air pollution in developing countries and acute lower respiratory infections in children. Thorax 55, 518–532. doi:10.1136/thorax.55.6.518
Tasic, M., Rajšic, S., Novakovic, V., and Mijic, Z. (2006). Atmospheric aerosols and their influence on air quality in urban areas. Facta Univer Phys. Chem. Technol. 4, 83–91. doi:10.2298/fupct0601083t
Theuri, D. (2009). Rural energy, stove and indoor air quality: the Kenya Experience. Available at: http://www.itdg.org (Accessed on April 15, 2020).
Tsegaye, T. (2007). “An overview of the forest ecosystems of Ethiopia: functions, trends and future directions,” in Environment for survival. Taking stock of Ethiopia’s environment. Green forum (Addis Ababa, Ethiopia).
USDOE (United States Department of Energy) (2011). Biomass cook stoves technical meeting: summary report. Energy efficiency and renewable energy. Washington, DC, United States: Biomass programme.
USEPA. 1995. Emission factor documentation for AP-42 section 10.7, charcoal: final report. Office of air quality planning and standards, emission factor and inventory group: EPA contract 68-D2-0159, work assignment No. II-04, MRI project No. 4602-04
USEPA (2002). Third external review draft of air quality criteria for particulate matter. Available at: http://cfpub.epa.gov/ncea/cfm/partmatt.cfm (Accessed on May 7, 2019).
Wang, J., Du, W., Lei, Y., Chen, Y., Wang, Z., Mao, K., et al. (2023a). Quantifying the dynamic characteristics of indoor air pollution using real-time sensors: current status and future implication. Environ. Int. 175, 107934. doi:10.1016/j.envint.2023.107934
Wang, J. W., Lei, Y., Duan, W., Mao, K., Wang, Z., Pan, B., et al. (2023b). Impacts of household PM2.5 pollution on blood pressure of rural residents: implication for clean energy transition. Sci. Total Environ. 884, 163749. doi:10.1016/j.scitotenv.2023.163749
Washington D.C (1991). Air quality criteria for carbon monoxide. United States: US Environmental Protection Agency, Office of Research and Development. 1991 (publication no. EPA-600/B-90/045F).
West, J. J., Smith, S. J., Silva, R. A., Naik, V., Zhang, Y., Adelman, Z., et al. (2013). Co-benefits of mitigating global greenhouse gas emissions for future air quality and humanhealth. Nat. Clim. Change 3 (10), 885–889. doi:10.1038/nclimate2009
WHO (1997). Health and environment in sustainable development: five years after the earth summit. Available at: https://apps.who.int/iris/bitstream/handle/10665/69477/WHO_SDE_PHE_OEH_06.02_eng.pdf;jsessionid=9B6A5A9416234144E05CCBACFAA831D7?sequence=1.87
WHO (2006). WHO Air quality guidelines for particulate matter, ozone, nitrogen dioxide and sulfur dioxide Global update 2005, 22.
WHO (2010). Guidelines for indoor air quality— selected pollutants. WHO guidelines, vol. 9. Copenhagen: WHO Regional Office for Europe. doi:10.1186/2041-1480-2-S2-I1
WHO (2016). Burning opportunity: clean household energy for health, sustainable development, and wellbeing of women and children. Geneva: World Health Organization.
WHO (2018). The Breathe Life Campaign (37) the campaign combines public health and climate change expertise with guidance on implementing solutions to air pollution in support of global development goals. Geneva, Switzerland: WHO/CCAC/UNEP.
WHO (World Health Organization) (2023). World Health Organization air quality guidelines 2021 (AQGs) and estimated reference levels (RLs). Geneva, Switzerland: European Environmental Agency.
Yang, Y. Y., Fan, L., Wang, J., Zhu, Y. D., Li, X., Wang, X. Q., et al. (2021). Characterization and exposure assessment of household fine particulate matter pollution in China. Indoor Air 31, 1391–1401. doi:10.1111/ina.12843
Zhao, N., Li, B., Li, H., Ahmad, R., Peng, K., Chen, D., et al. (2020). Field-based measurements of natural gas burning in domestic wall-mounted gas stove and estimates of climate, health and economic benefits in rural Baoding and Langfang regions of Northern China. Atmos. Environ. 229, 117454. doi:10.1016/j.atmosenv.2020.117454
Keywords: chimney, fuel inlet, indoor pollution, separate kitchen, emission and ventilation
Citation: Fekadu M, Molla M and Hailu B (2024) The effect of firewood combustion cookstove types on indoor air pollution and deforestation in selected rural houses of Ethiopia. Front. Environ. Sci. 12:1326517. doi: 10.3389/fenvs.2024.1326517
Received: 23 October 2023; Accepted: 23 September 2024;
Published: 24 October 2024.
Edited by:
Mounia Tahri, National Centre for Nuclear Energy, Science and Technology, MoroccoReviewed by:
Wei Du, Kunming University of Science and Technology, ChinaCopyright © 2024 Fekadu, Molla and Hailu. This is an open-access article distributed under the terms of the Creative Commons Attribution License (CC BY). The use, distribution or reproduction in other forums is permitted, provided the original author(s) and the copyright owner(s) are credited and that the original publication in this journal is cited, in accordance with accepted academic practice. No use, distribution or reproduction is permitted which does not comply with these terms.
*Correspondence: Miftah Fekadu, bWZrZWRpckBnbWFpbC5jb20=
Disclaimer: All claims expressed in this article are solely those of the authors and do not necessarily represent those of their affiliated organizations, or those of the publisher, the editors and the reviewers. Any product that may be evaluated in this article or claim that may be made by its manufacturer is not guaranteed or endorsed by the publisher.
Research integrity at Frontiers
Learn more about the work of our research integrity team to safeguard the quality of each article we publish.