- 1Department of Biodiversity, DSI-NRF SARChI Chair (Ecosystem Health), University of Limpopo, Limpopo, South Africa
- 2Department of Biological Sciences, University of Venda, Limpopo, South Africa
- 3Department of Environmental Sciences, University of South Africa, Florida, South Africa
Stream flow weirs disrupt river flow regimes, physical conditions, and biological composition of rivers, posing significant threat to the river’s functionality, integrity, and sustainability. Home to diverse aquatic organisms, there is a lack of impact assessment of weirs on the stream of the Luvuvhu River Catchment (LRC). The aim of this study was to examine the impact of six weirs on aquatic macroinvertebrate assemblages and composition across five streams. A total of six sampling surveys on macroinvertebrates and physico-chemical parameters were conducted upstream and downstream of six weirs in 12 months between June 2021 and June 2022. The South African Scoring System score (SASS score) and Average Score per Taxon (ASPT) indices were used to compare organisms’ response between upstream and downstream of weirs. Generalized linear mixed models (GLMM) were used to determine the effect of physico-chemical variables on macroinvertebrate assemblage, while the CCA was employed to assess similarities between downstream and upstream sampling points across various sites. A total of 18,914 macroinvertebrate individuals were observed from 65 families during the survey. In upstream sections, the SASS score was driven by habitat integrity, electrical conductivity, and water depth, while ASPT responded significantly to habitat integrity, pH, dissolved oxygen and electrical conductivity. In the downstream sections, the SASS score increased with an increasing habitat integrity score and river width, while decreasing with elevation and pH levels. ASPT increased with increasing habitat integrity and elevation in the downstream sections, while decreasing with increasing river width and water temperature. This study’s findings suggest habitat integrity is largely driving aquatic abundance and assemblage in the LRC, and it is therefore important to maintain the ecological integrity of rivers.
1 Introduction
Streamflow is one of the predominant variables that drives the ecological condition of flowing water ecosystems and thus the life history characteristics of aquatic biota (Power et al., 1995; Poff and Zimmerman, 2010; Schneider and Petrin, 2017). Natural flow regimes are significant for rivers’ effective ecological functionality and sustainability (Bunn and Arthington, 2002; Belmar et al., 2012; Poff, 2018; Jumani et al., 2020; Carpenter-Bundhoo et al., 2023). However, the need for social, economic development and human demand for water resources have led to the transformation of many rivers (Principe, 2010; Hough et al., 2019; Leitner et al., 2021). Moreover, while both good water quality and ecosystem integrity are important for macroinvertebrate communities and human development, they have undergone modifications by humans (Hu et al., 2022; Ramulifho et al., 2023). Many weirs and dams were constructed in rivers with little or no cognisance of their ecological consequences or risk of modification (Mueller et al., 2011; Fencl et al., 2015; Winemiller et al., 2016). The construction of weirs changes the river flow regimes and disrupts the physical conditions, posing as one of the biggest threats to the river’s functionality, integrity, sustainability (Im et al., 2011; Mueller et al., 2011; Carpenter-Bundhoo et al., 2020), and connectivity (Grill et al., 2019; Casserly et al., 2020). Weirs and other in-stream structural barriers disrupt the natural continuity of a river, transforming it into a series of disconnected segments (Brooks et al., 2018; Seliger and Zeiringer, 2018). This alteration hinders the movement of organisms and results in the fragmentation of populations (Jager et al., 2001; Brooks et al., 2018). Therefore, weirs are structures (solid, watertight, and capable of withstanding flooding) on a river equipped and operated to provide the flow data from which systematic records of water level discharge or flow are extracted (Wessels and Rooseboom, 2009).
Aquatic macroinvertebrate organisms are very habitat specialised insects and are known for susceptible responsiveness to local-scale stressors mainly due to their soft life-history features and limited mobility (Cheimonopoulou et al., 2011). However, a mere reduction in flow velocity may eventually lead to either an increase or decrease in macroinvertebrate abundance (Dewson et al., 2007). This changes in hydrology and water quality causes differences in aquatic macroinvertebrate assemblages between upstream and downstream of dams or weirs (Chaves-Ulloa et al., 2014; Gezie et al., 2023). This is why the differences in macroinvertebrate assemblages have the potential to indicate free-flowing and impounded stream conditions (Linares et al., 2019), and their diversity can also mimic human impacts in river ecosystems (Gezie et al., 2023). The impact of flow variation caused by weirs on macroinvertebrate assemblages’ structure in rivers is not only limited to sites directly in the vicinity of the dams and weirs, but the effect could extend to some distance afar (Mueller et al., 2011; Munasinghe et al., 2021).
In South Africa, the South African Scoring System version 5 (SASS5) has been used as a rapid bioassessment tool to investigate water quality and the general state of riverine ecosystems, whereby each macroinvertebrate taxon is allocated a sensitivity score according to the water quality conditions it is feasible to tolerate (Chutter, 1998; Dickens and Graham, 2002; Dallas, 2007; Dallas, 2021). SASS is an effective rapid bioassessment method with the ability to detect water quality impact, and over the past years, the method has proved to be the most preferred general river health assessment tool in South Africa (Chutter, 1998; Dickens and Graham, 2002; Dallas, 2021). Dallas (2021) indicated that the SASS5 method has also been transformed for use in other African countries such as Zambia, Namibia, Tanzania, Uganda, Rwanda, Okavango Delta in Botswana and Kenya using the scoring system to assess water quality in rivers within their jurisdiction areas.
In South Africa, most of the research concerning aquatic macroinvertebrate species has primarily concentrated on large dams, with limited attention given to the bio-ecological impacts of weirs, streamflow gauging stations, and canals (Bredenhand and Samways, 2009; Hughes and Mantel, 2010; Mantel et al., 2010; Ramulifho et al., 2019; Janse van Vuuren and Swanepoel, 2023). The weirs and dams in the rivers may affect the macroinvertebrate assemblages and water quality (Gezie et al., 2023), and their impacts vary across regions and taxonomic groups (Wang et al., 2020). Little is known about the ecological impacts of weirs on river flow modification and aquatic macroinvertebrate assemblages. This is a case in point for the LRC, as there is a lack of studies that have documented clear impacts of flow regime and habitat modification on macroinvertebrate communities in rivers modified by the presence of weirs. The anthropogenic activities in the LRC caused severe changes in land use and cover and the landscapes (Kundu et al., 2015) where the weirs occur. As river systems and their associated aquatic biodiversity emerge as some of the most threatened ecosystems (Cantonati et al., 2020), the impact of weirs on macroinvertebrates in the Luvuvhu system remains unclear.
This study`s main aim was to examine the impact of river modification by stream flow gauging weirs on macroinvertebrate assemblages. This study also assessed if physico-chemical parameters differ above and below the stream flow gauging weirs.
2 Materials and methods
2.1 Description of the study area and sampling locations
The study was conducted at six weirs located within the LRC which is approximately 5,941 km2 at 1,312 m above sea level (Figure 1). The LRC is in the Vhembe District Municipality in the Limpopo Province and falls within the Limpopo Water Management area, in the north-eastern part of South Africa. The LRC hosts a variety of aquatic and riparian ecosystems, supporting diverse fauna and endemic plant species according to Brown (2014). The climate in this catchment exhibits spatial and temporal variations, falling under the classification of humid subtropical as indicated by Conradie (2012). The mean annual rainfall is 608 mm, while topography varies from 200 m to 1,500 m and greatly influences rainfall and run-off distribution in the catchment (Odiyo et al., 2015).
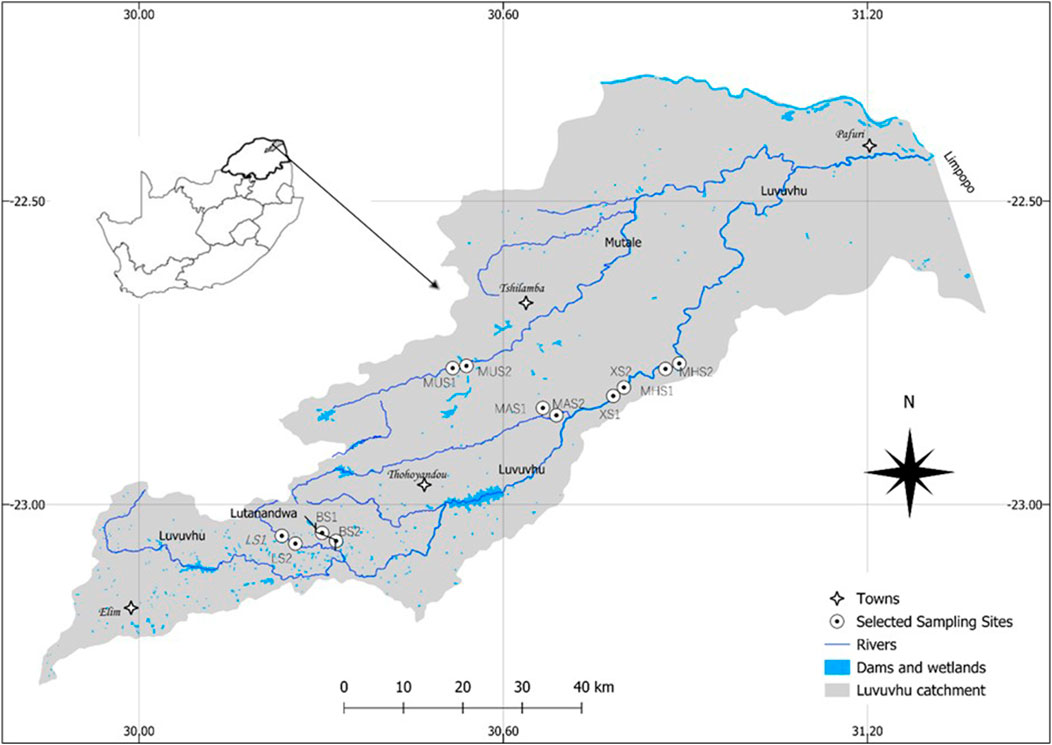
FIGURE 1. Study area map showing selected weirs within the Luvuvhu River catchment, while inset shows the context of the study area within Limpopo province, South Africa.
The highest rainfall occurs in the upper reaches where the Soutpansberg mountains are located, with little rainfall in the lower reaches around the Kruger National Park. Land-use activities in the LRC include forestry, agriculture, and settlements. The Luvuvhu River and its tributaries which include the Mutshundudi, Lutanandwa, Livhungwa and Mutale rivers rise in the Soutpansberg mountains (State of Rivers Report, 2001; Fouche, 2009; Nkuna and Odiyo, 2011). The catchment area and geographic coordinates emanating from the twelve sampling locations are shown in Table 1. The characterisation of six sampling weir’s sites including information of their size, age and intended construction purpose are shown in Table 2.
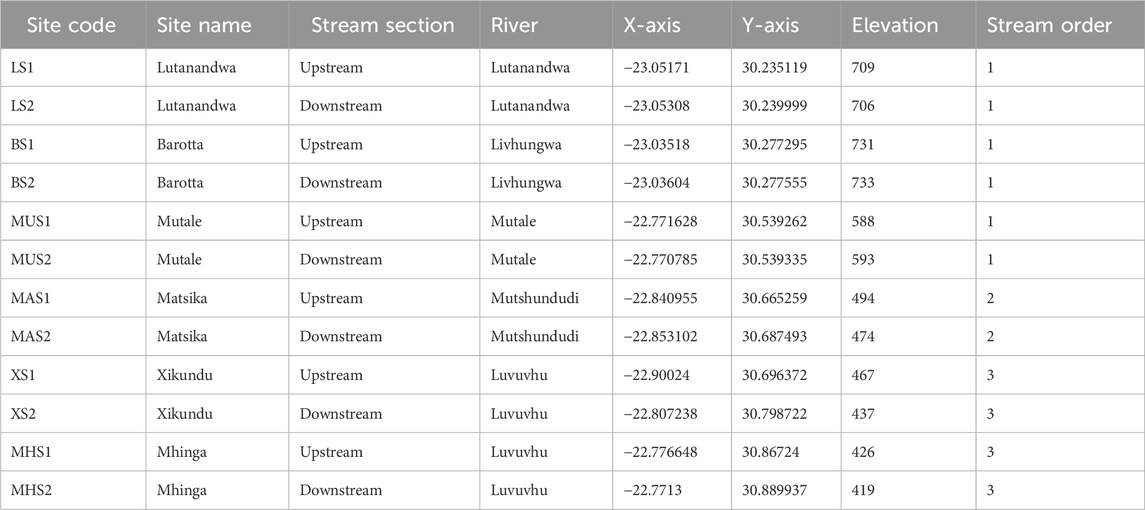
TABLE 1. The catchment area and geographic coordinates of the sampling sites of the six weirs in the Luvuvhu, Lutanandwa, Livhungwa, Mutale and Mutshundudi rivers.
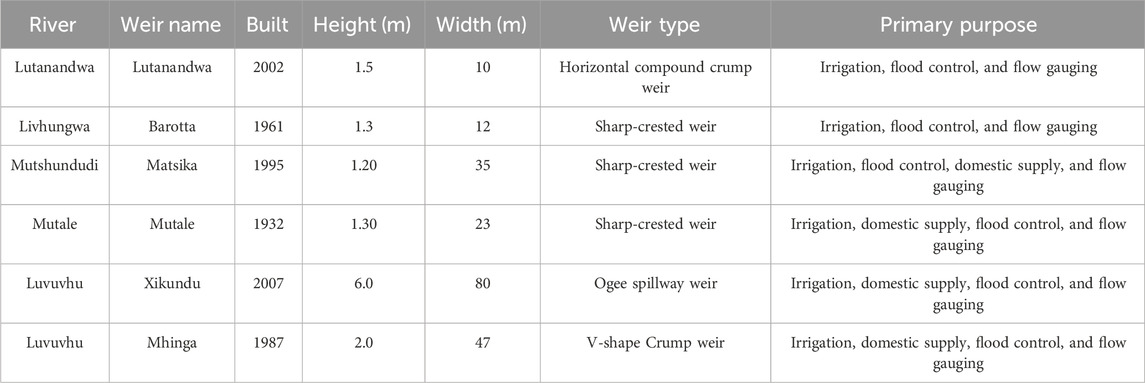
TABLE 2. Date of construction commission, age, height, width, design type and primary purpose of the weirs in the Luvuvhu River catchment.
2.2 Sampling points and measurement of physico-chemical parameters
This study considered weirs located across five rivers of this catchment, namely. The Livhungwa, Lutanandwa, Mutale, Mutshundudi, and Luvuvhu as primary sites. Sampling sites were located from 100 m above and below each primary site (weir). The sites in Lutanandwa, Barrota, and Mutale weirs exhibit near-natural conditions characterized by intact vegetation cover and minimal to no human impact. In contrast, the sites in Matsika, Mhinga, and Xikundu weirs within the catchment lack extensive vegetated areas along their banks. At each sampling site, a bi-monthly measurement (coincided with macroinvertebrates sampling) of water temperature (WT) (°C), dissolved oxygen (DO) (mg/L), pH, total dissolved solids (TDS) (mg/L), and electrical conductivity (EC) (mS/m) was taken using the YSI multiprobe meter in the upstream and downstream sections of the weirs. The meter was calibrated each time before any sampling event. Flow velocity (m.sˉ1) and water depth (cm) were measured using a flow meter FP101 (Global Water), while river width (m) and elevation (m) were measured using Google Earth. A Secchi disk was used to measure water transparency by determining the depth at which the disk becomes invisible as a function of clarity or transparency of water in centimetres (Bowers et al., 2020). The Integrated Habitat Assessment System (IHAS) which reflect the quantity, quality, and diversity of biotopes available was measured in this study using the total score of 100 points as developed and detailed in McMillan (1998).
2.3 Macroinvertebrate sampling
Macroinvertebrates were collected at each sampling point from the upstream and downstream of the weirs six times in 12 months between June 2021 and June 2022 on a 2-month interval, with the month of December 2021 excluded due to floods. Three main biotopes, stones-in-current (SIC), marginal vegetation (MV), and gravel/sand/mud (GSM) were targeted within a 200 m river stretch from the weir. Kick sampling was performed for 2 min in SIC biotopes, and for 1 min in GSM biotopes, while marginal vegetation was sampled along 2 m of riverbank according to the protocol by Dickens and Graham (2002). All sampling was performed moving from downstream to upstream, using a standard SASS kick net (30 cm × 30 cm wide x 50 cm deep with a 1 mm mesh). Once collected, each sample was visually assessed in a 50 cm × 30 cm white tray for up to 15 min. To obtain the SASS score and the ASPT, the SASS data sheet was used to record taxa that were present and to obtain their sensitivity scores. The collected macroinvertebrates were identified to family levels in the field using the field guides prescribed by Gerber and Gabriel (2002) and Fry (2021).
2.4 Data analysis
All statistical analyses were conducted using R statistical software (R Development Core Team, 2023). The spatio-temporal relationship between all physico-chemical parameters was measured using Pearson correlation analyses. Macroinvertebrate composition was analysed using the SASS score and ASPT as metrics of measurement. Macroinvertebrates estimate abundance values from the three biotopes at each site were pooled into single monthly SASS score and ASPT data. However, physico-chemical variables data were log-transformed to approximate normality prior to analyses. Standardization of physico-chemical data was necessary to reduce the influence of large differences and to normalize and render data homoscedastic (Ramulifho et al., 2023).
In order to assess the effects of weirs on SASS scores and ASPT at both the upstream and downstream sites, a generalized linear mixed model (GLMM) with negative binomial regression from the ‘lme4’ package and ‘glmer.nb’ function was used (Jamil and ter Braak, 2013; Nakagawa and Schielzeth, 2013). The effects of measured physico-chemical variables on SASS scores and ASPT were compared between upstream and downstream sites, based on a set number of 500 permutations in the data. The GLMM is an extension of the generalized linear model (GLM) in which the linear predictor contains random effects in addition to the usual fixed effects (Venables and Ripley, 2002). One full model of GLMM with all physico-chemical variables was run. During the analyses, sites were used as a random factor to account for temporal pseudo-replication, while all physico-chemical variables were included as fixed variables (Li et al., 2018). Best fitting models were selected in a stepwise backward manner according to their Akaike’s information criterion (AICs) (Li et al., 2018). Marginal r2 (variation explained by fixed effects only) and conditional r2 (variation explained by fixed and random effects) were calculated for the best and second-best models (Nakagawa and Schielzeth, 2013). The goodness-of-fit of the models was assessed using the relations between the residuals (the differences between observations and predictions by the retained model) and physico-chemical variables.
An initial detrended correspondence analysis (DCA) was employed on the macroinvertebrate dataset to assess the length of the gradient. The DCA axis I exhibited a gradient length exceeding 3.74 standard deviation units for the entire dataset, justifying the use of unimodal ordination canonical correspondence analysis (CCA) (Leps and Smilauer, 2003). Then CCA was employed to assess similarities between downstream and upstream sampling points across various sites, focusing on macroinvertebrates metrics and physico-chemical (Ter Braak and Verdonschot, 1995). CCA is commonly employed to identify the key environmental factors influencing the composition of the biological community at various sites. Data were log transformed to meet assumptions for normality, homoscedasticity and to prevent the undue influences of extreme values on the final CCA ordination. Monte Carlo permutation test with 999 random permutations was used in this CCA ordination. Measurements of macroinvertebrates metrics and physico-chemical variables were treated as predictors of similarities between sites during our analyses.
3 Results
3.1 Spatial-temporal variations of physico-chemical parameters
The values of WT, DO, EC, pH, TDS, river width, river depth, and velocity were averaged among the bi-monthly surveys at each sampling point (Table 3).
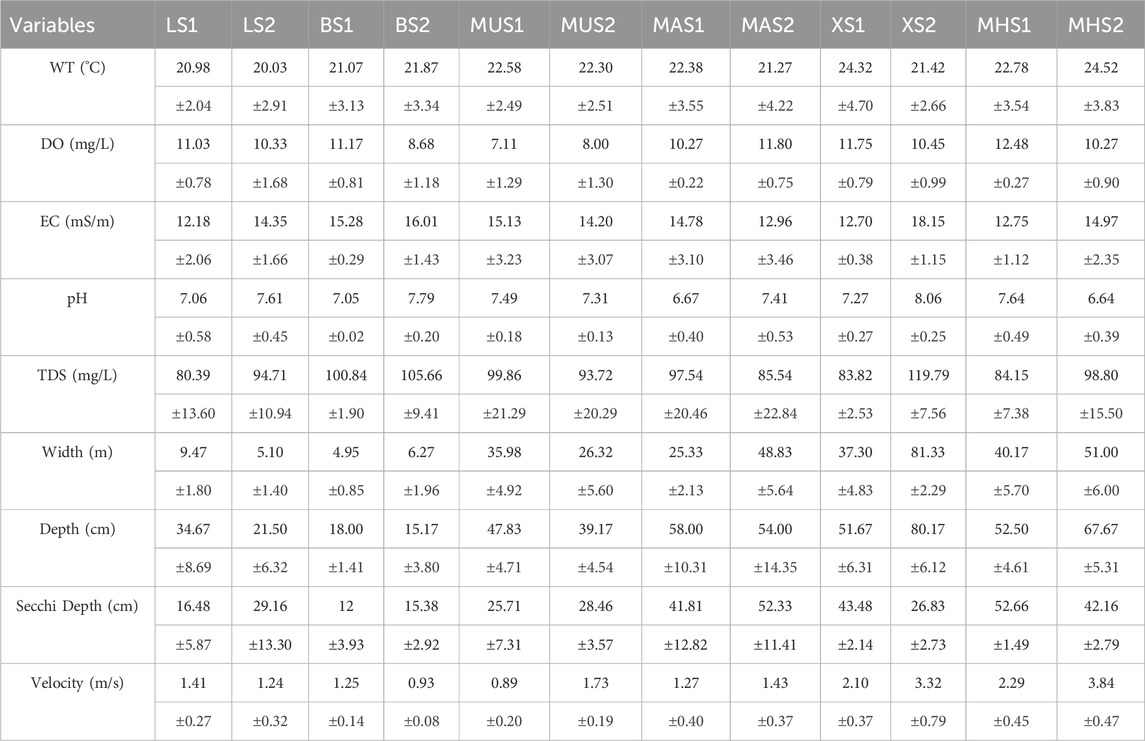
TABLE 3. Summary of mean values and standard deviation (±) of physico-chemical parameters for all the selected weirs in the Luvuvhu River during the study period.
Water depths in the downstream sections were generally reduced when compared to the upstream sections, except for sampling points XS2 and MHS2 (Table 3). The downstream sections exhibited relatively higher values of pH, EC, and TDS, while DO concentrations were comparatively higher in the upstream sections. Moreover, the average river width in the downstream sites witnessed an increase as opposed to the upstream sites, particularly noticeable at BS2, MAS2, XS2, and MHS2. The flow velocity in the downstream sites experienced a significant increase, attributed to elevated water turbulence discharge, except for LS2 and BS2, which demonstrated a notable reduction in velocity (Table 3).
More than half (51.5%) of physico-chemical variables correlations were negatively correlated in the upstream sites (Table 4). The highest negative correlations observed were between elevation and stream width (r2 = −0.85), stream depth (r2 = −0.74), and stream order (r2 = −0.74). Besides the best regression fit of r2 = 1 observed between EC and TDS, the next highest positive correction was between DO and IHAS (r2 = 0.81), Secchi depth and stream depth (r2 = 0.81), stream velocity, and stream order (SO) (r2 = 0.79). Elevation, TDS, and EC were variables with the most negative association compared to other physico-chemical variables at 72.7%. The most unlikely relationship observed was between pH and stream depth (r2 = 0.02) and between pH and IHAS (r2 = 0.02).
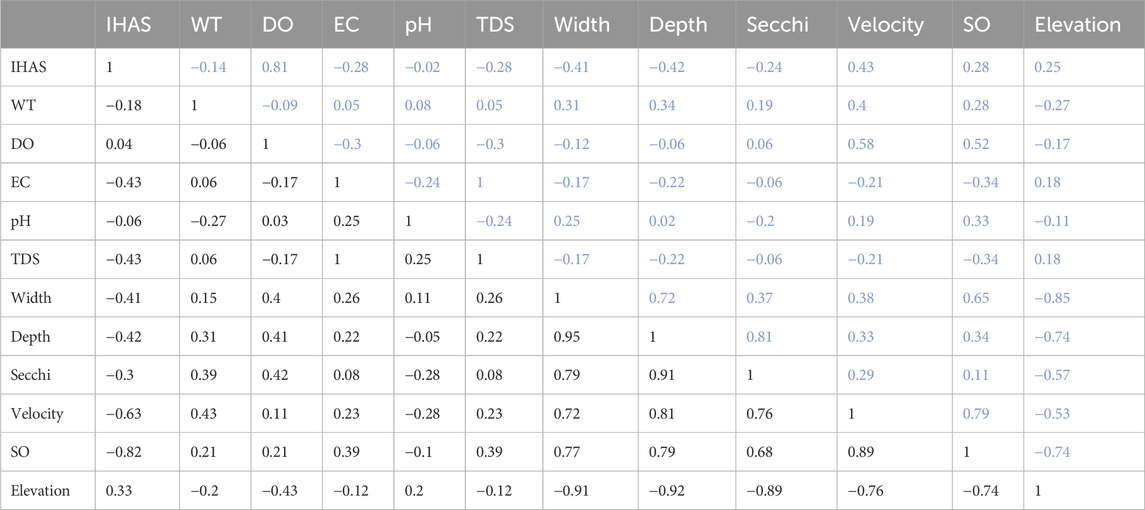
TABLE 4. The correlation coefficients (r2) between all physico-chemical variables measured using Pearson correlation analyses for upstream sites on the upper triangle of the correlation matrix table (blue values), while the downstream sites is shown on the lower triangle of the correlation matrix (black values).
On the downstream sites, more than 65% of physico-chemical variables were positively correlated. The highest positive correlation observed was between EC and TDS (r2 = 1), followed by a correlation between stream width and stream depth (r2 = 0.95), and a correlation between stream depth and Secchi depth (r2 = 0.91). The lowest negative correlation was observed between stream depth and elevation (r2 = −0.92) and between stream width and elevation (r2 = −0.91). The most unlikely relationship observed was between pH and DO (r2 = 0.03) and between DO and IHAS (r2 = 0.04). IHAS was the variable with the most negative association compared to other physico-chemical variables at 90.9%, followed by elevation with 81.8% (Table 4).
3.2 Macroinvertebrate response to weirs
An estimated total of 9,870 macroinvertebrate individuals were detected from 65 families during the 12-month survey at upstream sites. Baetidae was the most abundant family with an estimated total of 901 (9.13% of total estimated individuals) and was found in all the six upstream sites surveyed. Turbellaria, Oligoneuridae, Polymitarcidae, Lestidae, Psychomyiidae, Blephariceridae, and Physidae had the lowest number of individuals with only 1 specimen each. At downstream sections, an estimated total of 9,044 macroinvertebrate individuals were recorded. From the 60 families recorded, Baetidae also recorded the highest number of specimens at 950 individuals which is 10.50% of the total estimated individuals. Crambidae, Nepidae, and Psychomyiidae recorded the lowest number of individuals with only 1 specimen each (Supplementary Table S1).
In the upstream sections, the best model for the SASS score had eight physico-chemical variables (Table 5) and an AIC value of 350.4, while the second-best model yielded four physico-chemical variables and an AIC value of 355.2. For the best model, IHAS, EC and water depth were the most significant physico-chemical variables. Non-significant drivers of the SASS score included amongst other stream order, Secchi depth, and elevation. SASS scores increased with an increasing value of the IHAS score, while decreasing with EC, water depth and Secchi depth (Figure 2). For ASPT, the best model had an AIC value of 152.8 with four significant physico-chemical variables in the five model variables (Table 5). Significant variables included IHAS, pH, DO and EC, where the ASPT score only declined with EC (Figure 2). River depth was the only non-significant driver of ASPT. The second-best model of seven variables had an AIC value of 156.7 with five significant variables. Secchi depth and elevation were the two additional variables in the second-best model, hence when compared to the best model these were found not significant.
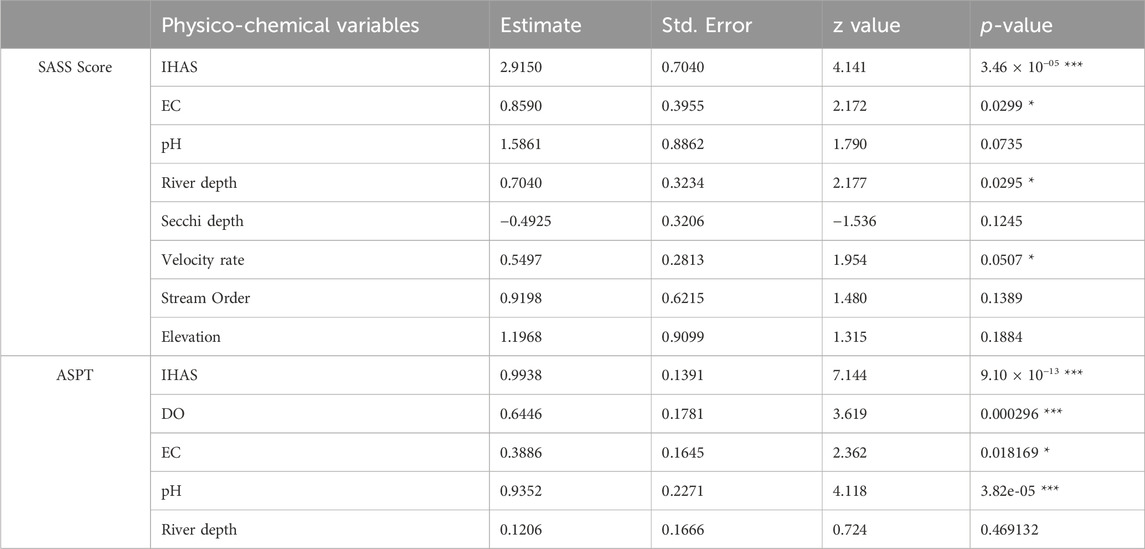
TABLE 5. Summary of models predicting drivers of observed changes for both SASS score and ASPT in the upstream sections of selected weirs in the LRC (significance: *p < 0.05, **p < 0.01, and ***p < 0.001).
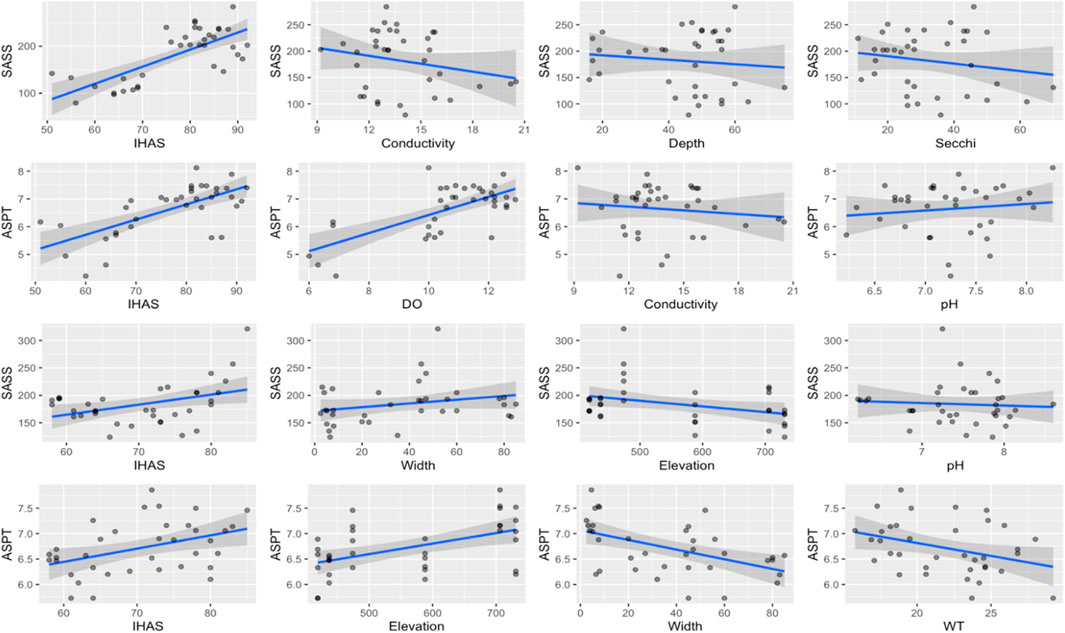
FIGURE 2. Regression plots showing the relationship between significant physico-chemical variables and both SASS Score and ASPT at upstream (Rows 1 and 2) and downstream sites (Rows 3 and 4).
In the downstream sections, the best model for the SASS score had an AIC value of 342.2 with four significant and two non-significant variables (Table 6). SASS scores increased with an increasing value of the IHAS score and river width while decreasing with elevation of site and pH concentration at a site (Figure 2). The most significant drivers of the SASS Score were IHAS, elevation, river width and pH. The second-best model had an AIC value of 343.6 with three significant variables in the eight physico-chemical variables that made up the model. For ASPT, the best model had an AIC value of 152.2 with all five physico-chemical variables as significant drivers of the ASPT score (Table 6). The ASPT increased with increasing IHAS and elevation while decreasing with increasing river width and water temperature. The second-best model of seven variables had an AIC value of 156.1 with only two significant variables (IHAS and elevation).
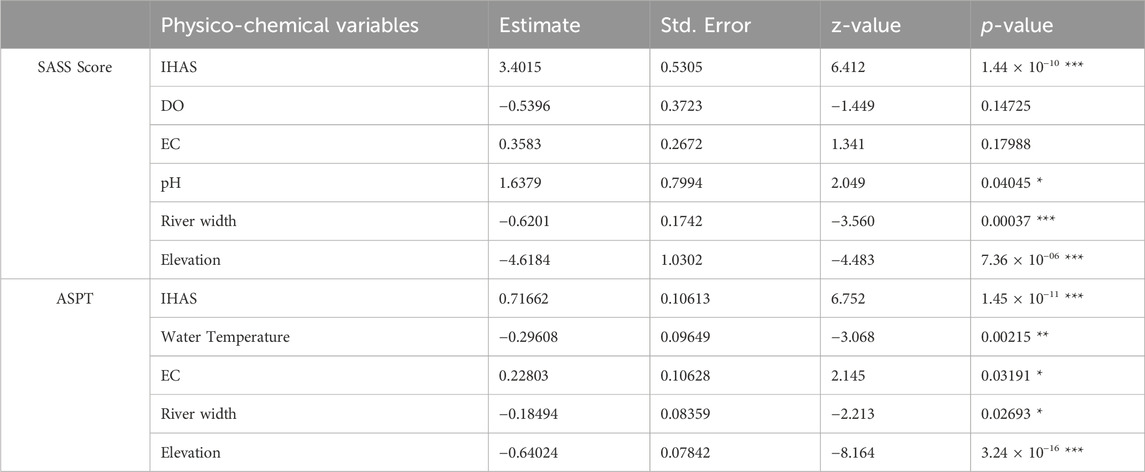
TABLE 6. Summary of models predicting drivers of observed changes for both SASS score and ASPT in the downstream sections of selected weirs in the LRC (significance: *p < 0.05, **p < 0.01, and ***p < 0.001).
3.3 Similarities between sampling sites
The cumulative percentage of variation explained by axis one to four of the correspondence analysis amounted to 72.92%, with the first and second axes explaining 57.37% and 12.02%, while third and fourth explained a combined 3.51% of total variation (Table 7). The Monte Carlo permutation test revealed statistical significance for all canonical axes (p < 0.05). Significant drivers of variation in structuring macroinvertebrates families within and across sampling points at both upstream and downstream included the water temperature, pH, river width, river depth, secchi depth, velocity rate and elevation (Figure 3). Some of the most abundance taxa in the Luvuvhu catchment such as baetidae, Potamonautidae, Gomphidae, Caenidae, Hydropsychidae and Veliidae were related to these variables (Supplementary Table S1).
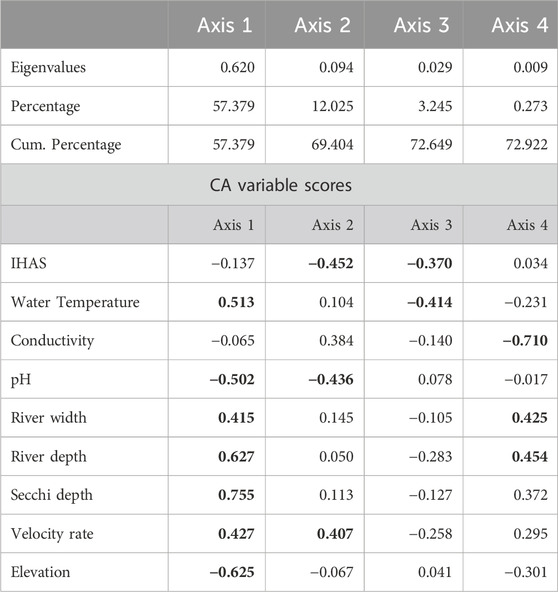
TABLE 7. Correlation coefficients based on macroinvertebrates metrics and physico-chemical variables and axes derived from CCA. Values in bold were considered important in structuring the sites.
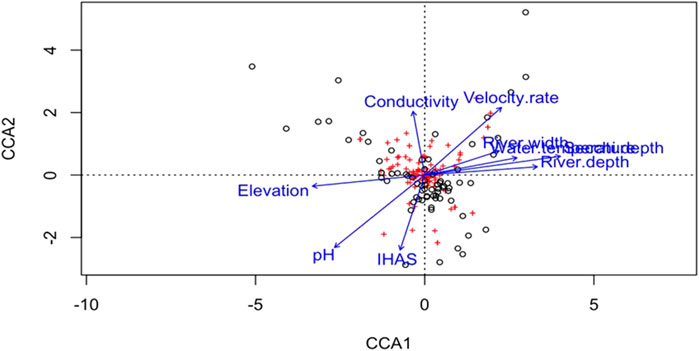
FIGURE 3. Result of CCA showing relationship between upstream and downstream sites based on macroinvertebrates metrics and physico-chemical variables. Open circles (o) depict site scores, while crosses (+) are macroinvertebrate families’ scores based on symmetric scaling. Variation explained: Axis 1 = 57.37%; Axis 2 = 12.02%; Axis 3 = 3.24%; and Axis 4 = 0.27%.
4 Discussions
4.1 Physico-chemical characteristics
We found that the upstream and downstream sections of the weirs had contrasting and unpredictable concentration of physico-chemical variables. In general, the results showed that in the upstream sections the physico-chemical variables were widely variable and therefore not largely associated, while in the downstream sites, the presence of the weir increased the similarity of most physico-chemical variables. The results of the physico-chemical variables of the current study are unlike the findings of Chaves-Ulloa et al. (2014) who reported high variation and extreme values of physico-chemical parameters downstream of the dam. This may be due to the size of weir and landuse type and extent on the upstream catchment as pointed out by Zaidel et al. (2021). Another reason may be that from a study by Kamidis et al. (2021) who noted that changes in physico-chemical variables vary with the volume of water flowing downstream of dams. Additionally, it is reported that in most cases damming of water is preventing the free passage of TDS to the downstream part (Stein et al., 2002; Kondolf and Yi, 2022). In the case of this study, an increase in physico-chemical parameters in the lower catchment due to land use such as agriculture and increased human access to rivers for various activities might have contributed to an increase in nutrient concentration, variation, and elevated values mostly measured downstream of the weirs.
High water temperatures at the upstream of Mutale, Matsika, and Xikundu could be attributed to loss of riparian vegetation that opened the canopy, resulting in direct heating from the sun as reported elsewhere (Wang et al., 2016; Zaidel et al., 2021). The high DO at the upstream sites of most of the weirs could be due to flow of water that created conditions of turbulence and recirculation, favouring their re-oxygenation at the water/air interface (Fernandes et al., 2014). The lower DO at downstream sites might be due to the decomposition of organic matter from soil and vegetation. The pH levels for all the sites were all within the recommended South African aquatic system pH ranges of 6–8 (DWAF, 1996). The higher EC values in the downstream of Lutanandwa, Xikundu and Mhinga could be due to increased loads of suspended substances from activities such as erosion and agricultural runoff that are also reported elsewhere (Adu and Oyeniyi, 2019), though the concentrations of EC at all the sites were within the recommended South African aquatic system for inland surface water (DWAF, 1996).
The results from this study further reflect the impacts of weirs on physico-chemical variables and this causes variation within these variables within and across sites. This study unravelled that most sampling points in the downstream sections of weirs had a clear trend for increased water temperature, pH, and EC. The Barotta, Lutanandwa, Xikundu and Mhinga weirs showed higher DO averages in the upstream than the downstream sections, whilst the Mutale and Matsika weirs do so in the downstream sections.
4.2 Aquatic macroinvertebrate assemblages
In general, the presence of weirs in the LRC had altered macroinvertebrate assemblages and abundance, while few sites such as the Lutanandwa, Mutale and Barotta weirs somewhat remained the same to a certain extent. These results indicate that weirs in mountainous areas have somewhat depicted only a moderate impact (low variation between upstream and downstream) compared to the weirs in the middle and lower reaches of the LRC. The similar behaviour of macroinvertebrate assemblages of these three sites (Lutanandwa, Mutale and Barotta) can be attributed to their less human activities and well vegetated streams. Riparian vegetation plays a crucial role in providing organic matter and structuring communities than other variables (Klonowska-Olejnik and Skalski, 2014; Mellado-Diaz et al., 2019; Gezie et al., 2023; Ramulifho et al., 2023), which might be the case in this study. However, in terms of variation at lower reaches, a related study by Bredenhand and Samways (2009) reported lower species richness downstream of the dam when compared to upstream section due to effect of the dam. These changes result in alteration of macroinvertebrate assemblages and their related functional services due to changes in stream flow rate, temperature, and oxygen. Weirs and dams, while important for human welfare cause ecologically cascading impact on biological organisms and other ecological services.
Four out of the six weirs (i.e., Lutanandwa, Barotta, Mhinga and Xikundu weirs) depicted high population of aquatic macroinvertebrates in the upstream sections rather than downstream sections. However, the Mutale and Matsika weirs showed the downstream sections as better-suited habitats to support a good population of aquatic macroinvertebrates despite the modification by these structures. Atique et al. (2020) argue that the alteration and disturbance activities in modified river systems impact the composition, structure, and function of aquatic macroinvertebrate communities, irrespective of their diversity and function. In general, the presence of weirs in the LRC have impact on macroinvertebrate assemblages and abundance in the downstream sections compared to the upstream sections. A related study by Krajenbrink et al. (2019) also reported that macroinvertebrate communities at monitoring sites downstream of the reservoirs differed from upstream sites in terms of abundance and community structure and was attributed to changes in flow.
5 Conclusion
This study aimed to assess the impacts of weirs on macroinvertebrate assemblages within the LRC by analysing physico-chemical water quality parameters. On physico-chemical water quality parameters, the findings revealed that the upstream sections, physico-chemical variables exhibited considerable variability and were not strongly correlated. Regarding macroinvertebrate assemblages, however, in the downstream sites, the presence of the weir enhanced the similarity of most physico-chemical variables with notable variations in the abundance and composition of macroinvertebrates between upstream and downstream sites of the weirs in the Luvuvhu River. Furthermore, assessments of SASS score, ASPT, IHAS, and physico-chemical variables in both sections of the weirs demonstrated significant differences. These variations are likely attributed to the presence of weirs, resulting in habitat integrity alterations, reduced flow velocity, and changes in turbidity levels.
Given the limited ecological information on weirs in the LRC, there is a pressing need for increased investment in macroinvertebrate assessment research, as these organisms serve as valuable indicators of environmental degradation. The study underscores the association between good biological and habitat conditions, emphasizing the importance of maintaining high water quality for enhanced macroinvertebrate assemblages and composition. Consequently, regular bioassessment of rivers and weirs in the LRC is crucial. It is therefore important to conduct regular bioassessment of rivers and weirs in the LRC. This study further highlights a need to improve, restore and preserve aquatic habitat quality and river connectivity in the upstream and downstream sections of weirs.
Data availability statement
The original contributions presented in the study are included in the article/Supplementary Material, further inquiries can be directed to the corresponding author.
Ethics statement
The manuscript presents research on animals that do not require ethical approval for their study.
Author contributions
HM: Conceptualization, Data curation, Formal Analysis, Investigation, Methodology, Project administration, Resources, Validation, Writing–original draft, Writing–review and editing. IB: Formal Analysis, Resources, Supervision, Validation, Writing–review and editing. AA-B: Software, Supervision, Validation, Writing–review and editing. PR: Formal Analysis, Resources, Software, Supervision, Validation, Visualization, Writing–review and editing. WL-P: Conceptualization, Funding acquisition, Project administration, Resources, Supervision, Validation, Writing–review and editing.
Funding
The author(s) declare financial support was received for the research, authorship, and/or publication of this article. This work is based on the research supported by the National Research Foundation of South Africa and the Department of Science and Innovation (Grant Number 101054; SARChI Chair in Ecosystem Health of the University of Limpopo). The research logistics and funds were also supported by the University of Venda, Biological Sciences Department and Directorate of Research and Innovation under the auspices of University Capacity Development Programme (UCDP) Cost Centre Number: D234.
Conflict of interest
The authors declare that the research was conducted in the absence of any commercial or financial relationships that could be construed as a potential conflict of interest.
Publisher’s note
All claims expressed in this article are solely those of the authors and do not necessarily represent those of their affiliated organizations, or those of the publisher, the editors and the reviewers. Any product that may be evaluated in this article, or claim that may be made by its manufacturer, is not guaranteed or endorsed by the publisher.
Supplementary material
The Supplementary Material for this article can be found online at: https://www.frontiersin.org/articles/10.3389/fenvs.2024.1308227/full#supplementary-material
References
Adu, B. W., and Oyeniyi, E. A. (2019). Water quality parameters and aquatic insect diversity in Aahoo stream, southwestern Nigeria. J. Basic Appl. Zoology 80 (15), 15–19. doi:10.1186/s41936-019-0085-3
Atique, U., Kwon, S., and An, K.-G. (2020). Linking weir imprints with riverine water chemistry, microhabitat alterations, fish assemblages, chlorophyll-nutrient dynamics, and ecological health assessments. Ecol. Indic. 117, 106652. doi:10.1016/j.ecolind.2020.106652
Belmar, o., Velasco, J., Gutierrez-Canovas, C., Mellado-Diaz, A., Millan, A., and Wood, P. J. (2012). The influence of natural flow regimes on macroinvertebrate assemblages in a semiarid Mediterranean basin. Ecohydrology 6 (3), 363–379. doi:10.1002/eco.1274
Bowers, D. G., Roberts, E. M., Hoguane, A. M., Fall, K. A., Massey, G. M., and Friedrichs, C. T. (2020). Secchi disk measurements in turbid water. J. Geophys. Res. Oceans 125, e2020JC016172. doi:10.1029/2020jc016172
Bredenhand, E., and Samways, M. J. (2009). Impact of a dam on benthic macroinvertebrates in a small river in a biodiversity hotspot: cape Floristic Region, South Africa. J. Insect Conservation 13 (3), 297–307. doi:10.1007/s10841-008-9173-2
Brooks, A. J., Wolfenden, B., Downes, B. J., and Lancaster, J. (2018). Barriers to dispersal: the effect of a weir on stream insect drift. River Res. Appl. 34 (10), 1244–1253. doi:10.1002/rra.3377
Brown, J. H. (2014). Why are there so many species in the tropics? J. Biogeogr. 41 (1), 8–22. doi:10.1111/jbi.12228
Bunn, S. E., and Arthington, A. H. (2002). Basic principles and ecological consequences of altered flow regimes for aquatic biodiversity. Environ. Manag. 30, 492–507. doi:10.1007/s00267-002-2737-0
Cantonati, M., Poikane, S., Pringle, C. M., Stevens, L. E., Turak, E., Heino, J., et al. (2020). Characteristics, main impacts, and stewardship of natural and artificial freshwater environments: consequences for biodiversity conservation. Water 12 (1), 260–285. doi:10.3390/w12010260
Carpenter-Bundhoo, L., Butler, G. L., Bond, N. R., Bunn, S. U., Reinfelds, I. V., and Kennard, M. J. (2020). Effects of a low-head weir on multi-scaled movement and behavior of three riverine fish species. Sci. Rep. 10, 6817. doi:10.1038/s41598-020-63005-8
Carpenter-Bundhoo, L., Butler, G. L., Bond, N. R., Thiem, J. D., Bunn, S. U., and Kennard, M. J. (2023). Fish movements in response to environmental flow releases in intermittent rivers. Freshw. Biol. 68, 260–273. doi:10.1111/fwb.14022
Casserly, C. M., Turner, J. N., O’Sullivan, J. J., Bruen, M., Bullock, C., Atkinson, S., et al. (2020). Impact of low-head dams on bedload transport rates in coarse-bedded streams. Sci. Total Environ. 716, 136908. doi:10.1016/j.scitotenv.2020.136908
Chaves-Ulloa, R., Umaña-Villalobos, G., and Springer, M. (2014). Downstream effects of hydropower production on aquatic macroinvertebrate assemblages in two rivers in Costa Rica. Rev. Biol. Trop. 62 (2), 179–201. doi:10.15517/rbt.v62i0.15787
Cheimonopoulou, M. T., Bobori, D. C., Theocharopoulos, I., and Lazaridou, M. (2011). Assessing ecological water quality with macroinvertebrates and fish: a case study from a small Mediterranean River. Environ. Manag. 47, 279–290. doi:10.1007/s00267-010-9598-8
Chutter, F. M. (1998). Research on the rapid biological Assessment of water quality Impacts in Streams and rivers. WRC Report No. 422/1/98. Pretoria, South Africa: Water Research Commission.
Conradie, D. C. U. (2012). “South Africa`s climate zones: today, tomorrow,” in International green building conference and exhibition; future trends and issues impacting on the built environment (Sandton: CSIR).
Dallas, H. F. (2007). “River health Programme: South African scoring system (SASS) data interpretation guidelines,” in Prepared for the institute of natural resources and the resource quality services river health department of water affairs and forestry (Cape Town, South Africa: The Freshwater Consulting Group).
Dallas, H. F. (2021). Rapid bioassessment protocols using aquatic macroinvertebrates in Africa – considerations for regional adaptation of existing biotic indices. Front. Water 3, 628227. doi:10.3389/frwa.2021.628227
Dewson, Z. S., James, A. B. W., and Death, R. G. (2007). A review of the consequences of decreased flow for instream habitat and macroinvertebrates. J. North Am. Benthol. Soc. 26, 401–415. doi:10.1899/06-110.1
Dickens, C. W. S., and Graham, P. M. (2002). The South African Scoring System (SASS) version 5 rapid bioassessment method for rivers. Afr. J. Aquatic Sci. 27, 1–10. doi:10.2989/16085914.2002.9626569
DWAF (Department of Water Affairs and Forestry) (1996). South African water quality guidelines volume 7. Pretoria: Aquatic Ecosystems.
Fencl, J. S., Mather, M. E., Costigan, K. H., and Daniels, M. D. (2015). How big of an effect do small dams have? Using geomorphological footprints to quantify spatial impact of low-head dams and identify patterns of across-dam variation. PLoS ONE 10 (11), e0141210. doi:10.1371/journal.pone.0141210
Fernandes, J. D. F., De Souza, A. T. L., and Tanaka, M. O. (2014). Can the structure of a riparian forest remnant influence stream water quality? A tropical case study. Hydrobiologia 724, 175–185. doi:10.1007/s10750-013-1732-1
Fouche, P. S. O. (2009). Aspects of the ecology and biology of the lowveld largescale yellowfish (labeobarbus marequensis, smith, 1843) in the Luvuvhu River, Limpopo river system, South Africa. PhD Thesis. South Africa: University of Limpopo.
Fry, C. (2021). A field guide to freshwater macroinvertebrates of Southern Africa. South Africa: Jacana Media (Pty) Ltd.
Gerber, A., and Gabriel, M. J. M. (2002). Aquatic invertebrates of South African rivers field guide. Pretoria: Institute of Water Quality Studies, Department of Water Affairs and Forestry.
Gezie, A., Goshu, G., and Tiku, S. (2023). Ecological effect of a small dam on the macroinvertebrate assemblage and water quality of Koga River, Northwest Ethiopia. Heliyon 9 (6), e17285. doi:10.1016/j.heliyon.2023.e17285
Grill, G., Lehner, B., Thieme, M., Geenen, B., Tickner, D., Antonelli, F., et al. (2019). Mapping the world’s free-flowing rivers. Nature 569, 215–221. doi:10.1038/s41586-019-1111-9
Hough, I. M., Warren, P. H., and Shucksmith, J. D. (2019). Designing an environmental flow framework for impounded river systems through modelling of invertebrate habitat quality. Ecol. Indic. 106, 105445. doi:10.1016/j.ecolind.2019.105445
Hu, X., Zuo, D., Xu, Z., Huang, Z., Liu, B., Han, Y., et al. (2022). Response of macroinvertebrate community to water quality factors and aquatic ecosystem health assessment in a typical river in Beijing, China. Environ. Res. 212, 113474. doi:10.1016/j.envres.2022.113474
Hughes, D. A., and Mantel, S. K. (2010). Estimating the uncertainty in simulating the impacts of small farm dams on streamflow regimes in South Africa. Hydrological Sci. J. 55 (4), 578–592. doi:10.1080/02626667.2010.484903
Im, D., Kang, H., Kim, K.-H., and Choi, S.-U. (2011). Changes of river morphology and physical fish habitat following weir removal. Ecol. Eng. 37 (6), 883–892. doi:10.1016/j.ecoleng.2011.01.005
Jager, H. I., Chandler, J. A., Lepla, K. B., and Van Winkle, W. (2001). A theoretical study of river fragmentation by dams and its effects on white sturgeon populations. Environmetal Biol. Fishes 60, 347–361. doi:10.1023/a:1011036127663
Jamil, T., and ter Braak, C. J. F. (2013). Generalized linear mixed models can detect unimodal species-environment relationships. PeerJ 1 (e95), e95–e14. doi:10.7717/peerj.95
Janse van Vuuren, S., and Swanepoel, A. (2023). Role of the lethabo weir in altering the phytoplankton community structure of the vaal river, South Africa. Afr. J. Aquatic Sci. 48 (3), 300–314. doi:10.2989/16085914.2023.2231513
Jumani, S., Deitch, M. J., Kaplan, D., Anderson, E. P., Krishnaswamy, J., Lecours, V., et al. (2020). River fragmentation and flow alteration metrics: a review of methods and directions for future research. Environ. Res. Lett. 15, 123009. doi:10.1088/1748-9326/abcb37
Kamidis, N., Koutrakis, E., Sapounidis, A., and Sylaios, G. (2021). Impact of river damming on downstream hydrology and hydrochemistry: the case of lower nestos River catchment (NE. Greece). Water 13, 2832. doi:10.3390/w13202832
Klonowska-Olejnik, M., and Skalski, T. (2014). The effect of environmental factors on the mayfly communities of headwater streams in the Pieniny Mountains (West Carpathians). Biologia 69 (4), 498–507. doi:10.2478/s11756-014-0334-3
Kondolf, M., and Yi, J. (2022). Dam renovation to prolong reservoir life and mitigate dam impacts. Water 14 (9), 1464–1523. doi:10.3390/w14091464
Krajenbrink, H. J., Acreman, M., Dunbar, M. J., Hannah, D. M., Laize, C. L. R., and Wood, P. J. (2019). Macroinvertebrate community responses to river impoundment at multiple spatial scales. Sci. Total Environ. 650, 2648–2656. doi:10.1016/j.scitotenv.2018.09.264
Kundu, P. M., Mathivha, F. I., and Nkuna, R. N. (2015). The use of GIS and remote sensing techniques to evaluate the impact of land use and land cover change on the hydrology of Luvuvhu River catchment in Limpopo province. Water research commission. WRC Report No. 2246/1/15: 1 – 154.
Leitner, P., Borgwardt, F., Birk, S., and Graf, W. (2021). Multiple stressor effects on benthic macroinvertebrates in very large European rivers – a typology-based evaluation of faunal responses as a basis for future bioassessment. Sci. Total Environ. 756, 143472. doi:10.1016/j.scitotenv.2020.143472
Leps, P., and Smilauer, P. (2003). Advanced use of ordination multivariate analysis of ecological data using CANOCO (1st). Cambridge: Cambridge University Press, 140–148.
Li, S., Yang, W., Wang, L., Chen, K., Xu, S., and Wang, B. (2018). Influences of environmental factors on macroinvertebrate assemblages: differences between mountain and lowland ecoregions, Wei River, China. Environ. Monit. Assess. 190 (152), 152–213. doi:10.1007/s10661-018-6516-7
Linares, M. S., Assis, W., de Castro Solar, R. R., Leitao, R. P., Hughes, R. M., and Callisto, M. (2019). Small hydropower dam alters the taxonomic composition of benthic macroinvertebrate assemblages in a neotropical river. River Res. Appl. 35, 725–735. doi:10.1002/rra.3442
Mantel, S. K., Muller, N. W. J., and Hughes, D. A. (2010). Ecological impacts of small dams on South African rivers Part 2: biotic-response – abundance and composition of macroinvertebrates communities. Water SA 36 (3), 361–370.
McMillan, P. H. (1998). “An integrated habitat assessment system (IHAS Version 2) for the rapid biological assessment of rivers and streams,” in CSIR research Report No. ENV-P-I 98132, water resources management Programme (Pretoria: Council for Scientific and Industrial Research).
Mellado-Diaz, A., Sanchez-Gonzalez, R., Guareschi, S., Magdaleno, F., and Velasco, M. T. (2019). Exploring longitudinal trends and recovery gradients in macroinvertebrate communities and biomonitoring tools along regulated rivers. Sci. Total Environ. 695, 133774. doi:10.1016/j.scitotenv.2019.133774
Mueller, M., Pander, J., and Geist, J. (2011). The effects of weirs on structural stream habitat and biological communities. J. Appl. Ecol. 48, 1450–1461. doi:10.1111/j.1365-2664.2011.02035.x
Munasinghe, D. S. N., Najim, M. M. M., Quadroni, S., and Musthafa, M. M. (2021). Impact of streamflow alteration on benthic macroinvertebrates by mini-hydro diversion in Sri Lanka. Sci. Rep. 11 (546), 1–12. doi:10.1038/s41598-020-79576-5
Nakagawa, S., and Schielzeth, H. (2013). A general and simple method for obtaining R2 from generalized linear mixed-effects models. Methods Ecol. Evol. 4 (2), 133–142. doi:10.1111/j.2041-210x.2012.00261.x
Nkuna, T. R., and Odiyo, O. J. (2011). Filling of missing rainfall data in Luvuvhu River Catchment using artificial neural networks. Phys. Chem. Earth, Part A/B/C 36 (14–15), 830–835. doi:10.1016/j.pce.2011.07.041
Odiyo, J. O., Makungo, R., and Nkuna, T. R. (2015). Long-term changes and variability in rainfall and streamflow in Luvuvhu River Catchment, South Africa. South Afr. J. Sci. 111 (7/8), 9. doi:10.17159/sajs.2015/20140169
Poff, N. L. (2018). Beyond the natural flow regime? Broadening the hydro-ecological foundation to meet environmental flows challenges in a non-stationary world. Freshw. Biol. 63 (8), 1011–1021. doi:10.1111/fwb.13038
Poff, N. L., and Zimmerman, J. K. (2010). Ecological responses to altered flow regimes: a literature review to inform the science and management of environmental flows. Freshw. Biol. 55 (1), 194–205. doi:10.1111/j.1365-2427.2009.02272.x
Power, M. E., Parker, G., Dietrich, W. E., and Wootton, J. T. (1995). Hydraulic food-chain models. Bioscience 45, 159–167. doi:10.2307/1312555
Principe, R. E. (2010). Ecological effects of small dams on benthic macroinvertebrate communities of mountain streams (Cordoba, Argentina). Int. J. Limnol. 46, 77–91. doi:10.1051/limn/2010010
Ramulifho, P., Ndou, E., Thifhulufhelwi, R., and Dalu, T. (2019). Challenges to implementing an environmental flow regime in the Luvuvhu River catchment, South Africa. Int. J. Environ. Res. Public Health 16, 3694. doi:10.3390/ijerph16193694
Ramulifho, P., Rivers-Moore, N., and Foord, S. (2023). Relationships between reference site quality and baetid mayfly assemblages in mountainous streams of the Luvuvhu catchment, South Africa. Water SA 49 (3), 251–259. doi:10.17159/wsa/2023.v49.i3.4023
R Development Core Team (2023). R: a language and environment for statistical computing. Vienna, Austria: R Foundation for Statistical Computing.
Schneider, S., and Petrin, Z. (2017). Effects of flow regime on benthic algae and macroinvertebrates – a comparison between regulated and unregulated rivers. Sci. Total Environ. 579, 1059–1072. doi:10.1016/j.scitotenv.2016.11.060
Seliger, C., and Zeiringer, B. (2018). “River connectivity, habitat fragmentation and related restoration measures,” in Riverine ecosystem management aquatic ecology series, 8. Editors S. Schmutz, and J. Sendzimir (Cham: Springer), 171–186.
State of Rivers Report (SoRR) (2001). South African river health Programme Letaba and Luvuvhu River systems WRC Report No. TT 165/01. Pretoria, South Africa: Water Research Commission.
Stein, J. L., Stein, J. A., and Nix, H. A. (2002). Spatial analysis of anthropogenic river disturbance at regional and continental scales: identifying the wild rivers of Australia. Landsc. Urban Plan. 60, 1–25. doi:10.1016/s0169-2046(02)00048-8
Ter Braak, C. J. F., and Verdonschot, P. F. M. (1995). Canonical correspondence analysis and related multivariate methods in aquatic ecology. Aquatic Sci. 57, 255–289. doi:10.1007/bf00877430
Venables, W. N., and Ripley, B. D. (2002). Modern applied statistics with S. Fourth Edition. New York: Springer, 183–208.
Wang, H., Chen, Y., Liu, Z., and Zhu, D. (2016). Effects of the “Run-of River” hydro scheme on macroinvertebrate communities and habitat conditions in a Mountain River of Northeastern China. Water 8 (31), 31–16. doi:10.3390/w8010031
Wang, J., Ding, C., Heino, J., Jiang, X., Tao, J., Ding, L., et al. (2020). What explains the variation in dam impacts on riverine macroinvertebrates? A global quantitative synthesis. Environ. Res. Lett. 15, 124028. doi:10.1088/1748-9326/abc4fc
Wessels, P., and Rooseboom, A. (2009). Flow-gauging structures in South African rivers: Part 1: an overview. Water SA 35, 1–10. doi:10.4314/wsa.v35i1.76643
Winemiller, K. O., McIntyre, P. B., Castello, L., Fluet-Chouinard, E., Glarrizzo, T., Nam, S., et al. (2016). Balancing hydropower and biodiversity in the amazon, Congo, and mekong. Science 351, 128–129. doi:10.1126/science.aac7082
Keywords: flow modification, habitat integrity, Luvuvhu River catchment, macroinvertebrate assemblages, weirs
Citation: Munzhelele HE, Barnhoorn IEJ, Addo-Bediako A, Ramulifho PA and Luus-Powell WJ (2024) Impact of weirs in altering benthic macroinvertebrate assemblages and composition structure in the Luvuvhu River Catchment, South Africa. Front. Environ. Sci. 12:1308227. doi: 10.3389/fenvs.2024.1308227
Received: 06 October 2023; Accepted: 06 February 2024;
Published: 20 February 2024.
Edited by:
Paweł Tomczyk, Wroclaw University of Environmental and Life Sciences, PolandReviewed by:
Marta Leiva, National University of Santiago del Estero, ArgentinaRenan De Souza Rezende, Regional Community University of Chapecó, Brazil
Augustine Ovie Edegbene, Federal University of Health Sciences Otukpo, Nigeria
Copyright © 2024 Munzhelele, Barnhoorn, Addo-Bediako, Ramulifho and Luus-Powell. This is an open-access article distributed under the terms of the Creative Commons Attribution License (CC BY). The use, distribution or reproduction in other forums is permitted, provided the original author(s) and the copyright owner(s) are credited and that the original publication in this journal is cited, in accordance with accepted academic practice. No use, distribution or reproduction is permitted which does not comply with these terms.
*Correspondence: Humbulani E. Munzhelele, ZWRkaHVtYnVAZ21haWwuY29t