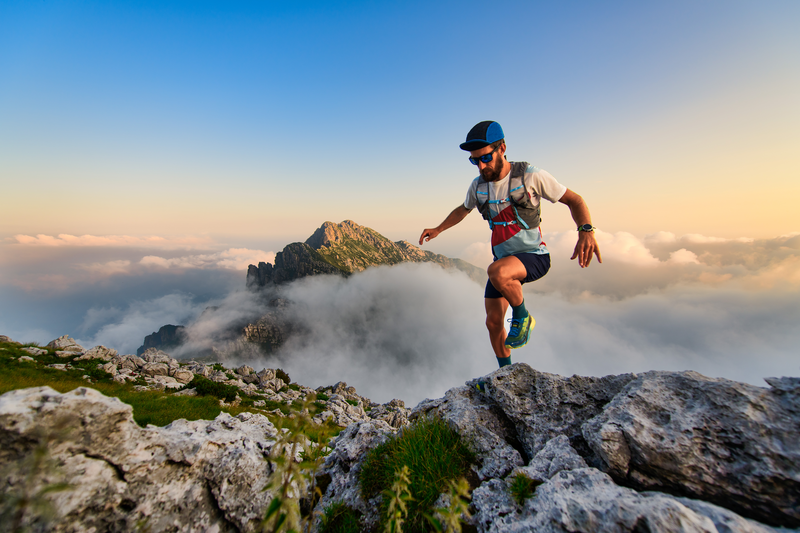
94% of researchers rate our articles as excellent or good
Learn more about the work of our research integrity team to safeguard the quality of each article we publish.
Find out more
PERSPECTIVE article
Front. Environ. Sci. , 02 February 2024
Sec. Toxicology, Pollution and the Environment
Volume 12 - 2024 | https://doi.org/10.3389/fenvs.2024.1307631
This article is part of the Research Topic Remediation and Health Risks of Heavy Metal Contaminated Soils View all 14 articles
The enhanced phytoremediation of metal contaminated soils holds great promise for the recovery of soil health and functionality, while providing a range of co-benefits, from an environmental and human health perspective, derived from the revegetation of the degraded sites and the concomitant delivery of ecosystem services. Due to diverse evolutionary co-selection mechanisms between metal resistance and antibiotic resistance in bacteria, metal contaminated soils are considered potential reservoirs of antibiotic resistant bacteria (ARB) which can contribute to the existing antibiotic resistance crisis. During the enhanced phytoremediation of metal contaminated soils, the application of organic wastes (e.g., manure, slurry, sewage sludge) as soil amendments can aggravate the risk of antibiotic resistance spread, because they often contain ARB which harbor antibiotic resistance genes (ARGs) that can then be propagated among soil bacterial populations through horizontal gene transfer (HGT). Due to the magnitude and criticality of the antibiotic resistance crisis, as well as the higher risk of spread and dispersal of ARB and ARGs (they make copies of themselves) compared to metals, it is proposed here to aim enhanced phytoremediation strategies towards decreasing the soil resistome (and, hence, the risk of its potential link with the human resistome), while reducing total and/or bioavailable metal concentrations and restoring soil health and the delivery of ecosystem services. To this purpose, a decalogue of practices is tentatively suggested. Finally, a proper management of plant and soil microbial compositions is a most crucial aspect, together with the selection of the right organic wastes and phytoremediation practices.
Metal phytoremediation strategies have important drawbacks that are hindering their commercial use (Wang and Delavar, 2023). Phytoextraction can reduce total metal concentrations, but it requires unacceptably long times to decrease such concentrations below regulatory limits (Tang, 2023). The main limitation of phytostabilisation is that it does not reduce total metal concentrations in soil, as it is based on the use of excluders that reduce metal bioavailability, but not total concentrations. Besides, phytoremediation is limited to the surface area and depth occupied by the roots and can increase the risk of metal accumulation up the trophic chain (El Rasafi et al., 2023). In an attempt to overcome these limitations, several enhanced phytoremediation strategies have been proposed. Thus, inorganic and organic amendments are used to assist the revegetation of metal contaminated soils (Moreira et al., 2021). Compared to inorganic amendments, organic amendments have certain advantages: high accessibility, low costs, reuse of wastes, increase of soil organic matter, supply of nutrients, improvement of soil structure, enhancement of microbial activity (which can then help control soilborne pathogens) (Janvier et al., 2007; Núñez-Zofío et al., 2011), etc. Another strategy, microbial-assisted phytoremediation, is based on the inoculation of plant growth-promoting bacteria (PGPB) (Waseem et al., 2024) and/or fungi (Ahmad et al., 2024), often as consortia, to facilitate plant growth and performance under the harsh conditions that characterize contaminated soils (Alkorta et al., 2017; Alkorta and Garbisu, 2021). The presence of fungi can promote bacterial distribution as they are known to use fungal hyphae to disperse in the soil under unsaturated conditions (Kohlmeier et al., 2005). Likewise, the combination of amendments, plants (phytoremediation), microorganisms (bioremediation), and earthworms (vermiremediation) is also promising for soil remediation (Lacalle et al., 2020). Interestingly, the phytoremediation field has recently shifted towards phytomanagement (Moreira et al., 2021), a concept focused on the use of plants to generate products and ecosystem services while remediating a contaminated soil.
Metal contaminated sites can conceal other risks different from those associated to metal toxicity. Relevantly, metal contaminated soils can be reservoirs of antibiotic resistant bacteria (ARB) and antibiotic resistance genes (ARGs) (Cai et al., 2023; Song et al., 2024). Due to the criticality of the antibiotic resistance crisis and the higher risk of spread and dispersal of ARB and ARGs, compared to metals, it is proposed here to aim enhanced phytoremediation strategies towards decreasing the soil resistome, while reducing metal concentrations and restoring soil health. This antibiotic resistance-centered approach to metal phytoremediation could promote its commercial use and interest for soil managers. Several papers can be found in the literature on i) the biological remediation of antibiotic contaminated soils, e.g., removal of antibiotics via bioremediation or microbial-assisted phytoremediation; or 2) the presence of ARB and ARGs in metal contaminated soils due to co-selection mechanisms. But, to our knowledge, the abovementioned proposal is a novel proposal.
In order to attract attention to a field of knowledge, it is convenient to point out its links with human health and/or wellbeing. Metals can be toxic to humans and, as such, they have always attracted our attention from a human health perspective (Tian et al., 2023). But, although metal toxicity by itself is more than enough reason to take the remediation of metal contaminated sites very seriously, to this risk, we must add the fact that these sites have been linked to one of the greatest threats to public health: the emergence and dissemination of ARB harboring ARGs (Edet et al., 2023).
Given the criticality of the antibiotic resistance crisis, the links between the soil resistome and the human resistome is currently a topic of much interest (Liao et al., 2022). The selective pressure that the presence of antibiotics may exert on ARB proliferation in soil is of particular concern, as soil contains a number of bacterial genera of clinical relevance (Serwecińska, 2020). Much interest exists on the relationship between the use of organic amendments of animal (manure, slurry) and/or urban (sewage sludge) origin as fertilizers and the soil resistome (Jauregi et al., 2021a; Jauregi et al., 2021b; Jauregi et al., 2023). Manure is acknowledged as a reservoir of ARB and ARGs (Zhu et al., 2013), which can be disseminated in the environment through horizontal gene transfer (HGT) among bacteria mediated by mobile genetic elements (MGEs) (Heuer et al., 2011; Urra et al., 2019). Once in the soil, the ARB initially present in the manure can die or survive and then inhabit the abiotic and/or biotic component of the soil matrix. The presence of antibiotics and their transformation products in the amendments can alter the composition of soil microbial communities with consequences for the soil resistome and mobilome (Gillings, 2013; Nesme and Simonet, 2015).
The link between metal contamination and AR is based on the fact that metal contamination can lead to the spread of AR through co-selection mechanisms, such as co-resistance (several resistance systems in the same genetic element), cross-resistance (one resistance system confers resistance to both a metal and an antibiotic), and co-regulation (when resistance to antibiotics and metals are controlled by a single regulatory gene) (Baker-Austin et al., 2006; Wales and Davies, 2015; Ohore et al., 2019). Due to the AR crisis and the degree of metal contamination in many environmental matrices, much attention is being paid to the study of the role of metal contamination as selective agent in the proliferation of environmental antibiotic resistance (Jauregi et al., 2021b). For instance, ARGs can be found in metal contaminated mines (Garbisu et al., 2018). Importantly, owing to the long residence times of metals in soil, they represent a recalcitrant selection pressure on ARB.
Many metal contaminated sites are not being remediated for economic and/or technical reasons. In an attempt to find ways to encourage the remediation of metal contaminated soils, here it is proposed to direct enhanced phytoremediation strategies to reduce the risk of the environmental resistome present in metal contaminated soils. This novel proposal is based on three facts: 1) soil is one of our most important resources, and as such we must do our best to restore its functionality when degraded; ii) the link between the soil resistome and the human resistome is a threat to the effectiveness of antibiotics in medicinal practice and we must do over best to disrupt such link; and iii) there is a higher risk of spread and dispersal of ARB and ARGs (they make copies of themselves), compared to metals. It is crucial to design enhanced phytoremediation practices taking into consideration the possibility to reduce the risk of antibiotic resistance in the metal contaminated site under remediation, as well as its propagation to other environmental matrices, while decreasing total and/or bioavailable metal concentrations, restoring soil functioning, and providing ecosystem services.
The following is a brief description of practices, in the form of a tentative decalogue (Figure 1), that must be taken into consideration during the implementation of enhanced phytoremediation strategies in order to minimize the antibiotic resistance risk, while addressing the risks associated with the presence of metals.
(1) Selection and pretreatment of organic amendments: it is desirable to select those amendments that have a lower content of metals and antibiotics (as well as of ARB, ARGs, and MGEs). Although, in most cases, a thorough analysis of the composition of the amendments is not available (especially regarding the content of antibiotics, not to mention the abundance of ARB, ARGs, or MGEs), from the origin of the amendments (urban versus animal origin, manure from one livestock species versus another, manure from an ecological versus an industrial farm, etc.), it is possible to estimate which ones represent a lower risk. However, the most important factor is probably the type of pretreatment applied to the amendment prior to its use: storage, composting, anaerobic digestion, etc. It is crucial to opt for amendments that have been properly treated for hygienisation purposes or composted. Manure pretreatment, by means of composting or anaerobic digestion, can reduce the burden of ARB and ARGs, as well as destroy antibiotic residues (Tran et al., 2021; Wang et al., 2021). Although neither composting nor storage completely eliminate the risk of antibiotic resistance, composting is normally more effective (Zalewska et al., 2023). A thermal hydrolysis pre-treatment combined with anaerobic digestion has been reported to reduce the abundance of ARGs and MGEs in sewage sludge (Sun et al., 2019). The use of exogenous additives, such as nanomaterials, during manure composting has been shown to reduce the ARG abundances (Jiang et al., 2023). Jiang et al. (2023) reported the effectiveness of the addition of SiO2 nanoparticles during manure composting to decrease the propagation of ARGs. Nnorom et al. (2023) reviewed the use of carbon- and iron-based conductive materials (biochar, activated carbon, zerovalent iron) as additives to mitigate the proliferation of ARGs during the anaerobic digestion of sludge and manure, and concluded that they can decrease ARG abundances in the digestate by easing selective pressure, changing microbial community structure, and diminishing HGT.
(2) Application of inorganic amendments to reduce metal bioavailability: another strategy to minimize the risk of co-selection between metal and antibiotic resistance is to decrease the levels of bioavailable metals in soil. A well-known strategy is the application of lime to increase soil pH and, hence, reduce bioavailable concentrations. Many other amendments (iron oxides, phosphates, ashes, etc.) have been used for chemical stabilization in metal contaminated soils (Kumpiene et al., 2019). When dealing with metal phytoremediation, one must decide whether to mobilize or immobilize the metals (Bolan et al., 2014), since mobilizing agents (chelating agents, desorbing agents) enhance plant metal uptake but at the same time increase the risk of metal leaching, while immobilizing agents (precipitating agents, sorbent materials) reduce both metal transfer to the food chain and leaching but require monitoring and management to guarantee the long-term stability of the immobilized metals.
(3) Planting metal excluders to reduce metal bioavailability: phytostabilisation with excluders is focused on the reduction of bioavailable concentrations, so that metal toxicity and mobility are decreased (Moreira et al., 2021). The level of bacterial exposure to metals is decreased and, concomitantly, the selection pressure to become tolerant to those metals, minimizing the appearance of antibiotic resistance via co-selection.
(4) Application of biochar to reduce the soil resistome: apart from the use of biochar aimed at modifying soil properties, including bioavailable concentrations (Moreira et al., 2021), much research has been done on the effect of biochar on the soil resistome (Cheng et al., 2021). Biochar has been reported to reduce the accumulation of ARGs in soil and their transfer to crops, among other reasons, due to the biochar´s ability to decrease, through sorption, the mobility and bioavailability of antibiotics and metals, as well as to its effect on microbial community composition derived from the induced changes on soil physicochemical properties (Ye et al., 2016; Duan et al., 2017; Chen et al., 2018; He et al., 2019; Cheng et al., 2021). Field aging can modify the biochar’s effect on the resistome of manured soil (Cheng et al., 2021). Finally, the addition of biochar during composting can accelerate antibiotics removal and reduce the accumulation of ARGs (Tong et al., 2022).
(5) Application of plant growth-promoting bacteria: the application of PGPB is becoming common practice during the phytoremediation of contaminated soils (Moreira et al., 2021). The application to soil of ecologically competitive PGPB could, theoretically speaking, outcompete the ARB present in the soil via, for instance, competition for resources and space. Antibiotic resistant bacteria introduced via the application of amendments can be viewed as invasive species and, as such, the possibility of their control by means of the introduction of highly competitive equivalents comes out as a potentially suitable strategy to reduce the risk of their dissemination. Biodiversity, and in particular soil biodiversity, can control invasive species (Bach et al., 2020). The generation of soil niches for potential microbial competitors of ARB derived from aboveground plant richness also emerges as a possibility for the control of ARB. It is important to emphasize that only antibiotic-susceptible PGPB must be used because PGPB often harbor ARGs (Mahdi et al., 2021).
(6) Bioaugmentation with antibiotic degraders: apart from the risk of the introduction of antibiotics to soil via organic amendments, many soil microbial species (particularly, actinobacteria and fungi) naturally produce antibiotics for competition purposes or as signalling molecules that facilitate intra- or interspecies interactions (Romero et al., 2011). Microorganisms have produced antibiotics for millions of years and, then, ARGs are often isolated from pristine environments, not subjected to antibiotic residues derived from human usage (Bhullar et al., 2012). In metal contaminated soils subjected to enhanced phytoremediation, this natural resistome must be added to the metal-derived resistome and the manured-associated resistome. The presence of natural or exogenous antibiotics in the soil causes a selective pressure on exposed bacteria to become resistant. The possibility of antibiotic bioremediation via bioaugmentation with microbial antibiotic degraders (Hong et al., 2020) can be a suitable option to decrease the risk of antibiotic resistance dissemination in soils under remediation. Apart from bacterial degraders, fungal-based remediation (mycoremediation) shows great potential for the removal of antibiotics (Čvančarová et al., 2015; Olicón-Hernández et al., 2017; Jalali et al., 2023). Interestingly, fungal hyphae serve as vectors for bacteria to travel across the soil, the so-called hyphal highways along which bacteria can swim in the water film that coats the hyphae or, alternatively, travel passively by settling at the tip of the growing hyphae (Guennoc et al., 2018).
(7) Increase plant richness to generate niches belowground: when ARB enter the soil during the application of manure, they can be regarded as invasive species whose success will rely on abiotic and biotic factors, such as, for instance, their ecological fitness, ecological valence under those conditions, dispersal ability, capacity for acclimation and adaptation, etc. Chen et al. (2019), in a study on the possibility of using microbial diversity against ARB invasion, reported that soil microbial diversity was negatively correlated with ARG abundance and concluded that a high soil microbial diversity works as a barrier against antibiotic resistance dissemination. Plant richness has been linked to belowground microbial diversity, probably due to the generation of niches derived from diverse litter types and root exudates (Wardle et al., 2004). In principle, the implementation of high plant richness aboveground (different species of grasses, shrubs, trees, and at different stages of growth and development) should increase the number of niches belowground and, concomitantly, a high diversity of microorganisms that could limit the growth of ARB or outcompete them. Besides, the promotion of biodiversity under phytoremediation has been reported to have many benefits (Garbisu et al., 2020).
(8) Establish a vegetation cover to control soil erosion: to avoid the dispersal of soil ARB to other environmental compartments (e.g., air, water), it is critical to establish a permanent full vegetation cover to minimize the risk of such dispersal through erosion. On the other hand, erosion has been reported to reduce soil microbial diversity (Qiu et al., 2021).
(9) Introduction of earthworms to reduce antibiotic resistance risk: although still a controversial issue, there are quite a few studies that have found a decrease in soil ARG abundances in the presence of earthworms. Li et al. (2022) found that earthworms can reduce the dissemination potential of ARGs by changing bacterial co-occurrence patterns in soil. Zhu et al. (2021) observed that the presence of earthworms decreased the abundance of ARGs in soils, suggesting that vermiremediation could be a suitable method to reduce risks associated to the presence of ARGs in soils. During vermicomposting of sewage sludge, earthworms have shown to decrease ARG abundance (Huang et al., 2020).
(10) Avoid or minimize the use of pesticides during phytoremediation: during phytoremediation, pesticides are often used to control weeds in the early stages of site management (Kidd et al., 2015). But herbicide use has been shown to be positively correlated with antibiotic resistance (Kurenbach et al., 2018; Xu et al., 2019), among other reasons, possibly due to changes in herbicide-mediated gene expression, leading to activation of bacterial ARGs (Staub et al., 2012). Liao et al. (2021) reported that the application of glyphosate, glufosinate, and dicamba increased the abundance of ARGs and MGEs in soil, without changes in the abundance or diversity of bacterial communities. Moreover, herbicide exposure increased conjugation frequency of multidrug resistance plasmids, thus promoting ARG spread among bacterial populations (Liao et al., 2021).
FIGURE 1. Tentative decalogue of practices that must be taken into account during the enhanced phytoremediation of metal contaminated soil in order to minimize the risk of antibiotic resistance.
Metal contaminated soils can be a reservoir of ARB and ARGs. The application of organic amendments during enhanced phytoremediation, as well as the inoculation of PGPB, can aggravate this problem. Here, it is proposed to aim enhanced phytoremediation strategies towards decreasing the soil resistome, while reducing total and/or bioavailable metal concentrations and restoring soil health and the delivery of ecosystem services. To this purpose, a decalogue of practices has been tentatively suggested. Nonetheless, it must be emphasized that the soil is a highly complex, heterogeneous, and dynamic ecosystem, whose functioning is still greatly unknown, and then one must be extremely cautious when proposing specific soil practices or measures as, quite often, they do not have the expected outcome or even show the opposite effect. In consequence, it is crucial to first test the suitability of the ten practices proposed here under the specific edaphoclimatic and contamination conditions of the site of interest. The ultimate goal of this perspective article has been to bring attention to the antibiotic resistance problem in metal contaminated soils subjected to enhanced phytoremediation. Importantly, this antibiotic resistance-centered approach to soil metal phytoremediation could promote its commercial use and interest for soil managers.
The raw data supporting the conclusion of this article will be made available by the authors, without undue reservation.
CG: Conceptualization, Writing–original draft, Writing–review and editing. IA: Conceptualization, Writing–original draft, Writing–review and editing.
The author(s) declare financial support was received for the research, authorship, and/or publication of this article. This work was supported by MCIN/AEI/10.13039/501100011033 (PID 2020-116495RB-I00), Basque Government (IT1578-22), and Euskampus–JRL Environmental Antibiotic Resistance.
The authors wish to thank support from Euskampus–JRL Environmental Antibiotic Resistance.
The authors declare that the research was conducted in the absence of any commercial or financial relationships that could be construed as a potential conflict of interest.
All claims expressed in this article are solely those of the authors and do not necessarily represent those of their affiliated organizations, or those of the publisher, the editors and the reviewers. Any product that may be evaluated in this article, or claim that may be made by its manufacturer, is not guaranteed or endorsed by the publisher.
Ahmad, J., Marsidi, N., Abdullah, S. R. S., Hasan, H. A., Othman, A. R., Ismail, N. I., et al. (2024). Integrating phytoremediation and mycoremediation with biosurfactant-producing fungi for hydrocarbon removal and the potential production of secondary resources. Chemosphere 349, 140881. doi:10.1016/j.chemosphere.2023.140881
Alkorta, I., Epelde, L., and Garbisu, C. (2017). Environmental parameters altered by climate change affect the activity of soil microorganisms involved in bioremediation. FEMS Microbiol. Lett. 364, fnx200. doi:10.1093/femsle/fnx200
Alkorta, I., and Garbisu, C. (2021). Reflections and insights on the evolution of the biological remediation of contaminated soils. Front. Environ. Sci. 9, 734628. doi:10.3389/fenvs.2021.734628
Bach, E. M., Ramirez, K. S., Fraser, T. D., and Wall, D. H. (2020). Soil biodiversity integrates solutions for a sustainable future. Sustainability 12, 2662. doi:10.3390/su12072662
Baker-Austin, C., Wright, M. S., Stepanauskas, R., and McArthur, J. V. (2006). Co-selection of antibiotic and metal resistance. Trends Microbiol. 14, 176–182. doi:10.1016/J.TIM.2006.02.006
Bhullar, K., Waglechner, N., Pawlowski, A., Koteva, K., Banks, E. D., Johnston, M. D., et al. (2012). Antibiotic resistance is prevalent in an isolated cave microbiome. PLoS ONE 7 (4), e34953. doi:10.1371/journal.pone.0034953
Bolan, N., Kunhikrishnan, A., Thangarajan, R., Kumpiene, J., Park, J., Makino, T., et al. (2014). Remediation of heavy metal(loid)s contaminated soils – to mobilize or to immobilize? J. Hazard. Mater. 266, 141–166. doi:10.1016/j.jhazmat.2013.12.018
Cai, P., Chen, Q., Du, W., Yang, S., Li, J., Cai, H., et al. (2023). Deciphering the dynamics of metal and antibiotic resistome profiles under different metal(loid) contamination levels. J. Hazard. Mater. 455, 131567. doi:10.1016/j.jhazmat.2023.131567
Chen, Q.-L., An, X.-L., Zheng, B.-X., Gillings, M., Peñuelas, J., Cui, L., et al. (2019). Loss of soil microbial diversity exacerbates spread of antibiotic resistance. Soil Ecol. Lett. 1, 3–13. doi:10.1007/s42832-019-0011-0
Chen, Q.-L., Fan, X.-T., Zhu, D., An, X.-L., Su, J.-Q., and Cui, L. (2018). Effect of biochar amendment on the alleviation of antibiotic resistance in soil and phyllosphere of Brassica chinensis L. Soil Biol. biochem. 119, 74–82. doi:10.1016/j.soilbio.2018.01.015
Cheng, J.-H., Tang, X.-Y., Su, J.-Q., and Liu, C. (2021). Field aging alters biochar's effect on antibiotic resistome in manured soil. Environ. Pollut. 288, 117719. doi:10.1016/j.envpol.2021.117719
Čvančarová, M., Moeder, M., Filipová, A., and Cajthaml, T. (2015). Biotransformation of fluoroquinolone antibiotics by ligninolytic fungi–metabolites, enzymes, and residual antibacterial activity. Chemosphere 136, 311–320. doi:10.1016/j.chemosphere.2014.12.012
Duan, M., Li, H., Gu, J., Tuo, X., Sun, W., Qian, X., et al. (2017). Effects of biochar on reducing the abundance of oxytetracycline, antibiotic resistance genes, and human pathogenic bacteria in soil and lettuce. Environ. Pollut. 224, 787–795. doi:10.1016/j.envpol.2017.01.021
Edet, U. O., Bassey, I. U., and Joseph, A. P. (2023). Heavy metal co-resistance with antibiotics amongst bacteria isolates from an open dumpsite soil. Heliyon 9, e13457. doi:10.1016/j.heliyon.2023.e13457
El Rasafi, T., Haouas, A., Tallou, A., Chakouri, M., Aallam, Y., El Moukhtari, A., et al. (2023). Recent progress on emerging technologies for trace elements-contaminated soil remediation. Chemosphere 341, 140121. doi:10.1016/j.chemosphere.2023.140121
Garbisu, C., Alkorta, I., Kidd, P., Epelde, L., and Mench, M. (2020). Keep and promote biodiversity at polluted sites under phytomanagement. Environ. Sci. Pollut. Res. 27, 44820–44834. doi:10.1007/s11356-020-10854-5
Garbisu, C., Garaiyurrebaso, O., Lanzén, A., Álvarez-Rodríguez, I., Arana, L., Blanco, F., et al. (2018). Mobile genetic elements and antibiotic resistance in mine soil amended with organic wastes. Sci. Total Environ. 621, 725–733. doi:10.1016/j.scitotenv.2017.11.221
Gillings, M. R. (2013). Evolutionary consequences of antibiotic use for the resistome, mobilome and microbial pangenome. Front. Microbiol. 4, 4. doi:10.3389/fmicb.2013.00004
Guennoc, C. M., Rose, C., Labbé, J., and Deveau, A. (2018). Bacterial biofilm formation on the hyphae of ectomycorrhizal fungi: a widespread ability under controls? FEMS Microbiol. Ecol. 94, fiy093. doi:10.1093/femsec/fiy093
He, Y., Liu, C., Tang, X., Xian, Q., Zhang, J., and Guan, Z. L. (2019). Biochar impacts on sorption-desorption of oxytetracycline and florfenicol in an alkaline farmland soil as affected by field ageing. Sci. Total Environ. 671, 928–936. doi:10.1016/j.scitotenv.2019.03.414
Heuer, H., Schmitt, H., and Smalla, K. (2011). Antibiotic resistance gene spread due to manure application on agricultural fields. Curr. Opin. Microbiol. 14, 236–243. doi:10.1016/j.mib.2011.04.009
Hong, X., Zhao, Y., Zhuang, R., Liu, J., Guo, G., Chen, J., et al. (2020). Bioremediation of tetracycline antibiotics-contaminated soil by bioaugmentation. RSC Adv. 10, 33086–33102. doi:10.1039/d0ra04705h
Huang, K., Xia, H., Zhang, Y., Li, J., Cui, G., Li, F., et al. (2020). Elimination of antibiotic resistance genes and human pathogenic bacteria by earthworms during vermicomposting of dewatered sludge by metagenomic analysis. Bioresour. Technol. 297, 122451. doi:10.1016/j.biortech.2019.122451
Jalali, F. M., Chahkandi, B., Gheibi, M., Eftekhari, M., Behzadian, K., and Campos, L. C. (2023). Developing a smart and clean technology for bioremediation of antibiotic contamination in arable lands. Sustain. Chem. Pharm. 33, 101127. doi:10.1016/j.scp.2023.101127
Janvier, C., Villeneuve, F., Alabouvette, C., Edel-Hermann, V., Mateille, T., and Steinberg, C. (2007). Soil health through soil disease suppression: which strategy from descriptors to indicators? Soil Biol. biochem. 39, 1–23. doi:10.1016/j.soilbio.2006.07.001
Jauregi, L., Epelde, L., Alkorta, I., and Garbisu, C. (2021a). Antibiotic resistance in agricultural soil and crops associated to the application of cow manure-derived amendments from conventional and organic livestock farms. Front. Vet. Sci. 8, 633858. doi:10.3389/fvets.2021.633858
Jauregi, L., Epelde, L., Alkorta, I., and Garbisu, C. (2021b). Agricultural soils amended with thermally-dried anaerobically-digested sewage sludge showed increased risk of antibiotic resistance dissemination. Front. Microbiol. 12, 666854. doi:10.3389/fmicb.2021.666854
Jauregi, L., Epelde, L., Artamendi, M., Blanco, F., and Garbisu, C. (2023). Induced development of oxytetracycline tolerance in bacterial communities from soil amended with well-aged cow manure. Ecotoxicology 32, 418–428. doi:10.1007/s10646-023-02650-x
Jiang, H., Zhang, L., Wang, X., Gu, J., Song, Z., Wei, S., et al. (2023). Reductions in abundances of intracellular and extracellular antibiotic resistance genes by SiO2 nanoparticles during composting driven by mobile genetic elements. J. Environ. Manage. 341, 118071. doi:10.1016/j.jenvman.2023.118071
Kidd, P., Mench, M., Alvarez-Lopez, V., Bert, V., Dimitriou, I., Friesl-Hanl, W., et al. (2015). Agronomic practices for improving gentle remediation of trace element-contaminated soils. Int. J. Phytorem. 17, 1005–1037. doi:10.1080/15226514.2014.1003788
Kohlmeier, S., Smits, T. H. M., Ford, R. M., Keel, C., Harms, H., and Wick, L. Y. (2005). Taking the fungal highway: mobilization of pollutant-degrading bacteria by fungi. Environ. Sci. Technol. 39 (12), 4640–4646. doi:10.1021/es047979z
Kumpiene, J., Antelo, J., Brännvall, E., Carabante, I., Ek, K., Komárek, M., et al. (2019). In situ chemical stabilization of trace element-contaminated soil - field demonstrations and barriers to transition from laboratory to the field - a review. Appl. Geochem. 100, 335–351. doi:10.1016/j.apgeochem.2018.12.003
Kurenbach, B., Hill, A. M., Godsoe, W., van Hamelsveld, S., and Heinemann, J. A. (2018). Agrichemicals and antibiotics in combination increase antibiotic resistance evolution. PeerJ 6, e5801. doi:10.7717/peerj.5801
Lacalle, R. G., Aparicio, J. D., Artetxe, U., Urionabarrenetxea, E., Polti, M. A., Soto, M., et al. (2020). Gentle remediation options for soil with mixed chromium (VI) and lindane pollution: biostimulation, bioaugmentation, phytoremediation and vermiremediation. Heliyon 6, e04550. doi:10.1016/j.heliyon.2020.e04550
Li, H., Luo, Q.-P., Pu, Q., Yang, X.-R., An, X.-L., Zhu, D., et al. (2022). Earthworms reduce the dissemination potential of antibiotic resistance genes by changing bacterial co-occurrence patterns in soil. J. Hazard. Mat. 426, 128127. doi:10.1016/j.jhazmat.2021.128127
Liao, H., Li, H., Duan, C.-S., Zhou, X.-Y., An, X.-L., Zhu, Y.-G., et al. (2022). Metagenomic and viromic analysis reveal the anthropogenic impacts on the plasmid and phage borne transferable resistome in soil. Environ. Int. 170, 107595. doi:10.1016/j.envint.2022.107595
Liao, H., Li, X., Yang, Q., Bai, Y., Cui, P., Wen, C., et al. (2021). Herbicide selection promotes antibiotic resistance in soil microbiomes. Mol. Biol. Evol. 38, 2337–2350. doi:10.1093/molbev/msab029
Mahdi, I., Fahsi, N., Hijri, M., and Sobeh, M. (2022). Antibiotic resistance in plant growth promoting bacteria: a comprehensive review and future perspectives to mitigate potential gene invasion risks. Front. Microbiol. 13, 999988. doi:10.3389/fmicb.2022.999988
Moreira, H., Pereira, S. I. A., Mench, M., Garbisu, C., Kidd, P., and Castro, P. M. L. (2021). Phytomanagement of metal(loid)-contaminated soils: options, efficiency and value. Front. Environ. Sci. 9, 661423. doi:10.3389/fenvs.2021.661423
Nesme, J., and Simonet, P. (2015). The soil resistome: a critical review on antibiotic resistance origins, ecology and dissemination potential in telluric bacteria. Environ. Microbiol. 17, 913–930. doi:10.1111/1462-2920.12631
Nnorom, M.-A., Saroj, D., Avery, L., Hough, R., and Guo, B. (2023). A review of the impact of conductive materials on antibiotic resistance genes during the anaerobic digestion of sewage sludge and animal manure. J. Hazard. Mat. 446, 130628. doi:10.1016/j.jhazmat.2022.130628
Núñez-Zofío, M., Larregla, S., and Carlos Garbisu, C. (2011). Application of organic amendments followed by soil plastic mulching reduces the incidence of Phytophthora capsici in pepper crops under temperate climate. Crop Prot. 30, 1563–1572. doi:10.1016/j.cropro.2011.08.020
Ohore, O. E., Addo, F. G., Zhang, S., Han, N., and Anim-Larbi, K. (2019). Distribution and relationship between antimicrobial resistance genes and heavy metals in surface sediments of Taihu Lake, China. J. Environ. Sci. 77, 323–335. doi:10.1016/j.jes.2018.09.004
Olicón-Hernández, D. R., González-López, J., and Aranda, E. (2017). Overview on the biochemical potential of filamentous fungi to degrade pharmaceutical compounds. Front. Microbiol. 8, 1792. doi:10.3389/fmicb.2017.01792
Qiu, L., Zhang, Q., Zhu, H., Reich, P. B., Banerjee, S., van der Heijden, M. G. A., et al. (2021). Erosion reduces soil microbial diversity, network complexity and multifunctionality. ISME J. 15, 2474–2489. doi:10.1038/s41396-021-00913-1
Romero, D., Traxler, M. F., López, D., and Kolter, R. (2011). Antibiotics as signal molecules. Chem. Rev. 111, 5492–5505. doi:10.1021/cr2000509
Serwecińska, L. (2020). Antimicrobials and antibiotic-resistant bacteria: a risk to the environment and to public health. Water 12, 3313. doi:10.3390/w12123313
Song, J., Chen, Y., Mi, H., Xu, R., Zhang, W., Wang, C., et al. (2024). Prevalence of antibiotic and metal resistance genes in phytoremediated cadmium and zinc contaminated soil assisted by chitosan and Trichoderma harzianum. Environ. Int. 183, 108394. doi:10.1016/j.envint.2023.108394
Staub, J. M., Brand, L., Tran, M., Kong, Y., and Rogers, S. G. (2012). Bacterial glyphosate resistance conferred by overexpression of an E. coli membrane efflux transporter. J. Ind. Microbiol. Biotechnol. 39, 641–647. doi:10.1007/s10295-011-1057-x
Sun, C., Li, W., Chen, Z., Qin, W., and Wen, X. (2019). Responses of antibiotics, antibiotic resistance genes, and mobile genetic elements in sewage sludge to thermal hydrolysis pre-treatment and various anaerobic digestion conditions. Environ. Int. 133, 105156. doi:10.1016/j.envint.2019.105156
Tang, K. H. D. (2023). Phytoremediation: where do we go from here? Biocatal. Agric. Biotechnol. 50, 102721. doi:10.1016/j.bcab.2023.102721
Tian, W., Zhang, M., Zong, D., Li, W., Li, X., Wang, Z., et al. (2023). Are high-risk heavy metal(loid)s contaminated vegetables detrimental to human health? A study of incorporating bioaccessibility and toxicity into accurate health risk assessment. Sci. Total Environ. 897, 165514. doi:10.1016/j.scitotenv.2023.165514
Tong, Z., Liu, F., Tian, Y., Zhang, J., Liu, H., Duan, J., et al. (2022). Effect of biochar on antibiotics and antibiotic resistance genes variations during co-composting of pig manure and corn straw. Front. Bioeng. Biotechnol. 10, 960476. doi:10.3389/fbioe.2022.960476
Tran, T. T., Scott, A., Tien, Y.-C., Murray, R., Boerlin, P., Pearl, D. L., et al. (2021). On-farm anaerobic digestion of dairy manure reduces the abundance of antibiotic resistance-associated gene targets and the potential for plasmid transfer. Appl. Environ. Microbiol. 87, 20. e0298020. doi:10.1128/AEM.02980-20
Urra, J., Alkorta, I., Lanzén, A., Mijangos, I., and Garbisu, C. (2019). The application of fresh and composted horse and chicken manure affects soil quality, microbial composition and antibiotic resistance. Appl. Soil Ecol. 135, 73–84. doi:10.1016/j.apsoil.2018.11.005
Wales, A. D., and Davies, R. H. (2015). Co-selection of resistance to antibiotics, biocides and heavy metals, and its relevance to foodborne pathogens. Antibiotics 4, 567–604. doi:10.3390/antibiotics4040567
Wang, J., and Delavar, M. A. (2023). Techno-economic analysis of phytoremediation: a strategic rethinking. Sci. Total Environ. 902, 165949. doi:10.1016/j.scitotenv.2023.165949
Wang, Y., Pandey, P. K., Kuppu, S., Pereira, R., Aly, S., and Zhang, R. (2021). Degradation of antibiotic resistance genes and mobile gene elements in dairy manure anerobic digestion. PLoS ONE 16, e0254836. doi:10.1371/journal.pone.0254836
Wardle, D. A., Bardgett, R. D., Klironomos, J. N., Setälä, H., Van Der Putten, W. H., and Wall, D. H. (2004). Ecological linkages between aboveground and belowground biota. Science 304, 1629–1633. doi:10.1126/science.1094875
Waseem, M., Khilji, S. A., Tariq, S., Jamal, A., Alomrani, S. O., and Javed, T. (2024). Phytoremediation of heavy metals from industrially contaminated soil using sunflower (Helianthus annus L.) by inoculation of two indigenous bacteria. Plant Stress 11, 100297. doi:10.1016/j.stress.2023.100297
Xu, X., Zarecki, R., Medina, S., Ofaim, S., Liu, X., Chen, C., et al. (2019). Modeling microbial communities from atrazine contaminated soils promotes the development of biostimulation solutions. ISME J. 13, 494–508. doi:10.1038/s41396-018-0288-5
Ye, M., Sun, M., Feng, Y., Wan, J., Xie, S., Tian, D., et al. (2016). Effect of biochar amendment on the control of soil sulfonamides, antibiotic-resistant bacteria, and gene enrichment in lettuce tissues. J. Hazard. Mater. 309, 219–227. doi:10.1016/j.jhazmat.2015.10.074
Zalewska, M., Błażejewska, A., Czapko, A., and Popowska, M. (2023). Pig manure treatment strategies for mitigating the spread of antibiotic resistance. Sci. Rep. 13, 11999. doi:10.1038/s41598-023-39204-4
Zhu, D., Delgado-Baquerizo, M., Su, J. Q., Ding, J., Li, H., Gillings, M. R., et al. (2021). Deciphering potential roles of earthworms in mitigation of antibiotic resistance in the soils from diverse ecosystems. Environ. Sci. Technol. 55, 7445–7455. doi:10.1021/acs.est.1c00811
Keywords: antibiotics, antimicrobials, bioremediation, metal pollution, resistome, soil
Citation: Garbisu C and Alkorta I (2024) Enhanced phytoremediation of metal contaminated soils aimed at decreasing the risk of antibiotic resistance dissemination. Front. Environ. Sci. 12:1307631. doi: 10.3389/fenvs.2024.1307631
Received: 04 October 2023; Accepted: 23 January 2024;
Published: 02 February 2024.
Edited by:
Qi Liao, Central South University, ChinaReviewed by:
Agnieszka Klimkowicz-Pawlas, Institute of Soil Science and Plant Cultivation, PolandCopyright © 2024 Garbisu and Alkorta. This is an open-access article distributed under the terms of the Creative Commons Attribution License (CC BY). The use, distribution or reproduction in other forums is permitted, provided the original author(s) and the copyright owner(s) are credited and that the original publication in this journal is cited, in accordance with accepted academic practice. No use, distribution or reproduction is permitted which does not comply with these terms.
*Correspondence: Itziar Alkorta, aXR6aS5hbGtvcnRhQGVodS5ldXM=
Disclaimer: All claims expressed in this article are solely those of the authors and do not necessarily represent those of their affiliated organizations, or those of the publisher, the editors and the reviewers. Any product that may be evaluated in this article or claim that may be made by its manufacturer is not guaranteed or endorsed by the publisher.
Research integrity at Frontiers
Learn more about the work of our research integrity team to safeguard the quality of each article we publish.