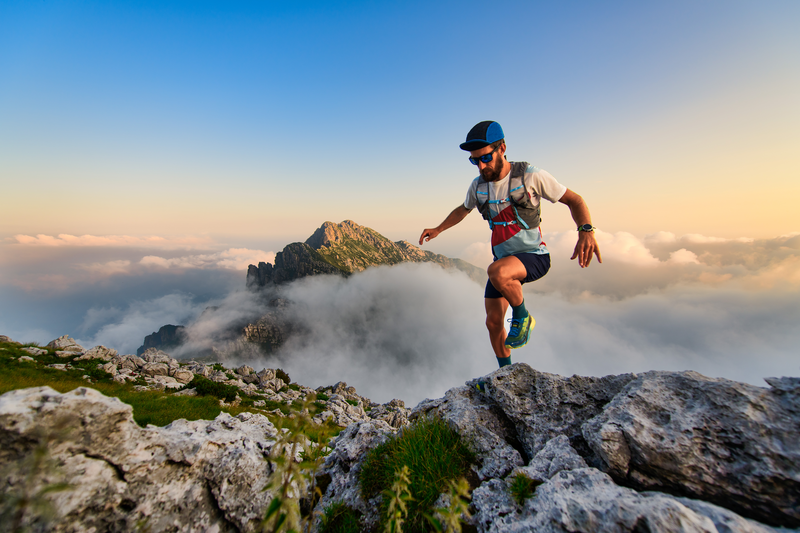
94% of researchers rate our articles as excellent or good
Learn more about the work of our research integrity team to safeguard the quality of each article we publish.
Find out more
ORIGINAL RESEARCH article
Front. Environ. Sci. , 16 January 2024
Sec. Atmosphere and Climate
Volume 11 - 2023 | https://doi.org/10.3389/fenvs.2023.1307426
This article is part of the Research Topic Crucial Air Quality, Atmospheric Environment, and Climate Change in Low- and Middle-Income Countries View all 8 articles
Introduction: Fine particulate matter (PM2.5) pollution is a global public health concern, particularly in arid and semi-arid regions that experience dust events. Exposure to PM2.5 has been associated with various short- and long-term health effects, which can be attributed to the composition of the fine particles. This study aimed to assess the concentration of bacteria and fungi in PM2.5 in the air of Isfahan, Iran which experiences air pollution.
Methods: A total of thirty-nine PM2.5 samples were collected over 1 year. Bacterial and fungal concentrations associated with PM2.5 were measured using both culture-based method and real-time polymerase chain reaction (PCR).
Results: The average concentration of PM2.5 was found to be 56 μg m−3, with many samples exceeding the recommended limit of 15 μg m−3 set by the World Health Organization for PM2.5 in ambient air. The mean culturable bacterial and fungal concentrations were 39 and 4 CFU m−3, respectively which showed a significant difference compared to the results obtained through real-time PCR. No significant correlation was found between the levels of bacterial and fungal cells and PM2.5 concentrations. The dominant phyla found in PM2.5 were Firmicutes and Actinobacteria, while Proteobacteria as Gram-negative bacteria were rarely detected.
Discussion: The findings suggest that the climate conditions in Isfahan, characterized by its semi-arid nature with low humidity content, influences the activity and viability of microbial populations, resulting in low concentrations of bioaerosols in PM2.5. Furthermore, it seems that desert dust events do not contribute to increasing the potential health risks associated with bacterial and fungal bioaerosols in PM2.5.
Air pollution is becoming a growing concern in certain regions of the world. The expansion of cities, urbanization, and industrial development, coupled with increased traffic, are the main factors contributing to higher levels of atmospheric pollution. This rapid decline in global air quality is having a detrimental effect on human health, the economy, and ecosystems (Shammi et al., 2021; World Health Organization, 2022; Taghizadeh et al., 2023). Air pollution caused by the presence of gas compounds and particulate matter (PM) in the atmosphere which the latter causes more health effects than any other pollutants (Shammi et al., 2021; World Health Organization, 2022). In recent years, arid and semi-arid regions such as the Middle East countries have been facing severe PM pollution resulting from increased desertification caused by climate change and drought (Goudarzi et al., 2016; Shammi et al., 2021). According to the 2020 world air quality report, Iran has recorded an average PM2.5 concentration of 27.2 μg m−3, which is more than double the WHO’s recommended exposure limit. Additionally, PM2.5 levels in Iran have increased from 24.3 to 27.2 μg m−3, showing a 10.6% increment from 2019 to 2020 (Taghizadeh et al., 2023). There is growing evidence that the increasing presence of PM has adverse health effects due to its diverse composition and chemical and biological properties (He et al., 2023; Tavangar et al., 2023). PM comprises inhalable particles and is classified as PM10, with the aerodynamic diameter of 10 μm or less, and fine particles with the aerodynamic diameter of 2.5 μm or less (PM2.5). PM10 as coarse particles are filtered by the nose and throat and therefore effectively removed by the upper respiratory tract. However, fine particles have the ability to penetrate deeply into the lungs, distribute within the lung’s alveolar districts, deposit in it and enter the blood system (World Health Organization, 2022; He et al., 2023). Exposure to PM2.5 pollution has significant detrimental effects on human health, leading to increased rate of illness, premature death, and the development of various diseases among affected populations. There is mounting evidence suggesting that fine particulate matter in the air can contribute to the onset and exacerbation of respiratory conditions, including chronic obstructive pulmonary disease (COPD), acute respiratory infections, cardiovascular diseases, type 2 diabetes, and even cancer (Shammi et al., 2021; World Health Organization, 2022; He et al., 2023). The World Health Organization (WHO) estimates that air pollution from PM2.5 is responsible for 4.2 million premature deaths globally every year (World Health Organization, 2022; He et al., 2023). A recent study conducted in Iran investigated the health burden and economic impact associated with PM2.5 pollution. The research estimated the crude death rate and the age-standardized death rate per 100,000 population for individuals aged 25 and above, attributed to ground-monitored PM2.5, were 97 and 125, respectively (Faridi, 2022).
PM2.5 is a complex mixture of solid and liquid particles containing organic and inorganic substances such as sulfate, nitrate, metals, ions, polycyclic aromatic hydrocarbons (PAHs), volatile organic compounds (VOCs); and microbial pollutants (bioaerosols) (He et al., 2023; Tavangar et al., 2023). It has been reported that bioaerosols, which are biological materials like bacteria and fungi, can significantly contribute, up to 25%, to PM (He et al., 2023). Zhang et al. (2022) found that bioaerosols accounted for 18.3% ± 10.6% and 13.7% ± 12.5% of PM10 and PM2.5 mass, respectively. As an important component of fine particles, bioaerosols play a crucial role in affecting human health, atmospheric chemistry, nucleation processes, and interactions within atmosphere. Exposure to the microbial components present in PM2.5 can lead to severe adverse effects, including infectious disease and respiratory tract disorders and allergic reactions. Additionally, microbes in fine PM can significantly influence the oxidative potential resulting from the inhalation of PM2.5. Respiratory symptoms such as asthma, hypersensitivity pneumonitis, and chronic bronchitis are commonly associated with exposure to non-infectious bioaerosols (Shammi et al., 2021; He et al., 2023).
Air pollution has become a major issue in many large Iranian cities, in recent years. Isfahan, the third-largest city in Iran, is particularly affected, with relatively high concentrations of PM2.5 contributing to its poor air quality (Taghizadeh et al., 2023). Looking at the data of air quality index (AQI) in 2019 shows that 4 months were classified as “Unhealthy for sensitive groups” due to elevated levels of PM2.5 (IQ Air, 2023). In addition, the average annual PM2.5 concentration from 26.4 μg m−3 in 2019 increased to 31.4 μg m−3 in 2020 (Taghizadeh et al., 2023). The city’s air pollution is primarily attributed to mobile sources and industrial activities. However, Middle East dust events, particularly during autumn with strong wind, also contribute to the high PM (Hosseini et al., 2020; Taghizadeh et al., 2023). A study on health burden attributable to fine particulate matter in Iran found that Tehran had the highest number of attributable deaths to ambient PM2.5, followed by Mashhad and Isfahan. The crude death rate and the age-standardized death rate per 100,000 population for individuals aged 25 and above, resulting from ground-monitored PM2.5 in Isfahan, were estimated to be 102 and 122, respectively (Faridi et al., 2022).
Given that the components and effects of PM2.5 can vary by location, it is crucial to have a correct knowledge about the physiochemical and biological compositions of fine particles to determine the role of PM pollution in potential health outcomes. While the impact of PM2.5 pollution and its physiochemical compositions on human health have been extensively studied (Faridi et al., 2022; He et al., 2023; Tavangar et al., 2023), very few studies have characterized the bioaerosols in PM2.5 and the effect of dust events on this important fraction of fine particles (Alghamdi et al., 2014; Du et al., 2018a; Aziza et al., 2018; Du et al., 2018b; Lu et al., 2018; Xu et al., 2019). Some studies have focused on investigating airborne bacteria and fungi in indoor and outdoor environments, as well as the influence of PM2.5 concentration and dust events on the concentration and type of bioaerosols (Jeon et al., 2011; Mirhosseini et al., 2015; Fang et al., 2016; Goudarzi et al., 2016; Harbizadeh et al., 2019; Hosseini et al., 2020). For instance, Hosseini et al. (2020) reported an average bacterial concentration of 76 CFU m−3 and a concentration of fungi of 46 CFU m−3 in the ambient air of Isfahan. Because of the health significance of microbial composition of fine particles and the need for relevant researches in this field especially in regions impact from dust events, this study was performed to investigate the microbial characteristics of the PM2.5 in an urban area that frequently experiences PM pollution. This study is the first of its kind in a semi-arid region with dry weather, providing insights into the abundance and types of bioaerosols present in fine particles.
This study was conducted in Isfahan, Iran, from October 2018 to November 2019. Isfahan with a population of 1.6 million, is a large city in central part of Iran with a semi-arid climate which frequently experiences the PM2.5 pollution. Sampling was performed in the city center, on the roof of a two-story building (32.65226 N, 51.67320 E). This location was selected because there were no multi-story buildings around it and it was located in a suitable distance from green spaces and other sources of aerosol generation. Figure 1 displays the sampling location.
PM2.5 was sampled via a PQ200 with a flow rate of 24 m3 day-1 for 24 h (from 9 a.m. to 9 a.m. of the next day). A quartz filter with the diameter of 47 mm was employed for sampling. Before the sampling, the filter was placed at 500°C for 1 h to eliminate its organic substances. Overall, 39 air samples were collected over a year (approximately one sample in 10 days). By measuring the filter’s primary weight and post-sampling secondary weight with precise scale, the PM2.5 concentration was calculated and reported as µg m−3. Meteorological parameters including relative humidity, temperature, UV index and wind speed during sampling period was obtained from the data of Meteorology Organization of Isfahan.
To evaluate the presence of bacteria and fungi in PM2.5, the filter was carefully placed into a sterile 15 mL centrifuge tube containing 7 mL of phosphate buffer solution with 0.02% Tween 20. The tube was then subjected to ultrasonic bath treatment for 5 min, followed by shaking for 30 min to separate the bioaerosols from the filter’s surface. To ensure complete separation, the filter washing process was repeated once more. Next, 250 µL aliquots of the resulting suspension were spread onto duplicate plates of tryptic soy agar (TSA) culture medium supplemented with nystatin for bacterial isolation, and malt extract agar (MEA) culture medium supplemented with chloramphenicol for fungal isolation. The TSA plates were incubated at 30°C for 2–3 days, while the MEA plates were incubated at room temperature for 3–5 days (Nikaeen et al., 2009). The colonies growing on the culture media were enumerated and reported as colony-forming units per cubic meter (CFU m−3). Bacterial and fungal colonies were stained and characterized based on the colony and cell morphology.
The concentration of total bacteria and fungi (culturable and non-culturable cells) associated with PM2.5 was determined using quantitative real-time PCR. DNA was extracted directly from the washed filter suspension using the freeze-thaw method followed by the application of QIAamp DNA Mini Kit (QIAGEN) as per the manufacturer’s instructions. Universal primers for the 16S rRNA and 18S rRNA (ITS) genes (Table 1) were used to amplify the DNA of bacteria and fungi, respectively, with real-time PCR. The concentration of bacterial and fungal cells was calculated by comparing the CT values of the amplified samples with the standard curve. Standard curve was plotted using the serial dilution of E. coli and Aspergillus flavus DNA (105–100 genome equivalents (GE)) as the bacterial and fungal indicators (An et al., 2006; Hospodsky et al., 2010). Briefly, the DNA of cultured Escherichia coli and A. flavus was extracted, the concentration of DNA was determined by Qubit fluorometer and the number of cells was calculated based on the GE which was calculated based on the average microorganism genome size.
To identify the predominant bacterial species, colonies displaying different morphology and gram staining characteristics were isolated and subcultured on TSA agar plates. The isolated colonies were then suspended in 100 μL of deionized water, and genomic DNA was extracted. Bacterial DNA was amplified using universal primers targeting the 16S rRNA gene, following the protocol described by Farhadkhani et al. (2014). The amplified gene was subjected to DNA sequencing, and the resulting sequences were analyzed using BLAST algorithms and databases available from the National Center for Biotechnology Information (http://blast.ncbi.nlm.nih.gov/Blast.cgi). The sequence data was subsequently deposited into GenBank (GenBank accession numbers: MZ661124-MZ661153).
Statistical analysis was performed in SPSS 22. Kolmogorov-Smirnov test was run to determine the normality of data distribution and determine whether parametric or non-parametric tests must be performed. Kruskal–Wallis test was used to compare the difference in the concentration of PM2.5-associated bioaerosols across seasons. Spearman rank-order correlation was used to evaluate the relationship between the analyzed parameters. p < 0.05 was regarded as statistically significant. The diagrams were plotted in Microsoft Excel.
Fine particles, known as PM2.5, can have detrimental effects on human health. During the sampling period, concentration of PM2.5 ranged from of 12–137 μg m−3, with the annual mean of 56 μg m−3 (table 2). In the studied region, it was found that only 5% (2 out of 39) of air samples met the WHO guideline limit of 15 μg m−3 for fine particle concentration. Additionally, it was observed that PM2.5 concentrations exceeded 100 μg m−3 on 9 days, further emphasizing the significance of air pollution caused by fine particles in the area. Looking at the data of air quality index (AQI) shows the condition of “Unhealthy for sensitive groups” for 4 months in 2019 due to relatively high levels of PM2.5 (IQAir, 2023). In recent years, desert dust events have emerged as one of the major contributor to very high concentrations (over 100 μg m−3) of the fine particles in Isfahan. The increased aridity in the Middle East, including Iran, as a result of climatic change and drought has led to more frequent dust events, exacerbating air pollution. Isfahan, being a semi-arid region with low humidity and relatively high temperatures (as shown in table 2) is affected by dust events in the Middle-East region. Alghamdi et al. (2014) reported a mean PM 2.5 concentration of 60 μg m−3 in Saudi Arabia. However, in a study conducted in Ahvaz, Iran, a city heavily impacted by intense Middle East dust (MED) storms, the mean PM2.5 concentration during the sampling period was reported as 85.5 μg m−3 on regular days and 475 μg m−3 on dusty days (Goudarzi et al., 2019). As depicted in Figure 2, high concentrations of PM2.5 were observed during autumn followed by winter. Overall, high PM2.5 concentrations (exceeding 100 μg m−3) were observed during summer (two samples), autumn (5 samples) and winter (4 samples) due to dust events in summer and autumn and thermal inversion in winter. Except for dusty days, high traffic density and industrial activates are the main sources of fine particles in Isfahan throughout the year.
TABLE 2. Concentration of PM2.5 and bioaerosols in PM2.5 and characteristics of meteorological parameters.
Biological particles, when inhaled alongside fine particles, have been linked to the development of various diseases (Shammi et a., 2021; He et al., 2023). Table 2 also presents the mean concentrations of bacterial and fungal aerosols associated with fine particles. The mean concentration of bacteria in PM2.5 was found to be 39 CFU m−3, while the mean concentration of fungi was and 4 CFU m−3. A study conducted on ambient bioaerosols in Isfahan also reported a mean concentration of 76 CFU m−3 for bacteria and 46 CFU m−3 for fungi (Hosseini et al., 2020). Bragoszewska et al. (2018) reported a range of 50–300 CFU m−3 for bacterial aerosols in the Netherlands, which is near to our values. However, a study by Li et al. (2017) in China reported higher mean concentrations of bacteria (565 CFU m−3) and fungi (399 CFU m−3). Similarly, Gao et al. (2015) found mean concentrations of airborne bacteria and fungi in Beijing, China to be 1110 and 948 CFU m−3, respectively. Furthermore, a study conducted in Ahvaz, Iran, which has an arid climate but relatively high humidity (45%–60%), reported a concentration of airborne bacteria ranging from 70 to 200 CFU m−3 (Haribzadeh et al., 2019). The variation in bioaerosol concentrations across different regions could be attributed to variations in sampling methods, growth media, geographical locations and, most importantly, the climatic and environmental conditions. Low humidity, high temperature, and intensity of UV radiation are major weather conditions that can impact the viability and consequently concentrations of bioaerosols in the ambient air (Kim et al., 2018a). The observed low concentrations of fungi in our study may be attributed to the low humidity content of the air in Isfahan (Table 2). Fungi concentrations are known to be particularly influenced by low humidity levels. This finding is consistent with the results reported by Mirhossein et al. (2015), who observed lower concentrations of fungi in indoor environments in a semi-arid region compared to other studies. Chen et al. (2012) investigated the concentration and size distribution of airborne microorganisms in Guangzhou, China, during the summer and concluded that concentration of bacteria was considerably higher than that of fungi, which aligns with our findings. The results of Alghamdi et al. (2014) also showed a higher concentration of bacteria-associated PM2.5 (45–591 CFU m−3) than fungi (4–28 CFU m−3). As suggested by Li et al. (2017), fungal spores are greatly influenced by nutrient availability, plant growth and climatic conditions, and their low concentration can be attributed to a decrease in nutrient sources in arid climates. On the other hand, fungi generally have larger size (2.1–3.3 µm) compared to bacteria (1.1–1.2 µm) which may contribute to their association with particles larger than 2.5 µm (Kim et al., 2018a). It is also worth noting that larger bioaerosols have higher precipitation velocities, which can explain their lower counts compared to bacteria (Fang et al., 2016; Du et al., 2018b). As mentioned earlier, the climate conditions of arid and semi-arid regions, including solar radiation, low humidity and high temperature, plays crucial roles in the survival as well as growth of airborne microorganisms. Since, Isfahan experiences a semi-arid climate with dry weather, our results showed a significant difference between the concentrations of bioaerosols detected by the conventional culture method and quantitative real-time PCR (p < 0.05) (Figure 3). The results show that many microbial species in the semi-arid climate of Isfahan are non-viable or in the viable but non-culturable (VBNC) state and therefore, do not detect via conventional culture methods. This finding indicates that climate conditions of arid regions may lead to VBNC state of bacteria especially Gram-negative ones. This result is supported by the high diversity of identified bacteria in studies which have used next-generation sequences for analysis of bioaerosols in ambient air (Jeon et al., 2011; Li et al., 2017; Lu et al., 2018).
FIGURE 3. Bioaerosols in PM2.5 (A) Bacterial and fungal concentrations based on culture method (CFU m−3) and quantitative real-time PCR (No. m−3) (B) ratio of detected number of bacteria and fungi by quantitative real-time PCR to culture method.
Based on Figure 4 and Figure 5, the most frequently detected bacteria were related to Gram-positive bacteria including gram positive Cocci and Bacilli as well as Actinomycetes with the higher frequency of Gram-positive Bacilli in the spring and summer (Figure 4). In total, the most frequently identified species were Actinomycetes, followed by Gram-positive Bacilli and Cocci. Only two Gram-negative species, Aquamicrobium aerolatum and Brevundimonas diminuta were found in the air samples (Figure 5). The dominant phyla in PM2.5 were Firmicutes and Actinobacteria, while Proteobacteria (Alphaproteobacterial class) as Gram-negative bacteria were rarely detected. In contrast, studies using molecular methods have identified a high abundance of Proteobacteria in air samples (Jeon et al., 2011; Li et al., 2017), which may be attributed to the VBNC state of Gram-negative bacteria. For instance, high-throughput sequencing analysis of microbial communities in PM2.5 in Beijing, China showed that Proteobacteria, with an abundance of 33%, were the most frequently detected bacteria (Li et al., 2017). However, when culture methods were used to detect airborne bacteria in locations with different ecological and environmental conditions, it was found that 70%–90% of isolates were generally Gram-positive Bacilli and Cocci as well as Actinomycetes (Alghamdi et al., 2014; Goudarzi et al., 2016; Shammi et al., 2021). An exception to this trend was reported by Fang et al. (2016), who detected relatively high numbers of Pseudomonas species in culturable airborne bacteria of Hangzhou, China. It is important to note that the analysis of biological composition of PM or bioaerosol samples using high-throughput sequencing revealed a different scenario compared to cultivation-based methods, since in several cases there was a predominance of Gram-negative bacteria, mainly belonging to Alpha-, Beta- and Gamma-proteobacteria and Bacteroidetes (Du et al., 2018a; Du et al., 2018b; Lu et al., 2018; Xu et al., 2019). This difference in bacterial community composition could support the hypothesis of low resistance of Gram-negative bacteria in the air and their presence in the VBNC form, which cannot be detected by culture methods. On the other hand, the higher frequency detection of Gram-positive bacteria could be attributed to their greater resistance in arid-conditions. Gram-negative bacteria, with their thin phospholipid layer, have less stability against adverse conditions such as high temperature, low humidity and solar radiation (Kim et al., 2018b). Du et al. (2018a) analyzed the concentration of bacterial and fungal species in the air of Beijing, China, and reported a higher prevalence of bacterial species in winter. Mirhosseini et al. (2015) also reported a higher frequency of detection of Gram-positive bacteria due to their greater persistence against high temperature and dryness. Bacterial spores can survive under unfavorable environmental conditions that are intolerable for the vegetative form of bacteria (Kim et al., 2018a). Since members of Bacillus are spore formers, capable of surviving under adverse conditions such as high temperature and desiccation, therefore an increase in the frequency of Gram-positive Bacilli detection in the summer was observed (Figure 4).
FIGURE 5. Phylogenetic tree of isolated bacteria from ambient air PM2.5 based on the 16S rRNA sequences.
During the year of sampling, black fungi had the highest frequency, while yeasts had the lowest frequency (Figure 6). The higher level of black fungi compared to other genera could be attributed to the presence of pigment, mainly melanin, in their cell membrane structure. Melanin provides protection against environmental stress and enhances their adaptation to the surrounding conditions. Melanin protects organisms from the damaging effects of UV radiation (Lang-Yona et al., 2012) which is high in the studied region during warm seasons. In autumn, winter and summer, black fungi were the most frequent, while Penicillium spp. were the most identified fungi in spring (Figure 6). Meklin et al. (2007) conducted a microbiological investigation on the air of Ohio and found that Cladosporium, Aspergillus and Penicillium had the highest counts. According to Alghamdi et al. (2014), Aspergillus and Penicillium were identified as the predominant fungal genera present in PM2.5 air samples from Jeddah, Saudi Arabia. The authors suggested that the prevalence of airborne Aspergillus, Penicillium, and Alternaria can be attributed to their adaptability to diverse substrates in all regions, regardless of weather conditions. Additionally, these fungi possess a high capability to produce and release a large number of spores into the air.
However, it is important to consider that factors such as climate conditions and geographical differences can influence the concentration and type of fungi isolated from the ambient air.
In the present study, no significant relationship was found between the increase in PM2.5 concentrations and bacterial and fungal numbers. Similar to our results, a study conducted in Beijing, China also found no correlation between bacterial and fungal abundance in PM2.5 and pollution levels of fine particles (Du et al., 2018b). Similarly, Alghamdi et al. (2014) reported that bacteria associated with PM2.5 seemed to be independent of PM mass concentrations. In contrast to our findings, some studies have identified a positive correlation between the number of bacterial colonies and PM concentrations (Jeon et al., 2011; Goudarzi et al., 2016; Shammi et al., 2021; Zhang et al., 2022). Jeon et al. (2011) reported a significant increase in airborne culturable bacterial concentration and a change in bacterial community structure during Asian dust events, with the mean concentration of culturable bacterial cells associated with PM10 on dusty days being seven times higher than that on normal days. Li et al. (2017) also evaluated the concentration and size of bioaerosol particles in the air of Qingdao, China and reported an increase in bioaerosols concentration during dust storms. A study conducted in Ahvaz, Iran also showed an increase in airborne bacterial concentrations during dust event days compared to normal days with concentrations being three times higher (Goudarzi et al., 2016). This difference across different regions could be attributed to variations in sampling methods (bioaerosol alone or bioaerosol in PM), geographical locations and, most importantly, the climatic and environmental conditions. However, according to table 3, high concentrations of PM2.5 could influence the predominant bacterial species associated with PM2.5. Bacillus spp., known for their high resistance, were more frequently identified on dusty days, while Staphylococcus spp. were not detected (Table 3). It has been reported that Bacillus species were more abundant in dusty days (almost 23.8%) than the non-dust days (Shammi et al., 2021). Certain microorganisms in the air can only survive in clean climatic conditions due to their cell structure. As PM2.5 concentration rises, both organic and non-organic compounds such as sulfate, nitrate and ammonia also increase, which can promote the metabolism and growth of some microorganisms (Madhwal et al., 2020; Wei et al., 2020). However, a further increase in particle concentration can result in the presence of chemical and toxic compounds within fine particles, which may inhibit the growth of sensitive microorganisms. Therefore, only microorganisms that can adapt to harsh conditions can survive. Bacilli and Actinomycetes are capable of surviving at high particle concentrations due to their cellular characteristics (Wei et al., 2020).
In the present study, several opportunistic pathogens were also identified. Staphylococcus epidermis, a coagulase-negative Staphylococci, was identified and is known to cause 75% of infections in this category. Pathogenic S. equorum and Staphylococcus gallinarum species were also identified (Qin et al., 2019). Streptomyces species, typically found in soil flora, have recently been identified as immune system suppressants which cause systemic diseases (Xu et al., 2019). Saccharomonospora spp. can also cause respiratory system infections. Nocardiopsis dassonvillei, an opportunistic pathogen, is known to causes infections in the nose, lung, biliary tract and skin (Lu et al., 2018). However, it is important to note that the concentrations of these pathogenic airborne bacteria were relatively low in the present study, making it difficult to assess their adverse effects on human health. Further research is needed to understand the potential risks associated with the presence of these opportunistic pathogens in the air.
The concentration of PM2.5 in a significant number of ambient air samples in Isfahan exceeded the recommended value by the WHO. However, the concentrations of bacteria and fungi in PM2.5 were lower than those reported in similar studies, and there was no correlation with PM2.5 concentrations. This could be attributed to the warm and arid climatic conditions in Isfahan, which may contribute to the lower concentration of airborne microorganisms. Additionally, the results of molecular examination revealed much higher concentrations of bacteria and fungi compared to the conventional culture methods, indicating the potential presence of VBNC bioaerosols. Although the molecular identification of isolated bacteria showed the presence of some pathogenic bacteria in the urban air, it appears that air pollution based on the elevated PM2.5 concentrations does not have health impacts in terms of bacterial and fungal bioaerosols. Further studies are required to determine the chemical properties of these particles and their health impacts.
The datasets presented in this study can be found in online repositories. The names of the repository/repositories and accession number(s) can be found in the article/Supplementary material.
MN: Conceptualization, Funding acquisition, Methodology, Project administration, Supervision, Writing–original draft, Writing–review and editing. HK: Formal Analysis, Investigation, Writing–original draft, Writing–review and editing. ZS: Data curation, Investigation, Methodology, Writing–review and editing. YH: Methodology, Writing–review and editing.
The author(s) declare financial support was received for the research, authorship, and/or publication of this article. This research was supported by the Vice Chancellery for Research at the Isfahan University of Medical Sciences (Grant No 399722 and 197050).
The authors declare that the research was conducted in the absence of any commercial or financial relationships that could be construed as a potential conflict of interest.
All claims expressed in this article are solely those of the authors and do not necessarily represent those of their affiliated organizations, or those of the publisher, the editors and the reviewers. Any product that may be evaluated in this article, or claim that may be made by its manufacturer, is not guaranteed or endorsed by the publisher.
Alghamdi, M. A., Shamy, M., Redal, M. A., Khoder, M., Awad, A. H., and Elserougy, S. (2014). Microorganisms associated particulate matter: a preliminary study. Sci. Total Environ. 479, 109–116. doi:10.1016/j.scitotenv.2014.02.006
An, H. R., Mainelis, G., and White, L. (2006). Development and calibration of real-time PCR for quantification of airborne microorganisms in air samples. Atmos. Environ. 40, 7924–7939. doi:10.1016/j.atmosenv.2006.07.020
Aziza, A. A., Leea, K., Parkb, B., Parkb, H., Parka, K., Choib, I., et al. (2018). Comparative study of the airborne microbial communities and their functional composition in fine particulate matter (PM2.5) under non-extreme and extreme PM2.5 conditions. Atmos. Environ. 194, 82–92. doi:10.1016/j.atmosenv.2018.09.027
Brągoszewska, E., and Pastuszka, J. S. (2018). Influence of meteorological factors on the level and characteristics of culturable bacteria in the air in Gliwice, Upper Silesia (Poland). Aerobiologia 34, 241–255. doi:10.1007/s10453-018-9510-1
Chen, X., Ran, P., Ho, K., Lu, W., Li, B., Gu, Z., et al. (2012). Concentrations and size distributions of airborne microorganisms in Guangzhou during summer. Aerosol.Air Qual.Res 12, 1336–1344. doi:10.4209/aaqr.2012.03.0066
Du, P., Du, R., Lu, Z., Ren, W., and Fu, P. (2018a). Variation of bacterial and fungal community structures in PM2.5 collected during the 2014 APEC summit periods. Aerosol Air Qual. Res. 18, 444–455. doi:10.4209/aaqr.2017.07.0238
Du, P., Du, R., Ren, W., Lu, Z., and Fu, P. (2018b). Seasonal variation characteristic of inhalable microbial communities in PM2.5 in Beijing city, China. Sci. Total Environ. 610, 308–315. doi:10.1016/j.scitotenv.2017.07.097
Fang, Z., Yao, W., Lou, X., Hao, C., Gong, C., and Ouyang, Z. (2016). Profile and characteristics of culturable airborne bacteria in Hangzhou, southeast of China. Aerosol Air Qual. Res. 16, 1690–1700. doi:10.4209/aaqr.2014.11.0274
Farhadkhani, M., Nikaeen, M., Adergani, B. A., Hatamzadeh, M., Nabavi, B. F., and Hassanzadeh, A. (2014). Assessment of drinking water quality from bottled water coolers. Iran. J. Public Health 43, 674–681.
Faridi, S., Bayat, R., Cohen, A. J., Sharafkhani, E., Brook, J. R., Niazi, S., et al. (2022). Health burden and economic loss attributable to ambient PM2.5 in Iran based on the ground and satellite data. Sci. Rep. 12, 14386. doi:10.1038/s41598-022-18613-x
Gao, M., Jia, R., Qiu, T., Han, M., Song, Y., and Wang, X. (2015). Seasonal size distribution of airborne culturable bacteria and fungi and preliminary estimation of their deposition in human lungs during non-haze and haze days. Atmos. Environ. 118, 203–210. doi:10.1016/j.atmosenv.2015.08.004
Goudarzi, G., Shirmardi, M., Naimabadi, A., Ghadiri, A., and Sajedifar, J. (2019). Chemical and organic characteristics of PM2.5 particles and their in-vitro cytotoxic effects on lung cells: the Middle East dust storms in Ahvaz, Iran. Sci. Total Environ. 655, 434–445. doi:10.1016/j.scitotenv.2018.11.153
Goudarzi, G., Soleimani, Z., Sorooshian, A., Marzouni, M. B., and Maleki, H. (2016). Impact of Middle Eastern dust storms on indoor and outdoor composition of bioaerosol. Atmos. Environ. 138, 135–143. doi:10.1016/j.atmosenv.2016.05.023
Harbizadeh, A., Mirzaee, S. A., Khosravi, A., Saleh Shoushtari, F., Goodarzi, H., Alavi, N., et al. (2019). Indoor and outdoor airborne bacterial air quality in day-care centers (DCCs) in greater Ahvaz, Iran. Atmos. Environ. 216, 116927. doi:10.1016/j.atmosenv.2019.116927
He, T., Jin, L., and Li, X. (2023). On the triad of air PM pollution, pathogenic bioaerosols, and lower respiratory infection. Environ. Geochem Health 45, 1067–1077. doi:10.1007/s10653-021-01025-7
Hospodsky, D., Yamamoto, N., and Peccia, J. (2010). Accuracy, precision, and method detection limits of quantitative PCR for airborne bacteria and fungi. Appl. Environ. Microbiol. 76, 7004–7012. doi:10.1128/AEM.01240-10
Hosseini, N., Hajizadeh, Y., Nikaeen, M., and Hatamzadeh, M. (2020). Spatiotemporal variation of ambient bioaerosols in a large and industrialized metropolis of Iran and their association with PM2.5 and meteorological factors. Aerobiologia 37, 105–117. doi:10.1007/s10453-020-09672-6
IQAir (2023). Air quality in esfahan. Goldach, Switzerland: IQAir. Available at: https://www.iqair.com/iran/isfahan/esfahan.
Jeon, E. M., Kim, H. J., Jung, K., Kim, J. H., Kim, M. Y., Kim, Y. P., et al. (2011). Impact of Asian dust events on airborne bacterial community assessed by molecular analyses. Atmos. Environ. 45, 4313–4321. doi:10.1016/j.atmosenv.2010.11.054
Johnson, E., and Choi, K. M. (2012). Lung cancer risk in workers in the meat and poultry industries–A Review. Zoonoses Public Health 59, 303–313. doi:10.1111/j.1863-2378.2012.01459.x
Kim, K. H., Kabir, E., and Jahan, S. A. (2018a). Airborne bioaerosols and their impact on human health. J. Environ. Sci. 67, 23–35. doi:10.1016/j.jes.2017.08.027
Lang-Yona, N., Dannemiller, K., Yamamoto, N., Burshtein, N., Peccia, J., Yarden, O., et al. (2012). Annual distribution of allergenic fungal spores in atmospheric particulate matter in the Eastern Mediterranean; a comparative study between ergosterol and quantitative PCR analysis. Atmos. Chem. Phys. 12, 2681–2690. doi:10.5194/acp-12-2681-2012
Li, Y., Lu, R., Li, W., Xie, Z., and Song, Y. (2017). Concentrations and size distributions of viable bioaerosols under various weather conditions in a typical semi-arid city of Northwest China. J. Aerosol Sci. 106, 83–92. doi:10.1016/j.jaerosci.2017.01.007
Lu, R., Li, Y., Li, W., Xie, Z., Fan, C., Liu, P., et al. (2018). Bacterial community structure in atmospheric particulate matters of different sizes during the haze days in Xi’an, China. Sci. Total Environ. 637, 244–252. doi:10.1016/j.scitotenv.2018.05.006
Madhwal, S., Prabhu, V., Sundriyal, S., and Shridhar, V. (2020). Ambient bioaerosol distribution and associated health risks at a high traffic density junction at Dehradun city, India. Environ. Monit. Assess. 192, 196. doi:10.1007/s10661-020-8158-9
Meklin, T., Reponen, T., McKinstry, C., Cho, S. H., Grinshpun, S. A., Nevalainen, A., et al. (2007). Comparison of mold concentrations quantified by MSQPCR in indoor and outdoor air sampled simultaneously. Sci. Total Environ. 382, 130–134. doi:10.1016/j.scitotenv.2007.03.031
Mirhoseini, S. H., Nikaeen, M., Khanahmad, H., Hatamzadeh, M., and Hassanzadeh, A. (2015). Monitoring of airborne bacteria and aerosols in different wards of hospitals-Particle counting usefulness in investigation of airborne bacteria. Ann. Agric. Environ. Med. 22, 670–673. doi:10.5604/12321966.1185772
Nikaeen, M., Hatamzadeh, M., Hasanzadeh, A., Sahami, E., and Joodan, I. (2009). Bioaerosol emissions arising during application of municipal solid-waste compost. Aerobiologia 25, 1–6. doi:10.1007/s10453-008-9102-6
Qin, T., Zhang, F., Zhou, H., Ren, H., Du, Y., Liang, S., et al. (2019). High-level PM2.5/PM10 exposure is associated with alterations in the human pharyngeal microbiota composition. Front. Microbiol. 10, 54. doi:10.3389/fmicb.2019.00054
Shammi, M., Rahman, M. M., and Tareq, S. M. (2021). Distribution of bioaerosols in association with particulate matter: a review on emerging public health threat in asian megacities. Front. Environ. Sci. 9, 698215. doi:10.3389/fenvs.2021.698215
Taghizadeh, F., Mokhtarani, B., and Rahmanian, N. (2023). Air pollution in Iran: the current status and potential solutions. Environ. Monit. Assess. 195, 737. doi:10.1007/s10661-023-11296-5
Tavangar, F. Z., Javeri, Z., Nikaeen, M., Sharafi, M., Mohammadi, M., Karimi, H., et al. (2023). Cytotoxicity and genotoxicity of fne particulate matter (PM2.5): a polluted city experiencing Middle East dust events. Air Qual. Atmos. Health. doi:10.1007/s11869-023-01480-4
Wei, M., Liu, H., Chen, J., Xu, C., Li, J., Xu, P., et al. (2020). Effects of aerosol pollution on PM2.5 associated bacteria in typical inland and coastal cities of northern China during the winter heating season. Environ. Pollut. 262, 114188. doi:10.1016/j.envpol.2020.114188
World Health Organisation (2022). Ambient (outdoor) air pollution. Available at: https://www.who.int/news-room/fact-sheets/detail/ambient-(outdoor)-air-quality-and-health.
Xu, C., Wei, M., Chen, J., Zhu, C., Li, J., Xu, X., et al. (2019). Profile of inhalable bacteria in PM2.5 at Mt. Tai, China: abundance, community, and influence of air mass trajectories. Ecotoxicol. Environ. Saf. 168, 110–119. doi:10.1016/j.ecoenv.2018.10.071
Keywords: air pollution, fine particles, bioaerosols, real-time PCR, dust events, health effect
Citation: Karimi H, Nikaeen M, Shamsizadeh Z and Hajizadeh Y (2024) Characterizing bioaerosols in PM2.5 in a semi-arid region experiencing desert dust events. Front. Environ. Sci. 11:1307426. doi: 10.3389/fenvs.2023.1307426
Received: 04 October 2023; Accepted: 26 December 2023;
Published: 16 January 2024.
Edited by:
Sirapong Sooktawee, Ministry of Natural Resources and Environment, ThailandReviewed by:
Heydar Maleki, Ahvaz Jundishapur University of Medical Sciences, IranCopyright © 2024 Karimi, Nikaeen, Shamsizadeh and Hajizadeh. This is an open-access article distributed under the terms of the Creative Commons Attribution License (CC BY). The use, distribution or reproduction in other forums is permitted, provided the original author(s) and the copyright owner(s) are credited and that the original publication in this journal is cited, in accordance with accepted academic practice. No use, distribution or reproduction is permitted which does not comply with these terms.
*Correspondence: Mahnaz Nikaeen, bmlrYWVlbkBobHRoLm11aS5hYy5pcg==
Disclaimer: All claims expressed in this article are solely those of the authors and do not necessarily represent those of their affiliated organizations, or those of the publisher, the editors and the reviewers. Any product that may be evaluated in this article or claim that may be made by its manufacturer is not guaranteed or endorsed by the publisher.
Research integrity at Frontiers
Learn more about the work of our research integrity team to safeguard the quality of each article we publish.