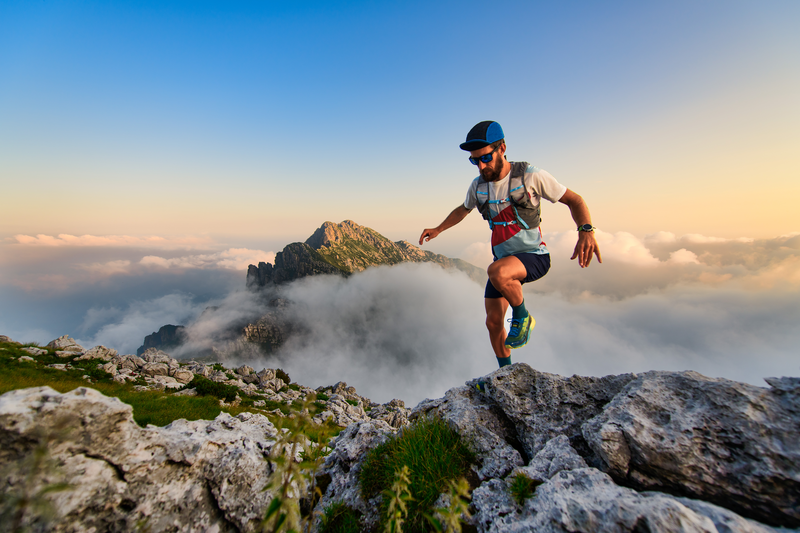
94% of researchers rate our articles as excellent or good
Learn more about the work of our research integrity team to safeguard the quality of each article we publish.
Find out more
ORIGINAL RESEARCH article
Front. Environ. Sci. , 06 November 2023
Sec. Environmental Economics and Management
Volume 11 - 2023 | https://doi.org/10.3389/fenvs.2023.1303792
This article is part of the Research Topic Sustainable Housing and New Perspectives in Urban and Rural Development View all 6 articles
This study explores optimizing waste resource efficiency through the Circular Economy (CE) framework. Motivated by the imperative to enhance resource efficiency and mitigate waste’s environmental impact, we examine the CE concept’s extension of product life cycles, while minimizing waste. We conduct a comprehensive review to explore the core CE principles across all stages of the product lifecycle and provide an in-depth analysis of waste treatment practices in Poland, emphasizing potential energy conversion methods like biogas production and incineration. Our findings underscore the importance of prioritizing waste incineration plant design for quicker payback, aligning with circular rational economic practices. Strategies such as improving biogas production, establishing solid bio-waste fermentation facilities, promoting sortable packaging, and incentivizing sustainable sorting emerge to optimize waste management. These findings highlight the pivotal role of economic, environmental, and energy considerations in shaping sustainable waste management strategies.
The concept of a circular economy (CE) has gained remarkable traction in recent years, presenting a compelling vision of our Earth as a closed-system spaceship where resources efficiently circulate. Within this grand metaphor, our global society, encompassing economic, environmental, social, and cultural facets, plays a pivotal role in maintaining equilibrium. One of the core tenets of CE, as advocated by the EU CE Strategy (EU COM/2015/0614), underscores the significance of waste management in achieving optimal environmental outcomes and reintroducing valuable materials into the economy to maintain sustainability. This article meticulously explores the waste management component within this celestial circular system.
Despite the prolific discussions surrounding CE, a universally accepted definition remains elusive, with over 200 variations scattered throughout scientific literature (Adamczak, 2022). CE, an evolutionary concept rooted in traditional waste management, holds relevance in both municipal and industrial domains. While scholarly discourse revolves around solid and liquid waste management, focusing on processing technologies, selective collection, and disposal methods, broader CE discussions, including the ambitious goal of achieving zero waste, often originate from the influential work of the Ellen MacArthur Foundation.
Curiously, amid these discussions, a significant research gap exists—the role of urban energy self-sufficiency within the CE framework. This research void has recently gained prominence, partly due to geopolitical events such as the Russia-Ukraine conflict, which cast a spotlight on concerns surrounding energy supply (Gabon et al., 2023; Apostu et al., 2023). Moreover, rapid economic and population growth over the past half-century has led to the excessive consumption of global resources, surpassing the Earth’s regenerative capacity (Popescu et al., 2022). Urbanization and population expansion have significantly escalated global material consumption, straining both individual and planetary resources. Remarkably, a mere 1% reduction in material and energy consumption across all sectors could generate an economy-boosting value of PLN 19.5 billion, underscoring the urgency of sustainable resource management (Deloitte, 2018). The extraction of raw materials, which has tripled from 1970 to 2017, now stands at a staggering 60 billion tons annually, equivalent to 22 kg per person daily (Fiksel, 2006). In the European Union alone, 2.2 billion tonnes of waste are generated annually, with municipal waste accounting for 27% of this total (Haglung, 2011). Alarmingly, resource extraction is responsible for 50% of human-generated carbon emissions and 90% of biodiversity impacts, with projections indicating a doubling of these impacts by 2060, according to the UN International Resource Panel (UN IRP) (Kamiński, 2020).
The central question arises: How can more reusable or recyclable fractions be extracted from this challenging mixed waste? Traditional solutions like increasing public education, while viable, may require substantial time for meaningful impact. Additionally, legal and financial restrictions may expedite educational efforts but could lead to social resistance and electoral consequences. These observations underscore the importance of redefining waste within the CE concept, emphasizing its potential as a valuable raw material (D’Admo et al., 2022; Luttenberger, 2020; Khan et al., 2023).
This article endeavors to bridge the gaps in waste management strategies within the broader context of the CE, with a specific focus on the potential for achieving energy self-sufficiency in cities. We assert that pursuing 100% recycling and reuse of all waste fractions is economically unwarranted. Instead, specific waste fractions, when transformed into biogas or incinerated for heat and electricity production, represent more effective waste management practices. Furthermore, the carbon dioxide emissions generated by combustion can be mitigated through urban transformation into green cities, where increased vegetation assists in absorbing carbon dioxide from the air.
To address these issues, we provide a comprehensive review focusing on the core principles of the CE and elucidating strategies for managing specific waste fractions. Our theoretical review provides a holistic perspective on the CE’s evolving discourse. Within this review, we delve into the multifaceted dimensions of the CE, dissecting its theoretical foundations, including principles of waste reduction, resource reuse, and the extraction of value from waste materials. This theoretical contribution enables a deeper understanding of the CE’s conceptual framework, offering valuable insights for researchers and policymakers engaged in sustainable waste management within this dynamic paradigm.
To illustrate the multifaceted dynamics of waste management within the context of CE principles, we present the Orli Staw installation plan, a municipal waste treatment facility in Poland serving 23 municipalities with 350,000 residents. This case exemplifies the innovative potential of the CE by harnessing kitchen and garden biowaste to produce biogas, thereby reducing waste, promoting resource reuse, and achieving local energy self-sufficiency. By focusing on bio-waste and employing a fermentation module for biogas generation, the plant redefines waste as a valuable resource. This groundbreaking approach not only aligns with CE principles but also addresses critical challenges related to resource scarcity and environmental sustainability. The Orli Staw plant’s transformation of waste into a valuable energy source offers a pioneering model for sustainable waste management, making it a significant case study with implications for circular economies worldwide.
The primary contribution of this article is the synthesis of insights into the role of waste management in achieving energy self-sufficiency within the CE framework. By highlighting waste management’s vital role in urban energy self-sufficiency, we emphasize the importance of a balanced approach to waste management and resource utilization. This synthesis provides practical insights into the integration of waste management strategies into the broader context of sustainable urban development, offering a nuanced perspective on this critical aspect of CE implementation.
In summary, our fundamental conclusion underscores the imperative for waste management to waste perceive as a dual asset: firstly, as a valuable raw material that can be reintegrated into the economy, and secondly, as a potent energy resource capable of enhancing the energy portfolio of cities. This dual perspective on waste not only aligns with the principles of CE but also offers a pragmatic solution to address contemporary challenges related to resource utilization and urban energy self-sufficiency.
The remainder of this paper unfolds as follows: In Section 2, we embark on a theoretical exploration, offering a comprehensive review of waste management within the context of the CE framework. Section 3 takes a deep dive into the practical realm, focusing on the Orli Staw case study in Poland, where we substantiate the concept of waste as a valuable resource for energy transformation with empirical evidence. Finally, Section 4 serves as the culmination of our discourse, where we draw our key findings, and articulate our conclusive remarks.
The view mentioned in the introduction that our planet is basically a huge spaceship speeding through space, recharged by solar energy and equipped with closed-circuit systems is becoming more and more popular in human society. There are many ways to understand and define the CE. One such definition defines the CE as a production and consumption model that involves sharing, borrowing, reusing, repairing, refurbishing and recycling existing materials and products for as long as possible. This extends the product life cycle. In practice, this means minimizing waste. When a product’s life cycle comes to an end, the raw materials and waste that comes from it should stay in the economy through recycling. They can be successfully reused, thus creating additional value. 1United Nations forecasts [based on the Food and Agriculture Organization (FAO) and the World Water Council] assume that by 2050 the world population will increase by 1/3, which will translate into the pace of moving to cities and a 50% increase in demand for water and energy during this period (Lasecki et al., 2021). Circular fashion has been present in the literature since the late 1960s. The concept of Circural Fashion appeared in 2014 and is currently one of the most popular concepts of sustainable development in fashion (Global Fashion Agenda’s 2020 Circural Fashion System Commitment was signed by 90 fashion brands). The phrases “spaceship Earth” (1960), “stocks for processing” (1966), The Closing Circle (1971), closed-loop economy (1981) alluded to CE (Meadows et al., 1973).
The CE paradigm began to develop in the early 1970s, when scientists, politicians and recruits gathered around the Club of Rome began research on the future of the earth’s natural environment, its limited raw material resources and the place occupied by humans in the ecosystem. The result of this research was the first report prepared for the Club of Rome entitled “Limits to Growth” (The Limits to Growth 1972). It was in this report that the idea of a CE emerged, where the authors of the report stated that: “Natural ecosystems can absorb many waste products of human activity and process them into substances useable, or at least harmless to other forms of life”. However, when a waste product is released in large quantities, natural absorption mechanisms can become saturated. The waste products of human civilization can accumulate in a given environment until they finally become visible, annoying and even harmful. Therefore, larger consuming countries can learn how to recover and regenerate used materials. They can develop new methods to increase the durability of products made from scarce raw materials. They can introduce social and economic patterns of behavior that would meet needs while minimizing (rather than maximizing) use and dissipation of irreplaceable substances that man currently possesses (Niutanen and Korhonen, 2003).
CE is an economic concept in which products, materials and raw materials should remain in the economy if possible, and the generation of waste should be minimized as much as possible (Oberle et al., 2019). This idea considers all stages of the product life cycle, starting from its design, through production, consumption, collection as waste, to its management. CE is a model of economic development in which–while maintaining the condition of efficiency–the following basic assumptions are met: 1) the added value of raw materials/resources, materials and products is maximized or the amount of waste generated is minimized, and the resulting waste is managed in accordance with the hierarchy of waste management methods (waste prevention, preparation for re-use, recycling, other recovery methods, disposal) (Pagotto and Halog, 2015)2.
The Ellen MacArthur Foundation3 states that the CE is based on three principles: 1) limit waste and pollution; 2) circulate products and materials (at their highest value)and 3) regenerate nature.
The first principle of the CE is to eliminate waste and pollution. Currently, our economy works in a take-make-waste system. We take raw materials from the Earth, we make products from them, and eventually we throw them away as waste. Much of this waste ends up in landfills or incinerators and is lost. This system cannot work in the long term because the resources on our planet are finite. The second principle of the CE is to circulate products and materials at their highest value. This means keeping materials in use, either as a product or, when that can no longer be used, as components or raw materials. This way, nothing becomes waste and the intrinsic value of products and materials are retained. The third principle of the CE is to regenerate nature. By moving from a take-make-waste linear economy to a CE, we support natural processes and leave more room for nature to thrive4.
The CE system diagram, known as the butterfly diagram illustrates the continuous flow of materials in a CE.5There are two main cycles–the technical cycle and the biological cycle. In the technical cycle, products and materials are kept in circulation through processes such as reuse, repair, remanufacture and recycling. In the biological cycle, the nutrients from biodegradable materials are returned to the Earth to regenerate nature5.
According to the Ellen MacArthur Foundation on the left-hand side of the butterfly diagram is the biological cycle, which is for materials that can biodegrade and safely return to the earth. 6This cycle mainly concerns products that are consumed, such as food. However, some other biodegradable materials, such as cotton or wood, may eventually make their way from the technical cycle into the biological cycle once they have degraded to a point where they can no longer be used to make new products. At the heart of the biological cycle is the concept of regeneration. It is the third principle of the CE. Instead of continuously degrading nature, as we do in the linear economy, in the CE we build natural capital. Once food is harvested and consumed, the nutrients in organic waste streams can be collected, and returned to the soil via processes like composting and anaerobic digestion. If nutrients are not returned, soil becomes depleted, meaning farmers are forced to rely increasingly on chemical fertilisers to keep farmland productive.
On the right-hand side of the butterfly diagram is the technical cycle, relevant for products that are used rather than consumed. The diagram shows that smaller inner loops are surrounded by the larger outer loops. These inner loops are where most value can be captured because they retain more of the embedded value of a product by keeping it whole. Take a smartphone, for example,–a working phone is worth more than the sum of its parts because the time and energy that went into making it is not lost. Therefore, inner loops like sharing, maintaining, and reusing should be prioritized above the outer loops that see the product broken down and remade. These loops also represent a cost saving to customers and businesses as they make use of products and materials already in circulation, rather than investing in making them new. 7The approach to CE is changing around the world. Not only as a problem to solve, but also as a business opportunity. For example, in Denmark, cooperation within the CE framework between business and industry is proposed in order to change the approach to production and consumption, creating new businesses and new jobs to make the lifestyle more sustainable (Luttenberger, 2020). Individual economic sectors are taking the essence of CE more and more seriously. One such example is the transformation from a linear to a circular approach in the food production industry in Australia. M. Pagotto and A. Halog (Pagotto and Halog 2015) present recommendations for this industry, based on the research of other scientists, which, among other things, are to improve environmental sustainability: “Our first recommendation is the implementation of a “sustainable resource management” (SRM) for the entire Australian agri-food industry chain. SRM is an integrated approach that manages materials/resource life cycles aiming to achieve economic efficiency and environmental sustainability (Fiksel, 2006). Implementing SRM will support the Australian food producers and suppliers in identifying inefficiencies in the production processes, reducing production and waste costs, increasing competitiveness and improving the producers. The second recommendation involves the implementation of integrated waste management, energy, and fertilizer production in large centralized (regional) units or smaller decentralized local units, such as farm scale or industrial districts (Niutanen and Korhonen 2003). This strategy can transform the current linear production system utilized in the Australian food industry to a roundput production system where the organic food wastes and wastewater are treated to produce energy, recycled water, and fertilizer, which are later returned as valuable inputs (Niutanen and Korhonen 2003). The third recommendation proposes the increase of biofuel/bioenergy production in Australia using available, nonfood biomass by-products generated by the agri-food industry. The Australian government should distribute financial incentives for constructing biorefineries. These biorefineries will transform organic materials, such as agricultural residues and food wastes, into valuable biofuels and chemicals (Pagotto and Halog, 2015). The last recommendation suggests that the Australian government should invest in research, professional training, and dissemination of the “mixed farming system” (MFS). This system will minimize the costs and emissions generated by transportation of feedstock that is required to feed the livestock raised in feedlots system (Pagotto and Halog, 2015).”
The diagram and its description present the essence of the CE in a nutshell. The practical application of this idea encounters some resistance in the societies of various countries. According to one of the laws of harmony presented by Karol Adamiecki: “The Law of Inertia of Habits and the Law of Contradiction,” any undertakings involving the introduction of specific innovations or improvements in the company evoke a certain resistance in the employee teams aimed at rejecting this change and restoring the current state. Therefore, it is of great importance to properly prepare changes, train employees and participate in the process of implementing improvements, limiting the scale of this resistance. 8,9The quoted law of inertia of habits and the law of contradiction can be applied not only to the employees of a given enterprise, but also to individual governments or population groups. One such exception is the Japanese city of Kamikatsu, where a special recycling center has been set up, where over 30 waste fractions are sorted. Thanks to such solutions, as much as 80 percent waste produced by the local community is reused, recycled or composted. Only 20 percent goes to landfills. Kamikatsu inspires other cities, including Turkey and San Diego, where a plan was announced to reduce landfilled waste by 75% by 2030. By 2040, it would become completely zero waste. New York has similarly ambitious plans—it wants to reduce the amount of garbage to zero in the next 15 years10.
Other examples of the transformation of waste management towards CE exemplified in Europe. Sweden use of heat generated in wastewater fermentation processes in heat pump systems. According to Gunnar Haglung, Haglung, 2011 about 80% of the heat in the Swedish district heating network, i.e. 40% of all heat, comes from energy sources that in many other countries of the world are not used at all but literally wasted. G. Haglung proposes that cities with up to 20,000 inhabitants residents invested in biomass-fired heating plants. In turn, cities with a population of 20,000 inhabitants up to 100 thousand inhabitants should invest in biomass-fired CHP plants, and in cities with more than 100,000 inhabitants, municipal waste should be managed on site, among others in incineration plants in cogeneration with the use of biomass. In addition, Sweden also introduced a tax on CO2 and for incineration plants operating without cogeneration in the generation of heat and electricity. Segregation at source popularized in Poland by Eng. Andrzej Bartoszkiewicz. In addition, since 2005, a full ban on landfilling has been in force, which was widely consulted with the Swedish society. Heat in Swedish cities equipped with district heating is supplied in addition to incineration plants, biogas, geothermal energy, heat pumps or fossil fuels from industrial heat waste. The Swedish example described shows the country’s determination towards CE and the pursuit of energy independence. The entire economic mechanism is that energy is produced from waste delivered to, e.g., incineration plants, the cost of which is lower compared to coal or gas. Those who deliver waste must pay for it, which on the one hand, as mentioned, reduces the costs of energy production, and on the other hand, in a way, stimulates society to produce less waste, for the collection of which they also must pay. Swedish society has agreed to this transformation. Other societies are slower to adopt similar solutions.
Due to the extensive discussion taking place on increasing the efficiency of the use of waste resources and thus converting energy, it is worth analyzing the possibilities of biogas production in solid waste processing installations. The presented case study concerns one of the most dynamically developing installations in Poland, which sorts waste, both municipal and industrial, for the recovery of the so-called commercial fractions and biodegradable waste for biogas production. Therefore, it seems that this example is worth disseminating where the preference is still mainly to store waste without considering it as a source of commercial products on the one hand, and on the other hand, the possibility of achieving energy independence first of all for a given installation, and then of other municipal entities or farms. homework.
In Poland, the first installations producing biogas from municipal waste were built in 2010-2017. Most of them use a biofraction separated from mixed/residual waste. Currently, most of them plan to change the input to selectively collected bio-waste. Some of them have undertaken investment activities in this area. Biogas produced from mixed waste is characterized by a high content of H 2 S reaching 2,000 ppm and a high content of silicon compounds (siloxanes), which requires its cleaning and makes it difficult to operate cogeneration engines. The average biogas productivity in such installations is in the range of 80–120 m3/Mg (source: Szewczyk’s own survey). So far, in Poland there is no installation producing biomethane from biogas, injected into the grid or used to drive means of transport in the form of bioCNG or bioLNG. This is due to the lack of favorable legislation enabling the injection of biomethane into the grid. Powering vehicles with biomethane is also at the planning stage. In all installations operating in Poland that process solid waste, the generated electricity and heat is used for its own needs, and surplus electricity is sold to the grid. One of the installations operates in the trigeneration mode and has a bromolite absorption refrigeration system. This system generates cold from the waste heat, which is used for air-conditioning the rooms.
Currently, in Poland, approximately 200,000 Mg/year of bio-waste is managed in operating fermentation installations. Generally, it is estimated that the amount of biowaste generated in Poland exceeds 2,000,000 Mg/year. With a conservative estimated average productivity of operating installations of approx. 100 m (Fiksel, 2006)/Mg, this gives a potential biogas production capacity of approx. 200 million m (Fiksel, 2006)/year. This biogas has a methane content of 50%–60%. These installations also have the ability to co-digestate other types of waste, not only from municipal sources, but also from trade, catering and agri-food processing, which can significantly increase Poland’s potential in this area. The advantage of this type of installation is their territorial dispersion as well as the stability and predictability of energy production, as well as its controllability, which, unlike other RES (wind, sun), stabilizes the power grid, reducing the need for its expansion.
In many societies processes like Kamikatsu proceed relatively slowly. This is illustrated by the example of the Municipal Waste Treatment Plant Orli Staw (ZUOK). This plant serves 23 municipalities with a population of approximately 350,000. These inhabitants produce approx. 120,000 Mg/year of municipal waste. All generated municipal waste goes to the ZUOK. This stream also includes selectively collected kitchen and garden biowaste. The installation in Orli Staw, as in other installations in Poland, the level of recycling and its structure are presented in Table 1.
TABLE 1. Practical possibilities of achieving recycling levels on the example of the installation in Orli Staw.
The data presented in Table 1 shows that the efficiency of waste segregation in some European societies is low. The so-called mixed waste (unsegregated) from 50%–80% in the structure of waste collected from residents. From a practical point of view, this waste is basically the easiest to landfill or burn to obtain electricity and heat. However, the question is how can more reusable or recyclable fractions be obtained from this mixed waste? The first answer that comes to mind is to increase the level of education of the inhabitants. This process may take many years. Legal and financial restrictions can also be applied, which theoretically should accelerate the education process, or trigger social protests, which then translate into the results of elections to central or local authorities. Generally, the idea of CE is to define waste not as waste, but as a raw material. In the Orli Staw plant, the focus is now on the energy use of bio-waste by installing a module for obtaining biogas from this waste (fermentation module). This module uses both green and kitchen waste. The technology used is so universal that it produces biogas even from contaminated biodegradable fractions. This biogas is then used to produce electricity and heat for the plant, and surpluses are stored for resale. From the residues of these wastes after the fermentation and composting process, solid and liquid fertilizer is obtained. According to P. Szewczyk, the solution used for selectively collected municipal bio-waste is better than in the case of rural biogas plants, which require a more stable and clean material input.
The fermentation installation at ZUOK Orli Staw was built in 2021-2022 and commissioned in 2023. After the first stage of implementation, it has the capacity to process 15,000 Mg/year. The input to the installation is mainly selectively collected municipal bio-waste. Since 2017, when the implementation of selective collection was successively started, their number has been gradually increasing. In addition, the fermentation charge can also be expired food and expired food products from trade and gastronomy. It is also possible to manage liquid waste from the agri-food industry and agriculture. The amount of delivered bio-waste is gradually increasing. In 2016, there were 4,122 Mg, in 2017–5,372 Mg, in 2018 already 6,332 Mg, in 2019–7,836 Mg. In 2020, the amount of biowaste supplied increased significantly to 14,442 Mg, a year later to 17,548, and in 2022 to 18,528 Mg. This phenomenon is shown in Figure 1.
FIGURE 1. Delivery of selectively collected municipal bio-waste to ZUOK (Orli Staw) in 2016-2022 Source: own study based on data from ZUOK (Orli Staw).
In the period from 1 March 2023 to 31 May 2023, during the start-up of the fermentation installation, the average biogas productivity was 98.96 m (Fiksel, 2006)/Mg of feedstock. At that time, after the injection of the inoculum and the start of the process, the amount of fed waste gradually increased, reaching the target value of approx. 41 Mg/day. This amount was corrected based on the analysis of parameters and the course of the fermentation process. The produced biogas has an average methane content of 60.17%. This content ranges from 59% to 62.2%.
The produced biogas has an H 2 S content of approx. 200 ppm. Before being directed to the cogeneration engine, the biogas is purified in a biological column and/or in columns filled with activated carbon. Biogas is also dried in refrigeration dryers. The cogeneration unit with a peak power of 550 kW operates with variable power depending on the current energy needs of the plant. The heat energy recovered from the cooling of the cogeneration engine and the cooling of exhaust gases is directed to the in-house network. It is used for the technological needs of the fermentation installation, mainly for heating the fermenter, heating the premises of the plant and producing hot water. The role of energy storage in the form of biogas is performed by a double-layer biogas tank with a capacity of approx. 2,500 m (Fiksel, 2006).
After the fermentation process, the digestate goes to the dewatering section consisting of two screw presses and one decanter centrifuge. The solid fraction goes to the aerobic process and then is a substrate to produce a natural organic fertilizer. The liquid fraction is directed to a buffer tank and then used in agricultural fields as an organic fertilizer.
The fermentation installation at the ZUOK, after extension with a second fermenter with a capacity of approx. 15,000 Mg/year, is planned to operate as a biomethane production installation in the form of bioCNG or bioLNG. It will be the fuel used to propel own means of transport and possibly also used as fuel for the needs of companies cooperating with the ZUOK collecting waste from residents.
Another possibility of energy use of waste are wastewater treatment plants where biogas is also obtained. An example is the treatment plant in Tychy in Silesia, where biodegradable industrial waste is added to municipal sewage, which improves the amount of biogas obtained. From this biogas, electricity and heat are obtained in such quantities that, apart from the wastewater treatment plant’s own consumption, electricity and heat are used to operate the municipal aqua park, and surplus electricity is sold to the power grid. The uniqueness of this solution lies in the fact that in the vast majority of sewage treatment plants energy and heat are used only for their own purposes.
The motivation of this paper stems from the use of CE in practice in the context of waste management. This concept is becoming more and more popular. Waste is no longer treated as unnecessary garbage, but as raw materials from which new goods can be produced or transformed, for example, into heat or electricity. Biogas can be obtained from other waste, which in turn can be used to power engines or produce electricity and heat. The transformation of many economies, including European ones, towards CE also seems to be a necessity due to the ongoing Ukrainian-Russian conflict, which has disrupted the established economic order consisting in, among other things, purchasing cheap energy carriers from Russia. This conflict thus highlighted the risk of energy carriers supplied from one or a few places. This also includes the potential risk of interrupting the supply of plutonium or uranium ore to nuclear power plants from their current mining sites. Using the potential of waste and popularizing the opportunities offered by broadly understood CE seems to be invaluable.
In addition, experience in implementing CE solutions may be useful for planned space travel. The example from Orle Staw shows dynamic changes in the approach to waste in Poland. From a financial point of view, the installation from Orly Staw generates profit, which is then transferred to the development of this company towards CE. More and more cities in Poland are starting to transform their installations in a similar way. It is a slow but steady process. Based on the example from Orle Staw and other examples described in the literature review, the following actions are recommended.
1. The design of incineration plants should assume the shortest possible payback period for investment outlays. In Sweden, for example, the payback period for the construction of a waste incineration plant is approximately 4–5 years, in Poland it is 20–30 years. This proves a more rational approach to the CE in this country from an economic point of view.
2. Such incineration plants should be built not only to dispose of waste while generating electricity and heat, but also as cement plants. The combustion temperature in a cement plant is higher than in an incinerator, which provides greater opportunities for environmentally safe disposal of hazardous waste, which may even be present in municipal waste. Moreover, the current Ukrainian-Russian war will end 1 day and the demand for cement will certainly increase, which rationally justifies the construction of such combined plants. In this way, flammable waste fractions can be used more effectively.
3. It is postulated to reconstruct each wastewater treatment plant so that it produces a larger amount of biogas than its own needs, also using industrial bio-waste.
4. Fermentation plants for the production of biogas from solid bio-waste should be built at each waste collection facility. This biogas would be used to produce heat and electricity for own needs and for sale, including as biofuel for engines.
5. Packaging should consist of materials that can be easily sorted. The effort here is also on the side of packaging manufacturers. In societies less convinced of such segregation at source, economic incentives to donate such packaging should be introduced. It would be worth applying an additional waste management hierarchy, i.e., separating cost-effective waste fractions such as aluminum, other metals, glass, waste paper, biodegradable and other economically viable fractions, and those that should only be treated as combustible material.
6. Collection points for such packaging should be located in places frequented by residents, e.g., shops, so that the resident does not feel the inconvenience of such a delivery of used packaging.
7. The logistics of collecting used packaging from such points should be improved. For example, each of the 14 distribution centers of one of the largest retail chains in Poland sends around 200 vehicles daily to deliver supplies to its retail outlets. These vehicles return to the city center lightly loaded or empty, covering around 20,000 km on the way back. Annually, this gives one center (assuming only 300 days of operation per year) 6,000,000 km, and for the entire network: 90,000,000 km. Fuel used when running empty: 20,700,000 L 22If those delivering products to stores collected used packaging at the same time, i.e., they would also provide collection services for such selected waste fractions, the number of so-called waste collections could be reduced. empty runs.
8. Disseminate the so-called sorting at source using efficient logistics. The solution proposed, among others, by A. Bartoszkiewicz assumes the employment of people in the so-called houses—sorting rooms located in multi-family buildings. These people would perform additional sorting of mixed waste on site. Separated materials would be collected, for example, by vehicles delivering products to nearby stores, in order to avoid empty journeys and reduce transport costs. You can also consider running a deposit system by the operators of such a sorting house.
The presented recommendations and examples, including the example of the Orle Staw installation, may constitute a model or anchor for improving the efficiency of the economy thanks to the CE concept. It is not a problem to transform the linear economy into a circular one by spending huge sums of money. The challenge and logical path is to do this while improving the economic efficiency of given industries or enterprises. One such example, apart from others mentioned in the article, is the constant transformation of the Orli Staw installation, which is slowly becoming an enterprise stimulating the effective management of solid waste. In the future, new activities in the CE area may appear at this installation based on economic calculations, involving even various social and business groups in conducting these activities, which will also allow for the expansion of the CE idea in Polish society.
The original contributions presented in the study are included in the article/supplementary material, further inquiries can be directed to the corresponding author.
HE: Conceptualization, Investigation, Methodology, Project administration, Supervision, Writing–review and editing. GZ: Conceptualization, Data curation, Formal Analysis, Methodology, Writing–original draft. W-CA: Project administration, Writing–review and editing. SP: Data curation, Investigation, Resources, Writing–original draft.
The author(s) declare that no financial support was received for the research, authorship, and/or publication of this article.
The authors declare that the research was conducted in the absence of any commercial or financial relationships that could be construed as a potential conflict of interest.
All claims expressed in this article are solely those of the authors and do not necessarily represent those of their affiliated organizations, or those of the publisher, the editors and the reviewers. Any product that may be evaluated in this article, or claim that may be made by its manufacturer, is not guaranteed or endorsed by the publisher.
1Circular Economy: Definition, Meaning and Benefits (Video) | News | European Parliament (europa.eu).
2https://www.gov.pl/web/Rozwoju-technologia/Gospodarka-o-ogu-zamknietym
3https://www.ellenmacarthurfoundation.org/circular-economy/concept
4What is a circular economy? | Ellen MacArthur Foundation
5The Butterfly Diagram: Visualizing the Circular Economy (ellenmacarthurfoundation.org)
6The biological cycle of the butterfly diagram (ellenmacarthurfoundation.org)
7The technical cycle of the butterfly diagram (ellenmacarthurfoundation.org)
8One Earth is not enough for us. We would use up five planets in a year - Forsal.pl
9Karol Adamiecki – Management Encyclopedia (mfiles.pl)
10https://smoglab.pl/miasteczka-zero-waste-first-dziala-w-japonii-kolejne-powzanie-w-turcji/
Adamczak, J. (2022). Restructuring a municipal enterprise towards a circular economy on the example of the waste management industry. Warsaw: Oficyna Wydawnicza SGH.
Apostu, S. A., Gigauri, I., Panait, M., and Martín-Cervantes, P. A. (2023). Is Europe on the way to sustainable development? Compatibility of green environment, economic growth, and circular economy issues. Int. J. Environ. Res. Public Health 20 (2), 1078. doi:10.3390/ijerph20021078
D'Adamo, I., Mazzanti, M., Morone, P., and Rosa, P. (2022). Assessing the relation between waste management policies and circular economy goals. Waste Manag. 154, 27–35. doi:10.1016/j.wasman.2022.09.031
Deloitte (2018). Closed loop – open opportunities, prospects for the development of the circular economy in Poland. Report: Closed loop - open opportunities | Deloitte Polska, entry date September 26, 2023).
Fiksel, J. (2006). A framework for sustainable materials management. JOM 5 (8), 15–22. doi:10.1007/s11837-006-0047-3
Gabor, M. R., López–Malest, A., and Panait, M. C. (2023). The transition journey of EU vs. NON-EU countries for waste management. Environ. Sci. Pollut. Res. 30 (21), 60326–60342. doi:10.1007/s11356-023-26686-y
Kamiński, A. (2020). The impact of the circular economy on refinery operations. Pol. J. Sustain. Dev. 24 (2).
Khan, S. A. R., Zia-Ul-Haq, H. M., Ponce, P., and Janjua, L. (2023). Re-investigating the impact of non-renewable and renewable energy on environmental quality: a roadmap towards sustainable development. Resour. Policy 81, 103411. doi:10.1016/j.resourpol.2023.103411
J. Kulczycka (Editor) (2019). “Circular economy in policy and scientific research,” Institute of mineral resources and energy management of the polish academy of sciences (Kraków). 978-83-955544-5-2. 978-83-956380-0-8.
T. Lasecki, T. Tamecki, M. Biernat-Kopczyńska, and R. Maruszkin (Editors) (2021). Circular Economy. Guide for small and medium-sized entrepreneurs (Warsaw: Polish Agency for Enterprise Development).
Luttenberger, L. R. (2020). Waste management challenges in transition to circular economy–case of Croatia. J. Clean. Prod. 256, 120495. doi:10.1016/j.jclepro.2020.120495
Meadows, D. H., Meadows, D. L., Randers, , and Behrens, W. W. (1973). The Limits of growth. Warsaw: National Economic Publishing House.
Niutanen, V., and Korhonen, J. (2003). Industrial ecology flows of agriculture and food industry in Finland: utilizing by-products and wastes. Int. J. Sustain. Dev. World Ecol. 10 (2), 133–147. doi:10.1080/13504500309469792
Oberle, B., Bringezu, S., Hatfield-Dodds, S., Hellweg, S., Schandl, H., and Clement, J. (2019). Global resources outlook 2019. Natural resources for the future we want. United Nations Environment Programme. International Resource Panel. 978-92-807-3741- 7 DTI/2226/NA UNEP 252.
Pagotto, M., and Halog, A. (2015). Towards a circular economy in Australian agri-food industry an application of input-output oriented approaches for analyzing resource efficiency and competitiveness potential. J. Industrial Ecol. 20, 1176–1184. Number 5, Yale University. doi:10.1111/jiec.12373
Popescu, C., Hysa, E., Kruja, A., and Mansi, E. (2022). Social innovation, circularity and energy transition for environmental, social and governance (ESG) practices—a comprehensive review. Energies 15 (23), 9028. doi:10.3390/en15239028
Szewczyk, P. (2023). Waste treatment system in the light of the implementation of legal requirements and their energy use case study - Orli Staw - conference materials: challenges and opportunities for the national municipal waste management system. Webinar Organ. by Finance Inst. Warsaw Sch. Econ. on June 6.
The business of a circular future (2021). How business models can accelerate the transition to a circular economy. WHITE PAPERS FOR A GREEN TRANSITION. Version 1.0.
Keywords: circular economy, resource efficiency, sustainability, energy conversion, waste management, resource optimization
Citation: Elroi H, Zbigniew G, Agnieszka W-C and Piotr S (2023) Enhancing waste resource efficiency: circular economy for sustainability and energy conversion. Front. Environ. Sci. 11:1303792. doi: 10.3389/fenvs.2023.1303792
Received: 28 September 2023; Accepted: 18 October 2023;
Published: 06 November 2023.
Edited by:
Klara Cermakova, University of Economics, CzechiaReviewed by:
Bozena Kaderabkova, Czech Technical University in Prague, CzechiaCopyright © 2023 Elroi, Zbigniew, Agnieszka and Piotr. This is an open-access article distributed under the terms of the Creative Commons Attribution License (CC BY). The use, distribution or reproduction in other forums is permitted, provided the original author(s) and the copyright owner(s) are credited and that the original publication in this journal is cited, in accordance with accepted academic practice. No use, distribution or reproduction is permitted which does not comply with these terms.
*Correspondence: Hadad Elroi, aGFkYWRlbEBzY2UuYWMuaWw=
Disclaimer: All claims expressed in this article are solely those of the authors and do not necessarily represent those of their affiliated organizations, or those of the publisher, the editors and the reviewers. Any product that may be evaluated in this article or claim that may be made by its manufacturer is not guaranteed or endorsed by the publisher.
Research integrity at Frontiers
Learn more about the work of our research integrity team to safeguard the quality of each article we publish.