- 1College of Agriculture and Bioengineering, Heze University, Heze, Shandong, China
- 2School of Pharmacy, Qingdao University, Qingdao, China
- 3Research and Development Department, NanoPeptide (Qingdao) Biotechnology Ltd., Qingdao, China
Micro/nano plastics (M/NPs) are emerging pollutants that have extensively infiltrated various aspects of human life, posing a significant threat to the natural ecological systems. M/NPs can enter the digestive system through the oral cavity and accumulate in various organs. The current research on M/NPs primarily relies on model organisms, and there remains a dearth of direct evidence concerning the impact of M/NPs on human health. Commonly utilized specific two dimensional (2D) cultured cell lines exhibit substantial disparities in physiological functions when compared to multicellular tissues in vivo. The conduct of animal experiments is a time-consuming process, constrained by ethical considerations, and also confronted with interspecies variations. A significant breakthrough in biology is the development of organoids derived from stem cells. Intestinal organoids can mimic the complex structure and functionality of tissue, and can generate cell-cell and cell-matrix interactions that closely resemble physiological responses in the body. As a result, they provide a more accurate reflection of toxic effects and mechanisms, and hold great potential for applications in the environmental toxicology assessment. However, the current research on the toxic mechanisms of M/NPs using intestinal organoids is still in its early stages. The focus of this review is on the application of intestinal organoids in toxicology studies of M/NPs, assessing the correlation between M/NPs and diseases, as well as elucidating the molecular mechanisms underlying toxic effects. Ultimately, we present the challenges and potential solutions for utilizing intestinal organoids as models to evaluate M/NPs-induced toxicity, aiming to provide valuable insights for future research.
1 Introduction
Micro/nano plastics (M/NPs) has permeated various facets of human existence and poses a pervasive and substantial threat to terrestrial and marine ecosystems (Kiran et al., 2022; Pires et al., 2022; Wang et al., 2022). These M/NPs are mainly ingested orally, absorbed by the human digestive system, and ultimately accumulate in the human body, posing a substantial risk to human health (Kaur et al., 2022; Thodhal et al., 2023). The long-term exposure and ingestion of pollutants may elevate the susceptibility of human beings to a range of diseases (Prata et al., 2020; Kumar et al., 2022). However, the direct effects of MPs on human health still lack sufficient data. Immortalized cells are commonly employed as a model for toxicity studies; however, due to their limited complexity, in vitro two-dimensional models fail to accurately depict toxic effects and predict actual in vivo reactions (Ben-David et al., 2018; Liu et al., 2019). Although animal models have long been considered the gold standard for toxicological research, in vivo experiments suffer from low throughput, high cost, labor-intensive procedures, time-consuming processes, and ethical concerns (Dothel et al., 2013; Faiola et al., 2015). Given the inherent interspecies differences and the limited capacity of animals to exhibit complex human responses to stimuli, the findings from animal experiments have been a subject of controversy (Bennekou, 2019). In recent years, with the rapid advancements in three dimensional (3D) culture technology and stem cell research, 3D organoids derived from adult stem cells, embryonic stem cells, and induced pluripotent stem cells have emerged as indispensable models for studying complex multicellular organ development (Clevers, 2016; Fernandes, 2023; Yang et al., 2023). These models effectively replicate morphogenesis, cell-cell interactions, and molecular processes during early human organ development (Yin et al., 2016; Dutta et al., 2017). The utilization of human intestinal organoids has been increasingly employed for the evaluation of the toxicity associated with various environmental pollutants, encompassing drug compounds, heavy metals, persistent organic pollutants, nanomaterials (Alexander et al., 2016; Forsythe et al., 2018; Cheng et al., 2022; Huang et al., 2022). However, the application of intestinal organoids in the field of toxicity assessment of environmental M/NPs is still in its early stages. This review focuses on intestinal organoids as a evaluating the association between M/NPs as well as elucidating the underlying molecular mechanisms. Lastly, we present the challenges and strategies involved in utilizing intestinal organoids as models to assess the toxicity of contaminants.
2 M/NPs in environment
Plastic pollution poses a significant threat to natural ecosystems, with an estimated production of 8.3 billion tons of virgin plastic as of 2017, and this figure is projected to double by 2050 (Geyer et al., 2017). These plastics tend to degrade into particles ranging from 1 to 1,000 μm in diameter, referred to as Microplastics (MPs), while plastic particles between 1 and 100 nm (or up to 500 nm in some cases) are known as Nanoplastics (NPs) (Campanale et al., 2020). The main chemicals of M/NPs include polyethylene, polystyrene, polypropylene, polyethylene terephthalate, and polyvinyl chloride (Avio et al., 2017). These M/NPs can be released from plastic waste, industrial emissions, and wastewater, and can infiltrate terrestrial, freshwater, and marine ecosystems spanning from the equator to the poles and from surface waters to deep-sea sediments. M/NPs are found in all environmental media including seafood, plants, animals, salts, and drinking water (Wang et al., 2016). Recent studies have revealed an average presence of 20 MPs (ranging from 50 to 500 µm) per every 10 g of human feces, with polypropylene and polyethylene terephthalate being the most prevalent types (Shen et al., 2019). This provides substantial evidence for the accumulation, ingestion, and excretion of M/NPs by humans (Schwabl et al., 2019). The inclusion of M/NPs in the United Nations Environment Programme (UNEP) yearbook for 2014 highlights their hazardous nature as one of the top ten urgent global challenges facing our planet today (Kiran et al., 2022). The presence of M/NPs in air, water, and food can lead to ecotoxicological issues. The introduction of M/NPs results in growth retardation, reduced fecundity, weakened immunity, and reproductive system malformations in both animals and humans. Additionally, M/NPs can serve as carriers for adsorbing persistent organic pollutants, trace metals, and harmful additives that are several times higher than those found in natural sediments. The primary route of exposure to M/NPs is through the consumption of contaminated food and water which has been linked to gastrointestinal diseases such as decreased epithelial permeability, local inflammatory processes, changes in intestinal microbiota composition (Waring et al., 2018; Fackelmann and Sommer, 2019), redox imbalance disruption of energy homeostasis and toxicity within the gut and kidneys (Deng et al., 2017). Inhalation of M/NPs can induce rapid bronchospasm, diffuse interstitial fibrosis, inflammatory and fibrotic alterations in bronchial and peribronchial tissues, as well as alveolar interstitial lesions (Mariano et al., 2021). The endocytic mechanism enables small polystyrene and polyvinyl chloride particles (<150 nm) to permeate the intestinal wall and eventually access the lymph nodes and bloodstream (Xu et al., 2020). The escalating plastic pollution along with increasing annual exposure to plastic particles has resulted in an elevated risk of human diseases. There is an urgent imperative to investigate the impact of M/NPs on internal organs in humans, which is pivotal for comprehending their pathogenic mechanisms and evaluating their potential threat to human health.
3 Intestinal organoids
Intestinal organoid construction technology utilizes induced pluripotent stem cells, embryonic stem cells, and adult stem cells to generate tissues that simulate normal intestinal function (Pleguezuelos-Manzano et al., 2020; Zhao et al., 2020; Tian et al., 2023). This approach enables investigation of personalized sources of intestinal cells to study intestinal diseases (Almeqdadi et al., 2019). The widely used method for culturing intestinal organoids was initially described by Sato and Clevers in 2009 (Sato et al., 2009). Small intestine crypts isolated in vivo are embedded in matrigel and cultured using medium containing various growth factors such as R-spondin 1, EGF, and Noggin. These crypts form closed structures with apoptotic cells within their lumen. Small intestine organoids accurately replicate both crypts and villi structures while encompassing all differentiated types of intestinal cells including paneth cells, absorptive enterocytes, colonic cells, goblet cells, and enteroendocrine cells. In 2011, Spence et al. (2011) initiated their study by utilizing pluripotent stem cells and employing a sequential manipulation of various growth factors to faithfully replicate the process of embry gut development in vitro. As a result, they successfully generated three-dimensional intestinal organoids that comprised polarized columnar epithelium forming villus-like and cryptlike structures. The study by Workman et al. (2017) represent the first successful integration of human pluripotent stem cell-derived neural crest cells with pluripotent stem cell-derived human intestinal organoids, enabling the replication of normal gut enteric nervous system development in vitro. Through migration into the mesenchium of intestinal organoids, these recombinant neural crest cells exhibit self-organization andial cell types, ultimately resulting in the construction of functional enteric nervous system within an intestinal organoid. Nikolaev et al. (2020) employed a hybrid microchip system by integrating a mixed colloid of type I collagen and Matrigel into a perfusable platform. This innovative system facilitated the generation of tubular epithelium accessible lumen and spatial arrangement resembling crypt and villus-like domains in vivo using intestinal stem cells. By connecting the microgut tube to an external pump system, it became perfusion-able, enabling continuous removal of dead cells to prolong tissue viability for several weeks. Additionally, this tube could be colonized with microorganisms to simulate host-microbe interactions. Intestine organoids have been utilized for investigations of gut physiology and disease exploration (Almeqdadi et al., 2019), including drug discovery via high-throughput screening (Du Y et al., 2020), exploration of host-microbe interactions by co-culturing with pathogens (Puschhof et al., 2021; Bozzetti and Senger, 2022; Adeniyi-Ipadeola et al., 2023), induction of somatic mutations using gene editing technologies such as CRISPR-Cas9 to target specific mutations (Martinez-Silgado et al., 2022). Intestinal organoids are crucial models for toxicological studies. Devall et al. (2021) assessed the impact of alcohol exposure on colonic organoids and demonstrated that alcohol had a modest effect on cell growth and viability while resulting in significantly distinct transcriptomic responses in treated versus control organoids. Graphene quantum dots can effectively reduce the size of intestinal organoids. Furthermore, intestinal organoids can be employed to evaluate the adverse effects of environmental pollutants on the intestine and investigate related mechanisms (Yu et al., 2019).
4 Intestinal oganoids for toxicity evaluation of M/NPs
The effects of M/NPs on human intestinal health have been extensively investigated using various in vitro and animal models, including zebrafish and mice as in vivo models (Bhagat et al., 2020; Zhao et al., 2021; Da et al., 2022), as well as Caco-2 and HT29-MTX cell models (Stock et al., 2019; Busch et al., 2021a; Busch et al., 2021b). However, there is still insufficient evidence regarding the intestinal toxicity of M/NPs in physiologically relevant human models (Forsythe et al., 2018). Organoids have a spatial organization structure composed of heterogeneous tissue-specific cells and cell-cell-extracellular matrix interactions (Almeqdadi et al., 2019). With the development of stem cell technology and 3D culture technology, the environmental toxicity assessment model has also been transformed from 2D model to 3D organoid model, which is helpful for high-throughput screening and toxicological application in vitro.
The intestinal epithelium is composed of a diverse array of differentiated epithelial cells, including enterocytes, goblet cells, enteroendocrine cells, paneth cells, and microfold cells (Sinagoga and Wells, 2015), which not only play a primary role in nutrient digestion and absorption but also provide a protective barrier against physical, chemical, and biological damage through apical intercellular junctions (Chen et al., 2015). The interaction between M/NPs and human intestinal cells has been mainly studied using monocultures of human colorectal adenocarcinoma epithelial cell lines Caco-2 or HT29-MTX (Visalli et al., 2021; Gautam et al., 2022). To achieve more physiological conditions for in vitro experiments, many researchers have used the Caco-2/HT29-MTX co-culture system as an experimental model (Fournier et al., 2021). Some investigators have even incorporated lymphoblastoid Raji-B cells to induce Caco-2 into microfold-like structures (Mariano et al., 2021). Although these studies have demonstrated a certain level of cellular uptake and epithelial transport of M/NPs, the majority of them have indicated minimal or insignificant cytotoxic effects, except at extremely high particle concentrations (Banerjee and Shelver, 2021). The toxic effect of M/NPs on the intestine may be disregarded or significantly underestimated in conventional culture systems (Liang et al., 2021). Human intestinal organoids can serve as viable substitutes for investigating the toxic effects of M/NPs on the human intestine. By employing morphological alterations, gene mutations, transcriptional analysis, and pathological examination, potential drug targets and pathways can be identified to facilitate the advancement of novel disease biomarkers and treatment strategies (Figure 1). Human intestinal organoids can be induced to differentiate into diverse cell types that compose the native intestinal by treating them with receptor activator of NF-κB ligand (RANKL, a common inducer of microfold-like structures) (Chen et al., 2023). During the exposure of M/NPs, microfold cells function as sensors, capturers, and transporters of larger particles. Epithelial cells internalize M/NPs in a size-, concentration-, and time-dependent manner. Exposure to high concentrations of M/NPs significantly triggers the secretion of various inflammatory cytokines associated with human inflammatory bowel disease. Intestinal stem cells play a crucial role in intestinal repair (Zhao et al., 2020; Takahashi et al., 2021). Therefore, it is highly significant to utilize intestinal organoids for studying the impact of M/NPs on intestinal stem cells and their important regulatory role in the occurrence and development of enteritis. In intestinal organoids, M/NPs can excessively activate the Notch signaling pathway, leading to increased expression levels of Lgr5, Bmi1, and Olfm4. This results in an increase in both the number and depth of crypts while causing an imbalance between proliferation and differentiation within colonic mucosal epithelium. Consequently, there is an imbalance in intestinal homeostasis along with a decrease in goblet cell and reduced expression levels of Muc2. Ultimately, this accelerates the onset and severity of colitis, demonstrating that by regulating the signaling pathway of intestinal stem cells (Xie et al., 2023), M/NPs can promote chronic intestinal diseases (Yan et al., 2022; Xie et al., 2023). Furthermore, apart from contributing to chronic enteritis development directly, M/NPs also exacerbate their impact on human health by absorbing toxic substances present in environmental pollutants, such as benzo [a] pyrene [B (a)P] (Gonzalez-Soto et al., 2019), fluoranthene (Magara et al., 2019), and anthracene (Kleinteich et al., 2018). B [a]P is a ubiquitous human carcinogen found in polluted air, water soil, and food, and can also adhere to polystyrene microplastics (Bukowska et al., 2022; Shaoyong et al., 2023). Compared to the treatment of mouse intestines and intestinal organoids with M/NPs alone, exposure to B [a]P-loaded polystyrene microplastics exhibited augmented cytotoxicity, including enhanced degradation of the colonic barrier, weight loss, shortened colon length, increased oxidative stress, autophagy induction, inflammation promotion, and bacterial translocation. Excessive activation of the Notch signaling pathway under conditions of increased oxidative stress further contributed to tight junction damage and mucosal barrier impairment. These findings elucidate potential molecular mechanisms by which B [a]P enhances microplastic-related intestinal toxicity. Studying the mechanism of intestinal organoids’ response to pollution is beneficial for researching effective drugs for treating intestinal diseases. Hou et al. (2022) proposed that combined exposure of intestinal organoids to polystyrene nanoparticles along with a clathrin-mediated endocytosis inhibitor (chlorpromazine), effectively inhibited nanoparticle accumulation in secretory cells. This suggests that inhibiting endocytosis can reduce the toxicity of polystyrene nanoparticles on the intestine.
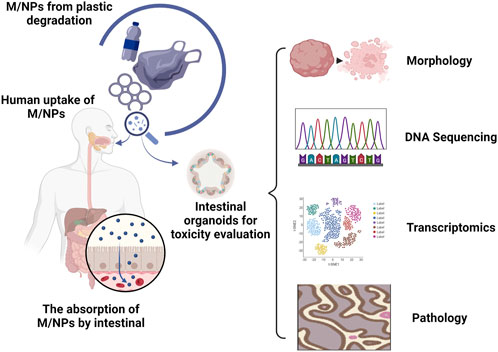
FIGURE 1. Organoids provide an in vitro model for studying the impact of M/NPs on the human intestine. The degradation of commonly used plastic products leads to the formation of small-sized M/NPs. The oral consumption of these particles by humans has the potential to cause harmful effects on the gastrointestinal tract. Human intestinal organoids can be utilized as substitutes to investigate the toxic effects of M/NPs on the human intestine. By utilizing morphological changes, genetic mutations, transcriptional analysis, pathological examination, identification of potential drug targets and pathways can be facilitated, thereby contributing to the advancement of novel disease biomarkers and treatment strategies.
5 Conclusion and perspectives
The issue of M/NPs are increasingly severe, yet the toxic effects on human health remain unclear. Intestinal organoids have emerged as a valuable tool for studying the toxicity of these pollutants, filling the gap left by traditional cell lines and animal models. However, further efforts are needed to establish intestinal organoids as models for environmental toxicology. The construction and culture methods of organoids vary among research groups, leading to inconsistencies in toxicity studies. Factors such as size, maturity, and cell composition can affect reproducibility and accuracy. To obtain more reliable results in organoid-based environmental toxicity detection, standardized culture and detection methods are required. Additionally, integrating liquid handling robots with automated high-throughput culture and analysis systems can optimize this technology for assessing environmental toxicity (Zhang et al., 2017; Schuster et al., 2020). The intestinal epithelium can be permeated by M/NPs, allowing them to enter the bloodstream and trigger an immune response (Liu et al., 2022). The intestinal organoids possess cell types and physiological structures that can partially replicate the in vivo intestinal epithelium (Almeqdadi et al., 2019); however, they are unable to fully emulate the complexity of the intestinal epithelium and its microenvironment due to the absence of immune cells and vascular tissues (Salewskij and Penninger, 2023). Recent advancements in co-culture, microfluidic, and 3D printing technologies have facilitated numerous studies on organoid-interstitial cell interactions, offering an improved platform for toxicity assessment (Cho and Yoon, 2017; Bein et al., 2018; Ronaldson-Bouchard et al., 2022). The mounting evidence suggests that M/NPs have the ability to penetrate the human body via the digestive tract and accumulate in various organs such as the liver, kidney, spleen, gastrointestinal tract, among others (Deng et al., 2017). Intestinal organoids alone are insufficient to capture systemic disease characteristics. The application of microfluidic technology has facilitated the development of human-on-chip systems (Lee et al., 2017; Oleaga et al., 2018; Ronaldson-Bouchard et al., 2022). Ronaldson-Bouchard et al. (2022) enabled the integration of multiple interconnected organoids on a single microfluidic chip. This innovative approach facilitates the assessment of drug-induced cardiotoxicity and holds great potential for evaluating systemic diseases. With ongoing advancements in organoid technology and its seamless integration with other cutting-edge techniques, organoids are poised to play increasingly pivotal role in environmental toxicity research.
Author contributions
QY: Writing–review and editing. YL: Writing–review and editing, Writing–original draft.
Funding
The author(s) declare financial support was received for the research, authorship, and/or publication of this article. This work was supported by the Postdoctoral Project of Qingdao (QDBSH20220202207).
Acknowledgments
Thanks to the related institutions for their help.
Conflict of interest
Author YL was employed by NanoPeptide (Qingdao) Biotechnology Ltd.
The remaining author declares that the research was conducted in the absence of any commercial or financial relationships that could be construed as a potential conflict of interest.
Publisher’s note
All claims expressed in this article are solely those of the authors and do not necessarily represent those of their affiliated organizations, or those of the publisher, the editors and the reviewers. Any product that may be evaluated in this article, or claim that may be made by its manufacturer, is not guaranteed or endorsed by the publisher.
References
Adeniyi-Ipadeola, G., Nwanosike, H., and Ramani, S. (2023). Human intestinal organoids as models to study enteric bacteria and viruses. Curr. Opin. Microbiol. 75, 102362. doi:10.1016/j.mib.2023.102362
Alexander, P. G., Clark, K. L., and Tuan, R. S. (2016). Prenatal exposure to environmental factors and congenital limb defects. Birth Defects Res. C Embryo Today 108, 243–273. doi:10.1002/bdrc.21140
Almeqdadi, M., Mana, M. D., Roper, J., and Yilmaz, O. H. (2019). Gut organoids: mini-tissues in culture to study intestinal physiology and disease. Am. J. Physiol. Cell Physiol. 317, C405–C419. doi:10.1152/ajpcell.00300.2017
Avio, C. G., Cardelli, L. R., Gorbi, S., Pellegrini, D., and Regoli, F. (2017). Microplastics pollution after the removal of the costa concordia wreck: first evidences from a biomonitoring case study. Environ. Pollut. 227, 207–214. doi:10.1016/j.envpol.2017.04.066
Banerjee, A., and Shelver, W. L. (2021). Micro- and nanoplastic induced cellular toxicity in mammals: a review. Sci. Total Environ. 755, 142518. doi:10.1016/j.scitotenv.2020.142518
Bein, A., Shin, W., Jalili-Firoozinezhad, S., Park, M. H., Sontheimer-Phelps, A., Tovaglieri, A., et al. (2018). Microfluidic organ-on-a-chip models of human intestine. Cell Mol. Gastroenterol. Hepatol. 5, 659–668. doi:10.1016/j.jcmgh.2017.12.010
Ben-David, U., Siranosian, B., Ha, G., Tang, H., Oren, Y., Hinohara, K., et al. (2018). Genetic and transcriptional evolution alters cancer cell line drug response. Nature 560, 325–330. doi:10.1038/s41586-018-0409-3
Bennekou, S. H. (2019). Moving towards a holistic approach for human health risk assessment - is the current approach fit for purpose? Efsa J. 17, e170711. doi:10.2903/j.efsa.2019.e170711
Bhagat, J., Zang, L., Nishimura, N., and Shimada, Y. (2020). Zebrafish: an emerging model to study microplastic and nanoplastic toxicity. Sci. Total Environ. 728, 138707. doi:10.1016/j.scitotenv.2020.138707
Bozzetti, V., and Senger, S. (2022). Organoid technologies for the study of intestinal microbiota-host interactions. Trends Mol. Med. 28, 290–303. doi:10.1016/j.molmed.2022.02.001
Bukowska, B., Mokra, K., and Michalowicz, J. (2022). Benzo[a]pyrene-environmental occurrence, human exposure, and mechanisms of toxicity. Int. J. Mol. Sci. 23, 6348. doi:10.3390/ijms23116348
Busch, M., Bredeck, G., Kampfer, A., and Schins, R. (2021a). Investigations of acute effects of polystyrene and polyvinyl chloride micro- and nanoplastics in an advanced in vitro triple culture model of the healthy and inflamed intestine. Environ. Res. 193, 110536. doi:10.1016/j.envres.2020.110536
Busch, M., Kampfer, A., and Schins, R. (2021b). An inverted in vitro triple culture model of the healthy and inflamed intestine: adverse effects of polyethylene particles. Chemosphere 284, 131345. doi:10.1016/j.chemosphere.2021.131345
Campanale, C., Massarelli, C., Savino, I., Locaputo, V., and Uricchio, V. F. (2020). A detailed review study on potential effects of microplastics and additives of concern on human health. Int. J. Environ. Res. Public Health 17, 1212. doi:10.3390/ijerph17041212
Chen, Y., Lin, Y., Davis, K. M., Wang, Q., Rnjak-Kovacina, J., Li, C., et al. (2015). Robust bioengineered 3d functional human intestinal epithelium. Sci. Rep. 5, 13708. doi:10.1038/srep13708
Chen, Y., Williams, A. M., Gordon, E. B., Rudolph, S. E., Longo, B. N., Li, G., et al. (2023). Biological effects of polystyrene micro- and nano-plastics on human intestinal organoid-derived epithelial tissue models without and with m cells. Nanomedicine-Uk 50, 102680. doi:10.1016/j.nano.2023.102680
Cheng, W., Li, X., Zhou, Y., Yu, H., Xie, Y., Guo, H., et al. (2022). Polystyrene microplastics induce hepatotoxicity and disrupt lipid metabolism in the liver organoids. Sci. Total Environ. 806, 150328. doi:10.1016/j.scitotenv.2021.150328
Cho, S., and Yoon, J. Y. (2017). Organ-on-a-chip for assessing environmental toxicants. Curr. Opin. Biotechnol. 45, 34–42. doi:10.1016/j.copbio.2016.11.019
Clevers, H. (2016). Modeling development and disease with organoids. Cell 165, 1586–1597. doi:10.1016/j.cell.2016.05.082
Da, S. B. W., Mutter, F., Wende, K., Cecchini, A. L., Schmidt, A., and Bekeschus, S. (2022). Consequences of nano and microplastic exposure in rodent models: the known and unknown. Part Fibre Toxicol. 19, 28. doi:10.1186/s12989-022-00473-y
Deng, Y., Zhang, Y., Lemos, B., and Ren, H. (2017). Tissue accumulation of microplastics in mice and biomarker responses suggest widespread health risks of exposure. Sci. Rep. 7, 46687. doi:10.1038/srep46687
Devall, M., Plummer, S. J., Bryant, J., Jennelle, L. T., Eaton, S., Dampier, C. H., et al. (2021). Ethanol exposure drives colon location specific cell composition changes in a normal colon crypt 3d organoid model. Sci. Rep. 11, 432. doi:10.1038/s41598-020-80240-1
Dothel, G., Vasina, V., Barbara, G., and De Ponti, F. (2013). Animal models of chemically induced intestinal inflammation: predictivity and ethical issues. Pharmacol. Ther. 139, 71–86. doi:10.1016/j.pharmthera.2013.04.005
Du, Y., Li, X., Niu, Q., Mo, X., Qui, M., Ma, T., et al. (2020). Development of a miniaturized 3d organoid culture platform for ultra-high-throughput screening. J. Mol. Cell Biol. 12, 630–643. doi:10.1093/jmcb/mjaa036
Dutta, D., Heo, I., and Clevers, H. (2017). Disease modeling in stem cell-derived 3d organoid systems. Trends Mol. Med. 23, 393–410. doi:10.1016/j.molmed.2017.02.007
Fackelmann, G., and Sommer, S. (2019). Microplastics and the gut microbiome: how chronically exposed species may suffer from gut dysbiosis. Mar. Pollut. Bull. 143, 193–203. doi:10.1016/j.marpolbul.2019.04.030
Faiola, F., Yin, N., Yao, X., and Jiang, G. (2015). The rise of stem cell toxicology. Environ. Sci. Technol. 49, 5847–5848. doi:10.1021/acs.est.5b01549
Fernandes, T. G. (2023). Organoids as complex (bio)systems. Front. Cell Dev. Biol. 11, 1268540. doi:10.3389/fcell.2023.1268540
Forsythe, S. D., Devarasetty, M., Shupe, T., Bishop, C., Atala, A., Soker, S., et al. (2018). Environmental toxin screening using human-derived 3d bioengineered liver and cardiac organoids. Front. Public Health 6, 103. doi:10.3389/fpubh.2018.00103
Fournier, E., Etienne-Mesmin, L., Grootaert, C., Jelsbak, L., Syberg, K., Blanquet-Diot, S., et al. (2021). Microplastics in the human digestive environment: a focus on the potential and challenges facing in vitro gut model development. J. Hazard Mater 415, 125632. doi:10.1016/j.jhazmat.2021.125632
Gautam, R., Jo, J., Acharya, M., Maharjan, A., Lee, D., Kim, C., et al. (2022). Evaluation of potential toxicity of polyethylene microplastics on human derived cell lines. Sci. Total Environ. 838, 156089. doi:10.1016/j.scitotenv.2022.156089
Geyer, R., Jambeck, J. R., and Law, K. L. (2017). Production, use, and fate of all plastics ever made. Sci. Adv. 3, e1700782. doi:10.1126/sciadv.1700782
Gonzalez-Soto, N., Hatfield, J., Katsumiti, A., Duroudier, N., Lacave, J. M., Bilbao, E., et al. (2019). Impacts of dietary exposure to different sized polystyrene microplastics alone and with sorbed benzo[a]pyrene on biomarkers and whole organism responses in mussels mytilus galloprovincialis. Sci. Total Environ. 684, 548–566. doi:10.1016/j.scitotenv.2019.05.161
Hou, Z., Meng, R., Chen, G., Lai, T., Qing, R., Hao, S., et al. (2022). Distinct accumulation of nanoplastics in human intestinal organoids. Sci. Total Environ. 838, 155811. doi:10.1016/j.scitotenv.2022.155811
Huang, Y., Liu, X., Feng, Y., Nie, X., Liu, Q., Du, X., et al. (2022). Rotenone, an environmental toxin, causes abnormal methylation of the mouse brain organoid's genome and ferroptosis. Int. J. Med. Sci. 19, 1184–1197. doi:10.7150/ijms.74569
Kaur, K., Reddy, S., Barathe, P., Oak, U., Shriram, V., Kharat, S. S., et al. (2022). Microplastic-associated pathogens and antimicrobial resistance in environment. Chemosphere 291, 133005. doi:10.1016/j.chemosphere.2021.133005
Kiran, B. R., Kopperi, H., and Venkata, M. S. (2022). Micro/nano-plastics occurrence, identification, risk analysis and mitigation: challenges and perspectives. Rev. Environ. Sci. Biotechnol. 21, 169–203. doi:10.1007/s11157-021-09609-6
Kleinteich, J., Seidensticker, S., Marggrander, N., and Zarfl, C. (2018). Microplastics reduce short-term effects of environmental contaminants. Part ii: polyethylene particles decrease the effect of polycyclic aromatic hydrocarbons on microorganisms. Int. J. Environ. Res. Public Health 15, 287. doi:10.3390/ijerph15020287
Kumar, R., Manna, C., Padha, S., Verma, A., Sharma, P., Dhar, A., et al. (2022). Micro(nano)plastics pollution and human health: how plastics can induce carcinogenesis to humans? Chemosphere 298, 134267. doi:10.1016/j.chemosphere.2022.134267
Lee, H., Kim, D. S., Ha, S. K., Choi, I., Lee, J. M., and Sung, J. H. (2017). A pumpless multi-organ-on-a-chip (moc) combined with a pharmacokinetic-pharmacodynamic (pk-pd) model. Biotechnol. Bioeng. 114, 432–443. doi:10.1002/bit.26087
Liang, B., Zhong, Y., Huang, Y., Lin, X., Liu, J., Lin, L., et al. (2021). Underestimated health risks: polystyrene micro- and nanoplastics jointly induce intestinal barrier dysfunction by ros-mediated epithelial cell apoptosis. Part Fibre Toxicol. 18, 20. doi:10.1186/s12989-021-00414-1
Liu, S., Li, H., Wang, J., Wu, B., and Guo, X. (2022). Polystyrene microplastics aggravate inflammatory damage in mice with intestinal immune imbalance. Sci. Total Environ. 833, 155198. doi:10.1016/j.scitotenv.2022.155198
Liu, Y., Mi, Y., Mueller, T., Kreibich, S., Williams, E. G., Van Drogen, A., et al. (2019). Multi-omic measurements of heterogeneity in hela cells across laboratories. Nat. Biotechnol. 37, 314–322. doi:10.1038/s41587-019-0037-y
Magara, G., Khan, F. R., Pinti, M., Syberg, K., Inzirillo, A., and Elia, A. C. (2019). Effects of combined exposures of fluoranthene and polyethylene or polyhydroxybutyrate microplastics on oxidative stress biomarkers in the blue mussel (mytilus edulis). J. Toxicol. Environ. Health A 82, 616–625. doi:10.1080/15287394.2019.1633451
Mariano, S., Tacconi, S., Fidaleo, M., Rossi, M., and Dini, L. (2021). Micro and nanoplastics identification: classic methods and innovative detection techniques. Front. Toxicol. 3, 636640. doi:10.3389/ftox.2021.636640
Martinez-Silgado, A., Yousef, Y. F., Puschhof, J., Geurts, V., Boot, C., Geurts, M. H., et al. (2022). Differentiation and crispr-cas9-mediated genetic engineering of human intestinal organoids. Star. Protoc. 3, 101639. doi:10.1016/j.xpro.2022.101639
Nikolaev, M., Mitrofanova, O., Broguiere, N., Geraldo, S., Dutta, D., Tabata, Y., et al. (2020). Homeostatic mini-intestines through scaffold-guided organoid morphogenesis. Nature 585, 574–578. doi:10.1038/s41586-020-2724-8
Oleaga, C., Riu, A., Rothemund, S., Lavado, A., McAleer, C. W., Long, C. J., et al. (2018). Investigation of the effect of hepatic metabolism on off-target cardiotoxicity in a multi-organ human-on-a-chip system. Biomaterials 182, 176–190. doi:10.1016/j.biomaterials.2018.07.062
Pires, A., Cuccaro, A., Sole, M., and Freitas, R. (2022). Micro(nano)plastics and plastic additives effects in marine annelids: a literature review. Environ. Res. 214, 113642. doi:10.1016/j.envres.2022.113642
Pleguezuelos-Manzano, C., Puschhof, J., van den Brink, S., Geurts, V., Beumer, J., and Clevers, H. (2020). Establishment and culture of human intestinal organoids derived from adult stem cells. Curr. Protoc. Immunol. 130, e106. doi:10.1002/cpim.106
Prata, J. C., Da, C. J., Lopes, I., Duarte, A. C., and Rocha-Santos, T. (2020). Environmental exposure to microplastics: an overview on possible human health effects. Sci. Total Environ. 702, 134455. doi:10.1016/j.scitotenv.2019.134455
Puschhof, J., Pleguezuelos-Manzano, C., Martinez-Silgado, A., Akkerman, N., Saftien, A., Boot, C., et al. (2021). Intestinal organoid cocultures with microbes. Nat. Protoc. 16, 4633–4649. doi:10.1038/s41596-021-00589-z
Ronaldson-Bouchard, K., Teles, D., Yeager, K., Tavakol, D. N., Zhao, Y., Chramiec, A., et al. (2022). A multi-organ chip with matured tissue niches linked by vascular flow. Nat. Biomed. Eng. 6, 351–371. doi:10.1038/s41551-022-00882-6
Salewskij, K., and Penninger, J. M. (2023). Blood vessel organoids for development and disease. Circ. Res. 132, 498–510. doi:10.1161/CIRCRESAHA.122.321768
Sato, T., Vries, R. G., Snippert, H. J., van de Wetering, M., Barker, N., Stange, D. E., et al. (2009). Single lgr5 stem cells build crypt-villus structures in vitro without a mesenchymal niche. Nature 459, 262–265. doi:10.1038/nature07935
Schuster, B., Junkin, M., Kashaf, S. S., Romero-Calvo, I., Kirby, K., Matthews, J., et al. (2020). Automated microfluidic platform for dynamic and combinatorial drug screening of tumor organoids. Nat. Commun. 11, 5271. doi:10.1038/s41467-020-19058-4
Schwabl, P., Koppel, S., Konigshofer, P., Bucsics, T., Trauner, M., Reiberger, T., et al. (2019). Detection of various microplastics in human stool: a prospective case series. Ann. Intern Med. 171, 453–457. doi:10.7326/M19-0618
Shaoyong, W., Jin, H., Jiang, X., Xu, B., Liu, Y., Wang, Y., et al. (2023). Benzo [a] pyrene-loaded aged polystyrene microplastics promote colonic barrier injury via oxidative stress-mediated notch signalling. J. Hazard Mater 457, 131820. doi:10.1016/j.jhazmat.2023.131820
Shen, M., Zhang, Y., Zhu, Y., Song, B., Zeng, G., Hu, D., et al. (2019). Recent advances in toxicological research of nanoplastics in the environment: a review. Environ. Pollut. 252, 511–521. doi:10.1016/j.envpol.2019.05.102
Sinagoga, K. L., and Wells, J. M. (2015). Generating human intestinal tissues from pluripotent stem cells to study development and disease. Embo J. 34, 1149–1163. doi:10.15252/embj.201490686
Spence, J. R., Mayhew, C. N., Rankin, S. A., Kuhar, M. F., Vallance, J. E., Tolle, K., et al. (2011). Directed differentiation of human pluripotent stem cells into intestinal tissue in vitro. Nature 470, 105–109. doi:10.1038/nature09691
Stock, V., Bohmert, L., Lisicki, E., Block, R., Cara-Carmona, J., Pack, L. K., et al. (2019). Uptake and effects of orally ingested polystyrene microplastic particles in vitro and in vivo. Arch. Toxicol. 93, 1817–1833. doi:10.1007/s00204-019-02478-7
Takahashi, T., Fujishima, K., and Kengaku, M. (2021). Modeling intestinal stem cell function with organoids. Int. J. Mol. Sci. 22, 10912. doi:10.3390/ijms222010912
Thodhal, Y. S., Hamid, N., Muhammad, J., Duan, J. J., and Pei, D. S. (2023). Micro(nano)plastics in commercial foods: a review of their characterization and potential hazards to human health. Environ. Res. 236, 116858. doi:10.1016/j.envres.2023.116858
Tian, C. M., Yang, M. F., Xu, H. M., Zhu, M. Z., Yue, N. N., Zhang, Y., et al. (2023). Stem cell-derived intestinal organoids: a novel modality for ibd. Cell Death Discov. 9, 255. doi:10.1038/s41420-023-01556-1
Visalli, G., Facciola, A., Pruiti, C. M., De Marco, G., Maisano, M., and Di Pietro, A. (2021). Acute and sub-chronic effects of microplastics (3 and 10 µm) on the human intestinal cells HT-29. Int. J. Environ. Res. Public Health 18, 5833. doi:10.3390/ijerph18115833
Wang, J., Lv, S., Zhang, M., Chen, G., Zhu, T., Zhang, S., et al. (2016). Effects of plastic film residues on occurrence of phthalates and microbial activity in soils. Chemosphere 151, 171–177. doi:10.1016/j.chemosphere.2016.02.076
Wang, W., Yuan, W., Xu, E. G., Li, L., Zhang, H., and Yang, Y. (2022). Uptake, translocation, and biological impacts of micro(nano)plastics in terrestrial plants: progress and prospects. Environ. Res. 203, 111867. doi:10.1016/j.envres.2021.111867
Waring, R. H., Harris, R. M., and Mitchell, S. C. (2018). Plastic contamination of the food chain: a threat to human health? Maturitas 115, 64–68. doi:10.1016/j.maturitas.2018.06.010
Workman, M. J., Mahe, M. M., Trisno, S., Poling, H. M., Watson, C. L., Sundaram, N., et al. (2017). Engineered human pluripotent-stem-cell-derived intestinal tissues with a functional enteric nervous system. Nat. Med. 23, 49–59. doi:10.1038/nm.4233
Xie, S., Zhang, R., Li, Z., Liu, C., Chen, Y., and Yu, Q. (2023). Microplastics perturb colonic epithelial homeostasis associated with intestinal overproliferation, exacerbating the severity of colitis. Environ. Res. 217, 114861. doi:10.1016/j.envres.2022.114861
Xu, S., Ma, J., Ji, R., Pan, K., and Miao, A. J. (2020). Microplastics in aquatic environments: occurrence, accumulation, and biological effects. Sci. Total Environ. 703, 134699. doi:10.1016/j.scitotenv.2019.134699
Yan, Z., Liu, Y., Zhang, T., Zhang, F., Ren, H., and Zhang, Y. (2022). Analysis of microplastics in human feces reveals a correlation between fecal microplastics and inflammatory bowel disease status. Environ. Sci. Technol. 56, 414–421. doi:10.1021/acs.est.1c03924
Yang, S., Hu, H., Kung, H., Zou, R., Dai, Y., Hu, Y., et al. (2023). Organoids: the current status and biomedical applications. Medcomm 4, e274. doi:10.1002/mco2.274
Yin, X., Mead, B. E., Safaee, H., Langer, R., Karp, J. M., and Levy, O. (2016). Engineering stem cell organoids. Cell Stem Cell 18, 25–38. doi:10.1016/j.stem.2015.12.005
Yu, L., Tian, X., Gao, D., Lang, Y., Zhang, X. X., Yang, C., et al. (2019). Oral administration of hydroxylated-graphene quantum dots induces intestinal injury accompanying the loss of intestinal stem cells and proliferative progenitor cells. Nanotoxicology 13, 1409–1421. doi:10.1080/17435390.2019.1668068
Zhang, Y. S., Aleman, J., Shin, S. R., Kilic, T., Kim, D., Mousavi, S. S., et al. (2017). Multisensor-integrated organs-on-chips platform for automated and continual in situ monitoring of organoid behaviors. Proc. Natl. Acad. Sci. U. S. A. 114, E2293–E2302. doi:10.1073/pnas.1612906114
Zhao, Q., Guan, J., and Wang, X. (2020). Intestinal stem cells and intestinal organoids. J. Genet. Genomics 47, 289–299. doi:10.1016/j.jgg.2020.06.005
Keywords: environmental pollution, micro/nano plastics, in vitro toxicity, stem cells, intestinal organoids, intestinal diseases
Citation: Yuan Q and Liu Y (2023) Utilization of intestinal organoid models for assessment of micro/nano plastic-induced toxicity. Front. Environ. Sci. 11:1285536. doi: 10.3389/fenvs.2023.1285536
Received: 30 August 2023; Accepted: 17 October 2023;
Published: 26 October 2023.
Edited by:
Nsikak U. Benson, Université Claude Bernard Lyon 1, FranceReviewed by:
Mohamed Ahmed Ibrahim Ahmed, Assiut University, EgyptCopyright © 2023 Yuan and Liu. This is an open-access article distributed under the terms of the Creative Commons Attribution License (CC BY). The use, distribution or reproduction in other forums is permitted, provided the original author(s) and the copyright owner(s) are credited and that the original publication in this journal is cited, in accordance with accepted academic practice. No use, distribution or reproduction is permitted which does not comply with these terms.
*Correspondence: Yajing Liu, yajingliu1128@163.com