- 1Department of Life Sciences, Bhakta Kavi Narsinh Mehta University, Junagadh, Gujarat, India
- 2Department of Zoology, College of Science, King Saud University, Riyadh, Saudi Arabia
- 3Department of Life Sciences, Hemchandracharya North Gujarat University, Patan, Gujarat, India
- 4Department of Veterinary Clinical Sciences, College of Veterinary Medicine, Iowa State University, Ames, IA, United States
This scientific manuscript presents a comprehensive investigation into the purification of municipal sewage water through the utilization of agricultural waste materials [Arachis hypogaea shells (AHS), Triticum aestivum straw (TAS), and Gossypium herbaceum shells (GHS)]. The treatment process involved a modified approach with 24 hs of aeration and the addition of 1 gm of agricultural waste biomaterials. The performance of the bio-coagulant was evaluated by monitoring the reduction of physico−chemical parameters. AHS exhibited remarkable turbidity removal efficiency of 93.37%, supported by pseudo−first and pseudo−second−order kinetic modelling. The application of agricultural waste materials significantly reduced key parameters, including solids (up to 70%–80%), dissolved oxygen (DO) (50%), biological oxygen demand (BOD) and chemical oxygen demand (COD) (up to 90%). Principal Component Analysis (PCA) showed the significant positive loading of PC1 (84.71%) that influencing the dual treatments of wastewater. Statistical analysis (p ≤ 0.05) confirmed the effectiveness of agricultural biomaterials in sewage water treatment compared to pre−treated water. The turbidity coagulation pseudo−first−order and pseudo−second−order kinetic modelling also revealed the efficiency against turbidity reduction in municipal sewage water. The findings underscore the significance of utilizing agricultural waste materials for sustainable and efficient purification of municipal sewage water, addressing water pollution and enhancing wastewater treatment processes.
1 Introduction
Municipal or sewage water pollution is a global issue that poses a significant threat to human health, aquatic life and the environment (Madhav et al., 2020; Ahmed et al., 2022). The discharge of waste and pollutants into water sources is a common practice in many parts of the world, particularly in developing nations where resources are limited, infrastructure is inadequate and there is a lack of awareness about the impact of such actions (Bijekar et al., 2022). As a result, water sources are becoming increasingly contaminated with a range of harmful substances, including chemicals, dyes (Agarwal et al., 2022), pesticides (Ajiboye et al., 2022), heavy metals (Ishwari et al., 2022; Uko et al., 2022; Yadav et al., 2023b), micro plastics (Rabari et al., 2023) and biological contaminants such as pathogens (Patel et al., 2022). Municipal wastewater is discharged from commercial, institutional and similar facilities (Nazari et al., 2021). Despite being a crucial resource, water bodies across the world are under threat due to the discharge of untreated sewage water (Zhao et al., 2021). In India, for example, according to recent report of CPCB (Central Pollution Control Board) that suggest only 13.5% of the wastewater is treated in the country and remaining 80%–85% wastewater is directly released into surface water. This trend is not unique to India but is a global problem that requires urgent attention (CPCB, 2021).
The practice of discharging untreated sewage water into water bodies is widespread in many countries and it is the most common cause of surface water and underground water pollution there by harming living organisms and directly impacting the food chain and the entire ecosystem (Bijekar et al., 2022; Jan et al., 2022). This can result in the loss of biodiversity and disrupt entire ecosystems. Furthermore, the accumulation of pollutants in the sediment and soil can lead to long−term contamination, which can persist for decades and affect the health of the surrounding community (Ahmad et al., 2021; Cui et al., 2021). In arid and semiarid countries where water demand is high and annual rainfall is low, reusing sewage water is an effective and sustainable approach to address water scarcity (Priyan, 2021; Yadav et al., 2023a). With the world population expected to increase by 2 billion in the next 30 years, from 8 billion currently, the demand for water will rise even higher, making sewage water treatment and reuse an essential part of water management strategies (United Nations, 2021United Nations, 2021).
Despite the importance of sewage treatment, many countries still struggle to safely treat household wastewater. According to the United Nations (2021), out of the total 270,674.505 million m3of household wastes generated worldwide, only 150,232.379 million m3 were safely treated. In India, average sewage water production is 72,368MLD (million liters per day). Sewage treatment plants (STPs) in India are able to treat a little more than a third of the sewage generated per day, according to the latest report of the Central Pollution Control Board, India. To address the problem of wastewater disposal, it is essential to implement effective sewage treatment systems. Treatment can remove or reduce the concentration of contaminants and pollutants present in sewage, making it safe for discharge into the environment or for reuse in non−potable applications such as irrigation.
There are different types of sewage treatment processes, including physical, chemical and biological processes, which can be used individually or in combination. Historically, wastewater management presented formidable hurdles; nevertheless, modern approaches have transformed, encompassing recycling that converges wastewater treatment and a source of renewable energy (Chahar et al., 2023). However, recently certain chemical or physical wastewater treatment methods have had some drawbacks and negative impacts, such as the requirements of reagents and high expenses, the need for energy–intensive treatment processes and the generation of hazardous secondary sludge. Therefore, wastewater reuse needs to be treated with a suitable wastewater treatment process. The invention of cost-effective technologies, particularly in wastewater treatment, has attracted tremendous interest in sustainable water resource management. Hence, the efficiency of natural biomaterials in the treatment of wastewater and water has been studied.
Coagulants have long been used in the treatment of wastewater, but natural−based biomaterials have gained attention in recent years (Bahrodin et al., 2021). Biomaterials are coagulants that are derived from natural polymers found in plants, animals, and other natural sources. These materials have several advantages, including being non−toxic, sustainable, naturally biodegradable and easily accessible (Joyce et al., 2021). Over 50 different types of natural coagulants have been investigated for use in water purification. Among them, biomaterials such as Prosopis juliflora, Azadirachta indica, Moringa oleifera (Jabar et al., 2020; Rai et al., 2022), Cocos nucifera (Luis-Zarate et al., 2018; Grass Ramírez et al., 2023), Saccharum officinarum (Keeping, 2017; Kerrou et al., 2021) and others have been studied for their potential in wastewater treatment.
There has been an increase interest in agricultural waste materials for a variety of purposes in recent years due to easily available, cheaper and eco-friendly in nature. The agricultural waste as wheat waste, groundnut shell, and cotton waste materials, for instance, have been found to exhibit highly rough, porous surface areas, attributed to their effective cellulosic network that includes lignin, proteins and other functional groups like phenolic hydroxyl groups, carboxyl and amino groups. These characteristics provide structural and porous surface areas with activated functional groups that make them suitable for water purification applications (Chen et al., 2020; Khan et al., 2020; Sathiparan et al., 2023).
Based on these characteristics, various research studies focused on the uses of agricultural biomaterials as source of the various applications. Moreover, some of the studies reported that application of biomaterials in water purification but treatment biomaterials was not vigorous and a limited number of observations. Therefore, the extensive literature report stated that no specific study was reported on the efficiency of agricultural waste materials as coagulants for the treatment of sewage water with broad range of physico−chemical parameters; hence, in this present investigation, a unique and innovative approach has been developed, integrating both primary treatment as aeration (24 h) and secondary treatment as bio-coagulants. This dual treatment approach distinguishes the research from previous studies. Furthermore, investigation includes a broader range of physicochemical parameters for determined the removal efficiency biomaterials in wastewater.
Therefore, this research work aimed to evaluate the characterization and removal efficiency of biomaterials such as agricultural waste materials (wheat waste, groundnut shell, and cotton waste) for sewage water treatment. The performance of coagulating agents was evaluated by measuring the physicochemical parameters of water, including pH, electrical conductivity (EC), turbidity, hardness (calcium and magnesium), dissolved oxygen (DO), biological oxygen demand (BOD), chemical oxygen demand (COD) and total solids (dissolved and suspended). The relationship between the physicochemical parameters were examined using different statistical analyses (hierarchical analysis, Principal component analysis and paired comparisons test). In addition, pseudo−first−order and pseudo−second−order kinetic models were employed to determine the removal efficiency of biomaterials.
2 Materials and methods
2.1 Collection of samples
Municipal sewage water samples were collected from 15 zones around the Junagadh region (Latitude: 21°31′29.78″N, Longitude: 70°27′28.60″E), Gujarat. The water samples were collected in an airtight plastic container. In accordance with APHA’s (2017) Standard Method, the wastewater samples were preserved, maintained, and transported to the laboratory.
The bio-coagulants (Arachis hypogaea shells, Triticum aestivum straw and Gossypium herbaceum shells) were collected from Zinzuda, located in Junagadh, Gujarat. Then, it was air-dried at room temperature before being sun−dried for 1–2 days. The dried Arachis hypogaea shells (AHS), Triticum aestivum straw (TAS) and Gossypium herbaceum shells (GHS) were grounded and sieved through a standard steel sieve to obtain a particle size of <100 mm. As biocoagulants, the AHS, GHS and TAS were not treated with any physical or chemical treatment. In order to facilitate further analysis, bio-coagulants are stored in clean, airtight polythene bags (Chen et al., 2020). There are various dosages of biocoagulants (viz., 0.1, 0.3, 0.5, 0.7, 0.9, 1, 1.3, 1.5, 1.7, and 1.9 gm) that have been used to treat sewage wastewater. Amongst them, 1.0 gm of biocoagulant concentration was found to be suitable for sewage wastewater (data not shown).
2.2 Experimental procedures
This study evaluated the effectiveness of agricultural waste as a coagulant in treating sewage water. The experiment involved untreated biomaterials and subjected the sewage water to primary and secondary treatments to enhance the treatment process. Aeration served as the primary treatment for 24 h. The secondary treatment involved using bio-coagulants (AHS, TAS and GHS) to treat the sewage water. Each beaker containing 500 mL of aerated sewage water and 1.0 gm of each agricultural bio-coagulant. The biocoagulant was thoroughly mixed with the sample solutions at varying agitation speeds 150 rpm for 10 min, followed by slowly at 50 rpm for 30 min and finally at 10 rpm for 20 min. The biocoagulants process increase gradually and forming large flocs that settled at room temperature. The stable particles were collected without disruption for subsequent analysis. The water suspension of these treatments was kept in dark conditions at room temperature for 20 days, and water suspensions were regularly analyzed at interval of 5 days. Physicochemical analysis was conducted on the treated sewage water to assess the coagulation-flocculation effect (Choudhary and Neogi, 2017; Ishak et al., 2021).
2.3 Wastewater analysis
The efficiency of bio-based coagulating materials (De et al., 2020; Yadav et al., 2022) has been assessed by monitoring the physicochemical parameters of sewage water, including pH, electrical conductivity (EC), turbidity, suspended solids (TSS), dissolved solids (TDS), dissolved oxygen (DO), biological oxygen demand (BOD), chemical oxygen demand (COD) and ion concentrations (Ca2+, Mg2+ and Cl−) of the suspension. After collecting the sample, we analyzed the pH of suspension using a digital 700 Eutech (India) pH meter and measured the conductivity using a 306 Systronics (India) conductivity meter. Solids, including TSS, TDS and total solids (TS) were evaluated by the gravimetric method. Moreover, DO and BOD measured the amount of oxygen required to remove waste organic matter from water in the decomposition process by Winkler’s method. The closed reflux method was used to determine the COD of the water (APHA, 2017).
The estimation of hardness (including calcium and magnesium) and ions such as calcium (Ca2+) and magnesium (Mg2+) were carried out by the EDTA titrimetric method. The argentometric method is used for estimating the amount of chloride in sewage water. The sewage water parameters, including TS, TSS, TDS, DO, BOD, COD, Ca2+, Mg2+, Cl− and total hardness (calcium and magnesium), were calculated as per (APHA, 2017).
2.4 Coagulation-flocculation kinetic modelling
It is well known that the fundamentals of the rate of aggregation started from the classic work of Smoluchowski (1917) (Ishak et al., 2021; Liu et al., 2022). After a period of the aggregation of particles, size distribution and shape of these particles can be changed fundamentally. To describe the aggregation rate of particle count based on the Brownian-controlled and rapid coagulation, the general differential equation can be shown below:
The final turbidity level in mg L−1 is represented by C, coagulation time in minutes is represented by t, and the nth order rate constant is represented by k. The kinetic order of the coagulation process is denoted by ‘α’ and its theoretical values lie within the range of 1 ≤ α ≤ 2 as described by Varsani et al. (2022), Naruka et al. (2021). The negative sign indicates that the turbidity level decrease with an increase in time.
If coagulation follows the first order kinetics where α = 1, the derive rate Eq. 2 is;
Considering the aforementioned information, by substituting the value of (α = 2) into Eq. 1 and performing integration while adhering to the specified boundary conditions, namely, the initial condition (t = 0, C = C0) and the final condition (t = t, C = C), we can derive Eq. 3.
The relationship between friction factor (β, m3 kg−1·s) and nth order coagulation rate constants calculated from Eq. 2 and Eq. 3, we can derive Eq. 4.
Brownian diffusion coefficient (D, kg2m−1·s) is determined by the Boltzmann constant (kB), temperature (K) and friction factor (Okey-Onyesolu et al., 2020). The diffusion coefficient is correlated by through Eq. 5.
Where kB = 1.3806452 × 10−23
The half-life period for the pseudo − first order kinetic reaction can be determined using Eq. 6, which is expressed as:
The half-life period for the pseudo − second order kinetic is calculated using Eq. 7.
2.5 Statistical analysis
In the present research work, Origin Pro software (version 2023), Minitab® (version 19.2020.1) and Prism 9 (version 9.5.1) were utilized for the various statistical (hierarchical analysis, principal component analysis and paired comparisons test) and kinetic (Pseudo−first−order and Pseudo−second−order) analyses.
3 Results and discussion
3.1 Effect on physico−chemical parameters of sewage water after primary treatment
Initially, untreated municipal sewage water was used for the analysis. The parameters pH of untreated water was found to be 7.77 (alkaline). After aeration, the concentration of protons decreased and the pH of the sewage water became more basic, measuring 8.75 due to turbulence in the wastewater and the release of carbon dioxide into the air, leading to a rise in proton concentration during aeration. After 24 h of aeration, the following removal efficiencies were observed: 3.31% for conductivity (from 1.479 to 1.430 mS/cm), 63.82% for turbidity (from 84.03 to 30.4 NTU) and 15.96% for hardness (from 7.33 to 6.16 mg/L).
Upon release of the air, the solids content of the sewage water, including both suspended and soluble solids, decreased by 40%–50%. After primary treatment (aeration), the concentration of chloride decreased by 54.16% (from 199.9 to 91.63 mg/L) and both the BOD and COD declined significantly by 36% (from 56.11 to 35.91 mg/L) and 16.38% (from 693.75 to 580 mg/L), respectively. These reduction of physico−chemical parameters in sewage water through agricultural waste materials are due to various reasons, including the biological activities of microorganisms present in sewage water, settling and separation process in which suspended particles and flocs are formed and allowed to settle. As these particles settle out of the water, they may carry ions and conductivity with them, resulting in a decrease in EC as well as the ion exchange reactions of biomaterials decrease the solids materials (Koul et al., 2022).
After primary (aeration) treatment, three agricultural waste products as AHS, GHS and TAS were used for the secondary treatments of the municipal sewage water.
3.1.1 Removal efficiency of AHS in sewage water
After primary treatments waste sample were used for secondary treatment by addition of the AHS particles. Primary treatments resulted the significant reduction on the physico−chemical parameters of wastewater. The use of bio-coagulants as AHS has been shown to impact several parameters such as pH, turbidity, calcium hardness, and COD after 5 days of treatment. The removal efficiencies of these parameters were 10.69%, 76.88%, 15%, and 10.18%, respectively, due to the aggregation of suspended particles, which caused them to settle and thus reduced turbidity and COD. Additionally, ions adsorbed on the surface of bio-coagulant and reduced the hardness of sewage water (Mohd Nor et al., 2017; Bahrodin et al., 2022). Removal efficiencies for pH, conductivity, turbidity, Chloride concentrations, COD and solid contents increased after 10 days of sewage water treatment (Figure 1). After 10 days of treatment, the reduction efficiency was 3.54% for pH, 5.68% for conductivity, 77.19% for turbidity, 22.71% suspended solids, 6.35% and 8.88% for chloride concentration and COD in sewage. After 15 days of analysis, no reduction was observed in pH, EC, hardness and ionic concentrations. According to Varsani et al. (2022) all the water−soluble components of coagulants dissolved in water and increased ions concentration. Hence, reduction level is lower after 10th and 15th day. Only 5%–6% of solids and 3%–4% of DO and BOD levels decreased after 15 days of sewage water treatment compared to primary treatment. On the 20th day, reductions were observed in pH (4.82%), turbidity (81.68%), TSS (67.28%), TDS (49.59%), TS (46.53%), and COD (94.30%).
According to Figure 1, dried AHS was able to reduce turbidity by around 90% (84.03–5.56 NTU), hardness by 13.69% (7.3–6.3 mg/L), solid content by 70%–80% (660–150 mg/L) and COD by 80%–85% (693.75–99.13 mg/L) in sewage water after 20 days of treatment compared to untreated sewage water. However, there was no observed reduction in BOD during the municipal sewage water treatment.
The use of AHS particles in the coagulation-flocculation process has been shown to reduce turbidity by 75.4%–85.0% and COD by 41.7%–53.2% in surface water (Wasify et al., 2023). According to Kingue et al. (2023) reported that the AHS extract has a capacity to removed more than 80% of the turbidity using a 500 mg/L of extract. Walanda and Napitupulu (2023) conducted research on the reduction efficiency of AHS in hospital wastewater, which resulted in a decrease of BOD from 72 to 24 g/L and COD from 46 to 14 g/L. Hence, the application of a dual treatment approach (primary and secondary) in sewage water treatment exhibited significant improvements, compared to previous studies.
The dried powder of M. oleifera seeds mixed with alum reduced BOD by 8.67% and COD by 50.40% in wastewater (Koul et al., 2022). Hence, this present research study demonstrated that AHS as a coagulant achieved even higher removal efficiency. This superior performance can be attributed to the extended duration of treatment, as results were obtained after a 20-day period, in contrast to the shorter−term findings reported by Koul et al. (2022). Additionally, the presence of a high amount of cellulose in AHS, as indicated by Varalalakshmi et al. (2021), may contribute to the enhanced coagulation effectiveness observed in our study.
When compared with other bio-coagulant such as M. oleifera seeds as coagulant, the dried powder of M. oleifera seeds mixed with alum reduced BOD by 8.67% and COD by 50.40% in wastewater (Koul et al., 2022). Hence, the % removal efficiency of COD and BOD were found to be maximum in the present investigation.
3.1.2 Removal efficiency of GHS in sewage water
Figure 2 presents the percentage removal efficiency of various physicochemical parameters in sewage water through GHS. After 5 days of treatment, sewage water exhibited reductions of 15.75% in pH, 51.20% in turbidity, 10.81% in hardness and 11.80% in dissolved oxygen (DO), respectively. However, conductivity, solids, biological oxygen demand (BOD), chemical oxygen demand (COD), calcium, magnesium and chloride ion concentrations did not show any significant reduction.
After 10 days of treatment, there was a reduction of 4%–5% in pH, 61.73% in turbidity, 26.15% in total solids (TS), 47.45% in total suspended solids (TSS), 25.24% in total dissolved solids (TDS), 12.64% in dissolved oxygen (DO) and 35.99% in chemical oxygen demand (COD) compared to the primary treatment. After 15 days of treatment, reductions of 67.10% in turbidity, 18%–20% in hardness, 30%–40% in ion concentration (Ca2+, Mg2+ and Cl−), 30%–50% in solids (TS, TSS and TDS), 24.14% in DO and 65.23% in COD in sewage water. Arulmathi et al. (2019) and DePaolis et al. (2023) studies reported that the GHS act as a coagulant, binding with suspended particles and forming flocs that settle down and reduced impurities in water through precipitation, sedimentation, ion exchange and adsorption onto surface by containing macromolecules such as protein and cellulosic materials. After 10 days reduction was gradually decreases compared to 5 days treatment, suggests that these parameters have stabilized and tries to reach at equilibrium stage. After 20 days of treatment, only pH showed a reduction of 8.64%, while DO, BOD and COD level decreased by 47.52%, 68.42% and 86.72%, respectively. However, other physicochemical parameters such as solids (TS, TSS and TDS) reduced 20%–40% increased after 20 days compared to primary (aeration) treatment.
By adding 1.0 gm of GHS to municipal sewage water, the turbidity can be reduced by 85.79% (from 84.03 to 11.93 NTU), hardness can be decreased by 16% (from 7.33 to 6.16 mg/L), total suspended solids can be reduced by 70%–80% (from 250 to 80 mg/L) and BOD and COD can be lowered by around 40% (from 56.11 to 34.02 mg/L) and 88% (from 693.75 to 77.01 mg/L), respectively, after 20 days of treatment.
The maximum removal of colour, COD, and turbidity by GHS was found to be 90.13, 72.96% and 85.48%, respectively, at pH 5.5, coagulant dose 2.2 g/L and contact time was 35 min (Arulmathi et al., 2019). Other biomaterials such as cactus leaves showed 78.28% reduction in TDS (from 1750 to 380 ppm) and 32% turbidity (from 50 to34 NTU) from wastewater (Majeed and Jasim, 2015). According to Varsani et al. (2022) activated M. olefera seeds reduced 60%–70% reduction in solid content. Hence, present research study determined that the AHS shows great efficacy of coagulation ability in sewage water.
3.1.3 Removal efficiency of TAS in sewage water
Figure 3 represents the reduction efficiency of wheat waste in sewage water. After 24 h of aeration (primary treatment), TAS biomaterial is provided as a secondary treatment for sewage water purification. After 5 days treatments, TAS material reduced the pH by 12.98%, turbidity by 44.40%, total hardness by 21.62% and dissolved oxygen (DO) level by 3.22% in sewage water. After 10 days, the conductivity level was reduced by 35.76%, turbidity by 23.07%, chloride ions by 72.50%, solids content by 52%, COD by 34.03% and there was a slight reduction in DO (0.96%) due to TAS bio-coagulant. According to Chen et al. (2019) research study reported that the TAS composed of macromolecules such as cellulose, hemicellulose, lignin and protein, which are composed of elements such as C, H, O and N. These macromolecules contain functional groups such as hydroxyl, carboxyl and amino groups with strong coordination and possess strong ability of coagulation. Total hardness (calcium and magnesium hardness) as well as ion concentrations of calcium and magnesium reduction was not observed in sewage water after 10 days of treatment when compared with primary treatment (aeration) by deposition of solids and ions in water during ion exchange mechanism of coagulation.
After 15 days of treatment with TAS bio-coagulant, only 10% reduction in hardness, while there was no reduction was observed in reduction in BOD level of sewage water due to organic deposition in sewage water. The physicochemical parameters, such as turbidity, TS, TSS, TDS and COD showed a reduction of 57.01%, 33.46%, 35.51%, 28.81% and 38.07%, respectively, in sewage water compared to primary treatment.
After 20 days of sewage water treatment, the reduction efficiency was 9.93% for pH, 25% for turbidity, 52.49% for total solids (TS), 51.69% for total suspended solids (TSS), 53.46% for total dissolved solids (TDS), 15.21% for DO and 51.25% for COD in sewage water compared to 15 days of treatment. Figure 3 proved that, with a comparison to untreated water, the TAS significantly minimized turbidity, hardness, solids and COD by 72.86% (84.03–22.8 NTU), 22.72% (7.33–5.66 mg/L), 60% (250–90 mg/L) and 59% (693.75–282.76 mg/L), respectively (Figure 3).
Kharel et al. (2016) reported that the reduction efficiency of TAS (wheat straw ash) was hardness around 81% in synthetic and fresh water. According to Tiwary et al. (2011), wheat straw treatment in wastewater responsible to reduced COD and BOD around 68% and 92% mg/L, respectively, while study revealed that sewage water treated with dual treatment (primary and secondary), it showed 60%–70% reduction in sewage water which is nearer to activated wheat straw treatment.
4 Turbidity coagulation kinetic modelling
The theoretical values of the order of the coagulation process (α) are in the range of (1≤ α ≥ 2). Basically, the aggregation process is predominant in the form of pseudo−first−order in which the collision is proportional to the product of the concentrations of two colliding species. Moreover, in real and empirical practice, extensive studies used (α = 2) and found that it was more logical in representing primarily the aggregation rate of particle count on the basis of a Brownian-controlled and rapid coagulation process.
The reduction of turbidity in sewage water by agricultural waste materials involves a multi−faceted process. Agricultural waste materials act as coagulants and flocculants, neutralizing the charges on suspended particles, bridging them together and adsorbing impurities. This results in the formation of larger flocs. As a result, these larger flocs settle more rapidly due to increased size and density, contributing to the removal of particles. Additionally, filtration is more effective with larger flocs. In addition, beneficial microbial activity is supported by the waste materials, aiding in the decomposition of organic matter and contaminants, further reducing turbidity.
Further analysis of the kinetic values indicated that TAS was least effective in reducing turbidity in sewage water treatment as compared to AHS and GHS. When comparing the coagulation kinetic model for TAS biocoagulant, the pseudo−first order was suitable (R2 = 0.18799, k1 = 0.065221 min−1 and t1/2 = 10.62 min) compared to pseudo−second−order to interpret the kinetic data. This interpreted data also proved in the primary dataset of removal efficiency that AHS reduced turbidity from 84.03 to 5.56 NTU (93.38%), while TAS reduced turbidity from 84.03 to 22.8 NTU (72.86%) (Figure 4).
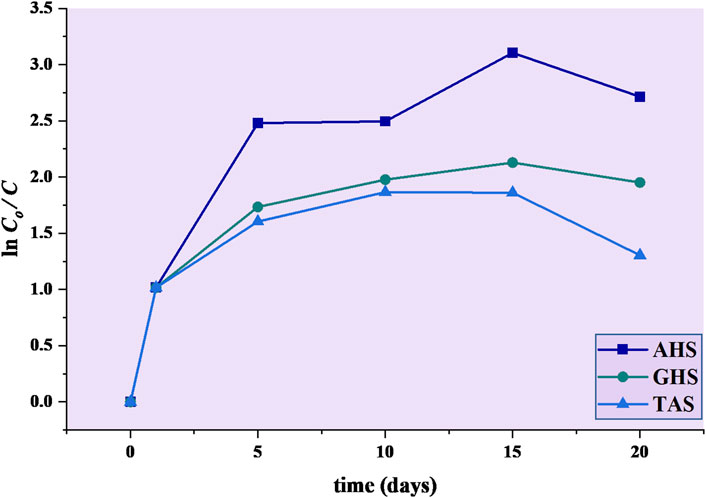
FIGURE 4. Pseudo−first−order kinetic model of agricultural waste during bio-coagulation in municipal sewage water.
Municipal sewage wastewater reported a significant reduction of turbidity as a result of addition of agricultural waste materials, including AHS, GHS, and TAS. Results from the current data (Figure 5) showed that pseudo−second order provided the finest description of the coagulation process. In this context, GHS as a biocoagulant was found (R2 = 0.6517) and AHS (R2 = 0.63386) exhibited a moderate coagulation effect (i.e., R2 = 0.6517) when compared to the other agricultural waste materials (TAS).
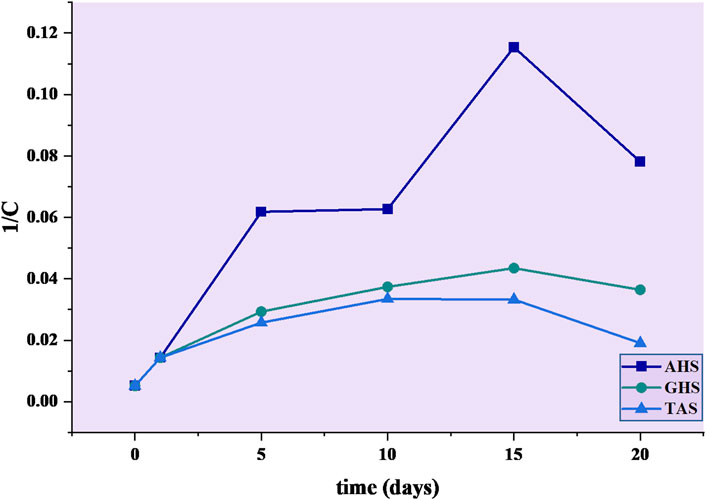
FIGURE 5. Pseudo−second−order kinetic model of agricultural waste during bio-coagulation in municipal sewage water.
However, the AHS and GHS displayed the best fit with a pseudo−second order kinetic model, as illustrated in Figure 5, which showed various kinetic variables such as the pseudo−second order rate constant (k2 = 0.003646 L mg−1 min−1), friction factor (β = 0.007 m3/kg·s), Brownian diffusion constant (D = 5.67 × 10−19 kg2/m·s), and half-life period (t1/2 = 1.41 min) were observed in AHS biocoagulant. It was also observed that k2 = 0.00156 L mg−1 min−1, β = 0.003 m3/kg·s, D = 1.32 × 10−19 kg2/m·s and a half-life period (t1/2 = 3.31 min) were reported in the GHS biocoagulant (Table 1).
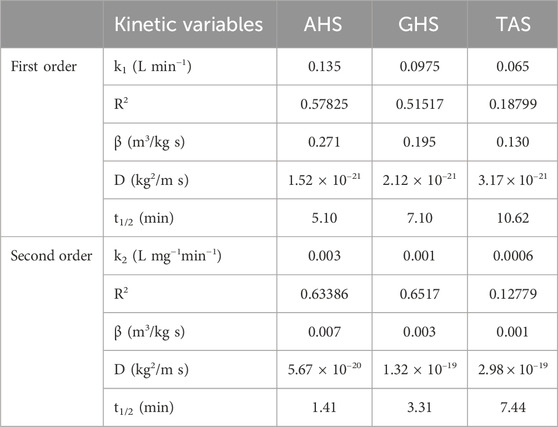
TABLE 1. Pseudo−first and second order kinetic constants during the bio-coagulation of sewage water through agricultural waste materials.
A similar kind of results were also observed in the coagulation kinetic of pseudo−second−order rate constant, which was higher in AHS biocoagulants and lower in TAS bio-coagulant as shown in Table 1. It was also confirmed by the kinetic variables, as R2 value and half-life (t1/2) of the biocoagulants. These findings suggest that amongst three agricultural waste materials, AHS could be an ideal biocoagulant for reducing turbidity in municipal sewage wastewater treatment (Figures 4, 5).
When compared with other biocoagulant such as Albizia lebbeck (L.), Clerodendrum inerme (10,000 mg/L), Azadirachta indica, Conocarpus lancifolius, Phoenix dactylifera (5,000 mg/L), Dianthus caryophyllus (3,000 mg/L) and Nerium oleander (1,000 mg/L) reported the rates of turbidity removal was 39.3%, 51.9%, 67.2%, 75.5%, 51.0%, 52.6%, and 57.2%, respectively (Salem et al., 2023). Hence, the agricultural bio-coagulants are seeming to be an effective biomaterial that has significant efficiencies in removal of turbidity in municipal sewage water.
5 Statistical analyses
5.1 Principal component analysis
PCA is a statistical technique used to reduce the dimensionality of data while preserving as much of the original variance as possible. It helps identify patterns and relationships between variables. PCA has been applied to understand the relationships and variation among the listed physico−chemical parameters. The importance of parameters in a PCA analysis is typically determined by their loading values on the principal components (Okoro et al., 2012). The loading values for PC1 and PC2 are shown in Figure 6. The loading values represent the contribution of each original variable (parameter) to the principal components (PC1 and PC2). Positive and negative values indicate the direction and strength of the relationship with the principal components.
Principal component analysis (PCA) was examined by the physico−chemical parameters of sewage water before the treatment, which explained 100% of total variance accounted by the PC1 (84.71%) and PC 2 (15.29%). Overall strength of interaction of the physico−chemical parameters showed in Figure 6. The parameters as pH, chloride, TS, TSS, TDS, DO, BOD and COD showed a positive loading with PC1. It was indicated that the PC1 represents a set of physico−chemical parameters that are positively correlated. Moreover, EC, total hardness (including calcium and magnesium), and calcium and magnesium showed a significant negative correlation with PC1. Specifically, the parameters such as pH, EC, turbidity, total hardness, TS, TDS and COD showed a significant positive loading on PC2. Additionally, BOD has a high negative loading value on PC2, indicating an inverse relationship (Figure 6).
So, the parameters that are important for PC1 and PC2 are as listed above, based on their loading values. These parameters are the ones that contribute most significantly to the variance and relationships within the dataset as captured by the two principal components. Hence, PC1 (84.71%) explains a significant loading in the total variance, it likely represents the most important underlying factor in the present study (Figure 6).
5.2 Paired comparison test
The Dunnett’s test is a suitable statistical method for conducting paired comparison tests to assess the efficacy of biomaterials. Figure 7 reports the results of a statistical analysis aimed at comparing three distinct agricultural waste materials (AHS, TAS and GHS), with a control group. The experiment consisted of treating these waste materials with municipal sewage water, and the p-values obtained for each of the samples were 0.0032, 0.0043 and 0.0039, respectively, indicating that each of the samples exhibits a highly significant (p < 0.05) difference from the control group. Therefore, it can be concluded that the agro bio-coagulants (AHS, GHS and TAS) are more effective in treating municipal sewage water.
The Dunnett’s test holds great significance with practical implications across various fields. This finding has practical implications for sewage water treatment facilities, as it highlights the potential of using agricultural waste materials as biocoagulants to improve treatment efficiency. Paired comparisons test offers a method for comparing multiple treatment groups to a single control group, simplifying complex comparisons. By providing p-values, it aids in identifying which treatments are significantly different from the control, crucial for evaluating intervention effectiveness. This statistical tool empowers researchers and analysts to make informed decisions, optimizing the selection of the most effective treatments. In scientific research, it ensures validation based on sound statistical principles. Industries like pharmaceuticals benefit from its application in quality control, maintaining consistent product quality. Moreover, it also plays a vital role in regulatory compliance, particularly in healthcare and environmental management.
6 Conclusion
The experimental utilization of agricultural waste materials as coagulants in sewage water treatment, employing a modified treatment approach, has notable and scientifically significant outcomes with direct application for the scientific community. The application of these materials has demonstrated remarkable turbidity removal efficiency, surpassing 85%–90%. Additionally, municipal sewage water treated using agricultural waste materials has exhibited reductions in key parameters. Notably, there was a 15% reduction in hardness, a 50%–55% in solid (TDS) content, and an 80%–90% decline in both BOD and COD levels after 20 days of treatment. Statistical analysis confirmed the effectiveness (p < 0.05) of agricultural biomaterials in sewage water treatment compared to pre−treated water. The Principal Component Analysis (PCA) underscores the significance of the first principal component (PC1) in explaining the majority of variance, capturing major factor influencing the treatment. The coagulation kinetic modelling indicated the potential of agricultural waste as a viable coagulant, offering promising results in terms of turbidity removal in municipal sewage wastewater treatment. The results of this study not only advance in the field of sewage water treatment but also present an opportunity for the broader scientific community to explore sustainable solutions to water quality challenges. By using agricultural waste materials, environmental sustainability can be enhanced and address the growing demand of clean water resources. These findings have practical applications for communities and industries striving for more efficient and eco-conscious wastewater treatment processes. The validated effectiveness of agricultural biomaterials, supported by statistical analysis, highlights the importance of data-driven approaches in environmental science and engineering. This research is a step towards a more sustainable and environmentally responsible future.
Data availability statement
The original contributions presented in the study are included in the article/Supplementary Material, further inquiries can be directed to the corresponding authors.
Author contributions
VV: Formal Analysis, Investigation, Methodology, Writing–original draft, Writing–review and editing. SV: Conceptualization, Supervision, Validation, Visualization, Writing–review and editing. VP: Data curation, Formal Analysis, Resources, Software, Writing–review and editing. DD: Data curation, Formal Analysis, Resources, Software, Writing–review and editing. SG: Data curation, Methodology, Resources, Validation, Writing–review and editing.DA: Data curation, Formal Analysis, Funding acquisition, Software, Writing–review and editing. VY: Conceptualization, Data curation, Methodology, Visualization, Writing–original draft, Writing–review and editing. DS: Conceptualization, Formal Analysis, Funding acquisition, Validation, Visualization, Writing–review and editing. AP: Conceptualization, Supervision, Visualization, Writing–original draft, Writing–review and editing.
Funding
The author(s) declare that no financial support was received for the research, authorship, and/or publication of this article.
Acknowledgments
The authors extend their appreciation to Researchers Supporting Project number (RSP2024R165), King Saud University, Riyadh, Saudi Arabia. Furthermore, the authors are grateful to the Department of Education, Government of Gujarat, for their support in the form of the SHODH fellowship.
Conflict of interest
The authors declare that the research was conducted in the absence of any commercial or financial relationships that could be construed as a potential conflict of interest.
Publisher’s note
All claims expressed in this article are solely those of the authors and do not necessarily represent those of their affiliated organizations, or those of the publisher, the editors and the reviewers. Any product that may be evaluated in this article, or claim that may be made by its manufacturer, is not guaranteed or endorsed by the publisher.
Supplementary material
The Supplementary Material for this article can be found online at: https://www.frontiersin.org/articles/10.3389/fenvs.2023.1280125/full#supplementary-material
References
Agarwal, N., Solanki, V. S., Gacem, A., Hasan, M. A., Pare, B., Srivastava, A., et al. (2022). Bacterial laccases as biocatalysts for the remediation of environmental toxic pollutants: a green and eco-friendly approach—a review. Water 14 (24), 4068. doi:10.3390/w14244068
Ahmad, W., Alharthy, R. D., Zubair, M., Ahmed, M., Hameed, A., and Rafique, S. (2021). Toxic and heavy metals contamination assessment in soil and water to evaluate human health risk. Sci. Rep. 11 (1), 17006. doi:10.1038/s41598-021-94616-4
Ahmed, M., Mavukkandy, M. O., Giwa, A., Elektorowicz, E., Katsou, E., Khelifi, O., et al. (2022). Recent developments in hazardous pollutants removal from wastewater and water reuse within a circular economy. Npj Clean. Water 5 (1), 12. doi:10.1038/s41545-022-00154-5
Ajiboye, T. O., Oladoye, P. O., Olanrewaju, C. A., and Akinsola, G. O. (2022). Organophosphorus pesticides: impacts, detection, and removal strategies. Environ. Nanotechnol. Monit. Manag. 17, 100655. doi:10.1016/j.enmm.2022.100655
Arulmathi, P., Jeyaprabha, C., Sivasankar, P., and Rajkumar, V. (2019). Treatment of textile wastewater by coagulation–flocculation process using Gossypium herbaceum and Polyaniline coagulants. Clean–Soil, Air, Water 47 (7), 1–10. doi:10.1002/clen.201800464
Bahrodin, M. B., Zaidi, N. S., Hussein, N., Sillanpää, M., Prasetyo, D. D., and Syafiuddin, A. (2021). Recent advances on coagulation-based treatment of wastewater: transition from chemical to natural coagulant. Curr. Pollut. Rep. 7 (3), 379–391. doi:10.1007/s40726-021-00191-7
Bahrodin, M. B., Zaidi, N. S., Kadier, A., Hussein, N., Syafiuddin, A., and Boopathy, R. (2022). A novel natural active coagulant agent extracted from the sugarcane bagasse for wastewater treatment. Appl. Sci. 12 (16), 7972. doi:10.3390/app12167972
Bijekar, S., Padariya, H. D., Yadav, V. K., Gacem, A., Hasan, M. A., Awwad, N. S., et al. (2022). The state of the art and emerging trends in the wastewater treatment in developing nations. Water 14 (16), 2537. doi:10.3390/w14162537
Chahar, M., Khaturia, S., Singh, H. L., Solanki, V. S., Agarwal, N., Sahoo, D. K., et al. (2023). Recent advances in the effective removal of hazardous pollutants from wastewater by using nanomaterials—a review. Front. Environ. Sci. 11. doi:10.3389/fenvs.2023.1226101
Chen, Y., Chen, Q., Zhao, H., Dang, J., Jin, R., Zhao, W., et al. (2020). Wheat straws and corn straws as adsorbents for the removal of Cr(VI) and Cr(III) from aqueous solution: kinetics, isotherm, and mechanism. ACS Omega 5 (11), 6003–6009. doi:10.1021/acsomega.9b04356
Choudhary, M., and Neogi, S. (2017). A natural coagulant protein from Moringa oleifera: isolation, characterization, and potential use for water treatment. Mater. Res. Express 4 (10), 105502. doi:10.1088/2053-1591/aa8b8c
CPCB (Central Pollution Control Board) (2021). Status of water quality in India: a report on wastewater management. Central Pollution Control Board, Min. of Environ. For. and Clim. Change., Gov. of India. Available at: https://cpcb.nic.in/.
Cui, H., Zhou, J., Li, Z., and Gu, C. (2021). Editorial: soil and sediment pollution, processes and remediation. Front. Environ. Sci. 9. doi:10.3389/fenvs.2021.822355
De, S., Mishra, S., Poonguzhali, E., Rajesh, M., and Tamilarasan, K. (2020). Fractionation and characterization of lignin from waste rice straw: biomass surface chemical composition analysis. Int. J. Biol. Macromol. 145, 795–803. doi:10.1016/j.ijbiomac.2019.10.068
DePaolis, M., De Respino, S., Samineni, L., Brighton, S., and Kumar, M. (2023). Cottonseed extract as a coagulant for water treatment. Environ. Sci. Adv. 2 (2), 227–234. doi:10.1039/D2VA00205A
Grass Ramírez, J. F., Muñoz, R. C., and Zartha Sossa, J. W. (2023). Innovations and trends in the coconut agroindustry supply chain: a technological surveillance and foresight analysis. Front. Sustain. Food Syst. 7. doi:10.3389/fsufs.2023.1048450
Ishak, K. A. S. S., Panneerselvam, A., Ambikapathy, V., Sathya, R., and Vinothkanna, A. (2021). An investigation of sewage water treatment plant and its physico-chemical analysis. Biocatal. Agric. Biotechnol. 35, 102061. doi:10.1016/j.bcab.2021.102061
Ishwari, B. K., Kumar, P. S., and Shivaprasad, K. H. (2022). Adsorption studies of heavy metals on coal fly ash samples from aqueous solutions. Appl. Ecol. Environ. Sci. 10 (1), 25–33. doi:10.12691/aees-10-1-5
Jabar, J. M., Odusote, Y. A., Alabi, K. A., and Ahmed, I. B. (2020). Kinetics and mechanisms of Congo red dye removal from aqueous solution using activated Moringa oleifera seed coat as adsorbent. Appl. Water Sci. 10 (6), 136. doi:10.1007/s13201-020-01221-3
Jan, I., Ahmad, T., Wani, M. S., Dar, S. A., Wani, N. A., Malik, N. A., et al. (2022). “Chapter 1 - threats and consequences of untreated wastewater on freshwater environments,” in Microbial consortium and biotransformation for pollution decontamination. Editors G. H. Dar, R. A. Bhat, H. Qadri, and K. R. Hakeem (Amsterdam, Netherland: Elsevier), 1–26. doi:10.1016/B978-0-323-91893-0.00009-2
Joyce, K., Fabra, G. T., Bozkurt, Y., and Pandit, A. (2021). Bioactive potential of natural biomaterials: identification, retention and assessment of biological properties. Signal Transduct. Target. Ther. 6 (1), 122. doi:10.1038/s41392-021-00512-8
Keeping, M. G. (2017). Uptake of silicon by sugar cane from applied sources may not reflect plant-available soil silicon and total silicon content of sources. Front. Plant Sci. 8, 760. doi:10.3389/fpls.2017.00760
Kerrou, M., Bouslamti, N., Raada, A., Elanssari, A., Mrani, D., and Slimani, M. S. (2021). The use of sugarcane bagasse to remove the organic dyes from wastewater. Int. J. Anal. Chem. 2021, 1–11. doi:10.1155/2021/5570806
Kharel, H. L., Sharma, R. K., and Kandel, T. P. (2016). Water hardness removal using wheat straw and rice husk ash properties. Nepal J. Sci. Technol. 17 (1), 11–16. doi:10.3126/njst.v17i1.25057
Khan, F. A., Tomar, A., Agarwal, Y. K., and Shukla, H. O. (2020). “Agricultural solid waste management: an approach to protect the environment and increase agricultural productivity,” in Handbook of solid waste management: sustainability through circular economy. Editors C. Baskar, S. Ramakrishna, S. Baskar, R. Sharma, A. Chinnappan, and R. Sehrawat (Singapore: Springer), 1–27. doi:10.1007/978-981-15-7525-9_28-1
Kingue, B., Njila, R., and Ndongo, B. (2023). Assessment of groundnut (Arachis hypogaea) as a natural coagulant for water treatment. Water Pract. Technol. 18 (1), 2057–2067. doi:10.2166/wpt.2023.127
Koul, B., Yadav, D., Singh, S., Kumar, M., and Song, M. (2022). Insights into the domestic wastewater treatment (DWWT) regimes: a review. Water. 14, 3542. doi:10.3390/w14213542
Liu, L., Meng, S., Li, C., and Li, Y. (2022). Revisiting the theory of coagulation of colloidal dispersions: an improved expression for the stability ratio. Langmuir 38 (3), 1131–1140. doi:10.1021/acs.langmuir.1c02790
Luis-Zarate, V. H., Rodriguez-Hernandez, M. C., Alatriste-Mondragon, F., Chazaro-Ruiz, L. F., and Rangel-Mendez, J. R. (2018). Coconut endocarp and mesocarp as both biosorbents of dissolved hydrocarbons in fuel spills and as a power source when exhausted. J. Environ. Manag. 211, 103–111. doi:10.1016/j.jenvman.2018.01.041
Majeed, M. R., and Jasim, H. A. (2015). Reducing the turbidity of wastewater by some plant based coagulant. Int. J. Sci. Res. 6, 1014–1016.
Madhav, S., Ahamad, A., Singh, A. K., Kushawaha, J., Chauhan, J. S., Sharma, S., et al. (2020). “Water pollutants: sources and impact on the environment and human health,” in Sensors in water pollutants monitoring: role of material. Editors D. Pooja, P. Kumar, P. Singh, and S. Patil (Singapore: Springer), 43–62. doi:10.1007/978-981-15-0671-0_4
Mohd Nor, N. A. N., Abbasiliasi, S., Marikkar, M. N., Ariff, A., Amid, M., Lamasudin, D. U., et al. (2017). Defatted coconut residue crude polysaccharides as potential prebiotics: study of their effects on proliferation and acidifying activity of probiotics in vitro. J. Food Sci. Tech. 54 (1), 164–173. doi:10.1007/s13197-016-2448-9
Naruka, A. K., Suganya, S., Kumar, P. S., Amit, C., Ankita, K., Bhatt, D., et al. (2021). Kinetic modelling of high turbid water flocculation using native and surface functionalized coagulants prepared from shed-leaves of Avicennia marina plants. Chemosphere. 272, 1–9. doi:10.1016/j.chemosphere.2021.129894
Nazari, L., Xu, C., Charles), , and Ray, M. B. (2021). “Nitrogen and phosphorous recovery from municipal wastewater and sludge,” in Advanced and emerging technologies for resource recovery from wastes. Editors L. Nazari, C. Xu, and M. B. Ray (Singapore: Springer), 97–125. doi:10.1007/978-981-15-9267-6_4
Okey-Onyesolu, C. F., Onukwuli, O. D., Ejimofor, M. I., and Okoye, C. C. (2020). “Kinetics and mechanistic analysis of particles decontamination from abattoir wastewater (ABW) using novel Fish Bone Chito-protein (FBC),” Heliyon, 6. doi:10.1016/j.heliyon.2020.e04468
Okoro, H. K., Adeyinka, A., Jondiko, O. E., and Ximba, B. J. (2012). Physico-chemical analysis of selected groundwater samples of Ilorin town in Kwara State, Nigeria. Sci. Res. Essays 7 (23), 2063–2069. doi:10.5897/SRE11.1889
Patel, H., Yadav, V. K., Yadav, K. K., Choudhary, N., Kalasariya, H., Alam, M. M., et al. (2022). A recent and systemic approach towards microbial biodegradation of dyes from textile industries. Sci. Res. Essays. 14, 3163. doi:10.3390/w14193163
Priyan, K. (2021). “Issues and challenges of groundwater and surface water management in semi-arid regions,” in Groundwater resources development and planning in the semi-arid region. Editors C. B. Pande,, and K. N. Moharir (Singapore: Springer International Publishing), 1–17. doi:10.1007/978-3-030-68124-1_1
Rabari, V., Patel, H., Patel, K., Patel, A., Bagtharia, S., and Trivedi, J. (2023). Quantitative assessment of microplastic contamination in muddy shores of Gulf of Khambhat, India. Mar. Pollut. Bull. 192, 115131. doi:10.1016/j.marpolbul.2023.115131
Rai, A., Amari, A., Yadav, V. K., Ismail, M. A., Elboughdiri, N., Fulekar, M. H., et al. (2022). A synergistic effect of Moringa oleifera-based coagulant and ultrafiltration for the wastewater treatment collected from final ETP. Adsorpt. Sci. Technol. 2022, 1–9. doi:10.1155/2022/1285011
Salem, A. K., Almansoory, A. F., and Al-Baldawi, I. A. (2023). Potential plant leaves as sustainable green coagulant for turbidity removal. Heliyon 9 (5), e16278. doi:10.1016/j.heliyon.2023.e16278
Sathiparan, N., Anburuvel, A., and Selvam, V. V. (2023). Utilization of agro-waste groundnut shell and its derivatives in sustainable construction and building materials – a review. J. Build. Eng. 66, 105866. doi:10.1016/j.jobe.2023.105866
Tiwary, K. N., Sharma, S. K., and Kumar, A. (2011). Anaerobic treatment of wheat straw wash water. Indian Pulp. Paper Technical Asso. 23 (1), 187–189.
Uko, C. A., Tijani, J. O., Abdulkareem, S. A., Mustapha, S., Egbosiuba, T. C., and Muzenda, E. (2022). Adsorptive properties of MgO/WO3 nanoadsorbent for selected heavy metals removal from indigenous dyeing wastewater. Process Saf. Environ. Prot. 162, 775–794. doi:10.1016/j.psep.2022.04.057
United Nations (2021). World population prospects 2021. United. Nation. Depart. of Econ. and Soc. Affairs., Popul. Div. Available at: https://population.un.org/wpp/Publications/Files/WPP2021_Highlights.pdf.
Varalalakshmi, V., Ponkalavani, S., Shajina, K., and Sangeetha, M. (2021). Biodegradable plastic from the shells of Arachis hypogaea (groundnut). Eur. J. Pharm. Med. Res. 8 (2), 511–517.
Varsani, V., Vyas, S. J., and Dudhagara, D. R. (2022). Development of bio-based material from the Moringa oleifera and its bio-coagulation kinetic modeling – a sustainable approach to treat the wastewater. Heliyon 8 (9), e10447. doi:10.1016/j.heliyon.2022.e10447
Walanda, R. M., and Napitupulu, M. (2017). Reduction of BOD and COD in hospital waste with biochar from peanut shells (Arachis hypogaea L.). Int. J. Res. Publ. Rev. 4 (6), 385–390.
Wasify, R. S., Hamed, S. R., and Ragab, S. (2023). Assessing the potential of de-oiled peanut (Arachis hypogea) seeds for surface water treatment: a sustainable alternative to chemical coagulants. Egypt. J. Aquatic Res. 49, 297–302. doi:10.1016/j.ejar.2023.04.003
Yadav, B., Parker, A., Sharma, A., Sharma, R., Krishan, G., Kumar, S., et al. (2023a). Estimation of groundwater recharge in semi-arid regions under variable land use and rainfall conditions: a case study of Rajasthan, India. PLOS Water 2 (3), e000061. doi:10.1371/journal.pwat.0000061
Yadav, V. K., Amari, A., Gacem, A., Elboughdiri, N., Eltayeb, L. B., and Fulekar, M. H. (2023b). Treatment of fly-ash-contaminated wastewater loaded with heavy metals by using fly-ash-synthesized iron oxide nanoparticles. Water 15 (5), 908. doi:10.3390/w15050908
Yadav, V. K., Gupta, N., Kumar, P., Dashti, M. G., Tirth, V., Khan, S. H., et al. (2022). Recent advances in synthesis and degradation of lignin and lignin nanoparticles and their emerging applications in nanotechnology. Materials 15 (3), 953. doi:10.3390/ma15030953
Keywords: municipal sewage water, agricultural waste materials, bio-coagulant, kinetic modelling, statistical analysis
Citation: Varsani VG, Vyas SJ, Parmar V, Dudhagara D, Gamit S, Ali D, Yadav VK, Sahoo DK and Patel A (2024) Efficient and eco-friendly treatment of wastewater through sustainable purification using agricultural waste and coagulation kinetic modelling. Front. Environ. Sci. 11:1280125. doi: 10.3389/fenvs.2023.1280125
Received: 19 August 2023; Accepted: 22 December 2023;
Published: 09 January 2024.
Edited by:
Wenyi Deng, Donghua University, ChinaReviewed by:
Marco Carnevale Miino, University of Insubria, ItalyAmin Mojiri, Hiroshima University, Japan
Copyright © 2024 Varsani, Vyas, Parmar, Dudhagara, Gamit, Ali, Yadav, Sahoo and Patel. This is an open-access article distributed under the terms of the Creative Commons Attribution License (CC BY). The use, distribution or reproduction in other forums is permitted, provided the original author(s) and the copyright owner(s) are credited and that the original publication in this journal is cited, in accordance with accepted academic practice. No use, distribution or reproduction is permitted which does not comply with these terms.
*Correspondence: Suhas J. Vyas, vsuhas.13@gmail.com; Virendra Kumar Yadav, yadava94@gmail.com; Dipak Kumar Sahoo, dsahoo@iastate.edu; Ashish Patel, uni.ashish@gmail.com