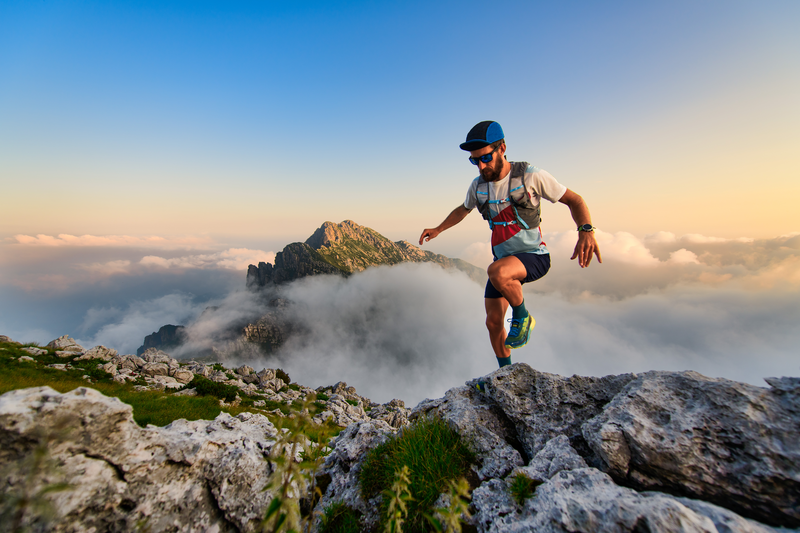
95% of researchers rate our articles as excellent or good
Learn more about the work of our research integrity team to safeguard the quality of each article we publish.
Find out more
ORIGINAL RESEARCH article
Front. Environ. Sci. , 09 January 2024
Sec. Environmental Economics and Management
Volume 11 - 2023 | https://doi.org/10.3389/fenvs.2023.1276382
This article is part of the Research Topic Collaborative economy CE5P (Planet, People, Partnership, Prosperity, Peace) View all 6 articles
Electric vehicles (EVs) are regarded as a key low-carbon technology to address sustainability challenges like climate change and air pollution. However, the real-world environmental impacts resulting from rapid EV adoption remain uncertain. This study develops a theoretical framework to quantify the impacts of EV adoption on energy consumption, carbon emissions, and air quality. Then we empirically investigates the influence of surging EV uptake in China using provincial panel data from 2015 to 2020. Our results indicate that increased EV stocks significantly reduced gasoline consumption but boosted coal-based power demand, shifting emissions and air pollutants from transportation to the electricity sector rather than yielding absolute reductions. We find important regional heterogeneity based on differences in grid generation profiles. In provinces more reliant on coal power, the environmental impacts were more severe. The findings also reveal a spatial spillover effect, with emissions transferred from net power-importing regions to exporters. Overall, the rapid EV transition alone appears insufficient to guarantee emissions cuts and environmental gains. Complementary efforts across sectors are essential to align industrial promotion with sustainability objectives. The empirical evidence informs integrated policy design and metrics to maximize decarbonization as EVs are deployed globally. Future research can build on this study by expanding geographical scope, incorporating projections, adopting a multi-disciplinary lens, leveraging microdata, and applying cutting-edge analytical techniques. Pursuing these directions will further advance knowledge on sustainable EV transitions.
Sustainable development goals have recently drawn a lot of attention. Reducing air pollution and slowed energy consumption growth have become China’s two most pressing concerns for sustainable development. China has promoted the use of the electric vehicles for this reason. In 2010, the Chinese government issued the “Decision on Accelerating the Cultivation and Development of Strategic Emerging Industries,” which listed the development of electric vehicles industry as one of the strategic emerging industries. Since then, the implementation of many policies has promoted the development of electric vehicle industry. China released the “Energy-saving and New Energy Automobile Industry Development Plan (2012–2020)” in July 2012 and noted that the demand for automobiles would continue to rise for a considerable amount of time, leading to an increase in energy consumption and more severe environmental pollution issues. Accelerating the development of new energy vehicles would effectively alleviate the pressure of energy consumption and environmental pollution, stimulate the transformation and upgrading of the automotive industry, and cultivate new economic growth source and international competitive advantage of strategic initiatives. In October 2020, the Chinese government released the “New Energy Vehicle Industry Development Plan (2021–2035),” striving to effectively promote the level of energy conservation and emission reduction and the efficiency of social operation, and achieve international advanced level of core technology and strong international competitiveness of quality brands of Chinese new energy vehicles. The rapid growth of China’s EV industry and great efforts made by the Chinese government attract substantial research attention (Hao et al., 2014; Zhang et al., 2017; Wu et al., 2021).
With rapid economic development and urbanization, China has become the world’s largest automobile market, with an average growth rate of 17.5% since 2000 (Hao et al., 2016). Air pollution was a common challenge in the urbanization of industrialized countries (IEA, 2019), and automobiles have become a major source of air pollution in China (He and Jiang, 2021). China has introduced a series of regulations to reduce the environmental impact of air pollutants emitted by automobiles. Liu et al. (2022) used the emission factor method to analyze automobile air pollutant emissions in 31 provinces from 2006 to 2016, and their results showed that pollutants emission efficiency of automobiles had a significant inhibitory effect on three major air pollutants. In terms of the structure of energy consumption in automobile industry, electric vehicles, as an effective means to reduce air pollution, have long been the focus of government attention (Su et al., 2021). Xie et al. (2021) explored the environmental impact of subsidies for electric vehicles on urban air pollution. Their results indicated that a 1% increase in the size of subsidies for electric vehicles would reduce air pollution levels by 0.15%. Su et al. (2021) found that electric vehicles could not be regarded as effective measures to reduce air pollution. They suggest that EVs still do not account for a high proportion of car sales, and therefore, its effect on environmental protection is not obvious.
Carbon emission has been one of the important research topics of sustainable development. Automobiles emit large amounts of carbon compounds, causing serious environmental pollution problems, and efforts have been made worldwide to address this. Klier and Linn (2015) analyzed new vehicle registration data from European countries and found that CO2 taxes reduced car registrations. In order to reduce automotive carbon emissions, China has also introduced several policies to develop EV industry. Zhao and Sun (2022) analyzed short- and long-term policy effects related to EV industry on carbon emissions in the local transportation sector. They found the increasing use of electric vehicles reduced carbon emission. Yu et al. (2022) found that the total life-cycle energy consumption and carbon emission of all electric vehicles are lower than those of gasoline vehicles.
China’s growing oil demand brings negative issues such as energy security concerns, carbon emissions and air pollution. It was important to control the total oil consumption to ensure the security of national energy supply, and to cope with climate change and environmental pollution (Jiang et al., 2022). The transportation sector was the main source of crude oil consumption and carbon emissions in China (Wang et al., 2019). According to the forecast of Ma et al. (2012), 64% of crude oil consumption by 2030 would be consumed by the transportation sector. If the Chinese government took no action on reducing oil demand, China’s oil demand would reach 808 million tons annually by 2030, with 43% of that demand coming from transportation. China’s reliance on oil imports would have increased to 80% by then (Oliver et al., 2009). Automobiles have emerged as one of the major contributors to the growth in oil consumption (Zhang et al., 2017). The use of electric vehicles could largely help reduce oil consumption. According to estimates made by Wu et al. (2012), coal power would reduce well-to-wheels (WTW) CO2 emissions by 17% compared to a low-private light passenger vehicle scenario by 2030, when it was projected to generate only 41% of the nation’s electricity.
Electric vehicles have been seriously considered as an industrial revolution toward sustainable transportation in China as a result of the serious environmental, energy security, and health issues that are being caused by the rapid increase in the number of automobiles (Wu et al., 2012). Zhao and Heywood (2017) investigated how electrification could help cut energy demand, oil dependence, and carbon emissions from transportation in China. Their findings showed that electric vehicles had a great potential to do so because of its high efficiency in energy use. The performance of electric vehicles varied across different countries. Holland et al. (2016) analyzed and compared the relevant data of gasoline-powered vehicles and electric vehicles in various states in the United States, revealing that the environmental benefits of electric cars differ due to local pollution and policies. As transportation was a significant contributor to global and local air pollution (Davis and Kilian, 2011), electric vehicles had a unique appeal in transforming individual transportation. Holland et al. (2021) used a dynamic model that captured the decreasing cost of electric cars to analyze this transition in terms of reducing electricity pollution and increasing vehicle substitutability. Their analysis of the US market showed that transitioning from gasoline cars was not optimal under current substitutability, as a ban on gasoline car production would result in significant unnecessary losses. Additionally, in the United Kingdom, the pleasure of driving and the identity derived from owning and using EVs also influenced drivers’ willingness to use electric cars (Schuitema et al., 2013).
Since 2009, China started to clarify the development of EVs and explicitly increased related financial support. In 2011, a comprehensive transition strategy for pure electric drive technology was proposed. The rollout took place in cities that qualified for the subsidy between 2009 and 2015. In 2016 the subsidy was expanded to the entire nation. Price subsidies decreased from 2016 to 2020. Financial support policies implied that the government believed the cost of EVs will fall as commercialization rose (Hao et al., 2014).
In the process of automobile electrification, electricity was mainly derived from thermal power generation, which was closely related to carbon emissions. Therefore, the use of coal should also be of concern. Li and Leung (2012) examined the relationship between coal consumption and real GDP in China and found that the relationship between coal consumption and GDP in eastern and central regions was bidirectional, and thus energy conservation measures may have dragged down the economies of eastern and central regions where coal-intensive industries were concentrated. Hofmann et al. (2016) applied a scenario analysis and argued that EV industry could contribute to the reduction of oil dependence, improvement of air quality, and reduction of carbon emissions only when their introduction was accompanied by aggressive electricity sector decarbonization.
Some literatures point out that the EV industry can hardly reduce air pollutants and GHG emissions (Ji et al., 2015; Mahmoud et al., 2016; Huo et al., 2010). If electricity is still generated by fossil energy, the reduced carbon emissions and air pollution of electric vehicles in the transportation sector will be partially offset by the increase in the electricity sector. The adoption of EVs can only transfer the pollution and carbon emission between departments, which is not conducive to reduce the total carbon emissions, environmental pollution and reliance on fossil energy. As electricity in China mainly comes from thermal power generation, the relationship between the use of electric, carbon emission, air pollution and energy consumption remain unclear. Thus, we investigate whether the development of EV industry help reduce carbon emission, air pollution and energy consumption in China.
This study addresses the pressing research question of how surging electric vehicle adoption in China is impacting energy consumption, carbon emissions, and air pollution. The primary objective is to provide empirical evidence on the holistic environmental sustainability impacts of EVs based on recent provincial data. Most prior studies are prospective and rely on scenarios, hence our work fills the knowledge gap by quantifying real-world outcomes from the expansion of EVs across provinces and over time. Investigating these dynamics is crucial given the central role of EVs in China’s carbon peaking and neutrality strategy. This research would help coordinate policy design and integrated metrics that align industrial growth with emissions mitigation across sectors.
Our findings suggest that increasing EV consumption significantly reduces gasoline consumption, which is consistent with previous studies that the problem of oil dependence has been alleviated (Hofmann et al., 2016; Zhao and Heywood, 2017). However, we find that an increase in EVs have a positive effect on coal consumption in general, implying that the electricity consumed mainly comes from thermal power generation, which uses coal as the main source. The impact of increased coal burning is much higher than that of reduction in gasoline burning, resulting in an increase in total carbon emissions in China. For the overall impacts on the air quality index, our results suggest EVs have no significant impact.
It is also interesting to note that for regions with high thermal power reliance, using more EVs does not change much of the total CO2 emission of the region. However, for regions with relatively lower levels of thermal power reliance, an increase in EVs shows a significant increase in total CO2 emissions. Low thermal reliance areas relied more on renewable or other clean energy to generate electricity, however, with a large influx of EVs, the original power capacity does not seem to be sufficient to supply the EV, therefore, these regions would start to use coal to satisfy the increased electricity need. In addition, our results suggest that EVs increase CO2 emissions in net electricity export regions but do not have a significant effect in net electricity import regions.
We make several contributions to the literature. First, we advance the literature by quantifying the impacts of EVs on oil dependence, carbon emissions, and air pollution. We find that EVs can increase overall coal consumption and result in higher carbon emissions and air pollution in China, which echoes the findings of Hofmann et al. (2016) and Su et al. (2021). Second, we provide new insight into how the initial state of energy profile (i.e., whether it uses coal or clean energy) affects the EVs-carbon emission relationship and find regions with a higher initial clean energy source actually contribute to most of the incremental increase in coal consumption in China. Last, we provide evidence showing that the EV-carbon emission/air pollution relationship depends on the location of the power plant, especially whether the region has a net electricity export or a net electricity import.
This paper is organized as follows. Section 2 provides a literature review on EVs and their environmental impacts. Section 3 develops the theoretical framework to ground empirical analysis. Section 4 describes the data used in the empirical analysis. Section 5 develops the empirical models. Section 6 discusses the main results and conducts heterogeneity investigation. Section 7 discuss the contributions, policy implications and limitations. Section 8 concludes.
Compared to conventional gasoline vehicles, the tailpipe emissions of EVs can drastically reduce chemical substances, such as CO2, Nitrogen oxides (NOx), and Sulphur dioxide (SO2). A large body of literature has documented the favorable impacts of electric vehicles on the environment. For example, by applying quantile-on-quantile regression on monthly data, Xu et al. (2021) find that EVs reduce CO2 emissions in eight leading countries. Using the method of moments quantile regression, Kazemzadeh et al. (2022) find that both battery electric vehicles and plug-in hybrid electric vehicles can significantly reduce air pollutants PM2.5 in 28 European countries from 2010 to 2019. Using a similar quantile regression method, Fuinhas et al. (2021) and Koengkan et al. (2022) find that electric vehicles can also reduce greenhouse gas emissions in European countries. Using an Electricity Supply and Emissions Model, Zhao and Heywood (2017) forecast that the electrification of light-duty vehicles can reduce the energy demand, oil dependence, and greenhouse gas emissions in China in the feasible future. Analyzing from a life cycle perspective, Yu et al. (2022) find the life cycle total energy consumption and greenhouse emissions of all-electric vehicles are lower compared to gasoline internal combustion engine vehicles.
Since government energy and environment policies can impose constraints or provide incentives to car manufacturers and vehicle consumers, the discussion on the relationship between electric vehicles and environments often extends to evaluations of the government’s policies. For instance, Government policies, such as imposing a CO2 tax can reduce car registrations and greenhouse gas emissions (Klier and Linn, 2015). Holland et al. (2021) find that simply put a gasoline vehicle production ban would not be optimal given the current U.S. market condition. A cumulative gasoline vehicle production quota and an electric vehicle purchase subsidy will have a smaller deadweight loss and are more desirable. Klier and Linn (2015) find that the carbon dioxide (CO2) emission rate tax discourages new vehicle registration in France, Germany, and Sweden. He and Jiang (2021) find that the policy which restricts gasoline car purchases significantly slowed down the growth of daily PM10 in the policy-treated cities. Xie et al. (2021) find that the implementation of the new energy vehicle1 subsidy program, which in turn increases electric vehicle sales, could significantly improve urban air pollution in China from 2006 to 2018.
Although the direct reduction of air pollutants and greenhouse emissions from electric vehicles’ tailpipes is indisputable, their overall environmental impact is more complicated. The main reason is that the power source of electricity can come from burning fossil fuels, especially coal, which produces a large amount of air pollutants and emits greenhouse. Therefore, to evaluate the consequence of switching from conventional fossil fuel vehicles to electric vehicles in one region, it is imperative to understand its electricity-generating system. Holland et al. (2016) find in the United States; the environmental benefits of electric cars differ in different states due to the distributed nature of the electricity grid. California, for instance, can generate much of its electricity from clean energy sources, and it will be better off switching from gasoline to electric vehicles, while in North Dakota, where electricity is mainly generated from burning coal and natural gas, switching to electric vehicles can even increase air pollutants and greenhouse emissions. Ajanovic and Haas (2019) make a life cycle assessment of EV’s environmental impact and find the environmental benignity of EVs is most sensitive to the electricity mix. Increasing the share of renewable energy sources in electricity generation is crucial for EVs to have a beneficial environmental impact.
In China, there is also substantial evidence showing that with the current electricity sector profile, which is mainly from burning coal, massive adoption of electric vehicles can negatively impact the environment. For example, Wu et al. (2012) find that driving electric cars can help cut per-kilometer petroleum use substantially, but do not reduce CO2 emissions, especially in areas where coal is the main power source for electric generating. Ji et al. (2015) find that using electric vehicles in urban areas shifts air pollution to rural areas, where most power plants are located. Su et al. (2021) analyze the transportation sector and find no evidence that new energy vehicles help reduce air pollutants (PM2.5) in China from 2013 to 2020. Using an environmental Input-Out model and scenario analysis, Hofmann et al. (2016) find that if gasoline vehicles are replaced by electric vehicles, which are powered by 80% coal, the CO2 emission reduction in the petroleum sector will be offset by an increase in the electricity sector, leaving the total national CO2 emission unchanged.
Our study differs from previous literature in several ways. First, we make a refinement on the measurement of EV’s prevalence, including both the absolute quantity and relative quantity (as compared to gasoline vehicles) to better capture the adoption scale in different regions in China. Second, we not only analyze the total CO2 emission, but also distinguish CO2 generated from Gasoline from Coal in our main analysis and heterogeneity analysis, which provides quantitative evidence showing the how EVs transfer emissions from tailpipes to thermal power plants. Third, we consider EV’s impacts on carbon emissions and air pollution accounting for heterogeneous electricity generation profile and net export/import status using province level data, which have not been thoroughly studied in previous literature.
This section discusses the theoretical framework that identifies the relationships among vehicle usage, energy consumption, CO2 emission, and air pollution. First and foremost, we would like to pin down how vehicles use (both electric and internal combustion engine vehicles) can affect energy consumption. Our framework builds upon the work by Beenstock and Dalziel (1986) which analyzes factors that can affect energy demand. Hasanov (2021) provides a modified framework that incorporates demographic measure into the energy demand function. In this study, we extend previous model by including vehicles usage into the analysis.
The production of transportation service (measured by the usage of either electric and internal combustion engine vehicles) depends on the availability of energy, labor, and capital, which can be expressed as:
We assume that the production of transportation service takes the Cobb-Douglas type production function and Eq. 1 can be written as:
The natural logarithmic transformation of Eq. 2 can be expressed as:
The first order condition can be derived by cost minimization from Eq. 3. Then we can derive the energy demand equation:
where
Since that energy is the sum of fossil fuels energy (FE) and renewable energy (RE), and the vehicles include electric vehicles (EV) and internal combustion engine vehicles (ICEV) Eq. 4 can be written as:
Applying Taylor expansion to the both side and ignore high-order terms, we have
Next, we examine the relationship between CO2 emission, air pollutant emissions and vehicles usage. CO2 emission is proportional to fossil fuel energy use (Hasanov, 2021), and air pollution level is also directly linked with energy consumption and the transportation sector. Burning more fossil fuels, in particular, can increase air pollution level substantially. Therefore, we can express the relationship between CO2 and air pollutants emissions with fossil energy use:
Equations 6–8 show clear relationships between the use of EV with fossil energy consumption, carbon emission and air pollutants emissions. Thus, this study aims to figure out their relationship empirically.
We focus on the impact of EVs on air pollution, carbon emissions, and energy consumption in this paper. We collected the most recent data and our sample spans the years 2015–2020. This corresponds to the years of rapid EV adoption following major government promotion policies. By spanning the initial policy rollout through mass market uptake, our study period captures the environmental impacts of recent EV expansion triggered by industrial planning.
Our data on automobiles, which includes the number of electric and conventional vehicles, is collected from DaaS-Auto database. In terms of air pollution, we collect data on the air quality index (AQI), PM2.5, and PM10 from the China Air Quality Online Detection and Analysis Platform. The provincial air quality data is calculated by taking the average of the cities within each province. Figure 1 shows the historical trends of the distribution of average air quality index. Clearly, the air pollution has been mitigated during this period, due to substantial efforts in environmental protection made by the Chinese government. However, the impact of EV adoptions on air quality can hardly be concluded from Figure 1. Therefore, we employ the panel regression to uncover this issue.
Energy consumption data, such as gasoline and coal are gathered from CSMAR database and the National Bureau of Statistics of China. Total amount of carbon emissions, carbon emissions from gasoline consumption and coal consumption are gathered from CSMAR. These variables of energy consumption, emissions, and air pollutants are commonly examined in the literature, which align with our research objective of assessing the holistic environmental impact of EVs. Figure 2 shows the historical energy dependence structure during the sample period. Although the proportion of clean energy is significantly increasing, China still relies heavily on thermal power, implying that most of the electricity consumed from EV adoption and production come from coal consumption. Thus, the impact of EV adoption on SDGs remains unclear.
Our sample covers 32 provinces and districts in China mainland. Other control variables are collected from the National Bureau of Statistics of China which include GDP Per Capita, industrial added value, population density, afforestation area, proportion of urban population, treatment of waste gases, total energy consumption and employment. These control variables are widely used in previous studies. Their inclusion follows established models linking emissions, energy use, and growth. We also control for overall energy consumption based on prior work showing its importance in explaining environmental performance. Table 1 lists the definitions of the variables and Table 2 provides the summary statistics. As the recent panel literature states, non-stationarity, cross-sectional dependency, and heterogeneity issues should be addressed in empirical studies (Baltagi and Hashem Pesaran, 2007; Pesaran, 2015; Liddle and Hasanov, 2022). According to the Pesaran (2007) panel unit root test for heterogeneous panels (CIPS), some variables are non-stationary. Pesaran (2015) CD test shows that significant cross-sectional dependency exists in most variables. Therefore, we report cluster robust standard errors and test their cointegration relationship in the empirical analysis to address the cointegration and cross-sectional dependency issues.
Our study utilizes fixed effects panel regression models to estimate the impact of electric vehicle adoption on environmental outcomes. The fixed effects specification controls for unobserved heterogeneity across provinces that are time-invariant, such as geographical factors, resource endowments, or cultural differences. The fixed effects absorb these provincial differences so the models isolate the effects of predictors that change over time, like EV adoption and economic growth. Panel models with fixed effects are commonly used in policy evaluation and technology impact studies to control for time-invariant confounders and estimate causal relationships. The province and year fixed effects in our models account for unobserved provincial differences and temporal trends, allowing us to identify the influence of EV growth on emissions, energy, and air quality.
We first construct the following models to investigate the impact of EV holdings on energy consumption such as gasoline and coal.
where Energy refers to gasoline or coal consumption.
Then, we construct the following models to investigate how EV holdings affect carbon emissions.
where CO2 refers to total carbon dioxide emission, carbon dioxide emission from gasoline consumption or carbon dioxide emission from coal consumption. X is the vector of control variables. Here, the control variables are selected according to Wang et al. (2013) and Lin and Du (2015). Again
Last, we investigate the influence of EV holdings on air pollution by applying the following models
where air pollution is represented by air quality index (AQI), PM2.5 or PM10. X is the vector of control variables selected according to Xie et al. (2021) and He and Jiang (2021). The empirical analysis is conducted in Stata.
The procurement of electric vehicles is primarily dictated by consumer preferences and is independent of the variables of energy consumption, carbon emissions, and air pollution. Consequently, the analysis conducted in this study does not involve the contemplation of reverse causality, as the examined factors hold no discernible impact on the decision-making process regarding electric vehicle acquisition.
First, we examine the influence of EV holdings on energy consumption. Table 3 reports the results of Eqs 9, 10. To account for cross sectional dependency, cluster robust standard errors are reported. Clearly, we can observe that EV holdings have a significant negative impact on gasoline consumption and a positive impact on coal consumption, suggesting that the use of electric vehicles can significantly reduce gasoline consumption. However, since electricity mainly comes from thermal power generation, the use of electric vehicles significantly increased coal consumption. On the other hand, the fuel car holdings have no significant impact on gasoline consumption. That is because the Chinese government made great efforts to restrict the use of fuel cars. For example, license issuance for fuel car has been strictly restricted in Beijing and Shanghai, however, for electric vehicles, license issuance is unlimited with no license fee.
Then we replace the number of electronic and fuel cars by their ratios. We can observe that the EV/ICGV ratio has significant negative impact on gasoline consumption and positive impact on coal consumption. Totally, these results suggest that the use of EVs reduce the gasoline consumption, and thus reduce the reliance on oil import. However, EV cannot reduce the reliance on fossil energy. In addition, CIPS test shows that the residuals are stationary, suggesting the cointegration relationship holds.
Then, we examine the influence of EVs on carbon emissions. Table 4 represents these results from Eqs 11, 12. Obviously, the increase of EVs significantly reduced carbon emissions from gasoline consumption and increased carbon emissions from coal consumption. These results are in line with Table 3. In addition, the positive impact of EVs on carbon emissions from coal consumption is much larger than the negative impact on carbon emissions from gasoline consumption. As a consequence, we can observe that EV holdings significantly increased total carbon emission. That is because the production of EVs increased dramatically in recent years. In 2021, China sold over 3.5 million EVs, about 52% of the whole sales in the world. Although the increased carbon emissions from coal consumption by the use of EVs can be offset by the decrease of the use of fuel cars, the carbon emission in the production process of EVs still increased substantially. After replacing the number of EV and fuel car holdings by their ratio, similar results can be obtained. Totally, we can conclude that under the current stage, the development of EV industries does not help reducing carbon emissions.
Finally, we examine the influence of EV holdings on air pollution. Table 5 represents these results from Eqs 13, 14. Clearly, EVs has no significant impact on air quality index, PM2.5 and PM10. Given the fact that EV holdings would increase the consumption of coals, the air pollutants emission was transferred from the oil consumption to coal consumption. Thus, at the current stage, developing the EV industry cannot help mitigating air pollution.
In sum, we can conclude that the significant increase in electric vehicles help reducing the consumption of gasoline, and thus reduce the reliance on oil import. However, since the electricity is mainly generated by coal, the carbon emissions and air pollutants emissions are transferred from oil consumption to coal consumption. Therefore, the development of EV industry cannot help reducing carbon emissions and mitigating air pollution. Our empirical results suggest that unless the electricity industry decarbonized, the introduction of EVs would not contribute to air quality improvement and carbon emission reduction.
As concluded above, the primary reason that the development of the EV industry has not reduced carbon emissions and air pollutants emissions is the heavy reliance on thermal power generation. As a result, we investigate the regional heterogeneity of the influence of EV industry development based on thermal power reliance. Figure 3 shows the percentage of thermal power generation to total power generation in each province in 2021. We divide our sample into two groups—above and below the average level of thermal power reliance in China.
The findings of the heterogeneity study based on thermal power reliance are shown in Table 6. The EVs significantly cut gasoline consumption in Group A, which is heavily reliant on thermal power generation. However, coal consumption did not grow considerably. As a result, carbon emissions from gasoline use were greatly reduced, while overall carbon emissions and carbon emissions from coal consumption were not significantly raised. As a consequence, EVs has no discernible influence on air pollution.
On the other hand, EVs significantly reduced gasoline consumption and boosted coal consumption in group B, which relies less on thermal power generation. As a result, carbon emissions from gasoline consumption were greatly lowered, whereas overall carbon emissions and carbon emissions from coal consumption were significantly raised. In addition, the influence of the substantial increase in EVs on air pollutants emissions is limited.
These findings imply that coal consumption, carbon emissions, and air pollution emissions by industries are extremely high in regions that rely heavily on thermal power generation. Despite rapid growth in the EV sector in recent years, the quantity of coal consumed, carbon emissions, and air pollutants generated by the EV industry is fairly minor when compared to traditional heavy polluting industries. However, in regions where thermal power generation is less prevalent, basic coal consumption, carbon emissions, and air pollution emissions are comparatively low. As a result, the rapid development in EVs boosted coal consumption and carbon emissions in these locations. Because regions that rely less on thermal power do not suffer from severe pollution, the widespread use of electric vehicles could not considerably reduce air pollution.
In China, the disparities in economic growth and energy distribution among regions dictate the many linkages between electrical power generation and consumption. Electricity gaps (defined as electricity generation minus electricity consumption) are common in developed regions, although economically impoverished regions in the central and western regions usually generate extra electricity. As a result, the impact of promoting electric vehicles in certain regions will be determined by local power sources. If a region’s power supply is mostly provided by other provinces, an increase in electricity consumption caused by the promotion of electric vehicles in that region would have less of an impact on local carbon and air pollutants emissions, resulting in an increase in carbon and air pollutants emissions in electricity exporting provinces. Thus, based on net electricity import and export, we analyze the regional heterogeneity of the effect of EV sector growth. Figure 4 displays the difference between electricity generation and consumption in 2021. Our samples are divided into two categories: net electricity import regions and net electricity export regions.
The findings of the heterogeneity study based on net electricity import and export are presented in Table 7. Clearly, the growth of the EV sector has had no discernible influence on coal consumption or carbon emissions in net electricity import regions. However, the growth of the EV industry significantly increased overall carbon emissions and carbon emissions from coal consumption in net electricity export regions. These results indicate that China’s unequal distribution of electricity has a regional transfer effect on the impact of EV promotion on carbon emissions. The increased demand for electricity generated by the growth of EVs has also been transferred to electricity exporting areas. As a result, electricity exporting areas are forced to use thermal power to close the electricity imbalance in a short amount of time, increasing carbon emissions in exporting areas.
We develop a theoretical framework and empirically investigate the impacts of EV usage on energy consumption, carbon emission and air pollution. Our findings suggest that electric vehicles increase overall coal consumption and carbon emissions in China, contradicting evidence by Fuinhas et al. (2021) and Koengkan et al. (2022) that EVs can reduce greenhouse gas emissions in European countries. Our major findings are more consistent with prior research that finds negative environmental effects of EVs utilizing existing power generation methods (Wu et al., 2012; Ji et al., 2015; Holland et al., 2016; Su et al., 2021).
However, our research differ from these in several important respects. First, our study finds that switching to EVs increases carbon emissions but did not find evidence that switching to EVs would increase air pollution. Second, our study examines the heterogeneity in thermal power dependence and discovers that the provinces with low thermal power reliance (i.e., higher percentage of using clean energy in electricity generation) actually have experienced a significant increase in coal consumption and carbon emission from the usage of EVs. There are no significant changes in coal consumption and carbon emissions for provinces with high thermal power reliance, contrary to studies such as Holland et al. (2016), which find that switching from gasoline to electric vehicles is better in states with clean energy sources and worse in states that rely more on coals. Finally, our research employs the net electricity imports/exports categorization to characterize local electricity generating capability, which has not been comprehensively examined in earlier literature.
Our findings carry several important policy implications as China pursues its goals of peaking carbon emissions by 2030 and achieving carbon neutrality by 2060. First, the rapid growth of EVs driven by industrial promotion policies has not yet delivered proportionate emissions reductions due to increased coal power demand. This indicates that governments should adopt a policy mix that integrates the transition to EVs with broader decarbonization efforts across the economy, rather than a siloed approach. Policymakers should continue incentivizing EVs through subsidies, fuel economy regulation, charging infrastructure, and licensing preferences. But the fiscal and administrative support for EVs should be complemented with stronger measures to green the electric grid, improve efficiency across transport modes, expand public transit accessibility, implement carbon pricing mechanisms, and invest in natural climate solutions. A coordinated policy portfolio aligning EV adoption with power, industry, buildings, and land use emissions mitigation is imperative.
Second, our findings reveal the need to expand the metrics for evaluating the success of EV policies beyond just oil displacement. Targets and incentives for the EV industry should integrate indicators like life-cycle emissions, share of renewables in the EV charging mix, and emission intensity per kilometer travelled. This will encourage automakers and grid operators to maximize decarbonization outcomes as EVs are deployed. Transitioning the power supply for EVs and manufacturing toward low-carbon sources should become policy priorities.
Third, the regional spillover effects we find highlight the need for coordinated policymaking between provincial and central authorities. Local EV incentives should factor in grid capacity expansion needs and power trading dynamics to avoid unintended emissions increases in interconnected areas. Provinces with limited renewable potential should be prioritized for EV integration funding to avoid coal-based charging. Coordinating EV roadmaps between China’s regional power grids can address infrastructure and supply-demand imbalances.
Finally, the government has a vital signaling and convening role to play in aligning policies, technical standards, and industrial strategies between the EV and renewable energy sectors. The policy ecosystem should provide platforms for automakers, charging companies, battery manufacturers, generators, grid operators, and power suppliers to collaborate on maximizing the climate benefits of EVs throughout their life cycle.
Well-designed policies tailored to local contexts can enable China to simultaneously pursue the growth of its strategic EV industry and ambitious decarbonization agenda. Our study provides crucial policy insights for achieving the dual goals of EV leadership and sustainability leadership.
While this study provides valuable empirical evidence on the environmental impacts of electric vehicle adoption, it has certain limitations that present opportunities for future research.
Our analysis focuses solely on China given the rapid growth in EVs under state promotion policies. Expanding the empirical evaluation to other major EV markets such as Europe, the United States, and other developing economies such as India can identify commonalities and jurisdictional differences in sustainability outcomes. Comparative policy analyses enable knowledge sharing on optimal regulatory and industrial approaches for maximizing benefits.
We utilize provincial-level data which obscures intra-provincial differences across urban and rural areas. Future research could employ city-level data for a more granular analysis, especially comparing impacts across metropolitan areas with varying development levels, policy environments, and grid mixes. Studying distributional and spatial equity issues is vital.
Our study estimates observational relationships using provincial aggregate data rather than microdata with individual behavior. Future research could survey EV owners to gather primary data on charging patterns, electricity sourcing decisions, driving habits, and life cycle emissions to enrich understanding. Combining macro and micro perspectives provides analytical rigor and grounding.
We focus primarily on technological and environmental factors. Future work should adopt a broader socio-economic perspective to balance industrial development, energy transition, ecological sustainability, and social welfare goals within a systems framework. An interdisciplinary approach combining engineering, economics, sustainability science, and political economy could prove fruitful.
Finally, our analysis mainly employs panel regression techniques. Applying complementary methods like structural equation modeling, qualitative comparative analysis, and spatial econometrics could provide methodological triangulation and novel insights. Harnessing computational tools and big data analytics can also enable more sophisticated investigation of EV-environment linkages across spatial and temporal scales.
This study provides important empirical evidence on the linkage between electric vehicle adoption and environmental sustainability outcomes in China. While previous research has hypothesized this relationship using qualitative models and scenarios, our work utilizes recent panel data at the provincial level to quantify the impacts of surging EV uptake on energy consumption, emissions, and air pollution. This advances the field by establishing an evidence base during a crucial phase of EV expansion under major government promotion policies. Our findings can inform policy and industry strategies to align EV growth with climate goals.
Several key conclusions emerge from the analysis. First, the rapid adoption of EVs in China has significantly reduced gasoline consumption, lowering dependence on oil imports. However, the increased electricity demand has been primarily met through higher coal-based generation. As a result, the growth in EVs has shifted carbon emissions and air pollutants from the transportation sector to the power industry rather than yielding absolute reductions. This highlights the need for integrated sustainability metrics and policies that account for emissions across sectors and the full life cycle. The narrow focus on displacing oil understates the challenges of power sector decarbonization.
Second, our results reveal important heterogeneity based on regional power generation profiles. In provinces more reliant on renewable energy sources, the rise of EVs caused minimal increase in coal use and emissions. But in areas still dominated by coal power, the environmental impacts were significant. This indicates that transitioning the grid supply mix in tandem with increasing EV uptake is essential to maximize climate and air quality benefits. The policy emphasis needs to expand beyond just EV adoption targets and oil displacement.
Third, we find evidence of a spatial spillover effect due to unequal power trading patterns across provinces. Increased EV charging loads in net electricity importing regions transferred carbon emissions to exporting regions relying more heavily on coal generation. Managing these distributed effects requires coordination between national, provincial, and grid-level authorities when designing EV incentives and infrastructure policies. A systems perspective is imperative.
Overall, our findings indicate that the EV transition alone is insufficient to guarantee emissions reductions and environmental gains. Complementary efforts like proliferating renewable energy, modernizing grids, implementing carbon pricing, and investing in natural climate solutions are equally crucial. The policy mix should align industrial growth and technology incentives with sustainability objectives across sectors. The conclusions contribute timely empirical evidence and recommendations as China pursues its carbon peaking and neutrality goals.
Our study also reveals important avenues for future research. Assessing the holistic impacts of EVs across their life cycle from mineral extraction through production, use phase, and recycling can provide further insights into maximizing benefits. Evaluating EV policies and industry growth in other major markets such as Europe and the United States can identify jurisdictional best practices. Deepening the macroeconomic and fiscal policy analysis to balance industrial development, energy transition, and environmental sustainability priorities can inform integrated policy design. Expanding the empirical approach to incorporate projections and scenarios can improve understanding of long-term trajectories. Our findings and recommendations can help guide policy, industry strategy, and future scholarship to effectively align decarbonization and industrial transformation priorities. Managing this transition in a socially inclusive and environmentally sound manner is vital as countries pursue sustainable development.
The raw data supporting the conclusion of this article will be made available by the authors, without undue reservation.
PL: Data curation, Formal Analysis, Investigation, Methodology, Visualization, Writing–original draft, Conceptualization, Software. SH: Supervision, Validation, Writing–review and editing, Funding acquisition, Project administration. LS: Funding acquisition, Validation, Writing–review and editing. ST: Conceptualization, Formal Analysis, Investigation, Methodology, Project administration, Supervision, Validation, Writing–original draft.
The authors declare that no financial support was received for the research, authorship, and/or publication of this article.
The authors declare that the research was conducted in the absence of any commercial or financial relationships that could be construed as a potential conflict of interest.
The authors declared that they were an editorial board member of Frontiers, at the time of submission. This had no impact on the peer review process and the final decision.
All claims expressed in this article are solely those of the authors and do not necessarily represent those of their affiliated organizations, or those of the publisher, the editors and the reviewers. Any product that may be evaluated in this article, or claim that may be made by its manufacturer, is not guaranteed or endorsed by the publisher.
The Supplementary Material for this article can be found online at: https://www.frontiersin.org/articles/10.3389/fenvs.2023.1276382/full#supplementary-material
1The new energy vehicles refer to the vehicles powered by electricity in this context.
Ajanovic, A., and Haas, R. (2019). On the environmental benignity of electric vehicles. J. Sustain. Dev. Energy, Water Environ. Syst. 7, 416–431. doi:10.13044/j.sdewes.d6.0252
Baltagi, B. H., and Hashem Pesaran, M. (2007). Heterogeneity and cross section dependence in panel data models: theory and applications introduction. J. Appl. Econ. 22 (2), 229–232. doi:10.1002/jae.955
Beenstock, M., and Dalziel, A. (1986). The demand for energy in the UK. Energy Econ. 8, 90–98. doi:10.1016/0140-9883(86)90033-2
Chai, J., Wang, S., Wang, S., and Guo, J. E. (2012). Demand forecast of petroleum product consumption in the Chinese transportation industry. Energies 5 (3), 577–598. doi:10.3390/en5030577
Davis, L. W., and Kilian, L. (2011). Estimating the effect of a gasoline tax on carbon emissions. J. Appl. Econ. 26 (7), 1187–1214. doi:10.1002/jae.1156
Fuinhas, J. A., Koengkan, M., Leitão, N. C., Nwani, C., Uzuner, G., Dehdar, F., et al. (2021). Effect of battery electric vehicles on greenhouse gas emissions in 29 European union countries. Sustainability 13 (24), 13611. doi:10.3390/su132413611
Hao, H., Ou, X., Du, J., Wang, H., and Ouyang, M. (2014). China’s electric vehicle subsidy scheme: rationale and impacts. Energy Policy 73, 722–732. doi:10.1016/j.enpol.2014.05.022
Hao, H., Wang, S., Liu, Z., and Zhao, F. (2016). The impact of stepped fuel economy targets on automaker's light-weighting strategy: the China case. Energy 94, 755–765. doi:10.1016/j.energy.2015.11.051
Hasanov, F. J. (2021). Theoretical framework for industrial energy consumption revisited: the role of demographics. Energy Rep. 7, 2178–2200. doi:10.1016/j.egyr.2021.04.002
Hasanov, F. J., Khan, Z., Hussain, M., and Tufail, M. (2021). Theoretical framework for the carbon emissions effects of technological progress and renewable energy consumption. Sustain. Dev. 1–13. doi:10.1002/sd.2175
He, K., Huo, H., Zhang, Q., He, D., An, F., Wang, M., et al. (2005). Oil consumption and CO2 emissions in China's road transport: current status, future trends, and policy implications. Energy policy 33 (12), 1499–1507. doi:10.1016/j.enpol.2004.01.007
He, X., and Jiang, S. (2021). Effects of vehicle purchase restrictions on urban air quality: empirical study on cities in China. Energy Policy 148, 112001. doi:10.1016/j.enpol.2020.112001
Hofmann, J., Guan, D., Chalvatzis, K., and Huo, D. (2016). Assessment of electrical vehicles as a successful driver for reducing CO2 emissions in China. Appl. energy 184, 995–1003. doi:10.1016/j.apenergy.2016.06.042
Holland, S. P., Mansur, E. T., Muller, N. Z., and Yates, A. J. (2016). Are there environmental benefits from driving electric vehicles? The importance of local factors. Am. Econ. Rev. 106 (12), 3700–3729. doi:10.1257/aer.20150897
Holland, S. P., Mansur, E. T., and Yates, A. J. (2021). The electric vehicle transition and the economics of banning gasoline vehicles. Am. Econ. J. Econ. Policy 13 (3), 316–344. doi:10.1257/pol.20200120
Huo, H., Zhang, Q., Wang, M. Q., Streets, D. G., and He, K. (2010). Environmental implication of electric vehicles in China. Environ. Sci. Technol. 44, 4856–4861. doi:10.1021/es100520c
IEA (2019). Global EV outlook 2019. Paris: IEA. Available at: www.iea.org/publications/reports/globalevoutlook2019.
Ji, S., Cherry, C. R., Zhou, W., Sawhney, R., Wu, Y., Cai, S., et al. (2015). Environmental justice aspects of exposure to PM2.5 emissions from electric vehicle use in China. Environ. Sci. Technol. 49, 13912–13920. doi:10.1021/acs.est.5b04927
Jiang, H. D., Liu, L. J., Dong, K., and Fu, Y. W. (2022). How will sectoral coverage in the carbon trading system affect the total oil consumption in China? A CGE-based analysis. Energy Econ. 110, 105996. doi:10.1016/j.eneco.2022.105996
Kazemzadeh, E., Koengkan, M., and Fuinhas, J. A. (2022). Effect of battery-electric and plug-in hybrid electric vehicles on PM2.5 emissions in 29 European countries. Sustainability 14 (4), 2188. doi:10.3390/su14042188
Klier, T., and Linn, J. (2015). Using taxes to reduce carbon dioxide emissions rates of new passenger vehicles: evidence from France, Germany, and Sweden. Am. Econ. J. Econ. Policy 7 (1), 212–242. doi:10.1257/pol.20120256
Koengkan, M., Fuinhas, J. A., Teixeira, M., Kazemzadeh, E., Auza, A., Dehdar, F., et al. (2022). The capacity of battery-electric and plug-in hybrid electric vehicles to mitigate CO2 emissions: macroeconomic evidence from European union countries. World Electr. Veh. J. 13 (4), 58. doi:10.3390/wevj13040058
Li, R., and Leung, G. C. (2012). Coal consumption and economic growth in China. Energy policy 40, 438–443. doi:10.1016/j.enpol.2011.10.034
Liddle, B., and Hasanov, F. (2022). Industry electricity price and output elasticities for high-income and middle-income countries. Empir. Econ. 62 (3), 1293–1319. doi:10.1007/s00181-021-02053-z
Lin, B., and Du, K. (2015). Modeling the dynamics of carbon emission performance in China: a parametric Malmquist index approach. Energy Econ. 49, 550–557. doi:10.1016/j.eneco.2015.03.028
Liu, S., Li, H., Kun, W., Zhang, Z., and Wu, H. (2022). How do transportation influencing factors affect air pollutants from vehicles in China? Evidence from threshold effect. Sustainability 14 (15), 9402. doi:10.3390/su14159402
Ma, L., Fu, F., Li, Z., and Liu, P. (2012). Oil development in China: current status and future trends. Energy Policy 45, 43–53. doi:10.1016/j.enpol.2012.01.023
Mahmoud, M., Garnett, R., Ferguson, M., and Kanaroglou, P. (2016). Electric buses: a review of alternative powertrains. Renew. Sustain. Energy Rev. 62, 62673–62684. doi:10.1016/j.rser.2016.05.019
Oliver, H. H., Gallagher, K. S., Tian, D., and Zhang, J. (2009). China's fuel economy standards for passenger vehicles: rationale, policy process, and impacts. Energy Policy 37 (11), 4720–4729. doi:10.1016/j.enpol.2009.06.026
Pesaran, M. H. (2007). A simple panel unit root test in the presence of cross-section dependence. J. Appl. Econ. 22, 265–312. doi:10.1002/jae.951
Pesaran, M. H. (2015). Time series and panel data econometrics. Oxford, UK: Oxford University Press.
Schuitema, G., Anable, J., Skippon, S., and Kinnear, N. (2013). The role of instrumental, hedonic and symbolic attributes in the intention to adopt electric vehicles. Transp. Res. Part A Policy Pract. 48, 39–49. doi:10.1016/j.tra.2012.10.004
Su, C. W., Yuan, X., Tao, R., and Umar, M. (2021). Can new energy vehicles help to achieve carbon neutrality targets? J. Environ. Manag. 297, 113348. doi:10.1016/j.jenvman.2021.113348
Wang, Q. W., Zhou, P., Shen, N., and Wang, S. S. (2013). Measuring carbon dioxide emission performance in Chinese provinces: a parametric approach. Renew. Sustain. Energy Rev. 21, 324–330. doi:10.1016/j.rser.2012.12.061
Wang, X., Bai, M., and Xie, C. (2019). Investigating CO2 mitigation potentials and the impact of oil price distortion in China's transport sector. Energy Policy 130, 320–327. doi:10.1016/j.enpol.2019.04.003
Wu, Y., Yang, Z., Lin, B., Liu, H., Wang, R., Zhou, B., et al. (2012). Energy consumption and CO2 emission impacts of vehicle electrification in three developed regions of China. Energy Policy 48, 537–550. doi:10.1016/j.enpol.2012.05.060
Wu, Y. A., Ng, A. W., Yu, Z., Huang, J., Meng, K., and Dong, Z. Y. (2021). A review of evolutionary policy incentives for sustainable development of electric vehicles in China: strategic implications. Energy Policy 148, 111983. doi:10.1016/j.enpol.2020.111983
Xie, Y., Wu, D., and Zhu, S. (2021). Can new energy vehicles subsidy curb the urban air pollution? Empirical evidence from pilot cities in China. Sci. Total Environ. 754, 142232. doi:10.1016/j.scitotenv.2020.142232
Xu, B., Sharif, A., Shahbaz, M., and Dong, K. (2021). Have electric vehicles effectively addressed CO2 emissions? Analysis of eight leading countries using quantile-on-quantile regression approach. Sustainable Production and Consumption 27, 1205–1214.
Yu, Y., Xu, H., Cheng, J., Wan, F., Ju, L., Liu, Q., et al. (2022). Which type of electric vehicle is worth promoting mostly in the context of carbon peaking and carbon neutrality? A case study for a metropolis in China. Sci. Total Environ. 837, 155626. doi:10.1016/j.scitotenv.2022.155626
Zhang, X., Liang, Y., Yu, E., Rao, R., and Xie, J. (2017). Review of electric vehicle policies in China: content summary and effect analysis. Renew. Sustain. Energy Rev. 70, 698–714. doi:10.1016/j.rser.2016.11.250
Zhao, M., and Sun, T. (2022). Dynamic spatial spillover effect of new energy vehicle industry policies on carbon emission of transportation sector in China. Energy Policy 165, 112991. doi:10.1016/j.enpol.2022.112991
Keywords: electric vehicle, energy consumption, carbon emission, air pollution, sustainable development goals
Citation: Lu P, Hamori S, Sun L and Tian S (2024) Does the electric vehicle industry help achieve sustainable development goals?—evidence from China. Front. Environ. Sci. 11:1276382. doi: 10.3389/fenvs.2023.1276382
Received: 11 August 2023; Accepted: 14 December 2023;
Published: 09 January 2024.
Edited by:
Fakhri J. Hasanov, King Abdullah Petroleum Studies and Research Center (KAPSARC), Saudi ArabiaReviewed by:
Matheus Koengkan, University of Aveiro, PortugalCopyright © 2024 Lu, Hamori, Sun and Tian. This is an open-access article distributed under the terms of the Creative Commons Attribution License (CC BY). The use, distribution or reproduction in other forums is permitted, provided the original author(s) and the copyright owner(s) are credited and that the original publication in this journal is cited, in accordance with accepted academic practice. No use, distribution or reproduction is permitted which does not comply with these terms.
*Correspondence: Li Sun, c3VubGlAc2JzLmVkdS5jbg==; Shuairu Tian, dGlhbnNyQHNicy5lZHUuY24=
Disclaimer: All claims expressed in this article are solely those of the authors and do not necessarily represent those of their affiliated organizations, or those of the publisher, the editors and the reviewers. Any product that may be evaluated in this article or claim that may be made by its manufacturer is not guaranteed or endorsed by the publisher.
Research integrity at Frontiers
Learn more about the work of our research integrity team to safeguard the quality of each article we publish.