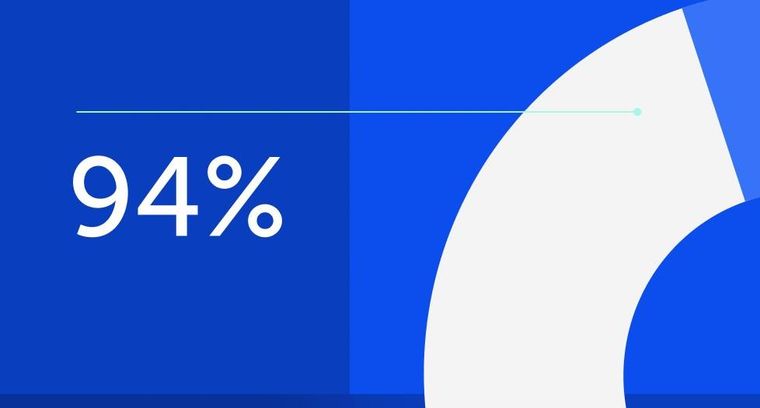
94% of researchers rate our articles as excellent or good
Learn more about the work of our research integrity team to safeguard the quality of each article we publish.
Find out more
ORIGINAL RESEARCH article
Front. Environ. Sci., 20 November 2023
Sec. Toxicology, Pollution and the Environment
Volume 11 - 2023 | https://doi.org/10.3389/fenvs.2023.1274997
This article is part of the Research TopicLegacy & Emerging Contaminants in the Aquatic EnvironmentView all 12 articles
Hexabromocyclododecanes (HBCDs), as brominated flame retardants, have increasingly drawn concern due to their detection in various marine organisms in recent years. The present test investigated the selective accumulation, depuration, and bioisomerization of HBCDs in different tissues and organs of Nibea albiflora, as well as the genetic metabolic behavior of HBCDs between parents and offspring. In a semi-static water environment, N. albiflora were exposed to individual HBCD diastereoisomers (α-HBCD, β-HBCD, or γ-HBCD) at a concentration of 0.4 μg/L for bioaccumulation, followed by clean food for depuration. During the exposure period, the highest concentrations of these HBCDs were observed in the intestine and gill of N. albiflora. α-HBCD was detected in all exposure groups, suggesting that both β-HBCD and γ-HBCD could be bioisomerized to α-HBCD in N. albiflora. In addition, there was no bioisomerization of α-HBCD to either β-HBCD or γ-HBCD, and there was no bioisomerization between β-HBCD and γ-HBCD. The half-lives (t1/2) were variable among different diastereoisomers, following an order of α-HBCD > γ-HBCD > β-HBCD. The most rapid depuration rate of HBCDs was discovered in the liver while muscle showed the least. Additionally, prior to spawning, the parent fish were exposed to contaminated feed (silkworms sprayed with 200 μL of a 30 μg/mL HBCD solution) for 6 days. By comparing the HBCDs concentrations in the maternal gonad, fertilized eggs, hatching eggs, larvae, and juveniles, it was determined that the maternal transfer coefficients for HBCDs were approximately 1, indicating dynamic equilibrium of HBCDs throughout the process from maternal tissue to progeny development. Differences in HBCDs pattern between organs observed in the experiment support a proposal of an organ-specific diastereomer accumulation.
Hexabromocyclododecanes (HBCDs), as non-bonded additive brominated flame retardants (BFRs), have been extensively utilized in construction, textiles, and electronic products due to their effective flame-retardant properties and minimal impact on material properties (Lopes and Elisa, 2023). During the lifecycle phases (i.e., production, application, transportation, or disposal) of these products, HBCDs can be easily released into the environment. In recent years, HBCDs have been widely detected in environmental (e.g., soil, water, and offshore sediments) (Goto et al., 2020; Pan et al., 2022; Wu et al., 2023), as well as in organisms (e.g., birds, aquatic animals, plants, and humans) (Fournier et al., 2012; Lu et al., 2019; Feiteiro et al., 2021; Tavoloni et al., 2021). HBCDs have even been detected in remote regions such as the South Pole and Arctic (Koch et al., 2015; Kim et al., 2018). Due to their long-distance transport, persistence, bioaccumulation and toxicity, HBCDs were included as persistent organic pollutants on the Stockholm Convention Persistent Organic Pollutants (POPs) list in May 2013. The widespread distribution of HBCDs in marine organisms has attracted great concerns, due to their adverse impacts on marine organisms and human health (Zhang et al., 2022; Ghzela et al., 2023).
The lipophilic and hydrophobic properties of HBCDs facilitates their bioaccumulation within organisms. Furthermore, HBCDs accumulated mainly in adipose tissues, with annual increments observed (Ruan et al., 2018). A wide detection spectrum of HBCDs in fish along China’s coastline revealed concentrations spanning from non-detectable levels to 2,970 ng/g lw (Zhang et al., 2022). Concentrations in Neophocaena phocaenoides and Sousa chinensis in China’s South Sea were significantly elevated compared to their environment. The accumulation of HBCDs in fish tissues followed the order: fat > liver > gill > muscle, exhibiting a positive correlation with tissue lipid content (Tang et al., 2015). Such observations underscore HBCDs’ proclivity for organismal fat layers. These compounds pose degradation challenges for organisms and can inflict extensive effects on various biota, including humans. HBCDs have been associated with toxic effects affecting various physiological systems, including the liver, nerves, and thyroid, as well as the immune, endocrine, and reproductive systems (Darnerud, 2003; Lilienthal et al., 2009; Koch et al., 2015). While previous research primarily examined the exposure to a mix of HBCDs, it is actually divided into many isomers, each with distinct properties. HBCDs exhibit isomer-selective bioaccumulation, metabolic and toxic effects. Therefore, a more nuanced analysis of individual HBCD isomers is warranted for a thorough and precise ecological risk assessment in environmental contexts.
Among the 16 possible stereoisomers, the HBCDs products contain predominantly three isomers: γ-(75–89%), α-(10–13%) and β-HBCD (1–12%) (Tavoloni et al., 2020). In addition, industrial HBCDs also contain slight amounts of δ-HBCD and ε-HBCD (Zoeller, 2012). The polarity, dipole moment, and water solubility of the three main isomers differ, resulting in variable environmental stability and biological uptake rates (Oh et al., 2014). The octanol-water partition coefficients (log Kow) of α-, β-, and γ-HBCD at 25°C were 5.59, 5.44, and 5.53, respectively (Gerecke et al., 2006; Goss et al., 2008; Oh et al., 2014), indicating a strong predisposition for these compounds to accumulate in organisms. In particular, α-HBCD has been identified as a preferred enantiomer in various species (Ruan et al., 2018; Ruan et al., 2018; Zhang et al., 2018), with its enantioselectivity varying among organisms (Zhang et al., 2014; Huang et al., 2017). It has been reported that γ- and β-HBCD may not bioaccumulate (Zhang et al., 2018). Brandon et al. (2020) notably observed that shrimp fed with whiteflies containing HBCDs exhibited neither enrichment nor accumulation but maintained a metabolic equilibrium. However, in-depth studies on the causes of selective enrichment are lacking.
Given the distinct behaviors of HBCDs in biota accumulation, disposition, and effects, understanding the mechanisms behind these processes is crucial for both regulatory and toxicological considerations. The selective enrichment of HBCD stereoisomers in environmental and biological samples, results from multiple factors. These include differences in exposure sources, water solubility of isomers, bioavailability, selective absorption, organs excreting the compound, biological metabolic rates, and selective conversion of stereoisomers within organisms. These factors must be comprehensively considered in the ecological hazard assessments of HBCDs, along with accurate qualitative and quantitative analyses of their stereoisomers. To understand the selective enrichment, transformation, and distribution mechanism of HBCDs in different tissues and organs of N. albiflora, as well as its genetic metabolic behavior between parents and offsprings, controlled laboratory accumulation studies are necessary. In the present study, N. albiflora were exposed to individual compounds in water and purification experiments were conducted. Additionally, parental exposure experiments using HBCD-containing feed were also conducted. The diastereomer-specific bioaccumulation, distribution, metabolic behavior, and mechanisms of each HBCD isomer in various organs or tissues of N. albiflora (including blood, gonad, liver, intestine, gallbladder, spleen, kidney, gill, brain, and muscle) were assessed, as well as the maternal transfer capability of HBCDs.
α-HBCD (CAS 134237-50-6), β-HBCD (CAS 134237-51-7) and γ-HBCD (CAS 134237-52-8), as 100.8 μg/mL in toluene (1 mL), were purchased from AccuStandard, Inc. (USA). HBCD (unequal mix of 3 isomers of 13C12, 99%, 50 μg/mL in toluene, 1.2 mL, CAS 25637-99-4) was purchased from Cambridge Isotope Laboratories, Inc. (United States). n-Hexane (CAS 110-54-3), acetone (CAS 67-64-1), acetonitrile (CAS 75-05-8), and anhydrous sodium sulfate (CAS 7757-82-6) were purchased from ANPEL Laboratory Technologies Inc. (Shanghai, China). Dichloromethane (CAS 75-09-2) was purchased from J. T. Baker (United States). Methanol (CAS 67-56-1) was purchased from Merck. (Germany). Concentrated sulfuric acid (95.0%–98.0%, CAS 7664-93-9) was purchased from Sinopharm Chemical Reagent Co., Ltd. (Shanghai, China).
HBCDs were pre-equilibrated at designated concentrations to eliminate any potential adsorption to the walls of fiberglass tanks. In a preliminary trial, N. albiflora were exposed to pollutants at concentrations of 0, 0.2, and 0.4 μg/L. A discernible accumulation in the organisms was observed at the 0.4 μg/L concentration within a brief duration. Notably, previous research reported an ambient HBCD concentration of 0.35 ± 0.06 ng/L in seawater from aquaculture areas (Pan et al., 2023). The exposure level of 0.4 μg/L was selected based on the concentration of HBCDs in natural environment, aiming to facilitate discernible accumulation of HBCD isomers within a short time. N. albiflora without obvious diseases and deformities were randomly selected for the investigation. After being acclimated for over 2 weeks, sixty adult N. albiflora were randomly divided into four groups, including three experimental groups and one control group. N. albiflora were exposed to 0.4 μg/L of α-HBCD, β-HBCD, or γ-HBCD using the semi-static water exposure method. Specifically, 50% of the water containing a single compound was renewed every day to maintain each isomer concentration. Acetone was used as the control. Fish were fed an amount equivalent to 1% of their body weight daily at a fixed time. The exposure period lasted 6 d. The exposure test lasted for 6 d followed by 9 d of depuration test. The depuration test was conducted in seawater without HBCDs. During the experiment, the tanks remained under the same conditions as acclimation process. Sufficient oxygen was provided, with a constant temperature at 24°C ± 1°C throughout the experiment. The health and behavior of the experimental fish were monitored daily.
Samples from various tissues and organs were collected at 1, 3, and 6 d post-exposure, and days 4 and 9 post-depuration. At each sampling time, 2 N. albiflora specimens were randomly selected from each tank to make up pooled samples, ensuring a representative analysis. After washing and removing surface moisture, the specimens were dissected to collect samples from different tissues and organs, including blood, liver, intestine, spleen, kidney, gallbladder, gill, brain, muscle, and gonad. These samples were sealed in glass containers and frozen at −20°C until HBCD chemical analysis.
Initially, the N. albiflora were cultivated in earthen ponds where they were fed a mixture of small fish, after which, they were transferred indoors for overwintering and given frozen squid as bait. The ponds floors were cleaned daily to maintain hygiene. During the pre-oviposition period, female and male brood fish were segregated and fed separately. They were fed with uncontaminated silkworms in the morning and with silkworms contaminated (sprayed with the 200 μL of 30 μg/mL HBCDs) in the afternoon. The exposure continued for 6 d. During this period, special attention was given to the brood N. albiflora, with emphasis on tracking their gonadal development. After gonadal maturation, males and females were transferred to seawater without HBCDs and allowed to interbreed, concentrating on spawning. Normal eggs were subsequently selected, counted, and hatched in fresh seawater. Both brood fish and fertilized eggs were utilized in subsequent analysis.
Fertilized eggs were incubated in standing water at 26°C, then reared in static water with micro-aeration at 22°C–25°C. After an embryonic development of 20–36 h, larval were separated from the membranes. From 3 to 4 d post-hatching, they were fed rotifers as opening bait for 8–10 d. Feeding occurred at 4-h intervals, with quantities adjusted according to consumption. Water quality was monitored daily, and any remaining membrane were removed if necessary. Following the rotifer-feeding period, water was renewed and maintained at a depth of 5 cm. By 14 d post-hatching, larvae grew to approximately 10 mm, with morphology and organs development resembling parent fish, marking their transition into the juvenile phase. Samples were collected and analyzed at 0 d (fertilized eggs), 1 d (hatching eggs), 3 d (larval fish, 2.84 mm), 10d (larval fish, ∼5 mm) and 14 d (juvenile fish, ∼10 mm).
After freeze-drying, 2.00 g of the homogenized sample was accurately weighed into a 50 mL centrifuge tube (Polypropylene, Corning Life Sciences (Wujiang) Co., Ltd.) with 2.0 g of anhydrous sodium sulfate to enhance sample dispersion and improve extraction efficiency. Subsequently, 100 μL of internal standard solution (mixed internal standard solution of 13C-α-HBCD, 13C-β-HBCD, and 13C-γ-HBCD with a mass concentration of 100 ng/mL) was added. A total of 30 mL of acetone/n-hexane (1:1, v/v) was added to the centrifuge tube, which were completely mixed by shaking for 2 min. After that, the tubes were ultrasonicated for 10 min, followed by centrifugation at 5,000 rpm for 5 min to separate the supernatant. The supernatant was then transferred to a rotary evaporator. The residue was re-extracted with the above-mentioned method. All separated supernatants were combined and reduced to dryness at 40°C using the vacuum-rotary evaporation. The residue was reconstituted with 10 mL of n-hexane and transferred to a centrifuge tube for sulfonation with 10 mL of concentrated sulfuric acid (China National Pharmaceutical Group, Beijing, China). The mixture was shaken and separated by centrifugation at 5,000 rpm for 5 min. The supernatant was transferred to a rotary evaporator. The lower layer was re-extracted with 10 mL of n-hexane. The whole organic extract was concentrated under reduced pressure in a vacuum concentrator to a volume of 1 mL of hexane for the cleanup step.
Sample cleanup procedure was performed using solid phase extraction (SPE). The SPE cartridges (silica, 1 g/6 mL, ANPEL Laboratory Technologies Inc., Shanghai, China) were previously conditioned with 5 mL of n-hexane. After loading the extracts, cartridges were cleaned with 1 mL of n-hexane. The HBCDs retained in the cartridges were eluted with 10 mL of a mixture n-hexane and dichloromethane (1:3, v/v). The eluent obtained from the cartridge was evaporated to dryness, and then the residue was redissolved in 1 mL of methanol, subsequently filtrated with a 0.22 μm organic phase membrane filter (13 mm × 0.22 μm, ANPEL Laboratory Technologies Inc., Shanghai, China) and transferred to an injection vial prior to ultra performance liquid chromatography-triple quadrupole tandem mass (UPLC-QqQ-MS/MS) analysis.
The separation and quantification of HBCDs were performed by UPLC-QqQ-MS/MS (Acquity-Quattro Premier XE, Waters, United States) with electrospray ionization. The mobile phase consisted of ultra-pure Milli-Q (with a resistivity of 18.2 MΩ cm) and methanol-acetonitrile (4:6, v/v). The composition and ramp of mobile phase were listed in Table 1. The separation of HBCDs in the extracts was performed on a BEH C18 column (100 mm × 2.1 mm × 1.7 μm, Waters, United States) with a mobile phase flow rate of 0.3 mL/min at 40°C. The injection volume was 10 μL. Electrospray ionization was performed in the negative ion mode. The identification and quantitation of HBCDs were performed using multiple reaction monitoring mode (MRM). The parent ions, daughter ions, and collision voltages were listed in Table 2. Specifically, the ion pair m/z 640.4-80.9 was monitored for quantitation, while the ion pair m/z 640.4-78.9 was monitored for qualitative analysis. The labeled HBCD isomers were identified using the ion pair m/z 652.3-78.9.
The HBCDs mixed standard solution was serially diluted with methanol to prepare six concentrations (0, 1.00, 5.00, 10.0, 20.0, and 50.0 ng/mL) of standard solutions. The concentration of the internal standard was set at 10 ng/mL. A standard curve was plotted based on these standard solutions. The correlation coefficients (r2) of all three isomers exceeded 0.99. The limit of quantitation (LOD) was calculated based on a 10-fold signal-to-noise ratio and was established as 0.20 μg/kg for the UPLC-MS/MS analytical method. Blanks and samples were analyzed concurrently, and no analytes were detected in any blank samples. At three spiked concentration levels of 0.5, 2.5, and 10 μg/kg, the recovery rate for HBCDs was 85.8%–97.3%, and the relative standard deviation was less than 9.10%. This method demonstrated good recovery, high accuracy, and excellent precision, fulfilling the requirements for subsequent experimental analyses.
A first-order kinetic model was used to fit the purification-stage data (Geng et al., 2019):
Purification half-lives (Li et al., 2017):
where, Ct is the analyte concentration in N. albiflora at time t, C0 is the analyte concentration in N. albiflora at time 0 of purification, and k is the purification rate constant, which also corresponds to the slope of regression curve.
Statistical analyses were performed using SPSS (Statistical Package for the Social Sciences; v 24, IBM). Kruskal–Wallis H tests and one-way analyses of variance were used to determine statistical significance. The criterion for statistical significance was set at p < 0.05 throughout the study.
Figure 1 shows the concentrations of α-, β-, and γ-HBCD in various tissues from N. albiflora following exposures of 3 and 6 days. These variations underscore the differential impacts of pollution sources and exposure durations on the accumulation of these compounds, as suggested by Bertucci et al. (2020). The HBCDs accumulated with time in all tissues (except the β- and γ-HBCD-treated group in gallbladder), though the rates were inconsistent. Exposed to α-HBCD for 3 d, the highest concentration were recorded in the intestine (125.20 ng/g dw) and gill (65.43 ng/g dw), whereas the lowest being in the brain. Following 6 d of exposure to α-HBCD, the concentrations of α-HBCD in the intestine, gill, and gonad increased significantly to 316.10, 294.90, and 194.9 ng/g dw, respectively, with the lowest concentration at this juncture was in the gallbladder (24.00 ng/g dw). After β-HBCD exposure for 3 d, the highest concentrations were observed in the intestine (65.93 ng/g dw) and gill (51.57 ng/g dw). In contrast, β-HBCD was undetectable in brain and kidney, whereas α-HBCD was detected, indicating a bioisomerization of β-HBCD to α-HBCD. After 6 d of exposure to β-HBCD, the concentrations in the intestine (137.85 ng/g dw) and gill (85.20 ng/g dw) were notably higher than in other tissues, with the gallbladder (5.71 ng/g dw) showing the lowest. Additionally, α-HBCD was detected in the kidney, intestine, and liver, indicating that β-HBCD was metabolized into α-HBCD in these tissues to varying degrees. Regarding γ-HBCD exposure at 3 d, its concentrations peaked in the intestine (60.65 ng/g dw) and gill (46.16 ng/g dw), while the spleen (13.67 ng/g dw) and kidney (17.22 ng/g dw) recorded the lowest concentrations. Interestingly, α-HBCD was identified in the brain, indicating that γ-HBCD was metabolized into α-HBCD. By the 6th day, the concentrations of γ-HBCD were more pronounced in the intestine (277.80 ng/g dw) and kidney (81.26 ng/g dw) compared to other tissues, with the gallbladder (1.40 ng/g dw) showing the lowest concentration. Furthermore, α-HBCD was detected in the brain, intestine, and gonad, indicating that γ-HBCD was metabolized into α-HBCD to varying degrees in these tissues. It may be attributed to conditions within the organism and the influence of thermal factors (Koeppen et al., 2008; Szabo et al., 2010). In summary, it is notable that α-HBCD was detected in all exposure groups, whereas no other diastereomers were found in the group spiked with β- or γ-HBCD only. Throughout the exposure, a bioisomerization of β- and γ-HBCD to α-HBCD was observed, whereas no isomerization of α-HBCD to β- or γ-HBCD was noted, aligning with previous studies (Law et al., 2006; Zhang et al., 2016; Sun et al., 2018).
FIGURE 1. Distribution characteristics and selective enrichment of α-HBCD, β-HBCD, and γ-HBCD in the tissues/organs of N. albiflora. Note: HBCD, hexabromocyclododecane; DW, dry weight.
On the 3rd and 6th days post-exposure, the total concentrations of the HBCD isomers were compared across the three exposure groups. α-HBCD emerged as the dominant isomer in N. albiflora, with its concentration significantly higher those of β- and γ-HBCD (p < 0.01). The results further support the proposal of selective bioaccumulation of HBCD diastereoisomers. Likewise, Du et al. (Du M et al., 2012) observed the highest accumulation of α-HBCD in their experiments with zebrafish. A similar selective enrichment was also reported by Wang et al. (Wang et al., 2021) in their studies on human cells, attributing to the high affinity of efflux protein P-gp. Beyond binding affinity, transcriptomic expression patterns, protein levels, and enzymatic activities are paramount in influencing HBCD metabolism and transport within organisms. Further molecular studies are required to elucidate these underlying mechanisms.
Regarding tissue enrichment patterns, all exposure groups exhibited the highest concentrations in the intestine and gill. The distribution variability across different tissues could be attributed to both dynamic and thermodynamic factors. From a dynamic standpoint, intestine and gill have a heightened exposure to chemicals in water. Chemicals predominantly enter the intestine via ingestion or the gill via respiration, subsequently reaching various tissues and organs via the bloodstream. From a thermodynamic standpoint, the elevated lipid content in gill and intestine might account for the pronounced accumulation of hydrophobic chemicals within these tissues (Zhang et al., 2014). Similarly, Sun et al. (2009) found that the bioaccumulation of arsenate occurred through gill and intestinal uptake. In Cyprinus carpio, the sequence for HBCDs accumulation in intake tissues as intestine > gill > skin and distributed them as adipose > liver > brain > muscle (Zhang et al., 2016). Li et al. (2017) consistently observed tissues concentrations in Eisenia fetida following the order of intestine > body fluid > body wall. The gill and intestine emerge as critical interfaces of aquatic contaminant exposure on fish.
Compared to the concentrations observed on the 3rd day post-exposure, the concentration of HBCDs in the intestine increased noticeably by day 6. In addition to lipid partitioning, which is the principal mechanism underlying the bioaccumulation of HBCDs (Shin et al., 2020; Hou et al., 2022), inter-tissue- and organ-specific metabolic variations also modulate pollutant enrichment within an organism (Wang et al., 2020). We hypothesized that HBCDs, similar to other highly lipophilic compounds, are incorporated into lipoproteins during cellular assembly within intestinal cells. They are then absorbed by chylomicrons, which influences their free concentration in vivo. Since most of these compounds are chelated, their bioavailability to metabolic enzymes diminishes (Jandacek et al., 2009). In the present study, on the 3rd day of α-HBCD exposure, the detected concentration of α-HBCD in the brain at a mere 0.626 ng/g, surging to 210.10 ng/g by the 6th day. Concentrations of HBCDs in the brain also showed an increasing trend in the β-HBCD- and γ-HBCD-exposure groups. These findings suggest that HBCDs possess the capability to traverse the blood-brain barrier and accumulate within the brain (Zhang et al., 2016), warranting further research.
Consistent with the findings of previous research (Susanne et al., 2011), we observed that the purification curve in each tissue or organ largely adhered to first-order kinetics. The k and t1/2 for α-HBCD, β-HBCD, and γ-HBCD, calculated from the fit of the experimental data to Eqs 1, 2, are detailed in Table 3.
The values of t1/2 for α-HBCD, β-HBCD and γ-HBCD were in the range of 12.36–29.62, 5.27–11.67, and 11.46–16.06 respectively, and the values of k were 0.0234–0.0561, 0.0594–0.1316, and 0.0431–0.0605 respectively. Therefore, α-HBCD persisted the longest within N. albiflora, while β-HBCD and γ-HBCD metabolized quicker. This is aligns with findings in both biota and liver cells, where α-HBCD exhibited the longest half-life (Gerecke et al., 2006; Huang et al., 2016). This finding supports the notion that α-HBCD is the dominant isomer accumulating within marine fish species. The distinct physicochemical properties of the three HBCD diastereomers may have contributed to the varying depuration capabilities of N. albiflora. Previous isomer metabolism experiments have revealed variations in the metabolic pathways of different diastereomers. For instance, α-HBCD mainly undergoes oxidation without evidence of debromination or stereoisomerization. In contrast, β-HBCD and γ-HBCD have exhibited multiple metabolic pathways, including stereoisomerization, oxidation, dehydrogenation, reductive debromination, and ring-opening (Szabo et al., 2010; Taylor et al., 2011; Heldur, 2016). These metabolic differences make α-HBCD the predominant isomer within biological tissues, as it is the most resistant to metabolism and accumulates through the stereoisomerization of the β- and γ-HBCD diastereomers. This observation is further substantiated by marine sample analyses (N et al., 2005; Zhang et al., 2018). Compared to β-HBCD and γ-HBCD, α-HBCD exhibits greater resistance to metabolic degradation and transformation within biological systems (Zhang et al., 2014). The results of single-dose exposure experiments in rodents, specifically involving α-HBCD and γ-HBCD, demonstrated distinct metabolic pathways for these two diastereomers, and the extent of metabolism of γ-HBCD substantially surpassed that of α-HBCD. Research on the biometabolites of HBCDs in rats and mice has shown that HBCDs can activate Phase I and II metabolic enzyme systems, particularly the cytochrome P450 enzyme system (CYP2B and CYP3A). These enzyme systems, which are involved in pollutant degradation, facilitate the debromination or hydroxylation of HBCDs. This leads to cumulative or magnified toxicological effects (Zegers et al., 2005; Germer et al., 2006; Hakk et al., 2012). Additionally, in contrast to the isomer depuration rates found in both zebrafish and human HepG2 cells (α-HBCD < β-HBCD < γ-HBCD) (Du et al., 2012; Wang et al., 2021), we observed the depuration rate for β-HBCD to be greater than that for γ-HBCD in N. albiflora. It is noteworthy that Zhang et al. (2016) found no conversion between HBCD diastereomers in incubation experiments with chicken liver microsome. Therefore, the ability of HBCD diastereomers to be metabolized varies between organisms. These suggest metabolic variability between organisms due to the unique interplay of metabolic enzymes and transport proteins.
In this study, marked differences were observed in the k and t1/2 values across different organs. The order of k for α-, β-, and γ-HBCD in different tissues was the same, liver > gonad > muscle. Specifically, the liver showed the highest depuration rate, supporting its role as the primary site of HBCDs metabolism (Topić Popović et al., 2023). Similar observations were made in experiments where E. fetida was exposed to soil containing HBCDs. For β-HBCD and γ-HBCD, the elimination rate constant (ke) values were found to be in the sequence of intestine > body fluid > body wall, whereas for α-HBCD, the ke values followed the order of body wall > intestine > body fluid (Li et al., 2017). Furthermore, Zhang et al. (2014) also reported the varying k2 values of HBCDs across tissues of C. carpio following aquatic exposure. Therefore, the tissue distribution of chemicals is influenced by multiple factors, encompassing both kinetic aspects and the properties of the tissues. In the context of HBCDs, this distribution becomes even more intricate due to the distinct metabolic and isomerization tendencies of the three diastereomers across various tissues.
Maternal gonads, fertilized eggs and fry were used to evaluate the transmissibility of HBCDs to offspring. By analyzing the contaminant concentration and isomer composition across the three maternal tissues (i.e., gonads, liver, and muscle), fertilized eggs, and fish fry, selecting the most representative tissue to evaluate maternal transfer. We found that the concentrations and isomer characteristics of gonads align more closely with fertilized eggs than with the liver and muscle. Therefore, maternal gonads were selected to represent maternal concentrations during the transfer assessment. In oviparous organisms, should organic pollutants maintain an equilibrium distribution within the body throughout the egg-laying process, the maternal transfer coefficient–defined as the ratio of pollutant concentration in the egg to that in the maternal tissue–would consistently equate to 1 (Ross et al., 2008). Intriguingly, the maternal transfer coefficient for α-, β-, and γ-HBCD did not follow a discernible trend, which might due to isomer transformations during biological processes. Therefore, analyzing the total concentration of compounds, rather than focusing solely on isomerism, provides a more accurate evaluation of the progeny transfer ability. The maternal transfer coefficient for HBCDs was determined by calculating the ratio of the total concentration of HBCDs across various developmental stages in the progeny to that in the gonads. As depicted in Figure 2, the maternal transfer coefficients of the total HBCDs were approximately 1, which indicates that HBCDs were balanced during the transfer process from the maternal tissue to the progeny and the development of the progeny.
The HBCD isomer composition remained consistent in maternal gonads, as well as fertilized and hatching eggs, indicating that the accumulation pattern of HBCDs remain static during the formation and development of fertilized eggs. Similarly, the concentrations and characteristics of the diastereomers were consistent between larvae and juveniles, suggesting a stable HBCD accumulation pattern across these developmental stages. However, transformations were observed among HBCD diastereomers during the transition from fertilized eggs to larvae and juveniles. The compositional patterns of diastereomers in maternal gonads, fertilized eggs, and various developmental stages are illustrated in Figure 3. Specifically, during the stages spanning maternal gonad to hatching eggs (gonad–1 d), the α-HBCD dominated with a concentration of approximately 45%. This was followed by β-HBCDs (∼40%) and γ-HBCD (∼15%). However, the composition changed dramatically during the larval to juvenile stage (3–14d). Here, α-HBCD accounted for only ∼15% of the total, being the least prevalent, β-HBCDs at around 10%. Notably, γ-HBCD emerged as the dominant isomer, comprising roughly 75% of the overall HBCDs.
FIGURE 3. Composition of HBCD diastereomers at different developmental stages of the N. albiflora. Note: (A), gonad–1d (gonad, fertilized eggs, eggs hatched for 1d); (B), 3–14d (larvae and juveniles).
By comparing the characteristics of diastereomers in maternal gonads, fertilized eggs, and fry, we found that a transformation occurred from α-HBCD and β-HBCD to γ-HBCD during the developmental transition from embryos to larvae and subsequently to juveniles. This observation challenges the conventional understanding that α-HBCD cannot transform into other diastereomers and β-HBCDs cannot convert into γ-HBCD. Huang et al. (2016) also found trace amounts of γ-HBCD in HepG2 cells 6 d after incubation with α- or β-HBCD. Similarly, in female C57L/6 mice exposed to 14C-labeled α-, β-, and γ-HBCD, β-HBCD could be bioisomerized to γ-HBCD (Sanders et al., 2013). While it is uncertain whether this conversion is attributed to microflora activity in the gut or metabolic activity within the tissues, the ability to convert HBCD diastereomers in N. albiflora does vary across their developmental stages. The precise underlying mechanism requires further exploration.
In exposure studies where N. albiflora was individually exposed to α-HBCD, β-HBCD, or γ-HBCD, α-HBCD was detected in all exposure groups, suggesting that both β-HBCD and γ-HBCD could be bioisomerized to α-HBCD. Diastereomers concentrations varied across tissues, with the intestine and gill exhibiting the highest levels. The half-lives sequence for the three diastereomers varied among tissues and organs, but consistently was α-HBCD > γ-HBCD > β-HBCD. Notably, the concentrations of HBCDs remained equilibrated during transmission from the maternal tissue to progeny and throughout progeny development. However, the mechanism of transformation among the three HBCD diastereomers differed at various developmental stages of N. albiflora.
The raw data supporting the conclusion of this article will be made available by the authors, without undue reservation.
The animal study was approved by the Zhejiang Marine Fisheries Research Institute, Key Laboratory of Sustainable Utilization of Technology Research for Fisheries Resources of Zhejiang Province, Zhoushan. The study was conducted in accordance with the local legislation and institutional requirements.
SS: Data curation, Software, Writing–original draft, Writing–review and editing, Conceptualization, Formal Analysis, Investigation. XS: Methodology, Project administration, Resources, Validation, Visualization, Writing–review and editing. XC: Conceptualization, Data curation, Investigation, Methodology, Writing–original draft. XP: Software, Writing–review and editing. QH: Formal Analysis, Writing–review and editing. HH: Methodology, Software, Writing–review and editing. JZ: Data curation, Investigation, Writing–review and editing. TL: Validation, Writing–review and editing. YG: Supervision, Writing–review and editing.
The author(s) declare financial support was received for the research, authorship, and/or publication of this article. This work was supported by the Zhejiang Basic Public Welfare Plan Project under contract LGF22B070004 and the National Natural Science Foundation of China under contract 21407127.
Author XP is employed by Zhejiang Huanneng Environmental Technology Co., Ltd.
The remaining authors declare that the research was conducted in the absence of any commercial or financial relationships that could be construed as a potential conflict of interest.
All claims expressed in this article are solely those of the authors and do not necessarily represent those of their affiliated organizations, or those of the publisher, the editors and the reviewers. Any product that may be evaluated in this article, or claim that may be made by its manufacturer, is not guaranteed or endorsed by the publisher.
Bertucci, J. I., Malala Irugal Bandaralage, S., and Hecker, M. (2020). Assessing the cytotoxic effect of hexabromocyclododecane (hbcd) on liver tissue cultures from fathead minnow (pimephales promelas). Aquat. Toxicol. 225, 105523. doi:10.1016/j.aquatox.2020.105523
Brandon, A. M., Abbadi, S. H. E., Ibekwe, U. A., Cho, Y. M., Wu, W. M., and Criddle, C. S. (2020). Fate of hexabromocyclododecane (hbcd), a common flame retardant, in polystyrene-degrading mealworms: elevated hbcd levels in egested polymer but no bioaccumulation. Environ. Sci. Technol. 54, 364–371. doi:10.1021/acs.est.9b06501
Darnerud, P. O. (2003). Toxic effects of brominated flame retardants in man and in wildlife. Environ. Int. 29, 841–853. doi:10.1016/S0160-4120(03)00107-7
Du, M., Lin, L., Yan, C., and Zhang, X. (2012). Diastereoisomer- and enantiomer-specific accumulation, depuration, and bioisomerization of hexabromocyclododecanes in zebrafish (danio rerio). Environ. Sci. Technol. 46, 11040–11046. doi:10.1021/es302166p
Feiteiro, J., Mariana, M., and Cairrao, E. (2021). Health toxicity effects of brominated flame retardants: from environmental to human exposure. Environ. Pollut. 285, 117475. doi:10.1016/j.envpol.2021.117475
Fournier, A., Feidt, C., Marchand, P., Vénisseau, A. S., Bizec, B. L., Sellier, N., et al. (2012). Kinetic study of γ-hexabromocyclododecane orally given to laying hens (gallus domesticus). Environ. Sci. Pollut. Res. Int. 19, 440–447. doi:10.1007/s11356-011-0573-6
Geng, J., Han, M., Yang, X., Li, Y., Bartlam, M., and Wang, Y. (2019). Different biotransformation of three hexabromocyclododecane diastereoisomers by pseudomonas sp. under aerobic conditions. Chem. Eng. J. 374, 870–879. doi:10.1016/j.cej.2019.05.232
Gerecke, A. C., Giger, W., Hartmann, P. C., Heeb, N. V., Kohler, H. E., Schmid, P., et al. (2006). Anaerobic degradation of brominated flame retardants in sewage sludge. Chemosphere 64, 311–317. doi:10.1016/j.chemosphere.2005.12.016
Gerecke, A. C., Law, R. J., Voorspoels, S., Kohler, M., Heeb, N. V., Leslie, H., et al. (2006). Hexabromocyclododecanes (hbcds) in the environment and humans: a review. Environ. Sci. Technol. 40, 3679–3688. doi:10.1021/es0602492
Germer, S., Piersma, A. H., van der Ven, L., Kamyschnikow, A., Fery, Y., Schmitz, H., et al. (2006). Subacute effects of the brominated flame retardants hexabromocyclododecane and tetrabromobisphenol a on hepatic cytochrome p450 levels in rats. Toxicology 218, 229–236. doi:10.1016/j.tox.2005.10.019
Ghzela, M., Ben, A. W., Philippe, S., Soufiane, T., Ridha, D. M., and Laure, J. (2023). Correction to: first study of bromophenols and hexabromocyclododecanes in seafood from north africa (case of bizerte lagoon, Tunisia): occurrence and human health risk. Environ. Sci. Pollut. Res. Int. 30, 67914–67917. doi:10.1007/S11356-023-27268-8
Goss, K. U., Arp, H. P. H., Bronner, G., and Niederer, C. (2008). Partition behavior of hexachlorocyclohexane isomers. J. Chem. Eng. Data 53, 750–754. doi:10.1021/je700595y
Goto, A., Tue, N. M., Isobe, T., Takahashi, S., Tanabe, S., and Kunisue, T. (2020). Nontarget and target screening of organohalogen compounds in mussels and sediment from hiroshima bay, Japan: occurrence of novel bioaccumulative substances. Environ. Sci. Technol. 54, 5480–5488. doi:10.1021/acs.est.9b06998
Hakk, H., Szabo, D. T., Huwe, J., Diliberto, J., and Birnbaum, L. S. (2012). Novel and distinct metabolites identified following a single oral dose of α- or γ-hexabromocyclododecane in mice. Environ. Sci. Technol. 46, 13494–13503. doi:10.1021/es303209g
Heldur, H. (2016). Comparative metabolism studies of hexabromocyclododecane (hbcd) diastereomers in male rats following a single oral dose. Environ. Sci. Technol. 50, 89–96. doi:10.1021/acs.est.5b04510
Hou, R., Huang, Q., Pan, Y., Lin, L., Liu, S., Li, H., et al. (2022). Novel brominated flame retardants (nbfrs) in a tropical marine food web from the south China sea: the influence of hydrophobicity and biotransformation on structure-related trophodynamics. Environ. Sci. Technol. 56, 3147–3158. doi:10.1021/acs.est.1c08104
Huang, H., Wang, D., Wan, W., and Wen, B. (2017). Hexabromocyclododecanes in soils and plants from a plastic waste treatment area in north China: occurrence, diastereomer- and enantiomer-specific profiles, and metabolization. Environ. Sci. Pollut. Res. Int. 24, 21625–21635. doi:10.1007/s11356-017-9792-9
Huang, X., Chen, C., Shang, Y., Zhong, Y., Ren, G., Yu, Z., et al. (2016). In vitro study on the biotransformation and cytotoxicity of three hexabromocyclododecane diastereoisomers in liver cells. Chemosphere 161, 251–258. doi:10.1016/j.chemosphere.2016.07.001
Jandacek, R. J., Rider, T., Yang, Q., Woollett, L. A., and Tso, P. (2009). Lymphatic and portal vein absorption of organochlorine compounds in rats. Merican J. Physiology. Gastrointest. Liver Physiology 296, G226–G234. doi:10.1152/ajpgi.90517.2008
Kim, J. T., Choi, Y. J., Barghi, M., Yoon, Y. J., Kim, J. H., Kim, J. H., et al. (2018). Occurrence and distribution of old and new halogenated flame retardants in mosses and lichens from the south shetland islands, Antarctica. Environ. Pollut. 235, 302–311. doi:10.1016/j.envpol.2017.12.080
Koch, C., Schmidt-Koetters, T., Rupp, R., and Sures, B. (2015). Review of hexabromocyclododecane (hbcd) with a focus on legislation and recent publications concerning toxicokinetics and -dynamics. Environ. Pollut. 199, 26–34. doi:10.1016/j.envpol.2015.01.011
Koeppen, R., Becker, R., Jung, C., and Nehls, I. (2008). On the thermally induced isomerisation of hexabromocyclododecane stereoisomers. Chemosphere 71, 656–662. doi:10.1016/j.chemosphere.2007.11.009
Law, K., Palace, V. P., Halldorson, T., Danell, R., Wautier, K., Evans, B., et al. (2006). Dietary accumulation of hexabromocyclododecane diastereoisomers in juvenile rainbow trout (oncorhynchus mykiss) i: bioaccumulation parameters and evidence of bioisomerization. Environ. Toxicol. Chem. 25, 1757–1761. doi:10.1897/05-445r.1
Li, B., Chen, H., Sun, H., and Lan, Z. (2017). Distribution, isomerization and enantiomer selectivity of hexabromocyclododecane (hbcd) diastereoisomers in different tissue and subcellular fractions of earthworms. Ecotoxicol. Environ. Saf. 139, 326–334. doi:10.1016/j.ecoenv.2017.01.004
Lilienthal, H., Ven, L. T. M. V., Piersma, A. H., and Vos, J. G. (2009). Effects of the brominated flame retardant hexabromocyclododecane (hbcd) on dopamine-dependent behavior and brainstem auditory evoked potentials in a one-generation reproduction study in wistar rats. Toxicol. Lett. 185, 63–72. doi:10.1016/j.toxlet.2008.12.002
Lopes, M. M., and Elisa, C. (2023). Occurrence and health effects of hexabromocyclododecane: an updated review. Toxics 11, 409. doi:10.3390/toxics11050409
Lu, H., Ma, X. J., Huang, X. J., Lu, S., Huang, Y. H., Mo, C. H., et al. (2019). Distribution, diastereomer-specific accumulation and associated health risks of hexabromocyclododecanes (hbcds) in soil-vegetable system of the pearl river delta region, south China. J. Environ. Manage 248, 109321. doi:10.1016/j.jenvman.2019.109321
Oh, J. K., Kotani, K., Managaki, S., and Masunaga, S. (2014). Levels and distribution of hexabromocyclododecane and its lower brominated derivative in Japanese riverine environment. Chemosphere 109, 157–163. doi:10.1016/j.chemosphere.2014.01.074
Pan, Y., Liu, S., Li, H., Lin, L., Hou, R., Cheng, Y., et al. (2023). Expanded polystyrene buoys as an important source of hexabromocyclododecanes for aquatic ecosystem: evidence from field exposure with different substrates. Environ. Pollut. 318, 120920. doi:10.1016/j.envpol.2022.120920
Pan, Y., Liu, S., Lin, L., Cheng, Y., Hou, R., Li, H., et al. (2022). Release behaviors of hexabromocyclododecanes from expanded polystyrene microplastics in seawater and digestive fluids. Gondwana Res. 108, 133–143. doi:10.1016/j.gr.2021.10.030
Ross, M. S., Verreault, J., Letcher, R. J., Gabrielsen, G. W., and Wong, C. S. (2008). Chiral organochlorine contaminants in blood and eggs of glaucous gulls (larus hyperboreus) from the Norwegian arctic. Environ. Sci. Technol. 42, 7181–7186. doi:10.1021/es8000147
Ruan, Y., Lam, J. C. W., Zhang, X., and Lam, P. K. S. (2018). Temporal changes and stereoisomeric compositions of 1,2,5,6,9,10-hexabromocyclododecane and 1,2-dibromo-4-(1,2-dibromoethyl)cyclohexane in marine mammals from the south China sea. Environ. Sci. Technol. 52, 2517–2526. doi:10.1021/acs.est.7b05387
Ruan, Y., Zhang, X., Qiu, J. W., Leung, K. M. Y., Lam, J. C. W., and Lam, P. K. S. (2018). Stereoisomer-specific trophodynamics of the chiral brominated flame retardants hbcd and tbech in a marine food web, with implications for human exposure. Environ. Sci. Technol. 52, 8183–8193. doi:10.1021/acs.est.8b02206
Sanders, J. M., Knudsen, G. A., and Birnbaum, L. S. (2013). The fate of β-hexabromocyclododecane in female c57bl/6 mice. Toxicol. Sci. 134, 251–257. doi:10.1093/toxsci/kft121
Shin, E., Jeong, Y., Barghi, M., Seo, S., Kwon, S. Y., and Chang, Y. (2020). Internal distribution and fate of persistent organic contaminants (pcdd/fs, dl-pcbs, hbcds, tbbpa, and pfass) in a bos taurus. Environ. Pollut. 267, 115306. doi:10.1016/j.envpol.2020.115306
Sun, H., Zhang, X., Zhang, Z., Chen, Y., and Crittenden, J. C. (2009). Influence of titanium dioxide nanoparticles on speciation and bioavailability of arsenite. Environ. Pollut. 157, 1165–1170. doi:10.1016/j.envpol.2008.08.022
Sun, R., Luo, X., Zheng, X., Cao, K., Peng, P., Li, Q. X., et al. (2018). Hexabromocyclododecanes (hbcds) in fish: evidence of recent hbcd input into the coastal environment. Mar. Pollut. Bull. 126, 357–362. doi:10.1016/j.marpolbul.2017.11.040
Susanne, E., Roland, B., Ronald, M., and Irene, N. (2011). Hexabromocyclododecane enantiomers: microsomal degradation and patterns of hydroxylated metabolites. Environ. Sci. Technol. 45, 3938–3944. doi:10.1021/es1039584
Szabo, D. T., Diliberto, J. J., Hakk, H., Huwe, J. K., and Birnbaum, L. S. (2010). Toxicokinetics of the flame retardant hexabromocyclododecane gamma: effect of dose, timing, route, repeated exposure, and metabolism. Toxicol. Sci. 117, 282–293. doi:10.1093/toxsci/kfq183
Tang, B., Zeng, Y. H., Luo, X. J., Zheng, X. B., and Mai, B. X. (2015). Bioaccumulative characteristics of tetrabromobisphenol a and hexabromocyclododecanes in multi-tissues of prey and predator fish from an e-waste site, south China. Environ. Sci. Pollut. Res. Int. 22, 12011–12017. doi:10.1007/s11356-015-4463-1
Tavoloni, T., Stecconi, T., Galarini, R., Bacchiocchi, S., Dörr, A. J. M., Elia, A. C., et al. (2021). Bfrs (pbdes and hbcds) in freshwater species from lake trasimeno (Italy): the singular case of hbcds in red swamp crayfish. Sci. Total Environ. 758, 143585. doi:10.1016/j.scitotenv.2020.143585
Tavoloni, T., Stecconi, T., Galarini, R., Bacchiocchi, S., Piersanti, A., Elia, A. C., et al. (2020). Bfrs (pbdes and hbcds) in freshwater species from lake trasimeno (Italy): the singular case of hbcds in red swamp crayfish. Sci. Total Environ. 758, 143585. doi:10.1016/j.scitotenv.2020.143585
Taylor, S. D., Diliberto, J. J., Hakk, H., Huwe, J. K., and Birnbaum, L. S. (2011). Toxicokinetics of the flame retardant hexabromocyclododecane alpha: effect of dose, timing, route, repeated exposure, and metabolism. Toxicol. Sci. Official J. Soc. Toxicol. 121, 234–244. doi:10.1093/toxsci/kfr059
Topić Popović, N., Čižmek, L., Babić, S., Strunjak-Perović, I., and Čož-Rakovac, R. (2023). Fish liver damage related to the wastewater treatment plant effluents. Environ. Sci. Pollut. Res. Int. 30, 48739–48768. doi:10.1007/s11356-023-26187-y
Wang, H., Huang, W., Gong, Y., Chen, C., Zhang, T., and Diao, X. (2020). Occurrence and potential health risks assessment of polycyclic aromatic hydrocarbons (pahs) in different tissues of bivalves from hainan island, China. Food Chem. Toxicol. 136, 111108. doi:10.1016/j.fct.2019.111108
Wang, X., Tan, X., Zhang, Y., Hu, X., Shen, C., Huang, Y., et al. (2021). The enantiomer-selective metabolism of hexabromocyclododecanes (hbcds) by human hepg2 cells. Sci. Total Environ. 768, 144430. doi:10.1016/j.scitotenv.2020.144430
Wu, T., Li, X., Zheng, Z., Liu, Z., Yang, M., Zhang, N., et al. (2023). Hexabromocyclododecanes in surface soil-maize system around baiyangdian lake in north China: distribution, enantiomer-specific accumulation, transport, temporal trend and dietary risk. J. Hazard Mater 451, 131180. doi:10.1016/j.jhazmat.2023.131180
Zegers, B. N., Mets, A., Van Bommel, R., Minkenberg, C., Hamers, T., Kamstra, J. H., et al. (2005). Levels of hexabromocyclododecane in harbor porpoises and common dolphins from western european seas, with evidence for stereoisomer-specific biotransformation by cytochrome p450. Environ. Sci. Technol. 39, 2095–2100. doi:10.1021/es049209t
Zhang, Y., Li, S., Zhang, Y., Chen, Y., Wang, X., and Sun, Y. (2022). Bioaccumulation and biomagnification of hexabromocyclododecane in marine biota from China: a review. Toxics 10, 620. doi:10.3390/toxics10100620
Zhang, Y., Lu, Y., Wang, P., and Shi, Y. (2018). Biomagnification of hexabromocyclododecane (hbcd) in a coastal ecosystem near a large producer in China: human exposure implication through food web transfer. Sci. Total Environ. 624, 1213–1220. doi:10.1016/j.scitotenv.2017.12.153
Zhang, Y., Sun, H., and Ruan, Y. (2014). Enantiomer-specific accumulation, depuration, metabolization and isomerization of hexabromocyclododecane (hbcd) diastereomers in mirror carp from water. J. Hazard Mater 264, 8–15. doi:10.1016/j.jhazmat.2013.10.062
Zhang, Y., Wang, L., Sun, H., Yao, T., Zhu, H., Xu, J., et al. (2016). Impacts of loach bioturbation on the selective bioaccumulation of hbcdd diastereoisomers and enantiomers by mirror carp in a microcosm. Chemosphere 163, 471–479. doi:10.1016/j.chemosphere.2016.08.065
Keywords: hexabromocyclododecanes, isomers, bioisomerization, selective-accumulation, distribution, depuration
Citation: Song S, Sun X, Cheng X, Peng X, Hao Q, Hu H, Zhu J, Li T and Guo Y (2023) The distribution and metabolism of hexabromocyclododecane isomers varies in the tissues of Nibea albiflora. Front. Environ. Sci. 11:1274997. doi: 10.3389/fenvs.2023.1274997
Received: 09 August 2023; Accepted: 07 November 2023;
Published: 20 November 2023.
Edited by:
Abdul Qadeer, Chinese Research Academy of Environmental Sciences, ChinaReviewed by:
Jingqian Xie, Shanghai Ocean University, ChinaCopyright © 2023 Song, Sun, Cheng, Peng, Hao, Hu, Zhu, Li and Guo. This is an open-access article distributed under the terms of the Creative Commons Attribution License (CC BY). The use, distribution or reproduction in other forums is permitted, provided the original author(s) and the copyright owner(s) are credited and that the original publication in this journal is cited, in accordance with accepted academic practice. No use, distribution or reproduction is permitted which does not comply with these terms.
*Correspondence: Xiumei Sun, eGl1bWVpc3VuMjUwNDM4NjY3QDE2My5jb20=
Disclaimer: All claims expressed in this article are solely those of the authors and do not necessarily represent those of their affiliated organizations, or those of the publisher, the editors and the reviewers. Any product that may be evaluated in this article or claim that may be made by its manufacturer is not guaranteed or endorsed by the publisher.
Research integrity at Frontiers
Learn more about the work of our research integrity team to safeguard the quality of each article we publish.