- 1Key Laboratory of Geological Processes and Mineral Resources of Northern Qinghai-Tibetan Plateau, Qinghai Geological Survey Institute, Xining, China
- 2Key Laboratory of Salt Lake Resources Exploration and Research in Qaidam Basin, Qinghai Province, Golmud, China
- 3Key Laboratory of Comprehensive and Highly Efficient Utilization of Salt Lake Resources, Qinghai Institute of Salt Lakes, Chinese Academy of Sciences, Xining, China
- 4Key Laboratory of Salt Lake Geology and Environment of Qinghai Province, Xining, China
- 5University of Chinese Academy of Sciences, Beijing, China
Ca-Cl brine is a highly mineralized special water body occurring in oceanic ridges or basins. The deep part of the western Qaidam Basin is rich in Ca-Cl brine, which is essential for the formation of (MgSO4-deficient) potash deposits. Previous studies on this type of brine in the Qaidam Basin focused on its source tracing, but different carriers led to different conclusions. Moreover, the genesis of Ca-Cl brines in inland basins still remains unclear. In essence, a solid understanding of the relatively abundant Ca sources for the formation of Ca-Cl brines in inland basins has not been reached. In addition, a metallogenic model for this type of brine has not yet been established. Through hydrochemical analysis as well as Band Li isotope tracing, this study clarified that the main elements (Na and Cl) in these Ca-Cl brines mainly originated from the dissolution of existing evaporites in the inner basin. Further, trace elements in Ca-Cl brines (such as Band Li) mainly originated from lixiviation; atmospheric rainfall infiltrated and interacted with ore-rich mother rocks along the basin-margin fracture system under suitable temperature-pressure conditions; alternatively, they may have originated from mixing with magmatic hydrothermal fluids. This study showed that Ca-Cl brines in the western part of the Qaidam Basin have a typical “binary structure” in terms of their sources. In addition, the sources of Ca in Ca-Cl brines were further discussed based on B and Li isotope data, thus exploring a new genesis of Ca-Cl brines in inland basins. Finally, by integrating the above findings, an ore-forming model of this type of water body in the western part of the Qaidam Basin is proposed. This study is valuable for understanding the genesis mechanism of Ca-Cl brines in inland basins, and also has practical significance for the prediction, exploration, and evaluation of this type of brine resource.
1 Introduction
Paleocene-Neogene strata in the Qaidam Basin are rich in Ca-Cl type brine (oilfield brine); this Ca-Cl brine is not only rich in Na, Ca, and Mg, but also in resource elements such as K, B, Li, and Br. Moreover, because of its special hydrochemical characteristics, Ca-Cl type brine significantly influences the formation of certain evaporite deposits and the evolution of certain evaporite-forming fluids (Lowenstein et al., 1989; Hardie, 1990; Lowenstein et al., 2001). Therefore, it is associated with substantial scientific research and resource development prospects.
Generally, research on the origin of Ca-Cl brine often involves contents such as the origin of the water body in the brine, the origin of each element in the brine, the migration process of the brine, and the final enrichment and preservation processes of the brine (Zhai, 2020). Various scholars have studied the Ca-Cl type brine resources in the western Qaidam Basin from different perspectives. Based on data obtained by isotopic tracing studies (e.g., H, O, and He), it has been proposed that the water in this type of brine shows the characteristics of atmospheric rainfall and mixing with fluids from deep magmatic hydrothermal sources (Tan et al., 2011; Li et al., 2022a). Moreover, for the storage environment and space of these Ca-Cl brines, findings from geochemical research and drilling cores have confirmed that these Ca-Cl brines in the Qaidam Basin are in a reducing environment (Tan et al., 2011; Li, 2013); the fault, fissure, and anticline structures in the western basin are often storage spaces (Li et al., 2015). The studies referenced above show that this type of brine is stored in a relatively closed underground space; to a certain extent, the Ca-Cl brine can often be transported through fracture and fissure structures inside the basin under specific hydrodynamic conditions. This knowledge provides a useful basis for understanding the origin of this type of brine. However, different methods often lead to different conclusions. For example, the application of hydrochemical analyses of Ca-Cl brine in the Qaidam Basin often leads to the conclusion that the main elements such as Na and Cl in the Ca-Cl brine often originate from the redissolution of existing evaporates in the basin (Li et al., 2014); research using isotopes concluded that certain elements of the Ca-Cl brine often originate from low temperature weathering or hydrothermal activity (Shi et al., 2018; He et al., 2020; Li et al., 2021). Different source conclusions of the same kind of Ca-Cl brine have caused great confusion in the understanding of the causes of this type of brine. In addition, how the Ca-Cl brine water formed in this basin remains unknown. Various scholars have put forward the following different explanations: 1) diagenetic transformation between different water bodies and surrounding rocks in the continental basin (albitization, dolomitization, and reduction) (Walter et al., 1990; Li et al., 2014); 2) residual, concentration, and evaporation processes of seawater from Ca-Cl in marine or continental basins during the geological period (Lowenstein, 2003; Bottomley et al., 2005); 3) hydrothermal origin (Hardie, 1990; Tan et al., 2011; Lowenstein et al., 2016). However, since the Cenozoic, the Qaidam Basin has been a typical continental basin (Zhang et al., 1987), and decades of research have not found any clear records of hydrothermal activity in the interior of the basin. A number of relevant questions remain unaddressed: Are the conditions suitable for hydrothermal supply within the basin? Can diagenetic transformation between different water bodies and surrounding rocks form Ca-Cl brine in the western continental basin? In addition, as a typical inland basin, evidence of seawater residual since the Cenozoic Qaidam Basin has not yet been found; many problems are also associated with the current knowledge on the causes of Ca-Cl brine formation by ancient seawater residual, evaporation, and concentration in the Qaidam Basin.
How could such a large amount of Ca-Cl brine have formed in the western Qaidam Basin, and what metallogenic model is the most meaningful. The lack of clear answers to these questions severely restricts the current understanding of the formation mechanism, thus hindering the prediction, exploration, and evaluation of these Ca-Cl brine resources. In fact, as a complex water and salt system that is rich in various resource elements, the origin of multiple elements in the Ca-Cl brine may often be complex. Based on an analysis of the hydrochemical characteristics as well as B and Li isotopes data, this paper presents the “binary structure” characteristics of the main sources of the Ca-Cl brine in the western Qaidam Basin. Furthermore, the formation mechanism of Ca-Cl brine is discussed, and a new metallogenic model is developed. The conclusions of this study are of great theoretical and practical significance both for a better understanding of the genetic mode of the Ca-Cl brine in the western basin and for guiding the prediction, exploration, and development of this type of brine resource.
2 Geological setting
2.1 The tectonic background
The Qaidam Basin is located in the northeast corner of the Qinghai-Tibet Plateau and has an irregular rhomboid shape. It has a length of 850 km from east to west, a width of 150–300 km from north to south, and an area of about 12.1 × 104 km2. Tectonically, the basin belongs to the northeast part of the Tethyan domain, with the Qilian orogenic belt to the north, the East Kunlun orogenic belt to the south, and the Altyn Tagh orogenic belt to the west. These orogenic belts have undergone long-term and complicated evolution and formed the complex tectonic-magmatic- hydrothermal framework of the surrounding mountain system (Figure 1; Dai and Cao, 2000; Tang et al., 2000; Tan et al., 2012).
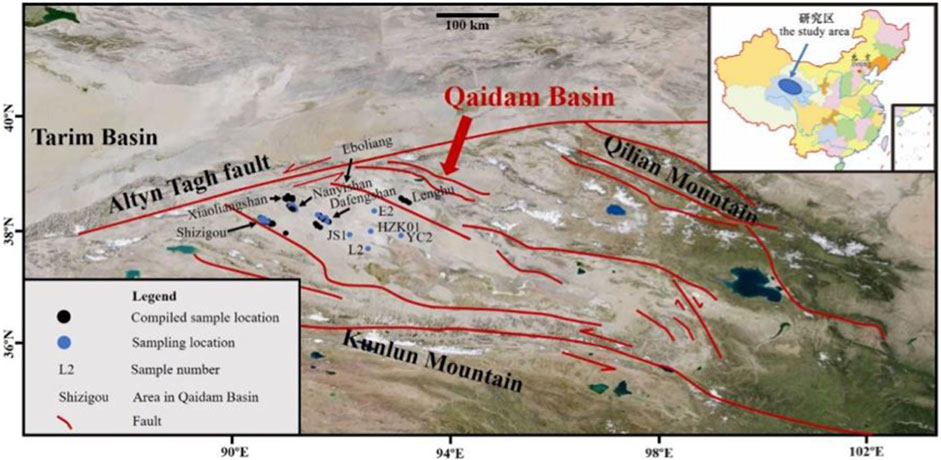
FIGURE 1. Map showing the sample locations [Note: All the blue circles represent the sampling locations for hydrochemical characteristic analysis in this study, the five blue circles with sample number represent the sampling locations for Li-isotope analysis in this study, The five blue circles with sample number, two blue circles (N13 and N6) from Nanyishan area and six blue circles from Shizigou area (S41H1-1-411, S41H2-1-411, S41H1-2-413, S41H1-1-511, S49H1-3-512, S38) represents the sampling locations for B-isotope analysis in this study], surrounding mountains, and other sites of the Qaidam Basin relevant for the paper.
During the Cambrian-Devonian period, the Qaidam Basin was a relatively independent Terrene (Tang et al., 2000). After the Indosinian tectonic event, the Qaidam terrene and its surrounding blocks gradually merged and transitioned to the tectonic stage of intra-continental basin development (Tang et al., 2000). Since the Cenozoic, the basin has widely received sediments and formed a set of overlying strata with a thickness of about 6,000–7,000 m and up to 10,000 m in certain areas (Zhao et al., 2013). Because of the influence of the “far-field effect” after the collision between the Indian plate and the Eurasian plate since the Cenozoic, the fault system at the margin of the basin was reactivated. Further, the Altyn Tagh fault to the western margin of the Qaidam Basin also began to be reactivated, and a series of NW-SE trending anticline and syncline structures developed in the west of the Qaidam Basin (Yin et al., 2007). These anticline and syncline structures can be identified, as the anticline structures are narrow, while the syncline structures are broad. This reflects the typical structural shape of the detachment system, indicating the existence of detachment in the deep part of the basin (Yin et al., 2007). These anticline structures in the western basin are rich in Ca-Cl brine resources (Li et al., 2015). Moreover, the fault system at the margin of the basin and part of the inner basement were also reactivated, and four types of fault systems were developed: the northern margin fault system of the basin, the northern margin fault system of the East Kunlun Mountain, the Altyn Tagh fault system, and the intra-basin fault system. Together, these faults controlled the Mesozoic and Cenozoic sedimentation of the basin (Luo and Pang, 2003).
2.2 Basin sediments and hydrogeology
The depositional strata since the Cenozoic can be divided into seven units from old to new: the Paleocene-Eocene Lulehe Formation (E1+21), the Oligocene Lower Ganchaigou Formation (E3g), the Miocene Upper Ganchaigou Formation (N1g), the Lower Youshashan Formation (N1y), the Pliocene Upper Youshashan Formation (N2g), the Shizigou Formation (N2S), and the Quaternary Qigequan Formation (Qp1+2) (Table 1). In recent years, with the accumulation of chronology data, especially high-precision paleomagnetic data, accurate ages of basin strata and chronology frameworks have been established (Table 1; Sun et al., 2004; Nie et al., 2019).
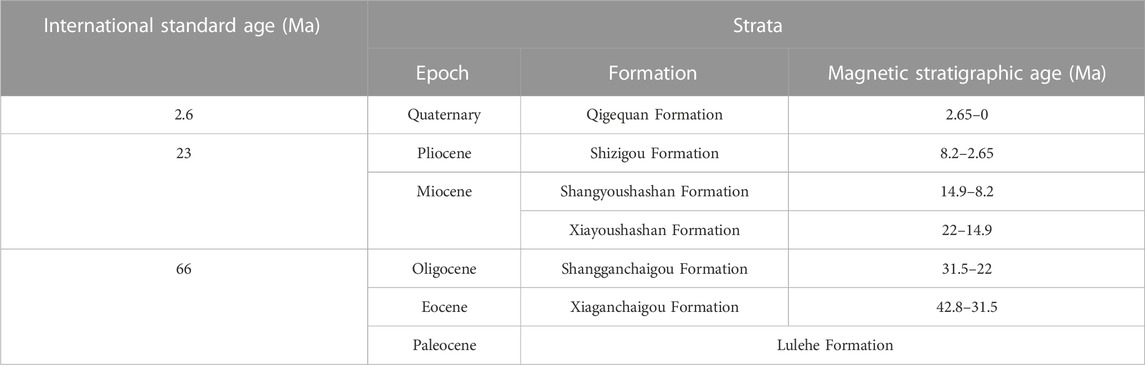
TABLE 1. Cenozoic magnetic stratigraphy of the Qaidam Basin (modified from Nie et al., 2019).
After the formation of the basin, widely distributed Cenozoic strata have occurred in Qaidam Basin. From the perspective of sedimentary sequences in the western Qaidam Basin, at the end of the Paleogene period, the Qaidam Basin has entered a stage of subsidence and the paleontological sedimentary environment and experienced processes of water body development from shallow to deep (Su et al., 2014). This means that from the Lulehe Formation to Xiaganchaigou Formation, the sedimentary facies are mainly developed from coastal-shallow to semi-deep lake subfacies (Su et al., 2014). The Neogene strata are mainly developed as lake facies, and the lithology is mainly alluvial rock, gray mudstone, and silty mudstone (Zeng et al., 2021). Shallow coastal lake facies primarily dominate the Quaternary strata, and semi-deep lake facies appeared in the middle part during the late stage (Dang et al., 2004).
According to drilling core data, a large number of evaporite deposits, represented by carbonate, sulfate, and chloride, have been developed in the western basin since the Eocene (Zeng et al., 2021). Among them, the oldest evaporate deposits are developed in the late Eocene Upper Xiaganchaigou Formation in the Shizigou area (Guo et al., 2018). These evaporate deposits can provide an abundant source for Ca-Cl brine during their migration. Moreover, very thick piedmont pluvial deposits occur at the end of the Paleogene, with the thickest strata exceeding 2,600 m (Zhang et al., 1987). Thus, the Ca-Cl brines (oil-field Ca-Cl brines) are produced in Paleocene–Neogene strata. These Ca-Cl brines have been found in many tectonically controlled units (e.g., anticlines, fractures, faults) and have high total dissolved salts (TDS) that coexist with petroleum in clastic rocks (Li et al., 2015). Commonly, the Ca-Cl brines in Paleocene formations are >1,000 min-depth (ranging from 1,000 to 1,500 m) and Neogene formation Ca-Cl brines are <1,000 m in-depth (ranging from 500 to 1,000 m). All Ca-Cl brines from petroleum wells have very high pressure resulting in constant self-eruption for several decades, and they have temperatures close to or exceeding 100°C and TDS >100 g/L. Minerals salts precipitate on the surface once the Ca-Cl brines discharge from production tubing.
3 Sampling and analytical methods
3.1 Sample collection and preparation
Most of the samples used for this study were collected from oilfield wells. A small number of samples (i.e., JS1, L2, E2, HZK01and YC2) from the middle of the basin were collected from Ca-Cl brine exploration wells (Figure 1). A final volume of each sample of 500 ml was ensured after measuring the specific gravity on site and sealing the samples for laboratory analysis.
Ninety-five samples from Nanyishan, Shizigou and Dafengshan areas as well as the central part of the basin were selected for hydrochemical characteristics analysis (Figure 1; sampling location). These samples basically cover the whole western region of the Qaidam Basin and are representative of the basic characteristics of the Ca-Cl brine in this basin. Considering the differences in the origin of different types of water bodies, only Ca-Cl water bodies in the study area were retained in this study. Firstly, the water types were defined according to the principle of published by Hardie and Eugster (1970) to eliminate non-Ca-Cl type hydrochemical data. Further, 63 sets of published hydrochemistry data of Ca-Cl brines from Nanyishan, Shizigou, Youdunzi, Youshashan, Xiaoliangshan and Lenghu areas were also compiled (Figure 1; compiled location; Liu et al., 2019; Tan et al., 2007; Li, 2007; Li, 2013; Li et al., 2015). Together, these hydrochemical data can reflect the hydrochemical characteristics of the Ca-Cl brine in the whole western Qaidam Basin; thirteen Ca-Cl brine samples from Shizigou (S38, S41H1-1-411, S41H2-1-411, S41H1-2-413, S41H1-1-511 and S49H1-3-512), Nanyishan (N6 and N13) and central basin (JS1, L2, E2, HZK01 and YC2) were subjected to B isotope analysis, these samples were distributed throughout the western basin and have a good representativeness. Considering the latest published Li-isotope data of Ca-Cl type brine in the western basin and the lack of related research of Ca-Cl brine in the central part of the basin, only five Ca-Cl type samples from the central basin were selected for analysis (Figure 1; JS1, L2, E2, HZK01 and YC2). The above data were integrated to explore the origin of Ca-Cl brine in the western Qaidam Basin.
3.2 Analytical methods
3.2.1 Major ion analyses of Ca-Cl brine samples
These Ca-Cl brine samples, which were analyzed for Na+, K+, Li+, Ca2+, Mg2+, SO42− and Cl− concentrations, as well as part of trace elements (such as the Li+, Br−, B2O3 and Sr) were examined at the Qinghai Institute of Salt Lakes, Chinese Academy of Sciences. The samples were measured by inductively coupled plasma optical emission spectrometry (ICAP 6500 DUO ICP-OES). Cl−, SO42−, and Br− concentrations were analyzed by ion chromatography (ICS-5000+). HCO3− concentrations were determined by general titration. The analytical method used in this study followed the procedures published by Qinghai Institute of Salt Lakes (1988). The analytical precision for major cations and anions exceeds ±2%. The trace elements were determined by Atomic Absorption Spectrometry (GBC—908) with an analytical error of ±5%. The results are shown in Supplementary Appendix SA.
3.2.2 Lithium isotopic analysis
Li isotope analysis was performed at the Institute of Earth Environment, Chinese Academy of Sciences (IEECAS). Chromatographic isolation of Li was carried out using AG50W-X8 cation exchange resin with a 100–200 mesh size, packed into customized PDF micro columns with an internal diameter of 6.4 mm and a column length of 25 cm. To purify Li, the samples were passed through columns and were eluted with 20 mL of 0.5 M HNO3, and Li was collected with 28 mL of 0.5 M HNO3. The column chemistry procedure was conducted twice to ensure recovery of pure Li solution, and the Na/Li ratio was less than 5 in all samples. The Li separation procedures followed the steps described by Guo et al. (2018).
Li isotope ratios were measured using the sample standard bracketing (SSB) method on a NEPTUNE Plus MC-ICP-MS at IEECAS. All samples were analyzed at a similar Li concentration and the same procedure as standards (L-SVEC; Flesch et al., 1973). Totals of 1% NaCl and 2% HNO3 were used to wash the introduction system. The total procedural blank of this method was less than 0.16 ng Li, which was deemed negligible relative to the 300 ng of Li analyzed in each sample (Gou et al., 2019). Values of δ7Li are reported in standard per mil (‰) notation relative to L-SVEC, where δ7Li = [(7Li/6Li) sample/(7Li/6Li) L - SVEC - 1] × 1000. The in-house Li standards USTC-L yielded an average value of δ7Li = −19.3‰ ± 0.1‰ (2 s.d., n = 45) and yielded as δ7Li = 12.2‰ ± 0.2‰ (2 s. d., n = 78) for SPEX-Li. GBW-Li and seawater reference standard materials were repeatedly measured to be δ7Li = 7.93‰ ± 0.2‰ (2 s.d., n = 10), δ7Li = 31.2‰ ± 0.7‰ (2 s.d., n = 21). In this experiment, the δ7Li of standards was measured in agreement with previously published data (Dellinger et al., 2015, Dellinger et al., 2017; Gou et al., 2019). Moreover, the long-term external reproducibility exceeds ±0.9‰ (2 s.d.) for δ7Li measurements (Gou et al., 2018). Detailed analytical methods for Li isotopes analysis have been described by Gou et al. (2019).
3.2.3 Boron isotopic analysis
The B isotope analysis was performed at the Salt Lake Analytical and Test Department of the Qinghai Institute of Salt Lakes, Chinese Academy of Sciences. A 2-column procedure was used for the B separation. For the first column, a mixed cation-anion resin column was used, and for the second column, an Amberlite IRA743 B-specific resin column was used. The mixed resin consists of a 200–400 mesh strongly acidic cation resin (Dowex 50 W × 8 H+, United States) and a 60–100 mesh weakly alkaline anion resin (Ion Exchanger II, HCO3−, Germany). Amberlite IRA 743 B-specific resin was first conditioned with an HCl solution. Then, the anion resin was leached with a saturated NaHCO3 solution. Finally, the column was washed with DI water to achieve a neutral pH before the subsequent use (Ma et al., 2011).
B purification and separation were accomplished according to the following three steps. The samples were passed through a special B adsorption resin column with a velocity of 2.5 mL min−1. Then the leachate was collected and processed with a B HCl elution (500 μL, 75°C, and 0.1 mol L−1). Next, 0.5 mL of the solution was concentrated and purified in a furnace at 60°C. Then, the purified solution was loaded into the mixed anion and cation exchange resin column, and the leachate was collected and processed with an elution of 15 mL of low B water. Appropriate amounts of Cs2CO3 and mannitol were added to keep the molar ratio of B/Cs at 2:1 and that of B/mannitol at 1:1. Then, they were concentrated to 1 mg mL−1 by heating at <60°C. The 11B/10B ratio was measured on a thermal ionization mass spectrometer (Thermo Fisher Finnigan, Germany). The B isotopic composition was calculated as follows (Eq. 1):
where the standard materials was NIST SRM951. The measured 11B/10B standard NIST SRM 951 = 4.05262 ± 0.00077 (2σ = 0.02%, n = 9) with an analytical uncertainty of 0.3‰.
4 Results
4.1 Major elements
The major element data are shown in Supplementary Appendix SA. Ca-Cl brine has high ion concentrations of Cl−, Na+, Ca2+, SO42−, Mg2+and HCO3−. The K+, B2O3, and Li+ contents in this Ca-Cl brine are relatively high and have potential development value.
To clarify the relationship between the different major elements, the Pearson correlation coefficients between the 12 major elements were calculated (Table 2).
The correlation coefficients (Table 2) between Na+ and Cl−, TDS and Na+, as well as TDS and Cl− in Ca-Cl brine are 0.96, 0.94, and 0.93, respectively. These high coefficients not only imply that the dissolution of NaCl provides the main source of Na+ and Cl− in Ca-Cl brines, but also that these two elements are the main ionic components causing the high TDS in Ca-Cl brines. The correlation coefficients between K+ and Na+, K and Ca2+, K+ and Cl−, K+ and B2O3, and K+ and Li+ in Ca-Cl brines are 0.54, 0.81, 0.70, 0.53, and 0.78, respectively. In combination with the high correlations between Na+ and Cl−, TDS and Na+, as well as TDS and Cl-, these correlation coefficients suggest that the K+ in Ca-Cl brines may originate from both the dissolution of existing evaporites in the basin and other sources. The correlation coefficients between Ca2+ and Li+, as well as Ca2+ and Sr2+ in Ca-Cl brines are 0.90 and 0.77, respectively. To a certain extent, this result suggests that the sources of Li+ and Sr2+ in Ca-Cl brines are most likely originate from the same source area and are related to minerals or rocks with high Ca2+ levels. The correlation coefficients between Mg2+ and SO42− as well as Mg2+ and HCO3− in Ca-Cl brines are 0.62 and 0.49, respectively, and the correlations between Ca2+ and SO42−, as well as Ca2+ and HCO3− are low. This result may be related to the incomplete dissolution of dolomite during the migration of Ca-Cl brine. In addition, the correlation between B2O3 and Li+ in Ca-Cl brines is 0.51. Some of the B3+ and Li+ elements in Ca-Cl brines may originate from bedrock weathering or water-rock interaction under hydrothermal conditions. Further, B2O3 correlates well with TDS, B2O3 correlates well with K+, B2O3 correlates well with Na+, and B2O3 correlates well with Cl−, indicating that the B2O3 in the Ca-Cl brine is partially derived from recharge after redissolution with existing evaporites in the basin. The Li+ in brines also shows similar characteristics with the boron. In fact, the moderate correlation between Cl− and Ca2+, Ca2+ and Na+ also supports the above inference. Together, these modes comprise the sources of B3+ and Li+ elements in the Ca-Cl brine.
4.2 Li isotope compositions
The δ7Li values of samples in the Qaidam Basin display significant variation, ranging from 10.48‰ to 15.97‰ (Table 3); this variation implies that the source of Li in Ca-Cl brine at the sampling site is relatively consistent. The corresponding Li content in the sample was relatively high.
4.3 B isotope compositions
The B isotopes of Ca-Cl brines in the western Qaidam Basin were also analyzed and the results are presented in Table 4. The variation of the B isotope composition in this region ranged from −5.68‰ to 26.69‰, with an average of 6.10‰, which is much lower than the value of seawater (39.5‰).
5 Discussion
5.1 Data analysis
According to the results of ion correlation analysis, the major elements (especially for Na and Cl) in Ca-Cl brines clearly originate from the dissolution of NaCl. If this conclusion is true, the process inevitably also dissolved other relatively soluble minerals during the Ca-Cl brine formation. The correlation between Mg2+, Ca2+ SO42− and HCO3− indicate incomplete dissolution of dolomite during Ca-Cl brine migration. When dolomite is completely dissolved, both Ca2+ and Mg2+ are completely released; however, when dissolution is incomplete, part of the Mg2+ is released earlier, and the remaining carbonate is precipitated as CaCO3. This preliminary dissolution process tends to result in a certain correlation between Mg2+ and CO32− and a lack of correlation between Ca2+ and CO32− (Eqs 2, 3). In addition, considering the correlations between K+, Na+, Ca2+, Cl−, B2O3 and Li+ as well as the correlations between Na+, Cl− and TDS, it is reasonable to speculated that the K+ in Ca-Cl brines may originate from both the dissolution of existing evaporites in the basin and other sources. Such dissolution can occur because of weathering or hydrothermal water-rock interaction (Li, 2013; Miao et al., 2022). Although similar geochemical mobility of ions also results in correlation between data, the supply of these ions by the source region is an indispensable and important factor. This conclusion is also supported by the observed correlation between Ca2+, Li+ and Sr2+. This correlation shows that the sources of Li+ and Sr2+ in Ca-Cl brines most likely originate from the same source area related to minerals or rocks with high Ca2+ levels. Furthermore, the correlation between B2O3 and Li+ in Ca-Cl brines is moderate. B3+ and Li+ elements have many similar geochemical properties and both tend to be enriched in bedrock in acidic granite bodies and in weathered sedimentary rocks. It appears that some of the B3+ and Li+ elements in Ca-Cl brines may originate from bedrock weathering or water-rock interaction under hydrothermal conditions (He et al., 2020; Miao et al., 2022). Also, B2O3 and K, B2O3 and Na as well as B2O3 and Cl− correlates much better (0.53, 0.73 and 0.75, respectively). This result indicates that the B2O3 in the Ca-Cl brine is partially derived from recharge after redissolution with existing evaporites in the basin. The moderate correlation, such as between Cl− and Ca2+, Ca2+ and Na+, also supports the above conclusion.
In brief, the findings present a typical “binary structure” in terms of sources. The main elements in Ca-Cl brines are mainly recharged by re-soluble evaporites, and they are possibly recharged by weathering or hydrothermal water-rock reactions, too. These recharge modes may represent the main supply pattern of elements within the Ca-Cl brines of this basin.
5.2 Indication of the origin of major elements via hydrochemical characteristics of brines
The main ion concentration combinations are Cl−, Na+, K+, Ca2+, SO42−, Mg2+, and HCO3−. Anions were dominated by Cl-with a content of up to 189.35 g/L, and cations were mainly Na+, with a content of up to 118.51 g/L; moreover, the linear relationship between the Na+ content and TDS was excellent, with a correlation coefficient of 0.92. This result suggests that the high TDS characteristic of Ca-Cl type brines is closely related to the evaporative concentration or dissolution of salt minerals. The correlation coefficient between Na+ and Cl− is 0.93, indicating that Na+ and Cl− in the Ca-Cl brine are mainly derived from the dissolution of halite within the basin (Figure 2).
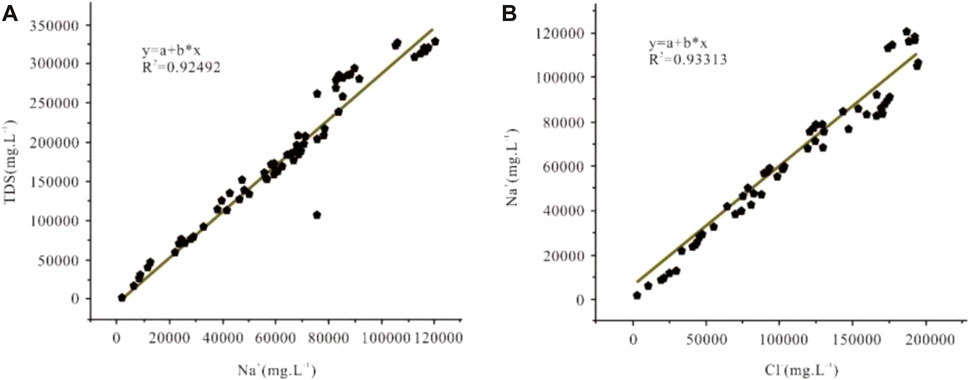
FIGURE 2. Relationship between Na+ content and TDS in Ca-Cl brines in western Qaidam Basin (A); relationship between Na+ and Cl− contents in Ca-Cl brines in the western Qaidam Basin (B).
5.3 Origin of B and Li elements in Ca-Cl brines
5.3.1 The main sources of B and Li elements in basin Ca-Cl brines
Ca-Cl brines are a particular type of fluid with high solids solubility and high abundance of K, B, Li and Sr, as well as many other components. The possible sources of Li and B elements in these Ca-Cl brines are mainly: 1) redissolution of existing evaporites during the Tertiary (Zhang et al., 1987; Risacher et al., 2003); 2) low-temperature weathering of Li-rich mother rocks in the mountains surrounding the basin (Zhang et al., 1987; Risacher et al., 2003; He et al., 2020); and 3) high-temperature water-rock exchange between Li-rich mother rocks and permeable fluids of atmospheric precipitation under specific temperature-pressure conditions and magmatic hydrothermal recharge (Zhang et al., 1987; He et al., 2020). These different sources of B and Li elements can be added to Ca-Cl brines, thus constituting their main sources.
5.3.1.1 Redissolution of evaporite deposits in the inner basin
The western part of the Qaidam Basin is rich in Tertiary evaporite sediments, which usually contain certain amounts of B, Li, and other elements. These early evaporites are dissolved by the groundwater in the form of a solution and then transport the B and Li elements in the evaporites to Ca-Cl brines. This will lead to an increase of B and Li elements in brines. It has been shown that the source of water bodies in Ca-Cl brines is mainly atmospheric precipitation, which inevitably dissolve some of the existing evaporites in the basin during infiltration, thus providing a certain amount of B and Li elements to the brines (Risacher and Fritz, 2008; Rissmann et al., 2015). The present study shows that the main elements in the Ca-Cl brines originate from the dissolution of existing evaporites in the basin, and this conclusion indicates that the dissolution of existing evaporites provides part of the B and Li elements in Ca-Cl brines. In the modern-day Li-rich saline lakes of the world, the input of weathered material is much lower than that of salt redissolution (Risacher et al., 2003). This highlights the importance of the contribution of salt redissolution to the individual elements in Ca-Cl brines.
5.3.1.2 Low-temperature weathering of lithium-rich mother rocks
Basins are usually composed of stable blocks that are often bounded by subduction-collisional orogenic belts. Many magmatic rock bodies that are rich in B, Li, and other elements have developed in the orogenic belt surrounding the Qaidam Basin (Weynell et al., 2017; He et al., 2020). As a residue of ocean-continent collision, continent-continent collision, and subduction belts (in which a large amount of ancient marine sediments remain), the orogenic belt is often an important site for the enrichment of B, Li, and other elements (Liu et al., 2021). Magmatic bodies with high B-Li content and ancient marine sediments that developed in the mountain systems around the basins inevitably carry some of the B and Li elements into the basin. These elements are carried in the form of river water from snowmelt, melting ice, rainwater, and other surface waters during weathering. These elements provide certain sources for Ca-Cl brines in the basins during the process of infiltration. This conclusion is supported by modern river data in the Qaidam Basin, where the Li content of the Nalenggele River at the southern margin of the Qaidam Basin gradually increases from 0.43 to 0.53 mg L−1 (He et al., 2020). With the flow of this river, the elemental content of Li is increasing in the downstream water (Miao et al., 2022). This means that rocks with high background values around the basin and their release of certain B, Li, and other resource elements into the river because of weathering form one mechanism. The other mechanism is the enrichment and migration rules of adsorption and desorption of clay and other minerals produced in this process. Both are important ways to cause the observed change of resource elements in rivers.
5.3.1.3 High-temperature water-rock exchange between lithium-rich mother rocks and fluids
Atmospheric precipitation is a deep cycle, which is heated under suitable temperature-pressure conditions, and finally reaches the surface as salt or hot springs (Feng et al., 2012). In this process, many elements such as Li, B, and K are desorbed from rocks with high background value through water-rock reactions under suitable temperature-pressure conditions (Cullen et al., 2019; He et al., 2020; Miao et al., 2022). During this circulation, magmatic hydrothermal fluids that are rich in B, Li, and other elements in the late magmatic stage sometimes provide many relevant resource elements (Cullen et al., 2019); eventually, these resource elements are brought into Ca-Cl brines through the fault system of the basin. With the circulating action of deep fluids and shallow groundwater, elements such as B and Li are gradually enriched. This process is the main source of the enrichment of Ca-Cl brines with elements such as B and Li. The most striking feature of geothermal waters is their relative enrichment with specific characteristic elements, such as Li, Rb, B and As, despite their relatively low or moderate TDS content (Elenga et al., 2021; Li et al., 2021). High concentrations of Rb, B, and Li in geothermal waters are often associated with magmatic hydrothermal fluids from regional crustal remelting rather than the desorption of these elements during water-rock processes (Brugger et al., 2005; Tassi et al., 2010).
In summary, as mentioned above, for Ca-Cl brine that is rich in B and Li, enrichment of B and Li elements is the result of the mixing of several sources. Among them, geothermal waters are the main source of water bodies rich in B and Li and play an important role in the enrichment and migration of B and Li elements. Geothermal waters not only dissolve salt deposits that formed earlier in the basin but also interact with surrounding rocks. Sometimes these waters mix with large amounts of magmatic hydrothermal fluids, thus adding B, Li, and other elements to the water bodies.
5.3.2 Indication of B and Li isotopes in Ca-Cl brines
The above analysis uncovers three possible ways for the source of B and Li in Ca-Cl brines in the study area. However, according to the distribution range of Li isotopes in Ca-Cl brines in the Qaidam Basin, they have a wide distribution (0.9‰–31.8‰; He et al., 2020; Li et al., 2021) and fall within that of rivers worldwide (6.1‰–43.0‰) (Figure 3; Huh et al., 1998; Vigier et al., 2009; Dellinger et al., 2015). It has been shown that silicate rocks are the main source of Li in rivers worldwide (Vigier et al., 2009; Dellinger et al., 2015). This implies that Li in Ca-Cl brines in the study area mainly originates from weathering of silicate rocks or water-rock exchange between atmospheric rainfall and silicates under suitable temperature-pressure conditions; an alternative origin is the mixing of magmatic hydrothermal fluids. Compared with the Li content in rivers, the Li content in Ca-Cl brines is higher. The available data indicate that an extremely low Li content in rivers will lead to great Li isotopic fractionation during migration (Pistiner and Henderson, 2003; Millot et al., 2010; Weynell et al., 2017). As rivers inevitably leach watershed rocks or other minerals, or may have new mineral precipitations, substantial isotopic fractionation results (Pistiner and Henderson, 2003; Millot et al., 2010; Godfrey et al., 2013; Weynell et al., 2017; Munk et al., 2018). This indication seems consistent with the wide range of Li isotopic distribution in Ca-Cl brines. The only way to reach high Li contents in water bodies under these conditions is to increase the Li element level in the water by evaporation (Li et al., 2021; Miao et al., 2022). However, such evaporation will lead to a corresponding increase in other elements (such as Br). The reason is that during evaporation, concentration, and deposition, Br elements tend to enter the Ca-Cl brine but the Br content in Ca-Cl type brine in the study area is very low (Li et al., 2021). Moreover, there is no significant positive correlation between Br, B, and Li elements in the Ca-Cl brine (Li et al., 2021). This indicates that the formation of these elements in Ca-Cl brine is not related to the evaporation and concentration of river migration. During epigenesis, the properties of Rb obstruct its entry into water bodies, which provides an explanation for its low content in surface water or normal salt lakes. However, the Rb content in the Ca-Cl brine of the Qaidam Basin is clearly elevated (Li et al., 2021), indicating that the formation of Ca-Cl brines is not strongly related to the genesis of rivers under silicate surface weathering. Furthermore, the results of element analysis also corroborate this viewpoint: the correlation between Na and Cl elements in the Ca-Cl brines is good enough, and the elemental ratio between them is basically 1. When a large amount of weathering material is involved, this correlation and the elemental ratio will certainly change with the continuous change of the ionic composition of the water bodies. Therefore, the effect of low-temperature weathering on the enrichment of Li contents in oilfield Ca-Cl brine is not very pronounced (He et al., 2020; Li et al., 2021).
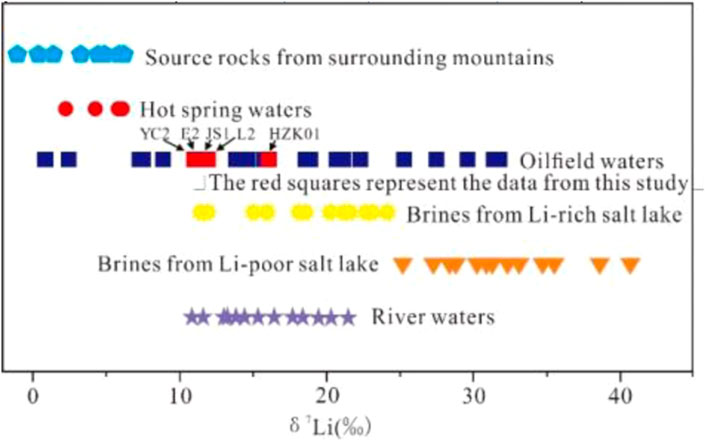
FIGURE 3. δ7Li values of various geological bodies in the Qaidam Basin: oilfield waters from the western Qaidam Basin (this study and Li et al., 2022b), source rocks from surrounding mountains (Weynell et al., 2017; He et al., 2020), hot spring waters (Xiao et al., 1994; Weynell et al., 2017; He et al., 2020), river waters (Xiao et al., 1994; He et al., 2020), Li-rich salt lake Ca-Cl brines (DaQaidam, Taijinar, and Bieletan salt lakes in the Qaidam Basin with Li contents exceeding 80 mg/L, Xiao et al., 1994; He et al., 2020), and Li-poor salt lake Ca-Cl brine (He et al., 2020; Li et al., 2021).
Previous studies have shown that subsurface hot water along faults driven by tectonic processes can provide large amounts of mineral elements (Tassi et al., 2010; Miao et al., 2022). Geothermal waters are special water bodies that form when fluids (such as atmospheric precipitation) infiltrate along regional fractures and fissures (He et al., 2020; Elenga et al., 2021). Over this process, these fluids capture large amounts of highly fluid-mobile elements after water-rock interaction with ore-bearing rocks under suitable temperature-pressure conditions or after mixing with magmatic hydrothermal fluids (Grimaud et al., 1985; Miao et al., 2022). This kind of water body tends to have a lower δ7Li value and high contents of B, Li and Rb, as well as other elements (Li et al., 2022a; Miao et al., 2022). These features of geothermal waters are similar to those of Ca-Cl brine in the study area. This similarity indicates that the water-rock interaction between fluids and ore-bearing rocks is one of the main reasons for the formation of Ca-Cl brine.
Generally, during epigenesis, the relative difficulty associated with the entry of Rb into water bodies results in a relatively low content of Rb in normal surface waters or salt lakes (Regenspurg et al., 2016; Li et al., 2021). However, the Rb content in the Ca-Cl brines in the study area is high (Li et al., 2021). Rb is one of the characteristic elements in geothermal water and has a certain correlation with B and Li in Ca-Cl brines (Thompson, 1983; Pang, 2009; Li et al., 2022b); on the one hand, this indicates that low temperature weathering is not the main reason for the formation of Ca-Cl brines; on the other hand, it also implies that water-rock interaction under suitable temperature-pressure conditions is an important mode of the formation of Ca-Cl brine with high contents of B and Li. When such a type of water body with a low δ7Li value and high levels of elements such as B, Li and Rb is mixed with fluids that are infiltrated by atmospheric precipitation, Ca-Cl brine can form. The conclusion of H and O isotope results further supports the conclusion that the water body in Ca-Cl brines originates from the mixing of atmospheric precipitation and deep fluids (Tan et al., 2011; Li, 2013).
Natural water bodies are rarely enriched with incompatible elements. Divalent ions, such as Ca2+, Mg2+ and Sr2+, sometimes also heavy metal ions, are enriched in groundwater bodies under strong water-rock reaction conditions (Elenga et al., 2021). However, elements such as B, Li, and Rb are not easily enriched in water bodies by water-rock interaction alone (Brugger et al., 2005); B, Li and Rb elements tend to have high levels in hot water (Elenga et al., 2021). This suggests that deep hot water recharge is an important mechanism for the formation of Ca-Cl brines in the western part of the basin. Judging from the characteristics of modern saline lakes, Li isotopes tend to present high values in Li-poor saline lakes (Figure 3; Li et al., 2021; He et al., 2020). These lakes exhibit low Li contents and high Li isotope levels during river recharge because of the low Li content of the river itself, which is further depleted via adsorption by clay minerals (Millot et al., 2010; Millot and Negrel, 2021; Garcia et al., 2020). In contrast, Li-rich salt lakes clearly exhibit low Li isotope values (Figure 3; Xiao et al., 1994; He et al., 2020). If Li elements in water bodies were recharged by normal river water alone, it would be difficult to achieve high Li enrichment because of the influence of clay mineral adsorption, and the Li isotope values would increase accordingly (He et al., 2020; Li et al., 2021; Miao et al., 2022). These phenomena further indicate that the enrichment of Li elements in Ca-Cl brines in the western part of this basin is the result of deep hot water recharge. Likewise, the B isotope values of Ca-Cl brines in the Qaidam Basin are ranging from −8.59 to 49.04 (this study and Li et al., 2022b). B-poor ancient brines have relatively high B-isotope values and a wide distribution range, while B-rich brines tend to have relatively low B isotope values (Figure 4; Xiao et al., 1999). Accordingly, the B isotope values of B-rich geothermal water in the Kunlun Mountains and Qilian Mountains have a low or very similar distribution range (Li et al., 2022a). Overall, when such B- and Li-rich subsurface hot water (possibly mixed with magmatic hydrothermal fluids) is mixed with brines, its B and Li isotope values must be lower than those of B- and Li-poor brines.
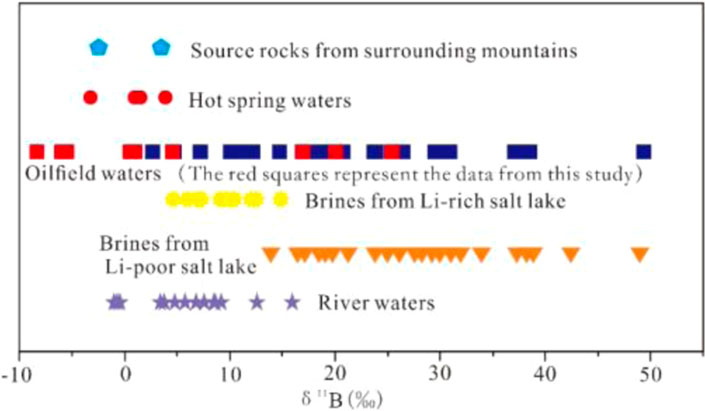
FIGURE 4. Δ11B values of various geological bodies in the Qaidam Basin; oilfield waters from the western Qaidam Basin (this study and Li et al., 2022b), source rocks from the surrounding mountains (Vengosh et al., 1995), hot spring waters (Vengosh et al., 1995; Xiao et al., 1999), river waters (Xiao et al., 1999), Li-rich salt-lake Ca-Cl brines (Xiao et al., 1999), Li-poor salt-lake Ca-Cl brines (Xiao et al., 1999).
This discussion above shows that the B and Li elements in the B- and Li-rich Ca-Cl brines of the western part of the Qaidam Basin originate from the hot water at the basin margin. B, Li, and other resource elements in this hot water originate not only from water-rock interaction under suitable temperature-pressure conditions, but also (partly) from the mixing of magmatic hydrothermal fluids.
5.4 Discussion on the Ca source in Ca-Cl brines in this inland basin
The genesis of Ca-Cl brines in inland basins has been studied before. The hydrochemical composition of this water body is rich in Ca2+ and poor in Mg2+; moreover, it contains small amounts of SO42− and HCO3−, and almost has no CO3- (Zhang et al., 1987; Lowenstein et al., 1989; Hardie, 1996; Lowenstein, 2003; Li et al., 2015). Ca-Cl brine is a special type of water body. Its hydrochemical composition is difficult to explain only by weathering between surrounding rock and atmospheric precipitation (river water), evaporation, and concentration on the Earth’s surface. However, it plays a crucial role in the formation of large ancient marine, rift, and terrestrial basin (Ca-Cl brine) potash deposits (Lowenstein et al., 1989; Hardie, 1990; Lowenstein et al., 2001).
The Qaidam Basin in China is a typical terrestrial salt-bearing basin; its western Tertiary strata are rich in Ca-Cl brines. How did this type of brine form? For the genesis of Ca-Cl brines, different scholars provided different explanations. Examples are the diagenetic transformation effect (albitization, dolomitization, and reduction), evaporation and concentration of ancient residual seawater, and hydrothermal genesis. Essentially, Ca-Cl brine is a water-salt system with high Ca2+ and low Mg2+ contents, suggesting that in nature, Ca-Cl brine is relatively enriched in Ca2+. According to the chemical composition as well as H, O, Sr, S, and B isotope data, scholars proposed that the water bodies in the Ca-Cl brine in the Qaidam Basin show characteristics of atmospheric rainfall and are mixed with deep fluids in the inner Earth (Tan et al., 2011; Li, 2013; Li et al., 2021). The hydrogeological data and B-Li isotope analysis presented above show that the B and Li elements in Ca-Cl brine have clearly originated from water-rock interaction at the basin margin. However, the main share of Na+ and Cl− in the Ca-Cl brine originates from the dissolution of existing evaporites in the basin during the migration of the water body. These findings clearly indicate that there are two origins of the relatively abundant Ca2+ in Ca-Cl brines in the western part of the Qaidam Basin: It can originate from the contribution of dissolution of the existing evaporites; It can also originate from the magmatic hydrothermal fluids contributed by the water-rock reaction under suitable temperature-pressure conditions in the deep part of the basin margin and the magmatic hydrothermal fluids in the orogenic belt of the basin margin during the orogenic period. In fact, the Ca2+ in Ca-Cl brine that originates exclusively from the dissolution of existing evaporites in the basin is often in balance with CO3− and SO42− in the evaporites. Consequently, the formation of Ca-Cl brines is prevented, and additional non-equilibrium Ca2+ input is required to allow the Ca-Cl brine to evolve into Ca-Cl type water bodies. Therefore, Ca2+ present in magmatic hydrothermal fluids contributed by water-rock interaction under appropriate temperature-pressure conditions at the deep basin margin and by magmatism at the basin margin orogenic belt during the orogenic period may be an important mechanism for the formation of this particular type of water. The results of ion correlation analysis further support this conclusion as correlation coefficients are 0.90 and 0.77 between Ca2+ and Li+, as well as between Ca2+ and Sr2+, respectively.
In the history of Ca-Cl brine research, Carpenter (1978) was the first to suggest that Ca-Cl brines in the basin were originally derived from marine evaporite. Carpenter further suggested that these Ca-Cl brines underwent a series of water-rock interactions with the surrounding rocks at normal temperature-pressure conditions during their burial, thus, resulting in Ca-Cl brines. However, the results of computer simulations indicate that the chemical composition of Ca-Cl brines that formed under such conditions differs significantly from that of Ca-Cl brines (Hanor, 1994). Therefore, Lowenstein. (2003) and Bottomley et al. (2005) re-evaluated the genesis of Silurian-Devonian Ca-Cl brines in the Illinois Basin in the United States and the western basin of Canada. Their results suggest that the Ca-Cl brines in these areas mainly inherited the chemical composition of Silurian-Devonian paleo seawater from a Ca-Cl type sea (Lowenstein et al., 2001; Holt et al., 2014), rather than being led by later water-rock interactions and transformation of hydrochemical characteristics. These studies highlight the importance of the chemical composition of paleo seawater with high Ca2+ and low Mg2+ contents in the Ca-Cl sea for the formation of Ca-Cl brines in the basin (Hardie, 1996; Lowenstein et al., 2001; Lowenstein, 2003; Bottomley et al., 2005). However, the Qaidam Basin in China is a typical terrestrial basin without residual or recharge of paleo seawater during its formation (Zhang et al., 1987). This rules out the possibility that Ca-Cl brines in the Qaidam Basin were formed by the diagenetic transformation effect (i.e., albitization, dolomitization, and reduction) and the evaporation and concentration of ancient residual seawater.
The concept that Ca-Cl brines are the cause of hydrothermal fluids has been proposed by many scholars (Hardie, 1990; Lowenstein and Risacher, 2008; Tan et al., 2011; Lowenstein et al., 2016). Hardie (1990) showed that Ca-Cl hydrothermal fluids that are rich in K+, B3+, and Li+ are distributed at mid-ocean ridges or terrestrial tectonic activity zones. Such hydrothermal fluids are driven by thermal anomalies or topographic dynamics to rise along fracture zones and emerge at the surface to form thermal springs or salt Ca-Cl brines. Example are areas such as the Reykjanes Ridge in Iceland (Bjornsson et al., 1972), the Salton Trough in the United States (Thompson, 1983; Hardie, 1990), and the Red Sea (Brewer and Spencer, 1969). In these areas, thermal Ca-Cl brine reacts under high temperature (100°C–400°C) conditions between seawater and ocean floor basalts and forms serpentinized peridotites and Ca-Cl hydrothermal Ca-Cl brines (Hardie, 1990). In terrestrial tectonic activity zones, deep-source hydrothermal fluids participate in and may be accompanied by albitization, chlorination, and epidotization, thus forming Ca-Cl brines (Hardie, 1990). Elemental composition and isotopic analyses by Tan et al. (2011) showed that both Ordovician thermal Ca-Cl brines in the central Tarim Basin and Tertiary oilfield Ca-Cl brines in the western Qaidam Basin have a deep source hydrothermal mixing genesis. Petrographic thin section studies and the components analysis of fluid inclusions in halite suggest that mantle-derived Ca-Cl hydrothermal fluids exert an important influence on the evolution of Ca-Cl brines and mineral assemblage therein (Lowenstein et al., 2016). The basement of the Qaidam basin is a relatively stable terrene (Tang et al., 2000), and decades of research have not found any clear records of hydrothermal activity in the interior of the basin. Therefore, the water-rock interaction in the deep part of the basin margin and the recharge and mixing of magmatic hydrothermal fluids during the orogenic period are important mechanisms for the formation of Ca-Cl brines in this basin. The conclusion of this study is important for a better understanding of the genesis of Ca-Cl brines in the western part of the Qaidam Basin.
6 Ore-forming model of Ca-Cl brines in the western Qaidam Basin
Research on the genesis of any kind of brine generally involves contents such as the origin of the water body, the origin of the elements, and its final migration, transformation, and positioning processes (Zhai, 2020). From the existing findings of Ca-Cl brine in the Qaidam Basin, the H and O isotope evidence indicates that this Ca-Cl brine originated from atmospheric rainfall and the mixing of magmatic hydrothermal fluids (Tan et al., 2011; Li, 2013). Moreover, He isotope and other research findings also show that this Ca-Cl brine has the characteristics of a mantle source (Li H. P. et al., 2022). This indicates that Ca-Cl brines in the western Qaidam Basin have characteristics of multi-source fluid mixing. The hydrochemical characteristics of each element in this Ca-Cl brine show that the Na and Cl elements mainly originate from the dissolution of a prior evaporite inner basin. The B and Li isotopes indicate that the source is related to water-rock interaction under suitable temperature-pressure conditions.
In fact, the Sr element in Ca-Cl brines also has a similar source with B and Li; all these elements have high mobility in fluids and show a certain similarity in geochemical properties. The correlation coefficients between Li and B, as well as between Li and Sr elements in Ca-Cl brines can reach 0.51 and 0.57, respectively, and prior studies proved this inference (Tan et al., 2011). Therefore, the Ca-Cl brine in the western Qaidam Basin shows a “binary structure” characteristic. This means that the main elements in the Ca-Cl brine often originated from the dissolution of existing evaporite in the basin, while the elements of B, Li, and Sr often originated from water-rock interaction under a suitable depth in the basin margin. Sometimes, the fluids of deep magmatic hydrothermal activity that formed in the surrounding orogenic zones mix.
The available seismic data confirm the existence of a deep detachment system and numerous fracture structures in the basin margin, and borehole data also confirmed that the anticline and fault structures in the western part of the Qaidam Basins are rich in Ca-Cl brine (Li et al., 2015). When atmospheric rainfall infiltrates along the fractures and faults in the basin margin, water-rock interaction with the ore-bearing mother rocks in the deep part of the basin margin happens under suitable temperature-pressure conditions. This interaction can wash out a large number of elements such as B, Li, Sr, and Ca, while at the same time, certain deep magmatic hydrothermal fluids mix during this process. When these ore-bearing fluids are transported along the fractures and fissures in the basin, they dissolve a large number of Tertiary evaporites in the western part of the Qaidam Basin and are then preserved in these suitable structural sites (anticline and fault structures), thus, forming the Ca-Cl brine in the western Qaidam Basin (Figure 5).
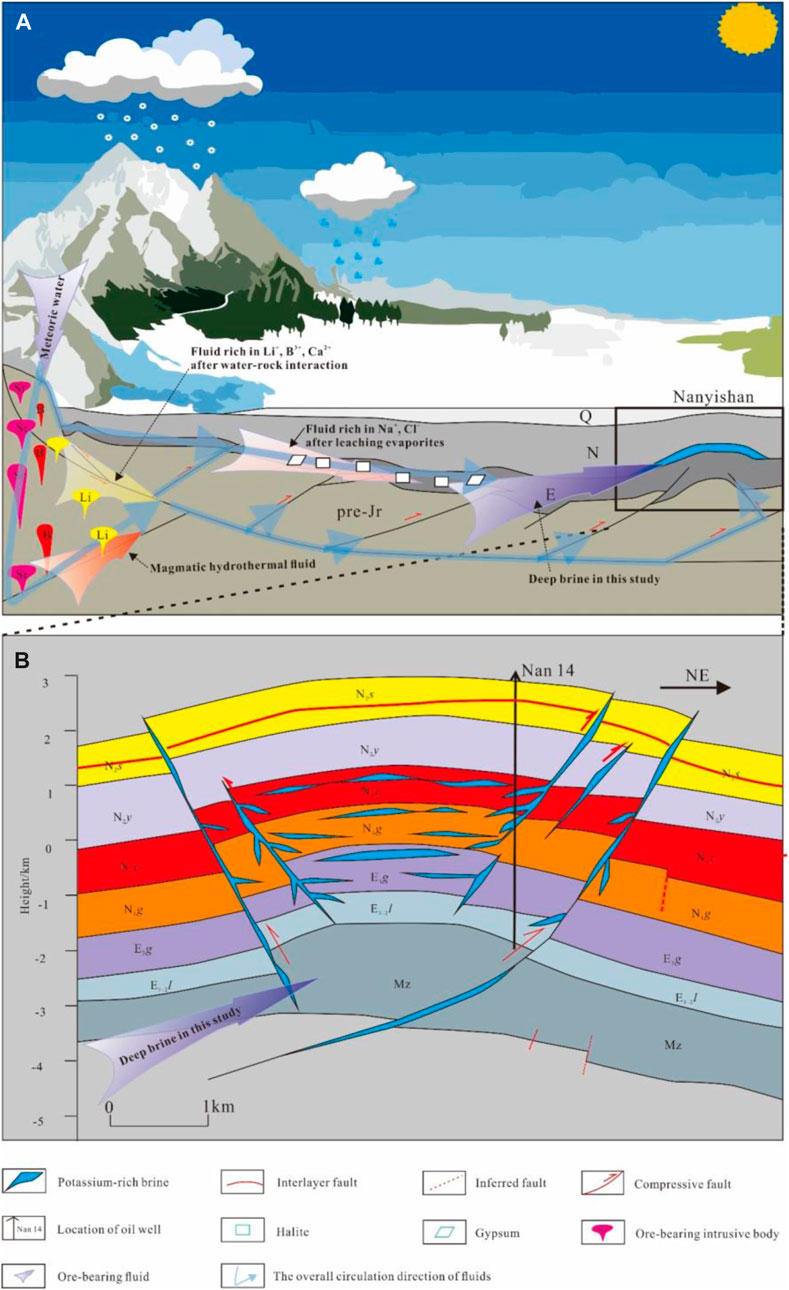
FIGURE 5. Schematic model, illustrating Ca-Cl brine formation processes in the western Qaidam Basin.
7 Conclusion
The origin of Ca-Cl brines has long been an object of scholarly discussion. As a typical inland basin, the origin of Ca-Cl brines has yet to be fully understood. Based on field samples, the characteristics of the Ca-Cl brine source, the genetic mechanism, and the metallogenic model are systematically proposed:
1) The source of Ca-Cl brines in the Qaidam Basin shows a “binary structure” characteristic. This means that the main elements (Na and Cl) often originate from the dissolution of the existing evaporite; B, Li, Sr, and other highly fluid-mobile elements often originate from water-rock interaction under the influence of hot water at a suitable depth in the basin margin; sometimes, the fluids of deep magmatic hydrothermal activity mix in the surrounding orogenic zones.
2) Through studying B and Li isotopes, combined with the actual geological characteristics of the basin, the genetic mechanism of Ca-Cl brines in the western Qaidam Basin is systematically explored, and a new model of Ca-Cl brine formation is established: Under suitable temperature-pressure conditions at the basin margin, Ca from water-rock interaction and that from hydrothermal fluids (contributed by the magmatic activity of surrounding orogenic belts) is key to the formation of this special type of Ca-Cl brine.
3) Through the analysis of hydrochemical data as well as B and Li isotopes, a model of Ca-Cl brine formation in the western Qaidam Basin is systematically established: In the process of atmospheric rainfall infiltration along basin margin fractures, the water body interacts with deep ore-containing mother rocks in the basin margin under suitable temperature-pressure conditions. This leads to the wash out of considerable amounts of B, Li, Sr, Ca, and other elements from the rocks. At the same time, in this process, certain magmatic hydrothermal fluids are mixed. When the ore-bearing fluid moves along the fracture and fissure in the basin, many Tertiary evaporites that remained in the western Qaidam basin are dissolved and then moved and preserved in certain appropriate structural sites (western anticline structures); consequently, Ca-Cl brines formed in the west of the Qaidam Basin.
Data availability statement
The original contributions presented in the study are included in the article/Supplementary Material, further inquiries can be directed to the corresponding author.
Author contributions
Conceptualization, YL and TP; methodology, WH; software, HL; validation, HM and HC; formal analysis, YL; investigation, YL, TP, HL, BL, XM, PZ, and HM; resources, YL; data curation, YL; writing—original draft preparation, YL; writing—review and editing, YL, PZ, TP, HL, HC, BL, and WH; visualization, WH and QY; supervision, HM; project administration, HM; funding acquisition, YL. All authors contributed to the article and approved the submitted version.
Funding
This research was funded by the Youth Program of the National Natural Science Foundation of China (41402082), the Qinghai Scholars Program of Qinghai Province (QHS201802), and the second Tibetan Plateau Scientific Expedition and Research (2019QZKK0806).
Conflict of interest
The authors declare that the research was conducted in the absence of any commercial or financial relationships that could be construed as a potential conflict of interest.
Publisher’s note
All claims expressed in this article are solely those of the authors and do not necessarily represent those of their affiliated organizations, or those of the publisher, the editors and the reviewers. Any product that may be evaluated in this article, or claim that may be made by its manufacturer, is not guaranteed or endorsed by the publisher.
Supplementary material
The Supplementary Material for this article can be found online at: https://www.frontiersin.org/articles/10.3389/fenvs.2023.1248294/full#supplementary-material
References
Bjornsson, S., Arnorsson, S., and Tomasson, J. (1972). Economic evaluation of Reykjanes thermal brine area. Iceland. doi:10.1016/0041-1647(71)90038-4
Bottomley, D. J., Clark, I. D., Battye, N., and Kotzer, T. (2005). Geochemical and isotopic evidence for a genetic link between Canadian Shield brines, dolomitization in the Western Canada Sedimentary Basin, and Devonian calcium-chloridic seawater. Can. J. Earth Sci. 42, 2059–2071. doi:10.1139/E05-075
Brewer, P. G., and Spencer, D. W. (1969). “A note on the chemical composition of the Red Sea brines,” in Hot brines and recent heavy metal deposits in the Red Sea. Editors E. T. Degens, and D. A. Ross (New York: Springer-Verlag), p, 174–p179.
Brugger, J., Long, N., McPhail, D. C., and Plimer, I. (2005). An active amagmatic hydrothermal system: the Paralana hot springs, Northern Flinders Ranges, South Australia. Chem. Geol. 222, 35–64. doi:10.1016/j.chemgeo.2005.06.007
Canadian Shield brines Clark, I. D., Battye, N., and Kotzer, T. (2005). Geochemical and isotopic evidence for a genetic link between Canadian Shield brines, dolomitization in the Western Canada Sedimentary Basin, and Devonian calcium-chloridic seawater. Can. J. Earth Sci. 42, 2059–2071. doi:10.1139/e05-075
Carpenter, A. B. (1978). “Origin and chemical evolution of brines in sedimentary basins,” in Thirteenth annual forum on the geology of industrial minerals: Oklahoma Geological Survey Circular. Editors K. S. Johnson, and J. A. Russell, 79, 60–77.
Cullen, J. T., Hurwitz, S., Barnes, J. D., Lassiter, J. C., Penniston-Dorland, S., Kasemann, S. A., et al. (2019). Temperature-dependent variations in mineralogy, major element chemistry and the stable isotopes of boron, lithium and chlorine resulting from hydration of rhyolite: constraints from hydrothermal experiments at 150 to 350 °C and 25 MPa. Geochimica Cosmochimica Acta 261, 269–287. doi:10.1016/j.gca.2019.07.012
Dai, J. S., and Cao, D. Y. (2000). Evolution characteristics of Cenozoic structural style in the Qaidam basin. Geol. Rev. 46, 455–460. (in Chinese with English abstract).
Dang, Y. Q., Zhang, D. W., Xu, Z. Y., and Hou, Z. S. (2004). Sedimentary facies and biogenic gas pool of the Quaternary of Sanhu area in Qaidam Basin. J. Palaeogeogr. 6, 110–118.
Dellinger, M., Bouchez, J., Gaillardet, J., Faure, L., and Moureau, J. (2017). Tracing weathering regimes using the lithium isotope composition of detrital sediments. Geology 45, 411–414. doi:10.1130/g38671.1
Dellinger, M., Gaillardet, J., Bouchez, J., Calmels, D., Louvat, P., Dosseto, A., et al. (2015). Riverine Li isotope fractionation in the Amazon River basin controlled by the weathering regimes. Geochimica Cosmochimica Acta 164, 71–93. doi:10.1016/j.gca.2015.04.042
Elenga, H. I., Tan, H. B., Su, J. B., and Yang, J. Y. (2021). Origin of the enrichment of B and alkali metal elements in the geothermal water in the Tibetan Plateau: evidence from B and Sr isotopes. Geochemistry 81, 125797–125815. doi:10.1016/j.chemer.2021.125797
Feng, Z. J., Zhao, Y. S., Zhou, A. C., and Zhang, N. (2012). Development program of hot dry rock geothermal resource in the Yangbajing Basin of China. Renew. Energy 39, 490–495. doi:10.1016/j.renene.2011.09.005
Flesch, G. D., Anderson, A. R., and Svec, H. J. (1973). A secondary isotopic standard for 6Li/7Li determinations. Int. J. Mass Spectrom. Ion. Phys. 12, 265–272. doi:10.1016/0020-7381(73)80043-9
Garcia, M. G., Borda, L. G., Godfrey, L. V., Steinmetz, R. L. L., and Losada-Calderon, A. (2020). Characterization of lithium cycling in the Salar De Olaroz, Central Andes, using a geochemical and isotopic approach. Chem. Geol. 531, 119–340. doi:10.1016/j.chemgeo.2019.119340
Godfrey, L. V., Chan, L. H., Alonso, R. N., Lowenstein, T. K., McDonough, W. F., Houston, J., et al. (2013). The role of climate in the accumulation of lithium-rich brine in the Central Andes. Appl. Geochem. 38, 92–102. doi:10.1016/j.apgeochem.2013.09.002
Gou, L. F., Jin, Z., Pogge von Strandmann, P. A. E., Li, G., Qu, Y. X., Xiao, J., et al. (2019). Li isotopes in the middle Yellow River: seasonal variability, sources and fractionation. Geochimica Cosmochimica Acta 248, 88–108. doi:10.1016/j.gca.2019.01.007
Gou, L. F., Jin, Z. D., Deng, L., He, M. Y., and Liu, C. Y. (2018). Effects of different cone combinations on accurate and precise determination of Li isotopic composition by MC-ICP-MS. Spectrochim. Acta Part B At. Spectrosc. 146, 1–8. doi:10.1016/j.sab.2018.04.015
Grimaud, D., Huang, S., Michard, G., and Zheng, K. (1985). Chemical study of geothermal waters of central Tibet (China). Geothermics 14, 35–48. doi:10.1016/0375-6505(85)90092-6
Guo, P., Liu, C. Y., Huang, L., Yu, M. L., Wang, P., and Zhang, G. Q. (2018). Palaeohydrological evolution of the late Cenozoic saline lake in the Qaidam Basin, NE Tibetan Plateau: tectonic vs. climatic control. Glob. Planet. Change 165, 44–61. doi:10.1016/j.gloplacha.2018.03.012
Hanor, J. S. (1994). “Origin of saline fluids in sedimentary basins,” in Geofluids: origin, migration and evo lution of fluids in sedimentary basins. Editor J. Parnell (London: Geological Society [London] Special Publication), 78, 151–174.
Hardie, L. A. (1990). The roles of rifting and hydrothermal CaCl2 brines in the origin of potash evaporites: an hypothesis. Am. J. Science. Journal Sci. 290, 43–106. doi:10.2475/ajs.290.1.43
Hardie, L. A. (1996). Secular variation in seawater chemistry: an explanation for the coupled secular variation in the mineralogies of marine limestones and potash evaporites over the past 600 m.y. Y. Geol. 24, 279–283. doi:10.1130/0091-7613(1996)024<0279:svisca>2.3.co;2
Hardie, L. A., and Eugster, H. P. (1970). “The evolution of closed-basin brines,” in Fiftieth anniversary symposia: mineralogy and petrology of the upper mantle, sulfides, mineralogy and geochemistry of non-marine evaporites. Editor B. A. Morgan (USA: Miner Soc Am Spec Pap), 3, 273–290.
He, M. Y., Luo, C. G., Yang, H. J., Kong, F. C., Li, Y. L., Deng, L., et al. (2020). Sources and a proposal for comprehensive exploitation of lithium brine deposits in the Qaidam Basin on the northern Tibetan Plateau, China: evidence from Li isotopes. Ore Geol. Rev. 117, 103277. doi:10.1016/j.oregeorev.2019.103277
Holt, N. M., García-Veigas, J., Lowenstein, T. K., Giles, P. S., and Williams-Stroud, S. (2014). The major-ion composition of Carboniferous seawater. Geochimica Cosmochimica Acta 134, 317–334. doi:10.1016/j.gca.2014.03.009
Huh, Y., Chan, L., Zhang, L., and Edmond, J. M. (1998). Lithium and its isotopes in major world rivers: implications for weathering and the oceanic budget. Geochimica Cosmochimica Acta 62, 2039–2051. doi:10.1016/s0016-7037(98)00126-4
Li, H. P., Pan, T., Li, Y. S., Jin, F., Han, G., and Wang, G. C. (2022a). Geochemical composition and origin tracing of structural fiss ure and pore brine in Western Qaidam Basin. Earth Science-Journal China Univ. Geosciences 47 (1), 36–47. (in Chinese with English abstract). doi:10.3799/dqkx.2021.225
Li, H. P., Zheng, M. P., Hou, X. H., and Yan, L. J. (2015). Control factors and water chemical characteristics of potassium-rich deep brine in Nanyishan structure of western Qaidam Basin. Acta Geosci. Sin. 36, 41–50. doi:10.3975/cagsb.2015.01.05
Li, J. S. (2013). The chemical and isotope characteristics of the Tertiary oilfield brine in the western Qaidam Basin and its origin and evolution. Germany: The University of Chinese Academy of Sciences, 52. (in Chinese with English abstract).
Li, J. S., Cai, J. F., Fan, Q. S., Shan, F. S., Ling, Z. Y., Han, Y. H., et al. (2022b). Metallogenic geochemical system of K, B and Li resources in salt lakes of Qaidam Basin. J. Salt Lake Res. 30, 12–20. (in Chinese with English abstract). doi:10.12119/j.yhyj.202203002
Li, J. S., Chen, F. K., Ling, Z. Y., and Li, T. W. (2021). Lithium sources in oilfield waters from the Qaidam Basin, Tibetan Plat eau: geochemical and Li isotopic evidence. Ore Geol. Rev. 139, 104481. doi:10.1016/j.oregeorev.2021.104481
Li, J. S., Li, T. W., Peng, X. M., Han, Y. H., Li, Z. P., and Ma, H. Z. (2014). Hydrogeochemical behaviors of oilfield water in the Tertiary in western Qaidam Basin. Oil Gas Geol. 35, 50–56. (in Chinese with English abstract). doi:10.11743/ogg20140107
Li, T. W. (2007). The origin analysis by hydro-chemical characters and Sr isotope of oil field brines in west of Qaidam Basin. Graduate Sch. Of Chin. Acad. Of Sci., 48. (in Chinese with English abstract).
Liu, C. L., Yu, X. C., Yuan, X. Y., Li, R. Q., Yao, F. J., Shen, L. J., et al. (2021). Characteristics, distribution regularity and formation model of brine-type Li deposits in salt lakes in the world. Acta Geol. Sin. 95, 2009–2029. (in Chinese with English abstract). doi:10.19762/j.cnki.Dizhixuebao.2021230
Liu, X. X., Yue, X., Yuan, W. H., Fan, Z. L., Yu, X. L., Dong, Q. W., et al. (2019). Hydrochemical characteristics and evolutionary process of deep brines from Shizigou anticline structure in Qaidam Basin, China. J. Salt Lake Res. 27, 73–81. (in Chinese with English abstract). doi:10.12119/j.yhyj.201901008
Lowenstein, T. K., Dolginko, L. A. C., and García-Veigas, J. (2016). Influence of magmatic-hydrothermal activity on brine evolution in closed basins: searles Lake, California. Geol. Soc. Am. Bull. 128, 1555–1568. doi:10.1130/b31398.1
Lowenstein, T. K., Hardie, L. A., Timofeeff, M. N., and Demicco, R. V. (2003). Secular variation in seawater chemistry and the origin of calcium chloride basinal brines. Geology 31, 857–860. doi:10.1130/g19728r.1
Lowenstein, T. K., and Risacher, F. (2008). Closed Basin brine evolution and the influence of Ca–Cl inflow waters: death valley and bristol dry lake California, Qaidam Basin, China, and salar de Atacama, Chile. Aquat. Geochem. 15, 71–94. doi:10.1007/s10498-008-9046-z
Lowenstein, T. K., Spencer, R. J., and Zhang, P. X. (1989). Origin of ancient potash evaporites: clues from the modern nonmarine Qaidam Basin of western China. Science 245, 1090–1092. doi:10.1126/science.245.4922.1090
Lowenstein, T. K., Timofeeff, M. N., Brennan, S. T., Hardie, L. A., and Demicco, R. V. (2001). Oscillations in Phanerozoic seawater chemistry: evidence from fluid inclusions. Science 294, 1086–1088. doi:10.1126/science.1064280
Luo, Q., and Pang, X. Q. (2003). The characteristics of fault system and its control role to petroleum zone in Qaidam Basin. J. southwest petrleum Inst. 25, 1–6.
Ma, Y. Q., Xiao, Y. K., He, M. Y., Xiao, J., Shen, Q., and Jiang, S. X. (2011). Boron isotopic composition of Paleozoic brachiopod and coeval coral calcites in Yunnan-Guizhou Plateau, China. Sci. China Earth Sci. 54, 1912–1925. doi:10.1007/s11430-011-4263-5
Miao, W. L., Zhang, X. Y., Li, Y. L., Li, W. X., Yuan, X. L., and Li, C. Z. (2022). Lithium and strontium isotopic systematics in the Nalenggele River catchment of Qaidam basin, China: quantifying contributions to lithium brines and deciphering lithium behavior in hydrological processes. J. Hydrology 614, 128630–128714. doi:10.1016/j.jhydrol.2022.128630
Millot, R., and Négrel, P. (2021). Lithium isotopes in the Loire River Basin (France): hydrogeochemical characterizations at two complementary scales. Appllied Geochem. 125, 104831–831. doi:10.1016/j.apgeochem.2020.104831
Millot, R., Vigier, N., and Gaillardet, J. (2010). Behaviour of lithium and its isotopes during weathering in the Mackenzie Basin, Canada. Geochimica Cosmochimica Acta 74, 3897–3912. doi:10.1016/j.gca.2010.04.025
Munk, L. A., Boutt, D. F., Hynek, S. A., and Moran, B. J. (2018). Hydrogeochemical fluxes and processes contributing to the forma tion of lithium-enriched brines in a hyper-arid continental basin. Chem. Geol. 493, 37–57. doi:10.1016/j.chemgeo.2018.05.013
Nie, J. S., Ren, X. P., Saylor, J. E., Su, Q. D., Horton, B. K., Bush, M. A., et al. (2019). Magnetic polarity st ratigraphy, provenance, and paleoclimate analysis of Cenozoic strata in the Qaidam Basin, NE Tibetan Plateau. GSA Bull. 132, 310–320. doi:10.1130/b35175.1
Pang, X. P. (2009). A study of the hot springs water chemistry and sinter deposition in the Bukedaban, Hoh Xil region (Qinghai: University of Chinese Academy of Sciences), 24–26. Thesis Master.
Pistiner, J. S., and Henderson, G. M. (2003). Lithium-isotope fractionation during continental weathering processes. Earth Planet. Sci. Lett. 214, 327–339. doi:10.1016/s0012-821x(03)00348-0
Qinghai Institute of Salt Lakes (1988). The introduction to analyzing methods of brines and salt deposits. 2nd Edn. Beijing: Science Press, 29–71. (in Chinese).
Regenspurg, S., Feldbusch, E., Norden, B., and Tichomirowa, M. (2016). Fluid-rock interactions in a geothermal rotliegend/permo-carboniferous reservoir (north German basin). Appl. Geochem. 69, 12–27. doi:10.1016/j.apgeochem.2016.03.010
Risacher, F., Alonso, H., and Salazar, C. (2003). The origin of brines and salts in Chilean salars: a hydrochemical review. Earth-Science Rev. 63, 249–293. doi:10.1016/s0012-8252(03)00037-0
Risacher, F., and Fritz, B. (2008). Origin of salts and brine evolution of Bolivian and Chilean salars. Aquat. Geochem. 15, 123–157. doi:10.1007/s10498-008-9056-x
Rissmann, C., Leybourne, M., Benn, C., and Christenson, B. (2015). The origin of solutes within the groundwaters of a high Andean aquifer. Chem. Geol. 396, 164–181. doi:10.1016/j.chemgeo.2014.11.029
Shi, H. Y., Miao, W. L., Tang, Q. L., Zhang, X. Y., and Li, Y. S. (2018). Geochemical characteristics and ore-forming material source of celestite deposits in Dafeng Mountain, Northwestern Qaidam Basin. Acta Geologica Sinica 92, 1733–1752. (in Chinese with English abstract).
Su, N. N., Jin, Z. K., Song, F., Gu, J. F., Chen, Y., and Zhang, W. D. (2014). Sedimentary facies of the Paleogene in Qaidam basi n. J. China Univ. Petroleum 38, 1–9. doi:10.3969/j.issn.1673-5005.2014.03.001
Sun, Z. M., Yang, Z. Y., Ge, X. H., Pei, J. L., Guo, X. C., Li, W. M., et al. (2004). Advances in the study of the Paleogene magnetotratigraphy on the northwestern margin of the Qaidam basin. Geol. Bull. China 23, 899–902.
Tan, H. B., Cao, C. D., Li, T. W., and Fan, Q. S. (2007). Hydrochemistry characteristics and chemical evolution of oilfield brines of the Paleogene and Neogene in western Qaidam Basin. J. Palaeogeogr. 6, 313–319. (in Chinese with English abstract).
Tan, H. B., Chen, J., Rao, W. B., Zhang, W. J., and Zhou, H. F. (2012). Geothermal constraints on enrichment of boron and lithium in salt lakes: an example from a river-salt lake system on the northern slope of the eastern Kunlun Mountains, China. J. Asian Earth Sci. 51, 21–29. doi:10.1016/j.jseaes.2012.03.002
Tan, H. B., Rao, W. B., Ma, H. Z., Chen, J. S., and Li, T. E. (2011). Hydrogen, oxygen, helium and strontium isotopic constraints on the formation of oilfield waters in the western Qaidam Basin, China. J. Asian Earth Sci. 40, 651–660. doi:10.1016/j.jseaes.2010.10.018
Tang, L. J., Jin, Z. J., Zhang, M. L., Liu, C. Y., Wu, H. N., You, F. B., et al. (2000). An analysis on tectono-paleogeography of the Qaidam basin, NW China. Earth Sci. Front. 7, 421–429.
Tassi, F., Aguilera, F., Darrah, T., Vaselli, O., Capaccioni, B., Poreda, R. J., et al. (2010). Fluid geochemistry of hydrothermal systems in the Arica-Parinacota, Tarapacá and Antofagasta regions (northern Chile). J. Volcanol. Geotherm. Res. 192, 1–15. doi:10.1016/j.jvolgeores.2010.02.006
Thompson, G. (1983). “Hydrothermal fluxes in the ocean,” in Chemical oceanography. Editors J. P. Riley, and R. Chester (New York: Academic Press), 272–338.
Vengosh, A., Chivas, A. R., Starinsky, A., Kolodny, Y., Zhang, B. Z., and Zhang, P. x. (1995). Chemical and boron isotope compositions of non-marine brines from the Qaidam Basin, Qinghai, China. Chem. Geol. 120, 135–154. doi:10.1016/0009-2541(94)00118-r
Vigier, N., Gislason, S. R., Burton, K. W., Millot, R., and Mokadem, F. (2009). The relationship between riverine lithium isoto pe composition and silicate weathering rates in Iceland. Earth Planet. Sci. Lett. 287, 434–441. doi:10.1016/j.epsl.2009.08.026
Walter, L. M., Stueber, A. M., and Huston, T. J. (1990). Br-Cl-Na systematics in Illinois basin fluids: constraints on fluid origin and evolution. Geology 18, 315–318. doi:10.1130/0091-7613(1990)018<0315:bcnsii>2.3.co;2
Weynell, M., Wiechert, U., and Schuessler, J. A. (2017). Lithium isotopes and implications on chemical weathering in the catchment of Lake Donggi Cona, northeastern Tibetan Plateau. Geochimica Cosmochimica Acta 213, 155–177. doi:10.1016/j.gca.2017.06.026
Xiao, Y. K., Qi, H. P., Wang, Y. H., and Jin, L. (1994). Lithium isotopic compositions of brine, sediments and source water in Da Qaidam lake, Qinghai, China. Geochimica 23, 329–338. (in Chinese with English abstract). doi:10.19700/j.0379-1726.1994.04.003
Xiao, Y. K., Shirodkar, P. V., Liu, W. G., Wang, Y. H., and Jin, L. (1999). Boron isotopes geochemistry in salt lake, Qaidam Basin, Qinghai. Prov. Prog. Chin. Nat. Sci. 9 (7), 612–618. (in Chinese with English abstract).
Yin, A., Dang, Y. Q., Chen, X. H., Wang, L. Q., Jiang, W. M., Jiang, R. B., et al. (2007). Cenozoic evolution and tectonic reconstruction of the Qaidam basin: evidence from seismic profiles. J. geomechanics 13, 193–211. (in Chinese with English abstract).
Zeng, X., Lin, T., Zhou, F., Li, J., Zhang, Y., Shen, X. S., et al. (2021). Carbon and oxygen isotope characteristics of carbonate and Neogene depositional environment in the Yiliping area of Qaidam Basin. Nat. Gas. Geosci. 32, 73–85. doi:10.11764/j.issn.1672-1926.2020.09.005
Zhai, Y. S. (2020). On the method of thinking in studying mineral deposits. Earth Sci. Front. 27, 1–12. doi:10.13745/j.esf.sf.2020.3.6
Zhang, P. X. (1987). Salt Lake of Qaidam Basin. Beijing: Scientical Publishing House, 47–73. (in Chinese with English abstract).
Keywords: Ca-Cl brine, Li-B isotope, origin of Ca-Cl brine, inland basin, ore-forming model, Qaidam Basin, Qinghai-Tibetan Plateau
Citation: Li Y, Pan T, Li H, Cheng H, Zhang P, Han W, Li B, Yuan Q, Ma X and Ma H (2024) Source and genesis of Ca-Cl type brines in Qaidam Basin, Qinghai-Tibetan Plateau: evidence from hydrochemistry as well as B and Li isotopes. Front. Environ. Sci. 11:1248294. doi: 10.3389/fenvs.2023.1248294
Received: 27 June 2023; Accepted: 20 November 2023;
Published: 04 January 2024.
Edited by:
Daniel D. Snow, University of Nebraska-Lincoln, United StatesReviewed by:
Sabarathinam Chidambaram, Kuwait Institute for Scientific Research, KuwaitLiang Qiu, China University of Geosciences, China
Hua-Wen Cao, Chengdu University of Technology, China
Copyright © 2024 Li, Pan, Li, Cheng, Zhang, Han, Li, Yuan, Ma and Ma. This is an open-access article distributed under the terms of the Creative Commons Attribution License (CC BY). The use, distribution or reproduction in other forums is permitted, provided the original author(s) and the copyright owner(s) are credited and that the original publication in this journal is cited, in accordance with accepted academic practice. No use, distribution or reproduction is permitted which does not comply with these terms.
*Correspondence: Yongshou Li, yongshouli@isl.ac.cn; Tong Pan, pant66@163.com