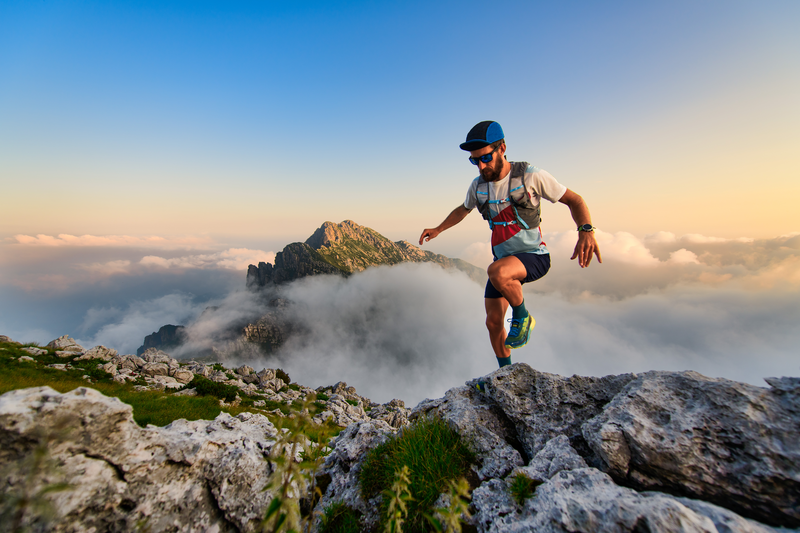
94% of researchers rate our articles as excellent or good
Learn more about the work of our research integrity team to safeguard the quality of each article we publish.
Find out more
ORIGINAL RESEARCH article
Front. Environ. Sci. , 04 September 2023
Sec. Environmental Systems Engineering
Volume 11 - 2023 | https://doi.org/10.3389/fenvs.2023.1248188
This article is part of the Research Topic Environmental Impacts of Materials and their Life Cycle View all 9 articles
In this study, the usability of the mixtures consisting of lignite coal taken from Tekirdag region and marble and travertine wastes taken from the marble factory in Gumushane region in local bean (Kelkit) cultivation was researched. First, control samples were created using only humus soil without coal, marble, or travertine dust. Then, for each coal or coal waste, coal/coal waste—waste marble dust and coal/coal waste—travertine dust mixtures were formed. These mixtures were obtained by mixing 25%, 50%, 75%, and 100% by weight of coal and 25%, 50%, and 75% by weight of marble and travertine dust. Afterwards, local beans were planted in these mixtures and their development was followed under laboratory conditions. Additionally, temperature and humidity values were recorded at certain intervals, pH and heavy metal analysis measurements were made on the mixtures before and after bean planting, the height and leaf measurement of the beans grown and the number of beans grown in these beans were also followed, and chlorophyll analysis was performed on the bean plants grown in these mixtures. As a result, 50% Coal-3D + 50% Travertine mixture had the maximum length increase, that 25% Coal Waste (Powder)-K3 + 75% Marble mixture had the highest number of beans, and that 50% Coal Waste -AK + 50% Marble mixture had the maximum leaf formation. In these mixtures, maximum 12 beans were grown and maximum 93 leaves were formed. Also, the bean plant was grown in all mixtures. The chlorophyll contents were at least as much as the values of the beans grown only in soil. However, heavy metal values do not exceed the permissible limit values in soil.
Limited mineral resources, the desire for more extraction, and population increases cause an increase in production in the mining sector (Luthra et al., 2022). It is stated that the waste produced by the mining industry is 7 billion tons per year, and according to the latest estimates, 19 billion tons of mining solid waste will accumulate by 2025, and due to its mixed physical and chemical structure, only 20% of this waste can be recycled (Marín et al., 2022).
Marble and travertine-producing countries in the world sell some of their products in the local market and export some to the foreign markets. 30% of the marble and travertine produced in Turkey are exported every year (Erkek and Özdemir, 2011; Adıgüzel, 2019). Since marble and travertine are used in different sizes, the formation of wastes such as blocks, pieces, dust wastes, mud, etc. During the production stage brings along serious environmental problems and these wastes cause the destruction of nature. Especially, the dust caused by the dimensional change of the marble blocks and the storage of these dusts are spread to the environment. Mining activities and subsequent processes, changes in the topographic structure and improper storage of wastes destroy the environment, causing water, soil and air pollution, and as a result, the biological environment and diversity in nature are damaged (Angotzi, 2005; Ashmole and Motloung, 2008; Rizzo et al., 2008). This situation, which causes environmental and soil pollution brings the expenditure of additional costs (Candeias et al., 2019; Galán, 2020; Jawadand and Randive, 2021).
Wastes of different sizes after marble mining cause deterioration on the environment. In particular, type of rock, geology and morphology of the marble quarry, texture and crystal structure of the operated rock, suitability and method of the tool used for the operation create a dimensional difference in the waste (Bağcı and Karataş, 2017). The wastes released in the marble quarry are generally in block size and are called rubble and cause a decrease in efficiency (Metin et al., 2012). Waste with a particle size of less than 2 µm is considered dust in the production process of marble and travertine. It is stated that 90% of the waste marble dust in different sizes are under 200 μm, 70% are under 100 μm and 40% are under 20 μm (Çelik and Tur, 2012). Additionally, the fact that the waste marble dust contains approximately 35%–45% water and is in muddy form causes a change in the precautions to be taken and the disposal methods. It is assumed that in the marble quarrying process, approximately 30% of the stone is released as pieces, and in the production phase, approximately 40% of the stone is released as pieces and dust waste. After that, approximately 60%–70% of the stone coming out of the quarry is evaluated as piece or dust waste (Kızıltepe and Şahin, 2021).
Recycling of mining waste helps to reduce the amount of waste to be disposed of, but it is not enough. New solutions such as the economic recycling and reusing of waste are necessary to ensure sustainability in the mining and metals extraction industries. Even if the waste contains heterogeneous, complex, and reactive mineral mixtures, there are different solution advantages. Marble wastes in block size are used against erosion in large-scale structures, in slopes, bridge abutments, retaining walls, concrete brick (briquette) and gas concrete production, development of geotechnical properties for clayey soils, road construction, aggregate production as raw material in concrete, and in stream improvement against flood hazards and in fight against floods (Aitcin and Mindess, 2011; Sivrikaya et al., 2014; Çavuş, 2015; Ural and Yakşe, 2016; Ghanbari et al., 2017). Waste marble dust is preferred as an alternative product used instead of cement and fine aggregate added to the concrete composition (Lakhani et al., 2014; Sakalkale et al., 2014; Singh and Bansal, 2015). Waste marble dust is also available for the stabilisation of expansive soils (SAYGILI, 2015; Öncü and Bilsel, 2018; Jain et al., 2020; Abdelkader et al., 2021; Abdulla and Majeed, 2021; Waheed et al., 2021; Amena and Kabeta, 2022).
Marble and travertine wastes are widely used in the construction sector in general, in terms of their chemical composition. However, there are also studies that give positive results by evaluating these wastes in different areas. For instance, the acidity of the soil was regulated by fine marble wastes (Tozsin et al., 2015; Fernández-Caliani et al., 2022). In addition, marble and travertine wastes, which are used as a kind of fertilizer and mineral source in agriculture, have shown an environmentally friendly approach to the disposal of lime-rich wastes (CaCO3) by improving acidic and calcium-deficient soils (Thakur et al., 2018). CaCO3, which is an effective method, was used to eliminate or reduce the harmful effects of heavy metal wastes in abandoned mine sites and to prevent damage to the vegetation and biodiversity in and around the mine area (Bade et al., 2012; Beesley et al., 2014; Ahmad et al., 2017; Holland et al., 2018; Benidire et al., 2020; Benidire et al., 2021; Benidire et al., 2022). They also added lime into the soil to increase soil pH (Mylona et al., 2000; Aarab et al., 2006; Potgieter-Vermaak et al., 2006; Kumpiene et al., 2008; Zornoza et al., 2012; Thakur et al., 2018). This increased the soil pH and gave it basic properties, as well as accelerating the development of plants and creating natural carbon dioxide gas to be used as fertilizer (McGrath and Lobell, 2013; Nakata et al., 2014). However, marble and travertine wastes are also used to reduce the sulfate ions activity and neutralize the acidity in remote areas where sulfide mine wastes are usually abandoned, such as acid mine drainage. Thus, these materials have the ability to neutralize acidity and the reaction surface, reduce the impact on the environment and increase agricultural productivity (Simandl and Paradis, 2018; Carrillo-González et al., 2022).
In this context, the usability of the mixtures formed as a result of mixing the dust released in the marble and travertine factory operated in Gumushane region, the low-calorie lignite coal taken from the Tekirdag region and the waste released after its production in certain proportions in local bean cultivation was tried to be revealed. With this study, it was aimed to reuse industrial mine wastes in agriculture. This specific research objectives are:
1. To reveal the usability of the mixtures formed by mixing the dust released in the marble and travertine factory operating in Gumuşhane region, low calorific lignite coal taken from Tekirdag region and the wastes released after production in certain ratios in local bean cultivation.
2. Eliminating or reducing the damages caused by these dusts to the environment.
3. To determine the most suitable mixture ratios that can be used in bean cultivation.
4. Determination of whether the heavy metal values of the prepared mixtures are suitable for use in soil.
5. Determination of chlorophyll content of beans grown in soil mixtures.
Marble and travertine dust waste taken from a marble factory located in Siran district of Gumushane province were not subjected to any processing since they were below 100 μ and were added to the mixtures as they were. Experiments were conducted according to the flow chart indicated in Figure 1.
Coal sources are located in Tekirdag, a province of Turkey. Coal samples from 3 different areas and waste from 2 different coal enrichment plants were brought to the laboratory to be used in the experiments. Large sizes of coal samples were first reduced to below −4 cm in a jaw crusher and then grinded to a size of −300 μ by ball milling for 15 min. Waste coal samples were used in the experiments without any treatment. Then, ash [TS ISO 1171 (TS ISO 1171, 2014)/ASTM D7582], moisture [TS 1051 ISO 1015 (TS 1051 ISO 1015, 2001)/ASTM ASTM D7582], volatile matter [TS ISO 562 (TS ISO 562, 2021)/ASTM D7582], fixed carbon (ASTM D3172-13), calories (ASTM D5865/D5865M-19), pH, humic + fulvic acid [TS ISO 5073 (TS ISO 5073, 2022)], organic matter and heavy metals analysis experiments were conducted on the lignite coal used in the study.
First, control samples were made using only humus soil without coal, marble or travertine dust. Then, coal—marble dust and coal—travertine dust mixtures were formed for each coal. These mixtures were obtained by mixing 25%, 50%, 75%, and 100% by weight of coal and 25%, 50%, and 75% by weight of waste marble dust. Coal/coal waste—travertine dust mixtures were obtained by mixing 25%, 50%, 75%, and 100% by weight of coal and 25%, 50%, and 75% by weight of travertine dust. The ratios and amounts of the mixtures prepared are given in Table 1.
These mixtures were mixed homogeneously and placed in pots measuring 14 cm × 17 cm in the laboratory environment and watered with 100 ml of distilled water every 3 days for 15 days. With this irrigation, the mixtures were saturated with water. Additionally, Kelkit bean grains, a local bean type, were left to sprout in humus soil in separate containers. A local bean plant was preferred in order to investigate the usability of these mixtures in agriculture. Then, the sprouting bean grains were planted in mixtures that contain water-saturated marble dust, travertine dust and coal. Planted beans were irrigated with 100 ml of distilled water every 3 days. In addition, temperature and humidity values were recorded at regular intervals. Furthermore, pH and heavy metal analysis measurements were performed on the mixtures before and after bean planting. Length and leaf measurement of the beans grown and the number of beans grown on them were also followed. Finally, chlorophyll analysis was carried out on bean plants grown in these mixtures. Experiments were conducted according to the stages in Figure 2.
Samples taken from each of the mixtures prepared according to the ratios in Table 1 were first diluted with distilled water at a ratio of 1:2.5 to form a suspension, and this suspension was mixed 3 times at 15-min intervals and then left for 15 min to be ready for analysis. Measurements were performed with a Neel brand pH meter, which was calibrated before the analysis. After harvesting of Kelkit beans, pH measurements were also performed on mixture samples taken from plant pots in the same way.
50 g samples were taken from each mixture prepared according to the ratios indicated in Table 1 both before planting and after harvesting beans. Heavy metal analyzes of these samples were carried out by using ICP-MS device in Gumustaş Madencilik A.S. The heavy metal values of Silver (Ag), Arsenic (As), Cadmium (Cd), Copper (Cu), Iron (Fe), Antimony (Sb), Lead (Pb), Zinc (Zn) metals that cause soil heavy metal pollution in these mixtures were examined.
Chlorophyll analyses were carried out according to the method of Witham et al. (1971), by harvesting beans in mixtures with healthy bean plants. In the analysis, 0.1 g dried leaf samples were extracted in 80% acetone and centrifuged at 10,000 rpm for 5 min. The supernatant was separated, and the remaining pulp was extracted in 80% acetone until it became colorless. After this, the collected supernatants of the suspension were read on the spectrophotometer at 645 and 663 wavelengths. Chlorophyll a, chlorophyll b, and total chlorophyll amounts were determined according to Eqs 1–3:
In the relevant formulae; V= Supernatant volume (ml), W = Extracted leaf weight (g), A = Absorbance values of wavelengths.
Results of the chemical and physical properties obtained from the analysis of marble and travertine used in bean mixtures are given in Table 2.
TABLE 2. Chemical and physical characteristics of marble and travertine (Demir Şahin et al., 2022).
As seen in Table 2, the content of marble and travertine waste consists of silicon dioxide, aluminum oxide, iron oxide, calcium oxide, magnesium oxide and sulfur trioxide. A large amount (about 52%) of marble and travertine waste consist of calcium oxide. The calcium oxide value of the marble waste is slightly higher than the travertine waste. Additionally, marble waste is thinner and has more specific surface area than travertine waste. However, density of travertine waste is higher than marble waste. Studies in the literature have shown that lime improves soil, eliminates its acidity and calcium deficiency and reduces or eliminates the harmful effects of heavy metals in it (Bade et al., 2012; Beesley et al., 2014; Tozsin et al., 2015; Ahmad et al., 2017; Holland et al., 2018; Thakur et al., 2018; Benidire et al., 2020; Benidire et al., 2021; Benidire et al., 2022). For this reason, the mixtures containing marble and travertine will contribute to the bean plant development and will provide improvements that reduce the effects of heavy metals in the wastes. Results of coal and coal wastes used are given in Table 3.
The highest volatile matter content is in Coal Waste -AK in Table 3. Other Coal Waste -K3, Coal-3C, Coal-4B and Coal-3D samples have volatile matter content less than this value. Ash values are Coal-4B, Coal-3D, Coal-3C, Coal Waste -AK and Coal Waste -K3 in descending order. Accordingly, the coal samples taken have higher ash content than waste coal samples. The sample with the highest fixed carbon value is Coal Waste -AK. Humic + Fulvic Acid, from highest to lowest, is Coal Waste -K3, Coal Waste -AK.
Coal-3C, Coal-3D and Coal-4B. It was determined that organic matter contents varied between 36.89%–78.19% and Coal Waste -K3 had the most organic matter content. Lastly, calorific values of the samples are Coal Waste -K3, Coal-3C, Coal Waste -AK, Coal-3D and Coal-4B, respectively.
Temperature and humidity changes during the development of beans planted in the mixtures are given in Figure 3. It was observed that the ambient temperature in which beans were grown was between 19.1°C and 26.7°C, and the ambient humidity values varied between 35% and 64%. These humidity and temperature values are sufficient for the bean plant to grow.
In Figure 4, it is seen that pH values of the mixtures prepared from mining waste were between 6.3 and 7.8 before the beans planted. When the ratio of the amount of coal in the mixture increased, pH values decreased and were measured below the reference pH value. With the addition of marble and travertine waste to the mixtures, pH values increased again and were generally measured above reference pH values. The lowest pH value of 6.3 was obtained only in the mixture containing Coal Waste -K3; the highest pH values of 7.8 were obtained in 25% Coal-3D + 75% Travertine and 25% Coal-3D + 75% Marble mixtures. The reason for the increase in the pH of these mixtures was due to the high CaO amounts of the marble and travertine used, and the hydrolysis of the carbonate over time. There was not much increase in pH measurement results of the prepared mixtures after bean planting (Table 6).
In Table 4, the elongation amount of the beans grown in the mixtures, the proportional elongation of the plant, the number of beans and leaves are given. It was determined that first height values of bean plant were between 9.5 and 57.5 cm, and the final height values were between 9.5 and 257 cm. Depending on these values, it was seen that proportional elongation amount varied between 1.75 and 14.42.
TABLE 4. Plant height, proportional extension, number of beans and leaves of cultivated bean plants.
It was determined that the maximum elongation was in the 50% Coal-3D + 50% Travertine mixture, and it exceeded the reference mixture. Considering the number of beans, it was observed that between 0 and 12 beans were grown in the mentioned mixtures. It was seen that more beans were obtained than the reference mixture in the 25% Coal Waste (Powder)-K3 + 75% Marble mixture, no beans were grown in the mixtures consisting only of coal except for the Coal-4B mixture, and in the remaining mixtures, fewer beans were grown than the reference sample.
When the number of leaves of the grown plants were examined, it was determined that 0–93 leaves formation was observed, the highest leaf formation was in the 50% Coal Waste -AK + 50% Marble mixture and exceeded the number of leaves in the reference sample, and the number of leaves was less in the mixtures with high coal content.
The values of Silver (Ag), Arsenic (As), Cadmium (Cd), Copper (Cu), Iron (Fe), Antimony (Sb), Lead (Pb), Zinc (Zn) metals that cause heavy metal pollution in the soil before and after bean planting are given in Tables 5, 6. Cu, As, Ag, Sb, Cd metals were determined below <0.01 in all prepared mixtures. Pb and Zn metals were measured as 0.01 mg/kg in some coal mixture samples. Fe mineral had values between 0.40 and 2.79 mg/kg. According to Regulation on Control of Soil Pollution and Point Source Contaminated Sites (source to be added), the limit values of Cu, Cd, Pb, Zn are 140, 3, 300, 300 mg/kg, respectively. The World Health Organization (WHO)/The Food and Agriculture Organization of the United Nations (FAO) values of As and Fe are 20 and 50,000 mg/kg, respectively. For Ag and Sb, there are no limit values determined by these regulations and organizations. Accordingly, it was determined that heavy metal values of all mixtures were below the heavy metal limit values allowed in the soil.
Post-harvest values of Silver (Ag), Arsenic (As), Cadmium (Cd), Copper (Cu), Iron (Fe), Antimony (Sb), Lead (Pb), Zinc (Zn) metals that cause heavy metal pollution in the soil are given in Table 6. Pb, Cu, As, Ag, Sb, Cd metals were determined below <0.01 in all prepared mixtures. Zn metals were measured as 0.01 mg/kg in some coal mixture samples. Fe mineral had values between 0.39 and 4.69 mg/kg.
When the Fe values in Tables 5, 6 are examined, it is seen that the value in the mixture generally decreases depending on the increase in the amount of marble and travertine dust in the mixtures (Figure 5). However, it is observed that the decrease in Fe value is higher in the mixtures with the addition of marble dust compared to the mixtures with the addition of travertine dust. It is considered that this situation is caused by the difference in Fe2O3 contents of marble and travertine dust in Table 2.
In the analyses following the harvesting of bean plants, it was observed that there were no serious changes in the Fe values. These metal values are important both in terms of the environment and heavy metal absorption that may occur in the plant, and the low values will allow the use of coal + marble and coal + travertine dust mixtures as soil (Figure 6).
As seen in Figure 7, chlorophyll analysis could be performed at the end of the harvest for the mixtures of Coal Waste (Powder)-K3, 50% Coal Waste (Powder)-K3 + 50% Marble, 25% Coal Waste (Powder)-K3 + 75% Marble, 75% Coal-4B + 25% Travertine and 50% Coal-4B + 50% Travertine, but not for the others due to lack of leaves. According to chlorophyll analysis of these mixture samples, chlorophyll a values were generally equal to or higher than the reference sample. Chlorophyll b values of Coal Waste (Powder)-K3 mixture were found to be higher than reference value, chlorophyll values of 75% Coal-4B + 25% Travertine mixture were found to be equal to reference value, and Chlorophyll b values of other mixtures were lower than reference values. The increase in the amount of marble in the mixtures caused an increase in the chlorophyll a value, whereas it did not cause a change in the travertine mixtures. On the other hand, chlorophyll b values generally decreased with the increase in the amount of marble and travertine in the mixtures.
According to the results of pH analysis performed on mixtures consisting of marble, travertine, coal or coal waste, it was determined that pH values were between 6.3 and 7.8, that pH value increased with the increase in the ratio of marble and travertine in the mixtures due to the CaO in the composition of marble and travertine dust, and that results of pH measurements performed on the mixtures after the bean harvest did not change much and these pH values were within the pH range required for growing beans. In addition, CaO contributed to the development of the bean plant; it helped to reduce the effects of heavy metals in wastes and thus a bean that does not harm the environment was grown. However, it was understood that 3 different coal and 2 different coal wastes from Tekirdağ/Turkey region can be used in the agricultural sector due to their Humic + Fulvic Acid and Organic Matter contents. On the other hand, it was understood that 50% Coal-3D + 50% Travertine mixture had the maximum length increase, that 25% Coal Waste (Powder)-K3 + 75% Marble mixture had the highest number of beans, and that 50% Coal Waste -AK + 50% Marble mixture had the maximum leaf formation. In these mixtures, maximum 12 beans were grown and maximum 93 leaves were formed.
It was observed that no beans were grown in the mixtures consisting of only coal, except for the Coal-4B mixture, while beans were grown in the remaining mixtures. It is thought that this is due to the fact that the organic matter content of Coal-4B is lower than that of other coals. It was determined that more leaves were formed in 50% Coal Waste -AK + 50% Marble mixture than in the reference sample, and the number of leaves were less in the mixtures with higher coal content.
As a result of the analyses made in the mixtures for Ag, As, Cd, Cu, Fe, Sb, Lead Pb, Zn metals that cause heavy metal pollution in the soil before and after bean harvest, it was determined that all the mixtures were below the heavy metal limit values allowed in the soil. Thus, it was proved that all mixtures are suitable for use in agriculture since their heavy metal values are below limit values allowed in the soil. When the Fe values were examined, it was understood that the value in the mixture generally decreased due to the increase in the amount of marble and travertine dust in the mixtures, that the decrease in the Fe value was higher in the mixtures with marble dust compared to the mixtures with travertine dust, and this was due to the differences in the Fe2O3 contents of marble and travertine dust. In the analysis after harvesting of bean plants, it was determined that there were no significant changes in Fe values, that these results were important both in terms of the environment and heavy metal absorption that may occur in the plant, and that the low values would allow the use of coal + marble and coal + travertine dust mixtures as soil.
Chlorophyll analysis could be performed at the end of the harvest on Coal Waste (Powder)-K3, 50% Coal Waste (Pow-der)-K3 + 50% Marble, 25% Coal Waste (Powder)-K3 + 75% Marble, 75% Coal-4B + 25% Marble, 25% Coal-4B + 75% Marble, 75% Coal-4B + 25% Travertine and 50% Coal-4B + 50% Travertine mixtures, but not on the others. According to chlorophyll analysis, chlorophyll values of these mixtures were higher or generally equal to the reference sample; chlorophyll b values of Coal Waste (Powder)-K3 mixture were higher than reference value, chlorophyll b values of 75% Coal-4B + 25% Travertine mixture were equal to reference value, and chlorophyll b values of other mixtures were lower than reference values. While the increase in the amount of marble in the mixtures caused an increase in the chlorophyll a value, it did not cause a change in the travertine mixtures. On the other hand, chlorophyll b values generally decreased with the increase in the amount of marble and travertine in the mixtures.
When all the data were examined, it was revealed that the bean plant were grown in the mixtures prepared using coal or coal waste and marble and travertine wastes, and that mine waste could be used in agricultural areas (Figure 8). The effect of marble and travertine dust on the growth and development of the bean plant is because most of their composition consists of CaCO3. CO2 gas, which plays an active role in the growth of the bean plant, helps to release excess water and energy accumulated in the plant’s leaves and contributes to the increase in the growth rate of the plant (McGrath and Lobell, 2013; Bishop et al., 2014; Nakata et al., 2014). The results obtained in this study show similarities to many studies. It was understood that the use of marble and travertine waste in the prepared mixtures in agriculture as a CO2 source causes an increase in the bean plant yield, especially in the bean formation and amount, the number of leaves, and the amount of lengthening.
Particularly organic matter and Humic + Fulvic Acid contained in coal or coal waste contribute to soil quality and plant growth. Obtaining better results in the prepared mixtures than the reference mixture is due to this organic matter and Humic + Fulvic Acid in the coal content (Song and Lee, 2010; Peña et al., 2015; Ultra et al., 2022; Bai et al., 2023; Feng et al., 2023; Jayapal et al., 2023; Yin et al., 2023).
It was determined that heavy metal values of all mixtures were below the heavy metal limit values allowed in the soil. Thus, it was understood that heavy metals in the content of the waste would not cause harm to human health, as in similar studies using different mine waste (Chen et al., 2019; Samsuri et al., 2019; Esteves-Aguilar et al., 2023; Jayapal et al., 2023; Nassiri et al., 2023; Tibane and Mamba, 2023; Zhang et al., 2023).
The increase in the amount of marble in the prepared mixtures caused an increase in the chlorophyll a value, while it did not cause a change in the travertine mixtures. On the other hand, chlorophyll b values generally decreased with the increase in the amount of marble and travertine in the mixtures.
In the study conducted by Chen et al. (2019), mining waste first increased chlorophyll a and chlorophyll b values up to a certain rate (5%) and then decreased. This situation reveals that chlorophyll a and chlorophyll b values in plants may differ depending on the type of waste.
In India, marble and mineral dust has adverse effects on plants and vegetation near marble industries. Trees and plants undergo biochemical, physiological and morphological changes due to the reduced amount of chlorophyll in plants due to these dusts, resulting in a 20% reduction in growth (Soni et al., 2017; Iqbal et al., 2022). It has been observed that these effects are seen in mixtures where marble dust is used too much and yields are decreased. However, dust from marble quarries in the Burdur region of Turkey mixes with the surface and ground waters in this region. Then, surface and ground waters are mixed into Lake Burdur and it has been determined that this situation decreases the quality of lake waters (Ozcelik, 2023). Furthermore, marble quarry dust is a significant source of air pollution, but its severity varies depending on factors such as local microclimatic conditions, dust particle concentration in ambient air, particle size and chemistry. Marble quarries produce highly alkaline and reactive dust as they contain limestone. Dust can have physical effects on surrounding plants, such as inhibiting and damaging their internal systems, corroding leaves and cuticles, as well as chemical consequences that can prevent long-term survival. It has long been known that dust from marble quarries around Lake Burdur damages vegetation and reduces crop yields, posing a danger to plant life in industrialised areas. Farmers report that this dust reduces growth, height and number of leaves (Gabarrón et al., 2019). Similar results were observed in this study due to the increase in the amount of marble and travertine dust used.
The results obtained in this study, in which the effects of the mixtures formed by the preparation of lignite coal and coal waste taken from Tekirdag/Turkey region and marble and travertine waste from the marble factory in Gumushane/Turkey on local Kelkit/Gumushane bean were examined, are given below:
(1) All mixtures are suitable for use in agriculture.
(2) Considering the elongation amount of the beans grown, the proportional elongation of the plant, the number of beans and leaves, it was observed that coal or coal wastes, marble and travertine waste contribute to the development of beans.
(3) Their heavy metal values are below limit values allowed in the soil.
(4) The chlorophyll contents of beans grown in the mixtures containing waste were found to be at least as much as the values of beans grown only in soil.
(5) Thus, it is understood that the damage caused by waste dust to the environment will be eliminated or reduced
According to the results obtained from this study, it was understood that coal, coal waste and marble and travertine waste could be used together in agriculture. Thus, it will help to reduce or eliminate environmental problems caused by mining waste and reduce the storage costs. In addition, mixtures containing coal or coal waste, marble and travertine can be used in restoration (renewal), rehabilitation (improvement) and reclamation works to be carried out after mining activities. Kelkit beans are widely cultivated in the Gümüşhane region and are a registered product in Turkey. The best mixture ratios obtained as a result of the study will be applied in the field. In addition to this, it is suggested that researchers who will study on this subject work on different types of coal, different types of marble and travertine waste and different plant species and use these mixtures in the rehabilitation and restoration stages of abandoned areas.
The original contributions presented in the study are included in the article/Supplementary Material, further inquiries can be directed to the corresponding author.
Ethical review and approval are not required for ethical issues related to the collection and use of waste materials in the study, as well as for their potential impact on the local environment and communities.
The author confirms being the sole contributor of this work and has approved it for publication.
I would like to thank the Cavit Kumaş, Demet Demir Sahin of Gumushane University, Akçelik Mining and Gumushane Cement Factory for their contributions to this study.
The author declares that the research was conducted in the absence of any commercial or financial relationships that could be construed as a potential conflict of interest.
All claims expressed in this article are solely those of the authors and do not necessarily represent those of their affiliated organizations, or those of the publisher, the editors and the reviewers. Any product that may be evaluated in this article, or claim that may be made by its manufacturer, is not guaranteed or endorsed by the publisher.
Aarab, T., Smeyers, M., Remy, M., Godden, B., and Delhaye, J. P. (2006). The storage of sewage sludge: Influence of liming on the evolution of copper chemical fractions. Waste Manag. 26, 1024–1032. doi:10.1016/j.wasman.2005.10.007
Abdelkader, H. A. M., Hussein, M. M. A., and Ye, H. (2021). Influence of waste marble dust on the improvement of expansive clay soils. Adv. Civ. Eng. 2021, 1–13. doi:10.1155/2021/3192122
Abdulla, R., and Majeed, N. (2021). Enhancing engineering properties of expansive soil using marble waste powder. Iraqi Geol. J. 54, 43–53. doi:10.46717/igj.54.1E.4Ms-2021-05-25
Adıgüzel, M. (2019). Investigation of Turkish marble sector and its competitive power. Third Sect. Soc. Econ. Rev. 54, 1530–1546. doi:10.15659/3.sektor-sosyal-ekonomi.19.09.1202
Ahmad, M., Lee, S. S., Lee, S. E., Al-Wabel, M. I., Tsang, D. C. W., and Ok, Y. S. (2017). Biochar-induced changes in soil properties affected immobilization/mobilization of metals/metalloids in contaminated soils. J. Soils Sediments 17, 717–730. doi:10.1007/s11368-015-1339-4
Amena, S., and Kabeta, W. F. (2022). Mechanical behavior of plastic strips-reinforced expansive soils stabilized with waste marble dust. Adv. Civ. Eng. 2022, 1–8. doi:10.1155/2022/9807449
Angotzi, G. (2005). World at work: Marble quarrying in tuscany. Occup. Environ. Med. 62, 417–421. doi:10.1136/oem.2004.018721
Ashmole, I., and Motloung, M. (2008). Rerclamation and environmental management in dimension stone mining. South. Afr. Inst. Min. Metallurgy 1, 155–178. Available at: https://js.ugd.edu.mk/index.php/NRT/article/view/124.
Bade, R., Oh, S., and Sik Shin, W. (2012). Assessment of metal bioavailability in smelter-contaminated soil before and after lime amendment. Ecotoxicol. Environ. Saf. 80, 299–307. doi:10.1016/j.ecoenv.2012.03.019
Bağcı, M., and Karataş, M. (2017). The investigation swot analysis of muğla marbles. Afyon Kocatepe Univ. J. Sci. Eng. 17, 736–752. doi:10.5578/fmbd.57409
Bai, Z., Wu, F., He, Y., and Han, Z. (2023). Pollution and risk assessment of heavy metals in zuoxiguo Antimony mining area, southwest China. Environ. Pollut. Bioavailab. 35, 35. doi:10.1080/26395940.2022.2156397
Beesley, L., Inneh, O. S., Norton, G. J., Moreno-Jimenez, E., Pardo, T., Clemente, R., et al. (2014). Assessing the influence of compost and biochar amendments on the mobility and toxicity of metals and arsenic in a naturally contaminated mine soil. Environ. Pollut. 186, 195–202. doi:10.1016/j.envpol.2013.11.026
Benidire, L., Madline, A., Pereira, S. I. A., Castro, P. M. L., and Boularbah, A. (2021). Synergistic effect of organo-mineral amendments and plant growth-promoting rhizobacteria (PGPR) on the establishment of vegetation cover and amelioration of mine tailings. Chemosphere 262, 127803. doi:10.1016/j.chemosphere.2020.127803
Benidire, L., Pereira, S., Aboudrar, W., Hafidi, M., Castro, P., and Boularbah, A. (2022). Remediation of metal-contaminated mine tailings by the application of organic and mineral amendments. J. Soils Sediments 22, 482–495. doi:10.1007/s11368-021-03081-z
Benidire, L., Pereira, S. I. A., Naylo, A., Castro, P. M. L., and Boularbah, A. (2020). Do metal contamination and plant species affect microbial abundance and bacterial diversity in the rhizosphere of metallophytes growing in mining areas in a semiarid climate? J. Soils Sediments 20, 1003–1017. doi:10.1007/s11368-019-02475-4
Bishop, K. A., Leakey, A. D. B., and Ainsworth, E. A. (2014). How seasonal temperature or water inputs affect the relative response of C 3 crops to elevated [CO 2 ]: A global analysis of open top chamber and free air CO 2 enrichment studies. Food Energy Secur 3, 33–45. doi:10.1002/fes3.44
Candeias, C., Ávila, P., Coelho, P., and Teixeira, J. P. (2019). “Mining activities: Health impacts,” in Encyclopedia of environmental health (Elsevier), 415–435.
Carrillo-González, R., Gatica García, B. G., González-Chávez, , Ma, D. C. A., and Solís Domínguez, F. A. (2022). Trace elements adsorption from solutions and acid mine drainage using agricultural by-products. Soil Sediment Contam. Int. J. 31, 348–366. doi:10.1080/15320383.2021.1942430
Çavuş, U. Ş. (2015). Use of marble wastes in flood walls and regulation and protection structures of river beds. Afyon Kocatepe Univ. J. Sci. Eng. 15, 19–28. doi:10.5578/fmbd.9746
Çelik, M. Y., and Tur, Ş. (2012). Investigation of the properties of the marble waste in the natural stone waste storage field of afyonkarahisar organized industrial zone. Afyon Kocatepe Univ. J. Sci. Eng. Sci. 12, 9–15.
Chen, D., Meng, Z., and Chen, Y. (2019). Toxicity assessment of molybdenum slag as a mineral fertilizer: A case study with pakchoi (Brassica chinensis L.). Chemosphere 217, 816–824. doi:10.1016/j.chemosphere.2018.10.216
Demir Şahin, D., Kumaş, C., and Eker, H. (2022). Research of the use of mine tailings in agriculture. J. Curr. Res. Eng. 8, 71–84.
Erkek, D., and Özdemir, S. (2011). Marble and travertine sector global and regional approach 2011, 42.
Esteves-Aguilar, J., Mussali-Galante, P., Valencia-Cuevas, L., García-Cigarrero, A. A., Rodríguez, A., Castrejón-Godínez, M. L., et al. (2023). Ecotoxicological effects of heavy metal bioaccumulation in two trophic levels. Environ. Sci. Pollut. Res. 30, 49840–49855. doi:10.1007/s11356-023-25804-0
Feng, J., Yang, Y., Ruan, K., Wu, D., Xu, Y., Jacobs, D. F., et al. (2023). Combined application of sewage sludge, bagasse, and molybdenum tailings ameliorates rare earth mining wasteland soil. J. Soils Sediments 23, 1775–1788. doi:10.1007/s11368-023-03437-7
Fernández-Caliani, J. C., Giráldez, I., Fernández-Landero, S., Barba-Brioso, C., and Morales, E. (2022). Long-term sustainability of marble waste sludge in reducing soil acidity and heavy metal release in a contaminated mine technosol. Appl. Sci. 12, 6998. doi:10.3390/app12146998
Gabarrón, M., Zornoza, R., Acosta, J. A., Faz, Á., and Martínez-Martínez, S. (2019). “Mining environments,” in Advances in chemical pollution, environmental management and protection, 157–205.
Galán, J. E. (2020). The benefits are at the tail: Uncovering the impact of macroprudential policy on growth-at-risk. J. Financial Stab., 100831. doi:10.1016/j.jfs.2020.100831
Ghanbari, M., Monir Abbasi, A., and Ravanshadnia, M. (2017). Economic and environmental evaluation and optimal ratio of natural and recycled aggregate production. Adv. Mater. Sci. Eng. 2017, 1–10. doi:10.1155/2017/7458285
Holland, J. E., Bennett, A. E., Newton, A. C., White, P. J., McKenzie, B. M., George, T. S., et al. (2018). Liming impacts on soils, crops and biodiversity in the UK: A review. Sci. Total Environ. 610–611, 316–332. doi:10.1016/j.scitotenv.2017.08.020
Iqbal, Q., Musarat, M. A., Ullah, N., Alaloul, W. S., Rabbani, M. B. A., Al Madhoun, W., et al. (2022). Marble dust effect on the air quality: An environmental assessment approach. Sustainability 14, 3831. doi:10.3390/su14073831
Jain, A. K., Jha, A. K., and Shivanshi, (2020). Geotechnical behaviour and micro-analyses of expansive soil amended with marble dust. Soils Found. 60, 737–751. doi:10.1016/j.sandf.2020.02.013
Jawadand, S., and Randive, K. (2021). “A sustainable approach to transforming mining waste into value-added products,” in Innovations in sustainable mining, 1–20.
Jayapal, A., Chaterjee, T., and Sahariah, B. P. (2023). Bioremediation techniques for the treatment of mine tailings: A review. Soil Ecol. Lett. 5, 220149. doi:10.1007/s42832-022-0149-z
Kızıltepe, U., and Şahin, Y. (2021). Investigation of usability of travertine waste as concrete aggregate. Fırat Univ. J. Eng. Sci. 33, 413–420. doi:10.35234/fumbd.850026
Kumpiene, J., Lagerkvist, A., and Maurice, C. (2008). Stabilization of as, Cr, Cu, Pb and Zn in soil using amendments – a review. Waste Manag. 28, 215–225. doi:10.1016/j.wasman.2006.12.012
Lakhani, R., Kumar, R., and Tomar, P. (2014). Utilization of stone waste in the development of value added products: A state of the art review. J. Eng. Sci. Technol. Rev. 7, 180–187. doi:10.25103/jestr.073.29
Luthra, S., Mangla, S. K., Sarkis, J., and Tseng, M.-L. (2022). Resources melioration and the circular economy: Sustainability potentials for mineral, mining and extraction sector in emerging economies. Resour. Policy 77, 102652. doi:10.1016/j.resourpol.2022.102652
Marín, O. A., Kraslawski, A., and Cisternas, L. A. (2022). Estimating processing cost for the recovery of valuable elements from mine tailings using dimensional analysis. Miner. Eng. 184, 107629. doi:10.1016/j.mineng.2022.107629
McGrath, J. M., and Lobell, D. B. (2013). Regional disparities in the CO 2 fertilization effect and implications for crop yields. Environ. Res. Lett. 8, 014054. doi:10.1088/1748-9326/8/1/014054
Metin, E., Yeşilkaya, L., and Gülseven, H. (2012). Comparison of the block and rubble CuttingProductivity in marble plants. TÜBAV Sci. J. 5, 1–10.Available at: https://dergipark.org.tr/en/pub/tubav/issue/21529/230986
Mylona, E., Xenidis, A., and Paspaliaris, I. (2000). Inhibition of acid generation from sulphidic wastes by the addition of small amounts of limestone. Miner. Eng. 13, 1161–1175. doi:10.1016/S0892-6875(00)00099-6
Nakata, K., Ozaki, T., Terashima, C., Fujishima, A., and Einaga, Y. (2014). High-yield electrochemical production of formaldehyde from CO 2 and seawater. Angew. Chem. Int. Ed. 53, 871–874. doi:10.1002/anie.201308657
Nassiri, O., Rhoujjati, A., Moreno-Jimenez, E., and Hachimi, M. L. E. L. (2023). Environmental and geochemical characteristics of heavy metals in soils around the former mining area of zeïda (high moulouya, Morocco). Water Air Soil Pollut. 234, 110. doi:10.1007/s11270-023-06103-3
Öncü, Ş., and Bilsel, H. (2018). Utilization of waste marble to enhance volume change and strength characteristics of sand-stabilized expansive soil. Environ. Earth Sci. 77, 461. doi:10.1007/s12665-018-7638-5
Ozcelik, M. (2023). Environmental effects of marble quarry operations in Burdur lake basin (Burdur-Turkey). J. Degraded Min. Lands Manag. 10, 4517–7. doi:10.15243/jdmlm.2023.103.4517
Peña, A., Mingorance, M. D., and Rossini-Oliva, S. (2015). Soil quality improvement by the establishment of a vegetative cover in a mine soil added with composted municipal sewage sludge. J. Geochem Explor 157, 178–183. doi:10.1016/j.gexplo.2015.06.014
Potgieter-Vermaak, S. S., Potgieter, J. H., Monama, P., and Van Grieken, R. (2006). Comparison of limestone, dolomite and fly ash as pre-treatment agents for acid mine drainage. Miner. Eng. 19, 454–462. doi:10.1016/j.mineng.2005.07.009
Rizzo, G., D’Agostino, F., and Ercoli, L. (2008). Problems of soil and groundwater pollution in the disposal of “marble” slurries in NW sicily. Environ. Geol. 55, 929–935. doi:10.1007/s00254-007-1043-9
Sakalkale, A. D., Dhawale, G. D., and Kedar, R. S. (2014). Experimental study on use of waste marble dust in concrete. Int. J. Eng. Res. Appl. 4, 44–50.
Samsuri, A. W., Tariq, F. S., Karam, D. S., Aris, A. Z., and Jamilu, G. (2019). The effects of rice husk ashes and inorganic fertilizers application rates on the phytoremediation of gold mine tailings by vetiver grass. Appl. Geochem. 108, 104366. doi:10.1016/j.apgeochem.2019.104366
Saygili, A. (2015). Use of waste marble dust for stabilization of clayey soil. Mater. Sci. 21. doi:10.5755/j01.ms.21.4.11966
Simandl, G. J., and Paradis, S. (2018). Carbonatites: Related ore deposits, resources, footprint, and exploration methods. Appl. Earth Sci. 127, 123–152. doi:10.1080/25726838.2018.1516935
Singh, J., and Bansal, R. S. (2015). Partial replacement of cement with waste marble powder with M25 grade. Int. J. Tech. Res. Appl. 3, 202–205.
Sivrikaya, O., Kıyıldı, K. R., and Karaca, Z. (2014). Recycling waste from natural stone processing plants to stabilise clayey soil. Environ. Earth Sci. 71, 4397–4407. doi:10.1007/s12665-013-2833-x
Song, U., and Lee, E. J. (2010). Environmental and economical assessment of sewage sludge compost application on soil and plants in a landfill. Resour. Conserv. Recycl 54, 1109–1116. doi:10.1016/j.resconrec.2010.03.005
Soni, A., Aseri, G. K., and Neelam, J. (2017). Impact of air pollution caused by mining and marble dust on foliar sensitivity through biochemical changes. Int. J. Eng. Res. Technol. 1, 1–4.
Thakur, A. K., Pappu, A., and Thakur, V. K. (2018). Resource efficiency impact on marble waste recycling towards sustainable green construction materials. Curr. Opin. Green Sustain. Chem. 13, 91–101. doi:10.1016/j.cogsc.2018.06.005
Tibane, L. V., and Mamba, D. (2023). Environmental risk assessment, principal component analysis, tracking the source of toxic heavy metals of solid gold mine waste tailings, South Africa. Environ. Forensics, 1–17. doi:10.1080/15275922.2023.2172478
Tozsin, G., Öztaş, T., Arol, A. İ., Kalkan, E., and Koç, E. (2015). The effects of marble wastes on acidic soil neutralization and hazelnut yield. J. Undergr. Resour., 29–36. doi:10.1016/j.jclepro.2014.06.0090
TS 1051 ISO 1015 (2001). Brown coals and lignites- determination of moisture content- direct volumetric method. Ankara, Turkey.
Ultra, V. U., Tirivarombo, S., Toteng, O., and Ultra, W. (2022). Enhanced establishment of colophospermum mopane (kirk ex benth.) seedlings for phytoremediation of Cu-Ni mine tailings. Environ. Sci. Pollut. Res. 29, 60054–60066. doi:10.1007/s11356-022-20116-1
Ural, N., and Yakşe, G. (2016). Waste marble partials expensing as way base material. Bilecik Seyh Edebali Univ. J. Sci. 2, 53–62. Available at: https://dergipark.org.tr/tr/pub/bseufbd/issue/22287/239047
Waheed, A., Arshid, M. U., Khalid, R. A., and Gardezi, S. S. S. (2021). Soil improvement using waste marble dust for sustainable development. Civ. Eng. J. 7, 1594–1607. doi:10.28991/cej-2021-03091746
Witham, F. H., Blaydes, D. F., and Devlin, R. M. (1971). Chlorophyll absorption spectrum and quantitative determination. Exp. plant physiology 1971, 162–167.
Yin, Y., Wang, X., Hu, Y., Li, F., and Cheng, H. (2023). Soil bacterial community structure in the habitats with different levels of heavy metal pollution at an abandoned polymetallic mine. J. Hazard Mater 442, 130063. doi:10.1016/j.jhazmat.2022.130063
Zhang, J., Liu, Y., Hong, S., Wen, M., Zheng, C., and Liu, P. (2023). Speciation analysis and pollution assessment of heavy metals in farmland soil of a typical mining area: A case study of dachang tin polymetallic ore, guangxi. Appl. Sci. 13, 708. doi:10.3390/app13020708
Keywords: marble, travertine, coal, waste, heavy metal analysis, beans, agriculture
Citation: Eker H (2023) Investigation of the usability of industrial mining wastes in agriculture. Front. Environ. Sci. 11:1248188. doi: 10.3389/fenvs.2023.1248188
Received: 26 June 2023; Accepted: 23 August 2023;
Published: 04 September 2023.
Edited by:
Vojtech Vaclavik, VSB-Technical University of Ostrava, CzechiaReviewed by:
Arvind Kumar Jha, Indian Institute of Technology Patna, IndiaCopyright © 2023 Eker. This is an open-access article distributed under the terms of the Creative Commons Attribution License (CC BY). The use, distribution or reproduction in other forums is permitted, provided the original author(s) and the copyright owner(s) are credited and that the original publication in this journal is cited, in accordance with accepted academic practice. No use, distribution or reproduction is permitted which does not comply with these terms.
*Correspondence: Hasan Eker, aGFzYW5la2VyQGthcmFidWsuZWR1LnRy
Disclaimer: All claims expressed in this article are solely those of the authors and do not necessarily represent those of their affiliated organizations, or those of the publisher, the editors and the reviewers. Any product that may be evaluated in this article or claim that may be made by its manufacturer is not guaranteed or endorsed by the publisher.
Research integrity at Frontiers
Learn more about the work of our research integrity team to safeguard the quality of each article we publish.