- 1Environmental Planning Program (EiABC), Addis Ababa University, Addis Ababa, Ethiopia
- 2GFA Consulting Group, GIZ Biodiversity, and Forests Program, Addis Ababa, Ethiopia
Assessment of habitat quality is important for the planning and management of conservation measures at a landscape level. The Alledeghi Wildlife Reserve (AWR) is an iconic wildlife conservation area because it not only contains charismatic wildlife species but also serves as a reliable livestock rangeland. The study aimed to assess habitat quality based on landscape-specific habitat threat information in the AWR using InVEST software. it is the first attempt to model the habitat quality of the landscape using expert-driven information. Six important threats were considered, namely, invasive species, bush encroachment, livestock incursion, fire, habitat destruction, and distance to roads. The quantified habitat quality was classified into low, moderate, and high. The results revealed that the quality of the habitat declined in the study area between 1998 and 2016. The high-quality habitat had a larger extent covering about 837 km2 (57.4%) in 1998 but it was reduced by 128 km2 (64%) during the study period. Conversely, moderate quality and low-quality habitats have increased from 78 km2 (5.35%) in 1998 to 206 km2 (14.12%) in 2016; and from 544 km2 (37.3%) in 1998 to 619 km2 (42.13%) in 2016 respectively. The decline in habitat quality was mainly associated with increased livestock incursion and expansion of invasive species which resulted in rapid land use changes. Thus, it is critical to undertake serious conservation measures to enhance biodiversity and ecosystem services in the AWR and to substantively contribute to the improved livelihood of the pastoral community.
1 Introduction
Biodiversity enhances ecosystem functionality which leads to improved ecosystem services through balancing and stabilizing ecological communities across scales (Cardinale et al., 2012; Oliver et al., 2015). The biodiversity of an area can be determined through habitat conditions whereas the importance of habitat depends on its quality (Basane and James, 2016). A particular habitat with special ecological importance is essential to the functioning of the wider ecosystem processes; such areas require extraordinary protection to safeguard the special value and vital ecosystem processes. Biological resources and the levels of biological reproduction and organism persistence have a greater effect on the ability of an ecosystem to provide living conditions for individual organisms and populations (Caro et al., 2020).
High-quality habitat is assumed as an indication of rich biodiversity (Norliyana and Mamat, 2020) and delivery of a suite of ecosystem services (Johnson, 2007; Stolton et al., 2010; Thomas et al., 2021). The state of biodiversity can be used as a basis proxy tool to measure the quality of a given habitat (Havlicek and Mitchell, 2014). Therefore, as a proxy for the condition of the state of biodiversity, quality habitat can indicate the capability of a given ecosystem to provide essential ecosystem services (Polasky et al., 2011) and as a determinant for measuring ecosystem health (Villamagna et al., 2013). The occurrence of diverse wildlife species is highly associated with the quality of habitat (Edmonds et al., 2021).
Habitat quality is an important indicator of regional ecological security (Zhu et al., 2015; Chen et al., 2016), which can reflect the level of regional biodiversity and ecosystem services (Tang et al., 2020; Zhu et al., 2020). Rigorous information on habitat quality is invaluable to making informed decisions on conservation planning and prioritization of conservation intervention strategies (Rouget et al., 2003; Baral et al., 2014; Simeneh et al., 2023) including expansion of important biodiversity areas, introduction and removal of species, and identification of principal habitat components (Basane and James, 2016) and determining of the key ecological attributes.
The landscape changes lead to corresponding modifications in the composition of the ecosystem and biodiversity (Liu et al., 2022). Further habitat quality changes affect the biodiversity and landscape pattern (Chu et al., 2018). Therefore, the occurrence of severe and complex ecological problems at landscape and species levels have a direct influence on the landscape pattern and habitat quality. Understanding the association between conservation challenges caused by land use change could provide a solution to ecological problems (Bai et al., 2019). Habitat loss consistently negatively affects species richness and population abundance (Laurance et al., 2002); and genetic diversity (Aguilar et al., 2008). The loss of critical habitats affects not only biodiversity but also directly impacts humans by decreasing the production of ecosystem services such as pollination (Potts et al., 2010), soil productivity and water provision (Bruijnzeel, 2004), and carbon storage and sequestration (Fargione et al., 2008).
The state of biodiversity, the range of habitats, and vegetation types across landscapes can be determined using the InVEST habitat quality and rarity models (Sharp et al., 2020; Liu et al., 2022). Thus, the changes in habitat quality are critical to the changes in ecosystem processes (Choudhary et al., 2021; Yang, 2021). Habitat quality monitoring provides robust information on ecological conditions and can be utilized as a basis for making habitat conservation interventions (Lin et al., 2016). Changes in habitat quality have tremendous implications for the conservation of wildlife species in savannah ecosystems (Kija et al., 2020) where the ecosystems are the principal habitats for diverse charismatic wildlife species and home to many iconic protected landscapes (Sinclair et al., 2007; Bohm and Hofer, 2018).
The Alledeghi Wildlife Reserve (AWR) is among the highly valued protected landscapes in Ethiopia which are highly pronounced with the assemblage of large mammals, but it is under severe conservation challenges, and the biodiversity endowment of the area particularly large mammals alarmingly declining (Fanuel, 2013; Simeneh et al., 2016). The important threats to biodiversity are steadily increasing such as the fast spread of invasive species, overgrazing, and bush encroachment (Almaz, 2009; Selamnesh, 2015) because of rapid Land Use Land Cover (LU/LC) changes, the ecosystem services values of the area have greatly declined (Simeneh, 2023). Moreover, urban development along the road is becoming an emerging conservation threat that will constrain the sustainability of the ecosystem (Almaz, 2009; Fanuel, 2013). Further, intensive charcoal production is well-pronounced in the entire area. Thus, this results in massive habitat destruction in the adjacent protected areas including the Awash National Park. Roadkill incidence has repeatedly occurred while wild animals are crossing the asphalted road in search of water (Simeneh et al., 2016). Fire incidence mainly in the highland forest is becoming a very common challenge for protected area management as local charcoal makers deliberately set fire to produce more charcoal.
The study hypothesizes that habitat quality declined over time in response to threat factors occurring in the study area. There is a lack of empirical studies conducted in the study area that assessed the status of the habitats to protect the values that the protected area possessed. Therefore, the novelty of this study is that it is the first attempt to model the quality of habitats of the protected landscape using expert-driven landscape threat information and analysis to indicate the state of the protected areas towards meeting its conservation goal. Therefore, this study aimed to assess the spatiotemporal changes in the quality of the habitat in the terrestrial ecosystems of AWR using InVEST software to provide a scientific basis for ecosystem planning interventions and prioritization of conservation management undertakings.
2 Methods and materials
2.1 Description of the study area
The AWR was established in the 1960 s (Hilliman, 1993). It is located in the Great Rift Valley in the northeastern region of the country between longitude 39°30′to 40°30′E and latitude 8°30′to 9°30′N, at 280 km east of Addis Ababa (Figure 1). The altitude ranges between 776 m and 2,445 m above sea level. The area is characterized by a semi-arid ecosystem with annual rainfall ranging between 400 and 700 mm. About 268 plant species and two types of ecosystems Dry evergreen montane forest and Acacia comiphora ecosystems (Addisu et al., 2017), 31 species of mammals, and over 140 avian species have been recorded (Hilliman, 1993; Fanuel, 2013) in the AWR. The most common wild animals inhabiting the reserve include the Grevy zebra (Equus grevyi), Beisa oryx (Oryx beisa beisa), Soemmering’s gazelle (Gazella soemmering), Gerenuk (Litocranius walleri) and lesser kudu (Tragelaphus imberbis) (Hilliman, 1993).
The major vegetation types in and around the reserve include grasslands, bushland, woodland, riverine forests, and highland forests (Almaz, 2009). The grassland plain stretching from the center of the reserve to the northwest was mainly occupied by grasses and occasionally with other herbs; the dominant species include Durfu (Chrysopogon plumulosus), Isisu (Chrysopogon schoenan) and Malif (Andropogon canaliculatus) (Almaz, 2009; Selamnesh, 2015). However, the rapid encroachment of shrub species and the rapid spread of invasive Prosopis juliflora and shrubs such as Combretum aculatum, Merua oblongflora, and Terminalia species have affected the grass species and the extent of the grassland habitat (Selamnesh, 2015; Simeneh, 2023). The bushland is an extensively increasing habitat type that possesses an assemblage of trees and shrubs (Simeneh, 2023). The habitat is mainly occurring in the southern, eastern, and northern edges of the landscape dominated by Acacia senegal (Almaz, 2010; Selamnesh, 2015). The common woody plant species in the AWR include Acacia tortilis, Acacia mellifera, Balanitis aegyptiaca, Cadaba, and Grewia species. The eastern mountainous section of the landscape is characterized by dense highland forest, common plant species include Cordia africana, Croton macrostachyus, Erythrina abyssinica, Juniperus procera, Olea europaea, Podocarpus falcatus, Pouteria altissima and Rhus vulgaris (Almaz, 2010). The riverine forests are a unique ecosystem and are important for the wild animals of the landscape. It is limited to seasonal streams and river courses where the water table is high.
2.2 Materials
2.2.1 Application of tools to assess habitat quality
Habitat quality can be assessed based on measured species diversity or through the analysis of the evolution of the habitat by parameter substitution (Andrus et al., 2021). In general, comparing observations to a standardized list of criteria can be used to assess the quality of a given habitat (Machado, 2004), and more recently the standardized modeling tool, particularly the Integrated Valuation of Ecosystem Services and Tradeoffs (InVEST) has been largely applied to measure the quality of habitat at various scales (Sharp et al., 2020). In this study, we assessed the state of habitats using InVEST software 3.11 version. The quantified habitat quality was classified by natural breaks into three classes (low, moderate, and high) (Kija et al., 2020).
2.2.2 The InVEST habitat quality model
The InVEST model provides good research methods and perspectives (Romero-Calcerrada and Luque, 2006; Terrado et al., 2016; Abreham et al., 2020). The model incorporates land use and biodiversity threats information to produce habitat quality maps. It uses the spatial extent of habitat quality as a proxy of biodiversity within the landscape, based on the proximity of the habitat to human-dominated land use and the intensity of disturbance caused by the land use (Sharp et al., 2020). The model considers that LU/LC with higher habitat quality is relatively intact and capable of supporting increased biodiversity and a lower habitat quality score indicates reduced biodiversity support and denotes a degraded landscape (Baral et al., 2014). The model is dependent on the relative impact of threats to the habitat, the distance between the threat sources and the habitat, and the sensitivities of the specific habitats to any possible threats, leading to habitat degradation (Sharp et al., 2020) (Table 1).
There are three key inputs to be considered for habitat quality mapping in InVEST model. First, the suitability of each LU/LC type (Hj) for providing habitat for biodiversity; second, anthropogenic threats that originate at pixel x (rx) affecting habitat quality; and third, the sensitivity of each LU/LC type to each threat (Table 1). For this study, six biodiversity threats were identified in the study area by following the approach of Terrado et al. (2016) and Wu et al. (2014). These were invasive species, bush encroachments, livestock incursion, fire, habitat destruction, and distance to roads (Table 2). The significance (weight) of each threat was prioritized based on the ecological and threat monitoring activities with two senior ecologists and five park rangers of the AWR between 3rd—4th December 2021 and the AHP method was applied to prioritize conservation threats following the approach by Terrado et al. (2016) and Wu et al. (2014) (Table 2; Figure 2).
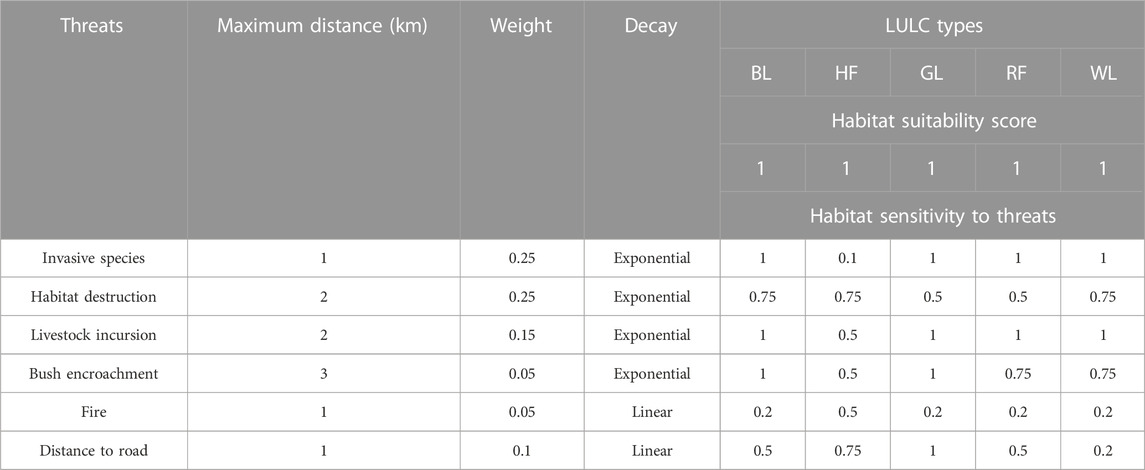
TABLE 2. Ecological habitat quality input data used for InVEST habitat quality model in the AWR (1998, 2016).
The total threat level in a grid cell x with LU/LCj is calculated as the relative habitat suitability score (Hj), from 0 to 1, where 1 indicates the highest suitability to species has been assigned to LU/LC types (Sharp et al., 2020). The last input of the model is the sensitivity of habitat type to different threats; helps to account for the differentiated impacts of threats to different habitats. The impacts of the threats on the habitat are determined by 1) the effect of the threat over space (irxy); 2) the relative weight of each threat’s importance compared to the others (wr), and 3) the relative sensitivity of each habitat to each threat (Sjr). The stress level Dxj of grid x with land-use type j is calculated as follows (Sharp et al., 2020).
Where, dxy is the linear distance between grid cells x and y, and drmax is the maximum effective distance of threats r’s across space.
where R is the number of threat factors, yr is the set of grid cells on r’s map, wr is the relative effect of each threat, θx is the level of accessibility to a grid cell x, and Sjr is the relative sensitivity of each habitat type to each threat.
The results of the model range from 0 to 1, with 1 representing the highest level of habitat quality (Sharp et al., 2020). The impacts of the threat on habitat decrease as the distance from the degradation sources increases, threats with higher destructive values (on the scale of 0-1) have higher impacts and the more sensitive a habitat type is to a threat (higher Sjr), the more degraded the habitat type could be by the threat.
Habitat quality is the environmental level that the ecological environment provides for the survival of individual organisms and populations. It is a continuous variable with a numerical range from low to high. The higher the quality of the habitat, the more stable the ecological structure and function of the patch. The way and intensity of human land use determines the quality of the habitat, and the more intense the land use, the more pronounced the decline in habitat quality (Almpanidou et al., 2014). Habitat quality was calculated based on the degree of habitat degradation, and the habitat quality score decreased with increasing habitat degradation score. The calculation formula for habitat quality is as follows:
Where, Qxj is the habitat quality of grid cell x in land cover type j; Hj is the habitat suitability of land cover type j; D2 xj is the level of habitat threat for grid cell x in land cover type j; k is the half-saturation factor, which is generally taken as half of the maximum value of D2 xj; and x is a constant.
The information obtained from expert judgment and AHP was verified by undertaking field assessments.
2.3 Data preparation and input for the InVEST habitat quality model
The data inputs (spatial and non-spatial) are required to run the InVEST habitat quality model (Figure 2). Thus, LU/LC maps, threat sources, and impacts, habitat types, habitat sensitivity to each threat, and half-saturation constant were the required inputs (Sharp et al., 2020). The information on LU/LC was obtained from the previous study made by Simeneh (2023) in the study area. All the required inputs such as LU/LC maps of the respective years (1998–2016), threat sources and impacts, habitat types, and habitat sensitivity to each threat were loaded to run the habitat quality model. Finally, habitat quality maps for each respective year were produced; the final habitat quality maps were classified into three classes (low, moderate, and high).
3 Results and discussions
The result revealed an overall habitat quality reduction during the study period (Table 3; Figure 3). The model showed that the ecosystem was dominated by a high-quality habitat of 837 km2 (57.4%) followed by a moderate-quality habitat of 544 km2 (37.3%), and a low-quality habitat of 78 km2 (5.35%) in 1998. In the subsequent 18 years (1998–2016), the low-quality and moderate-quality habitats increased by 128 km2 (62%), and 75 km2 (12%) respectively, while the high-quality habitats decreased by 203 km2 (32%). The extent of high-quality habitat largely declined during the study period from 837 km2 (57.4%) to 534 km2 (43.45%). Conversely, moderate-quality, and low-quality habitats have increased from 78 km2 (5.35%) in 1998 to 206 km2 (14.12%) in 2016; and from 544 km2 (37.3%) in 1998 to 619 km2 (42.13%) in 2016 respectively.
This study was the first to assess habitat quality using InVEST model and expert-driven approach in Ethiopia’s highly valued protected landscape. Thus, the study provides robust information that can be used for threat reduction planning and management intention in the study landscape. The habitat quality changes in the study area were highly associated with increased livestock incursion and expansion of invasive species resulting in severe changes in the healthy functioning of the ecosystems. The quality of habitat influences wildlife species diversity, density, distribution, and movement patterns in landscapes (Zhang et al., 2019; Dai et al., 2018). The decline in habitat quality is mainly attributed to increased conservation threats including the incursion of livestock and human interactions into wildlife habitats (Carter et al., 2014). Free grazing activities have an adverse negative impact on habitat quality (Su et al., 2020). Likewise, the quality of the habitat has been significantly declining particularly the grassland habitat was deteriorated by massive livestock incursion in the study landscape, which is a common prolonged problem in protected areas of Ethiopia (Mekbeb et al., 2022). Similar results were reported by Kija et al. (2020) that habitat quality has largely deteriorated by anthropogenic activities and land use policy changes in the Greater Serengeti Ecosystem of Tanzania.
Overall, high–quality 1998 became a moderate-quality and low-quality habitat during 1998–2016. The loss of habitat quality is well pronounced in the grassland habitat of the protected area which is the preferred feeding and breeding habitat for charismatic ungulate species and other wild animals of the AWR. The swift spread of invasive species coupled with livestock grazing and habitat destruction significantly affects the grassland habitat of the protected area. The savannah grassland habitat is the most preferred and suitable habitat for the charismatic plain animal of the reserve but under severe pressure, particularly invasive Prosophis juliflora in the grassland habitat is the principal conservation challenge for the protected area management. The communities are reliant upon livestock rearing and natural resources due to a lack of alternatives leading to overgrazing; unmanaged grazing practices are resulting in significant degradation of principal ecological habitats such as the grassland habitat in many protected area systems in Ethiopia. Due to the high livestock density in the area, the grassland habitat of the landscape has encountered severe grazing practices year after year. This has led to the deterioration of grassland habitat quality and a reduction in the capacity to provide forage for grassland-reliant wild animal species.
Land use and land cover changes can be taken as the prime factors for changes in habitat quality in the study area during the study period. The low-quality habitat has slightly shifted from the center of the highland forest to the center of the landscape which is occupied by the grassland habitat of the AWR (Figure 3); this is mainly due to the spread of invasive species, livestock incursion, and the closeness to the tarmac road. The highland forest has been unwisely utilized for various purposes mainly for charcoal production, however, improved management intervention in the highland forest contributed to the management of illegal activities thus the habitat has rapidly been restored (Simeneh, 2023). Conversely, the grassland habitat was largely converted into low habitat quality as unrestricted grazing led to reducing the quality of grassland habitat. The woodland, riverine forest, and partially bushland habitats have been unchanged in terms of quality and maintained high habitat quality during the study period. According to Fanuel (2013), the study landscape has lost about 52% of its quality to conserve the larger charismatic herbivores of the landscape. Similarly, this finding showed that only 634 km2 (43.45%) of the landscape sustains its high quality to possess charismatic species of the landscape. This indicates that the protected landscape is losing its quality habitats to possess the endangered iconic species. Maintaining high-quality habitats could enhance the stability of ecosystem structure and function and the quick recovery potential of habitats after disturbance (Schwarz et al., 2017; Wu et al., 2017).
Unlike most areas of Ethiopia in which invasive species were spread due to the road access; in the Afar area, where the landscape is located; Prosopis juliflora was introduced mainly for water and soil conservation and to support livestock forage in the dry season in the late 1970 s and early 1980 s (Ayanu et al., 2014; Kebede and Coppock, 2015; Hailu et al., 2019). Further, additional plantations were made between the 1980 s and 1990 s as shade and wind protection trees in villages, and the raw material was used for firewood fencing, and building materials (Ayanu et al., 2014). Livestock has been identified as the principal vector for the rapid spread of invasive species and the invasion become a serious problem that started rapidly invading the rangeland (Hailu et al., 2019). The invasion could significantly affect the ecosystem services and livelihood of pastoralist communities by reducing biodiversity, grazing land, and water supply (Shackleton et al., 2014).
4 Limitations of the study
The assessment of habitat quality using the InVEST model has been successfully employed for the maintenance of biodiversity and is invaluable for the management of the landscape and land-use planning (Sharp et al., 2020) but inadequate information about the spatial and temporal distribution of species across the protected landscape (Stephen et al., 2011) is the major limitations of the InVEST habitat quality model. It is, therefore, important to conduct a field-based habitat suitability assessment to obtain ecologically valid and robust information on the distribution of quality habitat and species abundance across the landscape (Nagendra et al., 2013).
5 Conclusion
This study has assessed the quality of habitat using expert-driven landscape habitat threat information in InVEST software in the most iconic but greatly threatened protected landscape of the AWR in Ethiopia. Assessing a landscape’s habitat quality has greater implications for the larger rangeland ecosystem management since it directly impacts landscape structure and spatial pattern. The most important factors for the decline of habitat quality include livestock incursion and the expansion of invasive species. Therefore, it can be concluded that the continued fast spread of invasive species and bush encroachment in the critical feeding and breeding habitat can largely influence the biodiversity and ecosystem services of AWR. Thus, it is critical to undertake serious conservation measures to maintain the ecosystem’s integrity and halt biodiversity loss. Further, the boundary of the AWR has not been clearly defined and has not been legally gazetted yet. Therefore, the findings of this study can be used to redefine the landscape boundary to encompass the most critical habitat under legal protection.
Data availability statement
The raw data supporting the conclusion of this article will be made available by the authors, without undue reservation.
Author contributions
SA designed and conceptualized the research collected relevant data, analyzed data, and wrote the draft manuscript.
Acknowledgments
The authors are thankful for the kind cooperation of ecological monitoring experts of the AWR and EWCA and rangers of AWR.
Conflict of interest
Author SA was employed by GFA Consulting Group.
Publisher’s note
All claims expressed in this article are solely those of the authors and do not necessarily represent those of their affiliated organizations, or those of the publisher, the editors and the reviewers. Any product that may be evaluated in this article, or claim that may be made by its manufacturer, is not guaranteed or endorsed by the publisher.
References
Abreham, B., Noszczyk, T., Soromessa, T., and Elias, E. (2020). The InVEST habitat quality model associated with land use/cover change in the winike watershed in the omo-gibe basin, southwest Ethiopia. Remote Sens. 12, 1103.
Addisu, A., Ali, S., and Ahmed, E. (2017). Floristic composition and community analysis of woody plants in hallideghie wildlife reserve, north-east Ethiopia. Ethiop. J. Environ. Stud. Manag. 10 (7), 917–930.
Aguilar, R., Quesada, M., Ashworth, L., Herrerias, D. Y., and Lobo, J. (2008). Genetic consequences of habitat fragmentation in plant populations: susceptible signals in plant traits and methodological approaches. Mol. Ecology17 17, 5177–5188. doi:10.1111/j.1365-294x.2008.03971.x
Almaz, T. (2009). Sustaining the Alledeghi grassland of Ethiopia: influences of pastoralism and vegetation change. (PhD dissertation). Logan: Utah State University.
Almpanidou, V., Mazaris, A. D., Mertzanis, Y., Avraam, I., Antoniou, I., Pantis, J. D., et al. (2014). Providing insights on habitat connectivity for male brown bears: a combination of habitat suitability and landscape graph-based models. Ecol. Model 286, 37–44. doi:10.1016/j.ecolmodel.2014.04.024
Andrus, R. A., Martinez, A. J., Jones, G. M., and Meddens, A. J. H. (2021). Assessing the quality of fire refugia for wildlife habitat. Ecol. Manage 482, 118868. doi:10.1016/j.foreco.2020.118868
Ayanu, Y., Jentsch, A., Müller-Mahn, D., Rettberg, S., Romankiewicz, C., and Koellner, T. (2014). Ecosystem engineer unleashed: Prosopis juliflora threatening ecosystem services? Reg. Environ. Chang. 15, 155–167. doi:10.1007/s10113-014-0616-x
Bai, L., Xiu, C., Feng, X., and Liu, D. (2019). Influence of urbanization on regional habitat quality: a case study of Changchun City. Habitat Int. 93, 102042. doi:10.1016/j.habitatint.2019.102042
Baral, H., Keenan, R. J., Sharma, S. K., Stork, N. E., and Kasel, S. (2014). Spatial assessment and mapping of biodiversity and conservation priorities in a heavily modified and fragmented production landscape in north-central Victoria, Australia. Ecol. Indic. 36, 552–562. doi:10.1016/j.ecolind.2013.09.022
Basane, C., and James, G. (2016). Habitat assessment for ecosystem services in South Africa. Int. J. Biodivers. Sci. Ecosyst. Serv. Manag. 12 (4), 242–254. doi:10.1080/21513732.2016.1217935
Bohm, T., and Hofer, H. (2018). Population numbers, density and activity patterns of servals in savannah patches of Odzala-Kokoua National Park, Republic of Congo. Afr. J. Ecol. 56, 841–849. doi:10.1111/aje.12520
Bruijnzeel, L. A. (2004). Hydrological functions of tropical forests: not seeing the soil for the trees? Agric. Ecosyst. Environ. 104, 185–228. doi:10.1016/j.agee.2004.01.015
Cardinale, B. J., Emmett Duffy, J., Gonzalez, A., Hooper, D. U., Perrings, C., Venail, P., et al. (2012). Michel loreau, James B. Grace, anne larigauderie, diane S. Srivastava and shahid naeem (2012). Biodiversity loss and its impact on humanity. Nature 486, 11148.
Carter, N. H., Viña, A., Hull, V., McConnell, W. J., Axinn, W., Ghimire, D., et al. (2014). Coupled human and natural systems approach to wildlife research and conservation. Ecol. Soc. 19, 43. doi:10.5751/es-06881-190343
Chen, Y., Qiao, F., and Jiang, L. (2016). Effects of land use pattern change on regional scalehabitat quality based on InVEST modelda case study in Beijing. Acta Sci. Nat. Univ. Pekin. 52, 553–562.
Choudhary, A., Deval, K., and Joshi, P. K. (2021). Study of habitat quality assessment using geospatial techniques in Keoladeo National Park, India. Environ. Sci. Pollut. Res. 28, 14105–14114. doi:10.1007/s11356-020-11666-3
Chu, L., Sun, T., Wang, T., Li, Z., and Cai, C. (2018). Evolution and prediction of landscape pattern and habitat quality based on CA-markov and InVEST model in hubei section of three gorges reservoir area (TGRA). Sustainability 11, 3854. doi:10.3390/su10113854
Claudia, C., João Carlos, M., Cunha, P. P., and Teixeira, Z. (2020). Ecosystem services as a resilience descriptor in habitat risk assessment using the InVEST model. Ecol. Indic. 115, 106426. doi:10.1016/j.ecolind.2020.106426
Dai, L., Li, S., Lewis, B. J., Wu, J., Yu, D., Zhou, W., et al. (2018). The influence of land use change on the spatial–temporal variability of habitat quality between 1990 and 2010 in Northeast China. J. For. Res. 30, 2227–2236. doi:10.1007/s11676-018-0771-x
Edmonds, N. J., Al-Zaidan, A. S., Al-Sabah, A. A., Le Quesne, W. J. F., Devlin, M. J., Davison, P. I., et al. (2021). Kuwait’s marine biodiversity: qualitative assessment of indicator habitats and species. Mar. Pollut. Bull. 163, 111915. doi:10.1016/j.marpolbul.2020.111915
Fargione, J., Hill, J., Tilman, D., Polasky, S., and Hawthorne, P. (2008). Land clearing and the biofuel carbon debt. Land Clear. Biofuel Carbon Debt 319 (5867), 1235–1238. doi:10.1126/science.1152747
Hailu, S., Bewket, W., Alamirew, T., Zeleke, G., Demel, T., Ketema, B., et al. (2019). Implications of land use/land cover dynamics and Prosopis invasion on ecosystem service values in Afar Region, Ethiopia. Sci. Total Environ. 675, 354–366. doi:10.1016/j.scitotenv.2019.04.220
Havlicek, E., and Mitchell, E. A. D. (2014). “Soils supporting biodiversity,” in Interactions in soil: promoting plant growth.
Hilliman, J. C. (1993). Compendium of wildlife conservation information: information on wildlife conservation areas. NYZS and EWCO. Addis Ababa.
Johnson, M. D. (2007). Measuring habitat quality: a review. Condor 109, 489–504. doi:10.1093/condor/109.3.489
Kebede, F. (2013). Ecology and Community Based Conservation of the Grevy’s zebra (Equus grevyi) and the African wild ass (Equus africanus) in the Afar region, Ethiopia PhD dissertation. Addis Ababa: Addis Ababa University.
Kebede, F., Afework, B., Moehlman, P. D., and Evangelista, P. H. (2012). Endangered Grevy’s zebra in the Alledeghi Wildlife Reserve, Ethiopia: species distribution modeling for the determination of optimum habitat. Endang Species Res. 17, 237–244. doi:10.3354/esr00416
Kebede, T., and Coppock, L. (2015). Livestock-mediated dispersal of Prosopis juliflora imperils grasslands and the endangered Grevy's zebra in Northeastern Ethiopia. Rangel. Ecol. Manag. 68, 402–407. doi:10.1016/j.rama.2015.07.002
Kija, H. K., Ogutu, J. O., Mangewa, L. J., Bukombe, J., Verones, F., Graae, B. J., et al. (2020). Spatio-temporal changes in wildlife habitat quality in the greater Serengeti ecosystem. Sustainability 12, 2440. doi:10.3390/su12062440
Laurance, W. F., Lovejoy, T. E., Vasconcelos, H. L., Bruna, E. M., Didham, R. K., Stouffer, P. C., et al. (2002). Ecosystem decay of Amazonian Forest fragments: a 22-year investigation. Conserv. Biology16 16, 605–618. doi:10.1046/j.1523-1739.2002.01025.x
Lin, Q., Mao, J., Wu, J., Li, W., and Yang, J. (2016). Ecological security pattern analysis based on InVEST and least-cost path model: a case study of Dongguan water village. Sustainability 8, 172. doi:10.3390/su8020172
Liu, S., Liao, Q., Xiao, M., Zhao, D., and Huang, C. (2022). Spatial and temporal variations of habitat quality and its response of landscape dynamic in the three gorges reservoir area, China. Int. J. Environ. Res. Public Health 19, 3594. doi:10.3390/ijerph19063594
Machado, A. (2004). An index of naturalness. J. Nat. Conservation 12, 95–110. doi:10.1016/j.jnc.2003.12.002
Mekbeb, T., Asefa, A., and Delelegn, Y. (2022). National biodiversity threat assessment: ranking major threats impacting Ethiopia’s biodiversity. Addis Ababa, Ethiopia: IUCN.
Nagendra, H., Lucas, R., Honrado, J. P., Jongman, R. H. G., Tarantino, C., Adamo, M., et al. (2013). Remote sensing for conservation monitoring: assessing protected areas, habitat extent, habitat condition, species diversity, and threats. Ecol. Indic. 33, 45–59. doi:10.1016/j.ecolind.2012.09.014
Norliyana, A., and Mamat, M. (2020). Spatial analysis model assessing habitat quality of selangor. IOP Conf. Ser. Earth Environ. Sci. 549, 012049. doi:10.1088/1755-1315/549/1/012049
Oliver, T. H., Heard, M. S., Isaac, N. J. B., Roy, D. B., Procter, D., Eigenbrod, F., et al. (2015). Biodiversity and resilience of ecosystem functions. Trends Ecol. Evol. 11, 673–684. doi:10.1016/j.tree.2015.08.009
Polasky, S., Nelson, E., Pennington, D., and Johnson, K. A. (2011). The impact of land-use changes on ecosystem services, biodiversity and returns to Landowners: a case study in the state of Minnesota. Environ. Resour. Econ. 48, 219–242.
Potts, S. G., Biesmeijer, J. C., Kremen, C., Neumann, P., Schweiger, O., and Kunin, W. E. (2010). Global pollinator declines: trends, impacts and drivers. Trends Ecol. Evol. 25, 345–353. doi:10.1016/j.tree.2010.01.007
Romero-Calcerrada, R., and Luque, S. (2006). Habitat quality assessment using Weights-of-Evidence based GIS modelling: the case of Picoides tridactylus as species indicator of the biodiversity value of the Finnish forest. Ecol. Model. 196, 62–76. doi:10.1016/j.ecolmodel.2006.02.017
Rouget, M., Richardson, D. M., Cowling, R. M., Lloyd, J. W., and Lombard, A. T. (2003). Current patterns of habitat transformation and future threats to biodiversity in terrestrial ecosystems of the Cape floristic region, South Africa. Biol. Conserv. 112, 63–85. doi:10.1016/s0006-3207(02)00395-6
Schwarz, N., Moretti, M., Bugalho, M. N., Davies, Z. G., Haase, D., Hack, J., et al. (2017). Understanding biodiversity-ecosystem service relationships in urban areas: a comprehensive literature review. Ecosyst. Serv. 27, 161–171. doi:10.1016/j.ecoser.2017.08.014
Selamnesh, T. (2015). Impact of Prosopis juliflora on plant biodiversity at Alledeghi wildlife reserve and surrounding local community, Ethiopia. MSc thesis. Addis Ababa: Ababa University.
Shackleton, R. T., Le Maitre, C., Pasiecznik, N. M., and Richardson, D. M. (2014). Prosopis: a global assessment of the biogeography, benefits, impacts, and management of one of the world's worst woody invasive plant taxa. AOB Plants 2014 (6), plu027. doi:10.1093/aobpla/plu027
Sharp, R., Douglass, J., Wolny, S., Arkema, K., Bernhardt, J., Bierbower, W., et al. (2020). InVEST user’s guide. The natural capital project. Stanford University, University of Minnesota, The Nature Conservancy, and World Wildlife Fund.
Simeneh, A. (2023). Assessing the impact of land use land cover changes on ecosystem services in the Alledighe Wildlife Reserve, Ethiopia: implication for rangeland ecosystem management. Manuscript under review.
Simeneh, A., Afework, B., and Assefa, A. (2016). Population size and structure of beisa oryx and its habitat overlap with sympatric species in Alledeghi wildlife reserve, NE, Ethiopia. Ethiop. J.Biol. Sci. 15 (1), 37–54.
Simeneh, A., Yeshitela, K., and Argaw, M. (2023). Assessing habitat quality using the InVEST model in the Dire and Legedadi watersheds, central highland of Ethiopia. Sustain. Environ., 2765–8511.
Sinclair, A. R., Mduma, S. A., Hopcraft, J. G., Fryxell, J. M., Hilborn, R., and Thirgood, S. (2007). Long-term ecosystem dynamics in the Serengeti: lessons for conservation. Conserv. Biol. 21, 580–590. doi:10.1111/j.1523-1739.2007.00699.x
Stephen, P., Erik Jeremy, N., Pennington, D. N., and Johnson, K. A. (2011). The impact of land-use changes on ecosystem services, biodiversity and returns to landowners: a case study in the state of Minnesota. Environ. Resour. Econ. 48 (2), 219–242.
Stolton, S., Mansourian, S., and Dundley, N. (2010). Valuing protected areas. Washington (DC): world Bank`s global environment facility coordination team. World Bank GEF Operations.
Su, X., Liu, Y., Zhou, W., and Liu, G. (2020). “An overview on status and importance of Tibetan Plateau steppe, China,” in Reference module in earth systems and environmental sciences (Amsterdam: Elsevier).
Tang, F., Fu, M., Wang, Li, and Zhang, P. (2020). Land-use change in Changli County, China: predicting its spatio-temporal evolution in habitat quality. Ecol. Indic. 117, 106719. doi:10.1016/j.ecolind.2020.106719
Terrado, M., Sabater, S., Chaplin-Kramer, B., Mandle, L., Ziv, G., and Acuna, V. (2016). Model development for the assessment of terrestrial and aquatic habitat quality in conservation planning. Sci. Total Environ. 540, 63–70. doi:10.1016/j.scitotenv.2015.03.064
Thomas, E., Jansen, M., Chiriboga-Arroyo, F., Wadt, L. H. O., Corvera-Gomringer, R., Atkinson, R. J., et al. (2021). Habitat quality differentiation and consequences for ecosystem service provision of an amazonian hyperdominant tree species. Front. Plant Sci. 12, 621064. doi:10.3389/fpls.2021.621064
Villamagna, A. M., Angermeier, P. L., and Bennett, E. M. (2013). Capacity, pressure, demand, and flow: a conceptual framework for analyzing ecosystem service provision and delivery. Ecol. Complex. 15, 114–121. doi:10.1016/j.ecocom.2013.07.004
Wu, C.-F., Lin, Y. P., Chiang, L. C., and Huang, T. (2014). Assessing highway’s impacts on landscape patterns and ecosystem services: a case study in Puli Township, Taiwan. Landsc. Urban Plan. 128, 60–71. doi:10.1016/j.landurbplan.2014.04.020
Wu, J., Yue, X., and Qin, W. (2017). The establishment of ecological security patterns based on the redistribution of ecosystem service value: a case study in the Liangjiang New Area, Chongqing. Geogr. Res. 36, 429–440.
Yang, Y. (2021). Evolution of habitat quality and association with land-use changes in mountainous areas: a case study of the Taihang Mountains in Hebei Province, China. Ecol. Indic. 129, 107967. doi:10.1016/j.ecolind.2021.107967
Yohannes, H., Soromessa, T., Argaw, M., and Dewan, A. (2021). Spatio-temporal changes in habitat quality and linkage with landscape characteristics in the Beressa watershed, Blue Nile basin of Ethiopian highlands. J. Environ. Manag. 281, 111885. doi:10.1016/j.jenvman.2020.111885
Zhang, H. B., Wu, F. E., Zhang, Y. N., Han, S., and Liu, Y. Q. (2019). Spatial and temporal changes of habitat quality in jiangsu yancheng wetland national nature reserve-rare birds of China. Appl. Ecol. Environ. Res. 17, 4807–4821. doi:10.15666/aeer/1702_48074821
Zhu, C., Zhang, X., Zhou, M., He, S., Gan, M., Yang, L., et al. (2020). Impacts of urbanization and landscape pattern on habitat quality using OLS and GWR models in Hangzhou, China. Ecol. Indic. 117, 106654. doi:10.1016/j.ecolind.2020.106654
Keywords: AWR, biodiversity, ecosystem quality, InVEST, rangeland
Citation: Admasu S (2023) Modeling habitat quality for rangeland ecosystem restoration in the Alledeghi Wildlife reserve, Ethiopia. Front. Environ. Sci. 11:1244238. doi: 10.3389/fenvs.2023.1244238
Received: 22 June 2023; Accepted: 25 October 2023;
Published: 07 November 2023.
Edited by:
Jie Luo, Zhejiang Lab, ChinaReviewed by:
Thabiso Sebolai, Department of Agricultural Research (DAR), MyanmarAbel Ramoelo, University of Pretoria, South Africa
Copyright © 2023 Admasu. This is an open-access article distributed under the terms of the Creative Commons Attribution License (CC BY). The use, distribution or reproduction in other forums is permitted, provided the original author(s) and the copyright owner(s) are credited and that the original publication in this journal is cited, in accordance with accepted academic practice. No use, distribution or reproduction is permitted which does not comply with these terms.
*Correspondence: Simeneh Admasu, c2ltZW5laDIwMDlAeWFob28uY29t, c2ltZW5laGEyMDA5QGdtYWlsLmNvbQ==