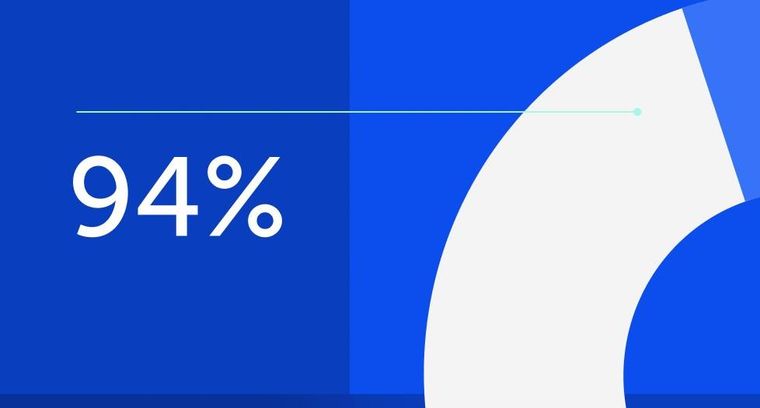
94% of researchers rate our articles as excellent or good
Learn more about the work of our research integrity team to safeguard the quality of each article we publish.
Find out more
ORIGINAL RESEARCH article
Front. Environ. Sci., 16 November 2023
Sec. Freshwater Science
Volume 11 - 2023 | https://doi.org/10.3389/fenvs.2023.1243777
Introduction: Climate change alters environmental and climatic conditions, leading to expansion or contraction and possible shifts in the geographical distribution of vectors that transmit diseases. Bulinus globosus and Biomphalaria pfeifferi are the intermediate host snails for human schistosomiasis in KwaZulu-Natal (KZN) province, South Africa.
Methods: Using the Maximum entropy (MaxEnt) model, we modelled the current and future distribution of human schistosomiasis intermediate host snails in KZN using two representation concentration pathways (RCP4.5 and RCP8.5) for the year 2085. Thirteen and ten bioclimatic variables from AFRICLIM were used to model the habitat suitability for B. globosus and B. pfeifferi, respectively. The Jack-knife test was used to evaluate the importance of each bioclimatic variable.
Results: Mean temperature warmest quarter (BIO10, 37.6%), the number of dry months (dm, 32.6%), mean diurnal range in temperature (BIO2, 10.8%), isothermality (BIO3, 6.7%) were identified as the top four bioclimatic variables with significant contribution to the model for predicting the habitat suitability for B. globosus. Annual moisture index (mi, 34%), mean temperature warmest quarter (BIO10, 21.5%), isothermality (BIO3, 20.5%), and number of dry months (dm, 7%) were identified as the four important variables for the habitat suitability of B. pfeifferi. Area under the curve for the receiving operating characteristics was used to evaluate the performance of the model. The MaxEnt model obtained high AUC values of 0.791 and 0.896 for B. globosus and B. pfeifferi, respectively. Possible changes in the habitat suitability for B. globosus and B. pfeifferi were observed in the maps developed, indicating shrinkage and shifts in the habitat suitability of B. pfeifferi as 65.1% and 59.7% of the current suitable habitats may become unsuitable in the future under RCP4.5 and RCP8.5 climate scenarios. Conversely, an expansion in suitable habitats for B. globosus was predicted to be 32.4% and 69.3% under RCP4.5 and RCP8.5 climate scenarios, with some currently unsuitable habitats becoming suitable in the future.
Discussion: These habitat suitability predictions for human schistosomiasis intermediate host snails in KZN can be used as a reference for implementing long-term effective preventive and control strategies for schistosomiasis.
Climate change threatens aquatic ecosystems including freshwater ecosystems which are at high risk, particularly where humans already exploit these systems De Necker (De Necker, 2020). According to the sixth assessment report (AR6) produced by the Intergovernmental Panel on Climate Change (IPCC), global surface temperature will reach or exceed 1.5°C in the near term (2021–2040) relative to 1860–1900 (pre-industrial era) even for the very low greenhouse gas emissions (Pörtner et al., 2022). Shifts in precipitation patterns, temperature rise, and increased frequency and severity of extreme climate events (e.g., droughts and flooding) are three major components of ongoing climate change (Stensgaard et al., 2019). Climate change leads to environmental changes that affect food security, air quality, the distribution and occurrence of various diseases. In South Africa’s interior, it is predicted that mean annual air temperatures will increase by 3°C–3.5°C while the coastal regions will experience a 1.5°C–2.5°C increase. In summer rainfall areas, the mean annual rainfall will increase by 40–80 mm per decade, while this will decrease by 20–40 mm per decade in winter rainfall areas. Limpopo, Mpumalanga, and Kwa-Zulu Natal (summer rainfall regions) will therefore experience hotter and wetter conditions in summer and autumn. In contrast, the Western Cape and southern parts of the Eastern Cape (winter rainfall regions) will experience hotter and drier winter conditions (De Necker, 2020).
Schistosomiasis is a chronic and debilitating neglected tropical disease caused by infection with parasitic blood flukes of the genus Schistosoma, which uses freshwater snails as intermediate hosts (Ahmad, 2022). Infection occurs in humans when they have contact with waterbodies that contain intermediate host snails contaminated with parasites. Parasites released from the contaminated snails penetrate human skin causing infection (Sokolow et al., 2016; De Necker, 2020). Schistosomiasis is endemic in 78 countries, with more than 800 million people at risk and over 200 million people infected, with the majority from sub-Saharan Africa (Sokolow et al., 2016; Ogongo et al., 2022). More than 4 million people are estimated to be infected with schistosomiasis in South Africa. Limpopo, Mpumalanga, Eastern Cape, and KwaZulu-Natal are the provinces where the disease is endemic (Magaisa et al., 2015; De Boni et al., 2021). Bulinus globosus and Biomphalaria pfeifferi are the intermediate host snails for Schistosoma haematobium which causes urogenital schistosomiasis, and Schistosoma mansoni which causes intestinal schistosomiasis in South Africa, respectively (Nwoko et al., 2022a).
Transmission of schistosomes depends on the spatial and temporal distribution of specific freshwater snails that are intermediate hosts and are the prerequisite that Schistosoma parasites to reach the development stage that infects humans. The global strategy endorsed by the World Health Organization to control schistosomiasis is the large-scale administration of the antischistosomal drug praziquantel to at-risk populations to prevent morbidity. The sustainability of this control strategy is now questionable as there is rapid re-infection after deworming. In recent years, a shift occurred from morbidity control to transmission control and local elimination, and hence, much attention is being given to control of intermediate host snails at transmission sites, along with primary prevention tailored to specific social-ecological systems (Walz et al., 2015).
The spatial and seasonal distribution of schistosomiasis intermediate host snails have been studied (Opisa et al., 2011; Manyangadze et al., 2021; Nwoko et al., 2022b; Nwoko et al., 2023). Physico-chemical properties, climatic and environmental factors such as water depth (Boelee and Laamrani, 2004), temperature, rainfall (Stensgaard et al., 2013) are known to affect the distribution, reproduction, fecundity, and survival of schistosomiasis intermediate host snails. Therefore, rising water temperatures and altered precipitation associated with climate change could considerably alter the distribution and abundance of the intermediate host snail and schistosome parasites, resulting in a shift in the transmission dynamics of the disease (McCreesh et al., 2015). Assessing the compounded impact of climate change are important challenges that will affect global health. Analysis of variance and generalized mixed effect models are commonly used to determine the spatial and seasonal distribution of schistosomiasis intermediate host snails but they do not predict the habitat suitability of the intermediate host snails (Opisa et al., 2011; Rabone et al., 2019). However, due to advances in technology, improved statistical and modelling techniques such as species distribution models, it is now possible to do that.
Species Distribution Model (SDM) is used to determine the potential distribution and habitat suitability of species based on the relationship between environmental factors, climatic factors and species occurrence (Liu et al., 2022). Among the SDMs, the maximum entropy (MaxEnt) model is a machine learning algorithm based on the principle that estimates the probability of species presence (Duan et al., 2014). MaxEnt model is a commonly used SDM because of its ability to model presence-only data as absence data are rarely available, and very unreliable when available (Phillips et al., 2006). Also, the model is robust in modelling data with small sample size as well as complex relations between the independent and dependent variables. Finally, the prediction results from a MaxEnt model is high, accurate and reproducible (Kaky et al., 2020; Gunawan et al., 2021). Several studies have used SDMs to predict the spatial distribution of schistosomiasis intermediate host snails on present-day climate, and to predict the distribution on a future climate using historical presence and absence data at national, continental, and global levels (Stensgaard et al., 2013; Pedersen et al., 2014; Manyangadze et al., 2016; Stensgaard et al., 2016).
Manyangadze, Chimbari (Manyangadze et al., 2016) modelled the spatial and seasonal distribution of suitable habitats of intermediate host for Schistosoma spp using the Maxent model without the aspect of climate change. However, this study was carried out in Ndumo area in uMkhanyakude district. It is in the north-eastern part of KwaZulu-Natal (KZN) province, bordered by Swaziland and Mozambique to the north-west and north, respectively. The area is approximately
This study was carried out in KZN province, South Africa. It is located in the southeast of the country, with a long shoreline on the Indian Ocean and sharing borders with three provinces (Free state, Eastern Cape, and Mpumalanga) and three countries; Mozambique, Eswatini, and Lesotho. It is the second-most populous province in South Africa with a population of about 11 million people and an area approximately 94,361 km2 (Khumalo et al., 2022). KZN comprises of ten district municipalities: Amajuba, iLembe, Ugu, uMgungundlovu, uThukela, uMkhanyakude, King Cetshwayo (formerly uThugulu), uMzinyathi, Harry Gwala (formerly Sisonke), and Zululand; and one metropolitan municipality: eThekwini (Figure 1). KwaZulu-Natal’s climate differs from subtropical to temperate with four seasons: rainy (December–February), post-rainy (March–May), cold/dry (June–August), and hot/dry (September–November). KwaZulu-Natal province is characterized by major rivers such as Mfolozi, Nyalazi, Tugela, Pongolo, and uMvoti amongst others. The province consists of three distinct geographical areas, namely, the lowland Indian Ocean coastal region, the central Natal Midlands and the mountainous areas of the Drakensberg and Lebombo Mountains (Ndlovu, 2013). Primary economic activities in the province include agriculture, mining and quarrying, manufacturing, trade, and tourism.
FIGURE 1. Map of South Africa showing KwaZulu Natal province as well as the occurrence data for B. globosus and B. pfeifferi.
We a conducted malacology survey in the 11 districts of KZN province for 12 months (September 2020–August 2021). Sampling sites were determined by proximity to schools (randomly selected) where parasitological surveys were carried out and where human water contact activities occurred. This was done because this study is a subset of a larger study and we needed to be able to trace infections. Snail sampling were done once in each season (that is, quarterly) at each site for 15 min by trained field staff. The trained field staffs used the combination of handpicking and the scoop nets described Appleton and Miranda (Appleton and Miranda, 2015) to collect the snails. The collected snails were identified morphologically using the key developed by Brown and Kristensen (Brown and Kristensen, 1989). Bulinus globosus and B. pfeifferi snails from each site were labelled, put in a container with water from the water body and transported to the processing site. Global positioning system was used to record the geographical coordinates of each site with the occurrence of B. globosus and B. pfeifferi. A total of 45 occurrence points of human schistosomiasis intermediate snail hosts were obtained in KwaZulu-Natal comprising of 38 occurrence points B. globosus and 17 occurrence points for B. pfeifferi (Figure 1).
Bioclimatic variables are important in determining species’ habitats. Therefore, a total of 17 bioclimatic variables were downloaded with a spatial resolution of 30 arc-second (923 m) from the AFRICLIM version 3 database (https://webfiles.york.ac.uk/KITE/AfriClim/GeoTIFF_30S/) for current and future projections of suitable habitats for B. globosus and B. pfeifferi (Table 1). These variables were downloaded because they are known to have influence on the habitat suitability of human schistosomiasis intermediate host snails (Mahmoud et al., 2022). We used the AFRICLIM ensemble model that comprised of ten general circulation models (GCMs) (GCM: CCCma-CanESM2, MPI-M-MPI-ESM-LR, CNRM-CERFACS-CNRM-CM5, ICHEC-EC-EARTH, NOAA-GFDL-GFDL-ESM2M, CSIRO-QCCCE-CSIRO-Mk3-6–0, IPSL- IPSL-CM5A-MR, MIROC-MIROC5, MOHC-HadGEM2-ES, and NCC-NorESM1-M), reduced to five regional climate models (RCM: CCCma-CanRCM4_r2, CLMcom-CCLM4-8-17_v1 (4 GCMs), DMI-HIRHAM5_v2, KNMI-RACMO22T_v1 (2 GCMs), SMHI-RCA4_v1 (10 GCMs)), the WorldClim contemporary baseline, under two representative concentration pathways of the IPCC-AR5 (RCP4.5 and RCP8.5). This ensemble model was used because it provides robust assessments of expected climate variability and reduces bias effectively (Platts et al., 2015). To predict the future distribution of habitat suitability of intermediate host snails in KwaZulu Natal, the Representative Concentration Pathways (RCPs) was used. RCPs are trajectories of four greenhouse gas emissions and concentrations adopted by the International Panel on Climate Change (IPCC). These pathways help to determine four possible future climates based on the amount of greenhouse gases emitted by 2085. RCP 2.6 is used in cases of minimum greenhouse gas emissions scenarios. RCP 4.5 and RCP 6.0 represents the intermediate scenarios of greenhouse gas emissions while RCP 8.5 represents the case of very high greenhouse gas emissions (Change, 2023). If additional efforts are not made to reduce emissions, the pathway will range between RCP 6.0 and 8.5 while RCP 2.6 represents a scenario where all efforts to reduce emission works and keeps global warming below 2°C above pre-industrial levels. Since RCP 2.6 is almost impossible to attain, this work uses RCP 4.5 and an extreme case of RCP 8.5 to predict changes in the spatial distribution of suitable habitats for schistosomiasis intermediate host snails.
TABLE 1. Bioclimatic variables included in the MaxEnt model for modeling the current and predict the future suitable habitats for B. globosus and B. pfeifferi, and their percentage contribution to model performance.
The MaxEnt version 3.3.3k (https://www.cs.princeton.edu/∼schapire/maxent) was used to create the habitat suitability maps for B. globosus and B. pfeifferi. For each species, the training data comprised of 75% of the presence data that was randomly selected while the test data comprised of the remaining 25% which was used in predicting the ability of the model (Phillips, 2007). Variable contribution analysis that gives a heuristic estimate of the relative contributions of the environmental variables to the MaxEnt model was carried out. In addition, a jackknife procedure, implemented in MaxEnt, was used to quantify each environmental variable’s explanatory power (importance) (Manyangadze et al., 2016). It reveals the predictive power of a model when trained under different scenarios (without a variable, with only a specific variable, and with all variables).
To assess the performance of the fitted MaxEnt model in appropriately discriminating suitable and unsuitable habitats for B. globosus and B. pfeifferi, the area under the curve (AUC) of the receiving operating characteristics (ROC) was used. AUC values range from 0 to 1, where a model with an AUC value of 1 indicates perfect discrimination whereas where a model with AUC values ≤ 0.5 indicate no difference in the discriminative power of the model versus a random prediction. A model with an AUC value closer to 1 has better discriminant power. A model with an AUC above 0.75 indicates sufficient discrimination and is useful (Ferson et al., 2000; Phillips and Dudík, 2008).
The habitat suitability index for B. globosus and B. pfeifferi were calculated. These values range between 0 and 1, where 0 implies that the habitat is unsuitable for the species to be found there and higher habitat suitability index values indicate higher habitat suitability for species occurrence (Du et al., 2021).
Among the 13 bioclimatic variables used in the prediction of habitats suitable for the distribution of B. globosus, the four highest variables in terms of contribution were mean temperature warmest quarter (37.6%), the number of dry months (32.6%), mean diurnal range in temperature (10.8%), and isothermality (6.7%), which quantifies the degree of day-to-night temperatures oscillation relative to the summer-to-winter (annual) oscillations. On the other hand, annual moisture index (34%), mean temperature warmest quarter (21.5%), isothermality (20.5%), and the number of dry months (7%) were the four variables that contributed most in the prediction of suitable habitats for the distribution of B. pfeifferi out of the 10 bioclimatic variables included in the model. Table 1 shows all the variables that were considered in this study and presents the percentage contribution of each bioclimatic variable in predicting the habitat suitability for B. globosus and B. pfeifferi distribution. Variables that had zero percentage contribution to the model were excluded (Table 1).
The result of the jack-knife test of variable importance is shown in Figure 2 below. The four environmental variables with the highest regularized training gain when used in isolation for modeling the habitat suitability of B. globosus are mean temperature warmest quarter (BIO10), number of dry months (DM), length of longest dry season (LLDS), and mean annual temperature (BIO1). Number of dry months (DM), mean temperature warmest quarter (BIO10), annual moisture index (MI), and Rainfall wettest quarter (BIO16) are the four environmental variables with the highest regularized training gain when used in isolation for modeling the habitat suitability of B. pfeifferi. This implies that the top four important variables above have the most information that is not present in the other variables. The environmental variable that decreases the gain the most when omitted is BIO10 for B. globosus and B. pfeifferi, which therefore appears to have the most information that is not present in the other variables. In Figure 2, the light blue bars (without variables) are not longer than the red bars (with all variables), this shows that the predictive performance of the model is better when the corresponding variables are used.
FIGURE 2. Jack-knife plots evaluating the environmental variables contribution in the MaxEnt model for B. globosus and B. pfeifferi (for full names of the variables, refer to Table 1).
The area under the curve (AUC) of the receiving operating characteristic (ROC) for B. globosus and B. pfeifferi are 0.791 and 0.896, respectively (Figure 3). The results obtained were above 0.75 indicating that the MaxEnt model had excellent predictive accuracy and satisfactorily reflects the distribution and habitat suitability for B. globosus and B. pfeifferi (Ferson et al., 2000; Phillips and Dudík, 2008).
FIGURE 3. The results of the AUC of the ROC in developing the habitat suitability model for (A) B. globosus and (B) B. pfeifferi.
The habitat suitability of B. globosus and B. pfeifferi in KwaZulu Natal under current climate conditions is presented in Table 2 and Figure 4. A total area of 14 096.01
FIGURE 4. (A) Probabilities of predicted suitable habitats and (B) habitat suitability of B. globosus, (C) Probabilities of predicted suitable habitats and (D) habitat suitability of B. pfeifferi under current climate conditions in KZN province, South Africa.
The habitat suitability predictions for B. globosus under the RCP4.5 and RCP8.5 climate scenarios in 2085 are shown in Figure 5. A significant difference was seen between the current suitable habitats and those predicted in 2085 across both RCP4.5 and RCP8.5 climate scenarios. The prediction shows a great expansion in suitable habitats for B. globosus towards the midlands (uMzinyathi, uMgungundlovu, and the entire uThukela districts). The predicted suitable habitats for the RCP8.5 climate scenario show more expansion compared to the RCP4.5 climate condition with the former projection having districts situated in the north-western and south-western regions of the province as suitable habitats. Under the RCP4.5 and RCP8.5 climate scenarios approximately 95.5% and 97.2% of the currently suitable areas will remain suitable while 32.4% and 69.3% of the currently unsuitable habitats will be suitable for B. globosus distribution in RCP4.5 and RCP8.5, respectively (Table 3).
FIGURE 5. Habitat suitability of B. globosus under future climate scenarios (A) Probabilities of predicted suitable habitats at RCP4.5 max (B) RCP4.5max (C) Probabilities of predicted suitable habitats at RCP8.5max and (D) RCP8.5max in KZN province, South Africa.
TABLE 3. Habitat suitability changes for B. globosus and B. pfeifferi under RCP4.5 AND RCP8.5 climate scenarios in 2085.
In 2085 under the RCP4.5 and RCP8.5 climate scenarios, there will be contraction, shifts, and expansion in the habitat suitability of B. pfeifferi, as regions that were formerly suitable such as Ugu, eThekwini, iLembe, and King Cetshwayo districts may no longer be suitable. On the other hand, when there is extreme greenhouse gas emission (RCP8.5), some parts of King Cetshwayo district are likely to become suitable for the distribution of B. pfeifferi. Conversely, uThukela and uMkhanyakude districts, will always be suitable habitats regardless of the amount of greenhouse gas emissions. If the greenhouse gas emissions increase moderately (RCP4.5), districts such as uMgungundlovu, eastern part of Harry Gwala and parts of uThukela districts that are currently not suitable may become suitable habitats for intermediate host snails. In addition, under the RCP4.5 and RCP8.5 climate scenarios approximately 65.1% and 59.7% of the currently suitable areas will likely become unsuitable while 11.9% and 13.8% of the currently unsuitable habitats may become suitable for B. pfeifferi distribution in RCP4.5 and RCP8.5, respectively (Table 3).
To our knowledge this study is the first to explore the impacts of climate change on the habitat suitability for human schistosomiasis intermediate host snails in all the districts of KwaZulu-Natal (KZN) province, South Africa. We used the MaxEnt to model and predict the current and future distribution of suitable habitats for B. globosus and B. pfeifferi under two RCPs (4.5 and 8.5) for the year 2085. MaxEnt model is effective at predicting distributions with small datasets (Yuan et al., 2015), thus it was used in predicting the habitat suitability of B. globosus and B. pfeifferi with 38 and 18 occurrence records, respectively. A high AUC for the ROC was obtained for predicting suitable habitats for the distribution of B. globosus (0.791) and B. pfeifferi (0.896) implying that the results obtained are reliable.
The four key variables influencing the distribution of B. globosus are mean temperature warmest quarter, number of dry months, mean diurnal range in temperature, and isothermality (that is precipitation and temperature). Number of dry months, mean temperature warmest quarter, annual moisture index, and annual wettest quarter (summarily, precipitation and temperature) were the four key bioclimatic variables identified to influence the distribution of B. pfeifferi. Malacological surveys that investigated the influence of bioclimatic variables on B. globosus and B. pfeifferi have shown similar findings (Moodley et al., 2003; Stensgaard et al., 2013). The effect of temperature on the survival of snails have been well documented (Kalinda et al., 2017). The optimal temperature where growth and reproduction is most favourable for B. globosus is between 200°C and 30°C while that of B. pfeifferi ranges from 220°C to 28°C (Adekiya, 2018). At lower temperatures, metabolism slows down, reproduction rate reduces, and the snails become less active. At high temperatures, usually above 30°C, the snails become stressed which increases their susceptibility to diseases and eventual mortality (McCreesh et al., 2014). Thus, high temperatures in an environment will reduce the distribution of snails in that region (McCreesh and Booth, 2013). Since temperature affects vegetation growth, oxygen level, water availability, this in turn affects snail’s habitat suitability (Oso and Odaibo, 2021). Seasonal variation also affects the abundance of snail in an environment (Gurarie et al., 2017). Warm and humid summers have been found to be favourable condition for both B. globosus and B. pfeifferi. These warm temperatures also favour their lifecycle from egg laying, to hatching and to the development of larvae as the snails become more active (Nandy and Aditya, 2022). Similarly, it is expected that the water levels in dams, lakes, ponds, and reservoir will reduce due to increase in average surface temperature in the coming years (Kalinda et al., 2017). This will result in decrease in the population of B. pfeifferi. However, in regions and seasons where temperature is between 20°C and 25°C, it is expected that there will be an increase in the population of B. pfeifferi. However, at high temperatures and in areas where B. pfeifferi’s population is small, there is the possibility of them dying.
The amount of water present in an environment determines the abundance of snails. When there is no water, snails cannot survive and where there is too much water, the abundance of snail population reduces. Therefore, when there is rainfall, temporary snail habitats are created as well as new habitats due to heavy rainfall that moves snails from one environment to another (Manyangadze et al., 2021). This implies when there is heavy rainfall, the population density of snails in that environment reduces due to transportation. If the speed of flowing water exceeds 0.3 m/s, snails will be washed away to a new environment (Ofulla et al., 2013). Cold and dry seasons as well as post rainy seasons have been found to be suitable habitats for B. globosus and B. pfeifferi while hot dry seasons reduces the abundance of snails due to pools and rivers drying up. Therefore, long period of rainfall or absence of rainfall as well as the intensity of rainfall will determine the abundance of snails in a region (Rubaba et al., 2016). However, it is quite difficult to accurately determine the spatial relationship between rainfall, snail population dynamics, and infection transmission due to varying rainfall effect on species as well as geographical location (Brooker, 2007). Therefore, in areas where rainfall plays important role in snail population, population of snails reduces after each rainy season as well as their transport from one location to another, thereby creating temporary habitat (Manyangadze et al., 2021).
The observation that all the eastern part of the province currently has suitable habitats for B. globosus may be attributed to the average annual temperature in that part of the province. Temperature increases south-easterly in South Africa, hence KwaZulu natal province situated in the eastern part of the country is generally warmer with temperatures of about 25°C that create suitable habitats for B. globosus (Ebhota and Tabakov, 2021). Our findings corroborate the findings from a parasitology study carried out in KwaZulu-Natal as the districts with current suitable habitats for human schistosomiasis snail hosts were found endemic for schistosomiasis (Nemungadi et al., 2022). Western parts of uMkhanyakude district and some parts of uThukela district also shows current habitat suitability of B. globosus. As observed for B. globosus, suitable habitat for B. pfeifferi can also be found in the eastern part of the province except around iLembe district and eastern part of uMkhanyakude. Compared to B. globosus, the current habitat is more suitable for the distribution of B. pfeifferi. Other parts of the province are not suitable habitats for the distribution of both B. globosus and B. pfeifferi. However, some of these districts may have suitable habitats depending on the season. It is expected that during spring and summer months temperatures are high, the districts might become suitable for both B. globosus and B. pfeifferi. These suitable areas for both species are characterised by temperate or subtropical climates with warm to hot summers and mild winters. Hence, the warm and humid climate might be the factors influencing the distribution of both species as the habitats that are currently unsuitable for the distribution of B. globosus and B. pfeifferi such as Amajuba, Sisonke, and uMgungundlovu districts are characterized by very cold temperatures during the winter and high temperatures in the summers.
In KZN province, annual average temperature and annual average rainfall have been projected to increase for the period 2021–2050 under the RCP 8.5 scenario. Increase in the number of rainfall days may result in an increase in flood and storms. This projected increase will affect the environment, socio-economy, water, and food quality (Le Roux et al., 2017).
Temperature affects the growth, reproduction and population of snails which influences the rate of parasite and host development (Kalinda et al., 2017). Climate change predictions may play an important role in determining high-risk areas as increased temperature increases the development of hosts and parasites but limiting their survival. However, there have been conflicting reports on how increase in temperature will affect snail population. McCreesh, Nikulin (McCreesh et al., 2015) suggested that increase in temperature will result in increase in suitable habitats resulting in increased disease prevalence while Pedersen, Midzi (Pedersen et al., 2014) suggested that increased temperature will result in decreased suitable habitat leading to a reduction in the transmission of the disease. There is also the possibility of snails adapting to higher temperature which will increase habitat suitability and disease prevalence (Stensgaard et al., 2019).
These contrasting views may all be valid for certain areas. For regions where temperatures were too low and hence presenting unsuitable habitats, increased temperature due to climate change may result in the habitat becoming suitable and areas currently presenting suitable habitats may be affected by increased temperature resulting in the areas becoming unsuitable. This is supported by Kalinda, Chimbari (Kalinda et al., 2017) who reported that snails can survive at about 15.5°C during cold seasons but with increased temperatures, there will be high snail proliferation resulting in increased disease prevalence.
Figure 5 shows the predicted habitat suitability of B. globosus for both RCP 4.5 and RCP 8.5 indicating that habitats around the northern part of Amajuba district are always not suitable. However, with increased temperature, the unsuitable areas may drastically reduce as observed in Figure 5D. Also, with moderate increase (RCP 4.5) in temperature, most parts of Harry Gwala district are likely to remain unsuitable for B. globosus and this may be the situation in most parts of uMgungundlovu, iLembe, and uMzinyathi districts. Under extreme increased temperatures (RCP 8.5), most of these areas will become suitable habitats for B. globosus. According to the Koppen Geiger climate classification in South Africa, these areas are currently classified as always unsuitable habitat for B. globosus fall under the temperate, cold summer with no dry season (Cui et al., 2021). With increased temperature, it is expected that the cold summer will be warmer resulting in increased snails and increased disease prevalence. Most of uThukela district and parts of Zululand, uMzinyathi, uMgungundlovu, and uMkhanyakude that are currently not suitable will become suitable in the future. With extreme global warming, more parts in the districts will become suitable habitats for B. globosus. Only a small part of King Cetshwayo that is currently suitable will become an unsuitable habitat under RCP 4.5 while small parts of Ugu districts currently suitable will become unsuitable under extreme conditions. The eastern part of the province and the western part of uMkhanyakude as well as parts of uThukela district will always present suitable habitats for B. globosus. According to the Koppen Geiger classification, this region falls under the temperate hot summer with no dry season (Cui et al., 2021). Since there is no dry season, water is always present, and temperatures are conducive for survival of snails.
Habitat suitability of B. pfeifferi for both RCP 4.5 and RCP 8.5 show that Harry Gwala, Amajuba, Zululand, uMzinyathi, parts of King Cetshwayo, uMgungundlovu, and uMkhanyakude districts are always not suitable habitat for B. pfeifferi (Figure 6). This may be attributed to the weather condition in this region. However, with increased temperature, some of these regions like parts of Ugu, uThukela, and uMkhanyakude districts that currently not suitable will become suitable with moderate increase in temperature. However, under extreme cases, only parts of uMgungundlovu district and parts of Amajuba district that are currently unsuitable will become suitable. Much of the eastern part of KwaZulu natal province currently suitable may later become unsuitable. Since the climate classification classifies this area as temperate, hot summer with no dry season, with increased temperatures, the summer months can become very hot such that the existing snails will be desiccated leading to a reduction in disease prevalence. The modelled climate change has different effects on the habitat suitability of B. globosus and B. pfeifferi. There is likely to be expansion in the habitat suitability of B. globosus while there will be contraction and shifts in the case of B. pfeifferi. This may be because of the high tolerance of B. globosus to higher temperatures compared to B. pfeifferi (Joubert et al., 1986; Manyangadze et al., 2021). Parts of uThukela and uMkhanyakude districts were shown to always have suitable habitats for the distribution of B. globosus and B. pfeifferi. Therefore, priority should be given to these districts when prevention and control strategies are implemented to interrupt the transmission of schistosomiasis.
FIGURE 6. Habitat suitability of B. pfeifferi under future climate scenarios (A) probabilities of predicted suitable habitats at RCP4.5 max (B) RCP4.5max (C) probabilities of predicted suitable habitats at RCP8.5max and (D) RCP8.5max in KZN province, South Africa.
A limitation of our study is that we only investigated the influence of climatic variables on the habitat suitability of B. globosus and B. pfeifferi. As such, some important predictor variables such as topographic (altitude and slope), biotic (competition and predators), and behavioural variables which are important in determining the habitat suitability for B. globosus and B. pfeifferi distribution might have been omitted.
Our study modelled and predicted the current and future distribution of suitable habitats for B. globosus and B. pfeifferi in KZN province, South Africa. The MaxEnt method was used in modelling the suitable habitats. Bioclimatic variables related to rainfall and temperature significantly contributed to the habitat suitability of B. globosus and B. pfeifferi. Climate change predictions regarding the future habitats of human schistosomiasis intermediate host snails in 2085 suggest that the habitats will become less favorable for B. pfeifferi and more favourable for B. globosus. This shift, expansion, and contraction will influence the transmission of schistosomiasis. We produced predictive habitat suitability maps that may guide public health measures and strategies to interrupt schistosomiasis transmission in the region through for instance the judicial allocation of limited resources (money, time, and effort), targeted control measures, environmental management, and behavioural interventions.
The raw data supporting the conclusion of this article will be made available by the authors, without undue reservation.
The University of KwaZulu Natal biomedical research ethics committee (BREC) issued the ethical approval (Ref. No: BREC/00001305/2020) for this study.
ON and TM conceptualised the study. ON collected the data, TM conducted statistical analysis, ON wrote the first draft. TM and MC read and edited the draft. All authors contributed to the article and approved the submitted version.
This research is also commissioned by the National Institute for Health Research, using Official Development Assistance (ODA) funding 16/136/33.
Many thanks to the people living in the communities where this project was carried out for giving us access to sample from the water bodies. We are also thankful to the community research assistants and colleagues at KwaZulu-Natal Ecohealth Programme (KEP) for their support during the data collection process.
The authors declare that the research was conducted in the absence of any commercial or financial relationships that could be construed as a potential conflict of interest.
All claims expressed in this article are solely those of the authors and do not necessarily represent those of their affiliated organizations, or those of the publisher, the editors and the reviewers. Any product that may be evaluated in this article, or claim that may be made by its manufacturer, is not guaranteed or endorsed by the publisher.
The views expressed in this publication are those of the authors and not necessarily those of the NHS, the National Institute for Health Research or the Department of Health. The funders had no role in the conception, study design, data collection and analysis, decision to publish or preparation of the manuscript.
Adekiya, T. A. (2018). Theoretical modelling of temperature and rainfall inuence on Schistosoma species population dynamics. China: University of Zululand.
Ahmad, G. (2022). Schistosomiasis: basic requirements for the development of a subunit vaccine, using genetic vectors. eBioMedicine, 82. doi:10.1016/j.ebiom.2022.104162
Appleton, C., and Miranda, N. (2015). Locating bilharzia transmission sites in South Africa: guidelines for public health personnel. South. Afr. J. Infect. Dis. 30 (3), 95–102. doi:10.1080/23120053.2015.1074438
Boelee, E., and Laamrani, H. (2004). Environmental control of schistosomiasis through community participation in a Moroccan oasis. Trop. Med. Int. Health 9 (9), 997–1004. doi:10.1111/j.1365-3156.2004.01301.x
Brooker, S. (2007). Spatial epidemiology of human schistosomiasis in Africa: risk models, transmission dynamics and control. Trans. R. Soc. Trop. Med. Hyg. 101 (1), 1–8. doi:10.1016/j.trstmh.2006.08.004
Brown, D., and Kristensen, T. J. D. B. L. p.n. (1989). A field guide to African freshwater snails, southern African species. Danish Bilharziasis Laboratory.
Change, I. P. o.C. (2023). Future climate changes, risks and impacts. Available at: https://ar5-syr.ipcc.ch/topic_futurechanges.php.
Cui, D., Liang, S., and Wang, D. (2021). Observed and projected changes in global climate zones based on Köppen climate classification. Wiley Interdiscip. Rev. Clim. Change 12 (3), e701. doi:10.1002/wcc.701
De Boni, L., Msimang, V., De Voux, A., and Frean, J. (2021). Trends in the prevalence of microscopically-confirmed schistosomiasis in the South African public health sector, 2011–2018. PLoS Neglected Trop. Dis. 15 (9), e0009669. doi:10.1371/journal.pntd.0009669
De Necker, L. (2020). Billharzia and its snail vectors under the spotlight in current study. Water wheel. 19 (6), 20–23.
Du, Z., He, Y., Wang, H., Wang, C., and Duan, Y. (2021). Potential geographical distribution and habitat shift of the genus Ammopiptanthus in China under current and future climate change based on the MaxEnt model. J. Arid Environ. 184, 104328. doi:10.1016/j.jaridenv.2020.104328
Duan, R.-Y., Kong, X. Q., Huang, M. Y., Fan, W. Y., and Wang, Z. G. (2014). The predictive performance and stability of six species distribution models. PloS one 9 (11), e112764. doi:10.1371/journal.pone.0112764
Ebhota, W. S., and Tabakov, P. Y. (2021). Assessment of solar PV potential and performance of a household system in Durban North, Durban, South Africa. Clean Technol. Environ. Policy 24, 1241–1259. doi:10.1007/s10098-021-02241-6
Ferson, S., Burgman, M., and Elith, J. (2000). Quantitative methods for modeling species habitat: comparative performance and an application to Australian plants. Quantitative methods conservation Biol., 39–58. doi:10.1007/0-387-22648-6_4
Frandsen, F., and Christensen, N. (1984). An introductory guide to the identification of cercariae from African freshwater snails with special reference to cercariae of trematode species of medical and veterinary importance. Acta trop. 41, 181–202.
Gunawan, G., Sulistijorini, S., Chikmawati, T., and Sobir, S. (2021). Predicting suitable areas for Baccaurea angulata in Kalimantan, Indonesia using Maxent modelling. Biodiversitas J. Biol. Divers. 22 (5). doi:10.13057/biodiv/d220523
Gurarie, D., King, C., Yoon, N., Wang, X., and Alsallaq, R. (2017). Seasonal dynamics of snail populations in coastal Kenya: model calibration and snail control. Adv. Water Resour. 108, 397–405. doi:10.1016/j.advwatres.2016.11.008
Hu, J., and Jiang, Z. (2011). Climate change hastens the conservation urgency of an endangered ungulate. PloS One 6 (8), e22873. doi:10.1371/journal.pone.0022873
Joubert, P., Pretorius, S., de Kock, K., and Van Eeden, J. (1986). Survival of Bulinus africanus (Krauss), Bulinus globosus (Morelet) and Biomphalaria pfeifferi (Krauss) at constant high temperatures. Afr. Zool. 21 (1), 85–88. doi:10.1080/02541858.1986.11447963
Kaky, E., Nolan, V., Alatawi, A., and Gilbert, F. (2020). A comparison between Ensemble and MaxEnt species distribution modelling approaches for conservation: a case study with Egyptian medicinal plants. Ecol. Inf. 60, 101150. doi:10.1016/j.ecoinf.2020.101150
Kalinda, C., Chimbari, M., and Mukaratirwa, S. (2017). Implications of changing temperatures on the growth, fecundity and survival of intermediate host snails of schistosomiasis: a systematic review. Int. J. Environ. Res. Public Health 14 (1), 80. doi:10.3390/ijerph14010080
Khumalo, G. E., Ntuli, S., Lutge, E., and Mashamba-Thompson, T. P. (2022). Geo-analysis: the distribution of community health workers in relation to the HIV prevalence in KwaZulu-Natal province, South Africa. BMC Health Serv. Res. 22 (1), 1–14. doi:10.1186/s12913-022-07707-x
Le Roux, A., Mans, G., van Huyssteen, E., and van Niekerk, W. (2017). Profiling the vulnerabilities and risks of South African settlements, 26–35.
Liu, X., Han, X., and Han, Z. (2022). Effects of climate change on the potential habitat distribution of swimming crab Portunus trituberculatus under the species distribution model. J. Oceanol. Limnol. 40, 1556–1565. doi:10.1007/s00343-021-1082-1
Magaisa, K., Taylor, M., Kjetland, E. F., and Naidoo, P. J. (2015). A review of the control of schistosomiasis in South Africa. South Afr. J. Sci. 111 (11-12), 1–6. doi:10.17159/sajs.2015/20140427
Mahmoud, M. M., Younes, A. A., El-Sherif, H. A., Gawish, F. A., Habib, M. R., and Kamel, M. (2022). Predicting the habitat suitability of Schistosoma intermediate host Bulinus truncatus, its predatory aquatic insect Odonata nymph, and the associated aquatic plant Ceratophyllum demersum using MaxEnt. Parasitol. Res. 121 (1), 205–216. doi:10.1007/s00436-021-07392-5
Manyangadze, T., Chimbari, M. J., Gebreslasie, M., Ceccato, P., and Mukaratirwa, S. (2016). Modelling the spatial and seasonal distribution of suitable habitats of schistosomiasis intermediate host snails using Maxent in Ndumo area, KwaZulu-Natal Province, South Africa. Parasites vectors 9 (1), 1–10. doi:10.1186/s13071-016-1834-5
Manyangadze, T., Chimbari, M. J., Rubaba, O., Soko, W., and Mukaratirwa, S. (2021). Spatial and seasonal distribution of Bulinus globosus and Biomphalaria pfeifferi in Ingwavuma, uMkhanyakude district, KwaZulu-Natal, South Africa: implications for schistosomiasis transmission at micro-geographical scale. Parasites vectors 14 (1), 222–229. doi:10.1186/s13071-021-04720-7
McCreesh, N., Arinaitwe, M., Arineitwe, W., Tukahebwa, E. M., and Booth, M. (2014). Effect of water temperature and population density on the population dynamics of Schistosoma mansoni intermediate host snails. Parasites Vectors 7, 503–509. doi:10.1186/s13071-014-0503-9
McCreesh, N., and Booth, M. (2013). Challenges in predicting the effects of climate change on Schistosoma mansoni and Schistosoma haematobium transmission potential. Trends Parasitol. 29 (11), 548–555. doi:10.1016/j.pt.2013.08.007
McCreesh, N., Nikulin, G., and Booth, M. (2015). Predicting the effects of climate change on Schistosoma mansoni transmission in eastern Africa. Parasites vectors 8, 4–9. doi:10.1186/s13071-014-0617-0
Moodley, I., Kleinschmidt, I., Sharp, B., Craig, M., and Appleton, C. (2003). Temperature-suitability maps for schistosomiasis in South Africa. Ann. Trop. Med. Parasitol. 97 (6), 617–627. doi:10.1179/000349803225001445
Nandy, G., and Aditya, G. (2022). Temperature dependent variations of life history traits of the land snail Allopeas gracile (Hutton, 1834)(Gastropoda: subulinidae). J. Therm. Biol. 108, 103297. doi:10.1016/j.jtherbio.2022.103297
Ndlovu, N. (2013). Analysis of the geographical patterns of malaria transmission in KwaZulu-Natal, South Africa using Bayesian spatio-temporal modelling.
Nemungadi, T. G., Furumele, T. E., Gugerty, M. K., Djirmay, A. G., Naidoo, S., and Kjetland, E. F. (2022). Establishing and integrating a female genital schistosomiasis control Programme into the existing health care system. Trop. Med. Infect. Dis. 7 (11), 382. doi:10.3390/tropicalmed7110382
Nwoko, O. E., Kalinda, C., and Chimbari, M. J. (2022a). Systematic review and meta-analysis on the infection rates of schistosome transmitting snails in Southern Africa. Trop. Med. Infect. Dis. 7 (5), 72. doi:10.3390/tropicalmed7050072
Nwoko, O. E., Manyangadze, T., and Chimbari, M. J. (2022b). Spatial distribution, abundance, and infection rates of human schistosome-transmitting snails and related physicochemical parameters in KwaZulu-Natal (KZN) province. South Africa: Heliyon.e12463
Nwoko, O. E., Manyangadze, T., and Chimbari, M. J. (2023). Spatial and seasonal distribution of human schistosomiasis intermediate host snails and their interactions with other freshwater snails in 7 districts of KwaZulu-Natal province, South Africa. Sci. Rep. 13 (1), 7845. doi:10.1038/s41598-023-34122-x
Ofulla, A. V., Adoka, S. O., Anyona, D. N., Abuom, P. O., Karanja, D., Vulule, J. M., et al. (2013). Spatial distribution and habitat characterization of schistosomiasis host snails in lake and land habitats of western K enya. Lakes Reservoirs Res. Manag. 18 (2), 197–215. doi:10.1111/lre.12032
Ogongo, P., Nyakundi, R. K., Chege, G. K., and Ochola, L. (2022). The road to elimination: current state of schistosomiasis research and progress towards the end game. Front. Immunol. 13, 1618. doi:10.3389/fimmu.2022.846108
Opisa, S., Odiere, M. R., Jura, W. G., Karanja, D. M., and Mwinzi, P. N. (2011). Malacological survey and geographical distribution of vector snails for schistosomiasis within informal settlements of Kisumu City, western Kenya. Parasites vectors 4 (1), 226–229. doi:10.1186/1756-3305-4-226
Oso, O. G., and Odaibo, A. B. (2021). Land use/land cover change, physico-chemical parameters and freshwater snails in Yewa North, Southwestern Nigeria. Plos one 16 (2), e0246566. doi:10.1371/journal.pone.0246566
Pedersen, U. B., Midzi, N., Mduluza, T., Soko, W., Stensgaard, A. S., Vennervald, B. J., et al. (2014). Modelling spatial distribution of snails transmitting parasitic worms with importance to human and animal health and analysis of distributional changes in relation to climate. Geospatial Health 8 (2), 335–343. doi:10.4081/gh.2014.23
Phillips, S. J., Transferability, sample selection bias and background data in presence-only modelling: a response to Peterson et al.(2007). Ecography. 31(2): p. 272–278. doi:10.1111/j.0906-7590.2008.5378.x
Phillips, S. J., Anderson, R. P., and Schapire, R. E. (2006). Maximum entropy modeling of species geographic distributions. Ecol. Model. 190 (3-4), 231–259. doi:10.1016/j.ecolmodel.2005.03.026
Phillips, S. J., and Dudík, M. (2008). Modeling of species distributions with Maxent: new extensions and a comprehensive evaluation. Ecography 31 (2), 161–175. doi:10.1111/j.0906-7590.2008.5203.x
Platts, P. J., Omeny, P., and Marchant, R. (2015). AFRICLIM: high-resolution climate projections for ecological applications in Africa. Afr. J. Ecol. 53, 103–108. doi:10.1111/aje.12180
Pörtner, H.-O., Roberts, D. C., Adams, H., Adler, C., Aldunce, P., Ali, E., et al. (2022). Climate change 2022: impacts, adaptation and vulnerability. USA: IPCC Sixth Assessment Report.
Rabone, M., Wiethase, J. H., Allan, F., Gouvras, A. N., Pennance, T., Hamidou, A. A., et al. (2019). Freshwater snails of biomedical importance in the Niger River Valley: evidence of temporal and spatial patterns in abundance, distribution and infection with Schistosoma spp. Parasites vectors 12, 1–20. doi:10.1186/s13071-019-3745-8
Rubaba, O., Chimbari, M., and Mukaratirwa, S. (2016). The role of snail aestivation in transmission of schistosomiasis in changing climatic conditions. Afr. J. Aquatic Sci. 41 (2), 143–150. doi:10.2989/16085914.2016.1145103
Sokolow, S. H., Wood, C. L., Jones, I. J., Swartz, S. J., Lopez, M., Hsieh, M. H., et al. (2016). Global assessment of schistosomiasis control over the past century shows targeting the snail intermediate host works best. PLoS neglected Trop. Dis. 10 (7), e0004794. doi:10.1371/journal.pntd.0004794
Stensgaard, A.-S., Booth, M., Nikulin, G., and McCreesh, N. (2016). Combining process-based and correlative models improves predictions of climate change effects on Schistosoma mansoni transmission in eastern Africa. Geospatial Health 11, 94–101. doi:10.4081/gh.2016.406
Stensgaard, A.-S., Utzinger, J., Vounatsou, P., Hürlimann, E., Schur, N., Saarnak, C. F., et al. (2013). Large-scale determinants of intestinal schistosomiasis and intermediate host snail distribution across Africa: does climate matter? Acta trop. 128 (2), 378–390. doi:10.1016/j.actatropica.2011.11.010
Stensgaard, A.-S., Vounatsou, P., Sengupta, M. E., and Utzinger, J. (2019). Schistosomes, snails and climate change: current trends and future expectations. Acta trop. 190, 257–268. doi:10.1016/j.actatropica.2018.09.013
Walz, Y., Wegmann, M., Dech, S., Vounatsou, P., Poda, J. N., N'Goran, E. K., et al. (2015). Modeling and validation of environmental suitability for schistosomiasis transmission using remote sensing. PLoS neglected Trop. Dis. 9 (11), e0004217. doi:10.1371/journal.pntd.0004217
Keywords: Schistosomiasis, B. globosus, B. pfeifferi, habitat suitability, climate change, representation concentration pathways (RCP), RCP4.5, RCP 8.5
Citation: Nwoko OE, Manyangadze T and Chimbari MJ (2023) Predicted changes in habitat suitability for human schistosomiasis intermediate host snails for modelled future climatic conditions in KwaZulu-Natal, South Africa. Front. Environ. Sci. 11:1243777. doi: 10.3389/fenvs.2023.1243777
Received: 21 June 2023; Accepted: 03 November 2023;
Published: 16 November 2023.
Edited by:
Jorge Contreras-Garduño, National Autonomous University of Mexico, MexicoReviewed by:
Ted Grantham, University of California, Berkeley, United StatesCopyright © 2023 Nwoko, Manyangadze and Chimbari. This is an open-access article distributed under the terms of the Creative Commons Attribution License (CC BY). The use, distribution or reproduction in other forums is permitted, provided the original author(s) and the copyright owner(s) are credited and that the original publication in this journal is cited, in accordance with accepted academic practice. No use, distribution or reproduction is permitted which does not comply with these terms.
*Correspondence: Onyekachi Esther Nwoko, bndva29ueWVrYWNoaUBnbWFpbC5jb20=
Disclaimer: All claims expressed in this article are solely those of the authors and do not necessarily represent those of their affiliated organizations, or those of the publisher, the editors and the reviewers. Any product that may be evaluated in this article or claim that may be made by its manufacturer is not guaranteed or endorsed by the publisher.
Research integrity at Frontiers
Learn more about the work of our research integrity team to safeguard the quality of each article we publish.