- 1Laboratory of Molecular Biology, Institute of Crop and Ecology, Hangzhou Academy of Agricultural Sciences, Hangzhou, China
- 2Hangzhou Agricultural Technology Extension Center, Institute of Husbandry, Hangzhou, China
- 3Institute of Fishers Institute, Hangzhou Academy of Agricultural Sciences, Hangzhou, China
- 4Zhejiang Everbright Agricultural Science and Technology Development Co., Ltd., Hangzhou, China
- 5Hangzhou Academy of Agricultural Sciences, Hangzhou, China
The widespread use of antibiotics in animal and human medication has raised global concerns over environmental contamination caused by antibiotic residues. This research investigated the occurrence of 12 kinds of antibiotics belonging to three groups, sulfonamides (SAs), tetracyclines (TCs), and fluoroquinolones (FQs), in chicken manure and the surrounding soil from different chicken farms in Hangzhou, South China, were detected in the samples via ultra-performance liquid chromatography-mass spectrometry (UPLC-MS/MS) and the contamination characteristics of each type of antibiotic and the correlations between each antibiotic and environmental factors were analyzed to identify the key influencing factors. An ecological risk assessment of antibiotics in the soil environment was conducted using the risk quotient (RQ) method to analyze the potential hazard level. The results indicated that: the total concentration of FQs was much higher than those of SAs and TCs in chicken manures and the soil samples. The mean concentration of FQs (39.17 μg·kg–1), TCs (34.37 μg·kg–1), and SAs (20.24 μg·kg–1) in chicken manure were significantly higher than those in the soil samples. Ciprofloxacin (CIP) was the main antibiotics detected in manure (19.42 μg·kg–1) and the soils samples (near soil: 18.42 μg·kg–1, far soil: 10.29 μg·kg–1), followed by enrofloxacin (ENR), tetracycline (TC), and ofloxacin (OFL). The redundancy analysis (RDA) showed that moisture content (MS), total organic carbon (TOC) and total nitrogen (TN) had a greater influence on the antibiotic concentration in the soil samples. Basis on the calculated risk quotient (RQs) values, the risk assessment indicated Sulfamerazine (SD) and Sulfamethoxazole (SMX) in soil posed a high risk, while trimethoprim (TMP), TC, CIP, and OFL in soils posed a medium or low risk. This research provides control and environmental monitoring data about chicken farms and scientific information that can be used to alleviate antibiotics pollution.
1 Introduction
The volume of livestock farming in China has dramatically increased since the beginning of the 21st century. In 2017, the total amount of livestock and poultry manure discharged in China was 1.64 × 109 t (FW) (Liu et al., 2020). Animal manure is an agricultural resource, that plays an important role in improving soil fertility and crop productivity (Peng et al., 2022). Antibiotics, are a class of naturally occurring, partially or fully synthesized compounds that can inhibit microorganisms, reproduce, or kill microorganisms (Wu et al., 2022). They are widely used in the prevention and treatment of infectious diseases in livestock and for promoting the animal growth (Li et al., 2015; Qiao et al., 2018; Chen et al., 2021). However, antibiotics applied to livestock are not fully absorbed by them, and up to 30%–90% of residual compounds are excreted through livestock manure or urine (Hanna et al., 2018). Owing to the limited availability of treatment processes and a lack of effective surveillance practices, the antibiotics that are present in manure are not completely eliminated (Congilosi and Aga, 2021). Animal manure may contain antibiotic residues and heavy metals residues (Muhammad et al., 2020; Sorinolu et al., 2021), when routinely applied to soil as a fertiliser, this manure can have indirect and direct toxic effects on microorganisms, animals and plants. Animal manure can also induce the emergence and spread of antibiotic-resistance genes that threatening human health (Binh et al., 2018; Ben et al., 2019; Zhang et al., 2023).
Studies have shown that tetracyclines (TCs), quinolones (QNs), and sulfonamides (SAs) antibiotics are particularly widely used (Sachi et al., 2019). Oxytetracycline (OTC) and tunicamycin (CTC) were the two most frequently recovered antibiotics in animal manure (Zhi et al., 2020). Fluoroquinolones (FQs) are widespread antimicrobial agents and have become the third most consumed antibiotic across the globe (Liu et al., 2022). They account for 17% of the global market (Van Boeckel et al., 2014) and are considered to be highly dangerous to plants, algae, and bacteria (Fang et al., 2023). Oxytetracycline (OTC) and tunicamycin (CTC) were the two antibiotics most frequently recovered from animal manure (Xu et al., 2023). As typical veterinary drugs, fluoroquinolone antibiotics (FQs) are widely used in the field of livestock and poultry breeding, however, these FQs escape to the surrounding soil through various pathways, polluting the soil through long-term accumulation (Zhan et al., 2023). SAs have a wide range of antimicrobial effects on Gram-negative and Gram-positive bacteria. TCs antibiotics are a group of broad-spectrum antibiotics with the same parent nucleus and are extremely widely used in clinical practice. In 2018, statistics from the Chinese Veterinary Yearbook of Animal Husbandry showed that TCs antibiotics accounted for the highest annual proportion of the administered veterinary antibiotics at 45.9%. Different categories of antibiotics in soil are largely influenced by their physicochemical properties. Due to their weak sorption to soil, SA residues are frequently detected in environmental media, such as surface water, groundwater, and even soil pores in livestock farms (Chen et al., 2021). It is important to note that residues of SAs residues in soil can be taken up by plants used in aquaculture and that grazing animals may be at risk. The TCs antibiotics are chemically stable and their relatively high solid-liquid sorption partition coefficient (Kd) makes them more susceptible to accumulation by sorption in soil, which means that they may be present in the soil for months to years (Jechalke et al., 2014). Consequently, livestock manure serves as an important reservoir of antibiotics in the aquaculture environment and is a major source of antibiotic emissions to the surrounding environment (Du et al., 2020). When untreated manure and urine from intensive poultry and other livestock operations are directly applied to the land, the antibiotics contained therein enter the soil and may migrate to deeper soil layers or nearby surface waters via vertical and lateral water flows, respectively (Li et al., 2023). In addition, the soil used for the long-term storage of livestock manure also accumulate heavy metals, leading to adverse effects on soil quality (Qian et al., 2018). However, data on the residues of FQs, TCs, and SAs residues on farms are limited.
In this study, five differently sized chicken farms in Hangzhou, China, were selected and three types of samples were collected. These samples were taken from fresh chicken manure, surrounding near soil, and control soils from further away (far soils) on the chicken farms. Three major antibiotic classes (TCs, SAs, and FQs) were detected in the samples via ultra-performance liquid chromatography-mass spectrometry (UPLC-MS/MS) and the contamination characteristics of each type of antibiotic and the correlations between each antibiotic and environmental factors were analyzed to identify the key influencing factors. An ecological risk assessment of antibiotics in the soil environment was conducted using the risk quotient (RQ) method to analyze the potential hazard level. This study provides a theoretical basis for preventing and controlling antibiotic contamination in livestock and poultry farming and information that can be used to improve the regulation of veterinary antibiotics on farms.
2 Materials and methods
2.1 Sample collection
Five large-scale chicken farms in Hangzhou were selected, namely, Lin’an Ailuo Poultry Co. Ltd. (S1), Fuyang Gu Xiang ecological farm (S2), Tonglu Shehong Poultry Co., Ltd. (S3), Tonglu Sishan Poultry Professional Cooperative (S4), and Fuyang Shuangjiang base (S5) (Figure 1). Three types of sample were collected. These were samples from the chicken manure, peripheral near-soil samples from the soil surrounding each chicken farm (10 m distance) and control soil samples from the soil in the area 50 m away from each chicken farm. Soil subsamples (at a depth of 0–20 cm in a 100 m2 area) were collected near the chicken farms. Eight parallel samples were taken as representative samples. The soil samples were put into valve bags, then stored in a refrigerator, and transferred to the laboratory, whereas the fresh manure samples were placed in plastic containers to be transferred to the laboratory. Before testing for antibiotics, all samples were freeze-dried at the temperature of −60°C and sieved. They were stored at −20°C until further analysis.
2.2 Chemicals and materials
In view of the widespread use of antibiotics in farms, 12 antibiotics from three classes were selected as target screening compounds in this study (TABLE. 1). These consisted of five SAs: sulfadiazine (SD), sulfamethoxazole (SMX), sulfachlorpyridazine (SCP), sulfadimidine (SM2), and trimethoprim (TMP); 4 TCs: tetracycline (TC), chlortetracycline (CTC), oxytetracycline (OTC), and doxycycline (DC); and three FQs: enrofloxacin (ENR), ciprofloxacin (CIP) and ofloxacin (OFL). The 12 kinds of antibiotics were purchased from the National Institute for the Control of Pharmaceutical Products, Beijing, China (purities >96%). Isotopically labeled internal standards were also obtained. These were tetracycline-d6 (TTCd6) and ciprofloxacin-d8 (CFX) from Witega (Berlin, Germany), and sulfadiazine-d4 (SDZ-d4) from the Laboratory of the Government Chemist (London, UK). HPLC grade methanol, for mic acid, and acetonitrile were obtained from Fisher Scientific (Waltham, MA, United States); hydrochloric acid (HCl) (guaranteed grade) was purchased from Nanjing Chemical Reagent Co., Ltd. (Nanjing, China); and disodium ethylenediamine tetraacetate (Na2EDTA) was purchased from Sinopharm Chemical Reagent Co., Ltd. (Shanghai, China). Unless special mention, the adopted chemicals belonged to analytical grade or above.
2.3 Analysis of the antibiotics
Sample pretreatment was conducted according to a preceding reported method (Hernando et al., 2006; Chen et al., 2018). Add 1 g soil sample into a 50 mL centrifuge tube, and then 15 mL of mixed extraction solution (methanol: EDTA-Mcllvaine = 2:1). The mixture was shaken for 20 min, extracted by ultrasonic extraction at low temperature for 15 min, centrifuged for 15 min (4,000 r·min–1), and collect the supernatant. The extraction was repeated three times and the supernatant was combined and diluted to 450 mL with water. The supernatant was then extracted with a solid phase extraction column activated by 6 mL of methanol and 6 mL of ultrapure water at a about 2–5 mL·min–1 at loading rate. Next, wash the column with 6 mL of ultrapure water and dry under vacuum for 30 min at negative pressure and elute with 3 mL methanol. Blow the eluate by nitrogen to nearly dry and then re-dissolved it with 1 mL of methanol. The sample was vortexed and shaken for 5 min and then the sample was fixed and passed through 0.22 μm organic filter membrane for analysis by UPLC.
The target antibiotics were analyzed via ultra-high performance liquid chromatography (UPLC, Thermo Ultimate, Waltham, MA, United States), together with a triple quadrupole mass spectrometer (MS, Thermo TSQ Quantum Access MAX, Walthman, MA, United States) equipped with an electrospray ionization (ESI) source in multiple reaction monitoring (MRM) mode. A total of 10 μL of the redissolved extracted was injected into the chromatographic system. The target antibiotics were separated by a C18 column (1.7 mm × 100 mm, 5 μm, Thermo, Waltham, MA, United States) maintained at 35°C. The flow rate for gradient elution was 0.20 mL·min–1 with phase A (Milli-Q water with 0.1% (v/v) formic acid) and Phase B (acetonitrile).
2.4 Quality control and quality assurance
Laboratory quality assurance and quality control methods (QA/QC) were implemented to ensure analytical quality, including solvent blanks, method blanks, quantification limits (MQLs), substitution use, recovery of spiked samples and duplicate analysis. Perform recovery tests with performed with chicken manure and soil (n = 3), and the recoveries for antibiotics in manure and soil ranged from 72% to 107% and 65%–116%, respectively. The relative standard deviation (RSD) percentages of the spiked measurements in manure and soil ranged from 1.3% to 13.4% and 2.4%–25.8%, respectively. Three samples were used to obtain the mean concentrations. The target compounds concentrations were calculated using the internal standard method. During the instrumental analysis, solvent blanks, procedure blanks, and known standards were injected every 15 samples to confirm the accuracy and reliability of the instruments.
2.5 Ecological risk assessment of the antibiotics
The RQ method was adopted to assess the potential risk of residues from the 12 antibiotic residues in soil to ecosystem resistance selection. The calculation of the expected no effect concentration in soil (PNECsoil) in this study, taking EU Technical Guideline Document on Risk Assessment and other relevant literature for reference.
The RQ values for the antibiotics were calculated as follows 1).
where MEC represents measured environmental concentration, μg·kg-1, and PNEC represents the predicted no-effect concentration (Nie et al., 2015) used for antibiotic resistance selection, μg·kg-1. Antibiotic toxicity data for soil are scarce and it is difficult to estimate PNECsoil values. Therefore, the PNEC values for antibiotics in soil were calculated using Equation 2 based on the PNEC values for antibiotics in water bodies:
where PNECsoil and PENCwater were used to predict the no-effect concentration of antibiotic resistance selection in soil and water environments, respectively, the PNECwater values (Table 1) were derived by collecting data from either notational or chronic toxicology experiments in conjunction with the US EPA ECTOX database, and Kdsoil was the soil-water partition coefficient, L·kg–1.
where EC50 is the acute toxicity reference factor, i.e., (Leung et al., 2012), the half maximum effect concentration (mg·L–1), and AF is the assessment factor, which is calculated using the five classification methods proposed in the EU Technical Guideline Document on Risk Evaluation. The target antibiotic data are based on only one short-term test and there are studies showing that the risk assessment AF for acute toxicity test is 1,000 and the chronic toxicity test risk assessment AF is 100. Therefore, the AF was taken to be 1,000 after comprehensive consideration (Bengtsson-Palme and Larsson, 2016). In order to maximize the estimation of the effect of antibiotics in soil, the lowest Kd value for various soil types in previous studies was selected as PNECsoil for each antibiotic soil in this study. The risk levels were classified into low risk (0.01 ≤ RQ < 0.1), medium risk (0.1 ≤ RQ < 1), and high risk (RQ > 1) (Hernando et al., 2006).
2.6 Soil properties
The pH, total phosphorus, total nitrogen, and organic matter in chicken manure were determined using the standard “Organic Fertilizer” (NY525-2012) (Ministry of Agriculture of PRC, 2012). The pH, total nitrogen, total phosphorus and organic matter in soil also determined according to “Soil Agrochemical Analysis” (Bao, 2000). Total nitrogen and phosphorus were determined by an automatic continuous flow analyzer (AA3), and organic matter was determined by the potassium dichromate capacitance method. The soil properties are shown in Table 2.
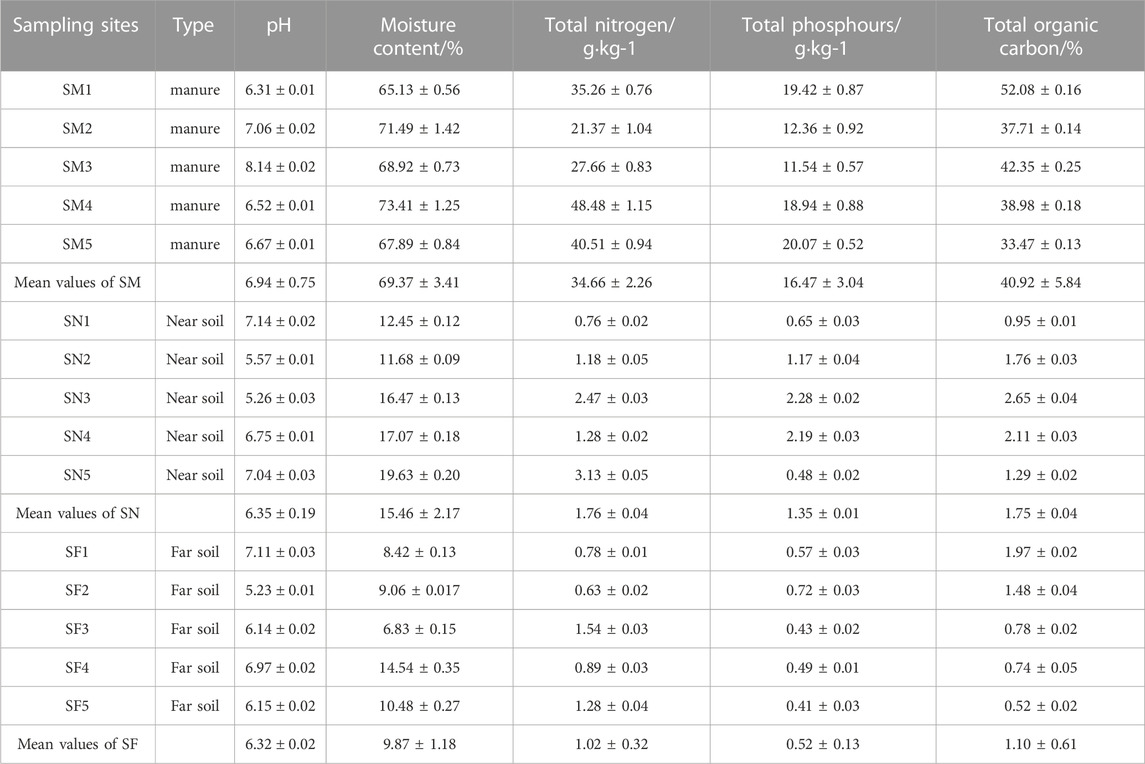
TABLE 2. Total detection levels of three classes of antibiotics in chicken farms and surrounding soil.
2.7 Statistical analysis
IBM PASW Statistics 18.0 (Chicago, I11, United States) was adopted to analyze all the statistics. Antibiotic concentrations were compared using the Kruskal-Wallis nonparametric test and statistically significant differences were considered to be significant at p < 0.05. A redundancy analysis (RDA) was performed to evaluate the relationship between physicochemical properties and antibiotic concentrations in the physicochemical environment of the soil in and around the chicken farms.
3 Results
3.1 Occurrence of antibiotics in chicken farms and in the surrounding soil
The detailed concentrations and detection frequencies of the 12 antibiotics on the five typical chicken farms are shown in Table 3. The detection frequencies of the 12 antibiotics were up to 100% in manure, indicating that antibiotics were widely present in the manure samples. Ciprofloxacin recorded the highest concentration of 19.23 μg·kg–1 (dry weight, dw), followed by ENR and OFL with average concentrations of 14.62 and 5.33 μg·kg–1, respectively. The 12 antibiotics were present at lower concentrations and detection frequencies in the surface soil than in the chicken manures, among which the detection frequencies of nine antibiotics (SD, SMX, CIP, TC, DC, TMP, ENR, and OFL) reached 100% in near soil, indicating that these antibiotics were widely present in the near soil where CIP (18.42 μg·kg–1) and ENR (12.38 μg·kg–1) were the most prevalent antibiotics. In terms of the far soil, the concentrations of target antibiotics and the detection frequencies showed seven antibiotics (SD, SMX, TC, OTC, CIP, ENR, and OFL) reached 100%, with mass fractions of 0.81, 0.39, 3.56, 1.06, 10.29, 7.28, and 2.67 μg·kg–1, respectively. Noticeably, CIP and ENR had much higher mass fractions than the other antibiotics in manure and the soil samples. Among the five SAs, SD had a higher mass fraction than the other four SAs in manure and the soil samples. The TC mass fraction was the highest detected in chicken manure and soil. Overall, the mass faction of the three antibiotic classes followed the order: FQs > TCs > SAs. Furthermore, the highest antibiotic concentrations were found in chicken manure, followed by near soil and far soil.
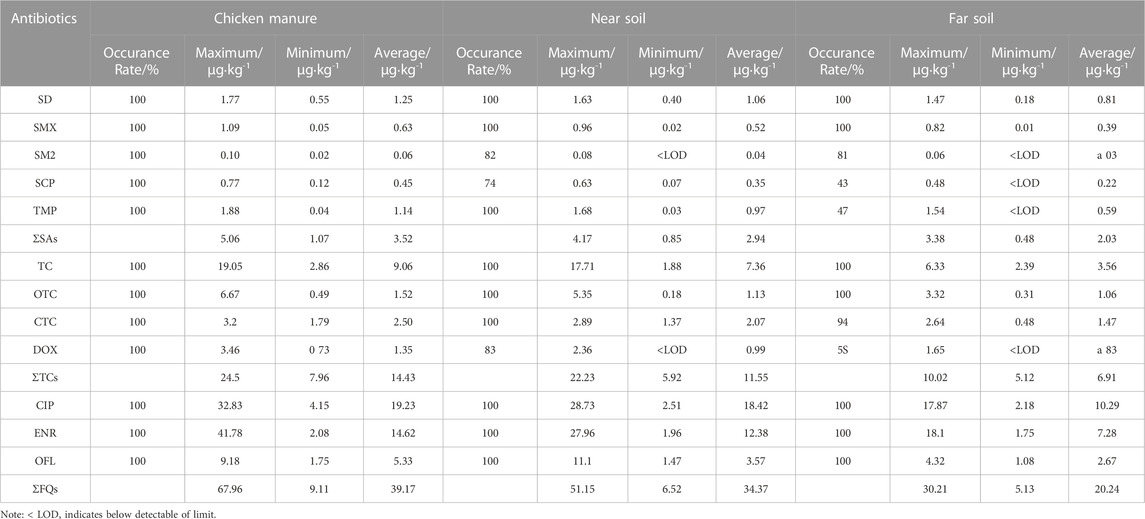
TABLE 3. Total detection levels of three classes of antibiotics in chicken farms and surrounding soil.
3.2 Properties distribution of antibiotics across the chicken farms and in the surrounding soil
Figure 2 shows that FQs were the largest contributors of the antibiotics in chicken manure and soil. Among the FQs, CIP was the major component in chicken manure and the soil from the five different chicken farms, where it accounted for 33.65%, 37.70%, and 35.26% of ∑FQs concentrations in chicken manure, near soil, and far soil, respectively, followed by ENR, TC, and OFL. The SM2 fraction was the lowest in the manure and soil samples. Overall, the distribution characteristics of the 12 antibiotics in chicken manure and soil were consistent, which suggests that the pollution sources affecting the chicken farms and surrounding soil are similar.
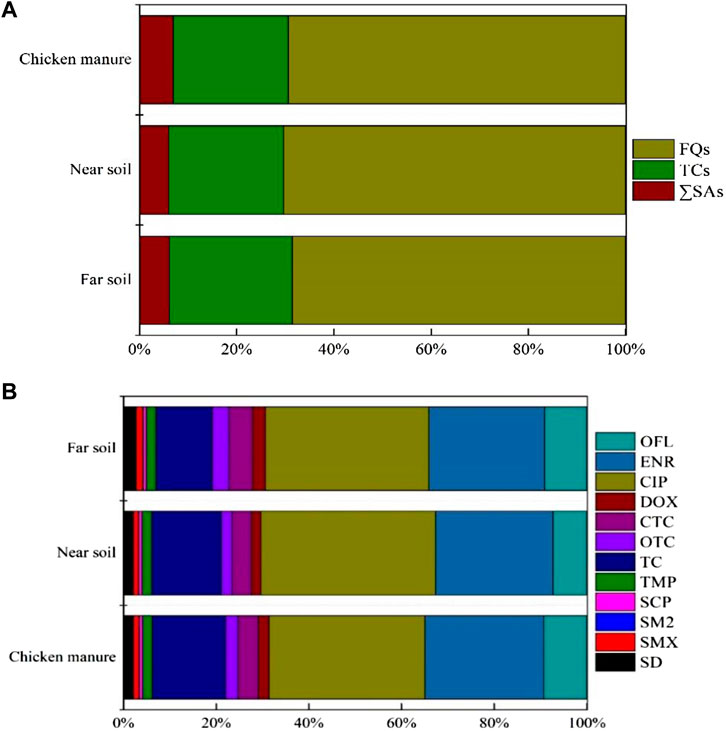
FIGURE 2. Composition of antibiotics in the Chicken manure and surrounding soil in 5 different chicken farms of Hangzhou, China. (A) Proportion of concentration of 3 classes antibiotics. (B) Proportion of concentration of individual antibiotic.
According to Figure 3 and the Kruskal-Wallis nonparametric test, significant differences were observed for the total concentration of antibiotics and ∑FQs, TC, CIP, ENR, and OFL, on the five different chicken farms in Hangzhou (p < 0.05). The total concentrations of antibiotics, and TC and OFL in chicken manure were significantly higher than that in the soil samples (p < 0.05), and ∑FQs, CIP, and ENR in chicken manure and near soil were significantly higher than those in far soil samples (p < 0.05). The results showed a tendency for antibiotic contamination to migrate to the surrounding soil.
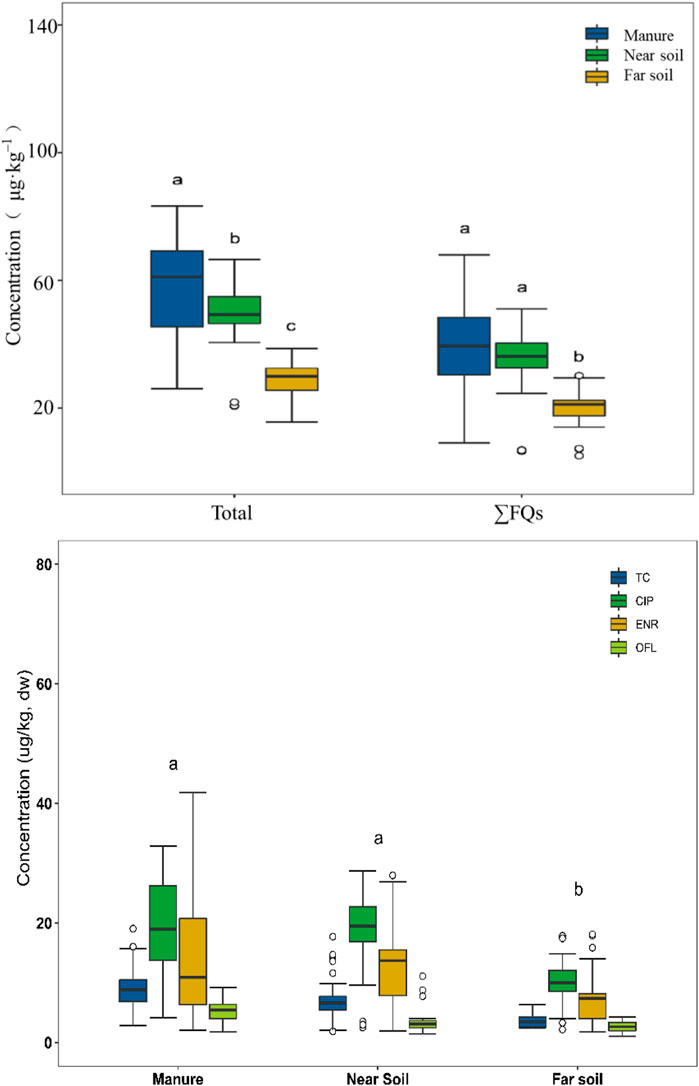
FIGURE 3. Box and whisker plots of antibiotics in the urban soil in different function areas of Hangzhou. Note: The horizontal black line in the box represented the median value and the low and upper edges of the box mark the 25th and 75th percentiles, respectively. The whiskers extending from the box show the highest and lowest values. “Singular values are represented by “circles,” which were beyond the 150th percentile of the difference between 25th and 75th percentiles.
3.3 Relationship between the soil characteristics and antibiotics concentrations
An RDA was used to evaluate the influence of environmental factors (pH, water content (MS), total nitrogen (TN), total phosphorus (TP), total organic carbon (TOC) on antibiotic concentrations in soils around the chicken farms. Figure 4 shows that in the I and II axes explained 53.44% and 6.59% of the total variance of the data for the 12 antibiotic concentrations in the soil samples, respectively, or 60.03% in total. The cumulative explanation of the relationship between antibiotic concentration and soil physicochemical factors amounted to 64.6%.
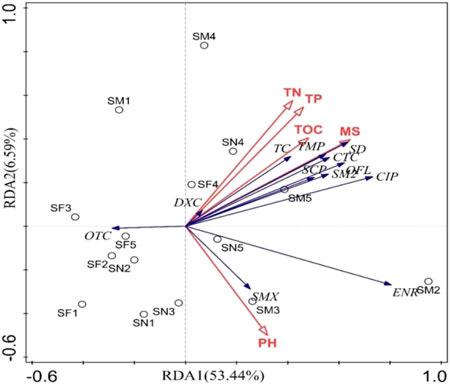
FIGURE 4. Redundant analysis of three major classes of antibiotics and environmental factors. Note: Blue rays represent antibiotics, red rays represent environmental factors; SM represents chicken manure samples, SN and SF represent near and far soil samples, respectively.
Therefore, axes I and II reflect the relationship between antibiotic concentration and environmental factors to some extent (Figure 4). The length of the rays in the RDA plot represents the magnitude of the model contribution: the longer the ray, the greater the contribution. The angle of the ray represents its correlation and the cosine of the angle is equal to the correlation coefficient between the two. The longer the vertical projection of the environmental factor ray on the antibiotic concentration ray, the greater the effect on the antibiotic concentration. Finally, the longer the vertical projection of the environmental factor rays on the antibiotic concentration rays, the greater the effect on the antibiotic concentration. The ray distribution of the 12 antibiotics in the soil samples around the chicken farm is shown in Figure 4. The correlation between TCs, SAs, and FQs antibiotics is not obvious, and the distribution of the antibiotics except for TCs is relatively concentrated. The rays for the environmental factors (TN, TP, MS, TOC, and pH) were longer and explained 14.00%, 2.4%, 24.5%, 14.00%, and 4.8% of the variability in antibiotic concentrations, respectively. Among them, TP had a strong positive correlation with DXC, MS had a strong positive correlation with SD, TMP and TN had a positive correlation with DXC, pH had a positive correlations with SMX and TOC had a positive correlation with TC. In conclusion, there was no significant pattern between the different antibiotics and most of the single environmental factors.
3.4 Ecological risk assessment of antibiotics
Combined with Figure 5, the RQ values for SMX, SD, and ENR in the near soil were much higher than 1 and all of them represent a high risk, among which the RQ values for SMX and SD reached 10.85 and 7.17, respectively, and both of them pose the highest ecological risk. The ecological risks due to SMX and SD in the far soil were the highest, the RQ values were much higher than 1, and both represent a high risk. Therefore, SMX and SD were the most important antibiotics in the chicken farm soil. The RQ values for SM2, SCP, TMP, TC, CIP, and OFL in the near soil were in the range 0.01–1 and represent a low to medium risk. The RQ values for OTC, CTC, and DXC were all less than 0.01 and represent no risk at the moment. The RQ values for SM2, TMP, TC, CIP, ENR, and OFL in the far soils were in the range 0.01–1. They represent a medium-low risk and should be taken seriously as they are harmful to soil microorganisms to some extent. The RQ values for SCP, OTC, CTC, and DXC were all less than 0.01, which means that they pose no risk at the moment.
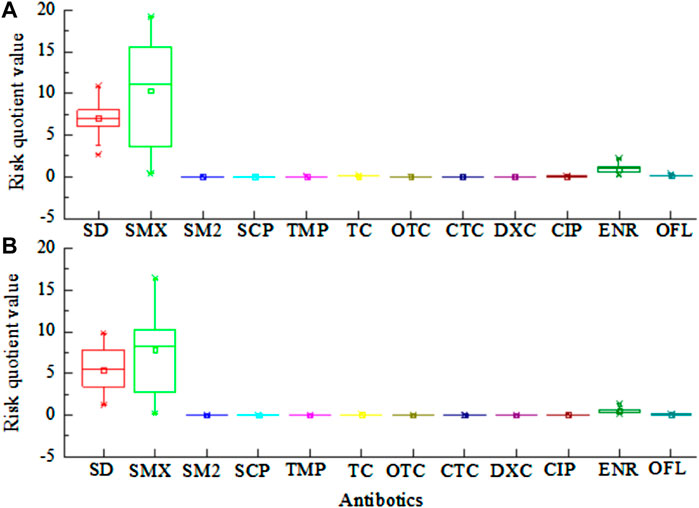
FIGURE 5. Risk quotient of predominant antibiotics in the soils near the chicken farm (A) and in the remote control soil (B).
The difference in the total contents of the three major antibiotics between the near and far (control) soils around the chicken farms was calculated to exclude the effect of exogenous antibiotics and to assess the effect of antibiotics on the surrounding near soils due to the chicken farms. Table 4 shows that the total RQ values for ∑SAs, ∑TCs, and ∑FQs in the near soil from the different chicken farms were greater than those of the control far soil, indicating that the control far soil on the chicken farms was not contaminated by external sources of SAs. Table 4 shows that the RQ values for the near soil around the chicken farms are higher than those of the control far soil, which may be related to the fact that the near soil around the farms can be directly exposed to chicken manure. The antibiotics contained in chicken manure can be directly transported and transformed into the peripheral near soil.
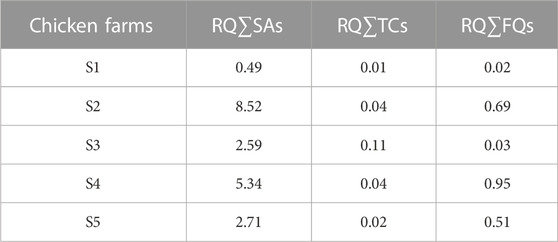
TABLE 4. Ecological risk difference of 3 major antibiotics in the near-soils and control remote soils around the chicken farm.
4 Discussion
4.1 Antibiotic contamination characteristics in manure and soil
The number and detection rates for the 12 antibiotics in manure were much greater than that in soil (TABLE 2). Their mean concentrations in manure and soil were in descending order: FQs > TCs > SAs respectively. Overall, the antibiotics with high concentrations in manure were also present at relatively high levels in the soil, which means their pollution sources are relatively similar.
In comparison with the other antibiotics, the higher Kd value for FQs indicates lower mobility and a tendency to accumulate in the soil. The SAs are widely used in livestock and aquaculture, and although they are used in small amounts, their detection concentrations in water are generally large due to their highwater solubility and their poor adsorption to soil and sediment (Ostermann et al., 2014; Wegst-Uhrich et al., 2014). The TCs and FQs are widely used as additives in livestock farming to prevent animal disease and promote animal growth, while SAs are relatively less used in intensive livestock farming (Chen et al., 2021). This may be the cause of the low concentration of SAs in this study. Among them, TCs have a strong affinity for soil particles and can be stably adsorbed to them. The concentration of TCs in this study was much lower than that of a previous study, indicating that TCs were relatively less applied on chicken farms in this study area. In terms of individual antibiotics, CIP was the major component in the chicken manures and soils from the five different chicken farms and accounted for more than 30% of the ∑FQs concentrations. Arun et al. indicated that CIP contributed to 55%–75% of the total FQs in dumpsite soil from Chennai City, China (Arun et al., 2020), which was consistent with our results. The higher CIP concentrations might be due to its longer half-life (2,310 ± 1,155 days) (Walters et al., 2010) in soil and lower log octanol-water partition (log Kow) coefficient (0.28) (Arun et al., 2020). The CIP molecule contains one-C=O, one-COOH, and one-N(CH3)2 and one-CONH2, which are all strongly adsorbed to soil. The prevalence of ENR, TC, and OFL could be due to their high rates of production and consumption rates in the Hangzhou area. Zhao et al. (2010) found that the concentrations of all SAs concentrations were measurable and the maximum SD concentration in chicken manure was 4.11 mg·kg–1, which was much higher than in this study (1.77 mg·kg–1) (Tu et al., 2020). In conclusion, the concentration and frequency of detection of the different antibiotics are mainly related to their use patterns, characteristics, and environmental behavior.
4.2 Effect of soil characteristics on the distribution of antibiotics in soil
Soil factors, such as heavy metal content, pH, and TOC, in addition to the specific characteristics of the antibiotics, have also been found to influence the adsorption of antibiotics in solid environmental matrices (Vasudevan et al., 2009). The soil pH is recognized as an important influential factor for that antibiotics adsorption onto soil, and can determine the form of veterinary antibiotics present (Hu et al., 2019) The TCs are a class of acid-base amphoteric compounds that are susceptible to denaturation reactions under both acidic and alkaline conditions. They are relatively stable under acidic conditions, but their degradation is promoted under alkaline conditions (Ben et al., 2019). In our study, the pH ranged from 5.23 to 7.14, which indicated a weak acid soil (TABLE 2). The chemical form of TCs in the soil at the sampling sites used in this study was relatively stable and not greatly influenced by other environmental factors. The RDA analysis showed that the SMX concentration in the soil was positively correlated with pH, but the remaining antibiotics were only weakly correlated with pH. SD and sulfachloropyridazine (SCP) adsorption in Galician soils was significantly and positively correlated with soil organic matter content, clay ratio and cation exchange capacity (CEC) (Conde-Cid et al., 2019). Gao et al. (2012) showed that NOR concentration and TOC were significantly and positively correlated in the surface soil samples from Shanghai (Gao et al., 2012). Our results showed that TC concentration was positively correlated with TOC, which indicated that there was a correlation between antibiotic concentration and soil organic carbon content. NOR, OFL, and CIP concentrations in top soils from Beijing were not significantly correlated with TOC (Gao et al., 2012), which was consistent with our findings. The reason for the inadequate relation to TOC may result from the easy adsorption of FQs onto metal oxides and soil minerals (Liu et al., 2022). However, this study suggests that there is a relationship between TOC and FQs in the soil. In addition, antibiotics undergo a series of degradation reactions after entering the soil, including photolysis, hydrolysis and biodegradation, which are important transformation processes. Usually, temperature, moisture, soil type and other factors affect the degradation of antibiotics in soil (Jiang, 2016). Studies have shown that the degradation of SAs in soil is mainly due to hydrolysis and biodegradation, and that the main degradation modes for TCs are photolysis and microbial degradation. Therefore, the concentrations of different antibiotics in the soil are influenced by a combination of soil microorganisms and environmental conditions such as light, water content, and soil properties.
4.3 Risk assessment of antibiotics in soil
This research showed that, the RQ values for SMX and SD in the surrounding near and far soils were much greater than 1 (Figure 5), with meant that they posed a high ecological risk. Studies have found that the excretion of SAs in animal manure was up to 90% and that this resulted in high environment drug exposure levels (Qian et al., 2022). Therefore, the serious ecological risks posed by SD and SMX may be related to the direct migration and transformation of antibiotics contained in chicken manure into the surrounding near soil. In this study, the RQ values of the far-soil and near-soil ENR were larger than 1, which is consistent with the results of the study by Gu et al. (2020). ENR is a chemically synthesised bacteriostatic agent belonging to the FQ class, which includes the four most-used antibiotics in China and are frequently detected in the water and environment (Zhang et al., 2023). Owing to their long half-life and strong adsorption capacities, they are retained in animal manure for a long periods, leading to a higher probability of translocation to the surrounding soil. In addition, FQs are amphiphilic compounds with strong ligands fluorine atoms, carboxyl groups, amino groups, and ion-exchanged carboxyl groups, which can interact with the soil through different mechanisms, and have a strong adsorption capacity in the soil (Chen et al., 2018). Overall, the RQ values of the proximal soils around chicken farms were higher than those of the farther and control soils, which could be related to the antibiotics contained in chicken manure being directly transported and transformed into the surrounding proximal soil (Wei et al., 2018).
5 Conclusion
The three major antibiotics are commonly detected in chicken manures and the soil, and the concentrations of these antibiotics were in descending order: chicken manure samples > near-soil samples > far-soil samples in the different sampling points, and the total concentrations of TCs, SAs, and FQs tended to decay sequentially, suggesting that there is a possibility of migration to the soil. The RDA showed that MS, TOC and TN had a greater influence on the antibiotic concentration in the soil samples. The ecological risk of near soil samples around chicken farms is higher than that of far soil samples. TC, CIP and OFL in soils posed a medium or low risk. The RQ difference of remote soil showed that each chicken farm had polluted the surrounding soil to different degrees. Basis on the calculated RQ values, the results of the risk assessment revealed that SD and SMX in soils pose a high risk, whereas TMP, TC, CIP, and OFL pose a medium or low risk. Additional studies are need to investigate the human exposure risk to antibiotics from organic vegetables grown near chicken farms.
Data availability statement
The raw data supporting the conclusion of this article will be made available by the authors, without undue reservation.
Author contributions
Conceptualization YZ, methodology, YZ and QL, validation, YC and HW; formal analysis, YG; YW, and HL investigation, YZ; resources, TZ. data curation, JF, writing–original draft preparation YZ, writing–review and editing, YZ; supervision, CZ, project administration QL and TZ, funding acquisition, QL and TZ. All authors contributed to the article and approved the submitted version.
Funding
This study was financially supported by the science and technology development project of Hangzhou (202003A02 and 202203A10) and Zhejiang Provincial key research and development project (2019C02035).
Conflict of interest
Author CZ was employed by Zhejiang Everbright Agricultural Science and Technology Development Co., Ltd.
The remaining authors declare that the research was conducted in the absence of any commercial or financial relationships that could be construed as a potential conflict of interest.
Publisher’s note
All claims expressed in this article are solely those of the authors and do not necessarily represent those of their affiliated organizations, or those of the publisher, the editors and the reviewers. Any product that may be evaluated in this article, or claim that may be made by its manufacturer, is not guaranteed or endorsed by the publisher.
References
Arun, S., Kumar, R. M., Ruppa, J., Mukhopadhyay, M., Ilango, K., and Chakraborty, P. (2020). Occurrence, sources and risk assessment of fluoroquinolones in dumpsite soil and sewage sludge from Chennai, India. Environ. Toxicol. Pharmacol. 79, 103410. doi:10.1016/j.etap.2020.103410
Ben, Y., Fu, C., Hu, M., Liu, L., Wong, M. H., and Zheng, C. (2019). Human health risk assessment of antibiotic resistance associated with antibiotic residues in the environment: A review. Environ. Res. 169, 483–493. doi:10.1016/j.envres.2018.11.040
Bengtsson-Palme, J., and Larsson, D. G. (2016). Concentrations of antibiotics predicted to select for resistant bacteria: proposed limits for environmental regulation. Environ. Int. 86, 140–149. doi:10.1016/j.envint.2015.10.015
Binh, V. N., Dang, N., Anh, N. T. K., Ky, L. X., and Thai, P. K. (2018). Antibiotics in the aquatic environment of Vietnam: sources, concentrations, risk and control strategy. Chemosphere 197, 438–450. doi:10.1016/j.chemosphere.2018.01.061
Chen, H. Y., Jing, L. J., Teng, Y. G., and Wang, J. S. (2018). Characterization of antibiotics in a large-scale river system of China: occurrence pattern, spatiotemporal distribution and environmental risks. Sci. Total Environ. 618, 409–418. doi:10.1016/j.scitotenv.2017.11.054
Chen, H., Zheng, W., Shen, X., Zhang, F., Zhou, X., Shen, J., et al. (2021). Occurrence, Distribution, and Ecological Risk Assessment of Antibiotics in Different Environmental Media in Anqing, Anhui Province, China. Int. J. Environ. Res. Public Health 18 (15), 8112. doi:10.3390/ijerph18158112
Conde-Cid, M., Novoa-Munoz, J. C., Nunez-Delgado, A., Fernandez-Sanjurjo, A., Arias-Estevez, M. J., and Alvarez-Rodriguez, E. (2019). Experimental data and modeling for sulfachloropyridazine and sulfamethazine adsorption/desorption on agricultural acid soils. Microsporous Mesoporous Mater. 228, 109601. doi:10.1016/j.micromeso.2019.109601
Congilosi, J. L., and Aga, D. S. (2021). Review on the fate of antimicrobials, antimicrobial resistance genes, and other micropollutants in manure during enhanced anaerobic digestion and composting. J. Hazard Mater 405 (5), 123634. doi:10.1016/j.jhazmat.2020.123634
Du, S., Shen, J. P., Hu, H. W., Wang, J. T., Han, L. L., Sheng, R., et al. (2020). Large-scale patterns of soil antibiotic resistome in Chinese croplands. Sci. Total Environ. 712, 136418. doi:10.1016/j.scitotenv.2019.136418
Fang, L. F., Chen, C. Y., Li, S. Y., Ye, P. P., Shi, Y. J., Sharma, G., et al. (2023). A comprehensive and global evaluation of residual antibiotics in agricultural soils: accumulation, potential ecological risks, and attenuation strategies. Ecotox Environ Saf. 262, 115175. doi:10.1016/j.ecoenv.2023.115175
Gao, L., Shi, Y., Li, W., Niu, H., Liu, J., and Cai, Y. (2012). Occurrence of antibiotics in eight sewage treatment plants in Beijing, China. Chemosphere 86 (6), 665–671. doi:10.1016/j.chemosphere.2011.11.019
Hanna, N., Sun, P., Sun, Q., Li, X., Yang, X., Ji, X., et al. (2018). Presence of antibiotic residues in various environmental compartments of Shandong province in eastern China: its potential for resistance development and ecological and human risk. Environ. Int. 114, 131–142. doi:10.1016/j.envint.2018.02.003
Hernando, M. D., Mezcua, M., Fernández-Alba, A. R., and Barceló, D. (2006). Environmental risk assessment of pharmaceutical residues in wastewater effluents, surface waters and sediments. Talanta 69 (2), 334–342. doi:10.1016/j.talanta.2005.09.037
Hu, S., Zhang, Y., Shen, G., Zhang, H. C., Yuan, Z. J., and Zhang, W. (2019). Adsorption/desorption behavior and mechanisms of sulfadiazine and sulfamethoxazole in agricultural soil systems. Soil Tillage Res. 186, 233–241. doi:10.1016/j.still.2018.10.026
Jechalke, S., Heuer, H., Siemens, J., Amelung, W., and Smalla, K. (2014). Fate and effects of veterinary antibiotics in soil. Trends Microbiol. 22, 536–545. doi:10.1016/j.tim.2014.05.005
Jiang, Y. (2016). Adsorption behavior of typical organic pollutants in loess. Beijing: China water resources and hydropower press.
Leung, H. W., Minh, T. B., Murphy, M. B., Lam, J. C., So, M. K., Martin, M., et al. (2012). Distribution, fate and risk assessment of antibiotics in sewage treatment plants in Hong Kong, South China. Environ. Int. 42, 1–9. doi:10.1016/j.envint.2011.03.004
Li, C., Chen, J., Wang, J., Ma, Z., Han, P., Luan, Y., et al. (2015). Occurrence of antibiotics in soils and manures from greenhouse vegetable production bases of Beijing, China and an associated risk assessment. Sci. Total Environ. 521-522, 101–107. doi:10.1016/j.scitotenv.2015.03.070
Li, M., Yang, L., Yen, H., Zhao, F., Wang, X., Zhou, T., et al. (2023). Occurrence, spatial distribution and ecological risks of antibiotics in soil in urban agglomeration. J. Environ. Sci. 125, 678–690. doi:10.1016/j.jes.2022.03.029
Liu, C., Chen, Y., Li, X., Zhang, Y., Ye, J., Huang, H., et al. (2020). Temporal effects of repeated application of biogas slurry on soil antibiotic resistance genes and their potential bacterial hosts. Environ. Pollut. 258, 113652. doi:10.1016/j.envpol.2019.113652
Liu, X. Y., Zhang, J. Q., Gbadegesin, L. A., and He, Y. (2022). Modelling approaches for linking the residual concentrations of antibiotics in soil with antibiotic properties and land-use types in the largest urban agglomerations in China: A review. Sci. Total Environ. 838, 156141. doi:10.1016/j.scitotenv.2022.156141
Ministry of Agriculture of PRC (2012). Agricultural industry standard of the People’s Republic of China: Organic fertilizer NY 525-2012. Beijing: China Agricultural Press.
Muhammad, J., Khan, S., Lei, M., Khan, M. A., Nawab, J., Rashid, A., et al. (2020). Application of poultry manure in agriculture fields leads to food plant contamination with potentially toxic elements and causes health risk. Environ. Technol. Inno 19 (1), 100909. doi:10.1016/j.eti.2020.100909
Nie, M., Yan, C., Dong, W., Liu, M., Zhou, J., and Yang, Y. (2015). Occurrence, distribution and risk assessment of estrogens in surface water, suspended particulate matter, and sediments of the Yangtze Estuary. Chemosphere 127, 109–116. doi:10.1016/j.chemosphere.2015.01.021
Ostermann, A., Gao, J., Welp, G., Siemens, J., Roelcke, M., Heimann, L., et al. (2014). Identification of soil contamination hotspots with veterinary antibiotics using heavy metal concentrations and leaching data--a field study in China. Environ. Monit. Assess. 186 (11), 7693–7707. doi:10.1007/s10661-014-3960-x
Peng, S., Zhang, H. Y., Song, D., Chen, H., Lin, X. G., Wang, Y. M., et al. (2022). Distribution of antibiotic, heavy metals and antibiotic resistance genes in livestock and poultry feces from different scale of farms in Ningxia, China. J. Hazard. Mater. 440, 129719. doi:10.1016/j.jhazmat.2022.129719
Qian, X. Y., Wang, Z. Q., Shen, G. X., Chen, X. H., Tang, Z. Z., Guo, C. X., et al. (2018). Heavy metals accumulation in soil after 4 years of continuous land application of swine manure: A field-scale monitoring and modeling estimation. Chemosphere 210, 1029–1034. doi:10.1016/j.chemosphere.2018.07.107
Qian, X. Y., Wang, Z. Q., Zhang, H. C., Gu, H. R., and Shen, G. X. (2022). Occurrence of veterinary antibiotics in animal manure, compost, and agricultural soil, originating from different feedlots in suburbs of Shanghai, East China. Environ. Monit. Assess. 194 (5), 379. doi:10.1007/s10661-022-10010-1
Qiao, M., Ying, G. G., Singer, A. C., and Zhu, Y. G. (2018). Review of antibiotic resistance in China and its environment. Environ. Int. 110 (1), 160–172. doi:10.1016/j.envint.2017.10.016
Sachi, S., Ferdous, J., Sikder, M. H., and Azizul Karim Hussani, S. M. (2019). Antibiotic residues in milk: Past, present, and future. J. Adv. Vet. Anim. Res. 6 (3), 315–332. doi:10.5455/javar.2019.f350
Sorinolu, A.J.L., Neha, T., Arun, K., and Mariya, M. (2021). Antibiotic resistance development and human health risks during wastewater reuse and biosolids application in agriculture. Chemosphere 265. doi:10.1016/J.CHEMOSPHERE.2020.129032
Tu, Q., Xu, Y., Li, E. H., Shi, R. G., Zheng, X. Q., and Geng, Y. G. (2020). Occurrence and risk assessment of antibiotics in typical Chicken farms and surrounding soils. J. Agro-Environment Sci. 39 (1), 97–107. (in Chinese). doi:10.11654/jaes.2019-0823
Van Boeckel, T. P., Gandra, S., Ashok, A., Caudron, Q., Grenfell, B. T., Levin, S. A., et al. (2014). Global antibiotic consumption 2000 to 2010: an analysis of national pharmaceutical sales data. Lancet Infect. Dis. 14 (8), 742–750. doi:10.1016/S1473-3099(14)70780-7
Vasudevan, D., Bruland, G. L., Torrance, B. S., Upchurch, V. G., and Mackay, A. A. (2009). pH-dependent ciprofloxacin sorption to soils: interaction mechanisms and soil factors influencing sorption. Geoderma 151 (3-4), 68–76. doi:10.1016/j.geoderma.2009.03.007
Walters, E., Mcclellan, K., and Halden, R. U. (2010). Occurrence and loss over three years of 72 pharmaceuticals and personal care products from biosolids-soil mixtures in outdoor mesocosms.Water Res. 44 (20), 6011–6020. doi:10.1016/j.watres.2010.07.051
Wegst-Uhrich, S. R., Navarro, D. A, Zimmerman, L., and Aga, D. S (2014). Assessing antibiotic sorption in soil: a literature review and new case studies on sulfonamides and macrolides. Chem Cent J. 8 (1. doi:10.1186/1752-153X-8-5
Wei, R., He, T., Zhang, S., Zhu, L., Shang, B., Li, Z., et al. (2018). Occurrence of seventeen veterinary antibiotics and resistant bacterias in manure-fertilized vegetable farm soil in four provinces of China. Chemosphere 215, 234–240. doi:10.1016/j.chemosphere.2018.09.152
Wu, Q. D., Zou, D. S., Zheng, X. C., Liu, F., Li, L. C., and Xiao, Z. H. (2022). Effects of antibiotics on anaerobic digestion of sewage sludge: performance of anaerobic digestion and structure of the microbial community. Sci. Total Environ. 845, 157384. doi:10.1016/j.scitotenv.2022.157384
Xu, S. L., Wang, W. Z., Deng, J. B., and Xu, W. H. (2023). The residue of tetracycline antibiotics in soil and Brassica juncea var. gemmifera, and the diversity of soil bacterial community under different livestock manure treatments. Environ. Geochem Health 45 (1), 7–17. doi:10.1007/s10653-022-01213-z
Zhan, L., Xia, Z. W., and Xu, Z. M. (2023). Thermal desorption behavior of fluoroquinolones in contaminated soil of livestock and poultry breeding. Environ. Res. 211, 113101. doi:10.1016/j.envres.2022.113101
Zhao, L., Dong, Y. H, and Wang, H. (2010). Residues of veterinary antibiotics in manures from feedlot livestock in eight provinces of China. Sci Total Environ. 408, 1069–1075doi:10.1016/j.scitotenv.2009.11.014
Zhang, Y., Cheng, D. M., Xie, J., Zhang, Y. T., Wan, Y., Zhang, Y. Q., et al. (2023). Impacts of farmland application of antibiotic-contaminated manures on the occurrence of antibiotic residues and antibiotic resistance genes in soil: A meta-analysis study.Chemosphere 311, 134529. doi:10.1016/j.chemosphere.2022.134529
Keywords: antibiotics, manure, soil, chicken farm, risk assessment
Citation: Zha Y, Li Q, Liu H, Ge Y, Wei Y, Wang H, Zhang L, Fan J, Chen Y, Zhang C, Zhou T and Zhang W (2023) Occurrence and ecological risk assessment of antibiotics in manure and the surrounding soil from typical chicken farms in Hangzhou, China. Front. Environ. Sci. 11:1241405. doi: 10.3389/fenvs.2023.1241405
Received: 16 June 2023; Accepted: 28 September 2023;
Published: 09 October 2023.
Edited by:
Hua-jun Huang, Jiangxi Agricultural University, ChinaReviewed by:
Hang Yu, Dalian Maritime University, ChinaZhihua Xiao, Hunan Agricultural University, China
Copyright © 2023 Zha, Li, Liu, Ge, Wei, Wang, Zhang, Fan, Chen, Zhang, Zhou and Zhang. This is an open-access article distributed under the terms of the Creative Commons Attribution License (CC BY). The use, distribution or reproduction in other forums is permitted, provided the original author(s) and the copyright owner(s) are credited and that the original publication in this journal is cited, in accordance with accepted academic practice. No use, distribution or reproduction is permitted which does not comply with these terms.
*Correspondence: Tiefeng Zhou, ZHVuMDgxNnFpQDE2My5jb20=; Weiwu Zhang, ZWNvc2NpaHp4aEAxNjMuY29t