- 1Laboratory of Molecular Biology, Institute of Crop and Ecology, Hangzhou Academy of Agricultural Sciences, Hangzhou, China
- 2Hangzhou Agricultural Technology Extension Center, Hangzhou, China
- 3Laboratory of Plant Molecular Biology and Proteomics, Institute of Biotechnology, Hangzhou Academy of Agricultural Sciences, Hangzhou, China
- 4Institute of Fishers Institute, Hangzhou Academy of Agricultural Sciences, Hangzhou, China
- 5Hangzhou Academy of Agricultural Sciences, Hangzhou, China
Abstract
Biochar is a versatile, carbon-rich, organic material that can effectively immobilize Cd in the soil. In this study, peanut shell biochar (SP), maize straw biochar (MS), and bamboo straw biochar (BS) were applied in different proportions to evaluate their effects on the remediation of Cd-contaminated farmland soil and plant growth. The results revealed that both single and mixed applications of biochar substantially increased corn biomass and chlorophyll content compared to the unamended control treatment, while the malondialdehyde (MDA) and proline contents were largely unaffected. The bamboo straw block biochar with maize straw biochar at a mass ratio of 2:1 (DBM) significantly increased the dry total biomass of maize (+107.24% compared to the unamended soil). SP application has highly increased the SPAD value. PB with BS application at a mass ratio of 1:1 (MSB) significantly decreased the soluble sugar content (+21.81% compared to the unamended control soil). Soil pH was increased by the application of biochar alone and in combination with feedstocks. The soil content of Fe/Mn oxide-bound (OX) and exchangeable-bound Cd (EX) was decreased, whereas that of carbonate-bound Cd (CA), residue-bound Cd (RE), and organic-bound Cd (OM) contents increased. The Cd content in corn grains under MSB and SP application was markedly reduced by 42.62% and 31.48%, respectively, compared to the unamended control soil. Overall, MSB and SP applications were effective in improving soil quality and crop growth.
1 Introduction
Many soils worldwide are contaminated with heavy metals due to industrial production, industrial activities, waste disposal, and improper management of pesticides and chemicals (Duan et al., 2016; Zhang A F et al., 2017; Zhang R H et al., 2017; Xie et al., 2021; Aown et al., 2022). Among the global environmental concerns related to soil, heavy metal pollution is particularly acute in China. According to a 2014 publication by the National Soil Pollution Survey Bulletin (NSPSB), approximately 19.4% of China’s arable soils are plagued by heavy metal contamination (about 2.0 × 107 ha of agricultural soil), of which cadmium (Cd) is the most concerning (Department of Environmental Protection, 2014). Cd in the soil can combine with soil colloids, organic matter, and minerals. It can also exist in the soil pore water environment in the ionic form (such as Cd2+, CdOH+, and CdCl+) (Wan et al., 2018). The Cd content in soil pores and its morphology are the main reasons for its biological effects on soil. Given the persistency of characteristics of heavy metals such as Cd and their detrimental impact on food security and human health, it is imperative to introduce stabilizing substances into the soil. The addition of stabilizing substances plays a key role in achieving this objective, preventing the entry of Cd into critical pathways of food crops (Zhao et al., 2015; Li et al., 2016).
Biochar is capable of binding heavy metal ions and reducing their harmful effects through surface precipitation, absorption, ion exchange, and electrostatic binding due to its high specific surface area; physicochemical properties, e.g., abundant surface oxygen-containing functional groups; and high pH (Liang et al., 2017; Michal et al., 2022). Crop straw is rich in organic carbon (OC), phosphorus (P), nitrogen (N), trace elements, and potassium (K) (Cui et al., 2019). In recent years, biochar prepared by straw pyrolysis has been widely used for agricultural carbon sequestration and soil fertility improvement (Holatko et al., 2022; Yang et al., 2022; Sun et al., 2023). In addition to remediating heavy metal and organic compound-contaminated soils, biochar also acts as a catalyst, positively influencing crop growth and enhancing yield (Xu et al., 2019; Zha et al., 2022). Cui et al. (2019) found that long-term incubation tests confirmed the stability of wheat straw biochar, which effectively decreased Cd bioavailability by approximately 90% compared to the control. The findings from Bashir et al. (2018a); Bashir et al. (2018b) revealed that the utilization of biochar triggered a substantial decline in the bioavailability of Cd and chromium (Cr), achieving reductions of 85% and 63%, respectively. China is the world’s largest producer of bamboo and has abundant bamboo charcoal resources (Liu et al., 2011; Xu et al., 2012). Peanut, being one of the crucial oil crops globally, boasts significant importance. Furthermore, peanut shell biochar exhibits a notable ability to uptake Cd (Christou et al., 2017). Maize straw efficiently adsorbs heavy metals and reduces Cd bioavailability in plants and soils (Gondek et al., 2018). However, biochar prepared from these different species has different physical and chemical properties (Michal et al., 2022; Ángela et al., 2022). Due to these differences in the physico-chemical properties of biochar, it is possible that biochar application alone will not alleviate soil heavy metal pollution.
As one of the primary staple crops in China, maize occupies a significant position in agriculture. Its remarkable growth rate and high yield make it the preferred cereal for phytoremediation of soil contaminated with heavy metals (Bai et al., 2017; Sarwar et al., 2017; Huerta et al., 2022). Previous studies have demonstrated that in maize cultivated in Cd-contaminated soil, the heavy metal concentration in the seeds of most maize varieties exceeds the safety standard (Yang et al., 2018; Zha et al., 2023). This study sought to establish an effective means of immobilizing Cd in contaminated soil, with the specific aim of ensuring the safety of maize cultivation. In this study, maize was used as an experimental material. The effect of the type of biochar and the amount needed to be applied to soil to control Cd pollution is unclear. Thus, the principal goal of this study was to investigate the practicality of utilizing different types of biochar in varying mass ratios and to assess the effects of different biochar combinations on soil and plant properties.
2 Materials and methods
2.1 Soil and biochar characterization
Surface soil samples (0–20 cm) were specifically gathered from a paddy field situated in Wangyan Village, Qiantan Town, Jiande City, which is positioned west of Hangzhou City in Zhejiang Province (29°39′25″ N, 119°35′38″ E). The soil samples collected from this area have been continuously impacted by surface runoff pollution from a nearby mine for a span of 30 years. Following an “S” pattern, five sampling points were carefully determined within the collection area to ensure representative coverage. To maintain uniformity, the soil was left to air-dry for 7 days and subsequently sieved using a 2-mm pore-size sieve. This preparatory step was performed prior to conducting various physico-chemical analyses. The soil analysis revealed a pH value of 6.78 and an electrical conductivity (EC) value of 1.58 dS m−1. Additionally, the organic matter content of the soil was determined to be 0.92%. Measured in mg kg−1 DW (dry weight), the total concentrations of Cd, Pb, Zn, and Cr in the soil were 4.57, 53.10, 176.98, and 135.93, respectively. According to the soil environmental quality agricultural land pollution risk control (GB15618-2018), the chosen agricultural land soil initially revealed contamination only by Cd, while the levels of other elements remained below the specified thresholds.
The carbonization of peanut shell biochar (SP) and bamboo biochar (BS) was carried out by Henan Lize Environmental Protection Technology Company at a temperature of 700°C. A continuous slow pyrolysis machine was employed to produce maize (variety: Qianjiangnuo NO.3) straw block biochar (MS) by subjecting it to a final temperature of 550°C for a retention time of 30 min at the Hangzhou Academy of Agricultural Sciences at approximately 700°C in a batch pyrolysis facility. The physicochemical properties of the biochars and heavy metal-contaminated soil are shown in Table 1.
2.2 Greenhouse experiment
The treatments included Cd-contaminated soil amended with single biochar (SP, BS, and MS), mixtures of two biochars in equal mass ratios (MSB, MSM, and MBM), and mixtures of two biochars in a 1:2 (w/w) ratio (DSB, DSM, and DBM) (Table 2). Under greenhouse conditions, after sterilizing the corn seeds with hydrogen peroxide, 10 seeds were sown in each pot. One week after seedling emergence, three uniformly growing corn plants were retained in each pot. The experimental setup involved growing maize in plastic pots filled with 20 kg of air-dried soil. The soil was enriched with a 5% mixture of biochars (Wbiochar/Wsoil). Unamended soil was designated as the control group. Five replicates were organized for each treatment. The experiment plants were maize (variety: Qianjiangnuo NO.3), which can accumulate heavy metals. In each pot, five maize seeds were sown. Following a 6-day germination period, three seedlings exhibiting robust growth were selected. Fertilizers (K2SO4–DAP–urea) were administered at a rate of 120–50–25 kg ha−1 applying irrigation water. Two rounds of urea fertilizer application were conducted (i.e., initially applied in conjunction with other fertilizers, and a subsequent application was conducted after a 15-day period).
After 82 days, the maize plants were harvested. The plants were dissected into four distinct parts (roots, stems, leaves, and grains). Each part was then gathered and prepared for laboratory analysis. A 15-min immersion of maize roots in a solution containing 20 mM of Na2EDTA was carried out to facilitate the removal of heavy metal ions attached to the root surface. Following the immersion, the roots underwent a comprehensive cleaning process using deionized water. All roots, stems, leaves, and grains underwent a thorough washing process, which was repeated three times. The four distinct plant parts were subjected to a drying process at 65°C for 72 h, allowing them to reach a constant weight. The biomass of each part was then confirmed using a balance (Setra Systems Inc. Boxborough, MA, and United States). The chlorophyll content was determined using the Soil and Plant Analyzer Development (SPAD) method (SPAD) (SPAD-TYS-A (Jinkeli, Inc., Beijing).
2.3 Analysis method of soil and plant
Soil and plant samples were collected simultaneously on the 82nd day after planting. Di-acid digestion was carried out on the dry weights (0.5 g) of both the soil and different maize parts. Nitric acid (HNO3) and perchloric acid (HClO4) were combined in a 1:3 ratio for the digestion process, ensued by heating on a hot plate at 250°C. Following filtration, the samples were subjected to Cd analyses using an atomic absorption spectrophotometer (AAS) (z2000, Hitachi, Tokyo, Japan). Samples of soil were digested by acidic solutions for available P and K analyses and were estimated in terms of the method elaborated by Page (1982). Soil Cd in the active state was determined by diethylenetriaminepentaacetic acid (DTPA) leaching and inductively coupled plasma–atomic emission spectrometry (ICP-AES) (HJ 804-2016). Soil samples were processed using the method reported by Tessier et al. (1979). The soil cadmium morphology can be segmented into five states: exchangeable state (EX), organic-bound state (OM), iron- and manganese oxide-bound state (OX), residual state (RE), and carbonate-bound state (CA).
The determination of proline content, MDA levels, and soluble sugar content involved was performed using the Saradhi method with acid ninhydrin solution (Aila and Saradhi, 1991), thiobarbituric acid (TBA) colorimetry (Duan et al., 2018), and anthrone colorimetry (Tashiro et al., 1997).
2.4 Statistical analysis
One-way analysis of variance (ANOVA) was performed using IBM SPSS 17.0 statistical software (SPSS Inc., Chicago, United States) to determine the significance of differences between the treatments. Tukey’s range test (p = 0.05) was employed to compare the mean values and detect significant differences between the treatments. All tables and figures were created using Excel and Origin 8.0, respectively.
3 Results
3.1 Effect of biochars on maize biomass
In this study, the maize total biomass and root, stem, and leaf biomass in all amended samples of biochar were significantly higher than those in the unamended control sample (p < 0.05) (Table 3). The DBM treatments resulted in the highest biomass, followed by the DSM and DSB treatments. The maize total biomass amended with DBM, DSM, and DSB was 3.47, 2.49, and 2.08 times, respectively, higher than that of the unamended control. Grains subjected to the BS, DSB, DSM, and DBM treatments displayed prominently higher biomass than the unamended control (p < 0.05). The bract biomass under BS, MS DSB, DSM, and DBM was significantly higher than that of the unamended control (p < 0.05). The highest total biomass and different parts of the biomass of maize were observed in DBM treatment. The application of DBM significantly increased the total biomass and root, stem, leaf, grain, and bract biomass in maize by 346.67%, 143.32%, 196.50%, 43.83%, 120.49%, and 107.24%, respectively.
3.2 Effect of biochars on maize physiology properties
As shown in Figure 1, both individual and mixed biochar treatments significantly increased maize chlorophyll content, as indicated by increased SPAD values (Figure 1A). The application of SP, DBM, and DSB significantly increased the SPAD in maize by 21.81%, 17.91%, and 17.51%, respectively. The findings deliver compelling evidence that the application of biochar had a significant alleviating influence on heavy metal stress.
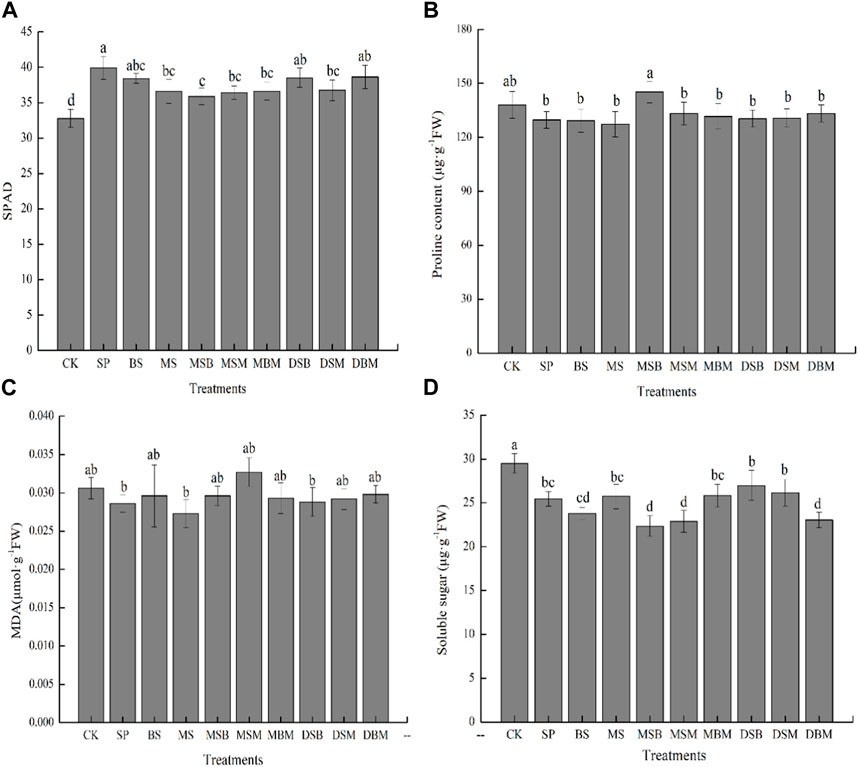
FIGURE 1. SPAD values (A), proline contents (B), MDA contents (C), and soluble sugar contents (D) of maize grown in Cd-contaminated soils amended with biochars. The abbreviations for biochar treatments are reported in Table 2. Error bars indicate standard deviation (n = 3). Different letters indicate statistically significant differences (p < 0.05).
In this study, the concentration of proline was lower in all amended treatments of biochars, except MSB treatments (Figure 1B). However, the difference was statistically nonsignificant. As shown in Figure 1C, the MDA concentration in all treatments of biochars was lower than in the control. Nevertheless, no statistically significant differences were observed between the biochar treatments and the control conditions. The soluble sugar concentration was significantly lower in all treatments than in the control (p < 0.05) (Figure 1D). The application of MSB, MSM, and DSB significantly decreased the soluble sugar concentration in maize by 24.24%, 22.37%, and 21.83%, respectively.
3.3 Effects of biochars on soil physical and chemical properties
Soil pH decreased significantly in the soil amended with BS, MS, and DSB (p < 0.05) (Figure 2A). Soil pH was positively influenced by the addition of SP, MSB, and MSM, surpassing the levels recorded in the BS-, MS-, and DBM-treated soils. Notably, MSB displayed the highest impact, resulting in a substantial increase of 0.34 units compared to the unamended soil.
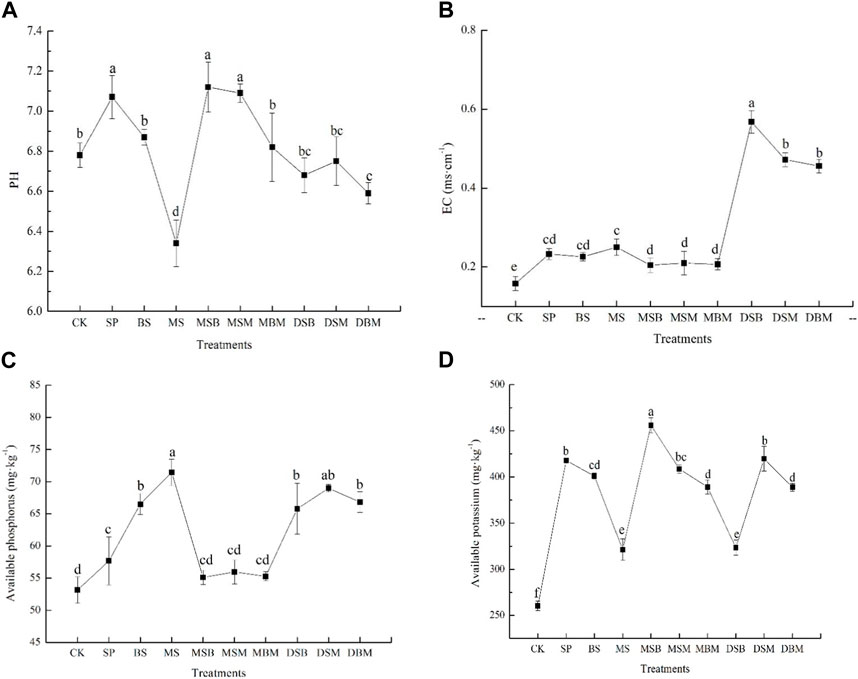
FIGURE 2. pH (A), EC (B), available phosphorus (C), and available potassium (D) in soils contaminated with Cd and amended with biochars. The abbreviations for biochar treatments are reported in Table 2. Error bars indicate standard deviation (n = 3). Different letters indicate statistically significant differences (p < 0.05).
The EC was significantly higher under all amended biochar samples than in the control (p < 0.05) (Figure 2B). The application of DSB, DSM, and DBM significantly increased the EC in soil by 259.49%, 198.73%, and 188.61%, respectively.
The available P content was significantly higher in soils amended with SP, BS, MS, DSB, DSM, and DBM than in the control (p < 0.05) (Figure 2C). The application of MS, DSM, and DBM markedly elevated the available P content in soil by 34.49%, 29.83%, and 25.78%, respectively. The available K content was significantly higher in soils after the application of biochars (p < 0.05) (Figure 2D). The soil treatments with MSB and SP exhibited significantly higher available K content than the other biochar treatments (p < 0.05). The application of MSB increased the available K content in the soil by 61.16%, while SP increased the available K content by 60.43%.
3.4 Effects of biochars on Cd content
In this study, the Cd content was higher in biochar-amended soils than in the unamended control soil. The Cd content amended with BS, MS, and DSB was significantly higher in amended soils than in the unamended control soil (p < 0.05) (Figure 3). Significant enhancements in soil Cd content were observed when BS and MS were used, with increases of 13.97% and 13.32%, respectively. All applications of biochar decreased the exchangeable Cd contents in soil. The inclusion of SP and DBM resulted in a substantial decline in the soil exchangeable Cd content, with reductions of 45.69% and 44.57%, respectively (p < 0.05).
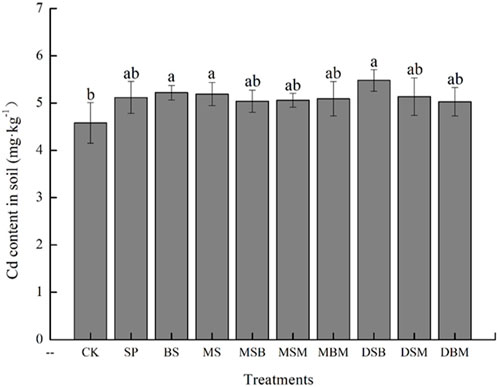
FIGURE 3. Cd content in soil in Cd-contaminated soil amended with biochars. The abbreviations for biochar treatments are reported in Table 2. Error bars indicate standard deviation (n = 3). Different letters indicate statistically significant differences (p < 0.05).
3.5 Effects of biochars on Cd morphology in contaminated soil
Because the Cd concentration varied greatly among the different biochars, studying the changes in the proportion of each form of Cd under the different biochar treatments could more intuitively reflect the effect of biochar on the morphological transformation of Cd. Figure 4 indicates a significant difference in soil’s exchangeable-bound (EX-bound) Cd content between the biochar treatments and the control (CK) (p < 0.05). The soil EX-bound Cd concentration was reduced by 45.30% and 46.37% under the DSB and DBM treatments, respectively (Figure 4A). The soil CA-bound Cd concentration under the biochar treatments was significantly higher than that of the CK and was considered too high for safe maize cultivation under the DSB treatment, with an increase of 96.32% compared to the CK (Figure 4B). The soil OX-bound Cd concentration under the MSB, MBM, DSB, DSM, and DBM treatments was significantly lower than that of the CK, with decreases of 19.49%, 22.68%, 34.28%, 22.17%, and 22.22%, respectively (Figure 4C). The soil OM-bound Cd concentration increased under the different biochar treatments compared to the CK, with increases of 105.53%, 175.60%, 78.75%, 81.00%, and 95.11% under the SP, BS, MS, DSM, and DBM treatments, respectively (Figure 4D). The soil RE-bound Cd concentration increased under the different biochar treatments, with 19.19%, 21.60%, 24.07%, 41.79%, 19.25%, and 13.96% increases compared to the CK under the MSB, MSM, MBM, DSB, DSM, and DBM treatments, respectively (Figure 4E). In conclusion, the application of biochar induced the conversion of soil Cd from the more active form to the more stable form, thus reducing the harmful effects of soil Cd.
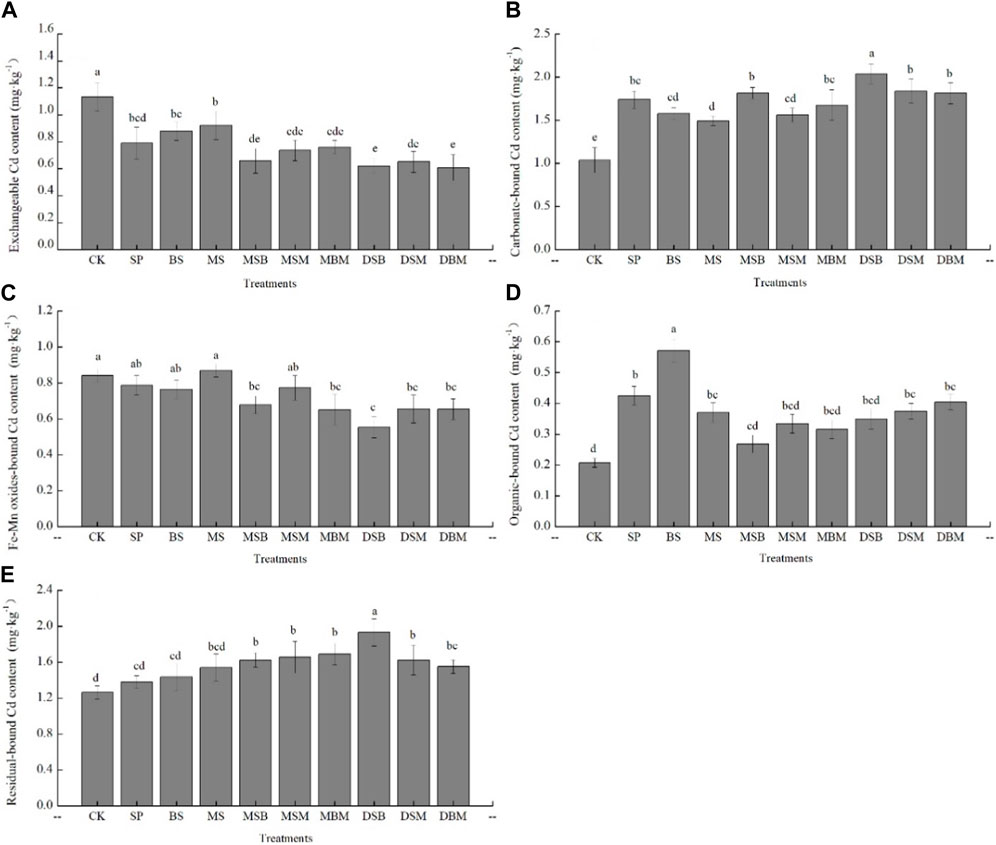
FIGURE 4. Exchangeable, carbonate, Fe-Mn oxides, organic and residual-bound Cd content in Cd-contaminated soil amended with biochars. The abbreviations for biochar treatments are reported in Table 2. Error bars indicate standard deviation (n = 3). Different letters indicate statistically significant differences (p < 0.05).
3.6 Effects of biochars on Cd accumulation in the tissues of maize
The Cd concentration in different parts of maize was as follows: root > leaf > stem > grains (Figure 5). In this study, the Cd content in different parts of maize was lower under biochar-amended samples than in the control. The application of SP, BS, MSB, MBM, DSB, DSM, and DBM significantly decreased the Cd content in maize root (p < 0.05) (Figure 5A). The lowest Cd content in maize root was observed in treatments of SP and DBM, which decreased the Cd content by 52.99% and 47.55%, respectively. However, the application of MS and MSM increased the Cd content in maize root by 1.90% and 65.22%, respectively. Treatment of the soil with SP, BS, MSB, and DBM significantly decreased the Cd content in maize leaf (p < 0.05) (Figure 5B). The lowest Cd content in maize root was observed in treatments of the application of SP and MSB, which decreased the Cd content in maize leaves by 42.62% and 31.48%, respectively. The application of SP, MSB, and DBM significantly decreased the Cd content in maize stem (p < 0.05) (Figure 5C). The lowest Cd content in maize stem was observed in treatments of MSB, which decreased the Cd content by 46.37%. Treatments of soil with SP, BS, MSB, MBM, DSB, and DBM significantly decreased the Cd content in maize grains (p < 0.05) (Figure 5D). The application of SP and DBM significantly decreased the Cd content in maize grain by 32.68% and 43.14%, respectively.
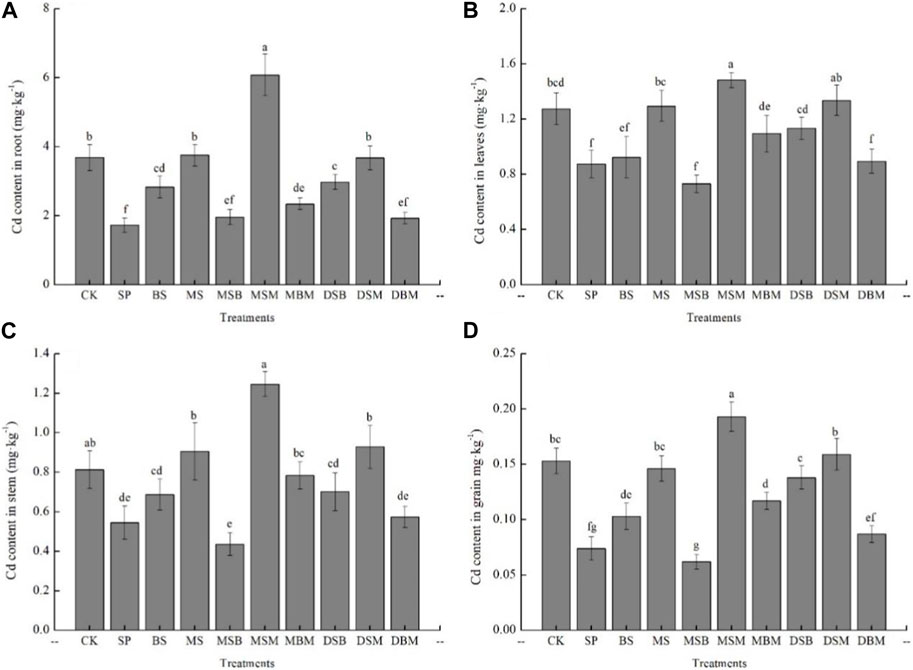
FIGURE 5. Cd content on the maize root, leave, stem and grain in Cd-contaminated soil amend with biochars. The abbreviations for biochar treatments are reported in Table 2. Error bars indicate standard deviation (n = 3). Different letters indicate statistically significant differences (p < 0.05).
4 Discussion
4.1 Effects of biochars on Cd accumulation in the tissues of maize
Biochar application can increase soil nutrient cycling, improve soil physicochemical properties, and increase soil N content, all of which positively affect plant growth (Jin et al., 2019; Yu et al., 2019; Ángela et al., 2022). Sun et al. (2023) found that N increased by 23.95% under 29.4 mg ha-1 biochar application, which substantially improved soil fertility. Higher levels of biochar application contribute to N retention in the soil and promote plant growth (Chan et al., 2007; Guerena et al., 2013). Another study found that the application of phosphorus fertilizer and biochar increased wheat yield, possibly owing to improvements in soil physical properties and organic matter (Mahmoud et al., 2017). The different types of biochar treatments in the present study increased maize plant biomass by varying degrees, which may be due to differences in the physicochemical properties of biochar prepared from peanut shells, bamboo, and maize stover (Michal et al., 2022). In this study, an increase in corn biomass corresponded to an increase in soil effective phosphorus and potassium. This suggests that biochar application is highly effective in improving soil fertility and promoting plant growth (Yang et al., 2022).
Chlorophyll is an important indicator of the plant response to different environmental stresses, and reactive oxygen species production disrupts protein complexes and inhibits chlorophyll production (Oliveira, 2012; Jia et al., 2018). In the present study, the chlorophyll content of maize plants was effectively increased by applying biochar alone or in combination. Related studies have found that the addition of biochar to heavy metal-contaminated soil can increase plant chlorophyll content and reduce oxidative stress, favoring plant growth.
Proline is an important physiological indicator for maintaining the osmotic balance between protoplasts and the environment and resisting the damage caused by heavy metal ions (Schat et al., 2010). The content of proline in plant leaves was lower under no-stress conditions (Liu et al., 2015). Ángela et al. (2022) found that biochar improved antioxidant defenses in plants, which is a clear advantage for plant growth under stress conditions. Another study concluded that the addition of biochar reduced Cr toxicity by decreasing antioxidant enzyme activity. Plants were, therefore, less stressed (Younis et al., 2015). The addition of biochar to the soil improved the physiological characteristics of maize in heavy metal-contaminated soil, and these results may be related to the significant reduction in exchangeable Cd concentration in the contaminated soil after biochar addition. In this study, the effect of each treatment on maize proline was insignificant, probably because heavy metal stress itself had only a small effect on proline. MDA is an important physiological indicator of the degree of damage to the plant membrane system (Abdelhafez et al., 2014), and the difference in the MDA content between different biochar treatments in this study was not significant, probably because the amount of biochar applied was less. Soluble sugar is a sensitive index of plant response to heavy metal stress, and its content decreases with the increase in heavy metal concentration (Zhang et al., 2014). In this study, the soluble sugar content under the MSB, MSM, and DSB treatments was prominently lower than that of the single-biochar strategy, which indicated that the biochar application was effective in reducing soil Cd stress.
4.2 Effects of biochar on soil properties
Throughout the biochar production process of pyrolysis, alkaline cations (such as Ca, Mg, K, and Na) present in the biomass are converted into distinct alkaline compounds (e.g., ash comprising oxides, hydroxides, and carbonates). This process results in the dissolution of these alkaline compounds, thereby increasing the pH level of the soil (Houben et al., 2013; Kelly et al., 2014). The soil pH under the SP, MSB, and MSM treatments was significantly higher than that of the untreated soil, which may be because peanut shell biochar has a high pH. In addition, the presence of iron and manganese oxides in soil contributes to its amphiphilic colloidal properties, with their adsorption primarily governed by the negative surface charge. Through its interaction with the soil, biochar plays a role in elevating soil pH by decreasing the concentrations of H+, Fe3+, Al3+, and Mn2+ ions in the soil solution (Liu et al., 2014). In this study, significantly higher EC values were uncovered in soils modified with multiple types of biochar compared to the control, implying that elevated pH levels could influence the movement of heavy metals and enhance the cationic exchange capacity of the soil. The current study showed that the addition of biochar affected Cd adsorption, which may be the key reason for the reduction in Cd bioavailability in maize compared to that in the control. Sousa et al. (2018) found that the addition of a composite mixture of biochar and manure affected the adsorption of Cr and reduced its translocation from roots to stems (Sousa et al., 2018). In addition, Xia et al. (2020) found that the continuous addition of biochar altered the physical properties of the soil, such as pH and moisture and water-holding capacity, which led to changes in environmental conditions for soil microbes and nitrogen (N) cycling. This may also be the main reason for the reduction in N loss and the increase in soil fertility (Thangarajan et al., 2018).
4.3 Effects of biochar on the total Cd concentration and soil Cd morphology
The research conducted by Yang et al. (2016) demonstrated an inverse correlation between soil pH and the CaCl2-extractable Cd concentration in heavy metal-contaminated soil, which was observed in soils that received supplementation with bamboo charcoal biochar and rice straw biochar (Yang et al., 2016). The biochar increased the soil pH, resulting in the precipitation of Cd as CdCO3 (Xu et al., 2016). Yin et al. (2016) used 1%–2% rice straw biochar to treat agricultural soils in mining areas and found a significant reduction in the soil inter-root pore water Cd concentration (Yin et al., 2016). Corn straw biochar prepared by Gao et al. (2017) reduced the extractable Cd content in agricultural soils by 91% (Gao et al., 2017). In this study, the increase in the soil Cd content and decrease in the effective Cd content under different biochar treatments were mainly related to the increase in pH. The introduction of biochar into the soil increased its pH, triggering the hydrolysis of heavy metal cations. Consequently, oxide precipitates were formed, leading to a decrease in the exchangeable Cd concentration within the soil. Additionally, multiple investigations have revealed that biochar implementation can significantly affect the sorption and desorption behavior of heavy metals in soil.
The biotoxicity and harmful effects of heavy metals are not only related to their contents but are also determined by their fugitive forms. Following soil amendment due to the application of different biochars, the CA-bound Cd, RE-bound Cd, and OM-bound Cd concentrations increased and the OX-bound Cd concentration decreased, indicating that the application of biochar promoted the conversion of soil Cd from the active to the stable state. Biochar can interact with soil Cd by electrostatic adsorption, ion exchange, complexation, co-precipitation, and cation–π-bonding interactions, thus changing the fugitive form of soil Cd from the highly active form to the less active form, and finally achieving the passivation of soil Cd (Xia et al., 2020; Zhang et al., 2020). Biochar treatment significantly reduced the oxide-bound metal fraction. Jiang et al. observed a similar metal immobilization effect of biochar. In this study, biochar application reduced the oxide-bound metal fraction, but the differences between treatments were small. The Fe and Mn oxide-bound Cd is a transitional form, which is generally not easily absorbed and utilized by plants, but it is converted to the active state with changes in soil environmental conditions.
It has been documented that an increase in soil pH leads to an increase in negative charges on clay minerals, hydrated oxides, and organic matter surfaces. Consequently, the sorption capacity of these components for Cd2+ boosts, facilitating the formation and precipitation of CdCO3 and Cd(OH)2. This may have been responsible for the increase in CA-bound Cd (Chen et al., 2020). The increase in the OM-bound Cd concentration in soil may have been due to the abundance of OM-bound Cd in soil and the high concentrations of carboxyl, aldehyde, ketone, and aromatic substances in the carboxyl groups of biochar and crop straw decomposition products. The ability to retain ions was enhanced, gradually manifesting distinct colloidal characteristics (Li et al., 2017). In this study, the OM-bound Cd content of biochar under the MSM and MBM treatments increased, probably due to the higher ion retention ability of biochar produced from peanut shells and corn stover and biochar applied with bamboo charcoal and corn stover.
Biochar application in acidic rice soils caused a higher residual fraction of Cd, which can be attributed to the robust inner-sphere complexation between the metals and biochar particles (Zhu et al., 2015; Cui et al., 2016). The increase in the reducible fraction of Cd after biochar application could be attributed to the adsorption of Fe/Mn oxides (Fang et al., 2016), which in turn further increased the adsorption of Cd onto Fe and Mn oxides (Li et al., 2013).
4.4 Effects of biochar on the total Cd concentration in maize
The findings of this investigation demonstrated that biochar significantly reduced the harmful biological effects of soil Cd, and thus the uptake and transport of Cd by maize. A significant reduction of Cd was achieved by adopting diverse biochar types in combination. The addition of biochar derived from bean stalk and rice straw, as highlighted by Zheng’s study, exhibited a significant reduction in the Cd content within rice roots, shoots, husks, and seeds (Zheng et al., 2012), which was proven to be consistent with the findings of this study. Liu et al. (2014) observed a substantial decline in the Cd concentration in crops following the application of bamboo stalk biochar. Aown et al. (2022) applied a biochar mixture to reduce bioavailable extractable Cr concentrations in soil by 36%, and soil Cd bioavailability was lower in the composite, amended treatment than in the control treatment (Aown et al., 2022). These findings suggested that biochar application can effectively immobilize Cd, reducing its mobility in the soil and inhibiting its uptake and accumulation in the aboveground tissues of crops. Additionally, Mohamed et al. (2018) found that the Cd concentration was higher in the belowground parts of wheat than in the aboveground parts (Mohamed et al., 2018), which was consistent with the pattern observed in this study. The Cd concentration in the root system of maize was higher than that in the straw, confirming that maize reduces the toxic effect of Cd by fixing it in the root system and inhibiting its transfer to the aboveground tissues. In summary, the utilization of biochar contributed to the mineral nutrition provided to the crop, which in turn weakened the synergistic effect between Cd and other cationic metals and nonmetals.
5 Conclusion
Both individual and mixed-biochar applications increased maize plant biomass to varying degrees and enhanced maize Cd stress tolerance by increasing leaf chlorophyll content and decreasing soluble sugar content. In addition, both individual and mixed applications of biochar effectively increased the soil pH, which might have led to the conservation of N nutrients and an increase in soil fertility, which is an obvious advantage for plant growth under heavy metal stress. The Cd content of maize grains under the SP treatment was reduced to 0.1 mg kg−1, which met the national standard for food safety and the limit of contaminants in food. This study confirmed that different mass ratios mixed with different biochars are important in immobilizing Cd in contaminated soil to ensure food security. Therefore, it can be concluded that the application of biochar in Cd-contaminated soil is an option for improvements in soil quality and crop growth. In the future, it will be necessary to evaluate the long-term effects of biochar application on soils and crops contaminated with heavy metals through field trials.
Data availability statement
The original contributions presented in the study are included in the article/Supplementary Material, further inquiries can be directed to the corresponding author.
Author contributions
Conceptualization: YZ; methodology: YZ and LZ; validation: YC and TN; formal analysis: XW; investigation: YZ; resources: JS; data curation: YZ; writing—original draft preparation: YZ; writing—review and editing: YZ; visualization: XB; supervision: EY and LZ; project administration: JS and TZ; funding acquisition: YZ. All authors contributed to the article and approved the submitted version.
Funding
This work is supported by the Natural Science Foundation of Zhejiang Province (LQ20C030007), the Hangzhou Agricultural and Social Development Scientific Research Project (20201203B108, 20201203B119), the Science and Technology Innovation Fund of Hangzhou Academy of Agricultural Sciences (2022HNCT-08), and the Zhejiang Provincial Key Research and Development Project (2019C02035).
Conflict of interest
The authors declare that the research was conducted in the absence of any commercial or financial relationships that could be construed as a potential conflict of interest.
Publisher’s note
All claims expressed in this article are solely those of the authors and do not necessarily represent those of their affiliated organizations, or those of the publisher, the editors, and the reviewers. Any product that may be evaluated in this article, or claim that may be made by its manufacturer, is not guaranteed or endorsed by the publisher.
References
Abdelhafez, A. A., Li, J., and Abbas, M. H. H. (2014). Feasibility of biochar manufactured from organic wastes on the stabilization of heavy metals in a metal smelter contaminated soil. Chemosphere 117 (1), 66–71. doi:10.1016/j.chemosphere.2014.05.086
Aila, P., and Saradhi, P. P. (1991). Proline accumulation under heavy metal stress. J. Plant Physiol. 138 (5), 554–558. doi:10.1016/S0176-1617(11)80240-3
Ángela, M. G., Jorge, P., and Carolina, E. (2022). Overview of the use of biochar from main cereals to stimulate plant growth. Front. Plant Sci. 13, 912264. doi:10.3389/fpls.2022.912264
Aown, A., Muhammad, A., Muhammad, N., Abdul, L., Saqib, B., Amjad, A., et al. (2022). Synergistic use of biochar and acidified manure for improving growth of maize in chromium contaminated soil. Int. J. Phytoremediation 22 (1), 52–61. doi:10.1080/15226514.2019.1644286
Bai, Y. C., Zuo, W. G., Zhao, H. T., Mei, L. J., Gu, C. H., Guan, Y. X., et al. (2017). Distribution of heavy metals in maize and mudflat saline soil amended by sewage sludge. J. Soils Sediments 17 (6), 1565–1578. doi:10.1007/s11368-016-1630-z
Bashir, S., Hussain, Q., Akmal, M., Riaz, M., Hu, H., Ijaz, S. S., et al. (2018b). Sugarcane bagasse-derived biochar reduces the cadmium and chromium bioavailability to mash bean and enhances the microbial activity in contaminated soil. J. Soil Sediments 18 (3), 874–886. doi:10.1007/s11368-017-1796-z
Bashir, S., Zhu, J., Fu, Q., and Hu, H. (2018a). Cadmium mobility, uptake and anti-oxidative response of water spinach (Ipomoea Aquatic) under rice straw biochar, zeolite and rock phosphate as amendments. Chemosphere 19, 579–587. doi:10.1016/j.chemosphere.2017.11.162
Chan, K. Y., Van Zwieten, L., Meszaros, I., Downie, A., and Joseph, S. (2007). Agronomic values of greenwaste biochar as a soil amendment. Soil Res. 45, 629–634. doi:10.1071/SR07109
Chen, X., He, H. Z., Chen, G. K., and Li, H. S. (2020). Effects of biochar and crop straws on the bioavailability of cadmium in contaminated soil. Sci. Rep. 10 (1), 9528. doi:10.1038/s41598-020-65631-8
Christou, A., Theologides, C. P., Coasta, C., Kalavrouziotis, I. K., and Varnavas, S. P. (2017). Assessment of toxic heavy metals concentrations in soils and wild and cultivated plant species in Limni abandoned copper mining site, Cyprus. J. Geochem. Explor. 178, 16–22. doi:10.1016/j.gexplo.2017.03.012
Cui, L., Noerpel, M. R., Scheckel, K. G., and Ippolito, J. A. (2019). Wheat straw biochar reduces environmental cadmium bioavailability. Environ. Int. 126, 69–75. doi:10.1016/j.envint.2019.02.022
Cui, L. Q., Pan, G. X., Li, L. Q., Bian, R. J., Liu, X. Y., Yan, J. L., et al. (2016). Continuous immobilization of cadmium and lead in biochar amended contaminated paddy soil: a five-year field experiment. Ecol. Eng. 93, 1–8. doi:10.1016/j.ecoleng.2016.05.007
Department of Environmental Protection (2014). Ministry of Land and Resources. National survey of soil pollution. Beijing: Department of Environmental Protection. Ministry of Land and Resources.
Duan, C., Fang, L., Yang, C., Chen, W., Cui, Y., and Li, S. (2018). Reveal the response of enzyme activities to heavy metals through in situ zymography. Ecotox Environ. Safe 156, 106–115. doi:10.1016/j.ecoenv.2018.03.015
Duan, Q. M., Lee, J. C., Liu, Y. S., Chen, H., and Hu, H. Y. (2016). Distribution of heavy metal pollution in surface soil samples in China: a graphical review. Bull. Environ. Contam. Toxicol. 97 (3), 303–309. doi:10.1007/s00128-016-1857-9
Fang, S. E., Tsang, D. C. W., Zhou, F. S., Zhang, W. H., and Qiu, R. L. (2016). Stabilization of cationic and anionic metal species in contaminated soils using sludge-derived biochar. Chemosphere 149, 263–271. doi:10.1016/j.chemosphere.2016.01.060
Gao, J. K., Lv, J. L., Wu, H. M., Dai, Y. C., and Nasir, M. (2017). Impacts of wheat straw addition on dissolved organic matter characteristics in cadmium-contaminated soils: insights from fluorescence spectroscopy and environmental implications. Chemosphere 193, 1027–1035. doi:10.1016/j.chemosphere.2017.11.112
Gondek, K., Mierzwa, H. M., and Kopeć, M. (2018). Mobility of heavy metals in sandy soil after application of composts produced from maize straw, sewage sludge and biochar. J. Environ. Manage 210, 87–95. doi:10.1016/j.jenvman.2018.01.023
Guerena, D., Lehmann, J., Hanley, K., Enders, A., Hyland, C., and Riha, S. (2013). Nitrogen dynamics following field application of biochar in a temperate North American maize-based production system. Plant Soil 365, 239–254. doi:10.1007/s11104-012-1383-4
Holatko, J., Hammerschmiedt, T., Kucerik, J., Baltazar, T., Radziemska, M., Havlicek, Z., et al. (2022). Soil properties and maize yield improvement with biochar-enriched poultry litter-based fertilizer. Basel) 15 (24), 9003. doi:10.3390/ma15249003
Houben, D., Evrard, L., and Sonnet, P. (2013). Mobility, bioavailability and Ph dependent leaching of cadmium, zinc, and lead in a contaminated soil amended with biochar. Chemosphere 92, 1450–1457. doi:10.1016/j.chemosphere.2013.03.055
Huerta, E. R., Arnienta, M., Dubrovsky, J., and Bernal, J. M. G. (2022). Bioaccumulation of heavy metals and as in maize (Zea mays L) grown close to mine tailings strongly impacts plant development. Ecotoxicology 31, 447–467. doi:10.21203/rs.3.rs-827219/v1
Jia, R., Qu, Z., You, P., and Qu, D. (2018). Effect of biochar on photosynthetic microorganism growth and iron cycling in paddy soil under different phosphate levels. Sci. Total Environ. 612, 223–230. doi:10.1016/j.scitotenv.2017.08.126
Jin, Z., Chen, C., Chen, X., Hopkins, I., Zhang, X., Han, Z., et al. (2019). The crucial factors of soil fertility and rapeseed yield - a five year field trial with biochar addition in upland red soil, China. Sci. Total Environ. 649, 1467–1480. doi:10.1016/j.scitotenv.2018.08.412
Kelly, C. N., Peltz, C. D., Stanton, M., Rutherford, D. W., and Rostad, C. E. (2014). Biochar application to hardrock mine tailings: soil quality, microbial activity, and toxic element sorption. Appl. Geochem 43, 35–48. doi:10.1016/j.apgeochem.2014.02.003
Li, H., Dong, X., da Silva, E, B., de Oliveira, L. M., Chen, Y., and Ma, L. Q. (2017). Mechanisms of metal sorption by biochars: biochar characteristics and modifications. Chemosphere 178, 466–478. doi:10.1016/j.chemosphere.2017.03.072
Li, M. Y., Ly, D., Zhang, Y., and Gao, Y. D. (2013). Influence of pyrolysis temperatures of biochar obtained from the rice straw on cadmium forms. J. Soil Water Conserv. 27 (6), 2511–2519. (in Chinese). doi:10.13870/j.cnki.stbcxb.2013.06.010
Li, S., Chen, J., Islam, E., Wang, Y., Wu, J., Ye, Z., et al. (2016). Cadmium-induced oxidative stress, response of antioxidants and detection of intracellular cadmium in organs of moso bamboo (Phyllostachys pubescens) seedlings. Chemosphere 153, 107–114. doi:10.1016/j.chemosphere.2016.02.062
Liang, J., Yang, Z. X., Tang, I., Zeng, G. M., Yu, M., Li, X. D., et al. (2017). Changes in heavy metal mobility and availability from contaminated wetland soil remediated with combined biochar-compost. Chemosphere 181, 281–288. doi:10.1016/j.chemosphere.2017.04.081
Liu, D., Li, S., Islam, E., Chen, J., Wu, J., Ye, Z., et al. (2015). Lead accumulation and tolerance of moso bamnoo (phyllostachys pubecens) seedlings: applications of phytoremediation. J. Zhejiang Univ. Sci. B 16 (2), 123–130. doi:10.1631/jzus.B1400107
Liu, X. L., Zeng, Z. X., Chen, Q. W., and Zou, H. L. (2014). Effects of biochar and lime additives on non-point load of heavy metals in paddy soil. J. Hydraul. Eng. 45, 682–690. doi:10.13243/j.cnki.slxb.2014.06.007
Liu, Y. X., Wang, Y. Y., Lu, H. H., Linson, L. P., Brar, S. K., and He, L. I. (2011). Lead accumulation and tolerance of moso bamboo (phyllostachys pubescens) seedlings: applications of phytoremediation. J. Zhejiang Univ. Sci. B, 123–130. doi:10.1631/jzus.B1400107
Mahmoud, E., Beshbeshy, T. R., El-Kader, A., El-Shall, B., and Khalafallah, N. (2017). Biochar impacts on physical properties and wheat yield of salt affected soils. Int. J. Sci. Res. Publ. 2 (1), 1–10.
Michal, K., Sarka, S., Jiri, S., Monika, T., Jan, H., Leona, K., et al. (2022). The effect of pyrolysis temperature and the source biomass on the properties of biochar produced for the agronomical applications as the soil conditioner. Mater. (Basel) 15 (24), 8855. doi:10.3390/ma15248855
Mohamed, I., Ali, M., Ahmed, N., Abbas, M. H. H., Abdelsalam, M., Azab, A., et al. (2018). Cow manure-loaded biochar changes Cd fractionation and phytotoxicity potential for wheat in a natural acidic contaminated soil. Ecotox Environ. Safe 2162, 348–353. doi:10.1016/j.ecoenv.2018.06.065
Oliveira, H. (2012). Chromium as an environmental pollutant: insights on induced plant toxicity. J. Bot. 2012, 1–8. doi:10.1155/2012/375843
Page, A. L. (1982). Method of soil analysis, part 2, Chemical and microbiological properties. Second ed. Wisconsin, USA: American Society of Agronomy, Inc. Soil Science Society of America, Inc.
Sarwar, N., Imran, M., Shaheen, M. R., Ishaque, W., Kamran, M. A., Matloob, A., et al. (2017). Phytoremedia-tion strategies for soils contaminated with heavy metals: modifications and future perspectives. Chemosphere 171, 710–721. doi:10.1016/j.chemosphere.2016.12.116
Schat, H., Sharma, S. S., and Vooijs, R. (2010). Heavy metal-induced accumulation of free proline in a metal-tolerant and a nontolerant ecotype of silene vulgaris. Physiol. Plant. 101 (3), 477–482. doi:10.1111/j.1399-3054.1997.tb01026.x
Sousa, R. S. D., Nunes, L., Lima, A. B. D., Melo, W. J. D., Antunes, J. E. L., and Araujo, A. (2018). Chromium accumulation in maize and cowpea after successive applications of composted tannery sludge. Acta Sci. Agron. 40, 35361–35460. doi:10.4025/actasciagron.v40i1.35361
Sun, J., Lu, X. R., Wang, S., Tian, C. K., Chen, G. S., Luo, N. N., et al. (2023). Biochar blended with nitrogen fertilizer promotes maize yield by altering soil enzyme activities and organic carbon content in black soil. Int. J. Environ. Res. Public Health 20 (6), 4939. doi:10.3390/ijerph20064939
Tashiro, C., Takahashi, H., Kanaya, M., Hirakida, I., and Yoshida, R. (1997). Hardening property of cement mortar adding heavy metal compound and solubility of heavy metal from hardened mortar. Cem. Concr. Res. 7 (3), 283–290. doi:10.1016/0008-8846(77)90090-4
Tessier, A., Campbell, P. G. C., and Bisson, M. (1979). Sequential extraction procedure for the speciation of particulate trace metals. Anal. Chem. 51 (7), 844–851. doi:10.1021/ac50043a017
Thangarajan, R., Bolan, N. S., Kunhikrishnan, A., Wijesekara, H., Xu, Y., Tsang, D. C. W., et al. (2018). The potential value of biochar in the mitigation of gaseous emission of nitrogen. Sci. Total Environ. 612, 257–268. doi:10.1016/j.scitotenv.2017.08.242
Wan, Y., Camara, A. Y., Yu, Y., Wang, Q., Guo, T., Zhu, L., et al. (2018). Cadmium dynamics in soil pore water and uptake by rice: influences of soil-applied selenite with different water managements. Environ. Pollut. 240, 523–533. doi:10.1016/j.envpol.2018.04.044
Xia, Y., Luo, H. N., Li, D., Chen, Z., Yang, S., Liu, Z., et al. (2020). Efficient immobilization of toxic heavy metals in multi-contaminated agricultural soils by amino-functionalized hydrochar: performance, plant responses and immobilization mechanisms. Enviro Pollu 261, 114217. doi:10.1016/j.envpol.2020.114217
Xie, Y. X., Dong, C., Chen, Z. Y., Liu, Y. J., Zhang, Y. Y., Gou, P. X., et al. (2021). Successive biochar amendment affected crop yield by regulating soil nitrogen functional microbes in wheat-maize rotation farmland. Environ. Res. 194, 110671. doi:10.1016/j.envres.2020.110671
Xu, P., Sun, C. X., Ye, X. Z., Xiao, W. D., Zhang, Q., and Wang, Q. (2016). The effect of biochar and crop straws on heavy metal bioavailability and plant accumulation in a Cd and Pb polluted soil. Ecotoxicol. Environ. Saf. 132, 94–100. doi:10.1016/j.ecoenv.2016.05.031
Xu, W. J., Mohammad, S., Penttinena, P., Shuzhen, H., and Liu, D. (2019). Bioavailability of heavy metals in contaminated soil as affected by different mass ratios of biochars. Enviro Techno 2019, 1609096. doi:10.1080/21622515.2019.1609096
Xu, Z. Y., Tang, M., Chen, H., Ban, Y. H., and Zhang, H. H. (2012). Microbial community structure in the rhizosphere of Sophora viciifolia grown at a lead and zinc mine of northwest China. Sci. Total Enviro 09 (435–436), 453–464. doi:10.1016/j.scitotenv.2012.07.029
Yang, Q., Li, Z., Lu, X. N., Duan, Q. N., Huang, L., and Bi, J. (2018). A review of soil heavy metal pollution from industrial and agricultural regions in China: pollution and risk assessment. Sci. Total Envir 642, 690–700. doi:10.1016/j.scitotenv.2018.06.068
Yang, W., Feng, G., Jia, Y. L., Yang, Y. X., Gao, X. Y., Gao, L. H., et al. (2022). Impact of single biochar application on maize growth and water-fertilizer productivity under different irrigation regimes. Front. Plant Sci. 13, 1006827. doi:10.3389/fpls.2022.1006827
Yang, X., Liu, J., McGrouther, K., Huang, H., Lu, K., Guo, X., et al. (2016). Effect of biochar on the extractability of heavy metals (Cd, Cu, Pb, and Zn) and enzyme activity in soil. Environ. Sci. Pollut. Res. 23, 974–984. doi:10.1007/s11356-015-4233-0
Yin, D. X., Wang, X., Chen, C., Peng, B., Tang, C. Y., and Li, H. L. (2016). Varying effect of biochar on Cd, Pb and as mobility in a multi-metal contaminated paddy soil. Chemosphere 152, 196–206. doi:10.1016/j.chemosphere.2016.01.044
Younis, U., Athar, M., Malik, S. A., Raza, S., and Mahmood, S. (2015). Biochar impact on physiological and biochemical attributes of spinach (Spinacia oleracea L) in nickel contaminated soil. Glob. J. Environ. Sci. Manage 1 (3), 245–254. doi:10.7508/gjesm.2015.03.007
Yu, H., Zou, W., Chen, J., Chen, H., Yu, Z., Huang, J., et al. (2019). Biochar amendment improves crop production in problem soils: a review. J. Environ. Manag. 232, 8–21. doi:10.1016/j.jenvman.2018.10.117
Zha, Y., Zhao, B., and Niu, T. X. (2022). Bamboo biochar and zinc oxide nanoparticles improved the growth of maize (Zea mays L) and decreased cadmium uptake in Cd contaminated soil. Agriculture 12 (9), 1507doi. doi:10.3390/agriculture12091507
Zha, Y., Zhao, L., Niu, T. X., Yue, E. K., Wang, X. B., and Shi, J. (2023). Multi-target element-based screening of maize varieties with low accumulation of heavy metals (HMs) and metalloids: uptake, transport, and health risks. Agriculture 13, 1123. doi:10.3390/agriculture13061123
Zhang, A. F., Cheng, G., Hussain, Q., Zhang, M., Feng, H., Dyck, M., et al. (2017). Contrasting effects of straw and straw-derived biochar application on net global warming potential in the Loess Plateau of China. Field Crop Res. 205, 45–54. doi:10.1016/j.fcr.2017.02.006
Zhang, A., Li, X., Xing, J., and Xu, G. (2020). Adsorption of potentially toxic elements in water by modified biochar: a review. J. Envir Chemi Engin 8 (4), 104196. doi:10.1016/j.jece.2020.104196
Zhang, R. H., Li, Z. G., Liu, X. D., Wang, B. C., Zhou, G. L., Huang, X. X., et al. (2017). Immobilization and bioavailability of heavy metals in greenhouse soils amended with rice straw-derived biochar. Ecol. Eng. 98, 183–188. doi:10.1016/j.ecoleng.2016.10.057
Zhang, X. K., He, L. Z., Sarmah, A. K., Lin, K. D., Liu, Y. K., Li, J. W., et al. (2014). Retention and release of diethyl phthalate in biochar-amended vegetable garden soils. J. Soil. Sediment. 14, 1790–1799. doi:10.1007/s11368-014-0929-x
Zhao, F. J., Ma, Y. B., Zhu, Y. G., Tang, Z., and McGrath, S. P. (2015). Soil contamination in China: current status and mitigation strategies. Environ. Sci. Technol. 49, 750–759. doi:10.1021/es5047099
Zheng, R. L., Cai, C., Liang, J. H., Huang, Q., Chen, Z., Huang, Y. Z., et al. (2012). The effects of biochars from rice residue on the formation of iron plaque and the accumulation of Cd, Zn, Pb, as in rice (Oryza sativa L) seedlings. Chemosphere 89, 856–862. doi:10.1016/j.chemosphere.2012.05.008
Keywords: biochar, cadmium, remediation, maize, soil properties
Citation: Zha Y, Zhao L, Wei J, Niu T, Yue E, Wang X, Chen Y, Shi J and Zhou T (2023) Effect of the application of peanut shell, bamboo, and maize straw biochars on the bioavailability of Cd and growth of maize in Cd-contaminated soil. Front. Environ. Sci. 11:1240633. doi: 10.3389/fenvs.2023.1240633
Received: 15 June 2023; Accepted: 08 August 2023;
Published: 05 September 2023.
Edited by:
Gaurav Pant, Graphic Era (Deemed to be University), IndiaReviewed by:
Vivek Verma, Thapar Institute of Engineering and Technology, IndiaSumit Pal, Indian Agricultural Research Institute (ICAR), India
Copyright © 2023 Zha, Zhao, Wei, Niu, Yue, Wang, Chen, Shi and Zhou. This is an open-access article distributed under the terms of the Creative Commons Attribution License (CC BY). The use, distribution or reproduction in other forums is permitted, provided the original author(s) and the copyright owner(s) are credited and that the original publication in this journal is cited, in accordance with accepted academic practice. No use, distribution or reproduction is permitted which does not comply with these terms.
*Correspondence: Tiefeng Zhou, ZHVuMDgxNnFpQDE2My5jb20=