- Technological University of Uruguay (UTEC), Water Engineering and Sustainable Development, ITR-CS, Durazno, Uruguay
Industrial sustainability is a process that has been gaining space in recent years. The use of microalgae for wastewater treatment could solve some environmental challenges, optimize resources, and generate value-added products in agriculture, biofuel, food, and feed. The use of High Rate Algal Pond (HRAP) presents economic benefits, by treating contaminated effluents and taking advantage of the microalgae biomass generated. The microalgae growth in wastewater can be limited by lighting energy or the easily assimilable carbon source, due to the high load of nutrients and organic matter present in these effluents. In the same way, other physical, chemical, and biological parameters must be controlled to guarantee that the process reaches its maximum performance. The technology applied with microalgae for the waste industrial treatment seeks to generate sustainable, economical, and efficient processes that guarantee the discharge of water under standard parameters that allow for preserving the environment, the quality of life of citizens and generating inputs such as biofertilizers that allow avoiding crucial problems such as NPK ratio imbalance, soil hardening, salinization, nutrient depletion, groundwater contamination and food for animal consumption that allows generating nutritional alternatives. In this way, the treatment of wastewater with microalgae is an opportunity to solve sanitary and environmental problems under a sustainable approach to obtain inputs, although some challenges must be solved for scale production. This document intends to show outstanding aspects related to effluent treatment, water reuse, and sustainable production of agricultural inputs through the use of microalgae.
1 Introduction
The accelerated population growth, agricultural intensification, industrialization, and greater production of agro-industrial and urban wastewater, have led to the exploitation of resources, to produce a greater number of foods, use more energy, consume more water, and emit pollutants into bodies of water, atmosphere and soil, proving to be one of the most critical environmental problems foreseen by humanity. Waterways can be affected by the discharge of pollutants and thus compromise the quality of the water, affecting the global supply of the resource (Obaideen et al., 2022). The lack of adequate wastewater treatment, coupled with poor water resource management, leads to a shortage of clean water supply. This has generated progress in recent decades in various technological processes that seek to recover resources from wastewater (Petrik et al., 2022).
The application of biological processes has been gaining space in recent years, focusing on the feasibility of coupling and taking advantage of wastewater treatment processes in systems that can evolve and promote processes adapted to the circular economy (Kundu et al., 2022). Environmental protection is a necessity, so the implementation of pollution control systems greatly influenced the global economic impact. In this way, establishing methodologies that balance the environment, economy and society vectors will be essential in treatment plants in the medium term. Wastewater contains several compounds, which will depend on the nature of the industrial, agricultural, or domestic activity involved, such as a high content of organic matter, inorganic impurities, macro and micronutrients, heavy metals, emerging pollutants, among others (Koul et al., 2022). Excessive discharge of nutrients such as nitrogen and phosphorus in bodies of water will generate eutrophication, altering the balance in the system that leads to a decrease in oxygen, death of aquatic organisms, generation of by-products and solid waste, emissions of compounds and odors into the atmosphere, reduction of biodiversity, and algal blooms. This can lead to bad taste and odor of the water, which threatens the safety of drinking water and aquatic foods, which stimulate the release of greenhouse gases, and degrade the social and cultural values of these water bodies (El-Sheekh et al., 2021).
Wastewater treatment can be at three levels where physical, chemical, or biological processes are implemented (Garrido-Cardenas et al., 2020). Primary level, removing settleable solids that can cause operational problems in subsequent steps. Secondary level, where a physical and/or biological process interacts consuming dissolved organic matter and oxidizing the main nutrients to nitrate and orthophosphate. Tertiary, consists of an advanced treatment that eliminates nitrates, phosphates, and trace organic compounds (Zhang et al., 2021). Normally, nitrogen is removed without recycling, converting it into N2 that will pass into the atmosphere (Qin et al., 2023). Phosphorus is mainly precipitated by adding cations such as calcium, aluminum, and iron, resulting in an expensive process (Christensen et al., 2022). As an alternative for the treatment, reduction, and/or elimination of nitrogen and phosphorus, the use of microalgae arises which, being a natural process, may seem simple and involve a series of complex metabolic processes that vary based on the conditions of the crops and the composition of the effluent to be treated (Dalvi et al., 2021). Biological treatment systems based on microalgae allow the reduction of nutrients such as potassium, nitrogen, phosphorus, and CO2, having a potential application to be used as an alternative in the treatment of effluents for the reduction of organic matter, COD, BOD, and elimination of nitrogen and phosphorus, as well as to reduce fecal pathogens and bacteria, removal of emerging contaminants and heavy metals (Amaro et al., 2023a). Microalgae have been widely applied in wastewater treatment, presenting good tolerance to the compounds present, which has allowed, in recent years, microalgae-based technology to attract attention for the treatment of domestic, municipal, industrial, and agro-industrial (Table 1) (Sepúlveda-Muñoz et al., 2023).
Considering the chemical nature of a large part of the effluents, the use of wastewater to cultivate microalgae could solve some of the main challenges facing modern society, such as the problems of demand for freshwater, the high cost of nutrients used for cultivation media and the need to treat contaminants to reduce or remove them significantly, providing a system that acts in the reuse and use of water resources, generating the return of cleaner water to bodies of water, productive systems and acting in reducing greenhouse gases. This type of technology, in addition to presenting environmental advantages, by reducing these polluting elements, provides the possibility of generating a better use of phosphorus, which is a non-renewable resource and which, when accumulated by microalgae, can be used as bioproducts with added value in agricultural processes, for example, the production of biofertilizers, biostimulants, food for animal feed and human consumption, chemical and pharmacological compounds, among others (Figure 1) (Liu and Hong, 2021; Osorio-Reyes et al., 2023).
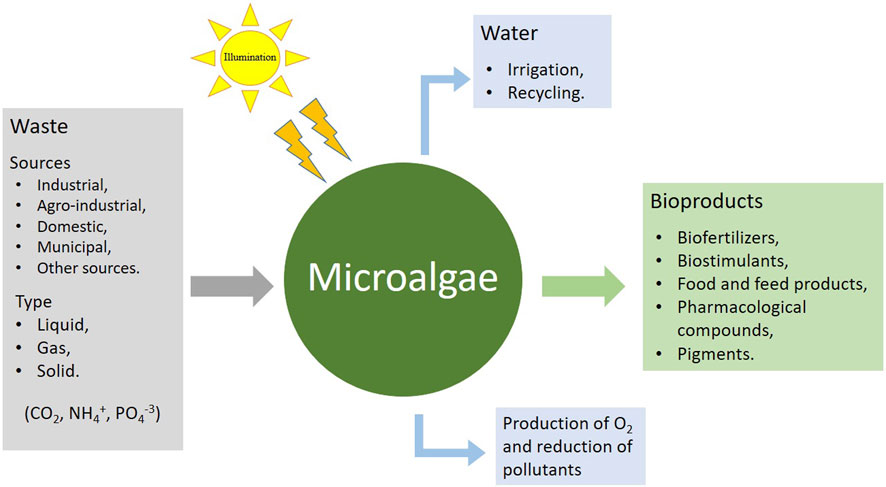
FIGURE 1. Technological scheme based on microalgae https://www.frontiersin.org/my-frontiers/overview.
The use of High Rate Algal Ponds (HRAP) presents economic benefits, by treating contaminated effluents and taking advantage of the microalgae biomass generated. The growth of microalgae in crops made up of wastewater can be limited by lighting energy or the easily assimilable carbon source, due to the high load of nutrients and organic matter present in these effluents. Similarly, other physical, chemical, and biological parameters must be controlled to guarantee that the process reaches its maximum performance (Saúco et al., 2021). The technology applied with microalgae for the treatment of industrial waste seeks to generate sustainable, economic, and efficient processes that guarantee the discharge of water under standard parameters that allow for preserving the environment, the quality of life of citizens and generating useable bioproducts, and thus avoid crucial issues such as NPK ratio imbalance, soil hardening, salinization, nutrient depletion, and groundwater contamination. In light of these challenges, the objective of this review is to show outstanding aspects, future perspective, and the challenges of aspects related to effluent treatment, and sustainable production of compounds with added value, showing the impact from the point of view of environmental remediation through technologies that involve the use of microalgae.
2 Microalgae strains and their metabolism
Despite the great diversity of microalgae in the world, estimated at three hundred thousand, only thirty thousand have been identified and of these approximately fifty species are used in biotechnological research, and ten for the commercial production of food compounds and derivatives (Richmond and Qiang, 2013; Cobos et al., 2020). Although biotechnological research applied to microalgae has been increasing, new strains of microalgae destined for the production of compounds of commercial interest have not been defined (Balasubramaniam et al., 2021). The search and collection of microalgae is relatively easy, although finding microalgae of biotechnological interest is more complicated. The strains of microalgae must present characteristics applicable to biotechnological processes that are attractive to entrepreneurs. Thus, strains that show high growth kinetics, with a high biomass yield, that are grown in wastewater or natural light and temperature conditions, that produce interesting and easily separable metabolites, would be desirable characteristics for the optimization of production processes (Zhuang et al., 2023).
Therefore, the selection of microalgae strains for the production of a commercial biproduct is of paramount importance and deserves to be considered. In the natural habitat, the species are well adapted to the local environmental conditions and their usefulness contributes to a more successful cultivation than non-native species. This is partly because the adaptability to a certain microenvironment makes the metabolism respond faster in similar conditions, this gives advantages to make them candidates in bioremediation processes (Deviram et al., 2020).
The effectiveness of microalgae use for bioremediation depends on the specific contaminants, the characteristics of the native strains, and the environmental conditions of the site. Additionally, regulatory and safety considerations must be taken into account when implementing bioremediation strategies. Overall, native microalgae strains can be valuable tools in the effort to clean up polluted environments and restore ecosystem health (Raklami et al., 2022). The use of native microalgae strains reduces the risk of invasive algae species and other microbial pathogens (Pereira et al., 2021). The cultivation of microalgae in wastewater is profitable in the production of microalgae biomass and helps in the elimination of nutrients (Huang et al., 2022).
The use of microalgae to purify pollutants in the agro-industrial and municipal sectors has been studied successfully (Vadiveloo et al., 2021; Koutra et al., 2021; Medeiros et al., 2020). For wastewater treatment, the strain must have a significant capacity for nutrient removal as well as be able to tolerate high levels of contaminants such as ammonia. Although some species, to a greater or lesser degree, can absorb polluting nutrients, it is essential to establish what the objective is since we can have strains with high effectiveness for the treatment of wastewater, but that are not interesting for obtaining products with value added. For this reason, it is important to determine yields and productivity in advance to successfully combine wastewater treatment and the production of a microalgae bioproduct of commercial interest (Torres-Franco et al., 2021).
Microalgae have a mainly photoautotrophic metabolism, obtaining energy for ATP synthesis from light and assimilating dissolved inorganic carbon (CO2 and CO32−), releasing oxygen in the process (Li et al., 2022). During this photosynthetic process, light reactions occur where photon energy is converted into chemical energy (ATP and NADP), and dark reactions where CO2 is reduced to carbohydrates by accumulated chemical energy (Wu et al., 2023). However, some microalgae can be forced to have heterotrophic or mixotrophic metabolism (Patel et al., 2021).
In addition to CO2, nitrogen, and phosphorus are essential for microalgal biosynthesis, with NH4+ being a bioavailable molecule for nitrogen and to a lesser extent NO3−, and for phosphorus dissolved orthophosphate (PO43−), N being essential for protein synthesis, and P for phospholipid and nucleic acid synthesis. The composition of the microalgae biomass is characterized by having a composition of C106H181O45N16P. These elements play critical roles in various biochemical processes within microalgae cells and act by forming macromolecules that are for microalgae metabolism and growth because they are involved in the synthesis of biomolecules, energy transfer, enzyme activation, and maintaining cellular structures and cell viability. The availability and balance of these macroelements in the growth medium can significantly impact the growth rate, biomass production, and overall health of microalgae cultures. This suggests that the adequate rate of available assimilable nitrogen, phosphorus, and carbon based on the C/N and N/P ratio would be 30 and 16 respectively, although variations have been reported (Bhatti et al., 2023).
3 Chemical alterations in the environment
Microalgae are highly sensitive to changes in their environment, including pH levels and nutrient competition. The pH changes and nutrient competition can impact microalgae in a different form. Extremely low pH levels can be detrimental to most microalgae species. Acidic conditions can disrupt the cell membranes and alter the proton balance within the cells, affecting photosynthesis and cellular metabolism. Some acid-tolerant species may thrive in these conditions, but overall, acidity can limit the growth and productivity of microalgae (Abiusi et al., 2022). On the other hand, high pH levels can also have negative effects on microalgae. Alkaline conditions can inhibit the uptake of essential nutrients like phosphorus and iron, leading to nutrient deficiencies (Yaakob et al., 2021). Additionally, high pH can disrupt the balance of CO2 in the water, which can hinder photosynthesis (Li et al., 2023). Different species of microalgae have varying tolerances to pH, so the impact of alkaline conditions may vary. Most microalgae species prefer a neutral to slightly alkaline pH range (around 7–9), where nutrient availability and photosynthesis are optimized. Maintaining pH within this range is crucial for the healthy growth of microalgae in controlled cultivation systems (Yu et al., 2022).
Competition for nutrients plays a crucial role; nitrogen (in the form of nitrates, nitrites, or ammonia) and phosphorus are two essential nutrients for microalgae growth. These nutrients are often found in limited supply in aquatic environments. When multiple microalgae species are present, they compete for these nutrients. Some species may outcompete others under specific conditions, leading to shifts in the microalgal community composition (Olofsson et al., 2019). Iron is another essential micronutrient for microalgae, especially for the production of chlorophyll. In iron-limited environments, microalgae may engage in intense competition for the available iron, with certain species having mechanisms to better scavenge and utilize this nutrient (Rana and Prajapati, 2021). Light and CO2 availability also play a significant role in nutrient competition among microalgae. Some species are better adapted to low light conditions, while others are more efficient at utilizing CO2 (Shekh et al., 2022).
Overall, the outcome of nutrient competition among microalgae is influenced by the specific nutrient concentrations, pH, light availability, and the relative competitive abilities of the different species present in a given ecosystem. Understanding these dynamics is essential for optimizing microalgae cultivation for various applications, including biofuel production, wastewater treatment, and aquaculture feed.
4 Role of microalgae in the biological treatment of wastewater
The use of microalgae in wastewater treatment has gained space in pilot and laboratory scale processes, being the most used for this purpose species of chlorophyceae, dinoflagellates, and cyanobacteria. Microalgae are used in wastewater treatment not only for their ability to significantly remove nitrogen and phosphorus but also for their efficiency in removing pathogenic organisms, due to the development of extreme pH and substances with antibacterial that can be excreted by them (Amaro et al., 2023b).
Bioremediation, bioaugmentation, and improvements in the process such as bioventilation and biostimulation, have shown to be very efficient in the purification of wastewater, eliminating organic and inorganic pollutants, such as nitrogen, phosphorus, and heavy metals, among others (Dubey et al., 2023). In addition, microalgae can biodegrade xenobiotic compounds, toxic synthetic products, not generated by natural biosynthetic processes, such as halocarbons, nitroaromatics, polychlorinated biphenyls, dioxins, alkylbenzyl sulfonates, petroleum hydrocarbons, and pesticides (Verma et al., 2019; Touliabah et al., 2022).
Bioremediation with microalgae has shown efficient, promising, and sustainable solutions in conventional effluent treatment systems, in turn providing bioproducts of industrial interest, and with added value, in addition to metabolizing potentially polluting compounds. Providing benefits both in the purification and in the collection and obtaining of microalgal biomass to produce bio-inputs, nutraceuticals, pigments, supplements and food or feed rich in lipids, proteins, polysaccharides, phenolic compounds, carotenoids, among others, which can be used as a source of ingredients for functional products in the food industry, which combined with microencapsulation processes provides greater stability (Kalra et al., 2021; Sadvakasova et al., 2023).
This type of procedure has generated a high purification of nutrients allowing them to reach low levels of discharge, considering that microalgae directly use N and P for their metabolic processes. In addition, the use of microalgae/bacteria consortia is increasing since during the absorption of CO2 oxygen is released which allows the presence of heterotrophic bacteria that help in the degradation of organic carbon (Flores-Salgado et al., 2021; Sátiro et al., 2022).
For the development and optimization in the treatment of effluents with microalgae, some drawbacks must be corrected such as the recovery of metabolites and biomass, design and implementation of the appropriate photobioreactor, lighting intensity, quality, and C/N—N/P ratio of the effluent, temperature, pH, and adequate agitation supply, among others (Deprá et al., 2019; Hu et al., 2020; Sánchez-Zurano et al., 2021; Liu et al., 2022; Song, et al., 2022).
The wastewater can be very varied depending on the origin, for this reason, the kinetics and yields in terms of nutrient consumption vary and must be optimized for each type of specific strain. In systems with microalgae/bacteria consortia, light plays a crucial role in the assimilation of NH4+, organic matter, and PO43−, with greater efficiency in reducing total nitrogen and COD at low irradiation rates. When the light/dark cycle alternates, initial sludge/microalgae sedimentation improves, and there is less oxygen consumption, and nutrient and organic matter removal (Li et al., 2021).
The optimum temperature for the efficiency of the process is approximately 22°C and discrete increases in temperature lead to proliferation of nitrifying bacteria and nitrification above microalgae assimilation (González-Camejo et al., 2020). On the other hand, high pH values affect microalgae metabolism, decreasing it (Zhang et al., 2019; Salvatore et al., 2023). The proportions and type of nutrients present in the effluent must be evaluated for each specific strain, being able to mix with other effluents or water to find a balance and better conditions for the microalgae to assimilate the components present (Umetani et al., 2023).
In addition, the use of microalgae in effluent sanitation is crucial to reducing or eliminating pathogens through mechanisms such as pH changes, nutrient competition, fixation, sedimentation, presence of biocontrol agents (Dar et al., 2019). During photosynthesis, an increase in pH and oxygen concentration occurs, which affects the growth of pathogens such as total and fecal coliforms, Salmonella, Clostridium, and Enterococcus (Delanka-Pedige et al., 2019; Cho et al., 2022; Malcheva et al., 2022). Many produced microalgae metabolites have an antimicrobial effect, being more marked in gram-positive bacteria than in gram-negative bacteria, a difference that is linked to the changes evidenced in the type of cell wall structure (Ramírez-Mérida et al., 2015a; Polat et al., 2023).
Worldwide, there are some pilot-scale systems for the treatment of effluents that incorporate microalgae in their process. The wastewater treatment system, Life Algaecan, was installed in a vegetable and fruit processing company in Spain. This system proposes a sustainable treatment model for effluents generated during processing that combines the profitable cultivation of heterotrophic microalgae, capable of purifying wastewater, with its subsequent collection. With this system, approximately 2 m3 per day has been treated, obtaining a high-quality final effluent, which is reused and discharged into water courses, thus solving the problem of pollution that these effluents generate, since the quality The end of the effluent obtained allows it to be reused as irrigation water or for cleaning equipment and facilities. In turn, a product of commercial interest is obtained for the production of biofertilizers due to the good NPK content present in the biomass (Martín-Marroquín et al., 2023).
On the other hand, Ávila et al. (2022), when evaluating the integration of a system based on microalgae for the treatment of tertiary wastewater in an industrial WWTP, showed that the microalgae efficiently removed the nutrients from the secondary effluent. In turn, the co-digestion of residual activated sludge and the collected microalgae was 70% above the monodigestion with residual activated sludge and its application as a biofertilizer turned out to be effective.
5 High Rate Algal Ponds (HRAP)
This technology began in small rural communities, due to its simplicity in operation and its low cost, compared to activated sludge technology. These ponds allow the development of microalgae biomass as it takes advantage of all the nutrients present in the residual water, as well as the elimination of other contaminants. Biomass can be marketed to obtain various products of industrial interest. This helps to pay maintenance and construction expenses whose main benefit is an effective treatment of wastewater, not only of domestic origin but also at an industrial level (Bhatt et al., 2022). These systems take advantage of the capacity of microalgae to fix carbon dioxide, remove nitrogen and phosphorus, develop antibiotic substances to eliminate pathogens, and retain heavy metals (Carvalho et al., 2021; Xiong et al., 2021; Zhao et al., 2023).
The HRAPs present high productivity of microalgal biomass and, compared to the reference lagoons, they have a high capacity for wastewater treatment because their cell retention time is greater than the hydraulic retention time. Most of these systems measure approximately 1 m deep and 3 m long. Their shape is open and circular, which makes them dependent on external conditions, such as the weather. The mixing of the effluents is carried out continuously and completely. In the case of the hydraulic retention time it is 2–8 days, while the cell retention time is 4–13 days (Jinda et al., 2020). The operation of the HRAP is based mainly on the oxidation of organic matter through photosynthetic oxygenation provided by the microalgae of the system, which take advantage of solar energy and organic matter. The oxygen released during photosynthesis is used by the rest of the microorganisms that also collaborate in the mineralization of nutrients such as nitrogen and phosphorus. This system demonstrates the ability of microalgae to interact symbiotically with the microbial flora, jointly reducing water pollutants (Liu et al., 2023).
HRAP technology is used to treat effluents that have already undergone previous treatment, to eliminate polluting substances that persist with conventional methods. This is a consequence of the extraordinary capacity of microalgae to assimilate significant amounts of dissolved organic and inorganic nutrients, and they not only assimilate nutrients but also pollutants of different kinds (Gutiérrez et al., 2016). The use of HRAP has been reported for the treatment of effluents from pig farms, where a COD removal greater than 90% was achieved (Aguirre et al., 2011). This system has also been used for the treatment of urban wastewater in Rabat, Morocco, evidencing a high efficiency in the removal of COD, nitrogen, and phosphorus, of 80, 85, and 63% respectively. In addition, the elimination of 1.23 logarithmic units of fecal coliforms and the complete elimination of helminth eggs could be observed (El Hamouri, 2009).
The HRAP system not only removes contaminants through the nutrient assimilation mechanism but also through the volatilization of ammonium generated by large increases in pH in the water, a consequence of the intense photosynthetic activity during the day. On the other hand, phosphorus precipitation is achieved by binding with some polyvalent cations such as iron, calcium, and magnesium (Sukačová and Červený, 2017). In various inorganic nutrient removal processes with microalgae, phosphorus usually has the lowest yield. However, Xu et al. (2014) added a Chlorella sp-based membrane bioreactor to an HRAP system, achieving a removal of up to 83.1% of the phosphorus present in the water. Subsequently, the microalgae biomass can be removed and with it the phosphorus from the residual water. This finding allows the optimization of water treatment systems, increasing the level of phosphorus removal, a fundamental element for the eutrophication of water bodies, and consequently the loss of diversity.
Another use that the HRAP system has is its application as a secondary system for the treatment of leachate from sanitary landfills. Despite the reported success, a previous treatment is suggested for high leachate concentrations, a consequence of the fact that the medium can be toxic for the microalgal biomass. For the development of this system, Chlorella vulgaris, Scenedesmus quadricauda, Euglena gracilis, Ankistrodesmus convolutus, and Chlorococcum oviforme were used. It was observed that the microalgae achieved a good reduction of COD 91.0%, ammoniacal nitrogen 99.9%, and phosphates 86.0%, also achieving the accumulation of heavy metals such as zinc, arsenic, cadmium, copper, chromium, and lead. However, the high bioaccumulation of metals in the microalga prevents its biomass from being used as food or in biofertilizers, despite this it is possible to use it in the manufacture of biofuels, or simply limit its use to the recovery of these metals by desorption (Mustafa et al., 2012). The mechanism by which microalgae eliminate heavy metals consists of the production of a peptide capable of binding metals, forming organometallic compounds that accumulate in the vacuole to control the concentration of heavy metal ions in the cytoplasm, helping to prevent or neutralize the potentially toxic effects they possess (Priya et al., 2014).
HRAP technology has been developed mainly in countries with seasonal variations. When applying these systems it is essential to take into account environmental variables such as temperature, available sunlight, and rainfall, among others. This is because they are open systems whose ecological dynamics are affected by the environment. Some simple modifications in pond operation, such as series-operated systems, generate higher microalgal biomass yields and better wastewater quality without higher operating capital costs (Sutherland et al., 2020). Similarly, it has been shown that the microalgae commonly used for the development of these systems correspond to Scenedesmus sp., Microactinium sp., Ankistrodesmus sp., Euglena sp., Chlamydomonas sp., Desmodesmus sp., Chlorella sp., Dictyosphaerium sp. and Pediastrum sp. (Cho et al., 2017).
In traditional domestic wastewater, the ratios of carbon, nitrogen, and phosphorous (C:N:P) are 20:8:1, while the C:N:P ratios for microalgae are 50:8:1. The additional carbon necessary for microalgae photosynthesis is supplied from free carbon dioxide, which is the main limiting factor in HRAPs. Although bacteria in ponds generate a high concentration of CO2, a high photosynthetic rate can create a CO2 deficit. It is estimated that 30% of the total carbon required by microalgae can be supplied by incorporating CO2 into these ponds (Craggs et al., 2013).
6 Microalgae products
The particular characteristics of growth, yield, and production of metabolites of interest generated by microalgae make it an attractive organism for use in various sectors of the food, pharmaceutical, aquaculture, cosmetic, and environmental industries, etc. (Mobin et al., 2019). However, the studies that seek to integrate productive processes with sustainability, where the use of industrial effluents is a niche of interest, are limited to demonstrating whether the final product is suitable for use from a sanitary point of view. Starting from this, the use of what, in principle, were contaminants, for the production of biostimulants, biofertilizers and other by-products such as pigments allows the establishment of the concept of microalgal biorefinery using agroindustrial waste, which could mean important opportunities for the sustainable use of resources.
6.1 Biofertilizers
The use of biofertilizers in crops becomes an important variable that acts on the physiology of the plant in different ways: improving the vigor, yield, and quality of the crops. Taking advantage of their biological nature, the action of not emitting residues, and being safe for application, they are increasingly used in a variety of crops. Although there is a diversity of microorganisms with fertilizing potential, not all provide optimal results. Microalgae are one of the most promising sources for developing this type of stimulating products, by solubilizing inorganic phosphorus and, in the case of formulations composed of cyanobacteria, fixing atmospheric nitrogen.
They can be applied directly to the soil, to the seeds or to plant surfaces (Dineshkumar et al., 2018). Microalgae-based biofertilizers, due to their phytochemical composition, provide the availability of macro and micronutrients such as Cu, Mn, Fe, Ca, Mg, polysaccharides, compounds with antimicrobial action, amino acids, phytohormones (auxins, gibberellins, and cytokines), which when applied directly to the vegetable crop or as composting, they have a biostimulating effect on crops (Gonçalves, 2021).
Biofertilizers made from microalgae help to improve the use of nitrogen, phosphorus, potassium and other soil elements, increase crop resistance to stress (Guo et al., 2020), sequester atmospheric carbon dioxide (Mutum et al., 2022), improve soil porosity (Nayak et al., 2019), increase water retention, and produce auxins, gibberellins, vitamins and amino acids (Muniswami et al., 2021), improve the quality and yield of agricultural products, significantly inhibit the toxicity of plant pathogens in the soil (Guo et al., 2020). Microalgae in soil develop a photosynthetic biofilm that together with eukaryotic and prokaryotic organisms enhances the degradation of organic compounds (Marks et al., 2017).
The use of biofertilizers worldwide is on the rise, bringing several advantages such as environmental, marking, and cost, in turn it can give a better use of phosphorus considering that it can be accumulated in its cellular structure (Sampathkumar et al., 2019).
6.2 Biogas
The sludge generated during wastewater treatment can be transformed into methane by performing anaerobic digestion (Ávila et al. (2022)). Microalgae have great potential for the production of biofuels since they produce a large amount of carbohydrate-rich biomass that serves as raw material to generate bioethanol, lipids that after transesterification provide biodiesel, hydrogen by electrocoagulation, and methane by anaerobic digestion. The costs of drying and extraction of carbohydrates and lipids are relatively high, which makes large-scale production unfeasible (Oliveira et al., 2021).
The anaerobic digestion of microalgae converts its lipids and other organic compounds into biogas. Low and variable yields in methane production have been reported among different types of microalgae species. This is due to the variable cell biochemical composition of lipids, carbohydrates, proteins, and wall structure as it has a lower cellulose content, as well as high concentrations of intracellular N that inhibit ammonia (Qian et al., 2022; Tawfik et al., 2022).
Yields in methane production can be obtained by improving lipid accumulation, varying the nitrogen content in the substrate, or by changes in temperature (Llamas et al., 2020). Thus, it is evident that under limited nitrogen concentrations (1.5 g L−1 to 0.375 g L−1 NaNO3) Chlorolobion sp. and Chlorella sp reached a higher lipid yield of 227.84 and 151.14 mg L−1 d−1 respectively (Arguelles and Martinez-Goss, 2021), and higher lipid content of up to 62% under limited nitrogen conditions when compared to the sufficient content in Chlorella (Feng et al., 2020). Temperature affects key enzymatic activity to produce changes in its intracellular content, thus increases in temperature from 25°C to 30°C decrease lipid content to less than half in Nannochloropsis oculata strains (Converti et al., 2009).
However, care must be taken since excess lipids can cause inhibition of anaerobic microorganisms due to the accumulation of long-chain fatty acids (Qiu et al., 2020). A pretreatment of the microalgal biomass will help to break the cell wall to make the lipids more available for anaerobic digestion, temperature, ultrasound, and enzymatic pretreatment can be used (de Oliveira et al., 2022).
7 Production of microalgae pigment from agro-industrial waste
The main advantages of microalgae wastewater treatment are that no additional pollution is generated since the biomass is harvested and efficient recycling of nutrients is possible. Agroindustrial residues have low chemical risks, are potentially available on a large scale, and can generate biomass, rich in natural pigments and other biomolecules (Durvasula et al., 2015).
Microalgae are a source of various bioactive molecules. These organisms have metabolic pathways capable of generating primary and secondary metabolites that can be used for use in areas of the chemical, food, pharmaceutical, agricultural, and environmental industries (Zhu, 2015). The production of pigments through efficient and productive technologies has become a key point for economically competitive commercialization.
Among the three groups of pigments found in microalgae, we have carotenoids, phycobilins, and chlorophyll. Microalgae carotenoids have been used for commercial purposes due to their coloring properties and high antioxidant power associated with the prevention of cancer, arteriosclerosis, aging, and degenerative diseases (Raposo et al., 2013).
The use of β-carotene and lutein has been of great importance in agro-industry since it has been used with great advantages for poultry feeding and feeding in aquaculture (Ritu et al., 2023). Among the advantages of producing carotenoids of microalgal origin, is the fact that it is a natural product, unlike synthetic β-carotene, in addition, production by microalgae allows the formation of trans and cis isomers of carotenoids, which provides a wide advantage for processes such as antioxidants (Wang et al., 2022; Chini Zittelli et al., 2023).
Dunaliella salina was the first microalgae to be marketed as a high-value chemical source (approximately USD 300,00–1.500,00 kg−1), showing certain advantages such as the ability to grow in highly saline media, which avoids risks of contamination by other microorganisms and high β-carotene contents (Borowitzka, 2013).
Carotenoids are intracellular products, so their productivity depends on the amount of biomass achieved, and factors related to the culture medium, type of strain, and use of a suitable photobioreactor (Ramírez-Mérida et al., 2015b; 2015c). Biomass is the main bioproduct for the microalgae pigment biorefinery, in this sense, many initiatives have been investigated to produce future pigments from microalgae, especially in an experimental stage. Table 2 shows some pigments generated in the culture of microalgae.
Production processes based on heterotrophic cultures have a potentially low production cost, compared to the conventional production of pigments by microalgae using phototrophic culture (Morales-Sánchez et al., 2017), since the raw material used to feed the culture is negligible. By comparing the production of phycobiliproteins and chlorophyll-a of Aphanothece microscopica Nägeli cultivated in autotrophic and heterotrophic systems using effluents from the dairy industry. The highest pigment concentrations were observed in the logarithmic phase: highest concentrations of chlorophyll-a in autotrophic cultures and predominance of allophycocyanin and phycoerythrin in heterotrophic cultures.
The results showed the effect of the variables under study for the production of pigments, in the logarithmic growth phase, which indicates the possibility of producing approximately 3,185 tons/year of biomass in heterotrophic systems of Aphanothece microscopica Nägeli, for 3,127 kg/year chlorophyll, 232,825 kg/year phycocyanin, 47,775 kg/year allophycocyanin and 7,008 kg/year phycoerythrin, when effluent from the dairy industry is used as a culture medium (Streit et al., 2017).
Therefore, the potential to produce pigments from microalgae cultures using agro-industrial residues can be considered as an emerging platform for obtaining these products. It is known that Spirulina species can accumulate up to 0.8%–1.0% w/w of β-carotene. Dunaliella salina and D. bardawil species are among the main carriers of carotenoids, providing yields of 400 mg β-carotene/m2 under ideal conditions (Flassig et al., 2015).
Similarly, research on strains little studied for this purpose has shown promising results, thus Rodrigues et al. (2014) grew Phormidium autumnale strains in heterotrophic culture using slaughterhouse wastewater as the culture medium. A total of (70,22 μg g−1) trans-β-carotene, (26,25 μg g−1) trans-zeaxanthin, (21,92 μg g−1) trans-lutein, (19,87 μg g−1) trans-echinenone and (15,70 μg g−1) cis-echinenone were separated from the microalgal biomass, showing the possibility of obtaining 107.902,5 kg/year of carotenoid on an industrial scale.
8 Final considerations and future challenges
Wastewater treatment is a fundamental process to maintain an ecological, sanitary balance appropriate to established regulations. Sustainability, efficiency, and economy in wastewater treatment is today an option that is pursued. The biological treatment of wastewater with microalgae provides economic and environmental advantages to the operating process. In recent years, interest in the use of microalgae in wastewater treatment has increased because it does not induce secondary contamination, due to its ability to avoid the use of aeration in the process due to the symbiosis between microalgae and bacteria, due to the generation of bioproducts that provide added value during the bioprocess. The implementation of high-rate algae systems in wastewater treatment can provide efficient and low-cost tertiary treatment. In turn, it is possible to use the biomass of microalgae for the biofixation of CO2 and thereby help to maintain the carbon footprint. All this generates a self-sustaining process that provides advantages for the environment and the industry, benefiting the quality of life on the planet.
The application of wastewater treatment using microalgae presents some challenges that must continue to be studied and resolved until operational and reproducible systems are available.
Among these, we have:
(i) The appropriate design and type of photobioreactor, the configuration plays an important role in the correct homogenization of the necessary biomass so that the highest content of the substrate can be reached and absorbed, guaranteeing good yields and productivity, improving the ideal size conditions for better use of square meters and develop economic and efficient systems.
(ii) Advance more rapidly in studies on an industrial scale, in this way, it will be possible to expose and demonstrate the behavior of microalgae and their yields under high-volume conditions.
(iii) Identify robust strains adapted to the specific contaminant load that generates better yields and productivity in some type of bioproduct of interest and continue advancing in consortium studies with mixtures of microalgae, bacteria, fungi, and yeasts.
(iv) Solve mechanical problems in culture systems and/or HRAP that generate cell lysis, accumulation of sludge and debris.
(v) Couple dual-mass harvesting systems and reuse of culture medium in photobioreactors to make them more effective.
(vi) Carry out a greater number of life cycle analyses and planetary analyses based on microalgae or consortia and compare them with traditional wastewater management and treatment systems.
(vii) Advance in automation technology, remote and efficient monitoring, and troubleshooting in production systems.
In this way, the application of the concept of effluent treatment together with the microalgal biorefinery could mean important opportunities for the sustainable use of resources. There will always be the conversion of pollutants dissolved in wastewater, contributing to improving the environment during the production process once the compounds are stabilized for discharge into bodies of water.
9 Conclusion
The biological treatment of wastewater with microalgae provides economic and environmental advantages to the operation process. Its implementation can provide efficient and low-cost tertiary treatments where contaminating compounds such as nitrogen and phosphorus can be reduced in high proportions. At the same time, the ability of microalgae to biofix CO2 contributes to the mitigation of greenhouse gases to maintain the carbon footprint. The advantages it provides from an environmental and agroindustrial point of view come from the diversity of its chemical and metabolic composition. The use of microalgae at the agricultural level has focused attention in recent years considering that microalgae biomass has shown notable results at the agri-food level, which also provides economic advantages by providing products with added value from biomass that have various areas of application, marking the way for sustainable development processes.
Despite this, there are challenges in the microalgal process that must be corrected, which are associated with the design of reactors or culture systems, management of organic waste, physicochemical control variables, scaling, and microalgae harvesting, among others, therefore that it is still necessary to continue with research and testing on a medium and large scale, in addition to continuing to raise awareness of the benefits of the use of microalgae in areas of social interest and the global economy.
Author contributions
LR and RR, contributed to manuscript revision, read, and approved the submitted version. All authors contributed to the article and approved the submitted version.
Funding
Research and Development Directorate of the Technological University of Uruguay. Josh 1:9.
Conflict of interest
The authors declare that the research was conducted in the absence of any commercial or financial relationships that could be construed as a potential conflict of interest.
Publisher’s note
All claims expressed in this article are solely those of the authors and do not necessarily represent those of their affiliated organizations, or those of the publisher, the editors and the reviewers. Any product that may be evaluated in this article, or claim that may be made by its manufacturer, is not guaranteed or endorsed by the publisher.
References
Abiusi, F., Trompetter, E., Pollio, A., Wijffels, R. H., and Janssen, M. (2022). Acid tolerant and acidophilic microalgae: an underexplored world of biotechnological opportunities. Front. Microbiol. 27 (13), 820907. doi:10.3389/fmicb.2022.820907
Aguirre, P., Álvarez, E., Ferrer, I., and García, J. (2011). Treatment of piggery wastewater in experimental high-rate algal ponds. Rev. Latinoam. Biotecnol. Ambient. Algal 2 (2), 57–66.
Amaro, H. M., Salgado, E. M., Nunes, O. C., Pires, J. C. M., and Esteves, A. F. (2023a). Microalgae systems - environmental agents for wastewater treatment and further potential biomass valorisation. J. Environ. Manag. 337, 117678. doi:10.1016/j.jenvman.2023.117678
Amaro, H. M., Sousa, J. F., Salgado, E. M., Pires, J. C. M., and Nunes, O. C. (2023b). Microalgal systems, a green solution for wastewater conventional pollutants removal, disinfection, and reduction of antibiotic resistance genes prevalence? Appl. Sci. 13, 4266. doi:10.3390/app13074266
Arguelles, E. D., and Martinez-Goss, M. R. (2021). Lipid accumulation and profiling in microalgae Chlorolobion sp. (BIOTECH 4031) and chlorella sp. (BIOTECH 4026) during nitrogen starvation for biodiesel production. J. Appl. Phycol. 33, 1–11. doi:10.1007/s10811-020-02126-z
Aslan, S., and Kapdan, I. K. (2006). Batch kinetics of nitrogen and phosphorus removal from synthetic wastewater by algae. Ecol. Eng. 28, 64–70. doi:10.1016/j.ecoleng.2006.04.003
Ávila, R., Justo, Á., Carrero, E., Crivillés, E., Vicent, T., and Blánquez, P. (2022). Water resource recovery coupling microalgae wastewater treatment and sludge co-digestion for bio-wastes valorisation at industrial pilot-scale. Bioresour. Technol. 343, 126080. doi:10.1016/j.biortech.2021.126080
Balasubramaniam, V., Gunasegavan, R. D., Mustar, S., Lee, J. C., and Mohd Noh, M. F. (2021). Isolation of industrial important bioactive compounds from microalgae. Molecules 26 (4), 943. doi:10.3390/molecules26040943
Bhatt, P., Bhandari, G., Bhatt, K., and Simsek, H. (2022). Microalgae-based removal of pollutants from wastewaters: occurrence, toxicity, and circular economy. Chemosphere 306, 135576. doi:10.1016/j.chemosphere.2022.135576
Bhatti, S., Richards, R., Wall, C. L., MacPherson, M. J., Stemmler, K., Lalonde, C. G., et al. (2023). Phycoremediation and simultaneous production of protein-rich algal biomass from aquaculture and agriculture wastewaters. J. Chem. Technol. Biotechnol. 98, 1918–1935. doi:10.1002/jctb.7409
Bhuyar, P., Farez, F., Rahim, F., Maniam, G., and Govindan, N. (2021). Removal of nitrogen and phosphorus from agro-industrial wastewater by using microalgae collected from coastal region of peninsular Malaysia. Afr. J. Biol. Sci. 3 (1), 58–66. doi:10.33472/afjbs.3.1.2021.58-66
Borowitzka, M. (2013). High-value products from microalgae—their development and commercialisation. J. Appl. Phycol. 25, 743–756. doi:10.1007/s10811-013-9983-9
Cardozo, K., Guaratini, T., Barros, M., Falcão, V., Tonon, A., Lopes, N., et al. (2007). Metabolites from algae with economical impact. Comp. Biochem. Physiology - Part C Toxicol. Pharmacol. 146 (1), 60–78. doi:10.1016/j.cbpc.2006.05.007
Carvalho, V. C. F., Kessler, M., Fradinho, J. C., Oehmen, A., and Reis, M. A. M. (2021). Achieving nitrogen and phosphorus removal at low C/N ratios without aeration through a novel phototrophic process. Sci. Total Environ. 793, 148501. doi:10.1016/j.scitotenv.2021.148501
Chagas, A., Rios, A., Jarenkow, A., Marcílio, N., Ayub, M., and Rech, R. (2015). Production of carotenoids and lipids by Dunaliella tertiolecta using CO2 from beer fermentation. Biochemistry 50 (6), 981–988. doi:10.1016/j.procbio.2015.03.012
Chen, C., Hsieh, C., Lee, D., Chang, C., and Chang, J. (2016). Production, extraction and stabilization of lutein from microalga Chlorella sorokiniana MB-1. Bioresour. Technol. 200, 500–505. doi:10.1016/j.biortech.2015.10.071
Chini Zittelli, G., Lauceri, R., Faraloni, C., Silva, A. M., and Torzillo, G. (2023). Valuable pigments from microalgae: phycobiliproteins, primary carotenoids, and fucoxanthin. Photochem Photobiol. Sci. 22, 1733–1789. doi:10.1007/s43630-023-00407-3
Cho, D., Choi, J., Kang, Z., Kim, B., Oh, H., Kim, H., et al. (2017). Microalgal diversity fosters stable biomass productivity in open ponds treating wastewater. Sci. Rep. 7, 1979. doi:10.1038/s41598-017-02139-8
Cho, K. H., Wolny, J., Kase, J. A., Unno, T., and Pachepsky, Y. (2022). Interactions of E. coli with algae and aquatic vegetation in natural waters. Water Res. 209, 117952. doi:10.1016/j.watres.2021.117952
Christensen, M. L., Cvitanich, C., Quist-Jensen, C. A., Thau, M., and Malmgren-Hansen, B. (2022). Precipitation and recovery of phosphorus from the wastewater hydrolysis tank. Sci. Total Environ. 813, 151875. doi:10.1016/j.scitotenv.2021.151875
Cobos, M., Castro, J. C., Paredes, J. D., Pérez, S., Maddox, J. D., Estela, S. L., et al. (2020). “Isolation, characterization, and biotechnological potential of native microalgae from the Peruvian amazon,” in Microalgae - from physiology to application. London, United Kingdom: IntechOpen. doi:10.5772/intechopen.89515
Converti, A., Casazza, A. A., Ortiz, E. Y., Perego, P., and Del Borghi, M. (2009). Effect of temperature and nitrogen concentration on the growth and lipid content of Nannochloropsis oculata and Chlorella vulgaris for biodiesel production. Chem. Eng. Process. Process Intensif. 48 (6), 1146–1151. doi:10.1016/j.cep.2009.03.006
Craggs, R., Lundquist, T., and Benemann, J. (2013). “Wastewater treatment and algal biofuel production,” in Algae for biofuels and energy. Editors M. Borowitzka, and N. Moheimani (Murdoch, Australia: Springer), 153–163. doi:10.1007/978-94-007-5479-9
Dahmani, S., Zerrouki, D., Ramanna, L., Rawat, I., and Bux, F. (2016). Cultivation of Chlorella pyrenoidosa in outdoor open raceway pond using domestic wastewater as medium in arid desert region. Bioresour. Technol. 219, 749–752. doi:10.1016/j.biortech.2016.08.019
Dalvi, V., Naaz, F., Nigam, H., Jain, R., Samuchiwal, S., Kalia, S., et al. (2021). Removal of pollutants from wastewater via biological methods and shifts in microbial community profile during treatment process. Wastewater Treat. React. 2021, 19–38. doi:10.1016/B978-0-12-823991-9.00022-8
Dar, R. A., Sharma, N., Kaur, K., and Phutela, U. G. (2019). “Feasibility of microalgal technologies in pathogen removal from wastewater,” in Application of microalgae in wastewater treatment. Editors S. K. Gupta, and F. Bux (Cham: Springer). doi:10.1007/978-3-030-13913-1_12
Delanka-Pedige, H. M. K., Munasinghe-Arachchige, S. P., Cornelius, J., Henkanatte-Gedera, S. M., Tchinda, D., Zhang, Y., et al. (2019). Pathogen reduction in an algal-based wastewater treatment system employing Galdieria sulphuraria. Algal Res. 39, 101423. doi:10.1016/j.algal.2019.101423
de Oliveira, M. C., Bassin, I. D., and Cammarota, M. C. (2022). Microalgae and cyanobacteria biomass pretreatment methods: a comparative analysis of chemical and thermochemical pretreatment methods aimed at methane production. Fermentation 8, 497. doi:10.3390/fermentation8100497
Deprá, M. C., Ramírez-Mérida, L. G., de Menezes, C. R., Zepka, L. Q., and Jacob-Lopes, E. (2019). A new hybrid photobioreactor design for microalgae culture. Chem. Eng. Res. Des. 144, 1–10. doi:10.1016/j.cherd.2019.01.023
Deviram, G., Mathimani, T., Anto, S., Ahamed, T. S., Ananth, D. A., and Pugazhendhi, A. (2020). Applications of microalgal and cyanobacterial biomass on a way to safe, cleaner and a sustainable environment. J. Clean. Prod. 253, 119770. doi:10.1016/j.jclepro.2019.119770
Dineshkumar, R., Kumaravel, R., Gopalsamy, J., Azim Sikder, M. N., and Sampathkumar, P. (2018). Microalgae as bio-fertilizers for rice growth and seed yield productivity. Waste Biomass Valor 9, 793–800. doi:10.1007/s12649-017-9873-5
Dubey, S., Chen, C. W., Haldar, D., Tambat, V. S., Kumar, P., Tiwari, A., et al. (2023). Advancement in algal bioremediation for organic, inorganic, and emerging pollutants. Environ. Pollut. 317, 120840. doi:10.1016/j.envpol.2022.120840
Durvasula, R., Hurwitz, I., Fieck, A., and Subba, D. (2015). Culture, growth, pigments and lipid content of Scenedesmus species, an extremophile microalga from Soda Dam, New Mexico in wastewater. Algal Res. 10, 128–133. doi:10.1016/j.algal.2015.04.003
El Hamouri, B. (2009). Rethinking natural, extensive systems for tertiary treatment purposes: the high-rate algae pond as an example. Desalination water Treat. 4 (1-3), 128–134. doi:10.5004/dwt.2009.367
El-Sheekh, M., Abdel-Daim, M. M., Okba, M., Okba, M., Gharib, S., Soliman, A., et al. (2021). Green technology for bioremediation of the eutrophication phenomenon in aquatic ecosystems: a review. Afr. J. Aquat. Sci. 46 (3), 274–292. doi:10.2989/16085914.2020.1860892
Feng, P. Z., Xu, Z. B., Qin, L., Alam, M. A., Wang, Z. M., and Zhu, S. N. (2020). Effects of different nitrogen sources and light paths of flat plate photobioreactors on the growth and lipid accumulation of Chlorella sp. GN1 outdoors. GN1 Outdoors. Bioresour. Technol. 301, 122762. doi:10.1016/j.biortech.2020.122762
Flassig, R., Fachet, M., Rihko-Struckmann, L., and Sundmacher, K. (2015). Robust process design for the bioproduction of β-carotene in green microalgae. Comput. Aided Chem. Eng. 37, 2117–2122. doi:10.1016/B978-0-444-63576-1.50047-9
Flores-Salgado, G., Thalasso, F., Buitrón, G., Vital-Jácome, M., and Quijano, G. (2021). Kinetic characterization of microalgal-bacterial systems: contributions of microalgae and heterotrophic bacteria to the oxygen balance in wastewater treatment. Biochem. Eng. J. 165, 107819. doi:10.1016/j.bej.2020.107819
Garrido-Cardenas, J. A., Esteban-García, B., Agüera, A., Sánchez-Pérez, J. A., and Manzano-Agugliaro, F. (2020). Wastewater treatment by advanced oxidation process and their worldwide research trends. Int. J. Environ. Res. Public Health 17, 170. doi:10.3390/ijerph17010170
Gonçalves, A. L. (2021). The use of microalgae and cyanobacteria in the improvement of agricultural practices: a review on their biofertilising, biostimulating and biopesticide roles. Appl. Sci. 11, 871. doi:10.3390/app11020871
González-Camejo, J., Montero, P., Aparicio, S., Ruano, M. V., Borrás, L., Seco, A., et al. (2020). Nitrite inhibition of microalgae induced by the competition between microalgae and nitrifying bacteria. Water Res. 172, 115499. doi:10.1016/j.watres.2020.115499
Guo, S., Wang, P., Wang, X., Zou, M., Liu, C., and Hao, J. (2020). “Microalgae as biofertilizer in modern agriculture,” in Microalgae biotechnology for food, health and high value products. Editors M. Alam, J. L. Xu, and Z. Wang (Singapore: Springer). doi:10.1007/978-981-15-0169-2_12
Gutiérrez, R., Ferrer, I., González-Molina, A., Salvadó, H., García, J., and Uggetti, E. (2016). Microalgae recycling improves biomass recovery from wastewater treatment high rate algal ponds. Water Res. 106 (1), 539–549. doi:10.1016/j.watres.2016.10.039
Hu, X., Song, C., Mu, H., Liu, Z., and Kitamura, Y. (2020). Optimization of simultaneous soybean processing wastewater treatment and flue gas CO2 fixation via chlorella sp. L166 cultivation. J. Environ. Chem. Eng. 8 (4), 103960. doi:10.1016/j.jece.2020.103960
Huang, H., Zhong, S., Wen, S., Luo, C., and Long, T. (2022). Improving the efficiency of wastewater treatment and microalgae production for biofuels. Resour. Conservation Recycl. 178, 106094. doi:10.1016/j.resconrec.2021.106094
Jinda, K., Koottatep, T., Chaiwong, C., and Polprasert, C. (2020). Performance evaluation of novel attached-growth high rate algal pond system with additional artificial illumination for wastewater treatment and nutrient recovery. Water Sci. Technol. 82 (1), 97–106. doi:10.2166/wst.2020.329
Kalra, R., Gaur, S., and Goel, M. (2021). Microalgae bioremediation: a perspective towards wastewater treatment along with industrial carotenoids production. J. Water Process Eng. 40, 101794. doi:10.1016/j.jwpe.2020.101794
Komolafe, O., Velasquez Orta, S. B., Monje-Ramirez, I., Noguez, I. Y., Harvey, A. P., and Orta Ledesma, M. T. (2014). Biodiesel production from indigenous microalgae grown in wastewater. Technol 154, 297–304. doi:10.1016/j.biortech.2013.12.048
Kotoula, D., Iliopoulou, A., Irakleous-Palaiologou, E., Gatidou, G., Aloupi, M., Antonopoulou, P., et al. (2020). Municipal wastewater treatment by combining in series microalgae Chlorella sorokiniana and macrophyte Lemna minor: preliminary results. J. Clean. Prod. 271, 122704. doi:10.1016/j.jclepro.2020.122704
Koul, B., Yadav, D., Singh, S., Kumar, M., and Song, M. (2022). Insights into the domestic wastewater treatment (dwwt) regimes: a review. Water 14, 3542. doi:10.3390/w14213542
Koutra, E., Mastropetros, S. G., Ali, S. S., Tsigkou, K., and Kornaros, M. (2021). Assessing the potential of Chlorella vulgaris for valorization of liquid digestates from agro-industrial and municipal organic wastes in a biorefinery approach. J. Clean. Prod. 280 (1), 124352. doi:10.1016/j.jclepro.2020.124352
Kundu, D., Dutta, D., Samanta, P., Dey, S., Sherpa, K. C., Kumar, S., et al. (2022). Valorization of wastewater: a paradigm shift towards circular bioeconomy and sustainability. Sci. Total Environ. 848, 157709. doi:10.1016/j.scitotenv.2022.157709
Li, G., Xiao, W., Yang, T., and Lyu, T. (2023). Optimization and process effect for microalgae carbon dioxide fixation technology applications based on carbon capture: a comprehensive review. C 9, 35. doi:10.3390/c9010035
Li, S., Li, X., and Ho, S. H. (2022). How to enhance carbon capture by evolution of microalgal photosynthesis? Sep. Purif. Technol. 291, 120951. doi:10.1016/j.seppur.2022.120951
Li, W., Peng, S., Jiao, H., Zhang, J., Huang, Y., and Sun, L. (2021). Performance assessment of microalgal-based wastewater treatment (MBWT) system in response to operation modes, hydraulic retention time (HRT) and cyclical light. Desalination Water Treat. 229, 79–92. doi:10.5004/dwt.2021.27368
Lima, S., Villanova, V., Grisafi, F., Caputo, G., Brucato, A., and Scargiali, F. (2020). Autochthonous microalgae grown in municipal wastewaters as a tool for effectively removing nitrogen and phosphorous. J. Water Process Eng. 38, 101647. doi:10.1016/j.jwpe.2020.101647
Liu, X., Ji, B., and Li, A. (2023). Enhancing biolipid production and self-flocculation of Chlorella vulgaris by extracellular polymeric substances from granular sludge with CO2 addition: microscopic mechanism of microalgae-bacteria symbiosis. Water Res. 236, 119960. doi:10.1016/j.watres.2023.119960
Liu, X. Y., and Hong, Y. (2021). Microalgae-based wastewater treatment and recovery with biomass and value-added products: a brief review. Curr. Pollut. Rep. 7, 227–245. doi:10.1007/s40726-021-00184-6
Liu, X.-Y., Hong, Y., Zhao, G.-P., Zhang, H.-K., Zhai, Q.-Y., and Wang, Q. (2022). Microalgae-based swine wastewater treatment: strain screening, conditions optimization, physiological activity and biomass potential. Sci. Total Environ. 807 (3), 151008. doi:10.1016/j.scitotenv.2021.151008
Llamas, M., Magdalena, J. A., Tomás-Pejó, E., and González-Fernández, C. (2020). Microalgae-based anaerobic fermentation as a promising technology for producing biogas and microbial oils. Energy 206, 118184. doi:10.1016/j.energy.2020.118184
Malcheva, B. Z., Petrov, P. G., and Stefanova, V. V. (2022). Microbiological control in decontamination of sludge from wastewater treatment plant. Processes 10, 406. doi:10.3390/pr10020406
Marks, E. A. N., Miñón, J., Pascual, A., Montero, O., Manuel, L., and Rad, C. (2017). Application of a microalgal slurry to soil stimulates heterotrophic activity and promotes bacterial growth. Sci. Total Environ. 605-606, 610–617. doi:10.1016/j.scitotenv.2017.06.169
Martín-Marroquín, J. M., Garrote, L., Hidalgo, D., Moustakas, K., Barampouti, E. M., and Mai, S. (2023). Solar-powered algal production on vegetable processing industry wastewater at pilot scale. Clean. Techn Environ. Policy 1–13. doi:10.1007/s10098-023-02505-3
Medeiros, V. P. B., Pimentel, T. C., Varandas, R. C. R., dos Santos, S. A., Pedrosa, G. T. S., Sassi, C. F. C., et al. (2020). Exploiting the use of agro-industrial residues from fruit and vegetables as alternative microalgae culture medium. Food Res. Int. 137, 109722. doi:10.1016/j.foodres.2020.109722
Mobin, S. M. A., Chowdhury, H., and Alam, F. (2019). Commercially important bioproducts from microalgae and their current applications – a review. Energy Procedia 160, 752–760. doi:10.1016/j.egypro.2019.02.183
Moondra, N., Jariwala Namrata, D., and Christian, R. A. (2020). Sustainable treatment of domestic wastewater through microalgae. Int. J. Phytoremediation 22, 1480–1486. doi:10.1080/15226514.2020.1782829
Morales-Amaral, M., Gómez-Serrano, C. F., Acién, G., Fernández-Sevilla, J. M., and Molina-Grima, E. (2015). Production of microalgae using centrate from anaerobic digestion as the nutrient source. Algal Res. 9, 297–305. doi:10.1016/j.algal.2015.03.018
Morales-Sánchez, D., Martinez-Rodriguez, O. A., and Martinez, A. (2017). Heterotrophic cultivation of microalgae: production of metabolites of commercial interest. J. Chem. Technol. Biotechnol. 92, 925–936. doi:10.1002/jctb.5115
Muniswami, D. M., Chinnadurai, S., Sachin, M., Jithin, H., Ajithkumar, K., Surya Narayanan, G., et al. (2021). Comparative study of biofertilizer/biostimulant from seaweeds and seagrass in Abelmoschus esculentus crop. Biomass Conv. Bioref 13, 11005–11022. doi:10.1007/s13399-021-01881-4
Mustafa, E. M., Phang, S. M., and Chu, W. L. (2012). Use of an algal consortium of five algae in the treatment of landfill leachate using the high-rate algal pond system. J. Appl. Phycol. 24 (4), 953–963. doi:10.1007/s10811-011-9716-x
Mutum, L., Janda, T., Ördög, V., and Molnár, Z. (2022). Biologia Futura: potential of different forms of microalgae for soil improvement. Biol. Futura 73, 1–8. doi:10.1007/s42977-021-00103-2
Nayak, M., Swain, D. K., and Sen, R. (2019). Strategic valorization of de-oiled microalgal biomass waste as biofertilizer for sustainable and improved agriculture of rice (Oryza sativa L.) crop. Sci. Total Environ. 682, 475–484. doi:10.1016/j.scitotenv.2019.05.123
Nobre, B., Villalobos, F., Barragán, B., Oliveira, A., Batista, A., Marques, P., et al. (2013). A biorefinery from Nannochloropsis sp. microalga – extraction of oils and pigments. Production of biohydrogen from the leftover biomass. Bioresour. Technol. 135, 128–136. doi:10.1016/j.biortech.2012.11.084
Obaideen, K., Shehata, N., Sayed, E. T., Abdelkareem, M. A., Mahmoud, M. S., and Olabi, A. G. (2022). The role of wastewater treatment in achieving sustainable development goals (SDGs) and sustainability guideline. Energy Nexus 7, 100112. doi:10.1016/j.nexus.2022.100112
Oliveira, C. Y. B., Oliveira, C. D. L., Prasad, R., Ong, H. C., Araujo, E. S., Shabnam, N., et al. (2021). A multidisciplinary review of Tetradesmus obliquus: a microalga suitable for large-scale biomass production and emerging environmental applications. Rev. Aquac. 13, 1594–1618. doi:10.1111/raq.12536
Olofsson, M., Lindehoff, E., and Legrand, C. (2019). Production stability and biomass quality in microalgal cultivation - contribution of community dynamics. Eng. Life Sci. 27 (5), 330–340. doi:10.1002/elsc.201900015
Osorio-Reyes, J. G., Valenzuela-Amaro, H. M., Pizaña-Aranda, J. J. P., Ramírez-Gamboa, D., Meléndez-Sánchez, E. R., López-Arellanes, M. E., et al. (2023). Microalgae-based biotechnology as alternative biofertilizers for soil enhancement and carbon footprint reduction: advantages and implications. Mar. Drugs. 21, 93. doi:10.3390/md21020093
Pasquet, V., Chérouvrier, J., Farhat, F., Thiéry, V., Piot, J., Bérard, J., et al. (2011). Study on the microalgal pigments extraction process: performance of microwave assisted extraction. Process Biochem. 46, 59–67. doi:10.1016/j.procbio.2010.07.009
Patel, A. K., Singhania, R. R., Sim, S. J., and Dong, C. D. (2021). Recent advancements in mixotrophic bioprocessing for production of high value microalgal products. Bioresour. Technol. 320, 124421. doi:10.1016/j.biortech.2020.124421
Pereira, A. G., Fraga-Corral, M., Garcia-Oliveira, P., Lourenço-Lopes, C., Carpena, M., Prieto, M. A., et al. (2021). The use of invasive algae species as a source of secondary metabolites and biological activities: Spain as case-study. Mar. Drugs 19, 178. doi:10.3390/md19040178
Petrik, L. F., Ngo, H. H., Varjani, S., Osseweijer, P., Xevgenos, D., van Loosdrecht, M., et al. (2022). From wastewater to resource. One Earth 5 (2), 122–125. doi:10.1016/j.oneear.2022.01.011
Polat, E., Yavuztürk-Gül, B., Ünver, H., and Altınbaş, M. (2023). Biotechnological product potential of Auxenochlorella protothecoides including biologically active compounds (BACs) under nitrogen stress conditions. World J. Microbiol. Biotechnol. 39, 198. doi:10.1007/s11274-023-03642-z
Priya, M., Gurung, N., Mukherjee, K., and Bose, S. (2014). Microalgae in removal of heavy metal and organic pollutants from soil. Microb. Biodegrad. Bioremediation 23, 519–537. doi:10.1016/B978-0-12-800021-2.00023-6
Qian, J., Zhang, J., Jin, Z., Cheng, J., Li, J., Song, H., et al. (2022). Enhancing algal yield and nutrient removal from anaerobic digestion piggery effluent by an integrated process-optimization strategy of fungal decolorization and microalgae cultivation. Appl. Sci. 12, 4741. doi:10.3390/app12094741
Qin, Y., Wang, K., Xia, Q., Yu, S., Zhang, M., An, Y., et al. (2023). Up-concentration of nitrogen from domestic wastewater: a sustainable strategy from removal to recovery. Chem. Eng. J. 451, 138789. doi:10.1016/j.cej.2022.138789
Qiu, Y., Frear, C., Chen, S., Ndegwa, P., Harrison, J., Yao, Y., et al. (2020). Accumulation of long-chain fatty acids from Nannochloropsis salina enhanced by breaking microalgae cell wall under alkaline digestion. Renew. Energy 149, 691–700. doi:10.1016/j.renene.2019.12.093
Raklami, A., Meddich, A., Oufdou, K., and Baslam, M. (2022). Plants—microorganisms-based bioremediation for heavy metal cleanup: recent developments, phytoremediation techniques, regulation mechanisms, and molecular responses. Int. J. Mol. Sci. 23, 5031. doi:10.3390/ijms23095031
Ramírez-Mérida, L. G., Zepka, L. Q., de Menezes, C. R., and Jacob-Lopes, E. (2015a). Microalgae as nanofactory for production of antimicrobial molecules. J. Nanomedicine Nanotechnol. s6, 004. doi:10.4172/2157-7439.S6-004
Ramírez-Mérida, L. G., Zepka, L. Q., and Jacob-Lopes, E. (2015b). Why does the photobioreactors fail? J. Bioprocess. Biotech. 5, 7. doi:10.4172/2155-9821.1000239
Ramírez-Mérida, L. G., Zepka, L. Q., and Jacob-Lopes, E. (2015c). Current status, future developments and recent patents on photobioreactor technology. Recent Pat. Eng. 9 (2), 80–90. doi:10.2174/1872212109666150206235316
Rana, M. S., and Prajapati, S. K. (2021). Resolving the dilemma of iron bioavailability to microalgae for commercial sustenance. Algal Res. 59, 102458. doi:10.1016/j.algal.2021.102458
Raposo, M., Morais, R., and Morais, A. (2013). Health applications of bioactive compounds from marine microalgae. Life Sci. 93, 479–486. doi:10.1016/j.lfs.2013.08.002
Richmond, A., and Qiang, H. (2013). Handbook of microalgal culture: applied phycology and biotechnology. 2nd ed. Hoboken, NJ, USA: John Wiley & Sons, Ltd, 719. doi:10.1002/9781118567166
Ritu, J. R., Ambati, R. R., Ravishankar, G. A., Shahjahan, M., and Khan, S. (2023). Utilization of astaxanthin from microalgae and carotenoid rich algal biomass as a feed supplement in aquaculture and poultry industry: an overview. J. Appl. Phycol. 35, 145–171. doi:10.1007/s10811-022-02817-9
Rodrigues, D., Flores, É., Barin, J., Mercadante, A., Jacob-Lopes, E., and Zepka, L. (2014). Production of carotenoids from microalgae cultivated using agroindustrial wastes. Food Res. Int. 65 (B), 144–148. doi:10.1016/j.foodres.2014.06.037
Sadvakasova, A. K., Kossalbayev, B. D., Bauenova, M. O., Balouch, H., Leong, Y. K., Zayadan, B. K., et al. (2023). Microalgae as a key tool in achieving carbon neutrality for bioproduct production. Algal Res. 72, 103096. doi:10.1016/j.algal.2023.103096
Salvatore, M. M., Carraturo, F., Salbitani, G., Rosati, L., De Risi, A., Andolfi, A., et al. (2023). Biological and metabolic effects of the association between the microalga Galdieria sulphuraria and the fungus Penicillium citrinum. Sci. Rep. 13, 1789. doi:10.1038/s41598-023-27827-6
Sampathkumar, P., Dineshkumar, R., Rasheeq, A. A., Arumugam, A., and Nambi, K. S. N. (2019). Marine microalgal extracts on cultivable crops as a considerable bio-fertilizer: a Review. Indian J. Traditional Knowl. (IJTK). 18, 4. doi:10.56042/ijtk.v18i4.29031
Sánchez-Zurano, A., Morillas-España, A., Gómez-Serrano, C., Ciardi, M., Acién, G., and Lafarga, T. (2021). Annual assessment of the wastewater treatment capacity of the microalga Scenedesmus almeriensis and optimisation of operational conditions. Sci. Rep. 11, 21651. doi:10.1038/s41598-021-01163-z
Sátiro, J., Cunha, A., Gomes, A. P., Simões, R., and Albuquerque, A. (2022). Optimization of microalgae–bacteria consortium in the treatment of paper pulp wastewater. Appl. Sci. 12, 5799. doi:10.3390/app12125799
Saúco, C., Cano, R., Marín, D., Lara, E., Rogalla, F., and Arbib, Z. (2021). Hybrid wastewater treatment system based in a combination of high rate algae pond and vertical constructed wetland system at large scale. J. Water Process Eng. 43, 102311. doi:10.1016/j.jwpe.2021.102311
Sepúlveda-Muñoz, C. A., de Godos, I., and Muñoz, R. (2023). Wastewater treatment using photosynthetic microorganisms. Symmetry 15, 525. doi:10.3390/sym15020525
Shekh, A., Sharma, A., Schenk, P. M., Kumar, G., and Mudliar, S. (2022). Microalgae cultivation: photobioreactors, CO<sub>2</sub> utilization, and value-added products of industrial importance. J. Chem. Technol. Biotechnol. 97, 1064–1085. doi:10.1002/jctb.6902
Song, Y., Wang, L., Qiang, X., Gu, W., Ma, Z., and Wang, G. (2022). The promising way to treat wastewater by microalgae: approaches, mechanisms, applications and challenges. J. Water Process Eng. 49, 103012. doi:10.1016/j.jwpe.2022.103012
Streit, N., Ramírez Mérida, L., Zepka, L., Jacob-Lopes, E., and Queiroz, M. (2017). Producción de pigmentos por Aphanothece microscopica Nägeli a partir de resíduos industriales lácteos. Ingeniare Rev. Chil. Ing. 25 (2), 350–358. doi:10.4067/S0718-33052017000200350
Sukačová, K., and Červený, J. (2017). Can algal biotechnology bring effective solution for closing the phosphorus cycle? Use of algae for nutrient removal - review of past trends and future perspectives in the context of nutrient recovery. Eur. J. Environ. Sci. 7 (1), 63–72. doi:10.14712/23361964.2017.6
Sutherland, D., Park, J., Ralph, P., and Craggs, R. (2020). Improved microalgal productivity and nutrient removal through operating wastewater high rate algal ponds in series. Algal Res. 47, 101850. doi:10.1016/j.algal.2020.101850
Tawfik, A., Eraky, M., Alhajeri, N. S., Osman, A. I., and Rooney, D. W. (2022). Cultivation of microalgae on liquid anaerobic digestate for depollution, biofuels and cosmetics: a review. Environ. Chem. Lett. 20, 3631–3656. doi:10.1007/s10311-022-01481-2
Torres-Franco, A., Passos, F., Figueredo, C., Mota, C., and Muñoz, R. (2021). Current advances in microalgae-based treatment of high-strength wastewaters: challenges and opportunities to enhance wastewater treatment performance. Rev. Environ. Sci. Biotechnol. 20, 209–235. doi:10.1007/s11157-020-09556-8
Touliabah, H.E.-S., El-Sheekh, M. M., Ismail, M. M., and El-Kassas, H. A. (2022). A review of microalgae- and cyanobacteria-based biodegradation of organic pollutants. Molecules 27, 1141. doi:10.3390/molecules27031141
Tran, D., Van Do, T. C., Nguyen, Q. T., and Le, T. G. (2021). Simultaneous removal of pollutants and high value biomaterials production by Chlorella variabilis TH03 from domestic wastewater. Clean. Techn Environ. Policy 23, 3–17. doi:10.1007/s10098-020-01810-5
Umetani, I., Sposób, M., and Tiron, O. (2023). Indigenous green microalgae for wastewater treatment: nutrient removal and resource recovery for biofuels and bioproducts. Bioenerg. Res. 1–11. doi:10.1007/s12155-023-10611-9
Vadiveloo, A., Foster, L., Kwambai, C., Bahri, P. A., and Moheimani, N. R. (2021). Microalgae cultivation for the treatment of anaerobically digested municipal centrate (ADMC) and anaerobically digested abattoir effluent (ADAE). Sci. Total Environ. 775, 145853. doi:10.1016/j.scitotenv.2021.145853
Verma, N., Sharma, S., Dhasmana, A., and Kumar, V. (2019). “Phycoremediation of pollutants for ecosystem restitution,” in Phyto and rhizo remediation microorganisms for sustainability. Editors N. Arora, and N. Kumar (Singapore: Springer). doi:10.1007/978-981-32-9664-0_3
Wan, M., Zhang, Z., Wang, J., Huang, J., Fan, J., Yu, A., et al. (2015). Sequential heterotrophy–dilution–photoinduction cultivation of haematococcus pluvialis for efficient production of astaxanthin. Bioresour. Technol. 198, 557–563. doi:10.1016/j.biortech.2015.09.031
Wang, J., Hu, X., Chen, J., Wang, T., Huang, X., and Chen, G. (2022). The extraction of β-carotene from microalgae for testing their health benefits. Foods 11, 502. doi:10.3390/foods11040502
Wu, W., Tan, L., Chang, H., Zhang, C., Tan, X., Liao, Q., et al. (2023). Advancements on process regulation for microalgae-based carbon neutrality and biodiesel production. Renew. Sustain. Energy Rev. 171, 112969. doi:10.1016/j.rser.2022.112969
Xiong, Q., Hu, L. X., Liu, Y. S., Zhao, J. L., He, L. Y., and Ying, G. G. (2021). Microalgae-based technology for antibiotics removal: from mechanisms to application of innovational hybrid systems. Environ. Int. 155, 106594. doi:10.1016/j.envint.2021.106594
Xu, M., Bernards, M., and Hu, Z. (2014). Algae-Facilitated chemical phosphorus removal during high-density Chlorella emersonii cultivation in a membrane bioreactor. Bioresour. Technol. 153, 383–387. doi:10.1016/j.biortech.2013.12.026
Yaakob, M. A., Mohamed, R. M. S. R., Al-Gheethi, A., Aswathnarayana Gokare, R., and Ambati, R. R. (2021). Influence of nitrogen and phosphorus on microalgal growth, biomass, lipid, and fatty acid production: an overview. Cells 14 (2), 393. doi:10.3390/cells10020393
Yu, H., Kim, J., Rhee, C., Shin, J., Shin, S. G., and Lee, C. (2022). Effects of different pH control strategies on microalgae cultivation and nutrient removal from anaerobic digestion effluent. Microorganisms 3 (2), 357. doi:10.3390/microorganisms10020357
Zhang, J., Shao, Y., Wang, H., Liu, G., Qi, L., Xu, X., et al. (2021). Current operation state of wastewater treatment plants in urban China. Environ. Res. 195, 110843. doi:10.1016/j.envres.2021.110843
Zhang, Y., Ren, L., Chu, H., Zhou, X., Yao, T., and Zhang, Y. (2019). Optimization for Scenedesmus obliquus cultivation: the effects of temperature, light intensity and pH on growth and biochemical composition. Microbiol. Biotechnol. Lett. 47 (4), 614–620. doi:10.4014/mbl.1906.06005
Zhao, D., Cheah, W. Y., Lai, S. H., Ng, E. P., Khoo, K. S., Show, P. L., et al. (2023). Symbiosis of microalgae and bacteria consortium for heavy metal remediation in wastewater. J. Environ. Chem. Eng. 11 (3), 109943. doi:10.1016/j.jece.2023.109943
Zhu, L. (2015). Biorefinery as a promising approach to promote microalgae industry: an innovative framework. Renew. Sustain. Energy Rev. 41, 1376–1384. doi:10.1016/j.rser.2014.09.040
Keywords: bioproducts, biofertilizer, COD, HRAP, pigment, effluents
Citation: Ramírez Mérida LG and Rodríguez Padrón RA (2023) Application of microalgae in wastewater: opportunity for sustainable development. Front. Environ. Sci. 11:1238640. doi: 10.3389/fenvs.2023.1238640
Received: 12 June 2023; Accepted: 01 December 2023;
Published: 13 December 2023.
Edited by:
Eduardo Jacob-Lopes, Federal University of Santa Maria, BrazilReviewed by:
Shamshad Ahmad, Babasaheb Bhimrao Ambedkar University, IndiaAhmed Abdelhafez, The New Valley University, Egypt
Gamal Ammar, City of Scientific Research and Technological Applications, Egypt
Copyright © 2023 Ramírez Mérida and Rodríguez Padrón. This is an open-access article distributed under the terms of the Creative Commons Attribution License (CC BY). The use, distribution or reproduction in other forums is permitted, provided the original author(s) and the copyright owner(s) are credited and that the original publication in this journal is cited, in accordance with accepted academic practice. No use, distribution or reproduction is permitted which does not comply with these terms.
*Correspondence: Luis G. Ramírez Mérida, bHVpcy5yYW1pcmV6QHV0ZWMuZWR1LnV5, bHVpc2d1aWxsZXJtb2xncm1AZ21haWwuY29t