- 1School of Ecology and Environment, Inner Mongolia University, Hohhot, China
- 2Inner Mongolia Key Laboratory of Environmental Pollution Prevention and Waste Resource Recycle, Hohhot, China
- 3Ministry of Education Key Laboratory of Ecology and Resource Use of the Mongolia Plateau, Hohhot, China
The present study was developed to explore nitrogen removal performance and associated microbial mechanisms of action in vertical flow constructed wetlands (VFCWs) when using external carbon sources. These analyses ultimately revealed that alkali-soaked Phragmites australis (P. australis) could serve as an effective plant carbon source, exhibiting the lower levels of total nitrogen (TN) release and the highest chemical oxygen demand (COD) of all tested carbon sources. Nitrogen removal efficiency improved following the addition of plant carbon sources, and under carbon/nitrogen (C/N) rations of 2, 4, 5, and 7, the VFCW system was able to remove 43.69%–75.76% TN, with the highest removal rate being observed at a C/N of 5. The abundance of denitrifying microorganisms such as Thiobaillus and Halomonas were also more enriched in VFCW1 than VFCW0, with stronger correlations in the microbial network community. A qPCR approach was used to analyze functional genes involved in denitrification, revealing that the addition of plant carbon sources was associated with increases in total gene abundance and the abundance of the denitrifying gene nirS, whereas no corresponding increase in amoA or nxrA abundance was observed. Higher total gene, amoA, and nxrA abundance were observed in the upper levels of these VFCW systems as compared to the lower layers, whereas nirS exhibited the opposite abundance pattern. Overall, these findings suggested that short-range denitrification is likely to be the primary denitrification process active in this VFCW system.
1 Introduction
Agricultural production efforts generate large volumes of rainfall-runoff derived from farmlands that (Leng et al., 2021), owing to the excessive application of chemical fertilizers and pesticides, carry large amounts of nitrogen (N) that can cause serious surface water pollution (Butkovskyi et al., 2021). Nutrient levels in surface bodies of water that are derived from such receding farmland water runoff can rise as high as 60%–80% (Hu, Yang, Han and Wang, 2019), making these waters the primary non-point source of agriculture-associated pollution (Lavrnić et al., 2018; Jabbar and Grote, 2019; Kaandorp et al., 2021; Sandström et al., 2021). China is a large nation with an extensive agricultural system that relies on the use of large quantities of chemical fertilizers, posing a major risk to Chinese surface water quality owing to the potential for farmland-derived runoff pollution. Agricultural sources are thought to account for 85%–93% of the N found in surface waters (Li et al., 2018), so it is vital that decrease the N levels in the water as much as possible before farmland-derived runoff mixes with surface waters, as the purification of these water sources will yield both environmental and economic benefits by mitigating water pollution.
Constructed wetlands (CWs) are a relatively recent sewage treatment strategy that are increasingly deployed to help treat farmland runoff as they exhibit good purification efficacy, are well suited to sewage with a low carbon/nitrogen (C/N) ratio, and provide an array of economic and environmental benefits (Gordon et al., 2021). CW technologies have developed steadily throughout much of China where they can now be used to treat over 20 kinds of wastewater (Zhang et al., 2012). However, the use of CWs to treat farmland runoff can be limited by their relatively low denitrification efficiency as they are a carbon-poor system, hampering effective denitrification in traditional forms of this system (Zhou et al., 2017). The addition of external carbon sources, however, can augment microbial denitrification activity, thereby improving the ability of CWs to decontaminate N-rich wastewater (Chand, Kumar and Suthar, 2022). Traditional carbon sources for CW applications such as glucose, sucrose, acetic acid, and methanol are not appropriate for use in the processing of farmland runoff owing to their toxicity and costs (Wu et al., 2018). Given its ease of access, low cost, and high organic matter content, plant biomass has been increasingly employed as a carbon source capable of enhancing denitrification activity in CWs (Sun et al., 2019; Xiong et al., 2020). However, adding this plant biomass results in the initial release of large quantities of N, C, phosphorus, and organic matter followed by a much more gradual C release at later time points. In an effort to overcome this issue, researchers have employed acidic or alkaline treatments to modify plant biomass. Alkaline treatment can disrupt lignin structural integrity and promote cellulose hydrolysis while also promoting the formation of more irregular polygonal fiber cross-sections and increasing plant porosity and specific surface area, with all of these effects ultimately benefiting the release of organic matter from these plants for their use as a carbon source, Therefore, alkali treatment was more suitable as a pretreatment method for the preparation of the plant carbon source than acid treatment (Zheng et al., 2021).
Ulansuhai is a representative lake in the Yellow River Basin and an important component of the hydraulic engineering system in the Hetao Irrigation Area, receiving over 90% of farmland drainage from this area such that agricultural and rural region-derived waste has become the primary source of pollution in the Ulansuhai Lake. Increases in nitrogen and phosphorus levels have driven the eutrophication of this lake water and associated reductions in overall water quality. Nuen et al. (2020) found that the total nitrogen (TN) intake in the Ulansuhai basin has risen annually such that the region now faces a severe pollution load, although the among of total phosphorus (TP) inflow remains relatively limited (Nuen et al., 2020). Given this issue, it is critical that strategies be developed to remove or mitigate nitrogen pollution of farmland runoff before this water enters the lake ecosystem. Phragmites australis (P. australis) and Schoenoplectus tabernaemontani (S. tabernaemontani) are plants that are frequently found in CWs and are the dominant aquatic plant species in Ulansuhai Lake. As such, they were chosen as the plant carbon sources for use in the present study.
The present study was thus designed to explore the use of modified plant biomass as an external carbon source with the goal of improving the performance of vertical flow constructed wetlands (VFCWs) when used to treat receding waters from Ulansuhai farmland and to explore the denitrification efficiency of these CW systems. To better understand the mechanistic basis for observed nitrogen removal, differences in the composition of microbial communities and the abundance of important functional genes (amoA, nxrA, nirS, and 16S rDNA) were analyzed. These findings provide a theoretical foundation for subsequent research focused on the use of artificial wetland technologies to control water pollution in Ulansuhai, while also offering general guidance for efforts to use VFCWs to manage lake eutrophication.
2 Materials and methods
2.1 The preparation of plant carbon sources
Following the collection of P. australis and S. tabernaemontani specimens, they were rinsed using ultrapure water, dried to a constant weight in a 50°C oven, cut into 1–2 cm pieces, and separated into three different pretreatment sample groups. For P. australis, one group was left untreated (UN-P), while another was soaked for 24 h in 2% NaOH as an alkali-soaked treatment condition (AS-P), and the third was soaked in 2% NaOH for 1 h in a 90°C water bath as an alkali-heat treatment group (AH-P). S. tabernaemontani was treated using these three same approaches, yielding untreated, alkali-soaked, and alkali-heat treated samples (UN-S, AS-S, and AH-S, respectively). After treatment, these plant materials were rinsed with water, adjusted to a neutral pH, and dried to a constant weight at 50°C for subsequent utilization (Figure 1).
2.2 Static immersion experiments
A 2 g aliquot of each of these pretreated samples was added to Erlenmeyer flasks in triplicate, with 250 mL of deionized water then being added to these flasks followed by incubation at 30°C in a 100 rpm incubator. Over the course of a 20-day experiment, the total nitrogen (TN), ammonium (NH4+-N), nitrate (NO3−-N), nitrite (NO2−-N), and chemical oxygen demand (COD) concentrations in this original soaking solution were analyzed every other day via the use of standard methods (State Environmental Protection Administration of China, 2002), followed by replacement of the removed volume with deionized water (Figure 1).
2.3 Constructed wetland experiment
Figure 2 provides a schematic overview of the vertical flow constructed wetland (VFCW) system used for this study. Prior to VFCW construction, farmland water from the Ulansuhai region was used for 2 months to facilitate microbial colonization. Water was fed into the system through intermittent inflow facilitated by a peristaltic pump sequence beginning in July 2020, the water residence period in VFCW is 3d. This water was treated to simulate the receding water of Ulansuhai farmland (C/N = 2) by adding C6H12O6, KNO3, NH4Cl, and KH2PO4 to tap water. The mass concentrations for each pollutant after configuration were as follows: COD = 28.00 mg/L, TN = 12.00 mg/L, NH4+-N = 1.50 mg/L, NO3−-N = 10.50 mg/L, and TP = 1.50 mg/L. To evaluate the purifying abilities of this CW system at different C/N values, 30 g (VFCW1), 35 g (VFCW2), or 60 g (VFCW3) of alkali-heat treated P. australis was added to these VFCWs for respective C/N values of 4, 5, and 7, with the control condition without a plant carbon source exhibiting a C/N value of 2 (VFCW0).
2.4 Analyses of the microbial mechanisms governing VFCW denitrification activity
2.4.1 Sample collection and DNA extraction
Sewage was introduced into the constructed wetland pilot system (VFCW1), with samples being collected using a sampler at 15 cm, 30 cm, and 50 cm from the top of the system. For each sample depth, a ∼100 g sample was collected and stored at −80°C. A FastDNA® Spin Kit for Soil (MP Biomedicals, United States) was used to extract total DNA from these samples, after which the quality and concentration of the resultant DNA were analyzed with a NANO PHOTO METER P330 spectrophotometer (ShengKe, Shanghai, China). The V3-V4 hypervariable region of the bacterial 16S rDNA gene was amplified using the 338 F (5′-ACTCCTACGGGAGGCAGCA-3′) and 806 R (5′-GGACTACHVGGGTWTCTAAT-3′) primer pair (Cai et al., 2017). A 50 µL PCR reaction was prepared, and purified PCR products were subjected to high-throughput sequencing with the Illumina MiSeq platform by Majorbio (Shanghai, China). Sequence data were processed using the Quantitative Insights into Microbial Ecology (QIIME, v1.8.0) pipeline, and high-quality sequences were clustered into operational taxonomic units (OTUs) at 97% sequence identity via the q2-vsearch method.
2.4.2 Quantitative analyses of functional genes
Functional genes including 16S rDNA, amoA, nirS, and nxrA were analyzed via qPCR with a BIO-RAD CFX96 CONNECT system (Shanghai, China) to assess their relative abundance using primers published previously by Zhi and Ji (2014) and Ma et al. (2019). Individual 20 μL reaction mixtures for the detection of amoA abundance included 10 μL of Premix Ex Taq V2.0+ dye (Beijing, China), 1 μL of template DNA, 1.6 μL of each primer (F + R), and nuclease-free water. For the three other functional gene targets, each 20 μL reaction mixture contained 10 μL of Premix Ex Taq V2.0+ dye (Beijing, China), 2 μL of template DNA, 1 μL of each of primer (F + R), and nuclease-free water. All analyses were performed in triplicate.
2.5 Statistical analysis
Excel 2016 was used for data analyses, while SPSS 26.0 was used for correlation analyses, and Figures were constructed in Adobe Illustrator 2022 and Origin 2022. Spearman’s correlation network construction was achieved by selecting those genera exhibiting an average abundance >0.2%, with a Spearman’s correlation p-value <0.05 being considered indicative of a significant interaction. Gephi 0.9.2 was used to construct and visualize the resultant network.
3 Results
3.1 Carbon and nitrogen release in static immersion experiments
3.1.1 The release of COD from different plant carbon sources
Initially, COD release from S. tabernaemontani and P. australis biomass samples that had been treated under different conditions was assessed (Figure 3). The maximum carbon release levels for the UN-P, AS-P, and AH-P samples were 111.91, 128.72, and 143.17 mg/L, respectively, while for UN-S, AS-S, and AH-S samples these respective values were 336.01, 340.05, and 179.41 mg/L, respectively.
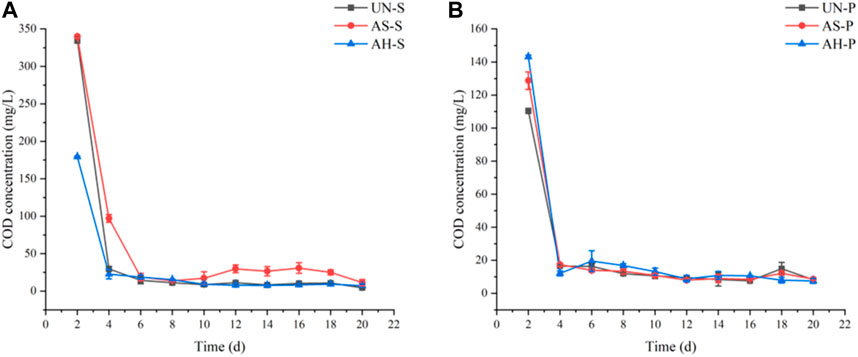
FIGURE 3. COD release from S. tabernaemontani (A) and P. australis (B) under different treatment conditions.
3.1.2 The release of N from different plant carbon sources
The rapid release of large amounts of N can contribute to a drop in water quality in VFCW systems (Figure 4). The amount of Various nitrogen release was largest during the initial phases of this experiment, gradually decreasing until day 6. The total nitrogen of Mugu and reeds released the most in the second day. Then, in the next 6 days, it quickly declined and kept stable on the eighth day. The release of ammonia nitrogen of xiangpu is also higher than the amount of reeds. The ammonia nitrogen of Mugu and reeds was released the second day were 3.81–6.46, 1.84–5.48. The release volume of the next day was 3.81–6.46, 1.84–5.48. The release of S. tabernaemontani nitrate nitrogen and nitrite nitrogen was 0–0.11, 0.01–0.06 in 20 days. The release of P. australis nitrogen nitrogen and nitrite nitrogen nitrogen nitrogen nitrogen is 0–0.20, 0.01–0.05 in 20 days, respectively.
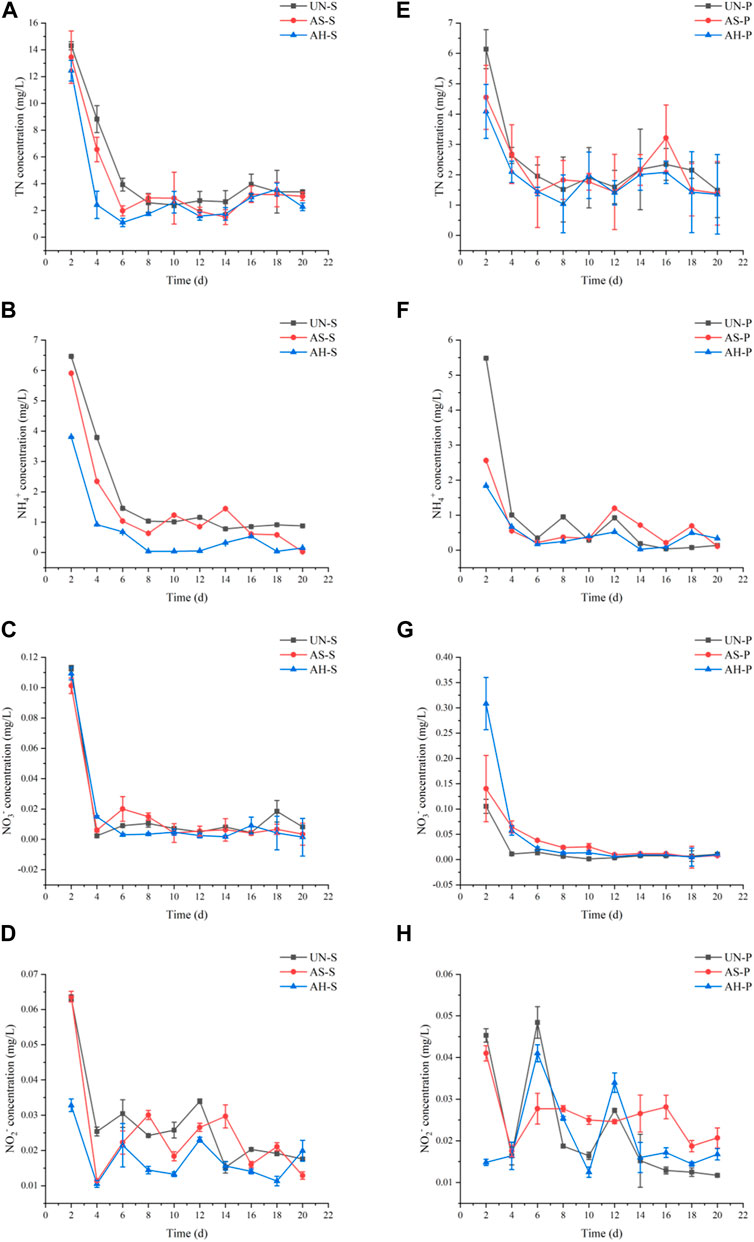
FIGURE 4. N release from S. tabernaemontani (A–D) and P. australis (E–H) under different treatment conditions.
3.2 The impact of carbon source on VFCW performance
A 30 g sample of alkali-heat treated P. australis was added to the VFCW1 system, with the VFCW0 system serving as a control to evaluate the impact of exogenous carbon source addition on the VFCW-mediated treatment of farmland-derived water. The average COD removal rates for VFCW0 and VFCW1 systems were 73.58% and 63.30% (Figure 5). Effluent COD changes for these two systems exhibited similar changes, initially rising for 9 days before slowly decreasing and stabilizing after day 10. The average TN removal rates for the VFCW0 and VFCW1 systems were 43.69% and 71.91%. Average nitrate removal rates for these two VFCWs were 65.19% and 85.27%, respectively, in line with the NH4+-N removal rate trends.
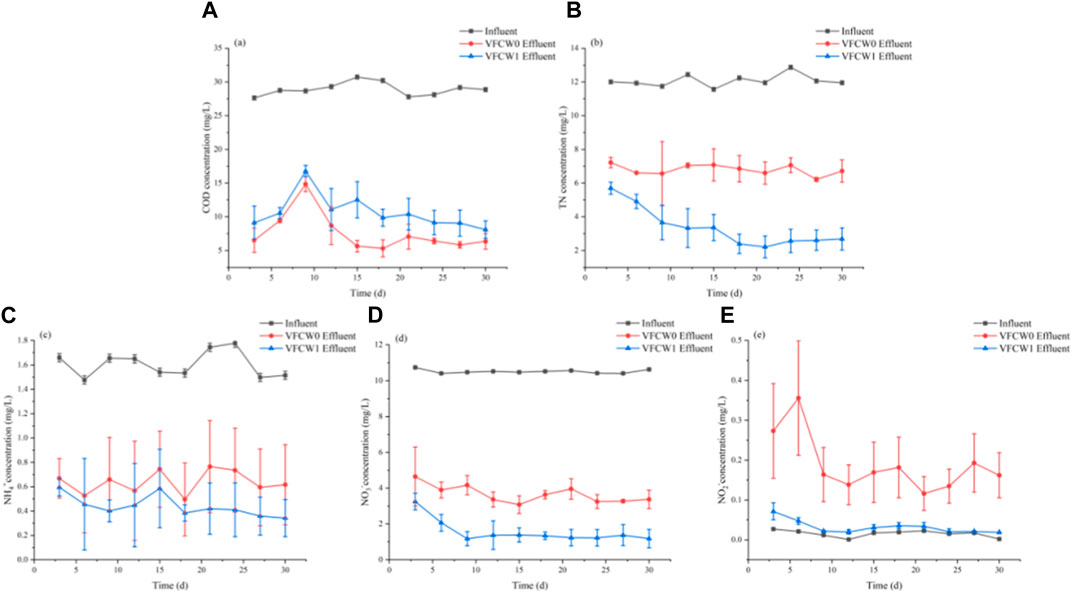
FIGURE 5. The impact of carbon source addition on COD (A), TN (B), NH4+-N (C), NO3−-N (D), and NO2−-N (E) removal in different VFCW systems.
3.3 The impact of plant carbon sources on COD and nitrogen removal at different C/N ratios
The effects of different C/N ratio values on the efficacy of COD and N removal rates for VFCWs to which alkali-heat treated P. australis biomass had been added were next explored. Average COD removal rates at the four tested C/N ratios were 73.58% (C/N = 2, VFCW0), 63.30% (C/N = 4, VFCW1), 55.19% (C/N = 5, VFCW2), and 45.68% (C/N = 7, VFCW3), respectively (Figure 6), with the VFCW0 and VFCW1 groups exhibiting higher removal rates than the two other groups.
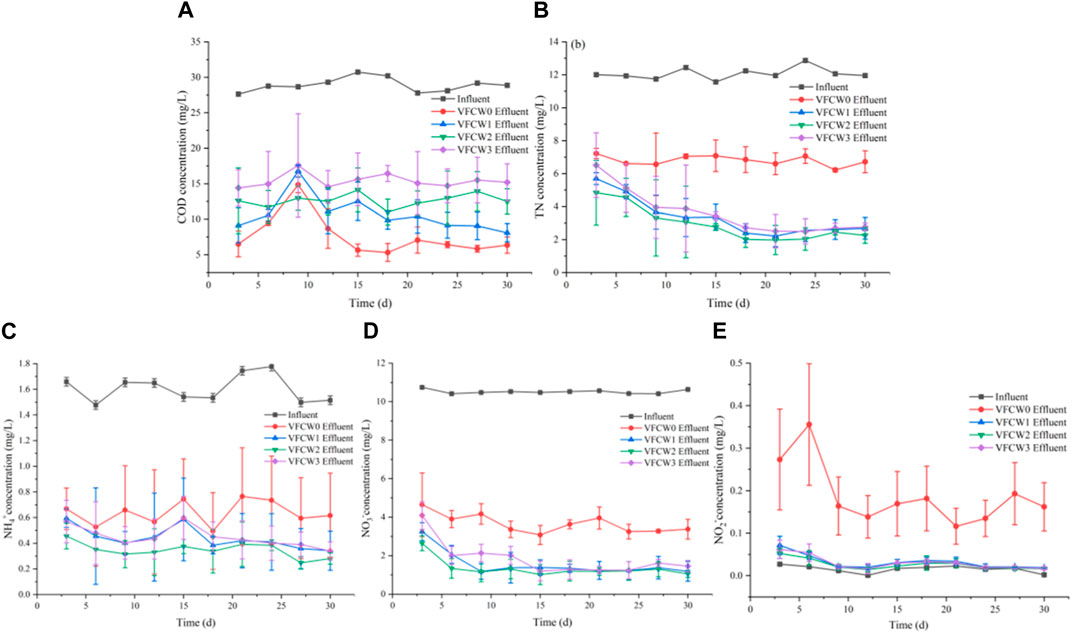
FIGURE 6. The impact of plant carbon sources on COD (A) and N (B–E) removal under different C/N ratios.
Average TN removal rates for these four VFCWs were 43.69%, 71.91%, 75.76%, and 70.06%, with maximal efficacy at a C/N ratio of 5. Average NH4+-N and NO3−-N removal rates across these four VFCW groups were consistent with the observed TN removal trends. NO2−-N removal effects for this system did not differ substantially with carbon source addition. When the C/N ratio was increased to 7, no significant improvements in TN removal rates were observed. From an economic perspective, increasing carbon source levels is not advantageous when seeking to minimize the waste of resources. Based on these results, a C/N ratio of 5 appears to be optimal when using VFCW systems and seeking to maximize N removal efficiency.
3.4 Analyses of the structure of microbial communities in VFCWs
3.4.1 Microbial structure analysis
Microbial nitrification and denitrification are the primary transformative processes responsible for N removal from VFCWs (Mesquita et al., 2017), and bacterial community composition is thus inextricably linked to denitrification performance. Samples of soil sediment from the VFCW0 and VFCW1 systems were thus collected for high-throughput sequencing analyses and the evaluation of functional gene abundance with the goal of determining how plant carbon source addition can improve N removal efficiency. The coverage results for these sequencing analyses indicated that the results were likely to be representative of the actual microbial distributions in both VFCW0 and VFCW1 (Table 1). In total, 5,002 and 5,101 OTUs were detected in the VFCW0 and VFCW1 samples. The Shannon (7.21), ACE (4,787.36), Chao (4,685.71), and Sobs (3,850.67) index values in VFCW1 samples were higher than in VFCW0 samples, consistent with improved microbial diversity and richness following plant carbon source addition. The structure of the microbial community in VFCW1 samples was thus more complex, having been strengthened by the addition of alkali-heat treated P. australis biomass as a source of carbon.
Microbial community composition at the phylum level in the VFCW0 and VFCW1 systems is shown in Figure 7A. The most dominant flora in these two respective systems were Proteobacteria (24.58% and 29.32%), Actinobacteriota (18.01% and 18.79%), Chloroflexi (14.12% and 11.27%), Acidobacteriota (9.27% and 5.98%), Desulfobacterota (7.57% and 7.96%), Bacteroidota (6.12% and 5.96%), and Firmicutes (5.86% and 7.69%).
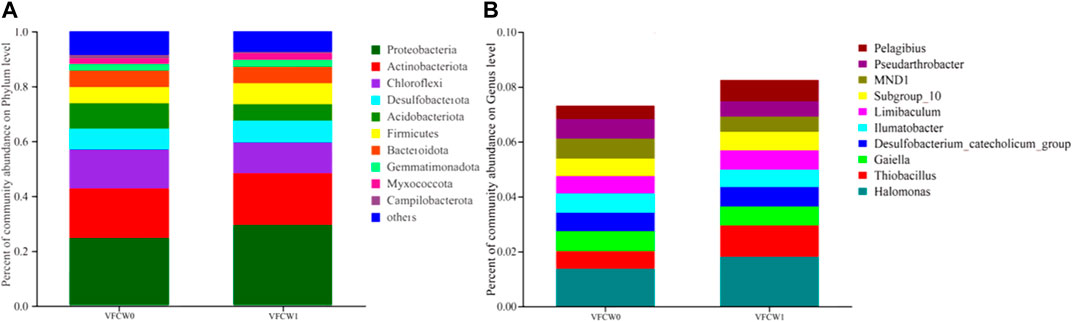
FIGURE 7. The composition of microbial communities in VFCWs. (A) Phylum level community composition (Species with a relative abundance <1% are classified as “others”) (B) Genus level community composition, excluding unnamed and unclassified gener.
The top 10 most abundant genera in these different systems were also evaluated, as shown in Figure 7B.
3.4.2 Microbial correlation network analysis
Ecological networks were next used to analyze the microbial communities in these VFCW systems based on Spearman correlations among genera (relative abundance >0.2%). In total the VFCW1 network included 69 nodes and 741 edges, whereas the VFCW0 network was smaller (58 nodes and 463 edges) (Figure 8).
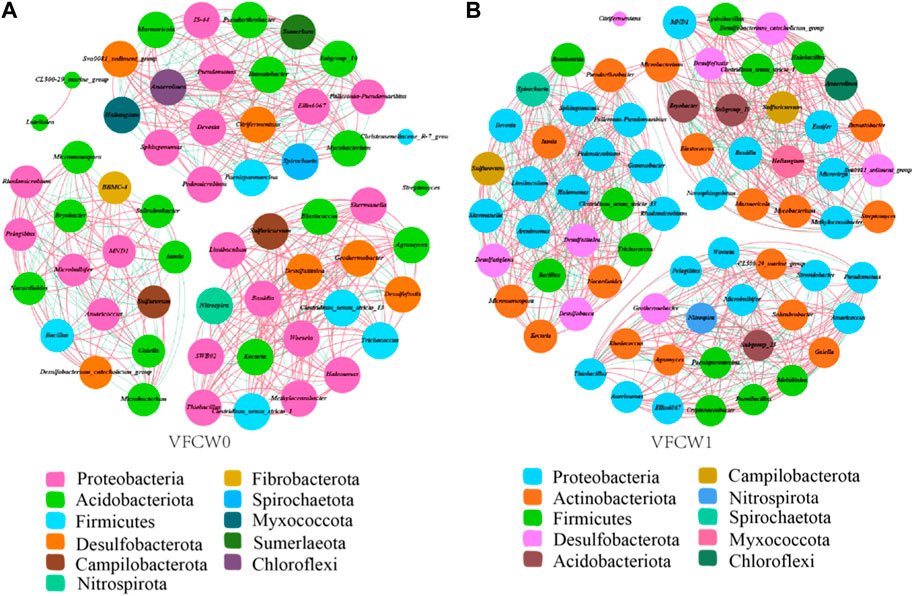
FIGURE 8. Network interactions for the 16S rDNA gene-based bacterial communities in the VFCW0 (A) and VFCW1 (B) system.
3.4.3 The distribution of denitrification-related genes in VFCWs
The distributions of denitrification-related functional genes in these VFCW systems are shown in Figure 9. Specifically, total bacterial abundance (16S rDNA) was analyzed, as was the abundance of nitrifying genes (amoA, nxrA) and a denitrifying gene (nirS).
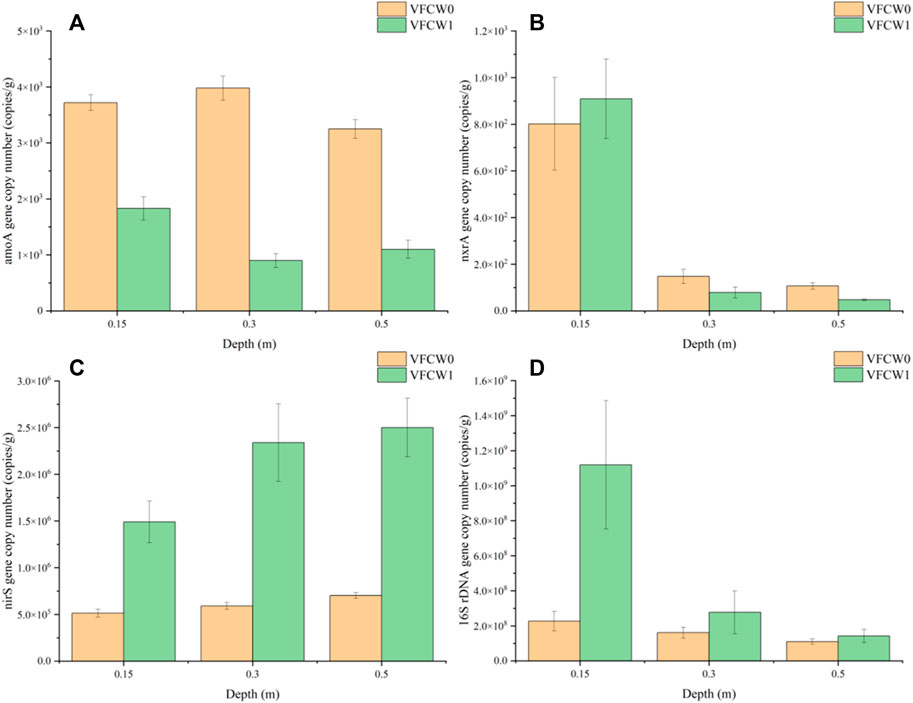
FIGURE 9. Denitrifying microorganism distributions in the VFCWs. (A) amoA, (B) nxrA, (C) nirS, (D) 16S rDNA.
Analyses of nxrA and amoA expression were used to gauge the abundance of microbes involved in the process of nitrification (Dionisi et al., 2002; Hu et al., 2019). amoA encodes an ammonia monooxygenase, which facilitates NH4+-N conversion to NO2−-N (Johnston et al., 2019), while nitrite oxidase (nxrA) facilitates nitrite oxidation (NO2−-N→ NO3−-N) (Poly et al., 2008). The amoA and nxrA distributions in these VFCWs are shown in Figures 9A, B, revealing significantly higher levels of both of these genes in the upper layer of the VFCW0 and VFCW1 systems as compared to the lower layer (p < 0.05), with significantly higher levels in VFCW0 samples as compared to those from VFCW1. Aerobic microbes express amoA and nxrA, and the abundance of amoA and nxrA was significantly increased in the surface layer of these VFCWs relative to the bottom layer, in line with the results published previously by Wang et al. (2020). The abundant plant-derived organic matter in VFCW1 can compete with amoA and nxrA-expressing microbes for dissolved oxygen, inhibiting the growth and proliferation of bacteria expressing amoA and nxrA in the system.
The nirS gene facilitates nitrite reduction and NO2−- N conversion into N2O and NO (Kandeler et al., 2006). The distribution of nirS gene abundance in these VFCWs is shown in Figure 9C, demonstrating that this gene was more abundant in the bottom layers of both the VFCW0 and VFCW1 systems as compared to the surface layer. The abundance of nirS was also significantly higher in VFCW1 as compared to VFCW0 (p < 0.05), which is because that nirS is expressed by heterotrophic microorganisms, heterotrophic microorganisms require large quantities of organic carbon to serve as electron donors in order to maintain microbial viability and to facilitate denitrification (Sukias et al., 2018). The addition of plant biomass to the VFCW1 system as a carbon source was thus conducive to the enhanced growth and proliferation of microbes expressing nirS in this CW system.
Variations in total bacterial load in these VFCW systems are shown in Figure 9D. The largest numbers of bacteria were detected in the upper layers of the VFCW0 and VFCW1 systems, with the total bacteria number in VFCW1 being significantly higher than in VFCW0 (p < 0.05). The abundance of organic matter in VFCW1 can enable rapid microbial growth and reproduction. These plants can also serve as carriers for microbial growth, with the resultant microbial proliferation being conducive to enhanced pollutant transformation and degradation within these CW systems.
4 Discussion
4.1 The choice of plant carbon sources
COD release from both untreated and alkali-treated P. australis and S. tabernaemontani reached maximum levels on day 1 before decreasing rapidly within 4 days and then stabilizing. This is attributable to the fact that water-soluble compounds including sugars, organic acids, and soluble inorganic salts on plant surfaces dissolve relatively rapidly, releasing carbon sources. COD release from S. tabernaemontani samples was initially significantly higher than from P. australis (p < 0.05), likely as the surface of S. tabernaemontani contains higher levels of organic carbon and other organic particles that are readily shed (Chang et al., 2016). Less carbon was released from AH-S samples as compared to UN-S samples, potentially because the relatively soft and fragile nature of S. tabernaemontani samples results in carbon loss upon exposure to high temperatures and alkaline conditions. Conversely, for P. australis samples, greater COD release was observed for alkali-treated samples, with the best release being evident for AH-P samples. Alkali treatment can result in cellulose swelling and reduced cellulose crystallinity, resulting in the breakdown of the structural links between lignin and carbohydrates. Alkaline conditions can also eliminate components that can interfere with cellulose accessibility including acetyl groups, lignin, and furfural acid substitutes (Zheng et al., 2021). The highest and lowest cumulative release levels were observed for AH-P and UN-P samples, respectively, suggesting that alkali-heat treatment can improve carbon source release, making the resultant plant biomass more suitable for addition to VFCWs as an external carbon source.
TN release from these two plant sources occurred in two main phases. Initially, soluble substances from the surface layer are rapidly dissolved, contributing to the release of large quantities of N. During later stages, insoluble substances dissolve more gradually to release N at a steady rate. NH4+ and TN exhibited similar release patterns, with NH4+ exhibiting the highest release ratio in the context of TN release such that it is the primary pollutant of concern. NO3− and NO2− release was limited, and they are not major pollutants of concern when using external carbon sources. N release from alkali-heat treated samples was lower than that from other samples, making these plant biomass samples the most appropriate exogenous carbon source for VFCWs.
4.2 The removal effect after adding plant carbon sources
Effluent COD levels for these two systems were lower than those in the inlet water and relatively stable. VFCW1 exhibited better COD removal, thus confirming that alkali-heat treated P. australis can be added as a plant carbon source in VFCW systems without resulting in any decline in water quality.
During the later stages of the experiment, microbes proliferated substantially and were able to absorb and transform organic matter, with effluent COD concentrations gradually decreasing and stabilizing in line with data published previously by Jia et al. (2020). While VFCW0 also exhibited COD removal activity, the effluent COD concentrations were lower than those for VFCW1 owing to the addition of a plant carbon source to the VFCW1 system. As cellulose and hemicellulose derived from plant biomass are gradually hydrolyzed, organic matter is released and cannot be completely degraded by aerobic microorganisms, leading to higher effluent COD concentrations (Jin et al., 2021).
TN concentrations for VFCW0 remained largely unchanged over the study period, whereas the effluent concentrations for VFCW1 gradually declined, demonstrating that plant carbon source addition significantly improved TN removal efficacy. The C/N ratio for the VFCW0 system was low such that insufficient electron donors were available, suppressing microbial denitrification activity and reducing NO3−-N and NO2−-N removal rates in this system, thus contributing to reductions in TN removal efficiency.
The average ammonia nitrogen rates for these two VFCWs were 60.36% and 71.89%, respectively. Such NH4+-N removal in CW systems primarily results from microbial transformation, plant absorption, substrate adsorption, and volatilization. When the water in these systems has an alkaline pH value, NH4+-N will undergo volatilization, whereas this effect will be negligible at neutral pH values. The higher NH4+-N removal rate in VFCW1 is thus attributable to microbial transformation and utilization. The addition of alkali heat-treated P. australis to VFCW1 was beneficial to the ability of microbes to remove ammonia nitrogen (Lyu et al., 2017; Li et al., 2018), and these alkali-treated plants are also able to remove some NH4+-N from the water via adsorption (Fu et al., 2017), thus yielding overall better ammonia nitrogen removal activity than that observed for VFCW0.
The lower carbon levels and insufficient electron donors available in the VFCW0 system inhibit microbe-mediated denitrification and lower the NO3−-N removal efficiency of CW systems (Ma et al., 2020).
Yan et al. (2022) demonstrated that in the absence of a carbon source, the denitrification process stalls at the NO2−-N stage such that NO2-N accumulates, as NO3−-N can inhibit denitrification, bacterial intracellular nitrite reductase activity and NO2−-N reduction rates decline. Insufficient carbon availability is thus a key driver of NO2−-N accumulation. In the VFCW1 system, NO2−-N accumulation was minimal owing to the abundance of available carbon, with the alkali-heat treatment of P. australis strengthening microbial denitrification and nitrite removal efficacy for the VFCW1 system. NO2−-N accumulated at significantly higher levels in VFCW0 relative to VFCW1 (p < 0.05), suggesting that plant carbon source addition was conducive to complete denitrification, with the majority of the NO2−-N in the VFCW1 system undergoing conversion and removal.
4.3 Microbial community analysis
Proteobacteria were thus the most abundant in both of these systems, as has also been shown in prior studies of denitrification in VFCWs (Chen et al., 2015; Sánchez et al., 2017; Qiu et al., 2021). Members of the Proteobacteria phylum can facilitate C and N transformation and their relatively high levels of abundance can improve COD and N removal rates when the influent C/N ratio is relatively low (Chen et al., 2019; Sun, et al., 2019; Guo et al., 2020). Most β-Proteobacteria are capable of degrading macromolecular organic matter (Jiang et al., 2020), and many α-, β-, and γ-proteobacteria are involved in denitrification (Lu et al., 2013). The second most abundant bacterial phylum in these VFCW systems was Actinobacteriota. Ward et al. (2009) demonstrated that Acterinobacteriota species can utilize carbon sources and reduce nitrate to nitrite, thus facilitating nitrogen removal. Chloroflexi was the third most dominant phylum in these samples, and He et al. (2016) demonstrated that Chloroflexi species are dominant in VFCWs, suggesting that they may be key mediators of pollutant removal in these systems. Significant increases in the relative abundance of major denitrifying bacteria including Proteobacteria and Firmicutes were observed in VFCW1 as a result of plant carbon source addition relative to VFCW0 (p < 0.05). Increases in Proteobacteria abundance can enhance nitrogen removal efficiency and reduce N2O emissions. As Firmicutes species are capable of decomposing cellulose and hemicellulose into smaller components of organic matter (Chu and Wang, 2016), increased Firmicutes abundance in the VFCW1 system is conducive to organic matter removal. Plant biomass can thus be effectively broken down in VFCWs, accelerating N transformation and removal through nitrification and denitrification processes.
Man et al. (2021) found demonstrated that Halomonas microorganisms can achieve removal efficiency rates of over 85% for a single nitrogen source in sewage samples, with removal rates of 45% for various nitrogen sources. Thiobacillus species have been shown to be capable of simultaneously eliminating both nitrogen and sulfur (Zhang et al., 2022). Halomonas and Thiobacillus were detected in both of these VFCWs and were more abundant in VFCW1, indicating that carbon source addition can enrich the abundance of genera associated with denitrification. MND1 is an enriched member of the Nitrosomonas family, corresponding to a taxonomic unit that contains ammonia-oxidizing bacteria (Yu et al., 2021). Pseudarthrobacter are often present in environments with high levels of dissolved oxygen (Lennon et al., 2012), likely explaining the observed reduction in Pseudarthrobacter abundance in VFCW1 owing to the fact that carbon source addition resulted in competition for dissolved oxygen.
The VFCW1 network was more modular than the VFCW0 network, indicating greater stability for the VFCW1 microbial communities. The dominant genera Thiobacillus and Halomonas were connected to many other genera in these networks, highlighting their likely importance in this ecological context. Both Thiobacillus and Halomonas exhibited 18 connections in the VFCW0 network, including 14 positive correlations and 4 negative correlations. Halomonas was associated with 24 connections in the VFCW1 network, including 13 positive correlations and 11 negative correlations. Thiobacillus was associated with 20 connections in the VFCW1 network, including 12 positive correlations and 8 negative correlations. Negative correlation ratios for these two genera were larger in VFCW1, potentially owing to a reduction in the abundance of genera that failed to adapt to high C/N ratio values following carbon source addition such that they were negatively correlated with Thiobaillus and Halomonas.
4.4 Analyses of the microbial denitrification pathways active in VFCWs
The microbe-mediated denitrification pathways active in these VFCW systems are outlined in Figure 10. The ammonia monooxygenase (amoA), nitrite oxidoreductase (nxrA), and nitrite reductase (nirS) functional genes play roles in the processes of systemic nitrification and denitrification. Ji et al. (2013) demonstrated that in CW systems, the abundance of amoA, nxrA, and denitrifying bacteria are largely equivalent such that this system can facilitate both nitrification and denitrification reactions. However, in the present study the most abundant analyzed functional gene was nirS (2.11 × 106 copies/g), followed by amoA (1.27 × 103 copies/g), with nxrA being least abundant (3.45 × 102 copies/g), suggesting that denitrifying bacteria have an advantage when competing for NO2−-N, converting this N source into gaseous nitrogen (GN2), that is ultimately discharged from the wetland system. Jia et al. (2021) designed and studied a carbon self-sufficient vertical subsurface flow CW system and observed respective amoA, nxrA, and nirS gene abundances of 3.11 × 103, 5 × 102, and 7.23 × 106 copies/g in their system. In their studies of VFCWs, Fu et al. (2016) observed respective mean amoA, nxrA, and nirS gene abundances of 4.17 × 105, 6.85 × 102, and 7.50 × 107 copies/g, in line with the present results. The amoA gene enables NH4+-N conversion into NO2−-N, thereby promoting nitrification in VFCW systems, while nirS catalyzes NO2−-N conversion into gaseous nitrogen, thereby mediating denitrification. The full denitrification process is: NH4+-N→NO3−-N→NO2−-N→N2. Given the low measured abundance of nxrA, short-range nitrification and denitrification is likely to be the dominant nitrogen removal pathway in this VFCW system. The quantified expressions are:
5 Conclusion
In summary, the present results suggest that following alkali-heat treatment, P. australis biomass can continuously release carbon, enhancing the denitrification effects for VFCW systems with an average TN removal rate of up to 75.67%. The optimal C/N ratio for such carbon source addition in this study was 5, given that this value yielded the best denitrification activity. Additional plant carbon sources also contributed to higher levels of microbial community diversity and richness in these CWs. At the phylum level, the dominant microbes included Proteobacteria, Actinobacteria, and Chloroflexi, with Thiobaillus and Halomonas species playing important roles in the microbe-mediated denitrification activity of VFCWs. In the presence of sufficient carbon, these microbes exhibit robust denitrification activity. Of the analyzed VFCW systems, VFCW1 exhibited greater levels of microbial abundance and stronger microbial community network correlation values. Genes associated with aerobic microorganisms (amoA, nxrA) were primarily distributed in the upper layer of the VFCW system whereas genes associated with anaerobic microorganisms (nirS) were primarily detected in the lower layer of this system, highlighting the competitive and synergistic ecological relationships among these groups of microbes. Various nitrogen sources in this system were ultimately removed through short-range nitrification and denitrification. The lack of carbon source in the constructed wetland is not conducive to denitrification. In this study, the nitrogen removal rate increased after adding the carbon source of alkali heat treated reed plants. From the economic point of view, P. australis is the main water plant of Ulansuhai, which is easy to obtain, so it is feasible to use constructed wetland with plant carbon source to treat farmland drainage of the upstream of Ulansuhai.
Data availability statement
The raw data supporting the conclusion of this article will be made available by the authors, without undue reservation.
Author contributions
LnW: Conceptualization, Project administration, Funding acquisition. YL: Writing and editing. XL: Investigation, Formal analysis, Writing—Materials and methods. MZ: Investigation. XD: Data curation, Investigation. HL: Investigation. LxW: Supervision, Project administration. All authors contributed to the article and approved the submitted version.
Funding
This work was supported by the National Natural Science Foundation of China (NSFC) (Nos 42067037, 41701281, 51869014), Science and Technology Major Project on Lakes of Inner Mongolia (No. ZDZX2018054), Science and Technology Major Project of Inner Mongolia (No.2022YFHH0017), and National Natural Science Funds, P.R. China (No. 32160279, 32161143025).
Conflict of interest
The authors declare that the research was conducted in the absence of any commercial or financial relationships that could be construed as a potential conflict of interest.
Publisher’s note
All claims expressed in this article are solely those of the authors and do not necessarily represent those of their affiliated organizations, or those of the publisher, the editors and the reviewers. Any product that may be evaluated in this article, or claim that may be made by its manufacturer, is not guaranteed or endorsed by the publisher.
References
Butkovskyi, A., Jing, Y., Bergheim, H., Lazar, D., Gulyaeva, K., Odenmarck, S. R., et al. (2021). Retention and distribution of pesticides in planted filter microcosms designed for treatment of agricultural surface runoff. Sci. Total Environ. 778, 146114. doi:10.1016/j.scitotenv.2021.146114
Cai, W., Li, Y., Niu, L., Zhang, W., Wang, C., Wang, P., et al. (2017). New insights into the spatial variability of biofilm communities and potentially negative bacterial groups in hydraulic concrete structures. Water Res. 123, 495–504. doi:10.1016/j.watres.2017.06.055
Chand, N., Kumar, K., and Suthar, S. (2022). Enhanced wastewater nutrients removal in vertical subsurface flow constructed wetland: Effect of biochar addition and tidal flow operation. Chemosphere 286, 131742. doi:10.1016/j.chemosphere.2021.131742
Chang, Y., Wang, T., and Wang, H. Y. (2016). The long-term nitrogen removal efficiency from agricultural runoff in Phragmites australis packed surface flow constructed wetland[J]. J. Environ. Eng. Tecnnol. 6 (5), 453–461.
Chen, D., Gu, X., Zhu, W., He, S., Huang, J., and Zhou, W. (2019). Electrons transfer determined greenhouse gas emissions in enhanced nitrogen-removal constructed wetlands with different carbon sources and carbon-to-nitrogen ratios. Bioresour. Technol. 285, 121313. doi:10.1016/j.biortech.2019.121313
Chen, Y., Wen, Y., Tang, Z., Huang, J., Zhou, Q., and Vymazal, J. (2015). Effects of plant biomass on bacterial community structure in constructed wetlands used for tertiary wastewater treatment. Ecol. Eng. 84, 38–45. doi:10.1016/j.ecoleng.2015.07.013
Chu, L., and Wang, J. (2016). Denitrification of groundwater using PHBV blends in packed bed reactors and the microbial diversity. Chemosphere 155, 463–470. doi:10.1016/j.chemosphere.2016.04.090
Dionisi, H. M., Layton, A. C., Harms, G., Gregory, I. R., Robinson, K. G., and Sayler, G. S. (2002). Quantification of nitrosomonas oligotropha-like ammonia-oxidizing bacteria and Nitrospira spp. from full-scale wastewater treatment plants by competitive PCR. Appl. Environ. Microbiol. 68, 245–253.
Fu, G., Huangshen, L., Guo, Z., Zhou, Q., and Wu, Z. (2017). Effect of plant-based carbon sources on denitrifying microorganisms in a vertical flow constructed wetland. Bioresour. Technol. 224, 214–221. doi:10.1016/j.biortech.2016.11.007
Fu, G., Yu, T., Ning, K., Guo, Z., and Wong, M. H. (2016). Effects of nitrogen removal microbes and partial nitrification-denitrification in the integrated vertical-flow constructed wetland. Ecol. Eng. 95, 83–89. doi:10.1016/j.ecoleng.2016.06.054
Gordon, B. A., Lenhart, C., Peterson, H., Gamble, J., Nieber, J., Current, D., et al. (2021). Reduction of nutrient loads from agricultural subsurface drainage water in a small, edge-of-field constructed treatment wetland. Ecol. Eng. 160, 106128. doi:10.1016/j.ecoleng.2020.106128
Guo, F., Zhang, J., Yang, X., He, Q., Ao, L., and Chen, Y. (2020). Impact of biochar on greenhouse gas emissions from constructed wetlands under various influent chemical oxygen demand to nitrogen ratios. Bioresour. Technol. 303, 122908. doi:10.1016/j.biortech.2020.122908
He, T., Guan, W., Luan, Z., and Xie, S. (2016). Spatiotemporal variation of bacterial and archaeal communities in a pilot-scale constructed wetland for surface water treatment. Appl. Microbiol. Biotechnol. 100, 1479–1488. doi:10.1007/s00253-015-7072-5
Hu, Q., Yang, Y., Han, S., and Wang, J. (2019). Degradation of agricultural drainage water quantity and quality due to farmland expansion and water-saving operations in arid basins. Agric. Water Manag. 213, 185–192. doi:10.1016/j.agwat.2018.10.019
Jabbar, F. K., and Grote, K. (2019). Statistical assessment of nonpoint source pollution in agricultural watersheds in the Lower Grand River watershed, MO, USA[J]. Environ. Sci. Pollut. Res. 26, 1487–1506.
Johnston, J., Lapara, T., and Behrens, S. (2019). Composition and dynamics of the activated sludge microbiome during seasonal nitrification failure. Sci. Rep. 9, 4565. doi:10.1038/srep4565
Ji, G., He, C., and Tan, Y. (2013). The spatial distribution of nitrogen removal functional genes in multimedia biofilters for sewage treatment. Ecol. Eng. 55, 35–42. doi:10.1016/j.ecoleng.2013.02.009
Jia, L., Li, C., Zhang, Y., Chen, Y., Li, M., Wu, S., et al. (2020). Microbial community responses to agricultural biomass addition in aerated constructed wetlands treating low carbon wastewater. J. Environ. Manag. 270, 110912. doi:10.1016/j.jenvman.2020.110912
Jia, W., Yang, Y., Yang, L., and Gao, Y. (2021). High-efficient nitrogen removal and its microbiological mechanism of a novel carbon self-sufficient constructed wetland. Sci. Total Environ. 775, 145901. doi:10.1016/j.scitotenv.2021.145901
Jiang, L., Wu, A., Fang, D., Zhang, Y., Shen, Q., Xu, X., et al. (2020). Denitrification performance and microbial diversity using starch-polycaprolactone blends as external solid carbon source and biofilm carriers for advanced treatment. Chemosphere 255, 126901. doi:10.1016/j.chemosphere.2020.126901
Jin, M., Shi, Y., Du, X., Wu, S., Wang, L., Qiao, N., et al. (2021). High temperature treatment of hemicellulose in pulp-impregnated effluent improves the pre-hydrolysis efficiency. New J. Chem. 45 (21), 9629–9635. doi:10.1039/d1nj00536g
Kaandorp, V. P., Broers, H. P., Van Der Velde, Y., Rozemeijer, J., and de Louw, P. G. B. (2021). Time lags of nitrate, chloride, and tritium in streams assessed by dynamic groundwater flow tracking in a lowland landscape[J]. Hydrol. Earth. Syst. Sci. 25 (6), 3691–3711.
Kandeler, E., Deiglmayr, K., Tscherko, D., Bru, D., and Philippot, L. (2006). Abundance of narG, nirS, nirK, and nosZ genes of denitrifying bacteria during primary successions of a glacier foreland. Appl. Environ. Microbiol. 72 (9), 5957–5962. doi:10.1128/aem.00439-06
Lavrnić, S., Braschi, I., Anconelli, S., Blasioli, S., Solimando, D., Mannini, P., et al. (2018). Long-term monitoring of a surface flow constructed wetland treating agricultural drainage water in Northern Italy[J]. Water 10 (5), 644
Leng, P., Zhang, Q., Li, F., Kulmatov, R., Wang, G., Qiao, Y., et al. (2021). Agricultural impacts drive longitudinal variations of riverine water quality of the aral sea basin (amu darya and syr darya rivers), central asia. Environ. Pollut. 284, 117405. doi:10.1016/j.envpol.2021.117405
Lennon, J. T., Aanderud, Z. T., Lehmkuhl, B. K., Hu, Z., Liang, S., and Schoolmaster, D. R. (2018). Mapping the niche space of soil microorganisms using taxonomy and traits[J]. Ecology 93 (8), 1867–1879.
Li, J., Fan, J., Zhang, J., Hu, Z., and Liang, S. (2018). Preparation and evaluation of wetland plant-based biochar for nitrogen removal enhancement in surface flow constructed wetlands. Environ. Sci. Pollut. Res. 25, 13929–13937. doi:10.1007/s11356-018-1597-y
Li, W., Zhai, L., Lei, Q., Wollheim, W. M., Liu, J., Liu, H., et al. (2018). Influences of agricultural land use composition and distribution on nitrogen export from a subtropical watershed in China. Sci. Total Environ. 642, 21–32. doi:10.1016/j.scitotenv.2018.06.048
Lu, P., Chen, C., Wang, Q., Wang, Z., Zhang, X., and Xie, S. (2013). Phylogenetic diversity of microbial communities in real drinking water distribution systems. Biotechnol. Bioprocess Eng. 18, 119–124. doi:10.1007/s12257-012-0230-z
Lyu, W., Huang, L., Xiao, G., and Chen, Y. (2017). Effects of carbon sources and COD/N ratio on N2O emissions in subsurface flow constructed wetlands. Bioresour. Technol. 245, 171–181. doi:10.1016/j.biortech.2017.08.056
Ma, L., Liu, W., Tan, Q., Zhou, Q., Wu, Z., and He, F. (2019). Quantitative response of nitrogen dynamic processes to functional gene abundances in a pond-ditch circulation system for rural wastewater treatment. Ecol. Eng. 134, 101–111. doi:10.1016/j.ecoleng.2019.05.008
Ma, Y., Zheng, X., Fang, Y., Xu, K., He, S., and Zhao, M. (2020). Autotrophic denitrification in constructed wetlands: Achievements and challenges. Bioresour. Technol. 318, 123778. doi:10.1016/j.biortech.2020.123778
Man, Q., Zhang, P., Huang, W., Zhu, Q., He, X., and Wei, D. (2022). A heterotrophic nitrification-aerobic denitrification bacterium Halomonas venusta TJPU05 suitable for nitrogen removal from high-salinity wastewater. Front. Environ. Sci. Eng. 16, 69–10. doi:10.1007/s11783-021-1503-6
Mesquita, M. C., Albuquerque, A., Amaral, L., and Nogueira, R. (2017). Seasonal variation of nutrient removal in a full-scale horizontal constructed wetland. Energy Procedia 136, 225–232. doi:10.1016/j.egypro.2017.10.246
Nuen, J., Li, S., Cheng, L., and Swan, L. (2020). Analysis of nonpoint source pollution load in Ulansuhai Nur basin. Arabian J. Geosciences 13, 1138–1147. doi:10.1007/s12517-020-06144-6
Poly, F., Wertz, S., Brothier, E., and Degrange, V. (2008). First exploration of Nitrobacter diversity in soils by a PCR cloning-sequencing approach targeting functional gene nxrA. FEMS Microbiol. Ecol. 63 (1), 132–140. doi:10.1111/j.1574-6941.2007.00404.x
Qiu, Z., Zhang, S., Ding, Y., Zhang, W., Gong, L., Yuan, Q., et al. (2021). Comparison of Myriophyllum Spicatum and artificial plants on nutrients removal and microbial community in constructed wetlands receiving WWTPs effluents. Bioresour. Technol. 321,124469. doi:10.1016/j.biortech.2020.124469
Sánchez, O. (2017). Constructed wetlands revisited: Microbial diversity in the–omics era. Microb. Ecol. 73 (3), 722–733. doi:10.1007/s00248-016-0881-y
Sandström, S., Futter, M. N., O'Connell, D. W., Lannergård, E. E., Rakovic, J., Kyllmar, K., et al. (2021). Variability in fluvial suspended and streambed sediment phosphorus fractions among small agricultural streams. J. Environ. Qual. 50 (3), 612–626. doi:10.1002/jeq2.20210
State Environmental Protection Administration of China (2002). Water and wastewater monitoring methods. 4th ed. Beijing, China: Chinese Environmental Science-Publishing Press.
Sukias, J. P., Park, J. B., Stott, R., and Tanner, C. C. (2018). Quantifying treatment system resilience to shock loadings in constructed wetlands and denitrification bioreactors. Water Res. 139, 450–461. doi:10.1016/j.watres.2018.04.010
Sun, G., Wan, J., Sun, Y., Li, H., Chang, C., and Wang, Y. (2019). Enhanced removal of nitrate and refractory organic pollutants from bio-treated coking wastewater using corncobs as carbon sources and biofilm carriers. Chemosphere 237, 124520. doi:10.1016/j.chemosphere.2019.124520
Sun, Y., Guan, Y., Wang, H., and Wu, G. (2019). Autotrophic nitrogen removal in combined nitritation and Anammox systems through intermittent aeration and possible microbial interactions by quorum sensing analysis. Bioresour. Technol. 272, 146–155. doi:10.1016/j.biortech.2018.10.017
Wang, L., Pang, Q., Peng, F., Zhang, A., Zhou, Y., Lian, J., et al. (2020). Response characteristics of nitrifying bacteria and archaea community involved in nitrogen removal and bioelectricity generation in integrated tidal flow constructed wetland-microbial fuel cell. Front. Microbiol. 11, 1385. doi:10.3389/fmicb.2020.01385
Ward, N. L., Challacombe, J. F., Janssen, P. H., Henrissat, B., Coutinho, P. M., Wu, M., et al. (2009). Three genomes from the phylum Acidobacteria provide insight into the lifestyles of these microorganisms in soils. Appl. Environ. Microbiol. 75 (7), 2046–2056. doi:10.1128/aem.02294-08
Wu, S., Gao, L., Gu, J., Zhou, W., Fan, C., He, S., et al. (2018). Enhancement of nitrogen removal via addition of cattail litter in surface flow constructed wetland. J. Clean. Prod. 204, 205–211. doi:10.1016/j.jclepro.2018.09.036
Xiong, R., Yu, X., Zhang, Y., Peng, Z., Yu, L., Cheng, L., et al. (2020). Comparison of agricultural wastes and synthetic macromolecules as solid carbon source in treating low carbon nitrogen wastewater. Sci. total Environ. 739, 139885. doi:10.1016/j.scitotenv.2020.139885
Yan, C., Huang, J., Wang, Y., Lin, X., Cao, C., and Qian, X. (2022). Assessment on the treatment of nitrogen contaminant by constructed wetland exposed to different concentrations of graphene oxide. J. Clean. Prod. 338, 130567. doi:10.1016/j.jclepro.2022.130567
Yu, M., Su, W. Q., Huang, L., Parikh, S. J., Tang, C., Dahlgren, R. A., et al. (2021). Bacterial community structure and putative nitrogen-cycling functional traits along a charosphere gradient under waterlogged conditions. Soil Biol. Biochem. 162, 108420. doi:10.1016/j.soilbio.2021.108420
Zhang, R. C., Chen, C., Xu, X. J., Lee, D. J., and Ren, N. Q. (2022). The interaction between Pseudomonas C27 and Thiobacillus denitrificans in the integrated autotrophic and heterotrophic denitrification process. Sci. Total Environ. 811, 152360. doi:10.1016/j.scitotenv.2021.152360
Zhang, T., Xu, D., He, F., Zhang, Y., and Wu, Z. (2012). Application of constructed wetland for water pollution control in China during 1990–2010. Ecol. Eng. 47, 189–197. doi:10.1016/j.ecoleng.2012.06.022
Zheng, Y., Cao, T., Zhang, Y., Xiong, J., Dzakpasu, M., Yang, D., et al. (2021). Characterization of dissolved organic matter and carbon release from wetland plants for enhanced nitrogen removal in constructed wetlands for low C–N wastewater treatment. Chemosphere 273, 129630. doi:10.1016/j.chemosphere.2021.129630
Zhi, W., and Ji, G. (2014). Quantitative response relationships between nitrogen transformation rates and nitrogen functional genes in a tidal flow constructed wetland under C/N ratio constraints. Water Res. 64, 32–41. doi:10.1016/j.watres.2014.06.035
Keywords: vertical flow constructed wetland, plant carbon source, nitrogen removal, influent C/N ratio, microbiological mechanisms
Citation: Lin Y, Lu X, Zhao M, Ding X, Lv H, Wang L and Wu L (2023) The impact of external plant carbon sources on nitrogen removal and microbial community structure in vertical flow constructed wetlands. Front. Environ. Sci. 11:1233422. doi: 10.3389/fenvs.2023.1233422
Received: 05 June 2023; Accepted: 15 September 2023;
Published: 04 October 2023.
Edited by:
Hongbin Yin, Chinese Academy of Sciences (CAS), ChinaReviewed by:
Shiyang Zhang, Wuhan University of Technology, ChinaHaile Yang, Shanghai Aquatic Wildlife Conservation and Research Center, China
Copyright © 2023 Lin, Lu, Zhao, Ding, Lv, Wang and Wu. This is an open-access article distributed under the terms of the Creative Commons Attribution License (CC BY). The use, distribution or reproduction in other forums is permitted, provided the original author(s) and the copyright owner(s) are credited and that the original publication in this journal is cited, in accordance with accepted academic practice. No use, distribution or reproduction is permitted which does not comply with these terms.
*Correspondence: Linhui Wu, imuwulinhui@163.com; Lixin Wang, lLx_wimu@163.com