- 1State Key Laboratory of Severe Weather and Key Laboratory of Atmospheric Chemistry of CMA, Chinese Academy of Meteorological Sciences, Beijing, China
- 2Institute of Atmospheric Environment, China Meteorological Administration, Shenyang, China
- 3Laboratoire de Physique et Chimie de l’Environnement et de l’Espace (LPC2E), CNRS–Université Orléans–CNES, Orléans, France
- 4Max Planck Institute for Chemistry, Mainz, Germany
Editorial on the Research Topic
Physical and chemical processes within the planetary boundary layer and their impacts on air pollution
1 Introduction
Air pollution is a critical environmental problem that arises from diverse natural and anthropogenic emissions and poses significant threats to public health (Bu et al., 2021), ecosystems (Hu et al.), and climate change (Bellouin et al., 2020). Despite some progress in reducing emissions and improving air quality in certain regions, many parts of the world still experience dangerous levels of pollution (Sicard et al., 2023).
The planetary boundary layer (PBL) forms the lowest layer of the troposphere and is a key player in the complex dynamics of air pollution through various physical and chemical processes (Stull, 1988). A comprehensive understanding of these processes is critical to devising effective strategies to mitigate air pollution. This entails the optimization of emission reduction measures, as well as the development of accurate air quality forecasting models. Such knowledge can inform sound public policy decisions aimed at reducing air pollution levels and safeguarding public health.
2 Physical processes
The PBL is a highly intricate and multi-scale system, involving a variety of physical processes (Serafin et al., 2018; Miao et al., 2022), such as turbulence, convection, radiation, and advection. The exchange of heat, moisture, and momentum between the surface and the atmosphere is largely governed by the thermal stratification of the PBL and its underlying surface characteristics (Stull, 1988). This exchange strongly influences the transport and dispersion of air pollutants, including aerosols and trace gases, from the surface to the upper troposphere.
Turbulence is the dominant feature of the PBL and is responsible for mixing and transporting heat, moisture, and pollutants (Wei et al.). Convection, on the other hand, is responsible for the vertical transport of heat and moisture (Margairaz et al., 2020). Radiation plays a significant role in determining the energy balance of the PBL (Lee, 2018) and its interaction with aerosols (Xiong et al.). Meanwhile, advection involves the transport of air masses horizontally (Miao et al., 2022), and certain synoptic conditions and PBL dynamics can result in the long-range transport of pollutants (Colarco et al., 2004; Xiao et al., 2020).
The height and stability of the PBL determine the concentration of pollutants at the surface (Miao and Liu, 2019) and regulate their vertical distribution (Bourgeois et al., 2018; Banerjee et al., 2022). Therefore, understanding the dynamics and physical processes of the PBL is crucial in predicting air pollutant concentrations and developing effective air pollution mitigation strategies. For example, the interaction between aerosols and the PBL (Wang et al., 2022) is a critical factor in air pollution formation and dispersion. High concentrations of aerosols can enhance the stability of the PBL, resulting in decreased PBL height and exacerbating pollution levels (Petäjä et al., 2016). Additionally, aerosols can affect cloud adjustments and microphysical processes, leading to changes in the PBL height and the concentration of pollutants (Xiong et al.).
3 Chemical processes
Chemical processes within the PBL are the critical drivers in affecting the composition and concentration of atmospheric pollutants (Wang et al., 2017). Reactions between pollutants (Ma et al., 2012), including nitrogen oxides (NOx) and volatile organic compounds (VOCs), with the involvement of sunlight and other atmospheric compounds such as oxygen, can lead to the formation of secondary pollutants such as photo oxidants and aerosols. One of the most crucial processes is the photochemical production of ozone (O3) (Wang et al.), which occurs during the VOCs degradation process with the presence of NOx and sunlight (Du et al.). Additionally, the transformation of primary gaseous pollutants, including nitrogen monoxide (NO) and sulfur dioxide (SO2), into secondary particle pollutants (Wang et al.) such as nitric acid (HNO3) and sulfate aerosols (SO4) is another critical process. The other category triggering this transformation is atmospheric oxidants, including hydroxyl radicals (OH), O3, and nitrate radicals (NO3), and heterogeneous reactions, which are generated through various photochemical reactions (Brown and Stutz, 2012; Wang et al., 2017; Ye et al., 2022).
Meteorological conditions such as air temperature, humidity, and wind speed influence these chemical processes (Li et al.; Wang et al.). For example, higher temperatures and stronger solar radiation accelerate the photochemical reactions, leading to a higher production rate of O3 and other secondary pollutants, and finally causing photochemical pollution. In contrast, increased wind speed and turbulence can enhance the mixing and dispersion of pollutants within the PBL, leading to lower concentrations of these compounds distributed near the surface.
Thereby, understanding the mechanisms behind these PBL chemical processes and their links with meteorological conditions is crucial for predicting and mitigating air pollution.
4 Summary
In short, the physical and chemical processes occurring within the PBL have profound effects on air pollution (Table 1), and it is essential to comprehend these processes thoroughly. To achieve this goal, it is necessary to conduct extensive research, including field measurements (e.g., Guo et al.), laboratory experiments (e.g., Hao et al.), and model simulations (e.g., Ai et al.). Such research will enable us to develop a comprehensive understanding of the sources, transformations, and transport of atmospheric pollutants, benefitting the development of future air pollution mitigation strategies and further reducing adverse effects on human and ecosystem health.
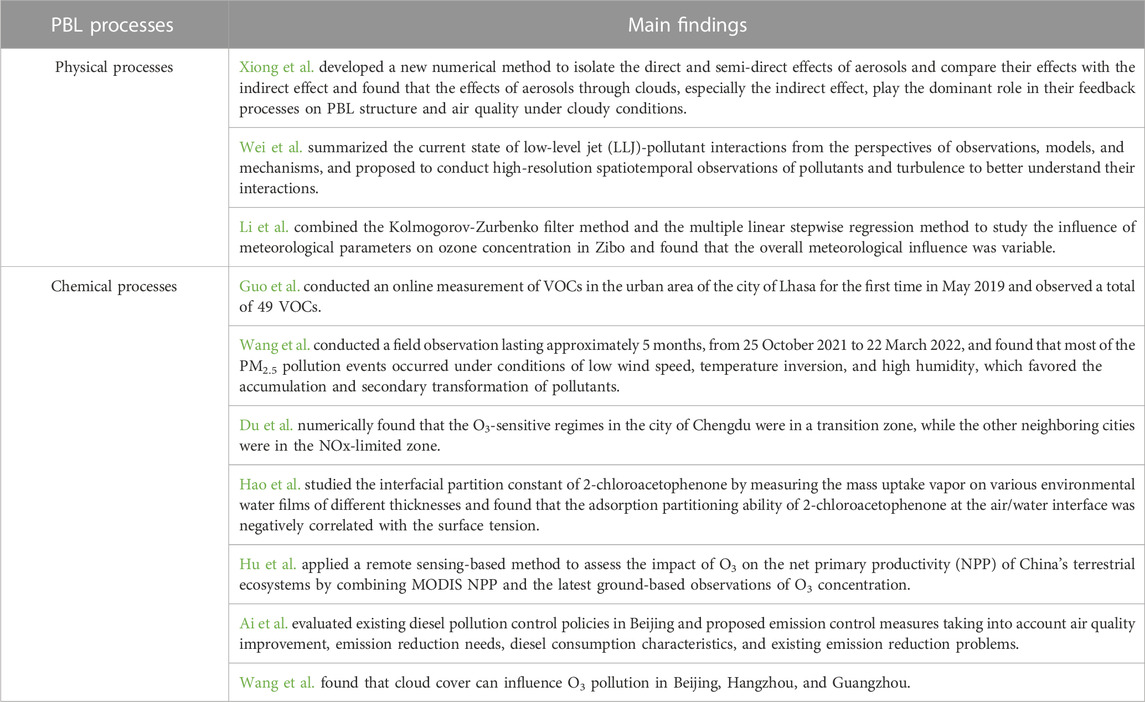
TABLE 1. Main findings from the published research papers within the Research Topic on “Physical and Chemical Processes within the Planetary Boundary Layer and Their Impacts on Air Pollution.”
Author contributions
YM and GZ wrote the editorial with inputs from XL and CX. All authors contributed to the article and approved the submitted version.
Funding
This work was supported by the National Natural Science Foundation of China (42275198) and China Scholarship Council (202205330018).
Conflict of interest
The authors declare that the research was conducted in the absence of any commercial or financial relationships that could be construed as a potential conflict of interest.
Publisher’s note
All claims expressed in this article are solely those of the authors and do not necessarily represent those of their affiliated organizations, or those of the publisher, the editors and the reviewers. Any product that may be evaluated in this article, or claim that may be made by its manufacturer, is not guaranteed or endorsed by the publisher.
References
Banerjee, T., Anchule, A., Sorek-Hamer, M., and Latif, M. T. (2022). Vertical stratification of aerosols over South Asian cities. Environ. Pollut. 309, 119776.
Bellouin, N., Quaas, J., Gryspeerdt, E., Kinne, S., Stier, P., Watson-Parris, D., et al. (2020). Bounding global aerosol radiative forcing of climate change. Rev. Geophys. 58, 660. doi:10.1029/2019RG000660
Bourgeois, Q., Ekman, A. M. L., and Renard, J.-B. (2018). How much of the global aerosol optical depth is found in the boundary layer and free troposphere? Atmos. Chem. Phys. 18, 7709–7720.
Brown, S. S., and Stutz, J. (2012). Nighttime radical observations and chemistry. Chem. Soc. Rev. 41 (19), 6405–6447. doi:10.1039/c2cs35181a
Bu, X., Xie, Z., Liu, J., Wei, L., Wang, X., Chen, M., et al. (2021). Global PM2.5-attributable health burden from 1990 to 2017: Estimates from the Global Burden of disease study 2017. Environ. Res. 197, 111123. doi:10.1016/J.ENVRES.2021.111123
Colarco, P. R., Schoeberl, M. R., and Doddridge, B. G. (2004). Transport of smoke from Canadian forest fires to the surface near Washington, DC: Injection height, entrainment, and optical properties. J. Geophys. Res. Atmos. 109 (D6). doi:10.1029/2003JD004248
Lee, X. (2018). Fundamentals of boundary-layer meteorology. Berlin, Germany: Springer International Publishing. doi:10.1007/978-3-319-60853-2
Ma, J., Xu, X., Zhao, C., and Yan, P. (2012). A review of atmospheric chemistry research in China: Photochemical smog, haze pollution, and gas-aerosol interactions. Adv. Atmos. Sci. 29, 1006–1026. doi:10.1007/s00376-012-1188-7
Margairaz, F., Pardyjak, E. R., and Calaf, M. (2020). Surface thermal heterogeneities and the atmospheric boundary layer: The relevance of dispersive fluxes. Boundary-Layer Meteorol. 175, 369–395. doi:10.1007/S10546-020-00509-W/FIGURES/12
Miao, Y., and Liu, S. (2019). Linkages between aerosol pollution and planetary boundary layer structure in China. Sci. Total Environ. 650. doi:10.1016/j.scitotenv.2018.09.032
Miao, Y., Zhang, X., Che, H., and Liu, S. (2022). Influence of multi-scale meteorological processes on PM2.5 pollution in wuhan, central China. Front. Environ. Sci. 10, 1–7. doi:10.3389/fenvs.2022.918076
Petäjä, T., Järvi, L., Kerminen, V. M., and Ding, A. J. (2016). Enhanced air pollution via aerosol-boundary layer feedback in China. Sci. Rep. 6 (1), 18998.
Serafin, S., Adler, B., Cuxart, J., De Wekker, S., Gohm, A., Grisogono, B., et al. (2018). Exchange processes in the atmospheric boundary layer over mountainous terrain. Atmosphere 9, 102. doi:10.3390/atmos9030102
Sicard, P., Agathokleous, E., Anenberg, S. C., De Marco, A., Paoletti, E., and Calatayud, V. (2023). Trends in urban air pollution over the last two decades: A global perspective. Sci. Total Environ. 858, 160064. doi:10.1016/J.SCITOTENV.2022.160064
Stull, R. B. (1988). in An introduction to boundary layer meteorology. Editor R. B. Stull (Dordrecht: Springer Netherlands). doi:10.1007/978-94-009-3027-8
Wang, T., Xue, L., Brimblecombe, P., Lam, Y. F., Li, L., and Zhang, L. (2017). Ozone pollution in China: A review of concentrations, meteorological influences, chemical precursors, and effects. Sci. Total Environ. 575, 1582–1596. doi:10.1016/j.scitotenv.2016.10.081
Wang, Z., Xue, L., and Liu, J. (2022). Roles of atmospheric aerosols in extreme meteorological events: A systematic review. Curr. Pollut. Rep. 8, 177–188. doi:10.1007/s40726-022-00216-9
Xiao, Z., Miao, Y., Du, X., Tang, W., Yu, Y., Zhang, X., et al. (2020). Impacts of regional transport and boundary layer structure on the PM2.5 pollution in wuhan, central China. Atmos. Environ. 230, 117508.
Keywords: turbulence, transport, dispersion, boundary layer height, chemical reactions
Citation: Miao Y, Zhang G, Li X and Xue C (2023) Editorial: Physical and chemical processes within the planetary boundary layer and their impacts on air pollution. Front. Environ. Sci. 11:1221546. doi: 10.3389/fenvs.2023.1221546
Received: 12 May 2023; Accepted: 16 May 2023;
Published: 23 May 2023.
Edited and reviewed by:
Tirthankar Banerjee, Banaras Hindu University, IndiaCopyright © 2023 Miao, Zhang, Li and Xue. This is an open-access article distributed under the terms of the Creative Commons Attribution License (CC BY). The use, distribution or reproduction in other forums is permitted, provided the original author(s) and the copyright owner(s) are credited and that the original publication in this journal is cited, in accordance with accepted academic practice. No use, distribution or reproduction is permitted which does not comply with these terms.
*Correspondence: Yucong Miao, bWlhb3l1Y29uZ0B5ZWFoLm5ldA==