- 1PSL Université Paris: CNRS-EPHE-UPVD, UAR 3278 CRIOBE, Université de Perpignan, Perpignan, France
- 2Laboratoire d’Excellence CORAIL, Perpignan, France
- 3Laboratoire des Sciences du Climat et de l’Environnement, LSCE/IPSL, CEA-CNRS-UVSQ, Université Paris-Saclay, Gif-Sur-Yvette, France
- 4INRAE, Aix Marseille University, RECOVER, Aix-en-Provence, France
- 5EDF R&D, LNHE—Laboratoire National d'Hydraulique et Environnement, Chatou, France
Introduction: Characterizing the thermal habitat of fish is key to understanding their ecological requirements in order to make appropriate management plans for the preservation of fish populations in the context of climate change. Little is known about the thermal habitat of the early life stages of fish (larvae and juveniles), as most methods have been designed for large, easily handled individuals. In particular, the study of otoliths holds great promise for unveiling the early thermal history of fish, although it is challenging to implement due to the very small size of the biological material.
Methods: The stable oxygen isotope content (δ18O) of biogenic carbonates (such as otoliths) can be used to reconstruct the life temperature of fish individuals. However, relationships between δ18O of otoliths and ambient temperature are scarce and mainly developed for commercial species. In this study, we assessed the δ18O of juvenile European chub (Squalius cephalus, Linnaeus 1758) living in a section of the lower Rhône River in France.
Results: The δ18O of otoliths showed significant relationship with ambient temperature [δ18Ooto(VPDB) - δ18Ow(VPDB) = 33.391 – 0.2641 * T (°C)], and the relationship between temperature and the fractionation factor (α) was 1,000 ln α = −43.472 + 21.205 [1,000/T(K)].
Discussion: As expected, back-calculated temperatures from these equations were in much better agreement with the measured in situ temperatures than previously published equations. This equation is the first to be developed for leuciscid fish in Europe and the second in the world.
1 Introduction
Among environmental parameters, water temperature plays a major role in physiological processes and life history traits of ectotherms (Magnuson et al., 1979; Van Der Have and De Jong, 1996; Małek et al., 2015) and this is especially true for fish (Payne et al., 2016). Temperature, along with physical factors, determines both the regional distribution (Buisson et al., 2008) and the fine-scale habitat requirement during ontogenesis of fish species (Schiemer et al., 2004). All these processes act on the fitness of individuals and determine their thermal niche. The thermal niche of ectotherms corresponds to the temperature conditions suitable for an organism to grow, feed, and reproduce, and it can differ between life stages (Hutchinson, 1957; Magnuson et al., 1979). According to a review by Gvoždík (2018), the thermal niche of a species has usually been determined using one of the following approaches: 1) by measurements of the thermal dependence of life history traits, 2) from laboratory experiments under controlled conditions to obtain thermal preferenda, or 3) by modelling biogeographic distributional. However, several studies have shown that the temperatures obtained using these approaches may differ from the in situ requirements of the species (e.g., Schrank et al., 2003). Therefore, a tool able to assess the thermal niche realized by individuals is required to identify essential habitats (Morrongiello et al., 2012).
In situ temperature measurements are often one-off in space and time and could be significantly different to the thermal habitat inhabited by individuals during the different stages of their life cycle. This is particularly true in large floodplain rivers, where the temperature of aquatic habitats used by fish is highly variable in space and time, both within the channel cross section and between waterbodies (Tonolla et al., 2012). For example, lithophilic riverine species show habitat shifts during their early life, from lotic gravel spawning grounds during embryogenesis and lentic inshore microhabitats used by larvae to more lotic and deeper waters for juveniles. This illustrates that individuals are exposed to contrasting thermal conditions throughout their life, most notably in their early life stages. In such conditions, reconstructing the thermal life history of fishes is challenging and requires methodological developments.
The lack of basic information on the actual thermal conditions experienced by aquatic ectotherms makes the determination of their thermal niche particularly imprecise. Many studies used data storage tags implanted directly on individuals to continuously record the water temperature of their immediate environment (e.g., Pálsson and Thorsteinsson, 2003; Hunter et al., 2004). However, such a tagging approach requires a minimum body length (and weight) of individuals to support this equipment, thus excluding larvae and small fish (juveniles or small-sized species). Natural proxies that accurately record the environmental conditions in which organisms lived could be an alternative solution. Among them, the isotopic signature of biogenic carbonates has often been used.
Otoliths are calcium carbonate structures of the inner ear of teleost fish that are metabolically inert (no dissolution or resorption) and grow throughout the life of the fish (see, Campana and Neilson, 1985; Campana, 1999; Campana and Thorrold, 2001). They form growth increments (daily and seasonal) and their chemical composition reflects the chemical composition of the surrounding water, fish diet, and metabolic rate (Campana, 1999). Otoliths are used notably for reconstructing environmental exposure (Elsdon and Gillanders, 2002, 2004). Among key environmental parameters, the reconstruction of thermal exposure history of individuals is of growing scientific interest in the context of climate change (Morissette et al., 2023). This topic is particularly relevant for better understanding the use of thermally changing habitats by individuals, for characterizing behavioral adaption of organisms and for developing appropriate local restoration measures that take account of the gradual rise in temperature. Most studies that have focused on the reconstruction of thermal history of organisms have considered the stable isotopic composition of oxygen in the otolith as a temperature-dependent tracer linked to both the stable isotopic composition of oxygen in the water and ambient temperature (Patterson et al., 1993; Thorrold et al., 1997). The oxygen isotope signature in otoliths has been used to reconstruct the thermal life history of fish in paleontological studies (Andrus et al., 2002; Surge and Walker, 2005; Price et al., 2009; West et al., 2012) as well as in contemporary ecological studies in freshwater and marine environments (Thorrold et al., 1997; Blamart et al., 2002; Darnaude et al., 2014; Gerdeaux and Dufour, 2015; Burbank et al., 2020; Nazir et al., 2023).
Temperature reconstruction from biogenic carbonates is based on thermodynamic predictions at equilibrium of the oxygen isotope ratio (18O/16O) between newly formed calcium carbonate and the surrounding environment (e.g., water). This fractionation at equilibrium is determined by thermodynamic properties of exchange between phases, but the natural mineral growth can create a disequilibrium (Watkins et al., 2013; Daëron et al., 2019). For oxygen isotopes, this shift from equilibrium can be interpreted in terms of the kinetic effect during otolith formation and during the exchange of isotopes between the different species of dissolved carbon dioxide and water involving hydration and hydroxylation processes (see Mcconnaughey, 1989).
For decades, the estimation of the living temperature of fish from their δ18O values (i.e., the relative variation of the ratio between 18O and 16O to a standard) of otoliths has been made using empirically determined equations (e.g., Thorrold et al., 1997; Darnaude et al., 2014; Burbank et al., 2020 and references therein). These equations have mainly been established on sagittae of fish species of commercial interest (mainly gadid and salmonid species). However, for the second-largest superorder of fish, Ostariophysi (which includes the species-rich orders Cypriniformes and Siluriformes), the fragility and the small size of their sagittae make their analysis tricky, if not impossible. The alternative solution that is usually opted for is to use the lapillus, the biggest otolith of Ostariophysi (e.g., Panfili et al., 2002; Bounket et al., 2019). To date, only two dedicated studies have looked at the oxygen isotope signature of lapilli. Smith and Jones (2006) in their study of Cynoscion nebulosus (Cuvier, 1830), a sciaenid juvenile, showed that sagittae and lapilli were not isotopically interchangeable, with an enrichment in δ18O of 0.44‰ on average for sagittae compared to lapilli. In a recent study, Burbank et al. (2020) developed an empirical oxygen isotope fractionation equation for Notropis photogenis (Cope, 1865), a North American leuciscid. They have shown that it is possible to work on the lapillus of small fish, and they provide a more realistic back-calculated temperature using the equation they developed compared to those found in the literature.
The Cypriniformes (minnows, carps, loaches and suckers) comprising around 4,300 species represent 6% of all vertebrate species and one third of freshwater fish species (Stout et al., 2016). The phylogeny of this order was recently resolved using molecular analyses, and some groups previously classified in the Cyprinidae family were elevated in family level, such as the Leuciscidae, the Danionidae, and the Gobionidae, for example, (Stout et al., 2016). To our knowledge, the equation developed by Burbank et al. (2020) is the only one specifically developed using the lapillus and considering a species from the Cypriniformes order. Understanding the relationship between temperature and the stable oxygen isotopic composition for other Cypriniformes from other freshwater hydrosystems is important for this overlooked order of fish. The European chub Squalius cephalus (Linnaeus, 1758), a potamodromous lithophilous species inhabiting both lotic and lentic freshwaters, is one of the most abundant and widespread Cypriniformes in Europe. This euryceous species lives in environments covering a wide range of temperatures. This makes it a relevant biological model to study the relationship between ambient temperature and the oxygen isotope signature of the lapillus, which would then be valid and applicable over a wide temperature gradient.
The aim of this study was first to estimate the relationship between the oxygen isotopic signature of lapillus and the mean temperature of the water in which juveniles have lived (i.e., over 3–6 months), and second to develop an empirical oxygen isotope fractionation equation valid for European chub. Finally, the performance of the identified relationship was assessed by comparison with other equations from the literature. This study was conducted on the reach of Caderousse (France), which is a 50-km-long section of the lower Rhône River. In this reach a previous study analyzed the microchemistry of water and chub otoliths and found that 70% of the young-of-the-year individuals sampled did not spread far away from their hatching area and can be considered as resident fish (Bounket et al., 2021). These individuals are therefore good candidates for studying the relationship between ambient temperature and the stable oxygen isotope content of otoliths in natural environments.
2 Material and methods
2.1 Study area
The study area is the reach of Caderousse, which is located between Montélimar and Orange on the lower Rhône River in south-eastern France (Figure 1). The reach of Caderousse is constituted by the two hydropower schemes of Donzère-Mondragon and Caderousse. This reach includes the by-passed section of the Donzère-Mondragon scheme, the reservoir of Caderousse, and the tailrace of the Bollène power plant. The by-passed section presents in its upstream part the lône de Malaubert, a backwater of the old Rhône River, and in its downstream part the confluence with the Ardèche River (a large typical Mediterranean tributary). The channelized section of the Donzère-Mondragon scheme receives heated cooling water on its right bank from the nuclear power plant of Tricastin. Four stations with contrasting thermal conditions were studied (Figure 1): 1) the Ardèche River at Pont-Saint-Esprit; 2) the lône de Malaubert; 3) a channelized section located upstream of the Tricastin nuclear power plant; and 4) a channelized section located downstream of the power plant of Bollène. Station 4 is located downstream of the release of heated cooling water from the Tricastin nuclear power plant, after complete mixing with the cooler water of the river.
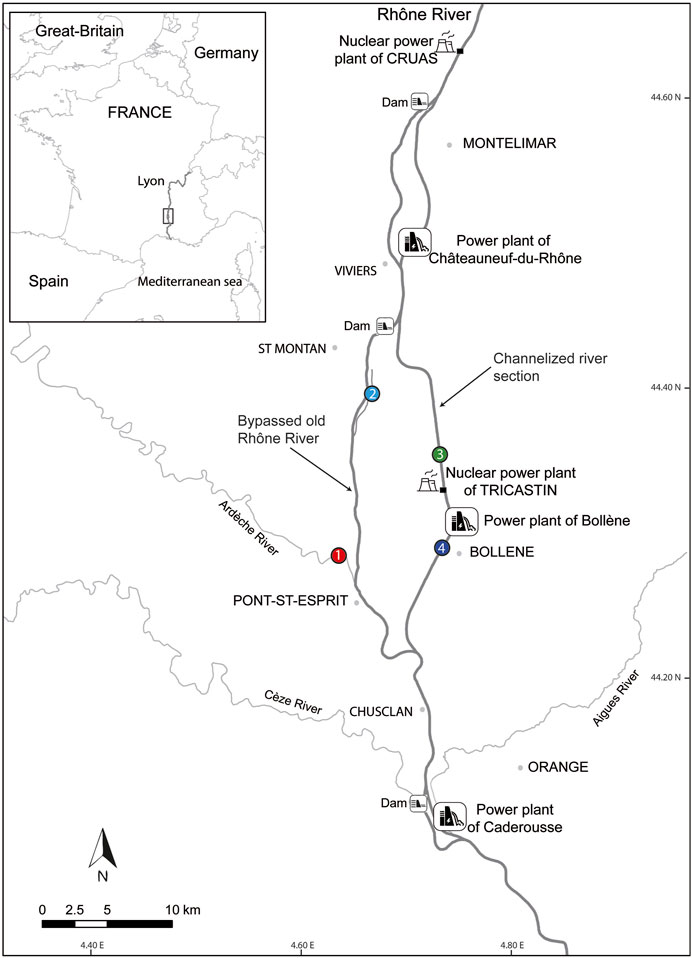
FIGURE 1. Map of the reach of Caderousse on the lower Rhône River. Water and fish were sampled at four stations: 1—the Ardèche River; 2—a backwater of the Rhône River—the lône de Malaubert; 3—the upstream section of the Tricastin nuclear power plant; and 4—downstream section of the Bollène hydroelectric power plant.
2.2 Water sampling
Water was sampled monthly from February to December 2016 to assess the temporal variation in oxygen isotopes of the Rhône River, the lône de Malaubert, and the Ardèche River. Water was sampled close to the riverbank at depths between 0.5 and 1.0 m; this habitat and depth correspond to typical juvenile habitats. A glass vial (150 mL) was rinsed several times using the river water before being filled and sealed underwater and then sent for stable oxygen isotope analysis.
Each station was equipped with HOBO® thermometers that recorded temperature every 15 min. Daily water temperature averages were then calculated for each station.
2.3 Fish sampling
Fish juveniles were collected by electrofishing in the reach of Caderousse (from 29 November to 2 December 2016) and their origin, i.e., resident or migrant, was determined using otolith and water microchemical analyses (Bounket et al., 2021). For reasons of feasibility, we focused on the juvenile stage (individuals aged between 3 and 6 months). Fish were considered as resident (i.e., individuals that were born and spent most of their life in the same environment where they were caught) if no significant difference was observed between the microchemistry of their otolith and the water microchemistry of the station where the fish were caught (see Bounket et al., 2021 for details). Among the fish used for the connectivity study by Bounket et al. (2021), 97% from the Ardèche River (30 out of 31 fish analyzed in station 1), 93% from the lône de Malaubert (28/30 in station 2), 20% from the upstream section of the Tricastin nuclear power plant (3/15 in station 3), and 37% from the downstream section of the Bollène hydroelectric power plant (3/8 in station 4) were identified as residents. Among these resident fish, 39 individuals were used for stable isotope analyses in order to evaluate the ability of lapilli to record water temperature (Table 1). In this study, the resident fish were used because they are the only fish for which the thermal life history can be verified and compared with in situ temperature. Indeed, contrary to migrant fish that have inhabited different environments during their life, resident fish have lived in the same environment throughout their life.
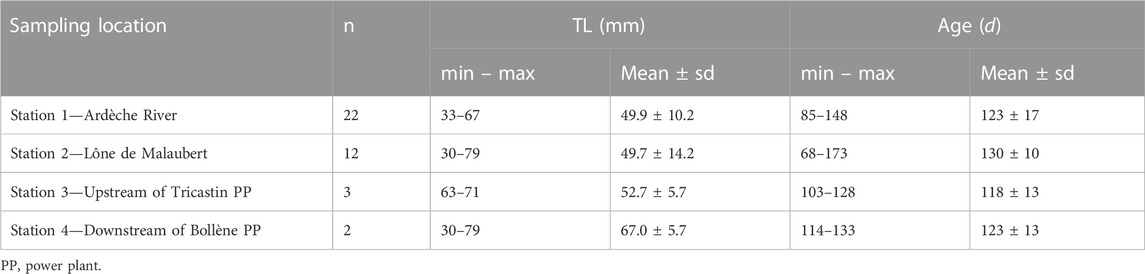
TABLE 1. Number of resident fish, total fish length (LT in mm), and estimated fish age (in days) by station.
2.4 Otolith growth rate
The growth rates of right otoliths were estimated based on the method described in previous studies (Bounket et al., 2019, 2021; Logez et al., 2021). Briefly, to expose the daily increments, the otolith was embedded in epoxy resin (Araldite 2020) and ground along the frontal plane using abrasive papers and diamond solutions. After digitization of the otolith, daily age and growth were estimated along a unique reading transect following the maximum growth axis. Fish age was estimated by counting the number of growth increments, and the growth rate was determined by measuring the distance between consecutive growth increments.
2.5 Stable isotope analysis
The left lapillus was used for the stable oxygen isotope analysis [the right lapillus was used for microchemistry and age analyses in Bounket et al. (2021)]. Lapilli were cleaned and baked at 380°C under vacuum for 45 min to avoid possible contamination by organic matter. Since chub lapilli are small, in toto analyses were performed from approximately 70 µg of otolith. All isotope analyses were carried out on an IRMS (isotopic ratio mass spectrometer) VG-OPTIMA coupled with an automated line of samples prepared in the “Laboratoire des Sciences du Climat et de l’Environnement (LSCE, Gif-sur-Yvette, France).” The results are given in the conventional (δ ‰) notation expressed in per mil against the VPDB (Vienna Pee Dee Belemnite) standard using Eq. 1:
where Rsample and Rstandard are the isotopic ratio 18O/16O of the sample and the standard, respectively. The reproducibility calculated from the calcite internal standard was 0.07‰ (1σ) for the oxygen.
The water samples were analyzed by cavity ring-down spectroscopy (CRDS) using a l2130i isotope and gas concentration analyzer (Picarro machine) at the LSCE. The samples (2 µL) are automatically injected into a vaporization module at 110°C before being sent into the laser cavity. The time needed to measure a sample is 9 min. The CRDS device is calibrated daily by measuring two in-house standards (themselves calibrated against the international standards SMOW and SLAP) whose isotopic compositions frame the samples to be measured. The oxygen isotope compositions are given against the SMOW standard and the external precision of the measurement (1s) is 0.2‰.
2.6 Isotopic fractionation
Since studies on the isotopic signatures of lapilli were scarce, isotopic fractionation must first be tested before being established. The isotopic fractionation is a modification of the ratio between both heavy and light isotopes during a chemical reaction or a state change, which are influenced by temperature. The theoretical values in δ18O of aragonite (δ18Oara) for each month were determined using Eq. 2 from Watkins et al. (2013). This equation was determined experimentally and enables the calculation of the isotopic values of δ18O considering the isotopic equilibrium between water and carbonate (without vital effect, i.e., without any influence of the organism metabolism or physiology on the mineralization). Although this equation was established for calcite, Lécuyer et al. (2012) concluded that biogenic calcium carbonate polymorphs precipitate with δ18O values very close to each other and not far away from isotopic equilibrium with seawater. Then, these theoretical δ18Oara values were weighted by the fish life span (δ18Ooto-weighted) and compared with the values of δ18O measured in otoliths (δ18Ooto).
where T is the temperature in Kelvin and δ18Ow the isotopic signature of the water expressed in SMOW.
For example, the δ18Oara-weighted of a fish aged 61 days, born on the 1 August and captured on 30 September, is obtained as shown in the mass balance Eq. 3.
Secondly, the otolith fractionation, the difference between the otolith and water δ18O values, was plotted against the inverse of the average weighted temperature. These two parameters were linked using Eq. 4:
where δ18Ooto (VPDB) and δ18Ow (VPDB) are the isotopic signatures of the otolith and water, respectively, expressed in VPDB, and a and b the coefficient of the linear regression. δ18Ow (VPDB) values were obtained using Eq. 5:
In the literature, the fractionation equation derives from a fractionation factor (α) as shown in Eq. 6.
Individual values of α expressed in 1,000 ln (α) were plotted against the inverse of the average temperature T expressed in Kelvin. This Arrhenius diagram allows one to obtain a species-specific equation linking these two parameters, as shown in Eq. 7:
where c and d are the coefficients of the linear relation.
2.7 Back-calculation of the temperature
The efficiency of the empirically established oxygen isotopic fractionation equation specifically defined for the European chub was evaluated by comparison to the back-calculated temperature obtained using nine equations from the literature and to the average weighted temperature from in situ measurement of each fish (Tweighted).
where Tweighted is the average temperature of the sample location calculated from the monthly temperature weighted by the life span of each individual, expressed in °C. The Tcal is the back-calculated temperature using equations from the literature (Table 2).
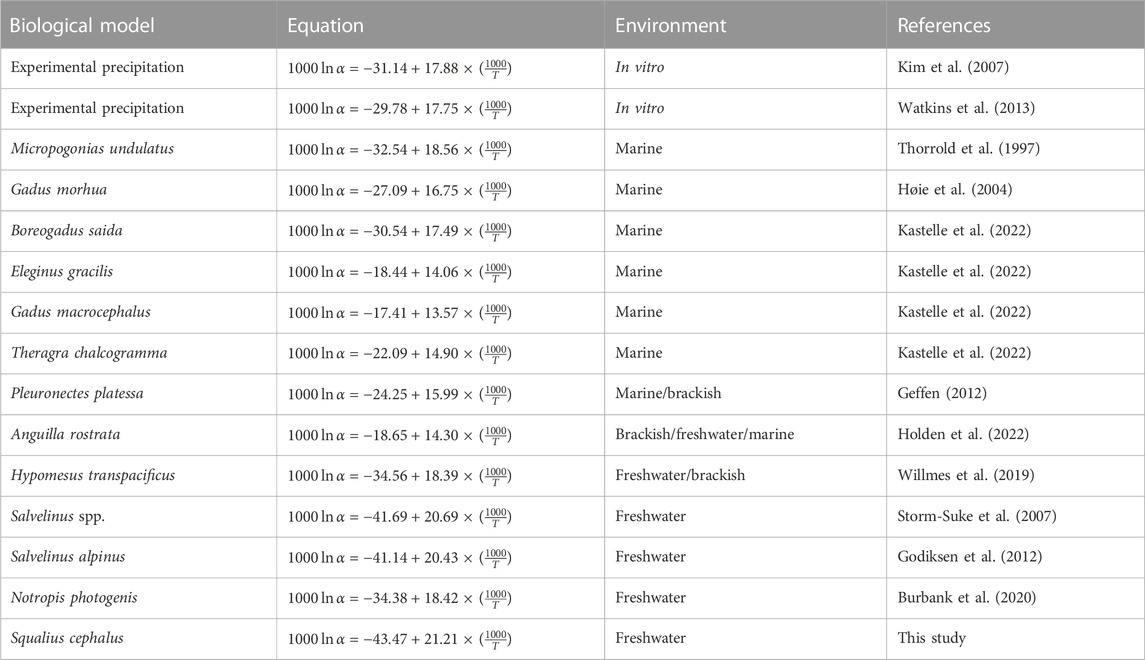
TABLE 2. Equations of oxygen isotopic fractionation obtained from the literature and investigated in the present study.
2.8 Statistical analysis
Kruskal-Wallis non-parametric tests were used to compare 1) the mean annual temperature, 2) the δ18Ow, and 3) the δ18Ooto between sites. Linear regressions were used to determine 1) the relationship between the fractionation factor and the temperature, and 2) the relationship between the temperature and the difference between the isotopic signature of otoliths (δ18Ooto) and water (δ18Ow). Spearman’s correlation test was used to examine the relationship between δ18Ooto and the average daily otolith growth rate, which is a proxy for average fish growth. The differences between the oxygen isotope signatures measured in otoliths and obtained theoretically were tested using the Friedman non-parametric test (χ2). Similarly, the differences between back-calculated temperatures from the 14 equations were also tested using the Friedman non-parametric test (χ2). The Friedman test was coupled with the Wilcoxon post hoc test to test for the significance of pairwise differences between equations. The size effect was assessed using the Kendall test (W). Kendall’s W varied from 0 (weak effect) to 1 (strong effect). All analyses were performed with the software R (v. 3.6.2) and the libraries “stats” and “rstatix.”
3 Results
3.1 Temperature and oxygen isotope signature of water
All stations showed a marked seasonality in water temperature (Figure 2A), with no significant difference in monthly averages between stations (χ2 = 2.69, df = 3, p = 0.44). The coolest water temperatures were observed in December for all stations, while the warmest month varied between stations: July for the Ardèche River and the lône de Malaubert, and August for the stations located upstream of the Tricastin power plant and downstream of the Bollène power plant.
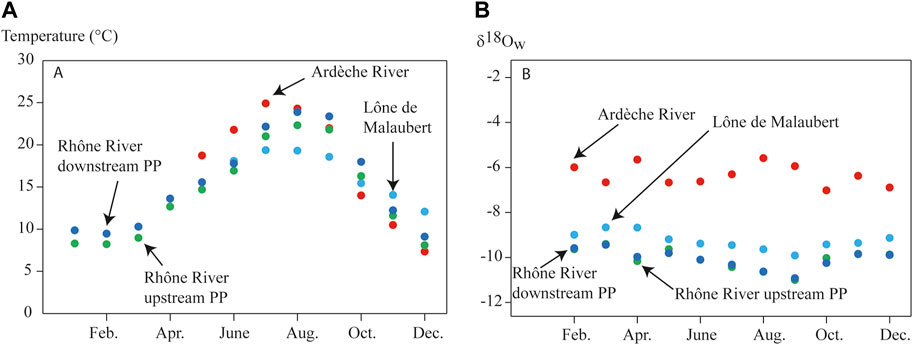
FIGURE 2. (A) Monthly water temperatures and (B) monthly oxygen isotope signatures of the water (δ18Ow SMOW) measured at the four sampling stations. PP, power plant.
The isotopic signatures of oxygen (δ18Ow) differed significantly among stations (χ2 = 32.97, df = 3, p = 3.27*10−7, Figure 2B). The Ardèche River showed the highest δ18Ow values from −7.02 to −5.59‰, the lône de Malaubert showed intermediate values from −9.91 to −8.66‰, and the stations located upstream of the Tricastin power plant and downstream of the Bollène power plant showed the lowest values from −11.00 to −9.40‰ and from −10.99 to −9.44‰, respectively. The low δ18Ow values of the Rhône River reflect the input throughout the year of ice-snow melted water from Lake Geneva.
3.2 Isotopic equilibrium and fractionation
Measured δ18Ooto signatures were lower than theoretical values of calcium carbonate by −2.24‰ ± 0.39‰ on average [Friedman test χ2 (1) = 39, p = 4.24*10−10]. This value is 37% lower than the average δ18O theoretical value. Although varying slightly among stations (Table 3), this difference suggests that the crystallization of calcium carbonate in lapilli is not in equilibrium with water, as the theoretical δ18O values are derived from the Watkins equation (without vital effect).
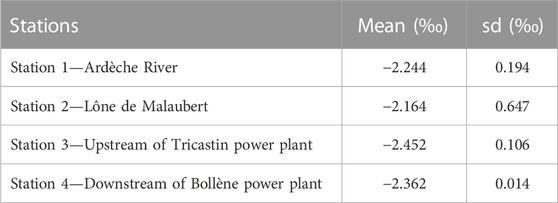
TABLE 3. Mean and standard deviation (sd) of the difference between δ18O measured in otolith and theoretical values calculated using the equation from Watkins et al. (2013) and weighted by fish lifetime.
The difference between the otolith and water δ18O values showed a negative linear relationship with temperature (F1,2 = 17.69, p < 0.05) (Figure 3A, Eq. 8b).
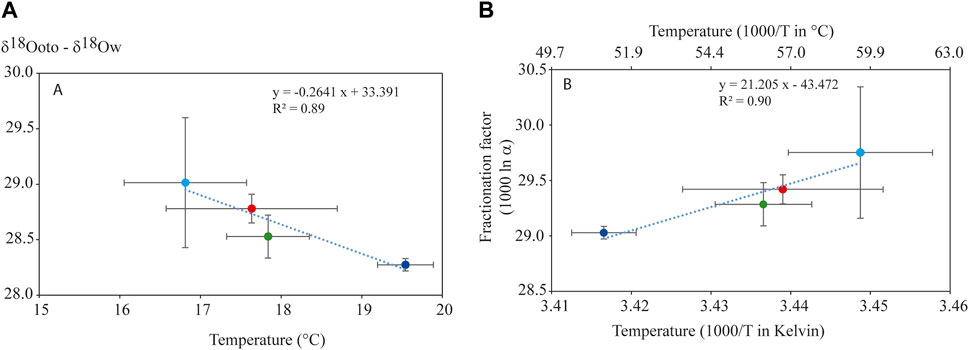
FIGURE 3. (A) Relationship between mean water temperature (T°C) weighted by each fish lifetime and otolith fractionation expressed as the difference between otolith and water δ18O values (δ18Ooto – δ18Ow). (B) Relationship between isotopic fractionation expressed in 1,000 ln α and the mean water temperature weighted by fish lifetime (expressed in 1,000/T in Kelvin or °C). Error bars represent the standard deviation. Ardèche River station (red), lône de Malaubert (light blue), upstream of the Tricastin power plant (green), and downstream of the Bollène power plant (dark blue).
The fractionation factors expressed in 1,000 ln α showed significant differences between stations (Friedman test χ2 = 13.889, df = 3, p = 0.003). A linear relationship was found with the weighted water temperature (expressed in Kelvin) (F1,2 = 19.04, p = 0.048) (Figure 3B, Eq. 9).
No significant relationship was found between δ18Ooto signatures and average daily otolith growth rates (Spearman correlation test, ρ = 0.304, p = 0.06; Figure 4) and between δ18Ooto signatures and fish age (Spearman correlation test, ρ = −0.09, p = 0.58).
3.3 Temperature back-calculation
Based on the measured temperature weighted by the lifetime of fish, significant differences were observed for the average temperature experienced by fish among stations (KW X2 = 11.22, df = 3, p = 0.01). The lowest values were observed in the lône de Malaubert (station 2), intermediate values in the Ardèche River (station 1) and in the upstream section of the Tricastin power plant (station 3), and the highest values were found in the downstream section of the Bollène power plant (station 4) (Table 4).
Back-calculated temperatures estimated from fractionation equations strongly varied depending on the equation used (Friedman test χ2 (10) = 380, p < 0.01, Table 4) with a large size effect (W = 0.974). All pairwise comparisons showed highly significant differences (Wilcoxon-tests, p < 0.0001) except in two cases. Non-significant differences were measured between the average weighted temperature and two equations: the one developed in the present study and the equation developed by Storm-Suke et al. (2007). The highest ΔT values were observed for back-calculated temperatures obtained from the Watkins et al. (2013) (−8.8°C) and the Thorrold et al. (1997) equations (−8.5°C) (Figure 5). The lowest ΔT values were obtained with the equation developed in this study (+0.01°C) and the one from Storm-Suke et al. (2007) (−0.02°C). The other equations yielded intermediate values (see Table 4 for the complete results).
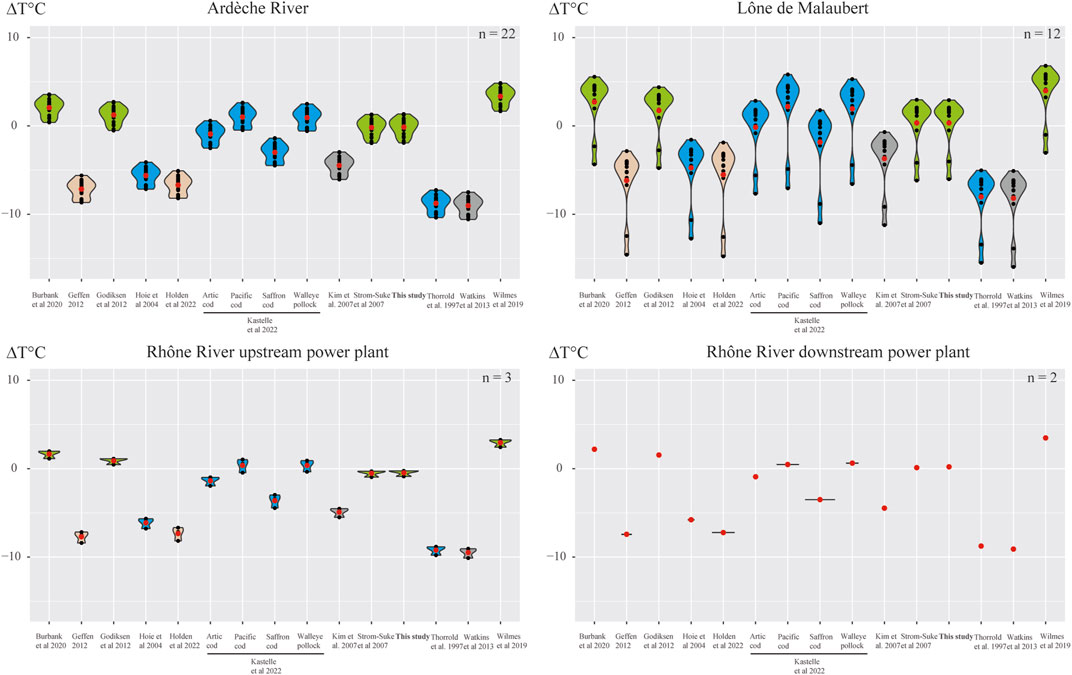
FIGURE 5. Violin plot of ΔT [difference between back-calculated temperature values and mean weighted temperatures (T°Cweighted)]. In green, fish from freshwater environments, in blue from marine environments, in brown from multiple environments (marine, brackish, freshwater) and in gray for in vitro experiments. The red dots represent the average values. Artic cod = Boreogadus saida, Pacific cod = Gadus macrocephalus, Saffron cod = Eleginus gracilis, Walleye Pollock = Theragra chalcogramma.
Back-calculated temperature values obtained from the chub-specific equation showed that the sampled individuals had lived in distinct thermal habitats. Individuals collected in the Ardèche River had back-calculated temperature ranging from 16.9°C to 18.7°C; individuals from the lône de Malaubert from 14.5°C to 21.6°C, and those from the channelized Rhône River (both stations) from 17.5°C to 19.5°C.
4 Discussion
The stable oxygen isotope content of otoliths is widely used to determine the thermal life history of fishes in marine, brackish, and freshwater environments. However, although Cypriniformes fish represent one third of freshwater fish species worldwide (Stout et al., 2016), our study and the one by Burbank et al. (2020) are the only studies to have established the relationship between ambient water temperature and the isotopic signature of lapilli. As expected, our study showed a clear fractionation between the otolith δ18O (δ18Ooto) and the water δ18O (δ18Ow) for lapilli as a function of temperature. Consequently, we developed a new oxygen fractionation equation specific to European chub. We found that the back-calculated temperature values derived from this equation were in much better agreement with the measured in situ temperatures than when using previously published equations.
To test the ability of the approach to identify non-resident (i.e., migrant) individuals, we performed a complementary analysis by back-calculating temperatures from the δ18Ooto signatures of the individuals identified as migrants by Bounket et al. (2021). These individuals showed much greater deviation from the average weighted ambient temperature compared to resident fishes (Supplementary Figure S1). This finding suggests that these individuals inhabited environments with different thermal conditions than the station where they were caught. Furthermore, it highlights how such an approach can help identify resident and migrant individuals, ideally alongside otolith and water microchemical analyses.
4.1 Performance of the fractionation equations
Fractionation equations developed for fish from freshwater environments (Storm-Suke et al., 2007, and the present study; Godiksen et al., 2012; Burbank et al., 2020) showed back-calculated temperatures closer to average weighted temperatures than those developed for fish from marine or brackish environments (Thorrold et al., 1997; Høie et al., 2004; Geffen, 2012; Holden et al., 2022) and from in vitro precipitation (Kim et al., 2007; Watkins et al., 2013). Kastelle et al. (2022) have recently proposed four species-specific equations for different marine species (three cod species and one pollock) among which the one for the Arctic cod Boreogadus saida (Lepechin, 1774) performed reasonably well in reconstructing the average temperature of the life span of the studied chub juveniles. The chub-specific equation developed in the present study showed the closest back-calculated temperature values (mean difference to Tweighted = 0.013°C), closely followed by the equation from Storm-Suke et al. (2007) (mean difference to Tweighted = −0.028°C). Values of temperature back-calculated using the equation developed by Burbank et al. (2020) for the freshwater leucicid Notropis photogenis underestimated by 2.21°C ± 1.80°C and 2.23°C ± 0.21°C the temperature obtained from the weighted average temperature and from the chub-specific equation, respectively. This was unexpected since this species is the closest relative of the European chub (in terms of phylogeny), for which a fractionation equation has been developed. Both Burbank et al. (2020) and the present study considered the totality of the otolith (in toto measurements) and provided an estimation of the temperature for the life span of the fish. However, Burbank et al. (2020) studied 0+ and 1+ fishes, whereas in our study only 0+ fishes, were studied. These differences in fish age [individuals about 6 months old for our study vs. individuals of older than 1 year in the study by Burbank et al. (2020)] have changed the isotopic signatures recorded in otoliths and have therefore induced differences in the estimated temperatures from the equations proposed in each study. It is worth noting that the slope and intercept of the oxygen isotope fractionation equations established for the sagittae of freshwater fish are of the same order of magnitude as those we established for the lapilli of European chub (Table 2). To further test the generalizability of the fractionation equation developed in the present study, an independent dataset combining the oxygen isotopic signature of water and chub lapilli would be needed. These results confirm the necessity 1) of establishing specific equations to provide reliable tools for estimating the thermal habitat used by fish, and 2) of developing experiments under known environmental conditions.
4.2 The consistent signature of δ18O within a station
Studies demonstrated that δ18Ooto was linked to δ18Ow and ambient temperature independently of fish growth (Smith and Jones, 2006; Burbank et al., 2018). We also found no significant relationship between δ18Ooto, average fish growth, and fish age. The station signature for δ18O of otoliths and water was very strong, with highly consistent values within each station and highly distinct values between stations (except for the two consecutive stations located on the channelized river section). A notable exception regarding δ18Ooto was two individuals sampled at the lône de Malaubert. They were identified as residents by Bounket et al. (2021) based on otolith microchemistry (strontium and barium), whereas their δ18Ooto values were very different from the other individuals sampled at this station but very similar to the δ18Ooto of individuals sampled in the channelized river section. As juveniles do not seem to be able to move between the lône de Malaubert and the stations located on the channelized river section, we hypothesize that these individuals sampled in the lône spent time in the main channel of the bypassed old Rhône River, whose δ18Ow is very similar to that of the channelized section (data not provided).
4.3 The promise of stable oxygen isotope analysis of otoliths and the challenges ahead
The congruence between estimated temperatures from the chub-specific equation and the weighted average temperature measured in situ confirmed that the oxygen isotope signature of otoliths is a promising tool for studying the thermal life and habitat use of fish. Furthermore, our work has highlighted the ability to study small otoliths and particularly the lapillus, of Cypriniformes juveniles, moreover. Several methodological and monitoring aspects should be the objective of future developments. First, a technical gap remains regarding our ability to perform multiple measurements of a single otolith (i.e., to quantify the δ18O at multiple depths along the otolith radius) in order to get access to several thermal snapshots throughout the life of fish. To the end, promising methods exist, such as micro-milling (Morat et al., 2014) or the use of secondary ion mass spectrometry (SIMS) on samples collected in situ (Matta et al., 2013; Helser et al., 2018). In most cases, micro-milling is not adapted to the small size of the otolith (e.g., micro-milling of spots up to 150 µm whereas the studied otoliths are only twice bigger). In our study, we tried the micro-milling approach of 150 µm spots but the lapilli were too fragile and systematically broken. The SIMS technique therefore remains the most suitable method for studying the thermal history (or at least for assessing the variability of temperatures experienced during the life of the fish) recorded in small otoliths but it is still underdeveloped (Von Leesen et al., 2021). Overall, multiple measurements would enable 1) a more accurate characterization of the habitats inhabited by the juveniles across their life, and 2) the possibility to consider fishes that are not exclusive residents of a single environment or station. Second, the implementation of a more comprehensive monitoring of water temperature of the sampled areas and of the directly connected habitats would be valuable in order to more accurately define the thermal context and improve our ability to associate back-calculated temperatures with specific local environments.
This study evidence the necessity of developing species-specific equations linking δ18O of otoliths and temperature. We created the second equation for cyprinid fish that allowed us to calculate the lifetime average temperature of juvenile chubs.
Data availability statement
The datasets presented in this study can be found in online repositories. The names of the repository/repositories and accession number(s) can be found below: https://doi.org/10.6084/m9.figshare.22708111.
Ethics statement
Ethical review and approval was not required for the animal study because All relevant international, national, and/or institutional guidelines for the care and use of animals were followed. Fish were sacrificed using anaesthetic overdose (eugenol) in accordance with the European Directive 2010/63/EU. Fish were collected in accordance with the authorisation numbers: 2015-217-004, 2015-229-DDTSE01, 2017-124-DDTSE01 and 2017-165-0007, delivered by the prefectures.
Author contributions
This study was designed by GC, FM, DB, CA, and AM. Field collections were made GC and BB. Otolith analyses were conducted by BB and DB. Statistical analyses were conducted by FM and AM. The manuscript was written by FM, AM, DB, CA, and GC. All authors contributed to the article and approved the submitted version.
Funding
This study was funded by the French Office for Biodiversity (OFB), by Electricity of France (EDF), and by the national research institute in science and technology for the environment and agriculture (INRAE).
Acknowledgments
The authors would like to thank everyone involved in fish sampling and data collection, in particular Virginie Diouloufet and Pierre Gibert. The authors also thank Isabella Athanassiou for proofreading the last version of this manuscript.
Conflict of interest
The authors declare that this study received funding from EDF. The funder was not involved in the study design, collection, analysis, interpretation of data, the writing of this article, or the decision to submit it for publication.
Publisher’s note
All claims expressed in this article are solely those of the authors and do not necessarily represent those of their affiliated organizations, or those of the publisher, the editors and the reviewers. Any product that may be evaluated in this article, or claim that may be made by its manufacturer, is not guaranteed or endorsed by the publisher.
Supplementary material
The Supplementary Material for this article can be found online at: https://www.frontiersin.org/articles/10.3389/fenvs.2023.1213239/full#supplementary-material
References
Andrus, C. F. T., Crowe, D. E., Sandweiss, D. H., Reitz, E. J., and Romanek, C. S. (2002). Otolith δ18O record of mid-Holocene sea surface temperatures in Peru. Science 295 (5559), 1508–1511. doi:10.1126/science.1062004
Blamart, D., Escoubeyrou, K., Juillet-Leclerc, A., Ouahdi, R., and Lecomte-Finiger, R. (2002). Composition isotopique δ18O-δ13C des otolithes des populations de Poissons récifaux de Taiaro (tuamotu, polynésie française): Implications isotopiques et biologiques. Comptes Rendus Biol. 325, 99–106. doi:10.1016/S1631-0691(02)01419-1
Bounket, B., Gibert, P., Gennotte, V., Argillier, C., Carrel, G., Maire, A., et al. (2019). Otolith shape analysis and daily increment validation during ontogeny of larval and juvenile European chub Squalius cephalus. J. Fish Biol. 95, 444–452. doi:10.1111/jfb.13976
Bounket, B., Tabouret, H., Gibert, P., Bareille, G., Pecheyran, C., Carrel, G., et al. (2021). Spawning areas and migration patterns in the early life history of Squalius cephalus (Linnaeus, 1758): Use of otolith microchemistry for conservation and sustainable management. Aquatic Conservation Mar. Freshw. Ecosyst. 31 (10), 2772–2787. doi:10.1002/aqc.3682
Buisson, L., Blanc, L., and Grenouillet, G. (2008). Modelling stream fish species distribution in a river network: The relative effects of temperature versus physical factors. Ecol. Freshw. Fish 17 (2), 244–257. doi:10.1111/j.1600-0633.2007.00276.x
Burbank, J., Drake, D. a. R., and Power, M. (2020). Field-based oxygen isotope fractionation for the conservation of imperilled fishes: An application with the threatened silver shiner Notropis photogenis. Endanger. Species Res. 42, 83–93. doi:10.3354/esr01040
Burbank, J., Kelly, B., Nilsson, J., and Power, M. (2018). Tests of size and growth effects on Arctic charr (Salvelinus alpinus) otolith δ18O and δ13C values. Rapid Commun. Mass Spectrom. 32 (17), 1557–1564. doi:10.1002/rcm.8198
Campana, S. E. (1999). Chemistry and composition of fish otoliths: Pathways, mechanisms and applications. Mar. Ecology-Progress Ser. 188, 263–297. doi:10.3354/meps188263
Campana, S. E., and Neilson, J. D. (1985). Microstructure of fish otoliths. Can. J. Fish. Aquatic Sci. 42, 1014–1032. doi:10.1139/f85-127
Campana, S. E., and Thorrold, S. R. (2001). Otoliths, increments, and elements: Keys to a comprehensive understanding of fish populations? Can. J. Fish. Aquatic Sci. 58 (1), 30–38. doi:10.1139/f00-177
Daëron, M., Drysdale, R. N., Peral, M., Huyghe, D., Blamart, D., Coplen, T. B., et al. (2019). Most Earth-surface calcites precipitate out of isotopic equilibrium. Nat. Commun. 10 (1), 429. doi:10.1038/s41467-019-08336-5
Darnaude, A. M., Sturrock, A., Trueman, C. N., Mouillot, D., Campana, S. E., Hunter, E., et al. (2014). Listening in on the past: What can otolith δ18O values really tell us about the environmental history of fishes? PloS one 9 (10), e108539. doi:10.1371/journal.pone.0108539
Elsdon, T. S., and Gillanders, B. M. (2004). Fish otolith chemistry influenced by exposure to multiple environmental variables. J. Exp. Mar. Biol. Ecol. 313 (2), 269–284. doi:10.1016/j.jembe.2004.08.010
Elsdon, T. S., and Gillanders, B. M. (2002). Interactive effects of temperature and salinity on otolith chemistry: Challenges for determining environmental histories of fish. Can. J. Fish. Aquat. Sci. 59 (11), 1796–1808. doi:10.1139/f02-154
Geffen, A. J. (2012). Otolith oxygen and carbon stable isotopes in wild and laboratory-reared plaice (Pleuronectes platessa). Environ. Biol. fishes 95 (4), 419–430. doi:10.1007/s10641-012-0033-2
Gerdeaux, D., and Dufour, E. (2015). Life history traits of the fish community in lake annecy: Evidence from the stable isotope composition of otoliths. Knowl. Manag. Aquatic Ecosyst. 416, 35. doi:10.1051/kmae/2015033
Godiksen, J. A., Power, M., Borgstrøm, R., Dempson, J. B., and Svenning, M. A. (2012). Thermal habitat use and juvenile growth of svalbard arctic charr: Evidence from otolith stable oxygen isotope analyses. Ecol. Freshw. Fish 21 (1), 134–144. doi:10.1111/j.1600-0633.2011.00533.x
Helser, T., Kastelle, C., Crowell, A., Ushikubo, T., Orland, I. J., Kozdon, R., et al. (2018). A 200-year archaeozoological record of Pacific cod (Gadus macrocephalus) life history as revealed through ion microprobe oxygen isotope ratios in otoliths. J. Archaeol. Sci. Rep. 21, 1236–1246. doi:10.1016/j.jasrep.2017.06.037
Høie, H., Otterlei, E., and Folkvord, A. (2004). Temperature-dependent fractionation of stable oxygen isotopes in otoliths of juvenile cod (Gadus morhua L). ICES J. Mar. Sci. 61, 243–251. doi:10.1016/j.icesjms.2003.11.006
Holden, C. V., Kyser, T. K., Longstaffe, F. J., Wang, Y., and Casselman, J. M. (2022). Oxygen isotope fractionation of otoliths formed across the maximum thermal range for somatic growth of the American eel Anguilla rostrata. Chem. Geol. 591, 120706. doi:10.1016/j.chemgeo.2021.120706
Hunter, E., Metcalfe, J., Arnold, G., and Reynolds, J. (2004). Impacts of migratory behaviour on population structure in North Sea plaice. J. animal Ecol. 73, 377–385. doi:10.1111/j.0021-8790.2004.00801.x
Hutchinson, C. (1957). Concluding remarks. Quant. Biol. 22, 415–427. doi:10.1101/sqb.1957.022.01.039
Kastelle, C. R., Helser, T. E., Laurel, B. J., Copeman, L. A., Stone, K. R., and Mckay, J. L. (2022). Oxygen isotope fractionation in otoliths: Experimental results from four North pacific and arctic gadid species. Mar. Ecol. Prog. Ser. 686, 159–175. doi:10.3354/meps13985
Kim, S.-T., O’neil, J. R., Hillaire-Marcel, C., and Mucci, A. (2007). Oxygen isotope fractionation between synthetic aragonite and water: Influence of temperature and Mg2+ concentration. Geochimica Cosmochimica Acta 71 (19), 4704–4715. doi:10.1016/j.gca.2007.04.019
Lécuyer, C., Hutzler, A., Amiot, R., Daux, V., Grosheny, D., Otero, O., et al. (2012). Carbon and oxygen isotope fractionations between aragonite and calcite of shells from modern molluscs. Chem. Geol. 332-333, 92–101. doi:10.1016/j.chemgeo.2012.08.034
Logez, M., Bounket, B., Gibert, P., Carrel, G., Diouloufet, V., Colombet, X., et al. (2021). The interannual variability of the fall size of young-of-the-year chub (Squalius cephalus): Influence of phenology, growth patterns and abiotic factors. Front. Environ. Sci. 9, 784751. doi:10.3389/fenvs.2021.784751
Magnuson, J. J., Crowder, L. B., and Medvick, P. A. (1979). Temperature as an ecological resource. Am. Zoologist 19 (1), 331–343. doi:10.1093/icb/19.1.331
Małek, D., Drobniak, S., Gozdek, A., Pawlik, K., and Kramarz, P. (2015). Response of body size and developmental time of Tribolium castaneum to constant versus fluctuating thermal conditions. J. Therm. Biol. 51, 110–118. doi:10.1016/j.jtherbio.2015.04.002
Matta, M. E., Orland, I. J., Ushikubo, T., Helser, T. E., Black, B. A., and Valley, J. W. (2013). Otolith oxygen isotopes measured by high-precision secondary ion mass spectrometry reflect life history of a yellowfin sole (Limanda aspera). Rapid Commun. mass Spectrom. 27 (6), 691–699. doi:10.1002/rcm.6502
Mcconnaughey, T. (1989). 13C and 18O isotopic disequilibrium in biological carbonates: II. in vitro simulation of kinetic isotope effects. Geochimica Cosmochimica Acta 53 (1), 163–171. doi:10.1016/0016-7037(89)90283-4
Morat, F., Blamart, D., Bounket, B., Argillier, C., Carrel, G., and Maire, A. (2023). Stable isotopic analysis of chub otolith from the Rhône River. figshare 2023, 22708111. doi:10.6084/m9.figshare.22708111.v1
Morat, F., Letourneur, Y., Blamart, D., Pécheyran, C., Darnaude, A. M., and Harmelin-Vivien, M. (2014). Offshore-onshore linkages in the larval life history of sole in the gulf of lions (NW-Mediterranean). Estuar. Coast. Shelf Sci. 149, 194–202. doi:10.1016/j.ecss.2014.08.023
Morissette, O., Trueman, C. N., Sturrock, A. M., Geffen, A. J., and Shirai, K. (2023). Limited evidence for species-specific sensitivity of temperature-dependent fractionation of oxygen stable isotope in biominerals: A meta-analysis. Methods Ecol. Evol. 00, 1719–1731. doi:10.1111/2041-210X.14122
Morrongiello, J. R., Thresher, R. E., and Smith, D. C. (2012). Aquatic biochronologies and climate change. Nat. Clim. Change 2 (12), 849–857. doi:10.1038/nclimate1616
Nazir, A., Ghosh, P., Sakthivel, T., and Khan, M. A. (2023). Stable isotopic analysis of long-whiskered catfish (Sperata aor) otoliths for characterization of their habitat and relationship with water temperature in the Ganges River. Curr. Chin. Sci. 3 (1), 57–66. doi:10.2174/2210298102666220909110222
Pálsson, Ó. K., and Thorsteinsson, V. (2003). Migration patterns, ambient temperature, and growth of Icelandic cod (Gadus morhua): Evidence from storage tag data. Can. J. Fish. Aquatic Sci. 60 (11), 1409–1423. doi:10.1139/f03-117
Panfili, J., De Pontual, H., Troadec, H., and Wright, P. J. (2002). “Manuel de sclérochronologie des Poissons,” in Coédition ifremer-IRD. Editors J. Panfili, H. de Pontual, H. Troadec, and P. J. Wright (France: IRD Éditions), 464.
Patterson, W. P., Smith, G. R., and Lohmann, K. C. (1993). Continental paleothermometry and seasonality using the isotopic composition of aragonitic otoliths of freshwater fishes. Clim. Change Cont. Isotopic Rec. 1993, 191–202. doi:10.1029/gm078p0191
Payne, N. L., Smith, J. A., Van Der Meulen, D. E., Taylor, M. D., Watanabe, Y. Y., Takahashi, A., et al. (2016). Temperature dependence of fish performance in the wild: Links with species biogeography and physiological thermal tolerance. Funct. Ecol. 30 (6), 903–912. doi:10.1111/1365-2435.12618
Price, G., Wilkinson, D., Hart, M., Page, K., and Grimes, S. (2009). Isotopic analysis of coexisting Late Jurassic fish otoliths and molluscs: Implications for upper-ocean water temperature estimates. Geology 37 (3), 215–218. doi:10.1130/g25377a.1
Schiemer, F., Keckeis, H., Nemeschkal, H., Schludermann, E., Winkler, G., and Zweimüller, I. (2004). Ontogenetic patterns in thermal adaptation of fish vs. long-term temperature trends in large rivers. Int. Congr. Ser. 1275, 209–217. doi:10.1016/j.ics.2004.09.039
Schrank, A. J., Rahel, F. J., and Johnstone, H. C. (2003). Evaluating laboratory-derived thermal criteria in the field: An example involving bonneville cutthroat trout. Trans. Am. Fish. Soc. 132 (1), 100–109. doi:10.1577/1548-8659(2003)132<0100:ELDTCI>2.0.CO;2
Smith, N. G., and Jones, C. M. (2006). Substituting otoliths for chemical analyses: Does sagitta= lapillus? Mar. Ecol. Prog. Ser. 313, 241–247. doi:10.3354/meps313241
Storm-Suke, A., Dempson, J. B., Reist, J. D., and Power, M. (2007). A field-derived oxygen isotope fractionation equation for Salvelinus species. Rapid Commun. Mass Spectrom. 21 (24), 4109–4116. doi:10.1002/rcm.3320
Stout, C. C., Tan, M., Lemmon, A. R., Lemmon, E. M., and Armbruster, J. W. (2016). Resolving Cypriniformes relationships using an anchored enrichment approach. BMC Evol. Biol. 16 (1), 244–313. doi:10.1186/s12862-016-0819-5
Surge, D., and Walker, K. J. (2005). Oxygen isotope composition of modern and archaeological otoliths from the estuarine hardhead catfish (Ariopsis felis) and their potential to record low-latitude climate change. Palaeogeogr. Palaeoclimatol. Palaeoecol. 228 (1-2), 179–191. doi:10.1016/j.palaeo.2005.03.051
Thorrold, S. R., Campana, S. E., Jones, C. M., and Swart, P. K. (1997). Factors determining δ13C and δ18O fractionation in aragonitic otoliths of marine fish. Geochimica Cosmochimica Acta 61, 2909–2919. doi:10.1016/S0016-7037(97)00141-5
Tonolla, D., Wolter, C., Ruhtz, T., and Tockner, K. (2012). Linking fish assemblages and spatiotemporal thermal heterogeneity in a river-floodplain landscape using high-resolution airborne thermal infrared remote sensing and in-situ measurements. Remote Sens. Environ. 125, 134–146. doi:10.1016/j.rse.2012.07.014
Van Der Have, T. M., and De Jong, G. (1996). Adult size in ectotherms: Temperature effects on growth and differentiation. J. Theor. Biol. 183 (3), 329–340. doi:10.1006/jtbi.1996.0224
Von Leesen, G., Bardarson, H., Halldórsson, S. A., Whitehouse, M. J., and Campana, S. E. (2021). Accuracy of otolith oxygen isotope records analyzed by SIMS as an index of temperature exposure of wild Icelandic cod (Gadus morhua). Front. Mar. Sci. 8. doi:10.3389/fmars.2021.698908
Watkins, J. M., Nielsen, L. C., Ryerson, F. J., and Depaolo, D. J. (2013). The influence of kinetics on the oxygen isotope composition of calcium carbonate. Earth Planet. Sci. Lett. 375, 349–360. doi:10.1016/j.epsl.2013.05.054
West, C. F., Wischniowski, S., and Johnston, C. (2012). Pacific cod (Gadus macrocephalus) as a paleothermometer: Otolith oxygen isotope reconstruction. J. Archaeol. Sci. 39 (10), 3277–3283. doi:10.1016/j.jas.2012.05.009
Keywords: water temperature, fractionation equation, European chub, Squalius cephalus, Rhône river
Citation: Morat F, Blamart D, Bounket B, Argillier C, Carrel G and Maire A (2023) Reconstructing the thermal history of fish juveniles using stable oxygen isotope analysis of otoliths. Front. Environ. Sci. 11:1213239. doi: 10.3389/fenvs.2023.1213239
Received: 27 April 2023; Accepted: 06 July 2023;
Published: 14 July 2023.
Edited by:
Maria Filomena Magalhães, University of Lisbon, PortugalReviewed by:
Florian Borgwardt, University of Natural Resources and Life Sciences Vienna, AustriaAafaq Nazir, National Taiwan University, Taiwan
Copyright © 2023 Morat, Blamart, Bounket, Argillier, Carrel and Maire. This is an open-access article distributed under the terms of the Creative Commons Attribution License (CC BY). The use, distribution or reproduction in other forums is permitted, provided the original author(s) and the copyright owner(s) are credited and that the original publication in this journal is cited, in accordance with accepted academic practice. No use, distribution or reproduction is permitted which does not comply with these terms.
*Correspondence: Fabien Morat, ZmFiaWVuLm1vcmF0QHVuaXYtcGVycC5mcg==