- 1Institute of Aquatic Ecology, Centre for Ecological Research, Budapest, Hungary
- 2National Laboratory for Water Science and Water Security, Institute of Aquatic Ecology, Centre for Ecological Research, Budapest, Hungary
- 3Doctoral School of Environmental Sciences, Eötvös Loránd University, Budapest, Hungary
- 4Faculty of Engineering, University of Pannonia, Veszprém, Hungary
Introduction: Unioniade is a species-rich family of freshwater mussels with an almost worldwide distribution. In Central European rivers Unio crassus, Unio tumidus and Unio pictorum have relatively high populations. In order to characterize the habitats of these suspension feeders, their nutrient supply and potential metal contaminants were investigated in near-bottom suspended (NBS) sediments and bottom sediments (BS) collected at four sampling locations along the Tisza River.
Methods: The grain size distribution of sediments was determined by laser-diffraction technique. The total organic carbon (TOC) and the total Kjeldahl nitrogen (TN) were measured by a TOC analyzer and automated colorimetry, respectively. The phosphorous and metal concentrations were determined by inductively coupled plasma mass spectrometry following a microwave assisted acidic extraction of sediments with aqua regia.
Results: The NBS sediments contained smaller grains than the BS samples, with roughly 85%–90% of the particles being less than 60 μm, allowing these mussels to catch them. Contrarily, only 2.47%–51.8% of BS samples were less than 60 μm. The NBS sediments contained 30, 23, and 15% more organic carbon, nitrogen, and phosphorous, respectively, than the BS samples. The rounded C:N:P molar ratios in the NBS sediments were 60:4:1, whereas they were 45:3:1 in the bottom sediments. The concentration of metals was also greater in the NBS sediments with the exception of Zn at Tokaj and Fe, Al, Mn, and Cr at Szeged, where prior contaminations transported by the tributaries Bodrog and Maros are stored in the top 10 cm of BS.
Discussion: On basis of the measured C:N:P molar ratios it can be stated that soil-derived organic materials are the main sources of the nutrient elements in the habitats of suspension feeders in the Tisza River. The finer NBS sediments have higher specific surface area than the BS and thereby contain more biofilms on the grains resulting in higher proportion of organic nutrients and metal ions bounded as complexes or chelates. Comparing the measured metal concentrations of sediments with the concentration limits summarized in the Consensus-Based Sediment Quality Guidelines developed for evaluation of potential risk of metal contaminants in sediments for the benthic-dwelling organisms, it can be established that only the Hg concentration exceeded the probable effect concentration.
1 Introduction
The freshwater mussels of the family Unionidae play a dominant role in freshwater ecosystems due to their suspension-feeding, waste excretion, and burrowing activities, as outlined by (Goldsmith et al., 2021). These mussels, which are partially embedded in the bottom sediments ingest organic nutrients, minerals, and microplastic particles from both the continuously flowing NBS sediments and the bottom sediments that have been resuspended by their burrowing. It implies a continuous interaction between the mussels and sediments in the benthic habitat. For instance, sediment impacts on mussels cause smothering, a drop in fish abundance, and decline in feeding and respiration. The increased concentration of suspended sediments could have a deleterious effect on mussel growth, survival, and reproduction, which could eventually result in changes in diversity (Box and Mossa, 1999). On the other hand, mussels-mediated nutrient dynamics, biodeposition, and bioturbation can alter the chemical composition and properties of sediments (Vaughn and Hakenkamp, 2001; Haag, 2012; Hoellein et al., 2017). Particulate nutrients (organic C, N, and P) and other key elements (Fe, Mn, and Si) have a substantial influence on the ecology and biochemistry of aquatic environments by regulating the availability of dissolved nutrients, affecting light availability, influencing phytoplankton stocks, growth, grazing rates and community structure, as well as affecting food webs (Turner and Millward, 2002; Beusen et al., 2005; Bilotta and Brazier, 2008; Hickey et al., 2010).
In order to characterize the habitats of mussels in rivers, it is necessary to investigate the physical and chemical properties of the water phase, the NBS sediment, and deposited (bottom) sediments. However, it should be noted that there is a dynamic feedback interaction between the river bed, flow, and mobile sediments (Shu et al., 2020). Despite the fact that there is limited possibility to uptake dissolved organic carbon and trace elements from the water phase, as was demonstrated by (Roditi et al., 2000) in Zebra mussels, the primary process of nutrition is based on the ingestion of suspended organic particles (Pan and Wen-Xiong, 2004).
To evaluate the nutrient supply of bottom-dwelling species, mostly the total organic carbon (TOC), total nitrogen (TN), total phosphorous (TP), as well as the TOC/TN concentration or molar ratios were measured in the bottom and suspended sediments. The majority of published data are on bottom sediments since sampling surface sediments using grab samplers or augers is a relatively straightforward process compared to sampling suspended sediments (Supplementary Table S1). On the basis of these selected literature data, it can be established that in the surface layer of bottom sediments in riverine environment, the concentrations of TOC, TN, and TP, as well as the TOC/TN ratio, changed in a relatively wide range of 0.14%–22%, 0.07%–1.45%, 0.04%–0.88%, and 0.9–111, respectively. At this point, it should be stressed that these nutrient concentration measurements only describe the surface sediments at the time of sampling, and we must estimate any short- or long-term changes brought on by physical and chemical (degradation) processes (Dalu et al., 2019; Islam et al., 2019).
In the case of suspended sediments (suspended particulate matter, SPM), which include both living (algae, bacteria) and non-living (e.g., inorganic minerals and organic detritus) compounds, we must take into account that their depth profiles in the rivers change continuously based on the hydrologic characteristics, rainfall or storm events, watershed pattern, vegetation, hydraulic conditions, physical characteristics (size, shape, and density) and hydrodynamic behaviour of particles (Kumar et al., 2021). Since the sampling procedure for suspended sediments is more sophisticated than for bottom sediments, it is challenging to receive reliable concentration data on depth profiles of nutrient elements and contaminants in rivers using different sampling devices and sampling strategies. Nevertheless, despite the measurement uncertainty of element concentrations, the C/N concentration ratios provide reliable information on the origin of organic compounds. The potential sources of organic materials include (1) living or dead algae and bacteria, (2) degraded soil organic matter, and (3) plant materials.
In addition to the transport of nutrients, it is also important to study the sediment-associated transport of essential and toxic metals across the mussel habitat. Since all particles are blanketed with biofilms (Mages et al., 2004; Prieto et al., 2016), the metal ions can be immobilized in the extracellular polymer matrix by a variety of mechanisms, including biosorption, precipitation as sulfides or phosphates, and reductive microbial precipitation (van Hullebusch et al., 2003). The biofortification factor of biofilms formed on artificial substrata in the Tisza River varied in the range of 103 and 105 for different heavy metals (Kröpfl et al., 2006). Because of these aforementioned processes in the biofilm layer, it is anticipated that the concentration of metals and metalloids will be higher in the finer suspended sediments due to decreasing grain size and increasing specific surface area of particles, as compared to the deposited bottom sediments. The relationship between the physico-chemical properties of sediments and their metal content was studied by (Dendievel et al., 2022) in seven Western European Rivers. On the basis of a dataset combining long-time monitoring and scientific data (>12,000 samples), they quantified the influence of key factors (sediment matrix type, TOC content, grain size distribution, fractionation, location, and time) on the metal content of sediments.
Some characteristic pseudo-total concentrations of the most widely measured metal contaminants (Zn, Cd, Pb, Ni, Cu, and Cr) in river surface sediments are listed in Supplementary Table S2. Their concentrations vary by many orders of magnitude in the range of 0.1–1,000 mg/kg, depending on lithogenic and anthropogenic sources, textural traits, organic matter contents, mineralogical composition, and depositional environment of sediment.
In this work, our objectives were (1) to determine the physical and chemical properties of NBS sediments and bottom sediments collected at four sampling locations along the Tisza River within the habitats of Unionidae mussels (Unio tumidus, Unio crassus, Unio pictorum), (2) to investigate the relationships between the grain size of sediments and concentrations of nutrient elements and metal contaminants (3) to identify the main sources of nutrient elements on basis of C/N ratios determined in sediments (4) to evaluate the potential risk of metal contaminants for mussels as bottom-dwelling animals considering the Concensus-Based Sediment Quality Guidelines (MacDonald et al., 2000).
2 Materials and methods
2.1 Sampling sites and techniques
The Tisza River, which originates in the Bukovina segment of the Carpathian Mountains, is the main tributary of the middle Danube River. Its length and drainage area are 966 km and 157,186 sq. km, respectively. Along the Hungarian section of the Tisza River, four sampling sites at the settlements of Tímár, Tokaj, Csongrád, and Szeged, which are located at flow km of 552, 544, 244, 160, respectively, were selected (Figure 1). The sediment samples were collected in the time period of August 2–4, when the algal population was relatively high and defined by relatively high chlorophyll-a levels (20 μg/L). At all sampling sites, three sampling points located about 6–8 m from the river bank and spaced roughly 4–5 m apart were selected. At these sampling points, 5 L of suspension was pumped into amber bottles from the 10–15 cm thick suspension layer streaming over the bottom of the riverbed using a portable, pressure-difference “SEDIMONER” sampler developed by Aqua-Terra Lab Ltd. (Veszprém, Hungary). Although this sampling method does not produce an isokinetic sample, it is more accurate when sediments are fine (<63 µm) and flows are turbulent. Van Veen grab sampler was used for sampling bottom sediments, allowing samples to be collected from a nearly 10 cm thick surface layer of bottom sediments. About 1 kg of sample was collected at each sampling point and transferred into plastic bags with zip-fastener. The suspension and sediment samples were stored on ice during transport to the laboratory.
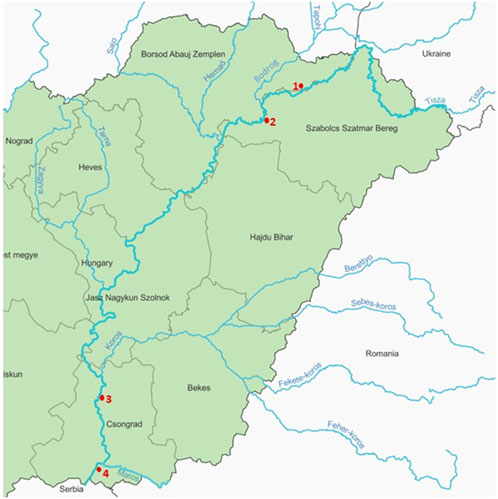
FIGURE 1. Hydrographic map of Tisza River and its tributaries arriving from Slovakia (Bodrog, Sajó) and Romania (Kőrös, Maros). The four sampling sites located at Timar (1), Tokaj (2), Csongrád (3), and Szeged (4) are denoted by a red circle.
For the characterization of the water phase, the water temperature, pH, dissolved oxygen concentration, redox potential, electric conductivity, and turbidity were measured on-site using a portable Ponsel-ODEON water quality meter (Fondriest Environmental, Ohio, United States). During the campaign, the flow velocity was measured by a rotating current meter (Global Water Flow Probe FP 211, Xylem, United States). The concentrations of selected anions, cations, as well as total organic C and N of water samples obtained after filtration of NBS sediments were determined by applying DIONEX 5000 ICS + dual channel ionchromatograph (Thermo Scientific, Massachusetts, United States) and a MULTI N/C 3100 TC/TN analyzer (Analytik Jena, Jena, Germany), respectively. The elemental concentrations were quantified using an inductively coupled plasma mass spectrometer (Analytik Jena, Jena, Germany). These parameters are listed in the Supplementary material (Supplementary Tables S3, S4).
2.2 Chemicals and reagents
A WasserLab Automatic unit (Labsystem Ltd., Budapest, Hungary) was used for generating ultrapure water (resistivity of 18.2 MΩ cm−1). Nitric, hydrochloric, and sulphuric acids, hydrogen peroxide, and solid natrium hydroxide of analytical grade were purchased from VWR International Ltd. (Debrecen, Hungary). The internal and multi-elemental standard solutions were produced by Sigma-Aldrich Ltd. (Missouri, United States).
2.3 Determination of total suspended solids
The suspension samples were homogenized and three samples of 200 mL each were separately filtered using pre-combusted glass fiber filters with a pore size of 0.7 µm. The empty filters were weighed before the filtration. The loaded filters were dried at 80°C for 12 h and re-weighed to determine the dry mass of solid particles for calculation of the mass concentration of TSS.
2.4 Determination of grain size distribution
The bottom sediments were wet-sieved over a 2 mm mesh to eliminate large detritus and benthic organisms and dried at 80°C for 12 h in a laboratory oven together with the suspended sediments. Three replicates of both sediment samples were individually homogenized. About 30–90 mg of these samples were resuspended in 200 cm3 ultrapure water and subjected to 5 min of ultrasonic treatment at a frequency of 32 kHz. The cumulative and differential distribution functions were determined with the use of a Shimadzu SALD-2300 laser diffraction particle size analyzer (Shimadzu, Kyoto, Japan).
2.5 Chemical characterization of sediment samples
For measuring the TOC contents, 300–500 mg dried and homogenized sediment samples were analyzed using a Multi N/C 3100 analyzer (Analytik Jena, Jena, Germany). The total Kjeldahl nitrogen (TN) of dried sediments was measured by automated colorimetry with preliminary distillation/digestion on the basis of Standard Method 1,687 (EPA-821-R-01-004, January 2001).
To determine the pseudo-total concentration of phosphorus (TP), arsenic, and different metals, 500 mg dried sediments were treated with 8 cm3 aqua regia at a temperature of 200°C for 20 min in a TopWave microwave-assisted digestion system (Analytik Jena, Jena, Germany). Three replicates were prepared for all sediment samples. Following the sedimentation of solid particles (predominantly silicates), 2 cm3 of the clear solution was removed by a pipette and diluted 25-fold with ultrapure water. After the addition of internal standards (Sc, Y, In) in concentration of 20 μg/L, the main (Al, Fe, Mn, P) and trace elements (As, Cd, Co, Cr, Cu, Hg, Li, Ni, Pb, Sn, Zn) were determined using a Plasma Quant Elite inductively coupled plasma mass spectrometer (Analytik Jena, Jena, Germany). The operating conditions are listed in Supplementary Table S5. To characterize the reliability of this analytical procedure a recovery test was carried out for 8 elements (Cd, Cr, Cu, Hg, Mn, Ni, Pb, Zn) analyzing the BCR-146R (Sigma Aldrich, Missouri, United States) certified reference material. The recovery values changed in the range of 82% and 114%.
2.6 Statistical methods
Data were visualized using R statistical software (R Core Team, 2019). The relationship between TOC and metal/metalloid concentrations was examined by Person’s correlation after checking the assumption of the normal distribution. Figures 3A–D were drawn with the tkplot function of the “igraph” package (Csardi and Nepusz, 2006) using the Pearson’s correlation coefficients and p-values as input data.
3 Results and discussion
3.1 Physical characterization of sediments
The mass concentration of NBS sediment particles fluctuated between 209 and 274 mg/L. These values are similar to TSS concentrations of 70–247 mg/L measured at middle depth in the Yellow River, however, considerably lower than the TSS concentrations in the 2:1:1 ratio mixed subsurface, middle and near-bed water samples of Yangtze River (Wang et al., 2012).
The grain size distribution functions of suspended and bottom sediments originating from the same sampling site are depicted in Figures 2A–D. The bottom sediments can be characterized by a high maximum value within the typical sand size range of 60–500 μm, while the NBS-sediments primarily consist of silt (size range 2–60 µm). In its grain size distribution functions, three different grain size groups can be observed, signifying the existence of particles originating from various sources. In all NBS sediments the maximum values are observable approximately at particle sizes of 6, 20, and 60 μm. Considering the particle capture efficiency of mussels, even this size range is important for the nutrient supply of Unionidae mussels (Kryger and Riisgård, 1988). However, (Tuttle-Raycraft and Ackerman, 2018), concluded in their paper that particle quality rather than size is what modulates suspension feeding in turbid waters. In the typical clay grain size range (<2 µm), particles were not detectable in the bottom sediments collected at Tokaj and Szeged. This phenomenon can be explained by the hydrodynamic sorting of the rivers (higher stream velocities at these sampling points (0.25 vs. 0.1 m/s). However, in all NBS sediment samples, around 5%–10% of the particles were found to be in this size range. In conclusion, silt predominates among the NBS sediments investigated, which is consistent with the finding made by (Bouchez et al., 2011) in the instance of the Amazon River.
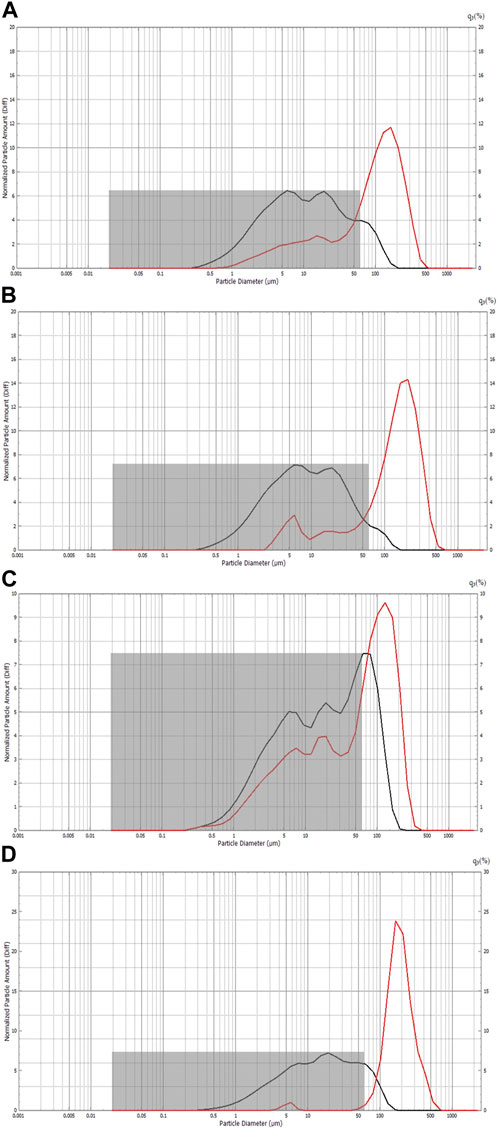
FIGURE 2. Grain size distribution of NBS sediments (black) and bottom sediments (red) collected at sampling sites Tímár (A) Tokaj (B), Csongrád (C), and Szeged (D).
It should be noted that our data on grain size measurements do not accurately reflect the grain size distributions that originally existed in the mussel habitats. This is because the cohesive suspended sediments are transported primarily as flocculated material (Droppo, 2001; Deng et al., 2019), but the flocs were damaged during the sample preparation by drying and resuspension of solid particles applying ultrasonic treatment before the grain size measurements. It implies that the effect of flocculation processes governed by extracellular polymeric substances (Lee et al., 2017) is partly eliminated. In order to avoid these undesirable disturbances and to obtain a true picture of the particle sizes of suspended sediments, it would be necessary to apply in-situ methods (e.g., submersible particle size analyzer and submersible digital holographic camera), as was demonstrated by (Safar et al., 2022). In the absence of such devices during our investigations, the suspended particles were collected and analysed in the conventional manner described in subchapters 2.1 and 2.4.
3.2 Chemical characterization of sediments
3.2.1 Study of nutrient elements
The suspension-feeder mussels can take the main nutrients (C, N, P) from three different sources: (1) particulate organic matter, e.g., algae, plant residues, bacteria, (2) biofilms formed on the surface of all inorganic and organic particles existing in the water, (3) dissolved organic compounds from the water phase. This third source plays only a negligible role in the supply of nutrients, as it was established by Roditi et al. (2000) in case of Zebra mussels. However, the biofilms containing microbial cells and extracellular polymeric substances (polysaccharides, lipids, nucleic acids, etc.) serve as a well utilized source of nutrients. The chemical investigations were focused on the determination of nutrients (TOC, TN, and TP) and the C:N:P molar ratios. The measured concentrations of these three nutrient elements in the NBS and the bottom sediments are listed in Table 1. It can be established that the TOC concentrations in the NBS sediments were higher by a factor of 1.7–3.4, compared to the bottom ones. An exception was the sampling site Csongrád, where the river’s velocity was extremely low (≤0.1 m/s) in a small muddy bay. It should be noted that the TOC values of bottom sediments were consistent across all sampling sites and only varied in a narrow concentration range of 9.1–10.6 mg/g.
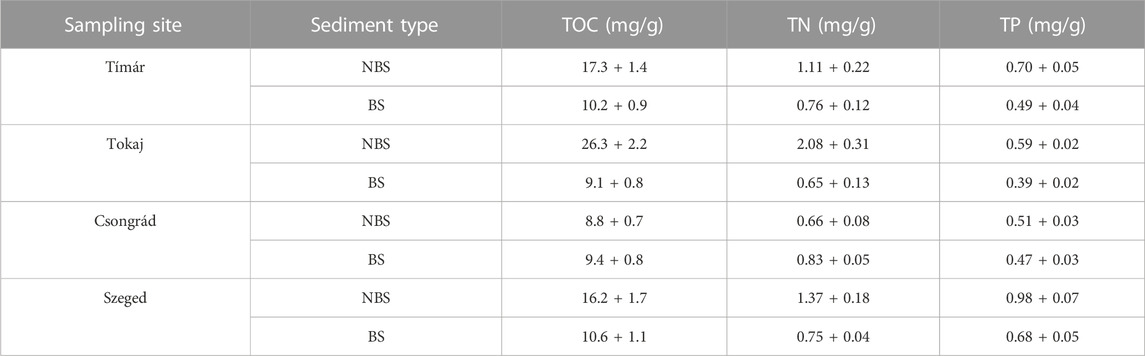
TABLE 1. Total organic carbon (TOC), total nitrogen (TN), and total phosphorous (TP) concentrations of NBS and bottom sediments (BS) in the Tisza River.
In the case of TN and TP content, the tendency of concentration fluctuations between the NBS and bottom sediments is similar to the TOC. The highest TOC and TN values were measured at the sampling site Tokaj, below the mouth of the tributary Bodrog, whereas the highest TP values were recorded at Szeged, below the mouth of the tributary Maros. Summarizing, it can be stated that the nutrient concentrations in the Tisza River’s NBS sediments at sampling sites with flow rates of 0.1–0.25 m/s are higher than those in the bottom sediments.
The C/N molar ratios were calculated (Table 2) to provide information on the bacterial-algal, soil-derived, or vascular land-plant origins of sedimentary organic matter. In reality, organic molecules from all three sources can participate in the formation of the current C/N values, albeit in varying proportions. Bacteria and algae typically have C/N molar ratios between 4 and 7, soil-derived organic compounds have between 8 and 20, and vascular land plants have 20 or greater (Meyers, 1994; Tyson, 1995). This distinction arises from the absence of cellulose in bacteria and algae and its abundance in vascular plants and the consequent relative richness of proteinaceous material in the aforementioned microorganisms. Since the C/N molar ratios in the NBS sediments and bottom sediments varied in the range of 13.7–18 and 13.1–16.3, respectively, it can be stated that the soil-derived organics are the dominant sources of organic matter in these samples. Similar C/N values (10–12) were published for Amazon (Hedges et al., 1986), St. Lawrence (Pocklington and Tan, 1987), Yellow and Yangtze Rivers (Wang et al., 2012) as well as for rivers in Central United States (Onstad et al., 2000). Our higher ratios can be attributed to the near-bottom sampling, while these literature data are related to surface or middle depth water samples.
The C/P and N/P molar ratios were also higher in the NBS sediments, with the exception of the muddy bay at sampling site Csongrád, and their highest values were also detected at sampling site Tokaj. This indicates that the highest nutrient supply for the suspension-feeder mussels is available at this settlement below the inflow of the tributary Bodrog.
3.2.2 Study of metal contaminants
The various metal and As contaminants (Table 3) were typically present in higher concentrations in the NBS sediments at Timár, Tokaj, and Csongrád than in the bottom sediments, with exception of Zn in samples collected at Tokaj. This metal is likely stored in the bottom sediments as a result of a former Zn contamination delivered by the tributary Bodrog. In the case of sediments collected at Szeged, a tributary effect of the Maros River was evident, resulting in higher concentration of Al, Mn, Cr, and Sn in the bottom sediments. The differences between the measured metal concentrations in the NBS sediments and the bottom sediments can be characterized by factor 1.1–2.2. The highest deviations for most metals in the suspended and deposited sediments were measured at the sampling site Tokaj, where the TOC and TN concentrations also had maximum values (Table 1). To evaluate these measurement data it should also be considered, that the biofilms due to their free–COOH and–OH groups are able to bind dissolved metal ions as chelates or complexes. Therefore, decreasing grain size of sediment particles and increasing specific surface area of biofilms grown on sediment particles leads to increasing metal concentrations of the finer NBS sediments. The network representation of relationships between the concentrations of the investigated components in NBS and bottom sediments are demonstrated in Figure 3, while the calculated coefficients are listed in Supplementary Table S6.
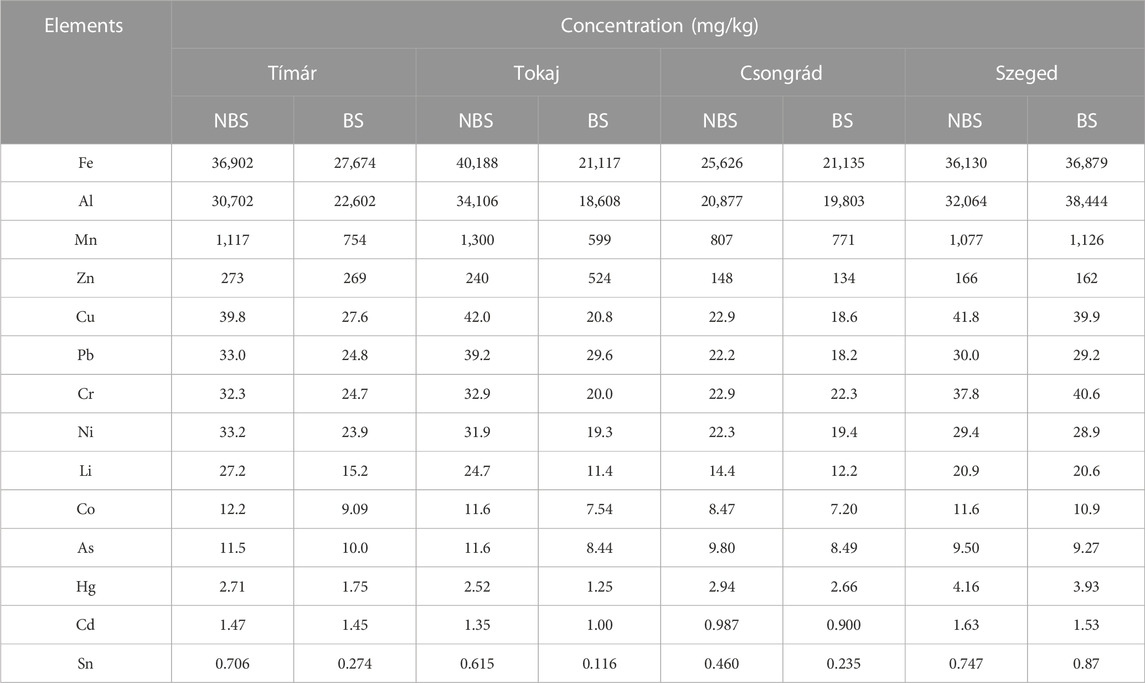
TABLE 3. Mean pseudo-total concentrations of major and trace elements in the NBS sediments and bottom sediments (BS) of Tisza River at four different sampling sites.
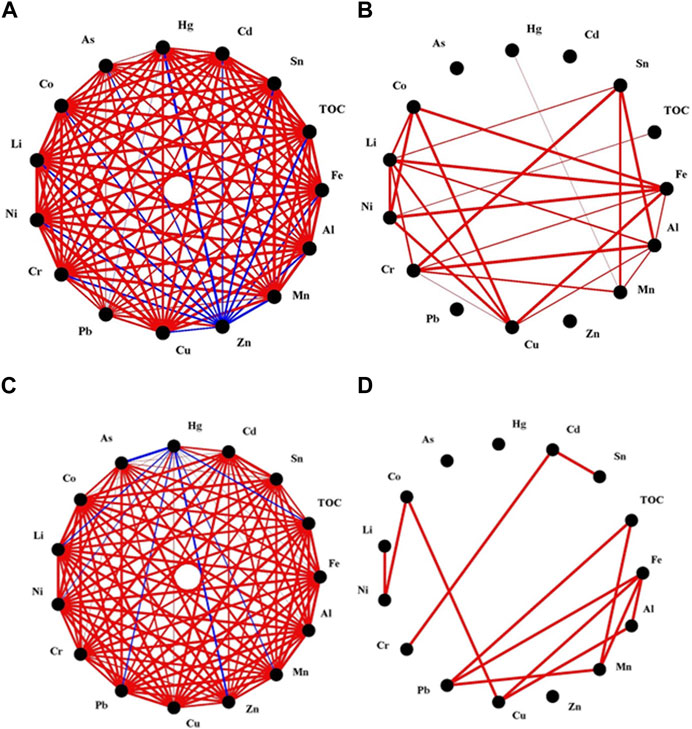
FIGURE 3. Network representation of the relationships between the concentrations of elements investigated and TOC in the NBS (A,B) and bottom (C,D) sediments. Elements appear as nodes, while the connecting lines between them represent the correlations. In subfigures (A,C), all correlations are shown, while in subfigures (B,D), only the significant correlations (p < 0.05) are depicted. The colours of the links indicate the direction of the relationship: red links denote positive correlations while blue links signify negative correlations. The width of the links is correlated with the strength of the correlation (i.e., the absolute values of the Pearson’s correlation coefficients).
On the basis of these statistical data, it can be stated that the majority of the metals investigated, with exception of Cd, Cr, Hg, and Sn in NBS and Pb and Zn in bottom sediments, displayed a strong positive correlation with TOC (correlation coefficient “r” ranged from 0.68 to 0.98). However, significant positive correlations (r = 0.98, p = 0.04) with TOC were found solely in the case of Mn and Pb in the NBS and Ni in the bottom sediments. Only Hg in NBS and Zn in bottom sediments showed negative correlation with TOC. Our findings concur with those of (Dendievel et al., 2022) and (Miranda et al., 2021), who found that the key factors influencing metal concentrations of sediments are their grain size and TOC content.
Correlations between different elements revealed distinct pictures, depending on the sediment type. For instance, in the NBS and bottom sediments, Fe had significant correlations with Al, Co, Cu, Li, Ni, and Al, Cu, Mn, Pb, respectively. In the NBS sediments, Zn demonstrated negative correlations with all other elements (except Pb), while in the bottom sediments, Hg displayed negative correlations with As, Li, Mn, Ni, Pb, and Zn. However, As and Zn concentrations did not display any significant correlations with any other elements in both sediment types.
The Consensus-Based Sediment Quality Guidelines (CBSQGs) developed by MacDonald et al., 2000, might be usefully viewed in the context of evaluating the potential risk of metal contaminants. The CBSQGs contain threshold effect concentrations (TEC) and probable effect concentrations (PEC) for 10 elements, which are the concentrations at which toxicity to benthic-dwelling organisms is predicted to be unlikely and probable, respectively. The concentration range of metal contaminants determined in the NBS and bottom sediment samples, as well as the TEC and PEC values are listed in Table 4. It can be established that the measured concentrations of As, Cd, Cr, Cu, Pb, and Ni in both type of sediments was lower than their PEC values. The Fe, Mn, and Zn concentrations were mostly between their respective TEC and PEC limits. However, the risk of Hg contamination can be predicted since its concentration in all sediment samples exceeded the PEC value.
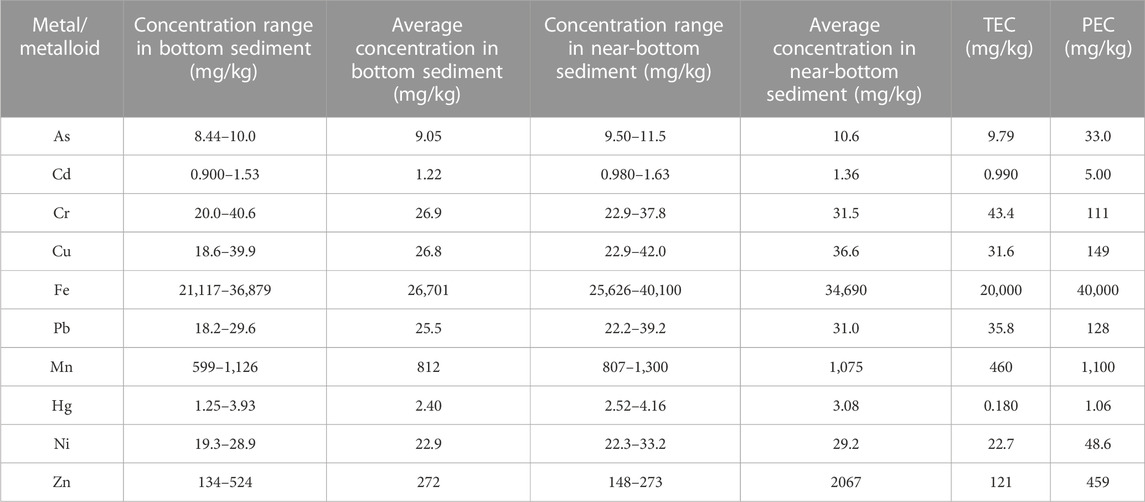
TABLE 4. Average and concentration range of metals determined in the bottom sediments (BS) and near-bottom sediments (NBS) of Tisza River and the recommended sediment quality guideline values for metals and associated levels of concern to be used while conducting sediment quality assessment.
4 Conclusion
It should be emphasized that the chemical information preserved by bottom sediments characterize a longer integration period of nutrients and contaminants, such as a few months or years, depending on the thickness of the investigated sediment layer. The sampling and investigation of the NBS sediments provide information on the momentary (temporal) concentration of contaminants and simultaneously on the actual concentration of seasonally changing nutrients. Our findings with regards to the bottom sediments sampled in sizable shellfish fields in the Tisza River illustrate the geochemical background of this catchment area, the “zoo-geochemical additives” of bottom-dwelling animals, and anthropogenic contaminants originating from different industrial and agricultural sources. In addition, the soil-derived organic materials contributed the major nutrients in the habitat of Unionidae mussels and their concentrations were considerably influenced by the tributaries. Considering the concentration limits listed in CBSQGs for heavy metals in sediment matrices, only the Hg contamination can generate a probable toxic effect in Unionidae mussels as benthic dwelling organisms.
Data availability statement
The raw data supporting the conclusion of this article will be made available by the authors, without undue reservation.
Author contributions
PD wrote the first draft of the manuscript. WA, ÁI were involved in the analytical experimental work. DT, SS, and ZK were participated in the sampling and sample preparation. AE conducted the statistical data analyses and data visualization. GZ contributed to conception and design of the study and gave final approval of the version to be published. All authors contributed to the article and approved the submitted version.
Funding
This research was supported by the Economic Development and Innovation Operational Program (Grant No. GINOP-2.2.1-15-2017-00070). The research presented in the article was carried out within the framework of the Széchenyi Plan Plus program with the support of the RRF 2.3.1 21 2022 00008 project.
Conflict of interest
The authors declare that the research was conducted in the absence of any commercial or financial relationships that could be construed as a potential conflict of interest.
Publisher’s note
All claims expressed in this article are solely those of the authors and do not necessarily represent those of their affiliated organizations, or those of the publisher, the editors and the reviewers. Any product that may be evaluated in this article, or claim that may be made by its manufacturer, is not guaranteed or endorsed by the publisher.
Supplementary material
The Supplementary Material for this article can be found online at: https://www.frontiersin.org/articles/10.3389/fenvs.2023.1209118/full#supplementary-material
References
Aguilar Pesantes, A., Peña Carpio, E., Vitvar, T., María Mahamud López, M., and Menéndez-Aguado, J. (2019). A multi-index analysis approach to heavy metal pollution assessment in river sediments in the ponce enríquez area, Ecuador. Water 11, 590. doi:10.3390/w11030590
Barhoumi, B., Beldean-Galea, M. S., Al-Rawabdeh, A. M., Roba, C., Martonos, I. M., Bălc, R., et al. (2019). Occurrence, distribution and ecological risk of trace metals and organic pollutants in surface sediments from a Southeastern European river (Someşu Mic River, Romania). Sci. Total Environ. 660, 660–676. doi:10.1016/j.scitotenv.2018.12.428
Beusen, A. H. W., Dekkers, A. L. M., Bouwman, A. F., Ludwig, W., and Harrison, J. (2005). Estimation of global river transport of sediments and associated particulate C, N, and P. Glob. Biogeochem. Cycles 19. doi:10.1029/2005GB002453
Bilotta, G. S., and Brazier, R. E. (2008). Understanding the influence of suspended solids on water quality and aquatic biota. Water Res. 42, 2849–2861. doi:10.1016/j.watres.2008.03.018
Bouchez, J., Gaillardet, J., France-Lanord, C., Maurice, L., and Dutra-Maia, P. (2011). Grain size control of river suspended sediment geochemistry: Clues from Amazon River depth profiles. Geochem. Geophys. Geosyst. 12. doi:10.1029/2010GC003380
Box, J. B., and Mossa, J. (1999). Sediment, land use, and freshwater mussels: Prospects and problems. J. North Am. Benthol. Soc. 18, 99–117. doi:10.2307/1468011
Csardi, G., and Nepusz, T. (2006). The igraph software package for complex network research. InterJournal, Complex Syst. 1695, 1.
Dalu, T., Wasserman, R. J., Magoro, M. L., Froneman, P. W., and Weyl, O. L. F. (2019). River nutrient water and sediment measurements inform on nutrient retention, with implications for eutrophication. Sci. Total Environ. 684, 296–302. doi:10.1016/j.scitotenv.2019.05.167
de Paula Filho, F. J., Marins, R. V., de Lacerda, L. D., Aguiar, J. E., and Peres, T. F. (2015). Background values for evaluation of heavy metal contamination in sediments in the Parnaíba River Delta estuary, NE/Brazil. Mar. Pollut. Bull. 91, 424–428. doi:10.1016/j.marpolbul.2014.08.022
Dendievel, A.-M., Grosbois, C., Ayrault, S., Evrard, O., Coynel, A., Debret, M., et al. (2022). Key factors influencing metal concentrations in sediments along western European rivers: A long-term monitoring study (1945–2020). Sci. Total Environ. 805, 149778. doi:10.1016/j.scitotenv.2021.149778
Deng, Z., He, Q., Safar, Z., and Chassagne, C. (2019). The role of algae in fine sediment flocculation: In-situ and laboratory measurements. Mar. Geol. 413, 71–84. doi:10.1016/j.margeo.2019.02.003
Droppo, I. G. (2001). Rethinking what constitutes suspended sediment. Hydrol. Process. 15, 1551–1564. doi:10.1002/hyp.228
Gielar, A., Helios-Rybicka, E., Möller, S., and Einax, J. W. (2012). Multivariate analysis of sediment data from the upper and middle Odra River (Poland). Appl. Geochem. 27, 1540–1545. doi:10.1016/j.apgeochem.2012.04.004
Goldsmith, A. M., Jaber, F. H., Ahmari, H., and Randklev, C. R. (2021). Clearing up cloudy waters: A review of sediment impacts to unionid freshwater mussels. Environ. Rev. 29, 100–108. doi:10.1139/er-2020-0080
Haag, W. R. (2012). North American freshwater mussels: Natural history, ecology, and conservation. Cambridge [England] ; New York: Cambridge University Press.
Hedges, J. I., Clark, W. A., Quay, P. D., Richey, J. E., Devol, A. H., and Santos, M. (1986). Compositions and fluxes of particulate organic material in the Amazon River1: Amazon River particulate material. Limnol. Oceanogr. 31, 717–738. doi:10.4319/lo.1986.31.4.0717
Hickey, B. M., Kudela, R. M., Nash, J. D., Bruland, K. W., Peterson, W. T., MacCready, P., et al. (2010). River influences on shelf ecosystems: Introduction and synthesis. J. Geophys. Res. 115, C00B17. doi:10.1029/2009JC005452
Hoellein, T. J., Zarnoch, C. B., Bruesewitz, D. A., and DeMartini, J. (2017). Contributions of freshwater mussels (Unionidae) to nutrient cycling in an urban river: Filtration, recycling, storage, and removal. Biogeochemistry 135, 307–324. doi:10.1007/s10533-017-0376-z
Ilie, M., Marinescu, F., Anghel, A.-M., Ghiță, G., Deák, G., Raischi, M., et al. (2016). Spatial distribution of heavy metal contamination in surface sediments from the Danube River. Int. J. Environ. Sci. 1.
Islam, M. J., Jang, C., Eum, J., Jung, S., Shin, M.-S., Lee, Y., et al. (2019). C:N:P stoichiometry of particulate and dissolved organic matter in river waters and changes during decomposition. J. Ecol. Environ. 43, 4. doi:10.1186/s41610-018-0101-4
Kraft, C., von Tümpling, W., and Zachmann, D. W. (2006). The effects of mining in Northern Romania on the heavy metal distribution in sediments of the rivers Szamos and Tisza (Hungary). Acta hydrochim. hydrobiol. 34, 257–264. doi:10.1002/aheh.200400622
Kröpfl, K., Vladár, P., Szabó, K., Ács, É., Borsodi, A. K., Szikora, S., et al. (2006). Chemical and biological characterisation of biofilms formed on different substrata in Tisza river (Hungary). Environ. Pollut. 144, 626–631. doi:10.1016/j.envpol.2006.01.031
Kryger, J., and Riisgård, H. U. (1988). Filtration rate capacities in 6 species of European freshwater bivalves. Oecologia 77, 34–38. doi:10.1007/BF00380921
Kumar, R., Sharma, P., Verma, A., Jha, P. K., Singh, P., Gupta, P. K., et al. (2021). Effect of physical characteristics and hydrodynamic conditions on transport and deposition of microplastics in riverine ecosystem. Water 13, 2710. doi:10.3390/w13192710
Lee, B., Hur, J., and Toorman, E. (2017). Seasonal variation in flocculation potential of river water: Roles of the organic matter pool. Water 9, 335. doi:10.3390/w9050335
MacDonald, D. D., Ingersoll, C. G., and Berger, T. A. (2000). Development and evaluation of consensus-based sediment quality guidelines for freshwater ecosystems. Archives Environ. Contam. Toxicol. 39, 20–31. doi:10.1007/s002440010075
Mages, M., Óvári, M., Tümpling, v. W., and Kröpfl, K. (2004). Biofilms as bio-indicator for polluted waters?: Total reflection X-ray fluorescence analysis of biofilms of the Tisza river (Hungary). Anal. Bioanal. Chem. 378, 1095–1101. doi:10.1007/s00216-003-2291-5
Meyers, P. A. (1994). Preservation of elemental and isotopic source identification of sedimentary organic matter. Chem. Geol. 114, 289–302. doi:10.1016/0009-2541(94)90059-0
Miranda, L. S., Wijesiri, B., Ayoko, G. A., Egodawatta, P., and Goonetilleke, A. (2021). Water-sediment interactions and mobility of heavy metals in aquatic environments. Water Res. 202, 117386. doi:10.1016/j.watres.2021.117386
Morillo, J., Usero, J., and Gracia, I. (2002). Heavy metal fractionation in sediments from the tinto river (Spain). Int. J. Environ. Anal. Chem. 82, 245–257. doi:10.1080/03067310290009523
Nagy Szabó, A., Szabó, J., and Vass, I. (2018). An assessment of water and sediment quality of the Danube river: Polycyclic aromatic hydrocarbons and trace metals. Int. J. Innov. Sci. Res 12, 230–236. doi:10.5281/ZENODO.1316073
Onstad, G. D., Canfield, D. E., Quay, P. D., and Hedges, J. I. (2000). Sources of particulate organic matter in rivers from the continental USA: Lignin phenol and stable carbon isotope compositions. Geochimica Cosmochimica Acta 64, 3539–3546. doi:10.1016/S0016-7037(00)00451-8
Pan, J.-F., and Wen-Xiong, W. (2004). Differential uptake of dissolved and particulate organic carbon by the marine mussel Perna viridis. Limnol. Oceanogr. 49, 1980–1991. doi:10.4319/lo.2004.49.6.1980
Pocklington, R., and Tan, F. C. (1987). Seasonal and annual variations in the organic matter contributed by the St lawrence river to the gulf of St. Lawrence. Geochimica Cosmochimica Acta 51, 2579–2586. doi:10.1016/0016-7037(87)90308-5
Pourabadehei, M., and Mulligan, C. N. (2016). Effect of the resuspension technique on distribution of the heavy metals in sediment and suspended particulate matter. Chemosphere 153, 58–67. doi:10.1016/j.chemosphere.2016.03.026
Prieto, D. M., Devesa-Rey, R., Rubinos, D. A., Díaz-Fierros, F., and Barral, M. T. (2016). Biofilm Formation on river sediments under different light intensities and nutrient inputs: A flume mesocosm study. Environ. Eng. Sci. 33, 250–260. doi:10.1089/ees.2015.0427
R Core Team (2019). R: A Language and Environment for Statistical Computing. R Foundation for Statistical Computing. Available at: https://www.r-project.org/.
Relić, D., Đorđević, D., and Popović, A. (2011). Assessment of the pseudo total metal content in alluvial sediments from Danube River, Serbia. Environ. Earth Sci. 63, 1303–1317. doi:10.1007/s12665-010-0802-1
Roditi, H. A., Fisher, N. S., and Sañudo-Wilhelmy, S. A. (2000). Uptake of dissolved organic carbon and trace elements by zebra mussels. Nature 407, 78–80. doi:10.1038/35024069
Rügner, H., Schwientek, M., Milačič, R., Zuliani, T., Vidmar, J., Paunović, M., et al. (2019). Particle bound pollutants in rivers: Results from suspended sediment sampling in Globaqua River Basins. Sci. Total Environ. 647, 645–652. doi:10.1016/j.scitotenv.2018.08.027
Safar, Z., Chassagne, C., Rijnsburger, S., Sanz, M. I., Manning, A. J., Souza, A. J., et al. (2022). Characterization and classification of estuarine suspended particles based on their inorganic/organic matter composition. Front. Mar. Sci. 9, 896163. doi:10.3389/fmars.2022.896163
Sakan, S., Dević, G., Relić, D., Anđelković, I., Sakan, N., and Đorđević, D. (2015). Evaluation of sediment contamination with heavy metals: The importance of determining appropriate background content and suitable element for normalization. Environ. Geochem Health 37, 97–113. doi:10.1007/s10653-014-9633-4
Shu, C., Tan, G., Lv, Y., and Xu, Q. (2020). Field methods of a near-bed suspended sediment experiment in the Yangtze River, China. Arab. J. Geosci. 13, 1118. doi:10.1007/s12517-020-06124-w
Turner, A., and Millward, G. E. (2002). Suspended particles: Their role in estuarine biogeochemical cycles. Estuar. Coast. Shelf Sci. 55, 857–883. doi:10.1006/ecss.2002.1033
Tuttle-Raycraft, S., and Ackerman, J. D. (2018). Does size matter? Particle size vs. quality in bivalve suspension feeding. Freshw. Biol. 63, 1560–1568. doi:10.1111/fwb.13184
Tyson, R. V. (1995). Sedimentary organic matter. Dordrecht: Springer Netherlands. doi:10.1007/978-94-011-0739-6
Usman, Q. A., Muhammad, S., Ali, W., Yousaf, S., and Jadoon, I. A. K. (2021). Spatial distribution and provenance of heavy metal contamination in the sediments of the Indus River and its tributaries, North Pakistan: Evaluation of pollution and potential risks. Environ. Technol. Innovation 21, 101184. doi:10.1016/j.eti.2020.101184
van Hullebusch, E. D., Zandvoort, M. H., and Lens, P. N. L. (2003). Metal immobilisation by biofilms: Mechanisms and analytical tools. Re/Views Environ. Sci. Bio/Technology 2, 9–33. doi:10.1023/B:RESB.0000022995.48330.55
Varol, M., and Şen, B. (2012). Assessment of nutrient and heavy metal contamination in surface water and sediments of the upper Tigris River, Turkey. CATENA 92, 1–10. doi:10.1016/j.catena.2011.11.011
Vaughn, C. C., and Hakenkamp, C. C. (2001). The functional role of burrowing bivalves in freshwater ecosystems: Functional role of bivalves. Freshw. Biol. 46, 1431–1446. doi:10.1046/j.1365-2427.2001.00771.x
Vidmar, J., Zuliani, T., Novak, P., Drinčić, A., Ščančar, J., and Milačič, R. (2017). Elements in water, suspended particulate matter and sediments of the Sava River. J. Soils Sediments 17, 1917–1927. doi:10.1007/s11368-016-1512-4
Wang, H., Wang, J., Liu, R., Yu, W., and Shen, Z. (2015). Spatial variation, environmental risk and biological hazard assessment of heavy metals in surface sediments of the Yangtze River estuary. Mar. Pollut. Bull. 93, 250–258. doi:10.1016/j.marpolbul.2015.01.026
Wang, X., Ma, H., Li, R., Song, Z., and Wu, J. (2012). Seasonal fluxes and source variation of organic carbon transported by two major Chinese Rivers: The Yellow River and Changjiang (Yangtze) River: Flux and source of organic carbon. Glob. Biogeochem. Cycles 26, GB2025. doi:10.1029/2011GB004130
Woitke, P., Wellmitz, J., Helm, D., Kube, P., Lepom, P., and Litheraty, P. (2003). Analysis and assessment of heavy metal pollution in suspended solids and sediments of the river Danube. Chemosphere 51, 633–642. doi:10.1016/S0045-6535(03)00217-0
Yang, Y., Gao, B., Hao, H., Zhou, H., and Lu, J. (2017). Nitrogen and phosphorus in sediments in China: A national-scale assessment and review. Sci. Total Environ. 576, 840–849. doi:10.1016/j.scitotenv.2016.10.136
Keywords: riverine sediment, mussels, habitat, nutrients, metal contaminants
Citation: Dobosy P, Almeshal W, Illés Á, Tserendorj D, Sandil S, Kovács Z, Endrédi A and Záray G (2023) Particle-based nutrients and metal contaminants in the habitat of Unionidae mussels in the Tisza River (Hungary). Front. Environ. Sci. 11:1209118. doi: 10.3389/fenvs.2023.1209118
Received: 20 April 2023; Accepted: 06 June 2023;
Published: 15 June 2023.
Edited by:
Nsikak U. Benson, Université Claude Bernard Lyon 1, FranceReviewed by:
Hongbin Yin, Chinese Academy of Sciences (CAS), ChinaWei He, China University of Geosciences, China
Copyright © 2023 Dobosy, Almeshal, Illés, Tserendorj, Sandil, Kovács, Endrédi and Záray. This is an open-access article distributed under the terms of the Creative Commons Attribution License (CC BY). The use, distribution or reproduction in other forums is permitted, provided the original author(s) and the copyright owner(s) are credited and that the original publication in this journal is cited, in accordance with accepted academic practice. No use, distribution or reproduction is permitted which does not comply with these terms.
*Correspondence: Gyula Záray, emFyYXkuZ3l1bGFAZWNvbHJlcy5odQ==