- 1State Key Laboratory of Water Resources Engineering and Management, Wuhan University, Wuhan, China
- 2Yellow River Engineering Consulting Co., Ltd., Zhengzhou, China
Reservoir ecological operation has become an important means of ecological protection and restoration. The operation of reservoirs on sediment-laden rivers with water supply, sediment transport, flood control, wetland protection, and power generation as the primary objectives is a challenge in water resources management. Currently, most studies on reservoir ecological operation models involve a single ecological objective, and the inadequacy of the corresponding ecological constraint conditions makes it difficult to optimize reservoir ecological operation. To address these challenges, this study considers comprehensive water shortage, ecological water shortage, effective sediment transport in the river, and reservoir power generation as objective functions to establish an ecological operation model of sediment-laden river reservoirs for wetland protection. In this model, the ecological flow at key sections and water quantity discharged into the ocean are added as the constraint conditions to generate optimal operation schemes that reflect ecological benefits. The case study of the Xiaolangdi Reservoir (XLDR) on the Yellow River, China shows that with this model, the multi-objective requirements of water supply, ecology, sediment transport, flood control, and power generation in the XLDR could be met synergistically by optimizing the average daily discharge of the reservoir. Although the generated ecological operation schemes consider the demand for wetland protection, the reservoir flood control, sediment transport and power generation were not affected, and the latter two even showed improvement. In addition, this model has stronger applicability for large reservoirs. In dry years, small and medium reservoirs require water transfer, water-saving, and other measures required to alleviate water shortage.
1 Introduction
Reservoir construction is an important method of exploiting river water resources (Sawakuchi et al., 2021). The operation and storage functions of a reservoir offer great economic benefits to society (Maskey et al., 2022); however, they have an impact on river hydrology, hydrodynamics, and water ecology (Ngor et al., 2018; Maavara et al., 2020), especially in causing unnatural flow patterns (Grill et al., 2019). For maintaining the ecological health of rivers and adapting river flow processes subjected to the influence of reservoirs, the concept of ecological operation has been proposed (Zhou and Guo, 2013; Valeria and Alberto, 2015). The core of ecological operation is to meet both the social and economic water requirements and maintain the ecological health of the river. Ecological protection and restoration have gradually become some of the important objectives of reservoir operation (Suwal et al., 2020), and this concept has enabled the field of water-resource management to develop immensely (Wang et al., 2015; Ai et al., 2022). To date, the primary scientific problem faced in the ecological operation of reservoirs is the optimization of the operation to reconstruct the ecological flow and hydrodynamic processes of the river (Acreman et al., 2014; Poff, 2018); that is, to create hydrological regime and hydrodynamic conditions favorable to ecological protection and water environment improvement downstream of the reservoir and to provide water flow process for river-beach forest and grass irrigation, wetland ecology, and fish habitat using pulsed discharge from the reservoir (Huang et al., 2018; Deng et al., 2020; Katrien et al., 2020).
Currently, the ecological protection role of reservoir has received considerable attention (Baumgartner et al., 2020; Deng et al., 2020). Through the ecological operation of reservoirs, an artificial flow process is created to improve the ecological environment quality downstream, which has been studied with respect to the operations of many reservoirs (Gillespie et al., 2015). Sale et al. (1982) investigated the relationship between biological flow demand and river water intake using mathematical methods, and the concept of ecological operation was explored. Ecological operation concepts have been implemented in the Glen Canyon Dam and Colorado River Reservoir in the United States, and the combined objectives of river sediment transport, habitat restoration, and fish protection were attained (Chen et al., 2019; Dobson et al., 2019). Babel et al. (2005) developed a reservoir operation optimization model to maximize the total economic benefits, and this model met the water requirements of different departments including living, production, and ecology to the greatest extent and guaranteed the ecological water demand downstream. Shiau and Wu (2007) studied the ecological flow rates of the river over different years and used an optimization algorithm to determine the balance between economy and ecology. Several scholars have considered the calculated minimum ecological flow rate of a river as the constraint condition in reservoir (group) operation optimization models to realize their ecological benefits. In some studies, the power generation in hydropower stations under different ecological flow conditions were investigated to optimize the trade-off between ecology and power generation objectives (Castellitti et al., 2008; Hu et al., 2008; Steinschneider et al., 2014; Nyatsanza et al., 2015).
In 2017, a reservoir operation model that coupled the structural differences in the river runoff regime was proposed, and a runoff mode was designed to ensure the ecological integrity of the Mekong River and socio-economic demand in this model (Sabo et al., 2017). Since 1999, operations on the Yellow River in China have been unified. Ecological operation based on water and sediment in the lower reaches of the Yellow River was carried out, and this practice improved the biodiversity of the Yellow River Delta (Li, 2006). Yan et al. (2021) constructed a reservoir operation model with two defined ecological objectives of water quantity level and hydrological alteration with the economic objective of hydropower production. Li et al. (2022) constructed an operation model of the Three Gorges and Gezhouba reservoir groups, and the ecological demand of representative fishes in the Yangtze River was involved in this model. Based on a refined reservoir discharge strategy, appropriate conditions for fish spawning were created. The realization of a win-win situation for economic development and ecological protection by incorporating these into reservoir operations is at the cutting edge of research (Margaret and Albert, 2019), but in general, the ecological operations of reservoirs have been less studied (Poff and Schmidt, 2016; You et al., 2021).
A number of studies have confirmed the feasibility of ecological operation, but most of the current ecological operations have the characteristic of “high-flow-rate discharge in the flood period” (Lan et al., 2022). With the increase in ecological protection awareness, the fulfillment of ecological demand at a high level has become an important goal (Niu et al., 2021), and ecological operation has expanded from the guarantee of ecological base flow of a single section to considering the ecological water demand of the area downstream (Tsai et al., 2015; Zeng et al., 2022). Because different rivers have different hydrological, water-sediment, and hydro-ecological characteristics that are affected by the operation and storage capacity of the reservoir itself, an obvious antagonistic effect exists between the benefit and ecological objectives. Optimized, refined, dynamic, and operable ecological operations have become a challenge. Meanwhile, studies on ecological operation in China focus on the Yangtze River (Huang et al., 2019; Jiang et al., 2019), and few studies take the Yellow River as the object. It is noteworthy that the Yellow River is a typical sediment-laden river, and its problems such as water shortage, uncoordinated water-sediment relationship, and fragile ecological background increase the difficulty of ecological operations. Therefore, according to the characteristics of sediment-laden rivers, a reservoir ecological operation model for the wetland protection was established in this paper, and a case study related to the Xiaolangdi Reservoir (XLDR) of the Yellow River (This reservoir is a key project to control the water supply downstream, to regulate the water-sediment relationship, and to provide ecological water replenishment for the wetland of the Yellow River Delta.) was carried out.
2 Reservoir ecological operation modelling
The key objective of reservoir operation is to maximize comprehensive regional benefits by shaping the flow process in the reservoir area downstream. Sediment transport is an important goal for sediment-laden rivers. The reservoir operation model in this paper not only involves water supply, flood control, power generation and other objectives, but also considers the ecological protection and sediment-transport objectives. It is an operation optimization model that integrates multiple objectives. The average daily process was taken as the simulation scale of the model, and the diversion gate dam project involved in this model was generalized according to the diversion site.
2.1 Model objective functions
(1) Minimum comprehensive water shortage. Water supply efficiency is improved during the operation period to reduce water shortage and provide stable water resource guarantee for the domestic and production water demand of the water service area.
where θ(t) is the importance coefficient of water shortage on day t; Qd(t) and Qs(t) is the water demand and water supply on day t, m³, respectively;
(2) Minimum ecological water shortage. To ensure the water demand for maintaining the basic functions of the river channel downstream, simulate a reservoir discharge mode close to the natural hydrological situation, enhance the connectivity and operation of water system, meet the ecological water requirement of river channel, and maintain and improve the health of the river.
where W(t) and Weco(t) stand for the ecological water discharged from the reservoir and regional ecological water demand on day t, respectively, m³. W(t) is the decision variable.
(3) Maximum effective sediment transport of the river. To improve the water and sediment transport capacity of the river channel and maintain the stability of the river channel, sediment can be transported into the ocean as much as possible, and the quantity of sediments deposited in the reservoir and river channel can be reduced.
where Qout(t) is the flow rate of water discharged from the reservoir on day t, m³/s; Qout(t) ≥ [Qs(t) + W(t)]. St is the critical sediment content, and St = vQout(t)m, kg/m³; v and m are the parameters; St, as one of the key parameters to calculate the sediment transport for sediment-laden river, is expressed as the sediment content with the highest sediment transport efficiency and lowest sediment deposition risk in the reservoir operation process; and meanwhile, the sediment content in reservoir outflow cannot be higher than St to prevent sediment deposition and economize sediment-carrying water. Qout(t) is the decision variable.
(4) Maximum reservoir power generation. Maximum electricity is generated during the reservoir operation period to obtain the benefits of the reservoir.
where Z(t) stands for the water level in front of the dam on day t, m; Z(0) stands for the installation elevation of the reservoir motor floor, m; K is the output coefficient of generator unit; aep is the on-grid electricity price of reservoir power generation, U.S. dollar/kW·h. Z(t) is the decision variable.
2.2 Model constraint conditions
(1) Reservoir water balance constraint
(2) Reservoir capacity constraint
(3) Reservoir discharge constraint
(4) Ecological flow constraints at key sections
(5) Constraint of water quantity discharged into the ocean
Considering that certain rivers were directly connected to the ocean, a certain quantity of water is required to maintain the offshore ecosystem, and the quantity was calculated as follows:
(6) Reservoir power output constraint
(7) Non-negative constraints: all the variables are non-negative values
Where V(t+1) and V(t) represent the storage capacity of the water reservoir on day t+1 and day t, respectively, m³; Qin(t), qin(t), Qout(t) and Qd(t) represent the inflow rate on day t, inter-section catchment flow rate, reservoir discharge flow rate, and reservoir diversion flow rate (including reservoir water loss), m³/s. Vmin and Vmax are the lower and upper limits of reservoir capacity, m³. Qmin and Qmax stand for the lower and upper limits of flow rate discharged from the reservoir, respectively, m³/s. Qc(t) is the flow rate at the section on day t, and EF(t) is the ecological flow rate required in the section on day t, m³/s. IS the flow rate discharged into the ocean, m³, and IS(min) is the minimum value of IS. Nmin and Nmax represent the lower and upper limits of output of the reservoir generator units on day t, kW.2.3 Model solution
The reservoir ecological operation model proposed in the paper has four objective functions, the calculation process of multi-objective reservoir operation model has the characteristics of high dimension and multi-stage dynamics, which will be restricted and affected by different stakeholders (Zhao and Cai, 2020). The Non-dominated Sorting Genetic Algorithm-Ⅱ (NSGA-Ⅱ) is one method to solve optimization problems. However, the optimization efficiency and convergence speed of the NSGA-Ⅱ algorithm would be reduced by the model with three or more objective functions (Liu et al., 2021). The existing study has found that an integrated algorithm that includes the normal boundary crossing method, primal-dual interior-point method, and NSGA-Ⅱ can be used for lake or reservoir optimization, which is an effective way to reduce calculation time and enhance global convergence (Liu et al., 2021). Four objective functions of the proposed model can, in this study, be converted into two coupled objective functions using the normal boundary crossing method and the primal-dual interior-point method. Subsequently, the NSGA-Ⅱ is employed to solve the model with two objective functions and using the fuzzy satisfaction evaluation to filter the optimal solution in the end (Calculation process can be seen in Figure 1).
3 Case study
3.1 Study area
The XLDR is a large comprehensive water control project located at the outlet of the last gorge in the middle reaches of the Yellow River. The area of the basin upstream from the dam site is 694,200 km2, accounting for 92.2% of the total basin (excluding the noncontributing area). The annual average runoff was measured to be 37.75 billion m³, and the average annual sediment transport is 1.265 billion tons. Approximately 92.3% of the total area of the Yellow River basin, 87% of the natural runoff of the Yellow River, and 100% of sediment transport are controlled by the reservoir. The primary objective of the XLDR was flood (including ice) prevention and siltation reduction, with subsidiary objectives of water supply, irrigation, power generation, storing clear water and releasing muddy water, elimination of hazards and promotion of benefits, and comprehensive resource utilization (Shang et al., 2022). The normal impounded level, normal dead storage level, flood control level, and abnormal dead storage level of the reservoir are 275, 230, 254, and 220 m, respectively. Currently, the XLDR provides water for the wetland in the Yellow River Delta through large-scale discharge during the flood season.
3.2 Model input data
The inflow of the XLDR from 2000 to 2020 was arranged according to the frequency of runoff values, and the inflow runoff in the wet year (2020), normal year (2007) and dry year (2016) was selected in the model. The local inflow in the model included the Yiluo River and Qinhe River, and the local inflow was arranged according to the frequency of characteristics in the wet, normal, and dry seasons; The quantities of water required for regional living, production, and urban ecology were calculated according to the quantities of water consumed (excluding water withdrawal from the river). The monthly average water loss due to evaporation and leakage in the river was estimated to be 25 m³/s in the wet and normal years and 20 m³/s in the dry year. The generalized calculation units in this model are shown in Figure 2.
The wetland of the Yellow River Delta in the lower reaches of the XLDR is an important stopover, wintering, and breeding site for migratory birds around the Western Pacific and East Asia-Australia. This delta is the largest breeding place for the oriental white stork, which is the flagship species of wetland ecosystem, the second largest breeding place for the endangered species of black-headed gull, and the largest migration and stopover place for the eastern population of curly pelican, which is a first-class protected animal in China. Wetland, rare and protected birds and their habitats, and estuarine and offshore aquatic organisms and their habitats are the main ecological protection objects in this region. According to the studies undertaken by the Yellow River Engineering Consulting Co., Ltd., the ecological water demand for maintaining the stability of the ecosystem of the Yellow River Delta under different inflow conditions is listed in Table 1; Water is required from March to September; the period of April to June is critical for fish spawning in the Yellow River estuary, and the minimum amount of water discharged into the ocean from April to June in a year reaches 30 × 108 m³. Moreover, based on the existing study from the Yellow River Engineering Consulting Co., Ltd., the critical sediment concentration of the XLDR with the best sediment transport performance is as follows: St = 0.00294Qout0.804, where Qout is the amount of water discharged from the XLDR. According to the Ministry of Water Resources of China, the minimum ecological flow rate at the Lijin section is 50 m³/s. In addition, based on the relevant research results (Xia et al., 2021), the installation elevation Z(0) of the generator floor of XLDR is 129 m, and the output coefficient K of the generator units is 8.5. The on-grid electricity price a of the XLDR is 0.04562 U.S. dollar/kW·h.
3.3 Results
Due to space constraints, this paper directly presents the optimal ecological operation scheme under different reservoir inflow conditions. During a wet year (2020), the monthly average flow rate discharged from the reservoir reached a maximum of 2927.58 m³/s in July and a minimum of 214.13 m³/s in January. The monthly average flow rates discharged from March to September exceeded 1,000 m³/s, and those in July to August exceeded 2000 m³/s (see Table 2). The abundant water resource was regulated and stored by the reservoir, so the requirements of flood control and sediment transport in the flood period were met, and the flood could effectively support the ecological replenishment of the wetland in the Yellow River Delta. The utilization rate of flood was improved, and the higher requirements of regional ecological protection and restoration were met.
In a normal year (2007), the monthly average flow rate discharged from the reservoir reached a maximum of 1,543.08 m³/s in June and a minimum of 211.91 m³/s in January. The monthly average flow rates discharged in March to July 1,212.01, 1,178.99, 1,199.99, 1,543.08, and 1,344.11 m³/s, exceeded 1,000 m³/s (see Table 3). Through the optimization of operation, the requirements of day-to-day living, industry, agriculture, ecological water supply, ecological flow of Lijin section, and coastal ecological water supply in the reservoir area downstream could be met. Considering the smaller water inflow in the normal year compared to the wet year, the monthly average flow rates discharged in August to September were less than 1,000 m³/s. The storage rate of the reservoir was improved to guarantee water supply in non-flood seasons.
In another dry year (2016), the monthly average flow rate discharged from the reservoir reached a maximum of 1,151.86 m³/s in March and a minimum of 131.61 m³/s in January. The monthly average flow rates discharged in March and June 1,151.86 and 1,021.71 m³/s, exceeded 1,000 m³/s (see Table 4). This is the river-channel high-flow-rate pulse shaped by the water demand process. Due to the small inflow quantity in the dry year, the reservoir discharge was conservative. Therefore, the monthly average flow rates discharged from the reservoir in January to February, August to October, and November to December were less than 500 m³/s, and the water discharged aimed to meet the requirements of living and industrial water in the reservoir area downstream; the flow rates exceeded 500 m³/s in March to May to meet the requirement of agricultural irrigation in the reservoir area downstream.
The flow rates of the Lijin section reached or exceeded 50 m³/s under different inflow conditions, and the quantity of water discharged into the ocean from April to June of the year reached or exceeded 30 × 108 m³. But the flow rates in the Lijin section in December and January in the dry year were 54.78 and 50.3 m³/s, respectively, approaching the minimum-flow-rate requirement of the section. Therefore, operation in dry years is still an important concern. The optimized discharge processes of the reservoir in wet, normal, and dry years are shown in Figure 3. The maximum and minimum daily average flow rates discharged from the XLDR in the flood season of the wet year were 3843.25 and 315.51 m³/s. In the non-flood period, the maximum and minimum values were 3106.18 and 336.6 m³/s. The flood season in the middle and lower reaches of the Yellow River was distributed in July to August, and the peak of daily average flow rate took place on July 28. During the periods from June 29 to July 6 and from July 24 to August 1, the daily average flow rates discharged exceeded 3,000 m³/s. The maximum and minimum daily average flow rates discharged from the XLDR in the flood season of the normal year were 3,124.6 and 331.5 m³/s, respectively. In the non-flood period, the maximum and minimum values were 3,001.1 and 2,29.8 m³/s; the peak of daily average flow rate occurred on July 3. During the period from June 30 to July 5, the daily average flow rates discharged exceeded 3,000 m³/s. In the flood period of the dry year, the maximum and minimum daily average flow rates discharged were 2,862.84 and 126.59 m³/. In the non-flood period, the maximum and minimum values were 1,499.58 and 136.32 m³/s. The maximum was observed on July 7. During the period from July 5 to 9, the daily average flow rates discharged exceeded 2,000 m³/s to shape a limited high-flow-rate pulse process in the case of small quantity of inflow, to maintain the stability of the regional ecosystem. Irrigation, breeding of aquatic organisms in the river, and ecological water replenishment in the wetland are urgent in the lower reaches of the Yellow River in the period from March to May. By optimizing the reservoir operation process, the daily annual pulsed flow rate exceeding 1,000 m³/s could be successively shaped from March to May, regardless of the wet, normal, and dry years. This effect obviously mitigated the contradiction between irrigation and ecological water demand.
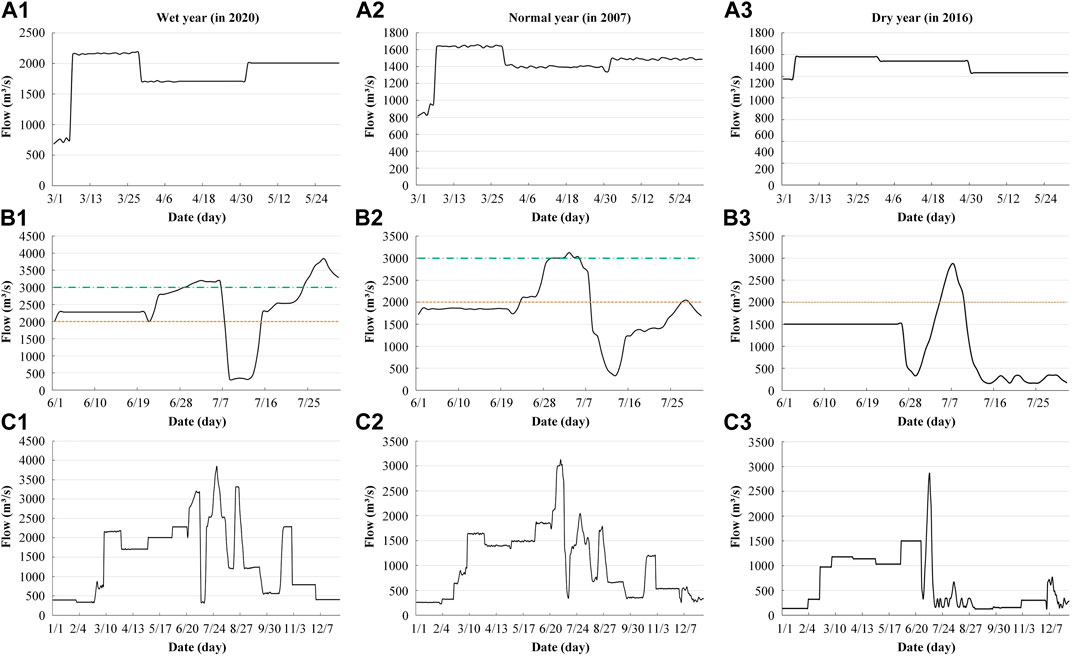
FIGURE 3. Reservoir outflow process in 2020, 2007 and 2016, respectively (a. March 1st ∼ May 31st; b. June 1st ∼ July 31st; c. Entire year).
4 Discussion
The optimized results from the model were compared with the actual operation processes of the reservoir. In 2020, 170 million tons of annual sediment transport and power generation of 9.067 billion kW·h with economic benefit of 0.399 billion U.S. dollar could be realized by the XLDR as per the model. In contrast, the actual annual sediment transport, power generation, and economic benefit in 2020 were 141 million tons, 9.011 billion kW·h, and 0.396 billion U.S. dollar, respectively. The power generation and economic benefits increased by 56 million kW·h and 300 thousand U.S. dollar. In 2007, 143 million tons of annual sediment transport and power generation of 6.707 billion kW·h with economic benefit of 0.295 billion U.S. dollar could be obtained as per the model. In contrast, the actual annual sediment transport, power generation, and economic benefit in 2007 were 141 million tons, 6.633 billion kW·h, and 0.291 billion U.S. dollar. The power generation and benefit were increased by 74 million kW·h and 400 thousand U.S. dollar. In 2016, the annual sediment transport, power generation, and benefit reached 26 million tons, 4.316 billion kW·h, and 0.190 billion U.S. dollar in the model. However, the actual values in 2016 were 0 ton, 4.133 billion kW·h, and 0.182 billion U.S. dollar. Power generation and benefit were increased by 183 million kW·h and 800 thousand U.S. dollar. Although the ecological operation scheme considered the objectives of ecological protection and restoration, reservoir flood control, sediment transport and power generation were not affected, and the sediment transport and power generation benefit were even improved, proving that the proposed model is reasonable and reliable.
According to the study, flood control is a key issue for the reservoir in the flood season. The water level should be decreased before the flood season, and the problem that a large quantity of water was discharged in a short time in the actual operation scheme could be addressed via the optimization of the model. Therefore, the discharge process of the reservoir in the flood season could be more stable. The damage to the river channel due to the high peak flow rate could be prevented, and the utilization rate of flood resources in the flood season could be improved. Especially, the ecological recovery and environmental improvement in the reservoir area downstream could be promoted with the abundant water resources in the wet year after the optimization of operation and storage of the reservoir. The inflow in the normal year was stable, and the normal operation met the requirements of reservoir operation. To improve the efficiency of power generation and sediment transport, the optimization scheme can be adopted. In the dry year, the shortage of inflow water had a negative impact on the supply and demand of water resources and ecological protection in the lower reaches of the Yellow River. In particular, the Yellow River began to run dry in the lower reaches in the 1970s, and this situation became serious after the 1990s. Especially in 1997, the Yellow River ran dry in the lower reaches for a total of 226 days, resulting in the shortage of domestic water, degradation of wetland in the Yellow River estuary delta, loss of biodiversity, and deterioration of ecological situation. The XLDR has become the last barrier to ensure the continuous flow in the lower reaches of the Yellow River. As per our results, the flood peak flow in the flood season of the dry year that had not been well utilized in the actual operation process was optimized, and the demand for irrigation, living and ecological water supply in the lower reaches of the Yellow River in the dry year were realized. Meanwhile, the quantity of sediment deposited in the reservoir was reduced, and the service life of the reservoir was increased in the optimized scheme. Nevertheless, the water shortage in the Yellow River is still an important issue that can be addressed through consistent water saving and inter-basin water transfer in the future.
The reservoir operation of sediment-laden river is complex, and the reservoir discharge not only aims to water supply and power generation, but also focuses on the flushing, carrying and transport of sand through the flow process. Meanwhile, as an effective ecological protection and restoration measure, reservoir operation of sediment-laden river becomes more complex with those ecological objectives involved (Huang et al., 2020). In this paper, a reserve economic operation model was built, and the case study of the XLDR revealed that the optimization and constructive collaboration of multiple objectives such as water supply, flood control, sediment transport, ecology, and power generation with interactions can be realized by the good operation and storage capacity of a large reservoir. The results showed that the model was applicable to large reservoirs. For the medium or small reservoirs, the limited storage capacity will be a major constraint during operation. In detail, the deposition of sediment will affect the storage capacity and the operation effect of the reservoir will be limited during the multi-objective optimization process, resulting in the situation that only a proportion of objectives are optimized while the benefits of remaining objective are reduced. This situation was beyond the scope of this paper. In the future, the applicability of the proposed model to medium and small reservoirs in sediment-laden rivers will be estimated, and the possibility of model evolution will be evaluated to provide improved techniques for the multi-objective optimization and operation of reservoirs in sediment-laden rivers.
5 Conclusion
Aiming at the core topic of multi-objective optimization and operation of reservoir of sediment-laden river with the consideration of ecological demand, the following conclusions are drawn.
(1) The most evident feature of sediment-laden river is the sediment content in the water, and its reservoir operation is very complex. With the consideration of ecological protection objectives, the multi-scale, multi-objective, and multi-level degrees of reservoir operation have become larger. For the multi-objective requirements of reservoir water supply, ecology, flood control, sediment transport and power generation, the minimum comprehensive water shortage, minimum ecological water shortage, maximum effective sediment transport of river, and maximum reservoir power generation were taken as the objective functions to establish an ecological operation model of reservoir of sediment-laden river for the wetland protection.
(2) The results related to the XLDR showed that a pulsed flow process with daily average flow rate continuously exceeding 1,000 m³/s was shaped in this model to meet the requirements of the river channel in the lower reaches of the Yellow River by correcting the sudden and violent discharge process of the reservoir, enhancing the operation and storage of flood peak water in the flood season, and weakening the contradiction between irrigation and wetland ecological water replenishment, ensuring the water supply for living, agriculture, industry and ecology under different inflow conditions of the reservoir, improving the efficiency of power generation and sediment transport of the reservoir, and controlling the flood in flood seasons.
(3) The operations of reservoirs in years with a small amount of inflow water should be studied in detail. For large reservoirs, the domestic water consumption and production should be of priority, and the water required should be appropriately allocated to ensure the stability of the river ecosystem and to maintain the biodiversity of wetland. For the medium and small reservoirs, the operations may jeopardize the agricultural and ecological water demand to a certain extent, and the water shortage problem can be addressed through water transfer and water saving.
(4) Reservoir ecological operation model is reasonable and reliable and can provide a reference for the study of reservoir operation with multiple objectives such as water supply, sediment transport, ecological conservation, flood prevention and power generation in China and other countries.
Data availability statement
The original contributions presented in the study are included in the article/Supplementary Material, further inquiries can be directed to the corresponding author.
Author contributions
Conceptualization, YY and BL, Methodology, YY and BL, Resources, BL, Writing-Original draft preparation, YY, Validation, YY and BL, Writing-Reviewing and Editing, YY and BL, Funding acquisition, BL. All authors have read and agreed to the published version of the manuscript. All authors listed have made a substantial, direct, and intellectual contribution to the work and approved it for publication. All authors contributed to the article and approved the submitted version.
Funding
This work was supported by the National Key R&D Program of China (2022YFC3202405-04), and the Development Funding Project by Water Youth Talent from Beijing Jianghe Water Development Foundation.
Acknowledgments
The research would not have been possible without the interest and cooperation of Yellow River Conservancy Commission of the Ministry of Water Resources.
Conflict of interest
Author BL was employed by the company Yellow River Engineering Consulting Co., Ltd.
The remaining author declares that the research was conducted in the absence of any commercial or financial relationships that could be construed as a potential conflict of interest.
Publisher’s note
All claims expressed in this article are solely those of the authors and do not necessarily represent those of their affiliated organizations, or those of the publisher, the editors and the reviewers. Any product that may be evaluated in this article, or claim that may be made by its manufacturer, is not guaranteed or endorsed by the publisher.
References
Acreman, M., Arthington, A. H., Colloff, M. J., Couch, C., Crossman, N. D., Dyer, F., et al. (2014). Environmental flows for natural, hybrid, and novel riverine ecosystems in a changing world. Front. Ecol. Environ. 12 (8), 466–473. doi:10.1890/130134
Ai, Y., Ma, Z., Xie, X., Huang, T., and Cheng, H. (2022). Optimization of ecological reservoir operation rules for a northern river in China: Balancing ecological and socio-economic water use. Ecol. Indic. 138, 108822. doi:10.1016/j.ecolind.2022.108822
Babel, M. S., Gupta, A. D., and Nayak, D. K. (2005). A model for optimal allocation of water to competing demands. Water Resour. Manag. 19 (6), 693–712. doi:10.1007/s11269-005-3282-4
Baumgartner, M. T., Pitágoras, A. P., Baumgartner, G., and Gomes, L. C. (2020). Storage or run-of-river reservoirs: Exploring the ecological effects of dam operation on stability and species interactions of fish assemblages. Environ. Manage. 65 (2), 220–231. doi:10.1007/s00267-019-01243-x
Castellitti, A., Pianosi, F., and Palmer, R. (2008). Water reservoir control under economic, social and environmental constraints. Automatic 44 (6), 1595–1607. doi:10.1016/j.automatica.2008.03.003
Chen, Z., Huang, P., and Zhang, Z. (2019). Interaction between carbon dioxide emissions and eutrophication in a drinking water reservoir: A three-dimensional ecological modeling approach. Sci. Total. Environ. 663 (1), 369–379. doi:10.1016/j.scitotenv.2019.01.336
Deng, M. J., Huang, Q., Chang, J. X., and Huang, S. Z. (2020). Large-scale ecological operation research and practice. J. Hydraul. Eng. 51 (7), 757–773. (in Chinese). doi:10.13243/j.cnki.slxb.20200326
Dobson, B., Wagener, T., and Pianosi, F. (2019). An argument-driven classification and comparison of reservoir operation optimization methods. Adv. Water. Resour. 128 (6), 74–86. doi:10.1016/j.advwatres.2019.04.012
Gillespie, B. R., Desmet, S., Kay, P., Tillotson, M. R., and Brown, L. E. (2015). A critical analysis of regulated river ecosystem responses to managed environmental flows from reservoirs. Freshw. Biol. 60 (2), 410–425. doi:10.1111/fwb.12506
Grill, G., Lehner, B., Thieme, M., Geenen, B., Tickner, D., Antonelli, F., et al. (2019). Author Correction: Mapping the world’s free-flowing rivers. Nature 569 (7755), E9–E221. doi:10.1038/s41586-019-1379-9
Hu, H. P., Liu, D. F., Tian, F. Q., and Ni, G. H. (2008). A method of ecological reservoir reoperation based-on ecological flow regime. Adv. Sci. 19 (3), 325–332. (in Chinese).
Huang, X. D., Wang, L., Han, P. P., and Wang, W. C. (2018). Spatial and temporal patterns in nonstationary flood frequency across a forest watershed: Linkage with rainfall and land use types. Forests 9 (6), 339. doi:10.3390/f9060339
Huang, L., Li, X., Fang, H. W., Yin, D., Si, Y., Wei, J., et al. (2019). Balancing social, economic and ecological benefits of reservoir operation during the flood season: A case study of the three gorges project, China. J. Hydrol. 572, 422–434. doi:10.1016/j.jhydrol.2019.03.009
Huang, X. D., Wang, D., Han, P. P., Wang, W. C., Li, Q. J., Zhang, X. L., et al. (2020). Spatial patterns in baseflow mean response time across a watershed in the loess plateau: Linkage with land-use types. For. Sci. 66 (3), 382–391. doi:10.1093/forsci/fxz084
Jiang, Z., Liu, P., Ji, C., Zhang, H., and Chen, Y. (2019). Ecological flow considered multi-objective storage energy operation chart optimization of large-scale mixed reservoirs. J. Hydrol. 577, 123949. doi:10.1016/j.jhydrol.2019.123949
Katrien, V. B., Patrick, M., Tim, S., Bram, D., Dries, B., Thomas, V., et al. (2020). Aligning biodiversity conservation and ecosystem ser-vices in spatial planning: Focus on ecosystem processes. Sci. Total. Environ. 712, 136350. doi:10.1016/j.scitotenv.2019.136350
Lan, L., Xu, Y. S., Mei, Y. D., Ren, Y. F., Tian, Y. F., and Zhang, X. (2022). Optimized flood control for the three gorges reservoir considering evolving flood propagation trends in the jingjiang reach of the Yangtze River. Front. Env. Sci-Switz. 10, 1013583. doi:10.3389/fenvs.2022.1013583
Li, Y. K., Lin, J. Q., Liu, Y., Yao, W., Zhang, D., Peng, Q., et al. (2022). Refined operation of cascade reservoirs considering fish ecological demand. J. Hydrol. 607, 127559. doi:10.1016/j.jhydrol.2022.127559
Li, G. Y. (2006). Regulation of water and sediment for the Yellow River based on joint operation of reservoirs and artificial intervention. J. Hydraul. Eng. 37 (12), 1439–1446. (in Chinese). doi:10.1061/(ASCE)0887-381X(2006)20:1(20)
Liu, B. J., Wang, Y., Xia, J., Quan, J., and Wang, J. (2021). Optimal water resources operation for rivers-connected lake under uncertainty. J. Hydrol. 595, 125863. doi:10.1016/j.jhydrol.2020.125863
Maavara, T., Chen, Q., Meter, K. V., Brown, L. E., Zarfl, C., Ni, J., et al. (2020). River dam impacts on biogeochemical cycling. Nat. Rev. Earth. Environ. 1, 103–116. doi:10.1038/s43017-019-0019-0
Margaret, P., and Albert, R. (2019). Linkages between flow regime, biota, and ecosystem processes: Implications for river restoration. Science 365 (6459), eaaw2087. doi:10.1126/science.aaw2087
Maskey, M. L., Dourado, G. F., Rallings, A. M., Rheinheimer, D. E., Medellin-Azuara, J., and Viers, J. H. (2022). Assessing hydrological alteration caused by climate change and reservoir operations in the San Joaquin River Basin, California. Front. Env. Sci-Switz. 10, 765426. doi:10.3389/fenvs.2022.765426
Ngor, P. B., Legendre, P., Oberdorff, T., and Lek, S. (2018). Flow alterations by dams shaped fish assemblage dynamics in the complex Me-kong-3S river system. Ecol. Indic. 88, 103–114. doi:10.1016/j.ecolind.2018.01.023
Niu, W. J., Feng, Z. K., Jiang, Z. Q., Wang, S., Liu, S., Guo, W., et al. (2021). Enhanced harmony search algorithm for sustainable ecological operation of cascade hydropower reservoirs in river ecosystem. Environ. Res. Lett. 16 (5), 055013. doi:10.1088/1748-9326/abf60c
Nyatsanza, F. F., Graas, S., and Zaag, P. (2015). The impact of dynamic environmental flow releases on hydropower production in the zambezi River basin. J. Am. Water. Resour. As. 51 (4), 1029–1042. doi:10.1111/jawr.12280
Poff, N. L., and Schmidt, J. C. (2016). How dams can go with the flow. Science 353 (6304), 1099–1100. doi:10.1126/science.aah4926
Poff, L. R. (2018). Beyond the natural flow regime? Broadening the hydro-ecological foundation to meet environmental flows challenges in a non-stationary world. Freshw. Biol. 63 (8), 1011–1021. doi:10.1111/fwb.13038
Sabo, J. L., Ruhi, A., Holtgrieve, G. W., Elliott, V., Arias, M. E., Ngor, P. B., et al. (2017). Designing river flows to improve food security futures in the lower Mekong Basin. Science 358 (6368), eaao1053. eaao1053. doi:10.1126/science.aao1053
Sale, M. J., Brill, E. D., and Herricks, E. E. (1982). An approach to optimizing reservoir operation for downstream aquatic resources. Water Resour. Res. 18 (4), 705–712. doi:10.1029/wr018i004p00705
Sawakuchi, H. O., Bastviken, D., Enrich-Prast, A., Ward, N. D., Camargo, P. B., and Richey, J. E. (2021). Low diffusive methane emissions from the main channel of a large Amazonian run-of-the-river reservoir attributed to high methane oxidation. Front. Env. Sci-Switz. 9, 655455. doi:10.3389/fenvs.2021.655455
Shang, W. X., Yan, D. M., Peng, S. M., Wang, Y., Ge, L., and Shang, Y. (2022). Analysis on the ecological impact of the Xiaolangdi reservoir on the Yellow River Delta wetland and coastal areas. Front. Env. Sci-Switz. 10, 953318. doi:10.3389/feart.2022.953318
Shiau, J., and Wu, F. (2007). Pareto-optimal solutions for environmental flow schemes incorporating the intra-annual and interannual variability of the natural flow regime. Water Resour. Res. 43 (6), 1–12. doi:10.1029/2006WR005523
Steinschneider, S., Bernstein, A., Palmer, R., and Polebitski, A. (2014). Reservoir management optimization for basin-wide ecological restoration in the Connecticut River. J. Water Res. Plan. Man. 140 (9), 04014023. doi:10.1061/(ASCE)WR.1943-5452.0000399
Suwal, N., Huang, X., Kuriqi, A., Chen, Y., Pandey, K. P., and Bhattarai, K. P. (2020). Optimisation of cascade reservoir operation considering environmental flows for different environmental management classes. Renew. Energ. 158, 453–464. doi:10.1016/j.renene.2020.05.161
Tsai, W. P., Chang, F. F., Chang, L. C., and Herricks, E. E. (2015). AI techniques for optimizing multi-objective reservoir operation upon human and riverine ecosystem demands. J. Hydrol. 530, 634–644. doi:10.1016/j.jhydrol.2015.10.024
Valeria, R., and Alberto, D. L. F. (2015). Assessing the link between environmental flow, hydropeaking operation and water quality of reservoirs. Ecol. Eng. 85, 26–38. doi:10.1016/j.ecoleng.2015.09.074
Wang, H., Brill, E. D., Ranjithan, R. S., and Sankarasubramanian, A. (2015). A framework for incorporating ecological releases in single reservoir operation. Adv. Water Resour. 78 (4), 9–21. doi:10.1016/j.advwatres.2015.01.006
Xia, J. Q., Chen, Y. J., Deng, S. S., Zhou, M. R., and Wang, Z. H. (2021). Coupled modeling of flow? Sediment transport and power generation in the Xiaolangdi reservoir. Adv. Eng. Sci. 53 (6), 113–121. (in Chinese). doi:10.15961/j.jsuese.202001085
Yan, M., Fang, G. H., Dai, L. H., Tan, Q. F., and Huang, X. F. (2021). Optimizing reservoir operation considering downstream ecological de-mands of water quantity and fluctuation based on IHA parameters. J. Hydrol. 600, 126647. doi:10.1016/j.jhydrol.2021.126647
You, J. J., Xue, Z. C., Lin, P. F., Jiang, Y. Z., and Wei, N. (2021). Study on the integrated river basin ecological operation based on Two-layer structure I: Methodology and Model. J. Hydraul. Eng. 52 (12), 1449–1457. (in Chinese). doi:10.13243/j.cnki.slxb.20210259
Zeng, C. F., Qi, W. Y., Mao, Y. Q., Liu, R., Yu, B. Y., and Dong, X. N. (2022). Water conservation ecological service function and its value response mechanism in a nested water conservancy project area. Front. Env. Sci-Switz. 10, 887040. doi:10.3389/fenvs.2022.887040
Zhao, Q. K., and Cai, X. M. (2020). Deriving representative reservoir operation rules using a hidden Markov-decision tree model. Adv. Water Resour. 146, 103753. doi:10.1016/j.advwatres.2020.103753
Keywords: ecological operation, reservoir, optimal operation scheme, wetland protection, Xiaolangdi reservoir, Yellow River
Citation: Yang Y and Liu B (2023) Reservoir ecological operation on sediment-laden river considering wetland protection. Front. Environ. Sci. 11:1207032. doi: 10.3389/fenvs.2023.1207032
Received: 17 April 2023; Accepted: 02 May 2023;
Published: 11 May 2023.
Edited by:
Xudong Huang, North China University of Water Conservancy and Electric Power, ChinaReviewed by:
Han Xiaole, Hohai University, ChinaDawei Zhang, China Institute of Water Resources and Hydropower Research, China
Jinshu Li, Stantec, United States
Copyright © 2023 Yang and Liu. This is an open-access article distributed under the terms of the Creative Commons Attribution License (CC BY). The use, distribution or reproduction in other forums is permitted, provided the original author(s) and the copyright owner(s) are credited and that the original publication in this journal is cited, in accordance with accepted academic practice. No use, distribution or reproduction is permitted which does not comply with these terms.
*Correspondence: Yichen Yang, hhuinnanjing@163.com