- 1State Key Laboratory of Urban and Regional Ecology, Research Center for Eco-Environmental Sciences, Chinese Academy of Sciences, Beijing, China
- 2University of Chinese Academy of Sciences, Beijing, China
- 3Faculty of Agronomy, University of Lubumbashi, Lubumbashi, Democratic Republic of Congo
- 4Key Lab of Mountain Surface Process and Ecological Regulation, Institute of Mountain Hazards and Environment, Chinese Academy of Sciences, Chengdu, China
- 5Laboratory of Environmental Engineering and Management, School of Chemical and Environmental Engineering, Technical University of Crete, Chania, Greece
- 6Department of Civil and Environmental Engineering, Federal University of Technology, Akure, Nigeria
- 7School of Environmental and Municipal Engineering, Xi’an University of Architecture and Technology, Xi’an, China
- 8Department of Environmental and Prevention Sciences, University of Ferrara, Ferrara, Italy
- 9Key Laboratory of Eco-Environments in the Three Gorges Reservoir Region (Ministry of Education), College of Life Sciences, Southwest University, Chongqing, China
- 10Institute of Food Science and Technology, Chinese Academy of Agricultural Sciences, Beijing, China
- 11Department of Biological Sciences, Olusegun Agagu University of Science and Technology, Okitipupa, Nigeria
Considerable time and funding have been committed to tackling nonpoint source (NPS) pollution in agricultural and urban watersheds . Notwithstanding all these efforts, the water quality in many AUWs has not significantly improved. Diffuse nutrient pollution involves the movement of these pollutants between soil and water. Excessive diffuse pollution has been accepted as one of the main causes of failure to attain favorable environmental conditions in freshwater systems. Recently, several technologies and practices have been implemented to manage diffuse pollution, namely: a) source reduction, b) pollutant retention over the movement process; c) nutrient recycling, and d) purification and restoration of the eutrophic water bodies. This paper synthesized the existing knowledge of key methods to tackle diffuse pollution from AUWs. Furthermore, the predominant purification mechanisms and impacting factors are explored, allowing a comprehensive and critical understanding of different control strategies to improve the management of diffuse pollution. Therefore, potential approaches for strengthening the performance of control technologies for diffuse pollution treatment and remediation are discussed. Although adopting source reduction strategies (e.g., the “4R” approach: right rate, right time, right source, and right placement of nutrients) could efficiently decrease surface runoff and pollutant levels, they may not stop runoff from entering nearby streams. Consequently, comprehensive treatment of agricultural runoff still requires extra process retention strategies. Overall, the findings of this paper showed that treatment system design and operational and environmental factors played crucial but variable roles in diffuse pollution treatment. Moreover, the results showed that combining or integrating constructed wetlands with other control technologies could enhance the comprehensive purification of diffuse pollution compared to using a single method. This review proposes a systematic approach for diffuse pollution control based on three components (water, soil and microbiota) and maximizing the regulating services of agroecosystems via land use/cover types. The current review contributes to the documentation of existing research trends. It sheds light on diffuse pollution control approaches in AUWs, and further encourages the development of this vital field.
1 Introduction
Diffuse nutrient pollution of surface waters constitutes a considerable risk to drinking water sources, human health, aquatic organisms, recreational activities, and economic productivity in watersheds (Kumwimba et al., 2023a; Diaz and Rosenberg, 2008; Carpenter, 2008). The limiting pollutant in aquatic ecosystems is usually a nutrient (e.g., phosphorus) and therefore, the mitigation of nitrogen N) and phosphorus P) pollution has been advocated worldwide in scientific and policy studies in aquatic environments (Chislock et al., 2013). Managing and mitigating non-point source (NPS) inorganic pollutants in waterbodies resolve multiple UN Sustainable Development Goals (Rasul, 2016; Metcalfe et al., 2017). In addition, mitigating these pollutants is also a substantial part of one of the Chinese government’s major challenges and other developed countries. While both point sources (PS) (Figure 1A) and NPS of nutrients (Figures 1A,B) could influence water quality, NPS inputs normally originate from vast land areas and can be delivered overland and shallow subsurface or even via the atmosphere to streams (Howarth et al., 2002; Kumwimba et al., 2017a), making them complicated and almost impossible to estimate, target, and remediate. Hence, attention has concentrated more on NPS mitigation, especially the role of farming. They have become the major contributor of nutrients in numerous aquatic ecosystems, such as lakes, rivers, streams, and estuaries. Because of the ease of assessing nutrient inputs and concentrations, PS (industry, sewage purification facilities) are easier to identify and manage by onsite treatment (Rissman and Carpenter, 2015). Consequently, PS pollution has been adequately controlled in many developed and developing nations, supported by many environmental regulations. In contrast, NPS pollution (e.g., urban and agricultural runoff and atmospheric deposition) (Figure 1) is dependent on the watershed and environmental characteristics and is often sporadic rather than continuous in nature (Wu et al., 2017). Furthermore, agricultural NPS of nutrients is principally impacted by agricultural activities and accelerated by precipitation. Nutrients are transported with the overland flow, given that the runoff futures in watersheds, its formation and convergence, show great spatial heterogeneity because of the different land use and difficult topography.
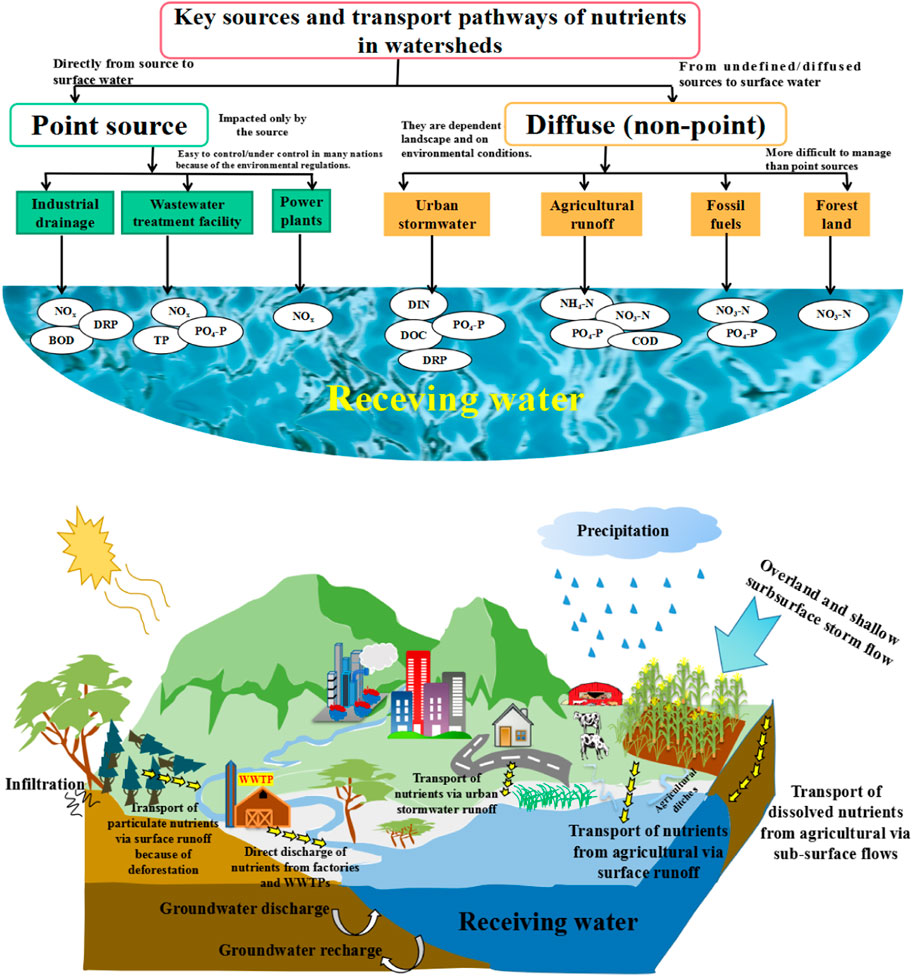
FIGURE 1. Hierarchy exhibiting the main sources and movement pathways of NPS pollution occurring in watersheds.
An assessment of the literature reveals that people around the world have continuously modified global nutrient cycling over the past few decades (Carpenter, 2008; Galloway et al., 2008; Gruber and Galloway, 2008). Additionally, eutrophication can be impacted by pollutant loads, water temperature, hydraulic features (e.g., low flow rate), etc., which, in turn, are influenced by socioeconomic development (Figure 1). In urban watersheds (UWs), for instance, the building of impervious surfaces alters the hydrobiogeochemical dynamics of rivers by limiting the infiltration of rainfall into the subsurface (Walsh et al., 2005). Septic system overflow and sanitary sewer discharges could also enhance NPS inorganic pollutant loads in urban regions (Mallin and McIver, 2012; Long et al., 2014). Agroforestry operations could enhance pollutant loads in forested watersheds by eliminating vegetation assimilation as a sink for pollutants (Wang et al., 2006). In agricultural watersheds (AWs) (Figure 1), practices including manure and inorganic fertilizer use, aquaculture, vegetation/crop residues, soils (that are washed off fields), atmospheric deposition, diffuse domestic sewage, managed animal feeding operations, overgrazing, rural runoff, etc., contribute NPS of nutrient loads to freshwater systems in a diffuse way (Lintern et al., 2018; Kumwimba and Meng, 2019). In China, for example, the discharges from cultivated lands, animal feeding operations, and aquaculture in 2007 were 13.24, 2.70, and 0.28 MTPY, respectively, for COD, TN and TP (Figure 2A). In particular, livestock and poultry were the major contributors to COD (12.68 MTPY), whereas total N and P were mostly generated from farmlands and livestock and poultry operations (Wu et al., 2017). Improper land uses in mountainous regions, and the resultant soil erosion and water loss can be responsible for these NPS of nutrients (Zhai et al., 2014; Liu et al., 2016a). In EU countries, published data have shown that agricultural fields account for approximately 33% of total water use and are the most important source of NPS nutrients in surface waters (EEA, 2012).
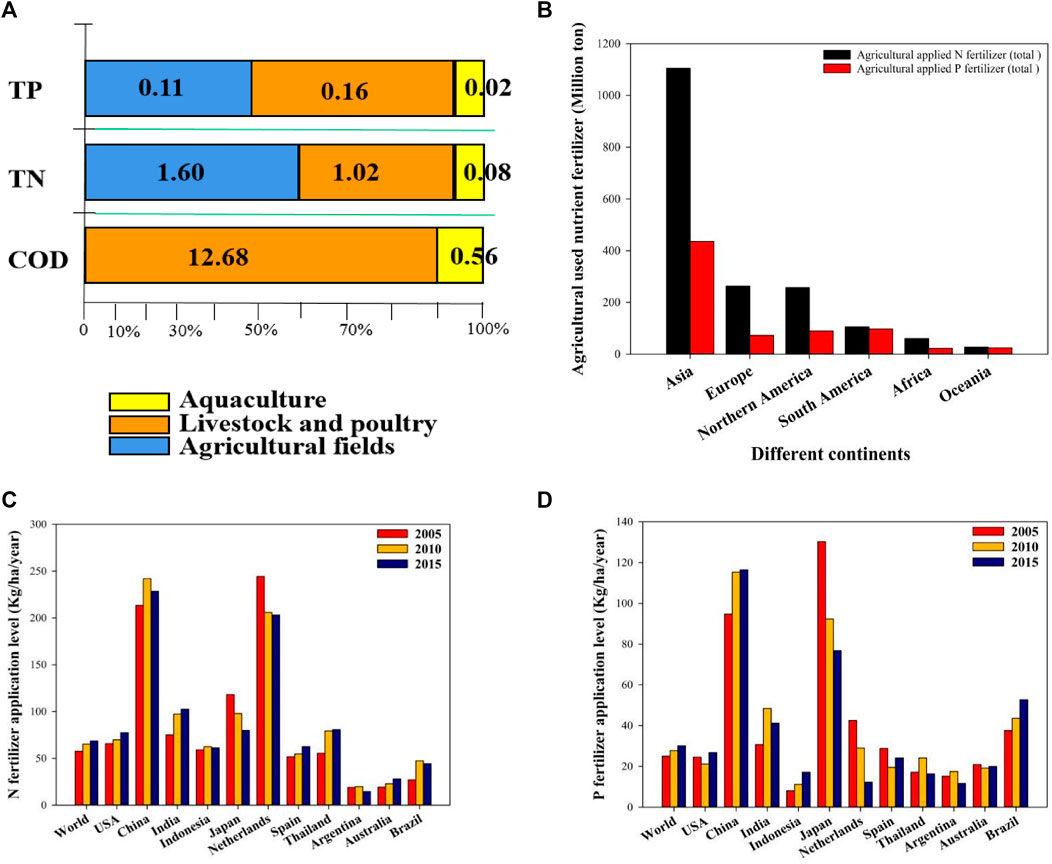
FIGURE 2. Pollutant discharges (Mt yr-1) from non-point sources, including cultivated lands, livestock and poultry, and aquaculture in China in 2007 (A) (Wu et al., 2017), the contribution of N and P (Mt) to the environment from various fertilizers applied on different continents (B) (FAOSTAT, 2021), and worldwide and country data on fertilizer application levels (C, D) (Information collected from Food and Agriculture).
Similarly, NPS pollution from agricultural fields is believed to be the principal source of nutrients in freshwater environments in the United States of America (Smith, 2003). Suitable management of agricultural runoff and animal waste is a serious issue for the U.S. administration and scientists. Similarly, the degradation of Lake Winnipeg in Canada was mainly caused by the uncontrollable NPS of nutrient enrichment (Tiessen et al., 2010). In short, despite years of investigations, technological progress, and heavy investments in protection, including several billions of US dollars spent over the last decade by governments worldwide (Environmental Working Group, 2018), NPS pollution continues to torment freshwater resources. The approach to managing diffuse pollution has been a hot and complicated subject in various areas, including environmental science, agricultural science, and ecology (Arif et al., 2022; Cao et al., 2023). Numerous researchers worldwide have been dedicated to this research field for decades. Undoubtedly, time and funds have been invested heavily into controlling NPS pollution in major and smaller watersheds worldwide. However, although water quality has been ameliorated, nutrient NPS problems persist and are indeed a wicked global problem (Ioannidou and Stefanakis, 2020; Lintern et al., 2020).
In an effort to alleviate the movement of NPS inorganic pollutants from land to freshwater systems and preserve peaceful, aesthetically appealing waterbodies while reducing their public health threats, natural resources managers and rural councilors have implemented a variety of control strategies and technologies over the years with varying degrees of success (Kumwimba et al., 2018; Xue et al., 2020; Addo-Bankas et al., 2022). However, most of these strategies concentrate more on treatment, rather than preventing NPS nutrient pollution, targeting only one contamination side. The single approach of nutrient reduction has been reported to be insufficient for NPS nutrient pollution mitigation. Furthermore, many review articles are restricted to one particular diffuse pollution treatment method (e.g., constructed wetlands, eco-ditches, vegetated buffer zones, retention ponds, etc.). In contrast, very few review papers have been performed to review ecotechnological methods for diffuse nutrient pollution comprehensively (e.g., Addo-Bankas et al., 2022). Therefore, finding an effective solution to organically merge the single approach to include all sides of diffuse nutrient pollution production is essential. A systematic and comprehensive control approach with related measures has been proposed to enhance the control of NPS nutrient pollution in both agricultural and urban watersheds. These approaches, based on the NPS nutrient pollution generation and evolution processes (e.g., generation-flow-sink), can be classified principally into a) source control strategies, 2) nutrient retention strategies, 3) nutrient reuse strategies, and 4) end purification and restoration of the contaminated water pathway.
Building upon these previous reviews, the main objectives of this review are to 1) comprehensively assess the overall research progress on using currently available control methods for managing NPS nutrient pollution and other water quality challenges; 2) clarify the major treatment processes and mechanisms in process retention technologies; 3) discuss the influence of system design, operational and environmental factors on NPS pollution treatment; 4) provide a panorama of the benefits and demerits of these control strategies and the possibility of combining and integrating. Methods; and 5) identify the possible knowledge gaps and future research directions related to the use of control technologies, as well as present recommendations for future research in this vital field. The current paper covers both urban and agricultural pollution control strategies.
1.1 Methodology
This study reviewed and analyzed the scientific literature to provide a clear and panoramic view of the pollution status across urban and agricultural watersheds. Moving beyond earlier reviews, this paper included studies across agricultural, urban, and mixed-use watersheds. We reviewed the literature that assessed NPS pollution control strategies intended to reduce NPS pollution, as well as their efficacies and advantages/disadvantages in watersheds. Both field-based studies and modeling studies were included in the current paper. The following criteria were utilized to choose the most relevant scientific publications performed around the world to encompass in this paper. The most common control technologies in both AUWs encompass constructed wetlands, ecological ditches, riparian buffer zones/vegetated filter strips, hedgerows and other field margin vegetation types, permeable pavement systems, green roofs, grassed planting trenches, stormwater wetlands, bioretention systems, media filters, infiltration basins/trenches, water dilution, aeration, flocculation, chemical precipitation (sponge iron and calcium nitrate), flotation beds, biofilm remediation, and microbial remediation. Relevant publications were identified and located using keywords associated with agricultural pollution and urban stormwater control technologies in the four well-known scientific databases (Web of Science database, Science Direct, Scopus and Google Scholar). The papers published in the last 23 years (2000–2023) were mostly chosen for a compressive review of research findings. Literature that fell within the scope of the review was summarized and sorted by method in an Excel spreadsheet. After a preliminary search, we reviewed all studies. Finally, we evaluated 298 pieces of literature by reading the abstracts and categorizing them according to the NPS pollution characteristics and control technologies. Because of the restriction of the main text length, the most relevant papers are critically reviewed to identify key knowledge gaps and make recommendations for making further advances in the field.
2 Essence of non-point sources of nutrient formation and development
The development of the agriculture sector is essential for increasing a country’s economic growth. It is of special importance to the most populous nations, including India, China, Indonesia, the EU, the USA, etc. (Cordell et al., 2009). Because of the huge need for food, commercial fertilizers have become crucial to sustaining high-yield agriculture in the past years (Huang et al., 2017; Yu et al., 2019). Data from the FAO (Figures 2C,D) indicate that, as of 2015, the global mean application of nitrogen and phosphorus fertilizer per cropland area has reached 68.6 and 30.1 kg ha-1 yr-1, respectively. The application of N and P fertilizers in the United States is still increasing, while China tops the chart for nations with the largest consumers and producers of fertilizers worldwide. Furthermore, the quantity of nitrogen and phosphorus applied in the farmlands of different continents was found to be greatest in Asia and North America (Figure 2B). Normally, agricultural sources such as cultivated lands, livestock and poultry, aquaculture, rural runoff, and untreated rural sewage are the primary contributors to diffuse nutrient pollution (Figure 2A), particularly in emerging nations (Ongley et al., 2010; Jia et al., 2016; Kumwimba et al., 2022). Freshwater resources around the world, such as Lake Taihu, Lake Erie, Chesapeake Bay, inland and Florida’s coastal waters, and the Baltic Sea, are damaged because of the rapid accumulation of harmful algal blooms that adversely impact water quality (Carpenter, 2008; Diaz and Rosenberg, 2008; Selman et al., 2008; Zhang et al., 2010; Osmond et al., 2019; Kumwimba et al., 2023b). The majority of the aquatic ecosystems get most of their diffuse nutrient pollution from cultivated lands, which have significantly contributed to NPS of nutrient losses impacting water quality for many years (Carpenter, 2008; Selman et al., 2008; Osmond et al., 2019).
With rapid social and economic growth over the past decades, the style of living and industrial structure in remote rural regions have continuously changed (Sun et al., 2012; Long and Liu, 2016). Consequently, diffuse nutrient discharges, encompassing those from rural areas and agricultural sources, have gradually increased and continually contaminated waterbodies (Yang et al., 2013a; Lintern et al., 2018; Xue et al., 2020). The formation and generation of diffuse pollutants in watersheds is a complicated physicochemical process, which is impacted by a number of factors, including topography, field slope, precipitation intensity, precipitation duration, runoff volume, land use and plant cover, application rate, application frequency, soil physical and chemical conditions, etc. Among these factors, rainfall features, topography, and land use patterns possess a larger influence on the formation and production of NPS pollution. NPS nutrients are often produced and transported over large areas, making it very complicated and almost impracticable to monitor their real origins, the periods of discharge, the nutrient levels and their spatial distributions (Ongley et al., 2010; Xue et al., 2020).
Rapid modernization, particularly intensive farming, normally defines the quintessence and features of creating and generating of diffuse nutrient pollution (Wu et al., 2017). This is particularly true since the 1980s, when the style of living and agricultural production structures in remote areas of China has undergone enormous modification, including the growing need for pig/cow meat, aquatic food products, poultry eggs, etc. (Sun et al., 2012). As a result, there has been a sharp rise in the source intensity and emission frequency of diffuse nutrient pollution, such as dispersed rural sewage, pollutant-rich runoff from farmlands, raw waste materials generated during farming activities and sewage from managed animal feeding operations (Sun et al., 2012; Xue et al., 2020).
With these transformations in lifestyle, rural residents began to seek improved economic advantages to support their existence. As a result, excessive chemical fertilizers and pesticides have been applied widely in farmlands. For major crops, rice and wheat, the N and P fertilizer application rates were 270–375 kg N ha−2 and 225–350 kg N ha−2, respectively, and 60–150 kg P ha−2 for both rice and wheat, with rice production reaching 21–28 kg kg−1 N and wheat production reaching only 11–13 kg kg−1 N in the southern area of the Yangtze River delta (Yang et al., 2013). Additionally, statistics show that the USA’s grain yield in California was approximately 33–42 kg−1 N (Eagle et al., 2000).
A 50-year trend assessment of nitrogen use efficiency (NUE) in global cropping systems showed that nitrogen loss was above 50 kg N ha−2 yr−1 in many populous nations, including India, China, the EU and the USA (Lassaletta et al., 2014). Minimum NUE enhanced discharges of N and P in overland flow from cultivated lands. The emission of these nutrients has become the most significant contributor and origin of diffuse pollutants (Wu et al., 2011a). In addition to the overland flow from cultivated lands, water and soil loss, particularly in highland areas, is an additional major contributor to diffuse pollution since these pollutants can generally be detached by the lost soil/sediment, which serves as the carrying substrate (Kumwimba et al., 2023a). In the Dianchi area, it has been estimated that water and soil loss could contribute 7.7% and 29.7% to N and P, respectively (Wu et al., 2011a). In short, the creation and generation of diffuse pollution includes the movement and conversion of nitrogen and phosphorus between soil and water. In conventional farming, nitrogen and phosphorus levels in soil and water are relatively greater than in water bodies. These circumstances could drive pollutant movement processes from cultivated lands into nearby waterbodies. The pollutant movement processes could be impacted by precipitation and fertilizer management and field slope, among other parameters.
3 Existing approaches to reducing diffuse nutrient pollution
To date, many control methods have been deployed around the world. Based on the formation and development processes of diffuse nutrient pollution (e.g., generation-flow-sink), four common groups of methods have been suggested to mitigate diffuse nutrient pollution (Figure 3), namely, a) source reduction technology, including the primary strategy of 4R management of fertilizer to utilize the right fertilizer source at the right rate, right time, and right place to achieve the economic, social, and environmental targets for each circumstance and prevention of water and soil loss (Figure 4C), b) nutrient retention technology during the movement process, c) nutrient reuse, and c) purification and restoration of eutrophic water bodies.
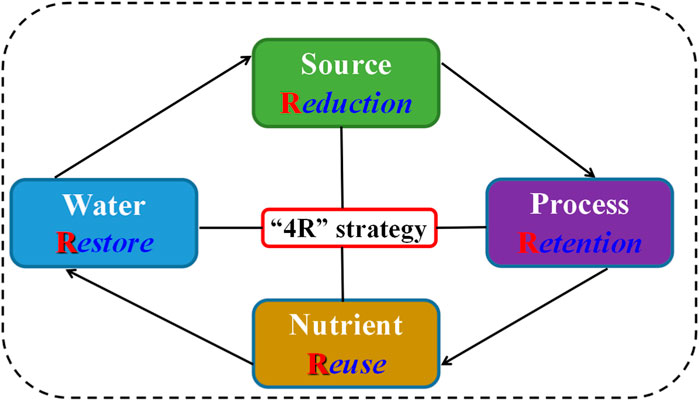
FIGURE 3. The systematic NPS pollution control approach encompassing its four stages (also known as the “4R” approach).
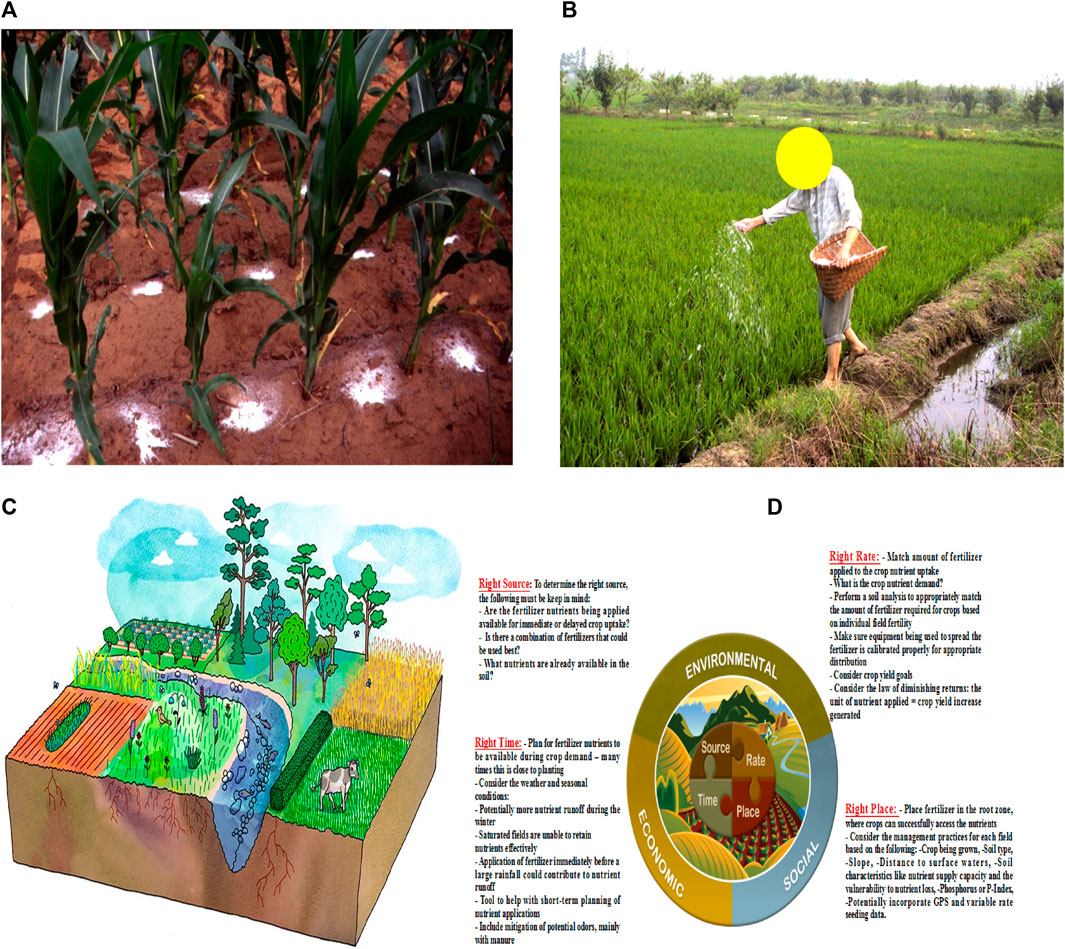
FIGURE 4. (A, B) The farmer applies chemical fertilizer in corn and rice fields (by Jianfeng Zhang), (C) different VBS used within and around fields (Picture: Gunilla Hagström/Form Nation), and (D) the basic strategy of 4R nutrient stewardship.
3.1 Current research on source reduction strategies
Pollution from crop cultivation (Figure 4A,B) can be a primary origin, and the control strategy is source reduction and process retention. Comparable to point source pollution control, the source reduction approach is the first line of defense for reducing the number of pollutants either dissolved from or bound to sediments, moving from agricultural lands during the rainfall or spring snowmelt periods and is the key to controlling diffuse agricultural pollution (Ribaudo et al., 2001). This approach (also known widely as the 4Rs) (Figure 4D) mostly refers to measures intended to manage the amount of nutrients released from highland erosion and runoff. Choosing and applying the right fertilizer source at the right rate, place, and time to attain the socioeconomic outcomes while minimizing ecological damage (Grant and Flaten, 2019) are important parts of all reasonable agricultural nutrient management plans. The principles of the 4Rs are relatively the same all over the world and are considered by many farmers as a precious tool to aid them in using nutrients productively. Excessive use of commercial fertilizers is directly ascribable to the discharge of nutrients from cultivated lands because of the low NUE (Sun et al., 2012). For this reason, the source control approach implies a change in farming operations, such as the optimizing nutrient, soil, and water management, NUE enhancement and lowering of farmers’ fertilizer input, water-saving irrigation, and runoff control. Additionally, water and soil conservation, including grass-crop rotation and other various tillage practices (Table 1) (Baulch et al., 2019), particularly in mountainous regions, can be other primary ways to decrease soil erosion and runoff and thus the generation of agricultural NPS of nutrients in farmlands.
In investigations of source control strategies, using a fertilizer with the recommended N rate decreased NO3-N leaching by approximately 29%–52% (Liu et al., 2016). Additionally, both organic matter and phosphorus addition enhanced soil nitrogen retention and decreased nitrogen loss (Wang et al., 2012; Mehnaz et al., 2019). Enhancing paddy fields could also decrease the threat of NPS pollution (Zhu et al., 2012). As discussed below, the establishment of hedgerows and other field margin vegetation types (e.g., beetle banks, trees, walls, shelterbelts, fences and gates preserved from the major crops within or around the cultivated land and installed to improve biodiversity and landscape aesthetic value as well as and soil erosion reduction, etc., can function as both source reduction and sink expansion) (Figure 4C) has been shown to enhance the soil structure and reduce the soil water and nutrient loss, thus decreasing the NPS pollution (Xia et al., 2013; Adhikary et al., 2017; Haddaway et al., 2018). Three lines of defense for diffuse nutrients: rice field source control, ecological ditches, and pond wetland treatment, could eliminate approximately 30% of the TN and 44% of the TP (Cai et al., 2017). Therefore, combining source reduction and process retention strategies can effectively decrease the diffuse nutrients from crop cultivation with heavy agricultural activities.
Credit: http://www.ipni.net/ipniweb/portal/4r.nsf/article/communicationsguide
3.1.1 Conservation tillage
Even though conservation methods like tillage may unavoidably perturb the soil surface, conservation tillage strategies, including reduced tillage (RT) and no-tillage (NT), can significantly decrease soil erosion rates (Daryanto et al., 2017). Other advantages of the conservation tillage (CT) include enhancing soil aggregation and increasing organic matter, thereby enhancing the water infiltration rates and water retention while encouraging biological activity (Plaza-Bonilla et al., 2013; Schmidt et al., 2019). Both RT and NT can be efficient methods of CT. For instance, a meta-data study of particulate P (PP) losses because of CT revealed 45% mean (concentration) and 55% (load) decreases relative to CT (Daryanto et al., 2017). However, these values did not reflect variations in climate, topography, cropping system, or length of tillage. Disaggregating the results showed that the efficacy of CT was reduced on more sloping lands (>4%) or with wetter antecedent states. Dodd and Sharpley (2016) reported a range for PP reduction from −33%–96% because of CT. Uusitalo et al. (2018) indicated that PP loads and levels were 27% and 55% lower, respectively, with NT compared to conventional till in Finland.
Clausen et al. (1996) investigated tillage influences on runoff for corn (Zea mays L.), demonstrating that RT decreased runoff by 64% and sediment losses by 99%. Adopting NT from a rice-planted watershed decreased the runoff volume and the TN and TP exports by 25.9, 8.5, and 7.8%, respectively (Liang et al., 2016). RT and NT decreased the intensity of tillage strategies and the effect of rainfall by conserving the soil surface using plant residues. Recently, it has been shown that ground covers and soil amendments like biochar, which improve soil aggregation and porosity, can be utilized to maintain the soil (Awad et al., 2012; Meier et al., 2017). Won et al. (2016) utilized rice straw mat cover, polyacrylamide, and gypsum to amend Chinese cabbage farmlands, which led to a decrease in suspended matter TN, and TP by 86.6%, 34.7% and 39.1%, respectively. Lee et al. (2015) assessed the effects of soil quality enhancement on soil loss. They found that the field soils treated with biochar and polyacrylamide decreased soil loss by 70.4% in a 33 mm d-1 natural rainfall, whereas there was no difference in the runoff. Lee et al. (2018) conducted an integrated assessment of the impacts of biochar application on runoff quality, soil losses, and agricultural productivity. They found that farm field soils amended with 4% biochar reduced runoff by 16.8%, soil loss by 25% and N loss (via runoff) by 41.8% (2018). Biochar has generally been applied in soil remediation and possesses enormous potential in agricultural runoff control. Further research on the impacts of biochar on soil aggregation and pollutant fixation is needed in the future (Prenarathna et al., 2019; Sun et al., 2020).
3.1.2 Rotation tillage
CT efficiently minimizes DN in overland flow (Liu et al., 2014). These practices, however, unavoidably cause soil compaction over the long term, resulting in phosphorus buildup on the soil surface and, therefore, an increase in phosphorus concentrations in the runoff. In the northern Great Plains of Canada, it was reported that CT decreased total nitrogen levels by 41%, whereas total phosphorus levels increased by 42% (Tiessen et al., 2010).
Another option to manage pollutant loss during agricultural runoff is reduced tillage (RT). Conversion of CT to RT to minimize phosphorus (both in surface soil and leachate from plant residues) losses during runoff was reported to be a good practice (Liu et al., 2014). Consequently, it decreased the levels of TDP by 46% and TP by 38% and reduced loads of TDP by 56% and TP by 42%. This is because tillage measures could reduce soil compaction and reduce phosphorus buildup in surface soil. In principle, plant residues in CT could increase the water holding capacity, resulting in higher runoff duration. Consequently, RT may diminish the exposure duration between plant residues and surface runoff which decreases phosphorus from plant residues. Daverede et al. (2003) assessed the impacts of two tillage methods (NT and chisel plough) and a range of soil P levels on the concentration and loads of dissolved reactive phosphorus (DRP). They showed that the latter practice could decrease DRP by 60%. Consequently, choosing tillage measures must be based on regional environmental circumstances, soil status, crops, and predominant eutrophication pollutants.
3.1.3 4R management of fertilization
To achieve these goals, the fundamental approach of 4R fertilizer management is to attain desired socioeconomic outcomes while minimizing negative ecological damage (Grant and Flaten, 2019). The principles of the 4Rs are relatively the same all over the world and are considered by many farmers as a precious tool to aid in the productive use of nutrients. N and P fertilizers are widely utilized in farming. Fertilizer-based NUE differs from crop to crop. For instance, the average nitrogen fertilizer utilization efficiencies of crops including wheat, rice, and maize are 18, 31% and 37%, respectively (Cassman et al., 2002). Consequently, it is essential to intentionally boost crop yield and agronomic efficiency while reducing adverse ecological consequences and maintaining N and P resources. Yao et al. (2018) studied the impacts of deep urea placement on N loss in the paddy land and found that deep urea placement decreased N loss by 50% while maximizing rice yield. Fertilization management plots, including band placement and hole placement, decreased TN by 63.6%–77% and TP by 42.8%–53.8% (Ye et al., 2016; Liao et al., 2017). Band placement could decrease contact with soil microbes and slow microbial nitrification. Zeng et al. (2008) measured N and P runoff losses from orchard soils as influenced by fertilization depths (surface, 10 cm and 20 cm) and rates and revealed that fertilization with chemical compound fertilizer at a soil depth of 20 cm decreased TN by 36.2% and TP by 31.4% in the runoff compared to both surface and 10-cm depth fertilization. Controlled-release fertilizer (CRF) can be another way to extend the duration of nutrient release and exploit the release rate, ideally to be compatible with the metabolic requirements of crops (Irfan et al., 2018). Tan et al. (2013) assessed the impact of fertilizer strategies on N loss via runoff on a wheat–maize rotation system surrounding Nansi Lake. They concluded that to decrease N loss, in parallel to securing continued good agricultural output levels, controlled-release N fertilizer combined with wheat straw integration must be promoted during corn production. Other research on the influences of controlled-release fertilizer reported a reduction in phosphorus loss in corn and paddy fields by 33% and 62%, respectively (Kun, 2012). Attention to the 4R stewardship would also manage the risk of loss of diffuse nutrients to the environment since the risk of pollutant loss from farmlands exhibits seasonal characteristics, with maximum pollutant loading in the rainy and fall seasons.
3.1.4 Water-saving irrigation
Intense rainfall and field drainage structures could drive overland flow. The rice‒wheat system is an essential potential N source for water pollution, accounting for up to 85% of cumulative nitrogen losses (Zhao et al., 2012). This is due to traditional flooding irrigation (TFI) continuing an elevated floodwater level in the farmlands. Water-saving irrigation methods can considerably decrease floodwater levels, enhancing the buffering capacity of farmland to aid in decreasing runoff and pollutant losses. In addition, water-saving irrigation improved root development by increasing grain production more than TFI (Aziz et al., 2018). Another technology that can be applied to decrease water use in irrigated lands is alternate wetting and drying (Hao et al., 2009; Matsuo and Mochizuki, 2009; Yang et al., 2009). This technology has been reported to decrease surface runoff by 30.2%–36.7% in compared to traditional methods (Liang et al., 2013). However, the levels of pollutants did not diminish with the reduction in surface runoff when alternate wetting and drying were utilized alone because the exposure period between water and soils could not decline. Therefore, integrating or combining irrigation management with farming practices and fertilization management should be the best approach.
All the aforementioned source reduction strategies can adequately address one or more nutrient loss pathways by decreasing the precipitation effect, enhancing infiltration, managing erosion, or decreasing leaching, agricultural runoff, and pollutant levels. However, they may not stop field runoff from reaching surface water. While source reduction strategies have considerably reduced the levels of agrochemicals in farm field runoff, it is still challenging to attain acceptable release levels. The long-term buildup of agrochemicals in waterbodies could also exacerbate the threat of eutrophication. Consequently, full control of farmland runoff requires extra process retention and end-treatment approaches.
3.2 Current studies on process retention strategies
As mentioned earlier, even after applying the primary strategy of 4R fertilizer stewardship, some nutrients unavoidably reach surface water via runoff and leaching during heavy rainfall or snowmelt. Consequently, the downstream ecosystems are substantially impacted because of the sensitivity of rivers to nutrient addition. Therefore, extending the water retention time and sequestering as much nutrients and sediment as possible before flowing into waterbodies is very crucial for NPS pollution control (Table 2; Table 3) to a greater degree, considering the high cost of conventional water contamination treatment.
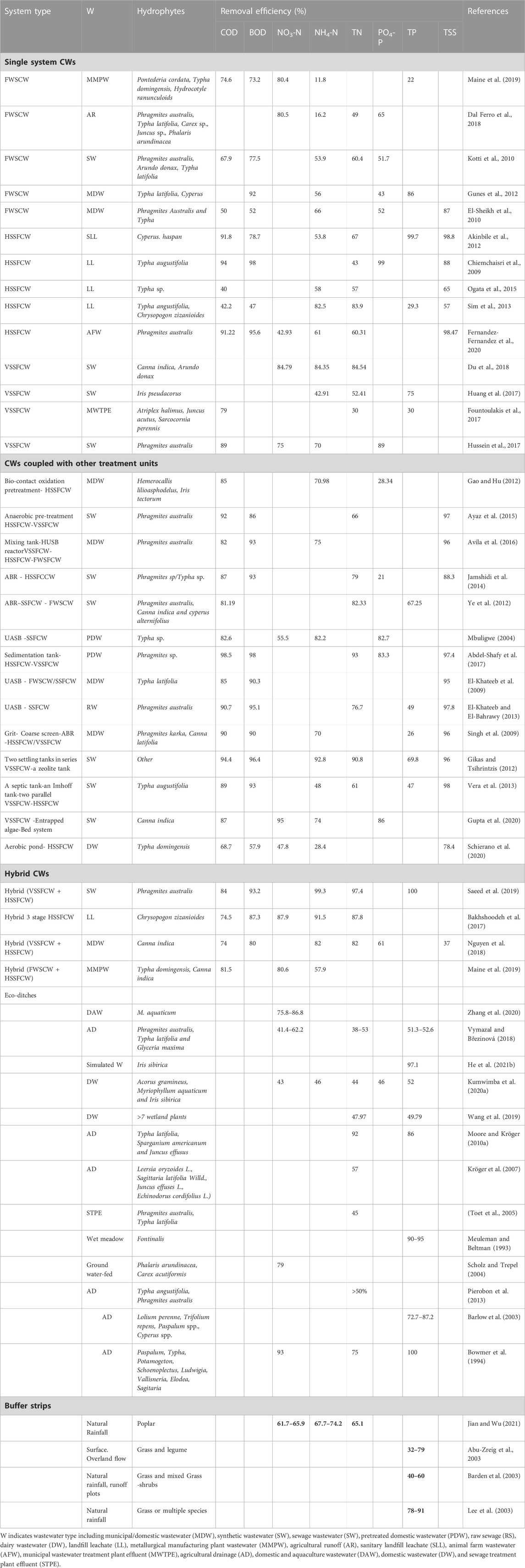
TABLE 3. Performance of constructed wetlands (e.g., single system, hybrid systems, and integrated or combined with other purification units), eco-ditches and buffer strips.
Process retention strategies offer a second defense against water contamination by intercepting and purifying pollutants during transport to streams by decelerating the overland flow velocities. The process retention strategies (Table 2; Figure 5) encompass ecological ditches/canals, vegetated buffer zones, grassed ponds, vegetation filter belts, constructed wetlands, etc. (Liu and Zhao, 2011; Castaldelli et al., 2015; Soana et al., 2017; 2019; Geng and Sharpley, 2019; Oral et al., 2021). Typically, ecological ditches are one of the most successful measures for intercepting pollutants from neighboring cropland. Before the pollutants are released into surface water, the ecological ditches could minimize agrochemicals in the overland flow, using a principle similar to an open-water constructed wetland. In addition, eco-ditches require less land area, and are inexpensive and relatively easy to implement. It is therefore considered to be a favorable measure for agricultural runoff control.
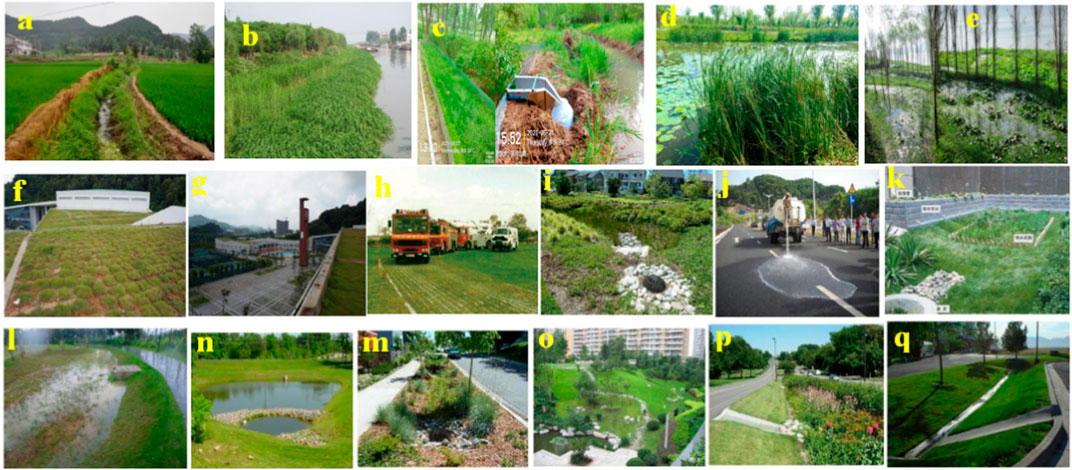
FIGURE 5. (A) Ecological ditches, (B, C) riparian buffer zones, (D) constructed wetlands, (E) vegetated ponds, (F) green roof, (G) permeable pavement, (H) permeable parking lot, (I) infiltration pond, (J) permeable road, (K) rain garden, (L) retention pond, (N) stormwater pond, (M) bioretention pond, (O) sinking square, (P) sinking greenland, (Q) rain water.
3.2.1 Ecological ditches
Agricultural drainage ditches are normally planned and built as a part of farm irrigation systems and can be an essential portion of agricultural watersheds. Their primary purposes encompass irrigation and draining extra water from agricultural lands (Kröger et al., 2007; Kumwimba et al., 2018). Ecological ditches are being converted from conventional farm drainage ditches (Wu et al., 2013) to minimize water flow, and intercept and mitigate pollutants and sediment from runoff (Otto et al., 2016; Kumwimba et al., 201b; Kröger et al., 2008; Cooper et al., 2004). Ignored in the past for their mitigation abilities, ecological ditches have great potential to minimize the movement of pollutants derived from cultivated lands in a similar way as constructed wetlands (CWs). Their acceptance could be ascribed to their small area requirements and possessing the characteristics of both free-water surface wetlands and streams (Kumwimba et al., 2023b).
Ecological ditches are referred to as transition zones between terrestrial and aquatic ecosystems. Depending on the conditions, ecological ditches could behave as a sink to retain pollutants and promote denitrification or as a source to release nutrients into the water column. Because of the substrate/sediment and macrophytes within these systems (Figure 6A), a unique substrate-macrophyte-microbial system is constructed, and complex physicochemical, and biological processes take place for contaminant removal in the system. Table 3 illustrates the typical ecological ditches with different purification efficiencies. Reduction rates of 38.3%–68.35% have been demonstrated for N and P by ecological ditches through hydrophyte assimilation, adsorption, and denitrification (Kröger et al., 2007; Yang et al., 2016; Vymazal and Březinová, 2018; Wang et al., 2019; Kumwimba et al., 2020b). Wang et l (2019) demonstrated NH4-N purification efficiencies of 26.7% (winter) and 51.9% (summer) for ecological ditches purifying rural wastewater. This is because macrophytes senesce and decay in water over the low winter temperatures, and the pollutants released from dead vegetation could further pollute the ecological ditch water (Kumwimba et al., 2023a).
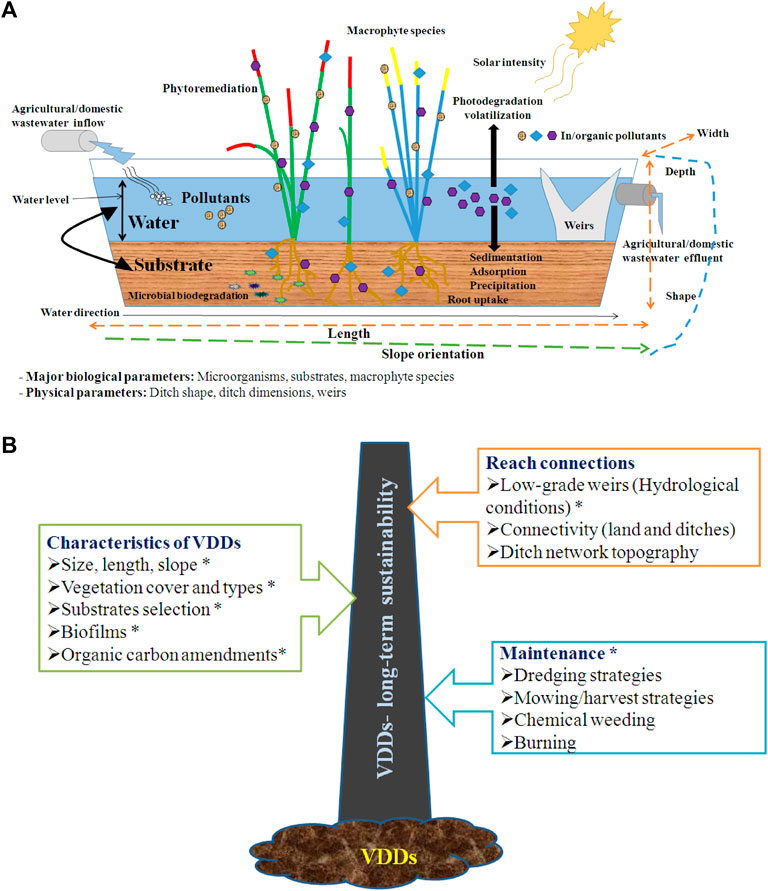
FIGURE 6. (A) Main functional features of ecological ditches/vegetated drainage ditches (VDDs) for the retention of pollutants and (B) future research needs for improving the sustainability of VDDs.
When well-managed, ecological ditches possess great potential to minimize nutrients like CWs while providing various ecosystem services (Dollinger et al., 2015; Kumwimba et al., 2017d). However, it should be noted that the agricultural runoff nutrient removal efficiency of ecological ditches and their sustainable long-term operation are increasingly perceived as crucial future challenges. The nutrient load of ecological ditches is determined by a complicated process referring to ditch characteristics (structural or functional features); and available maintenance (Kumwimba et al., 2018). Several management practices, including using low-grade sediment traps, various plant species, organic carbon barriers, etc., have been employed in agricultural ditches to enhance nutrient removal efficiency. Low-grade weirs can maintain water levels and improve HRT in ecological ditches, subsequently, offering reasonable nutrient purification rates in systems. Ecological ditches designed with low-grade weirs decreased 51%–83% of the influent TP loads (Flora and Kröger, 2014). On the one hand, ditch features (size, length, slope, hydrophyte cover, types, etc.) and ditch bed properties (substrates/soil media types) are key factors impacting the removal performance (Wu et al., 2013; Zhang et al., 2016a; Zhang et al., 2016b; Kumwimba et al., 2017c; Kumwimba et al., 2017e). On the other hand, the efficacy of ecological ditches in purifying agricultural runoff nutrients from farmlands is a complicated process that heavily relies on biofilms, connectivity between fields and ditches, hydraulic loading, depth, temperature, and ditch network topologies (Figure 6B), which in turn could lead to variations in the retention capacity of nutrients. In short, long-term research on the application of ecological ditches for both rural wastewater and farmland runoff treatment under various ditch management methods must be further studied.
3.2.2 Planted buffer areas
Planted buffer areas (PBAs) within and/or between farmlands and/or along water bodies can be seen as the last line/barrier to keep farm runoff and pollutants from reaching surface water bodies. A buffer zone is composed of three components: a) aboveground plants, b) belowground plant parts (roots and rhizomes), and c) subsoil. When agricultural runoff reaches buffer zones, water infiltration occurs. PBAs could diminish nutrient concentrations via filtration, infiltration, sorption, deposition of nutrient-bounded sediments, microbial transformation, etc. (Figure 7B). The removal efficiency of nutrients in PBAs is listed in Table 3. Various factors may impact the performance of PBAs, such as field parameters (e.g., type of nutrient, application rate, timing and frequency of nutrient application, rainfall intensity, runoff characteristics, slope of land, crop type), buffer vegetation type, soil characteristics, PBA dimension, drainage area to PBA ratio, season, management, PBA structure (presence of erosion rills), etc. (Lacas et al., 2005; Prosser et al., 2020; Kumwimba et al., 2023b) (Figure 7C). Most of these factors could enhance water infiltration, sedimentation, deposition, and biochemical reactions within PBAs. However, there is a current discussion about how wide the PBAs must be to prevent environmental consequences. Nevertheless, there is increasing uncertainty about the suitable PBA width for corridors or habitats, or what the minimum PBA width should be. Another issue is that their effectiveness in mitigating dissolved pollutants has been questioned (Figure 7B) (Kieta et al., 2018; Kumwimba et al., 2023a) since these practices can become a source of dissolved pollutants to sensitive waterbodies because of the saturation of PBA soil with nutrients. PBAs are not always effective in diminishing pollutant concentrations in cold climate regions. Excessive surface runoff events occur during spring snowmelt when PBA soils and PBA plants are frozen, and infiltration is highly restricted or absent and bacterial acclivities are low or nonexistent. Frozen/flattened vegetation can potentially contribute pollutants to runoff. Approximately 70%–80% of the surface runoff and pollutant losses take place during spring snowmelt in cold climate areas. Investigation of the removal of nutrients in buffer zones during snowmelt runoff is less frequent in comparison to rainfall-driven runoff. However, nominal absorption rates, incapacity for infiltration to take place or for sediments to be trapped within buffers, and the release of pollutants with snowmelt have been ascribed to frozen buffer zone soils and senesced plants. These circumstances hamper the capability of buffers to function as thought.
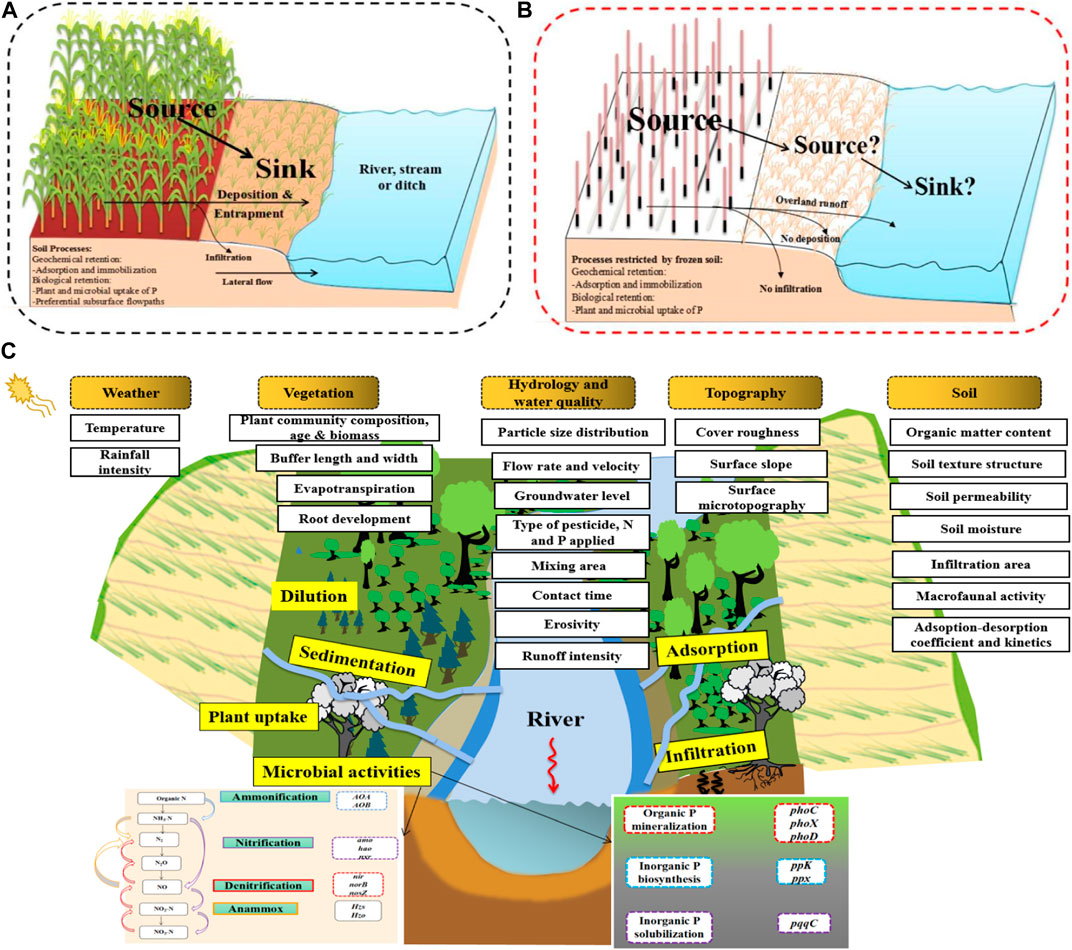
FIGURE 7. A conceptual diagram showing a number of the processes by which PBAs decrease nutrients in (A) warm and (B) cold climate regions (Kieta et al., 2018), and (C) yellow and white boxes indicate physical processes and parameters impacting the processes, respectively. Microbial nutrient-cycling networks in soil and related functional genes are shown in the lower left and right corners.
Satchithanantham et al. (2019) studied the pollutant retention performance of buffer zones by estimating inlet and outlet runoff pollutant levels during rainfall and snowmelt-driven runoff. The findings showed that pollutant levels of the soils in buffer zones could be the best indicator of whether the release or removal of pollutants could take place. The findings further indicated that VBZs act as a sink to sorb nutrients in runoff at low soil nutrient levels but as a source of nutrients at elevated soil nutrient levels. More variable responses were observed for NO3-N, but overall, the buffer zones trapped an important amount of NO3-N in the rainy period than in winter. This finding shows that the activities of vegetation and microorganisms in buffer zones are especially significant for nitrogen elimination. Vanrobaeys et al. (2019) assessed pollutant concentrations above and below the PBA. The results showed that PBAs are effective compared with cultivated land at minimizing sediment and sediment-bound phosphorus levels but do little to decrease dissolved forms of phosphorus. The efficacy of buffer zones in trapping phosphorus exhibits strong seasonality, as they possess certain abilities in the warm period but fail to perform during the spring snowmelt period. Therefore, it was suggested that the effectiveness of buffer zones must be improved in the summer when plants are actively developing. However, mowing plants at the end of autumn to minimize plant nutrient loss over these periods should be considered (Vanrobaeys et al., 2019). In cold climate areas, buffer design and management need careful assessment. Land managers and local governments must not greatly depend on buffer zones, as they may not function well for most times of the year to minimize pollutant transport. Further investigation must be carried out at a larger scale in natural systems and concentrate on optimizing buffer design and management methods.
To make PBAs more efficient, they must be “shaped” and smoothed out to encourage uniformly dispersed flow via more of the PBA’s surface, delay surface runoff, and trap chemicals in both dissolved form or attached to sediments. In addition, PBA management should be planned so that plants can be harvested and sequestrated pollutants can be eliminated. In low-lying zones where surface runoff takes place, it is advisable to put in a broad vegetated canal and remove/harvest the herbaceous plants. This implies that plants are inserted where the overland flow takes place and then mow the plants afterwards. Failing to do so, plants will senesce, die, and release back pollutants into the overland flow and streams. The PBA will not be as efficient without adequate management.
3.2.3 Constructed wetlands
Artificial or constructed wetlands (CWs) are engineered systems that imitate (or optimize) the biogeochemical and physical processes that take place in natural wetlands for cleaning sewage under controlled conditions (Vymazal, 2007; Kumwimba et al., 2021; Ajibade et al., 2023), while offering mutual gains such as ecosystem services and recreation. They can be categorized by application, like habitat formation, flood regulation and sewage purification. Nevertheless, from a practical or technical point of view, different configurations of constructed wetlands (e.g., free water surface flow, subsurface flow and floating wetlands) have been utilized to purify different types of water (Figure 8I).
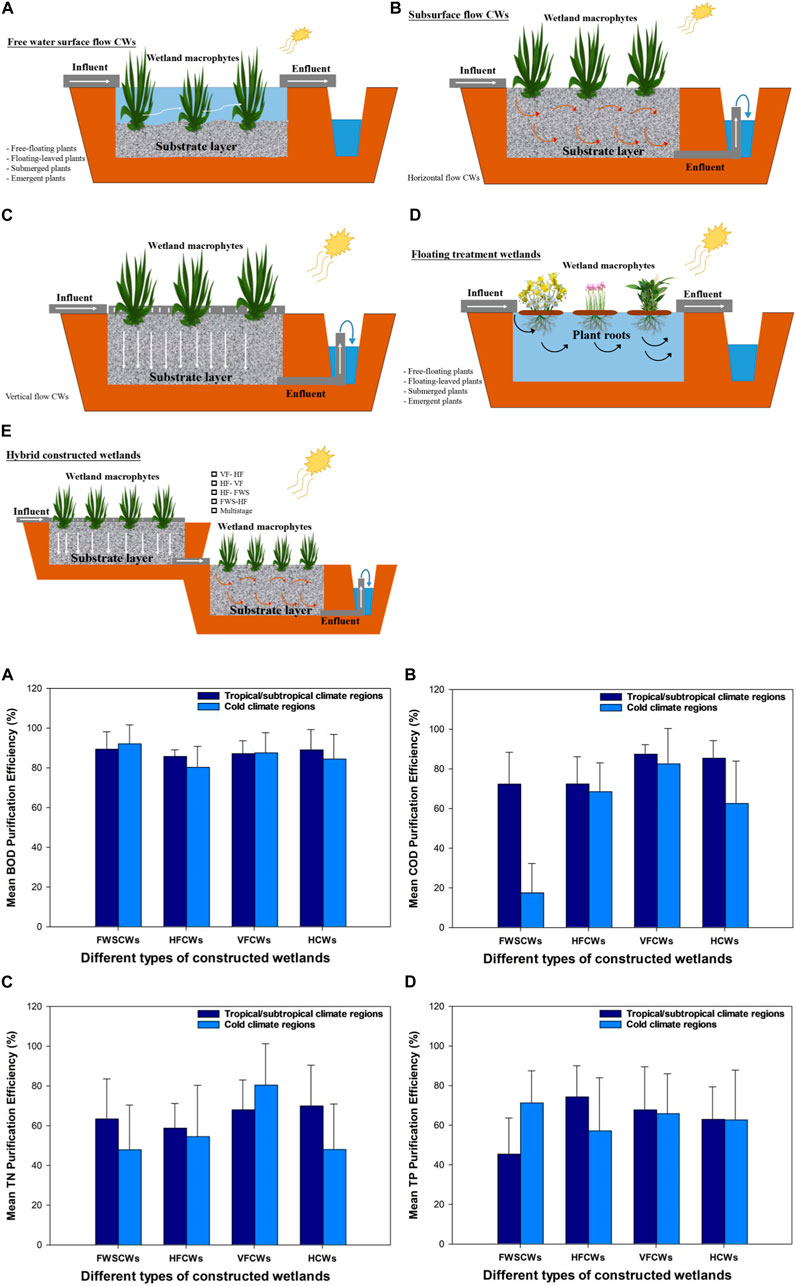
FIGURE 8. Schematics of I [(A) free water surface flow CWs (FWSCWs), (B) horizontal flow CWs (HFCWs), (C) vertical flow CWs (VFCWs), (D) floating CWs (FCWs), and (E) hybrid CWs (HCWs). They could possess various configurations with different fluid flow regimes and macrophyte systems. Each type possesses benefits, with hybrid/composite CWs designed to integrate these advantages, and II [(A) BOD, (B) COD, (C) TN, and (D) TP purification efficiencies in various CWs in tropical and cold climate regions. Different wastewater (Data source: Supplementary Table S1, S2)].
Sewage can be decontaminated in CWs via a sequence of processes (sedimentation, absorption, substrate/filler adsorption, precipitation, and microbial degradation, etc.) based on the synergistic actions of microorganisms, substrates and macrophytes (Brix, 1995; Vymazal, 2007; Stefanakis et al., 2014; Kujala et al., 2019; Kumwimba et al., 2020; Kumwimba et al., 2021). Fig. 8II shows the effectiveness of various constructed wetlands in tropical and cold climate regions. One of the great benefits of the CWs is the minimal operational and maintenance costs, in addition to many environmental advantages such as the minimum carbon footprint. This method may be used at small and large scales; nevertheless, CW requires relatively large land areas, depending on the design. Several factors could impact the performance of constructed wetlands, including feeding mode, HRT, HLR, pH, CW type, DO, inlet and outlet configuration, temperature, vegetation characteristics, etc. Hydrophytes, microbes, and substrates are responsible for the different treatment mechanisms (e.g., plant uptake, sedimentation, adsorption, filtration, microbial degradation, phytodegradation, phytoextraction, etc.) taking place in constructed wetlands. Undoubtedly, these mechanisms are impacted by climate conditions (Fig. 8II). These inherent weaknesses have usually limited their application and long-term stability. Valkama et al. (2017) assessed the daily and seasonal variations in pollutant retention performance of a boreal wetland. They showed that TP purification efficiency (28%) was most significant in summer and minimum (5.5%) in winter. Similarly, the TN purification efficiency (82%) was most remarkable in summer and minimal (3.5%) in winter. Certainly, the retention of N in constructed wetlands is a crucial question, particularly at low winter temperatures. The key nitrogen removal pathways are biological processes, which greatly rely on microbial nitrification-denitrification processes (Ji M. et al., 2020; Kujala et al., 2019; Varma et al., 2021; Ajibade et al., 2021a; b). An evaluation of most peer-reviewed papers on the performance of full-scale CWs globally showed that N was the most susceptible parameter to be easily impacted by low cold temperatures. In contrast, P, TSS and organic matter remain relatively less affected (Ji B. et al., 2020). Aiming to enhance the inadequate O2, low influent C/N ratio and low purification rate of constructed wetlands in cold temperatures, scholars have developed many modifications and different designs based on the system’s internal components (bacterial activities, macrophytes, substrate addition, and probable bioaugmentation) or external operational factors (heat loss, external aeration, operating conditions, and DO, etc.) (Figure 9; Figure 10). These modifications and technologies can promote water purification efficiency while keeping system ecological features and economic benefits.
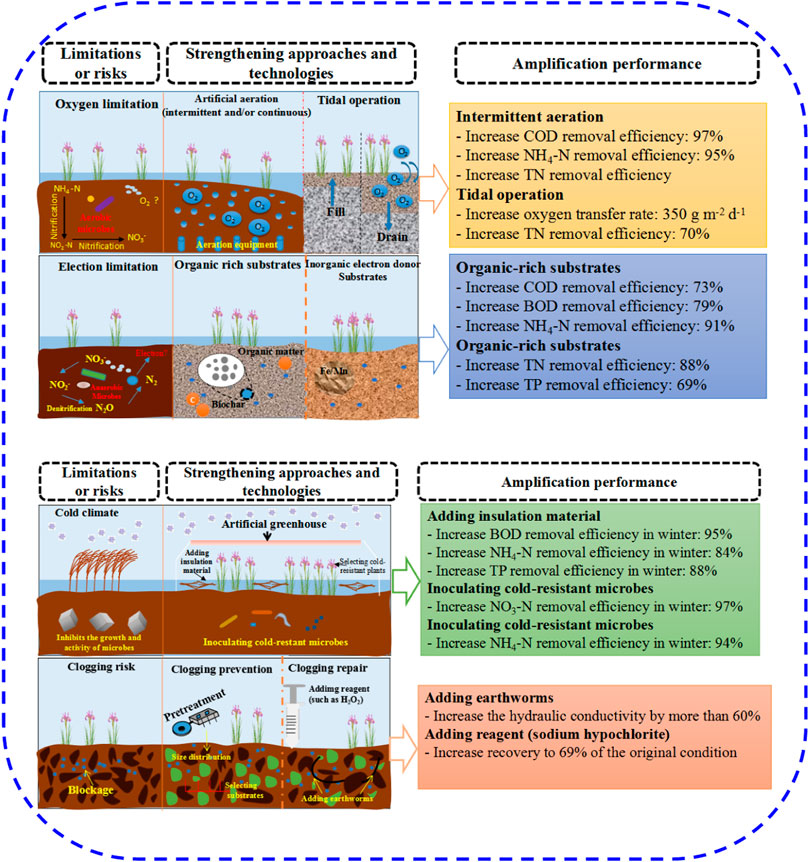
FIGURE 9. Modifications and technologies responded to the limitation or risks of constructed wetland performance at cold temperatures (Kumwimba et al., 2020a; Ji M. et al., 2020; Wu et al., 2023).
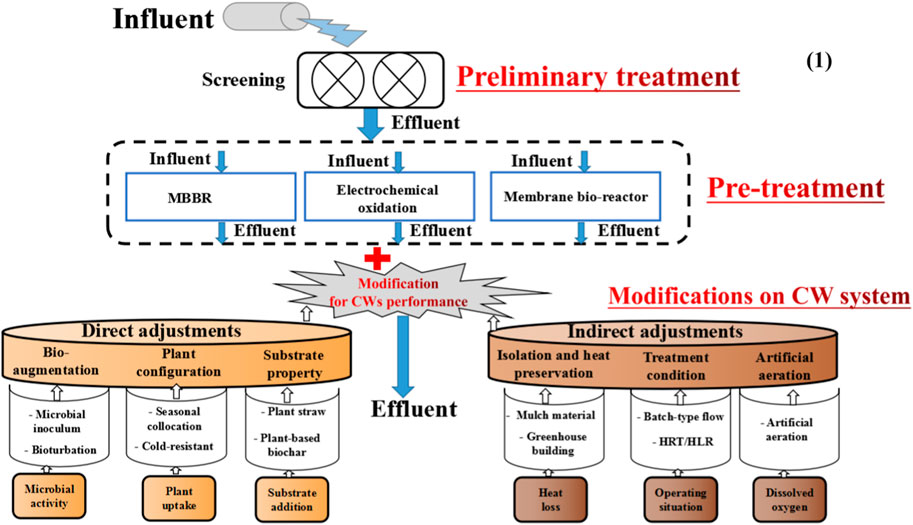
FIGURE 10. Recommended approaches for proper modification (1) to improve pollutants’ purification and recovery in constructed wetlands (Song et al., 2018; Ji B. et al., 2020; Kumwimba et al., 2021; Zhong et al., 2023).
Scaling has been one of the greatest challenges in CW research over the last few years. Extensive works have been conducted at the microcosm and mesocosm scales, but only a few research studies have been performed on full-scale CWs. Findings of these small-scale research or lab-based experiments are usually rarely applied to full-scale CWs, although few large-scale systems have been reported in the literature (Stefanakis, 2020; Alwahaibi et al., 2021). Furthermore, numerous findings are uncertain for full-scale CW systems, primarily from the operation, maintenance, and labor perspectives. The other key challenge is the investigation of the long-term performance of CWs. Existing studies are often brief and short, less than a year, which may not provide any valuable data concerning long-term performance. To improve the application of CWs, it is essential to perform more studies on full-scale CWs purifying actual sewage, specifically concentrating on long-term performance.
Growing interest in process retention strategies brings attention to vegetation-based water treatment and its role in diffuse pollution management. While understanding the suitable design, operation and management of vegetation-based water treatment systems is still depends on empirical parameters based on long-term assessment, there are complete global information gaps in understanding the system design, operation and maintenance. Knowledge of environmental conditions at the site (weather), sewage compositions, hydrology and hydraulic characteristics, substrate/filter medium types, vegetation type, system kinds and ages, full design aspects and water-quality parameters should be involved from both sources, e.g., scholars, and water treatment companies and city managers. There is still much room for developing and enhancing the predictive ability of models (related to both agricultural and urban conditions) for a deep understanding of the extra features, including transport and purification processes of diffuse nutrients and other contaminants on a watershed scale, and thereby helping to determine and target pollutant control strategies and to assess their relative efficacy. A thorough examination of ecological and health risks related to organic contaminants must be carried out in the plant-based treatment systems to remediate and manage the risks of water reuse through the use of a risk quotient approach and embracing measurable indices like the disability-adjusted life year. The application of plant-based treatment systems for goals other than eutrophic water purification could be another major field that the authors suggest for future studies. These systems could be essential in decreasing the carbon footprint of WWTP’s and developing a circular economy. For instance, the integration of plant-based treatment systems with bioelectrochemical systems to synchronously treat eutrophic water and produce power, which can reduce carbon transmissions. There are challenges in the approval and efficient operation of plant-based treatment systems, particularly in the least-developed nations. Developing practical and adaptable frameworks for design, building, operation and maintenance is required to orient and facilitate their use, particularly in least-developed nations. Future plant-based treatment system implementation must attempt to equalize the long-term sustainability of water purification and socioeconomic benefits, beginning from the design stage. In this context, plant-based treatment systems could and must be incorporated into city ecosystem growth by inserting characteristics including green roofs and green walls, further strengthening attempts to attain the global goals.
3.2.4 Ecological floating beds
Ecological floating beds (EFBs), also known as floating treatment wetlands, artificial or planted floating islands, are another nature-based purification method that uses buoyant structures to grow aquatic or terrestrial plants on the water’s surface. Pollutant removal in EFBs is accomplished using many mechanisms (Figure 11A). Constructed wetlands with floating hydrophytes have been extensively applied worldwide to purify stormwater, wastewater, landscape water, and rural river water and prevent harmful algal blooms with low operational and maintenance costs (Wang et al., 2020a; Kumwimba et al., 2020; Kumwimba et al., 2022). For instance, field-scale EFBs purified 60 million m3 per year of sewage at a cost of only CNY 0.0018 per m3 (Afzal et al., 2019). Similarly, the operation and maintenance cost of the hybrid EFBs (0.0138 CNY/m3) was much lower than that of traditional sewage treatment facilities (Kumwimba et al., 2023b). In contrast to other ecological purification measures, EFBs benefit from not requiring large land areas while directly purifying eutrophic river water in situ at the field scale. Furthermore, EFBs could provide amenity value by greening and beautifying the rivers and the surrounding environment. These ecological advantages with numerous environmental benefits have resulted in their broad application worldwide, becoming compulsory in certain regions to obtain improved water quality (Olguín et al., 2017; Kumwimba et al., 2020b; 2022; Colares et al., 2020).
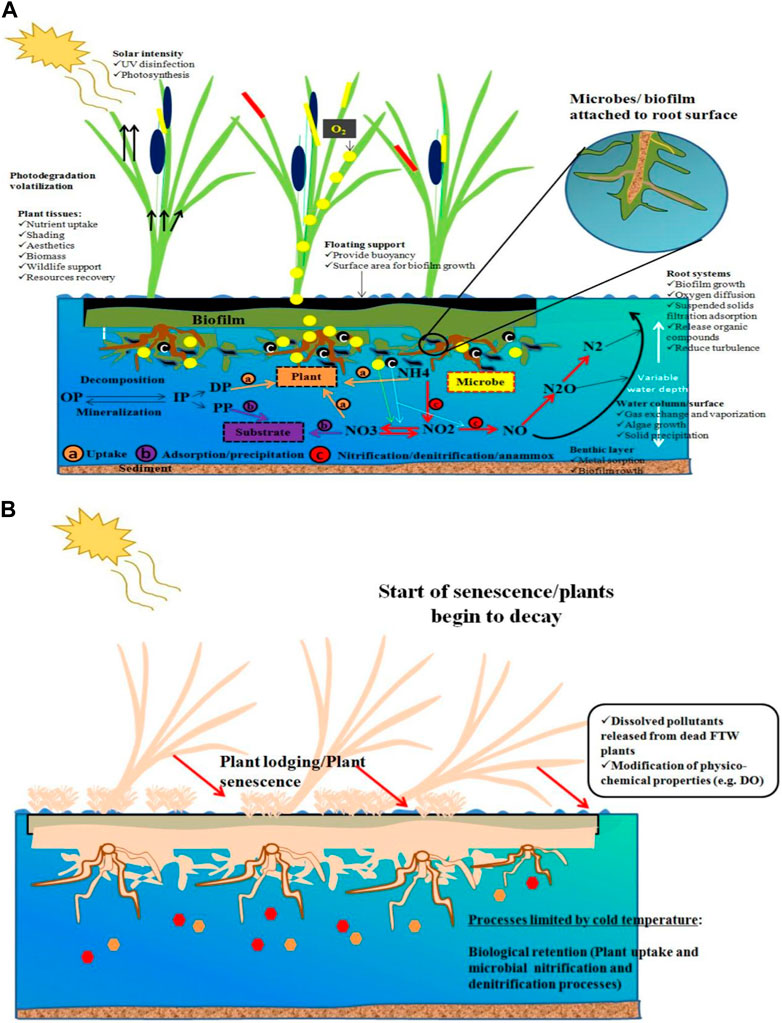
FIGURE 11. Conceptual schema displaying the different processes by which EFBs clean surface waters in summer when hydrophytes are actively developing (A) and at the beginning of autumn when hydrophytes begin to decompose during the rest of the year (B).
Like CWs, the purification performance of EFBs mainly relies on their hydrophyte roots (Figure 11). Nevertheless, several problems cast doubt on the use and long-term efficacy of EFBs (Figure 11B), especially their poor purification in cold temperature conditions due to the alteration of biological activities and hydrophyte absorption processes (Tharp et al., 2019; Wang et al., 2020a; Kumwimba et al., 2020; Kumwimba et al., 2022). EFBs are ineffective in deep and static water bodies as the macrophyte root lengths limit them. For instance, the mean length of hydrophyte roots in EFBs varies between 20 and 45 cm, making it unfit for use in deep waters, resulting in poor purification performance (Chen et al., 2016). The unsatisfactory EFB performance for carbon-limited wastewater treatment, especially in cold climates, is an inevitable limitation of the application of EFBs (Wu et al., 2021). Besides, insufficient carbon sources and/or electron donors could often limit the microbial denitrification process in EFBs, leading to poor pollutant removal performance. Nutrient removal in EFBs can be unsteady and insufficient during river remediation because of the restricted development of many floating macrophytes and the attached biomass (Sun et al., 2009).
In recent years, several technological innovations aimed at enhancing the purification performance of EFBs have been achieved (Figure 12). These encompass the incorporation of artificial biocarriers, artificial aeration, bioaugmentation, and the addition of various substrates (e.g., zeolite, alum sludge, biochar, woodchip, and stereo-elastic packing) and their combinations beneath the floating mats of EFB for the promotion of microbial attachments. Water depths of 0.6–1.1 m within EFBs and a rational bed coverage of 5%–38% were suggested for EFB designs, and the hydrophyte density should be inversely proportional to the hydrophyte size (Wang et al., 2020). Other studies also recommend up to 70% of needed bed coverage using Pontederia crassipes for a 0.25 m water depth and a contact time of 3–5 days to purify a eutrophic river (Gaballah et al., 2021). These results indicate that coverage must be expanded as much as possible for hypereutrophic rivers. For waterbodies with moderate eutrophication, the bed coverage could be minimized accordingly. Harvesting of plant biomass can be one of the essential operation parameters. Colares et al. (2020) reported that macrophyte harvesting could raise operation and maintenance expenses. However, the management of macrophyte biomass by harvesting could eliminate pollutants, and it can also be used in animal feed, green matter and compost, cosmetics, therapeutics, biosorbents, biofuels, and human food, thereby decreasing the overall system expenses. Another issue to address is the suitability of different hydrophytes for EFBs. Rigotti et al., 2021 reported that Typha domingensis was better at pollutant removal than Scirpus californicus in EFB treating urban runoff.
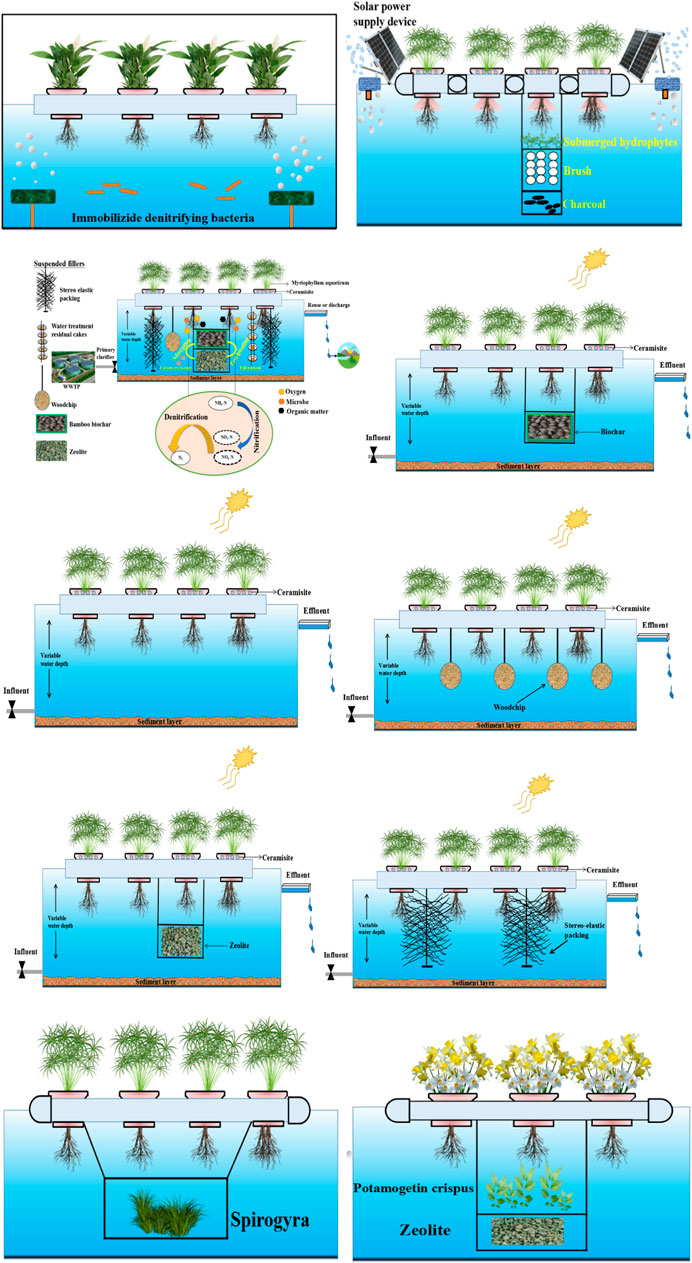
FIGURE 12. Various strengthening strategies of traditional ecological floating beds for improved purification efficiency.
3.2.5 Microbial biofilm-mediated bioremediation
The addition of particular bacterial cultures to a contaminated environment (also known as bioaugmentation) has become an effective strategy for controlling algal proliferation and eutrophication (Xu et al., 2017; Simon and Joshi, 2021) because of its efficient, robust, reliable, and cost-effective nature. Bioaugmentation greatly depends on the metabolic activity of microbes by breaking down and converting hazardous substances while improving environmental integrity. In bioaugmentation, bacteria are the most prevalent organisms. Biofilms, aggregates of microorganisms on biotic and abiotic surfaces, have also been demonstrated as a rather powerful constituent of environmental sewage purification technology.
Yang et al. (2019) effectively used a new plastic carbon fiber filler to develop a biofilm technology to treat eutrophic water. They found an excellent purification performance for eutrophic water pollutants (e.g., TN, TP, and COD). Common biofilm methods used to restore eutrophic water systems encompass filler contact oxidation, contact oxidation, fluidized bed aerated biological filters, thin-layer flow technology, suspended biofilm reactors (frequently also known as moving bed biofilm reactor), and steam injection approaches for enhancing geothermal steam containing pollutants (Qian et al., 2021). Many published benefits for the utilization of moving bed biofilm reactors include space savings, robust and simple reduction of contaminants, less temperature dependence, inexpensive and relatively easy processes, minimal operational and maintenance costs, low biomass loss, steady biofilm thickness, and minimal likelihood of clogging (Zhang et al., 2016). Hypereutrophic waterbodies and sewage can be treated by biological contact oxidation techniques, which have many benefits, such as promoting sludge thickening and the lack of bed clogging. Nevertheless, their effectiveness varies with seasonal temperatures. This method is eco-friendly and cost-effective in improving contaminant degradation (Simon and Joshi, 2021).
3.2.6 Constructed wetlands combined or integrated with other purification processes
With the goal of optimizing purification performance while limiting ecological risks, many scholars have evaluated the potential of exploiting the advantages of the combining or integrating several purification processes (Addo-Bankas et al., 2022). Table 1 illustrates different purification strategies, their corresponding purification mechanisms, and the advantages and disadvantages of each strategy. These integrations could enhance the purification performance for eutrophic water treatment compared to the individual approaches. Šereš et al. (2017) combined constructed wetlands and stabilization ponds to enhance secondary sewage purification, particularly dispersed village sewage or concentrated sewage in littoral areas. It is desirable to reduce building costs in regions with several ecological drainage ditches and conduits (Ham et al., 2004). Similarly, integrating an algae pond via the O2 enhancement of algae photosynthesis and a constructed wetland considerably improves nitrogen elimination (Zhao et al., 2016). Meanwhile, microorganisms could use the algae debris in the constructed wetland as a carbon source in treating sewage with a low carbon-to-nitrogen (C: N) ratio. Moreover, Lin et al. (2003) integrated aquaponics and greenhouse structures into constructed wetlands. Other scholars (Hu et al., 2012; Wu et al., 2014) established an integrated system of eco-wetland ponds and the extensive use of resources, which offered economic advantages to the regional freshwater aquaculture industries. Combining TiO2-photocatalysis and CWs to purify many specific contaminants in sewage effectively has also been demonstrated in recent research (Antoniadis et al., 2007; Arana et al., 2008; Herrera-Melian et al., 2012; Wu et al., 2016). The combination of CWs with ozonation was also found effective in the treatment of complex agro-industrial effluents such as the cork boiling wastewater (Gomes et al., 2020). Another fascinating research was performed by Huang et al. (2022), in which a bypass CW system integration method, including surface flow CWs, eco-gravel beds, eco-wetland multipond systems, and underwater forest ecosystems, was implemented to enhance river water quality. The results show that the integrated technology operates stably and could enhance contaminant removal in river water. For example, NH3-N, TP, and COD concentrations were less than 1.0, 0.2 and 20 mg L-1, respectively, while DO exceeded 5 mg L-1, and transparency exceeded 60 cm. Additional studies were performed on the potential of combining aeration and algae-eating fish for eutrophication and algal growth control in rivers. Integrating EFB and aluminum-based drinking water treatment residual enhanced its purification performance considerably (He et al., 2022). The findings exhibited improved N and P removal efficiencies, with mean retention of 53.3% for N and 45.3% for P, compared to the control with 27.3% for N and 12.8% for P. It was also revealed that combining fillers with EFB might enhance nutrient retention while boosting macrophyte development.
3.3 Nutrient reuse strategy for minimizing diffuse pollution
Here the reuse strategy refers to the reuse of nutrients in polluted water or drainage and solid wastes (straw and fecal) as much as possible prior to their transport to the waterbodies (Figure 13). Another strategy involves the collection of drainage or rural wastewater in ponds for use to either reirrigate agricultural fields, or even grow hydrophytes with economic importance, or released them into the wetland. This strategy can, therefore, save significant resources, minimize pollution, and enhance economic advantages (Yang et al., 2013). It can also achieve economic and environmental goals, leading to a win‒win situation. Hydrophytes harvested from polluted river water and drainage ditches could be composted and then recycled to farmlands as organic fertilizer. Up to 247.69 kg N and 35.09 kg P could be eliminated from waterbodies using this strategy (Xue et al., 2020), contributing to water quality enhancement. In addition, the compost also substituted 304 kg of urea and can decreased the nitrogen runoff loss by 11.2 kg. Moreover, rice production could be greatly enhanced compared to the farmers’ nitrogen management practices, while the soil’s total nitrogen and organic matter content were also enhanced by using organic fertilizer (Xue et al., 2014).
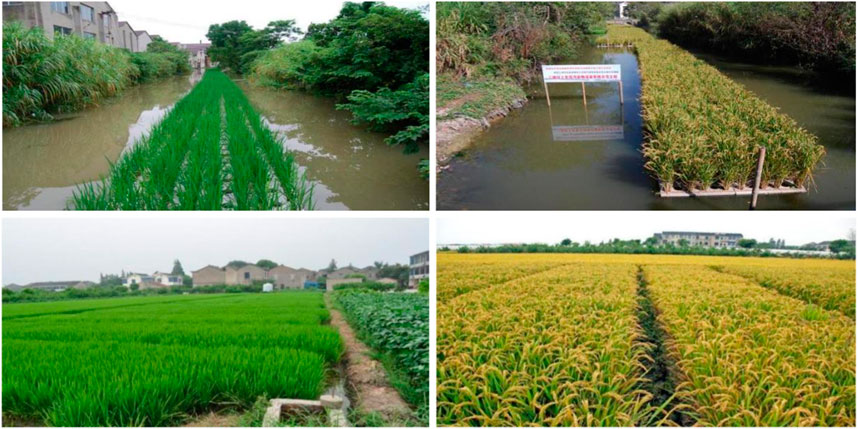
FIGURE 13. Rice planting to eliminate nutrients in waterways and wastewater treatment with paddy wetlands (Photograph taken by Jianfeng Zhang, Lin-Zhang Yang).
3.4 Aquatic environment restoration
This approach refers to eutrophic systems, including streams, canals, ditches and ponds in agricultural or urban settings, instead of the last destination water bodies such as lakes and reservoirs. Although methods could be adopted to minimize nutrient fertilizer inputs and trap nutrients during transport processes, an essential amount of these pollutants could inevitably be discharged into these water systems via which the runoff of diffuse nutrients enters (Liu et al., 2016c). Consequently, the purification of water bodies is required to restore the aquatic environments and enhance water quality. Purification and improvement of the streams, ditches and canals is the final method and defense for agricultural diffuse pollution control. Up to now, many restoration methods such as EFBs, eco-submersible dams, vegetated urban streams, and submerged plant purification methods have been proposed and extensively utilized (Wu et al., 2011b; Liu et al., 2016c; Kumwimba et al., 2023a). Nevertheless, these methods generally require prolonged HRTs for nutrient removal, restricting their use in restoring surface water.
3.5 Current urban stormwater runoff pollution control strategies
Stormwater runoff (SR) has significantly contributed to surface water pollution and the degradation of freshwater systems in city centers. Many effective methods have been developed for stormwater management in recent decades. These control methods could be categorized into source control (e.g., green roofs, permeable pavement systems), process control (e.g., concave green space, grass planting trench, planted buffer strips), and terminal treatment (rainwater wetlands, bioretention) according to the implementation stage of stormwater control. Figure 14 summarizes the efficiencies of various urban stormwater runoff pollution control strategies on runoff reduction and peak flow control.
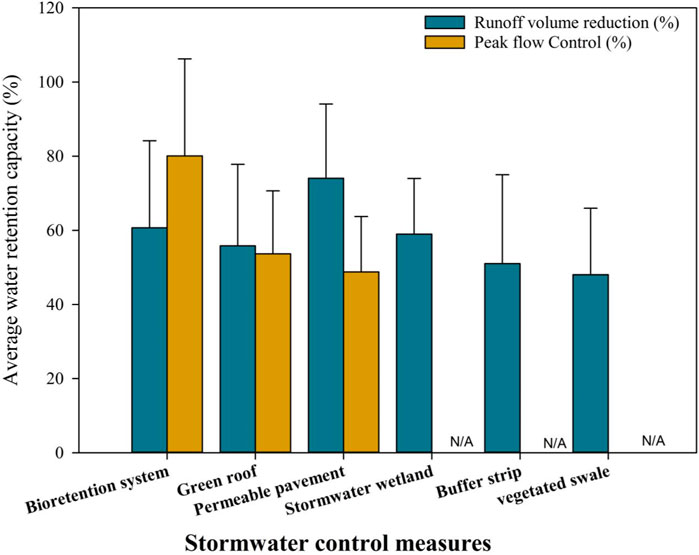
FIGURE 14. Summary of the average runoff reduction and peak flow control of different stormwater control measures.
Major processes in stormwater purification systems and factors that could influences these processes are shown in Figure 15. A significant portion of nutrient reduction is contributed by biotic processes involving vegetation and microbes. Design factors including area, depth, saturation, modifications, and vegetation could influence the efficacy of chemical and biological processes. Climate including temperature, rainfall intensity, and drying duration could influence nutrient reduction and health of microbes and vegetation that encourage nutrient retention from SW.
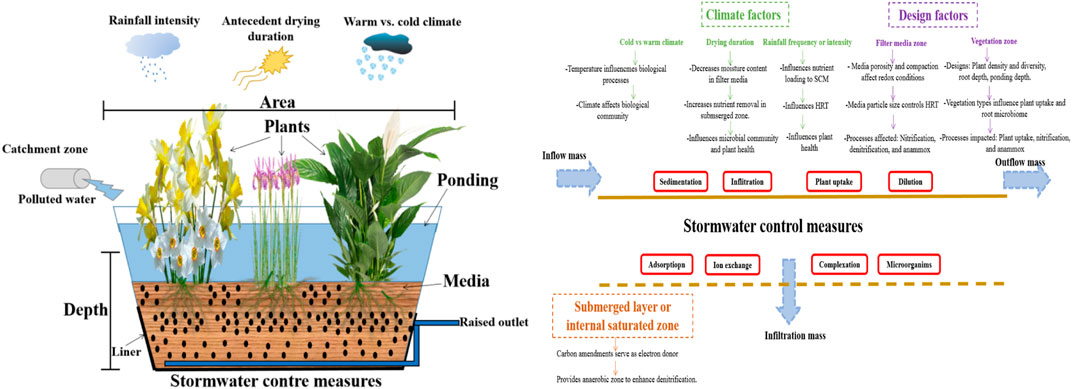
FIGURE 15. Pollutant removal processes (red solid boxes) in stormwater purification systems that might influence nutrient levels and the role of parameters (broken boxes: e.g., climate and design) in controlling these processes (Valenca et al., 2021).
Green roofs (GRs): (also called vegetated roofs or living roofs) have been widely considered the best method to manage SR in the urban areas where buildings occupy a vast space. SR is adequately sequestered and removed via a number of biochemical reactions within GR vegetation and substrates, which help to absorb carbon, keep energy and decrease heat island effects. Evapotranspiration from GRs cools the buildings, enhances air quality, expands the life of base roof materials, enhances biodiversity, boost the aesthetic quality of the building, enhance SR quality (Abass et al., 2020; Calheiros and Stefanakis, 2021). For instance, GR substrate sequestered organic contaminants from SR, improving the water quality (Berndtsson et al., 2006). Teemusk and Mander (2009) assessed the GR efficiency and found that the GR decreased the N level compared to runoff. On the other hand, many recent findings demonstrate that GRs degrade the SR quality (Moran et al., 2004; Hashemi et al., 2015). Berndtsson et al. (2006) reported that a large GR sequestered substantial amounts of organic pollutants, including 97% of Cu, and 96% of Zn. In North Carolina, USA, some supporting tests on GRs were performed to verify the effectiveness of water quality from the GRs (Moran et al., 2004). However, the findings showed that the level of TN was greater in the runoff from the GR. Furthermore, certain findings demonstrate that organic matter also influences the runoff water quality from GRs and that the organic pollutant levels in the outflow of GRs rely mainly on substrate layer thickness and components, roof slope and vegetation type (Chen, 2022; Zhang et al., 2022). Consequently, it is essential to conduct further studies on local substrate layer thickness components and plants that could enhance the SR quality and for low-cost GR expansion. Environmental conditions affecting the water retention ability are season, rainfall event characteristics and length of the antecedent dry weather period. The effectiveness of GRs is determined by two factors: 1) rainfall amount, which is partially sequestered by a substrate layer and absorbed by vegetation, returned to the atmosphere by evapotranspiration, and 2) runoff after peak flow reduction time, which is affected by rainfall intensity and roof saturation. In light of the aforementioned factors, when the rainfall intensity is low and the roof substrate is dry, there is almost no runoff and the retention rate is therefore 100%. When rainfall is intensive and the substrate is already saturated with water–runoff would be immediate, and the runoff retention rate would be consequently insignificant.
Permeable pavement (PP) systems: also called pervious or porous paving, are a type of paving material that sequester water or allows rainwater to infiltrate through the paving material for groundwater replenishment, runoff time extension, peak shaving, and SR treatment. Proved findings by Kamali et al. (2017) demonstrated that a PP system eliminated 79% and 12% of NH4-N and NO3-N, respectively; Drake et al. (2014) reported that the retention of P from three kinds of PPs: porous concrete, porous asphalt, and permeable interlocking concrete, was more than 75%, and the permeable interlocking concrete pavement demonstrated the highest P elimination efficiency. Furthermore, PPs possess a high achievement in terms of suspended solid and total N and could decrease hydrocarbon pollution by 98.7% (Coupe et al., 2003; NCDENR, 2005). Other scholars found that PPs could sequester more than 80% of sediments, 60% of P, and 80% of N (Melbourne Water, 2016). Several factors that influence the efficacy of PP systems include pavement type and its thickness and the porosity of the underlying bedding material. The application of PPs must be avoided when there is a high risk of clogging by silt loads on the surface. PPs offer attenuation storage and adequately help decrease SR and peak flow rates. To date, very little research has been conducted on enhancing nutrient elimination in PPs. Consequently, it is important to investigate the use of various materials as aggregates or in combination with aggregates in subsequent PP studies.
Concave green land (CGL). CGL, comparable to bioretention and rain gardens, is a major control measure of “Sponge Cities”. It refers to vegetated land that has a lower elevation compared to its surroundings and could enhance SR quality by sequestering contaminants through vegetation and soil. Considering its low investment cost and minimal operational and maintenance costs, researchers now promote the use of CGL as an additional stormwater control measure (Wang et al., 2016). Li et al. (2019) found that at the stormwater return periods of 3, 5, and 10 a, the interception rate and peak reduction effect of the depression green space at two various depths (0.15 and 0.2 m) and various green space rates (20%–45%) can exceeded 99.92%.
Vegetated swales (VSs). VSs are normally linear, planted in wide and shallow depressions (or open channels). In addition to their major role in transporting SR, they could also purify and reduce SR velocity through sedimentation and filtration by plant and vegetation material and infiltration through the soil. Furthermore, under the action of bacteria in the rhizosphere and substrate/soil, contaminants in runoff could be further degraded to decrease the pressure and pollution caused by rainwater in city centers (Li et al., 2019; Zhang, 2019). Plants should be chosen from native species (grasses and herbaceous species) that must be kept at the height of 7.5–15 cm. By encouraging infiltration, VSs aid in slowing down runoff volumes and retard runoff peaks and flow velocity. Several factors impact the efficacy of VSs, mostly encompassing HRT and length of the VS The HRT mostly influences the elimination of particulate contaminants, and the suspended solid elimination rates of 74.4% and 97.2% have been found for HRTs of 0.1 and 0.18 h, respectively (Yu et al., 2019). In addition, Summerfelt (2006) found a mean reduction of 2%–16%, 50%, 60%, and 46%–67%, 75%, 80%, for hydrocarbons, heavy metals, and SS, respectively, for a 30 and 60 m VS. length. In short, the performance of VSs is associated with their size and the intensity of rainfall events. With smaller rainfall events, the VS. normally produces no runoff. However, during intensive rain events, the VS. acts as a transport system that aids in retarding runoff peaks. Various researchers have found runoff volume reductions of 23%–48%.
Rainwater wetlands. Stormwater wetlands (SWs) are structural practices such as wet ponds that encompass wetland hydrophytes in a shallow pool. As SR enters the wetland, nutrient elimination is achieved by the synergistic physical, chemical, and biochemical effects of hydrophytes, sediment/substrates, and aerobic or anaerobic bacteria. While natural wetlands could sometimes be utilized to purify SR that has been correctly pretreated, SWs are basically different from natural wetland systems. SWs are built particularly for purifying SR and commonly have less biodiversity than natural wetlands in terms of both plant and animal life. Alihan et al. (2017) assessed the impact of SWs on controlling initial SR pollution on campus. They found suspended solid, total N, total P, and trace metal elimination rates of 60%–72%, 40%–50%, and 30%–80%, respectively. Additionally; Gill et al. (2017) investigated the impact of SWs on the elimination of trace metals from runoff and found that the enrichment of Cd, Cu, Pb, and Zn in SWs was 0.1 g/(a.m2), 15.6 g/(a.m2), 11.6 g/(a.m2), and 88.3 g/(a.m2), respectively.
Bioretention systems (BSs). Among the types of stormwater control measures reported, BSs are the most common and convenient, encompassing bioretention and infiltration basins. BS has an important role in urban SR control under various conditions because of its adaptability and availability. In BSs, water quality is improved through the principles of hydraulic flow with physical, chemical, and biological processes. BSs could stably eliminate most contaminants from the initial SR. Previous studies reported 46% of nitrate and 42% of dissolved N in research of N morphology in the outlet of a BS (Li and Davis, 2014). The major factors impacting the efficacy of BS encompass the kinds of plants, fillers/substrates, and structure. Sun and Davis (2007) showed that 88%–97% of trace metals were adsorbed by the substrates and 0.5%–3.3% were assimilated by the vegetation. To enhance the purification ability in BSs, organic matter, postwater purification residues, and aluminum sludge could be inserted into media as filler modifiers (Li et al., 2014). Kong et al. (2022) incorporated water purification residues into traditional BSs, and the outlet levels of total P and particulate P were considerably decreased, with the elimination of dissolved P rising by 60%.
In response to urban pluvial flooding and pollution, the Chinese government proposed a “sponge city (SC)” strategy in 2013 that aims to enhance urban stormwater management and promote sustainable urban development. Supplementary Figure S1 illustrates a comparison of different stormwater management measures for SC construction in urban watersheds (Urban Construction Department of the Ministry of Housing and Urban-Rural Development: Beijing, China, 2014). Thus, the SC concept has gained increasing public attention. SC refers to a city with sponge-like features for rainwater. However, up to now, SC construction is still in its exploratory phase. It is still unclear which models can be able to simulate the major processes (infiltration, purification, retention, discharge, and utilization) of SC measures. Its different advantages (socioeconomic and environmental advantages) have not yet been systematically assessed in the context of the SC. Although urban stormwater runoff control strategies have been widely investigated mainly in terms of effectiveness, potential improvements, obstacles, etc., there is still insufficient decisive knowledge concerning the effectiveness of runoff retention and purification. Of the studied stormwater runoff control strategies, GRs are widely investigated in terms of their rainwater retention ability. The major obstacles to expanding and executing urban stormwater runoff control strategies in city areas include lack of financial resources, path dependency, institutional fragmentation, lack of regulations, uncertainty concerning execution process and efficacy, and restricted land and time availability. The most appropriate means in heavily inhabited regions are solutions that could be constructed on top of the roof or to the walls, such as GRs, green walls and rainwater harvesting. These methods do not demand any land from the roads and thus are much simpler to execute.
4 Prospects for the control of NPS pollution
With the huge improvement in scientific research, numerous methods are undoubtedly accessible for NPS pollution control, which can be categorized into four groups (Figure 16B). These strategies have been effectively used in AUWs to manage NPS pollution with different levels of success. As mentioned above, most NPS pollution must be minimized from farmlands before reaching the surface water. Ongoing regional and global diffuse nutrient concerns have increased awareness of the need to identify watersheds and measures that are more vulnerable to diffuse nutrient loss and consequently at higher risk of further damaging water quality further (Figure 16A) (seventh International Nutrient Workshop, Sharpley et al., 2015). Undoubtedly, managing NPS pollution is an integrated matter without a single method to meet such strict demands. Consequently, the complete control of NPS pollution necessitates thoroughly using different control strategies. Since the formation and production of diffuse pollution differ temporospatially based on meteorological (e.g., precipitation) and irrigation events, the purification performance of systems also differs based on flow and pollutant levels. The processing load of control and NPS pollution control strategies must be planned to satisfy the peak processing needs. Given the essence of waterbodies, it is reasonable to apply ecologically sound or green methods. Many ecologically friendly methods provide additional benefits of enhancing water bodies’ aesthetic values while dealing with the proliferation of unsightly algae blooms and eutrophic water bodies. Nevertheless, a suitable NPS pollution control strategy must possess the following traits: a) flexibility for local and site climate conditions; b) ease of management without complicated operating processes or technical requirements; c) minimal investment and operational costs; d) adaptability to variations in water volume and nutrient levels; and e) process capability with full elimination abilities for N, P and organic matter.
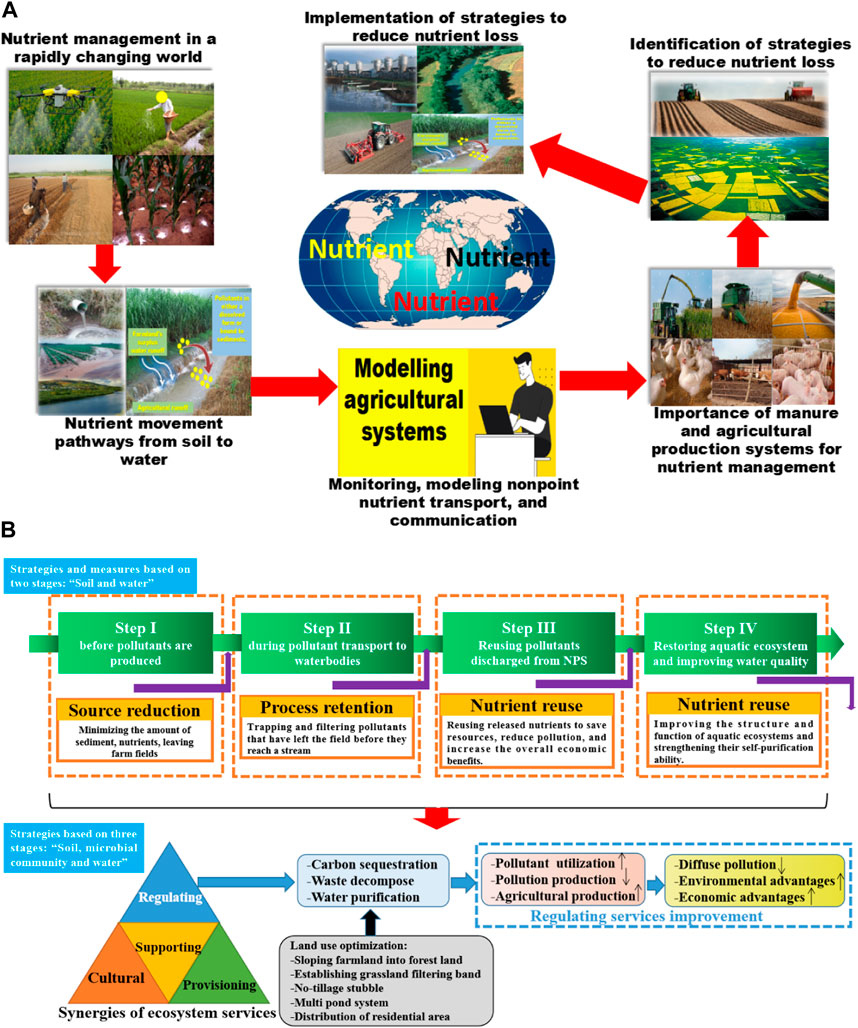
FIGURE 16. Conceptual framework of the (A) challenges and future research needs on agricultural nutrient use and water quality, (B) and ecosystem services synergies in an agroecosystem aimed at diffuse pollution abatement and enhancement of both environmental and economic advantages, based on land use optimization (drawn after the seventh International Nutrient Workshop, Sharpley et al., 2015; Wu et al., 2017).
To date, no current approach can comply with all these requirements. Although chemical and physical approaches have been effective and rapid in treatment, in some particular situations, prioritizing the use of bioeco-based technologies while embracing physicochemical treatments as an additional backup can provide a means to minimize the undesirable effects as well as the secondary pollution originating from their use. Source reduction strategies may minimize both water volume and contamination load via tillage, fertilization management strategies and water-saving irrigation and, thus, could be promoted through legislation. The subsequent processing load could be substantially reduced after the source reduction strategy. Process retention methods, including CWs and ecological drainage ditches, could complement the source reduction method. Although urban stormwater runoff control strategies have been widely investigated mainly in terms of effectiveness, potential improvements, obstacles, etc., there is still insufficient decisive knowledge concerning the effectiveness of runoff retention and purification. Of the studied stormwater runoff control strategies, GRs are widely investigated in terms of their rainwater retention ability. The major obstacles to expanding and executing urban stormwater runoff control strategies in city areas include lack of financial resources, path dependency, institutional fragmentation, lack of regulations, uncertainty concerning execution process and efficacy, and restricted land and time availability. The most appropriate means in heavily inhabited regions are solutions that could be constructed on top of the roof or to the walls, such as GRs, green walls and rainwater harvesting. These methods do not demand any land from the roads and thus are much simpler to execute.
Pollutant purification ability in plant-based water treatment systems is partially determined by the extent of vegetation and microbe action; thus, connecting operational aspects with dynamic biological activities must be investigated. For water containing higher levels of nutrients, it is suggested to utilize hybrid constructed wetlands to overthrow the defects of a single system. The effectiveness of plant-based water treatment systems at cold temperatures is not always encouraging because of the restricted pollutant purification ability. However, the current adjustments provided in the current paper demonstrate that enhancements are feasible, but they have intrinsic benefits and drawbacks. It is suggested that these adjustments, in addition to being executed separately, must be combined or integrated to attain case-specific improved efficiency. Additionally, bioremediation materials must be modified to improve bioremediation methods and their effectiveness in dealing with the problems of eutrophic systems. The bioremediation process must be assessed from different perspectives and hierarchies. The need for adjustments of constructed wetland-based systems lies in the connected deficiencies and preferred enhancement. For instance, a greenhouse structure could prevent water clogging because of bed freezing. Hydrophytes could be utilized as insulation material by deliberately covering the treatment systems. However, some scholars think that breeding cold-resistant hydrophytes by genetic methods is encouraging. Filler/substrate addition or bioaugmentation must be provided where microbes or hydrophytes stop working correctly under cold climates. The processes embraced in many works are complicated and require more studies and groundwork to confirm long-term ability. Other than that, the technical improvement via the process adjustments must also observe the economic feasibility. The cost engagement for adapting a complex or modified constructed wetland system must in coincidence with the employment of other equivalent methods in the field of water purification. In addition, adjustment strategies must also ensure the environmental, technical, and financial sustainability simultaneously.
As mentioned above, an assessment of the previous studies has demonstrated that chemical processes constitute an ecological issue and could cause secondary pollution, such as sludge. On the other hand, regarding mechanical technologies, the elevated cost of execution and the long-term ecological impacts, as reported in the case of water diversion, make these methods unsuccessful in providing a thorough solution to eutrophic water bodies. Bioeco-based technologies are more eco-friendly; however, these approaches require a longer time to offer the desired outcomes. Over the long run, further investigations must be carried out to identify rapid eutrophic water control and resolution by using more biotechnologically developed, cheap, and more possible approaches, which could back up the current rapid yet risky methods, offering a comprehensively greener, safer, more effective, and faster remedy for eutrophic water bodies.
4.1 Approach emerged based on soil-water- and microbiota stage
As stated before, pollutant movement and conversion between soil and water stages is the essence of diffuse pollution creation and generation. Conventionally, most of diffuse pollution control measures are based on two stages (e.g., soil and water). The third stage, the bio-stage made of several microbes including bacteria, microalgae and fungi between the soil and water stages in nature, particularly in shallow freshwater systems. Normally, the bio-stage is largely overlooked in the research about diffuse pollution creation and control, while it plays a considerable role between water and soil since several biochemical reactions engaged in pollutant conversion take place in the bio-stage. Microbes assimilate N and P for their development and sequester pollutants as part of their biomass. After microbes have died and biomass decomposed, N would be released back into soil as organic fertilizer, and the bio-stage could be seen as a short-term sink of pollutants and a slow-release fertilizer. Consequently, nutrient use efficiency is enhanced and pollutant release from cultivated lands is minimized.
The “three-stage” (soil, water and bio-stage) approach is commonly unbiased and closer to fact under field circumstances in comparison to the “two-stage” (water and soil stage). Correspondingly, besides the slow release biofertilizer, several other new methods could be built up based on soil-water- and bio-stages and possess great potential for diffuse pollution control at both sources and processes. Microbiota with elevated pollutant conversion ability could be chosen and grown on artificial fillers/substrates. Following their cultivation, microbiota could be placed in cultivated lands, such as rice paddy fields to enhance nutrient use efficiency and thus decrease fertilizer input. Microbiota with fillers/substrates could also be inserted in macrophyte based-treatment systems to sequester pollutants from eutrophic waters.
4.2 Management based on an approach of regulating services
Ecosystem services refer to the adnatages from all kinds of ecosystems (including agroecosystems) and are categorized into regulating, provisioning, supporting and cultural services (Figure 16B) Regulating services such as waste decomposition, water purification, and carbon storage could be adopted for diffuse pollution control. This will encompass diminution of diffuse pollution generation and sequestering nutrients during their movement process. That is to say, as a complex community consisted of crops, trees, animals, surface water and land, the agroecosystem a huge ability to sequester and consume pollutants itself.
Correspondingly, the measures for diffuse pollution control must be at a regional level, bearing in mind the entire region as an inseparable ecosystem and employing water and nutrient holding functions of the ecosystem. However, frequently utilized strategies including 4R fertilizer stewardship, water saving irrigation, conservation tillage, vegetated ditches, etc., only concentrate on an insignificant portion of the agroecosystem. Consequently, further research must be conducted at the ecosystem scale aim at keeping and enhancing the regulating services of the ecosystem, particularly boosting its water and pollutant sequestering ability with the final goal of minimizing diffuse pollution generation.
Given that the biogeochemical conversion processes of pollutants are strongly associated with soil properties and use, land use/cover types of an agroecosystem contribute significantly to the creation and generation of diffuse pollution. High concentrations of nutrients could lead to eutrophication and degradation of water quality, however, pollutants released from diffuse pollution could be viewed as a kind of resource if they would be adequately recycled/reused in both aquatic and terrestrial ecosystems. To fully use numerous functions of the agroecosystem for diffuse pollution control, proper use patterns must be categorized including changing sloping field land into forest, establishing hedgerows and other field margin vegetation types (e.g., beetle banks, trees, walls, shelterbelts, fences and gates preserved from the major crops within or around the cultivated land, no-tillage stubble, constructing multi-pond systems, and maximizing the distribution of residential zones. This would result in improved nutrient use ability, decrease in water and soil loss and domestic sewage generation, sequestration of pollutants in the ecosystem before getting to nearby streams, and boosted agricultural production (Figure 16B). Eventually, diffuse nutrient production is decreased with enhancements in both environmental and economic advantages, because of the improvement of several ecosystem services synergies.
5 Concluding remarks
Diffuse pollution of nutrients like N and P in agricultural and urban areas has been a challenging issue, the management of which a lot of time and money has been spent globally. Despite these efforts, the water quality in many catchments has not significantly improved. Nevertheless, dealing with a wicked matter, such as the issue of NPS of nutrient pollution of waterbodies, necessitates scholars to develop potential treatment strategies. To the best of the authors’ knowledge, this paper is one of the few that reviews various strategies to control diffuse nutrient pollution, which are generally addressed separately. Treatment plans combining two or more of the available ecotechnological methods are believed to be efficient. Source reduction strategies could reduce both water volumes and nutrient loads via different tillage practices, water-savings and 4R fertilizer stewardship. As such, they could be considered as one option, as part of a broader landscape-based approach to achieve the economic, social, and environmental goals. The subsequent pollution load would be significantly reduced after the source reduction strategy. Process retention strategy control technologies such as ecological ditches, buffer strips used within and around fields, vegetated ponds, and constructed wetlands could supplement source reduction strategies. To date, constructed wetlands have been shown to be an effective approach. However, in the face of frequent environmental degradation and increasingly stringent discharge criteria, artificial wetland systems operating as single approaches would struggle to meet new environmental requirements. Scientists are currently focusing on composite CWs, which could be used as an improved approach to current artificial wetlands. The construction of new CWs is becoming increasingly difficult due to the lack of arable land. In addition, studies in full-scale artificial wetlands and long-term studies are limited. Field studies in full-scale environments should also be carried out to improve the technology of artificial wetlands. Nutrients generated from diffuse pollution could be viewed as a resource if recycled and reused in a suitable manner in the agricultural and urban ecosystems. Further research and development may focus on a more detailed approach that integrates microbiota at the interface between soil and water to holistically develop innovative approaches for diffuse pollution control.
Author contributions
MNK conceptualized this review paper. MNK contributed to perform the literature search and data analysis and drafted the article. BZ, AS, FA, MD, ES, TW, MA, DK, and TDA contributed to reviewing and editing the manuscript. All authors contributed to the article and approved the submitted version.
Funding
This study was supported by the National Natural Science Foundation (NSFC) Project for International Young Scientists (42050410314), the China Postdoctoral Science Foundation (2020M670482), the Chinese Academy of Sciences President’s International Fellowship Initiative (PIFI) (2019PC0097), and the funding support for foreign experts through Foreign Young Talents Program of the Ministry of Science and Technology of China.
Conflict of interest
The authors declare that the research was conducted in the absence of any commercial or financial relationships that could be construed as a potential conflict of interest.
Publisher’s note
All claims expressed in this article are solely those of the authors and do not necessarily represent those of their affiliated organizations, or those of the publisher, the editors and the reviewers. Any product that may be evaluated in this article, or claim that may be made by its manufacturer, is not guaranteed or endorsed by the publisher.
Supplementary material
The Supplementary Material for this article can be found online at: https://www.frontiersin.org/articles/10.3389/fenvs.2023.1199923/full#supplementary-material
References
Abass, K., Buor, D., Afriyie, K., Dumedah, G., Segbefi, A. Y., Guodaar, L., et al. (2020). Urban sprawl and green space depletion: Implications for flood incidence in Kumasi, Ghana. Disaster Risk Reduct. 51, 101915. doi:10.1016/j.ijdrr.2020.101915
Abdel-Shafy, H. I., El-Khateeb, M. A., and Shehata, M. (2017). Blackwater treatment via combination of sedimentation tank and hybrid wetlands for unrestricted reuse in Egypt. Water Treat. 71, 145–151. doi:10.5004/dwt.2017.20538
Abu-Zreig, M., Ruda, R. P., Whiteley, H. R., Lalonde, M. N., and Kaushik, N. K. (2003). Phosphorus removal in vegetated filter strips. J. Environ. Qual. 32, 613–619. doi:10.2134/jeq2003.6130
Addo-Bankas, O., Zhao, Y., Gomes, A., and Stefanakis, A. I. (2022). Challenges of urban artificial landscape water bodies: Treatment techniques and restoration strategies towards ecosystem services enhancement. Processes 10 (12), 2486. doi:10.3390/pr10122486
Adhikary, P. P., Hombegowda, H. C., Barman, D., Jakhar, P., and Madhu, M. (2017). Soil erosion control and carbon sequestration in shifting cultivated degraded highlands of eastern India: Performance of two contour hedgerow systems. Agrofor. Syst. 91, 757–771. doi:10.1007/s10457-016-9958-3
Afzal, M., Rehman, K., Shabir, G., Tahseen, R., Ijaz, A., Hashmat, A. J., et al. (2019). Largescale remediation of oil-contaminated water using floating treatment wetlands. Clean. Water. Nat. 2 (1), 3. doi:10.1038/s41545-018-0025-7
Ajibade, F. O., Nwogwu, N. A., Lasisi, K. H., Ajibade, T. F., Adelodun, B., Guadie, A., et al. (2021b). “Removal of nitrogen oxyanion (nitrate) in constructed wetlands,” in Progress and prospects in the management of oxoanion polluted aqua systems. Editors N. A. Oladoja, and I. E. Unuabonah (Netherlands: The Netherlands, Springer Nature). doi:10.1007/978-3-030-70757-6
Ajibade, F. O., Wang, H., Guadie, A. A., Ajibade, T. F., Fang, Y., Sharif, H. M. A., et al. (2021a). Total nitrogen removal in biochar amended non-aerated vertical flow constructed wetlands for secondary wastewater effluent with low C/N ratio: Microbial community structure and dissolved organic carbon release conditions. Bioresour. Technol. 322, 124430. doi:10.1016/j.biortech.2020.124430
Ajibade, F. O., Yin, W., Guadie, A., Ajibade, T. F., Liu, Y., Kumwimba, M. N., et al. (2023). Impact of biochar amendment on antibiotic removal and resistant genes accumulation in microscale constructed wetlands for low C/N artificial wastewater treatment: Performance and mechanism. J. Chem. Eng. 459, 141541. doi:10.1016/j.cej.2023.141541
Alihan, J. C., Maniquiz-Redillas, M., Choi, J., Flores, P. E., and Kim, L.-H. (2017). Characteristics and fate of stormwater runoff pollutants in constructed wetlands. J. Wetl. Res. 19 (1), 37–44. doi:10.17663/jwr.2017.19.1.037
Alwahaibi, B., Jafary, T., Al-Mamun, A., Baawain, M. S., Aghbashio, M., Tabatabaei, M., et al. (2021). Operational modifications of a full-scale experimental vertical flow constructed wetland with effluent recirculation to optimize total nitrogen removal. J. Clean. Prod. 296, 126558. doi:10.1016/j.jclepro.2021.126558
Akinbile, C. O., Yusoff, M. S., and Zuki, A. A. (2012). Landfill leachate treatment using subsurface flow constructed wetland by Cyperus haspan. Waste Manag 32 (7), 1387–1393.
Anawar, Md.H., and Chowdhury, R. (2020). Remediation of polluted river water by biological, chemical, ecological and engineering processes. Sustainability 12, 7017. doi:10.3390/su12177017
Antoniadis, A., Takavakoglou, V., Zalidis, G., and Poulios, I. (2007). Development and evaluation of an alternative method for municipal wastewater treatment using homogeneous photocatalysis and constructed wetlands. Catal. Today 124 (3–4), 260–265. doi:10.1016/j.cattod.2007.03.044
Arana, J., Cabo, C. G. I., Rodriguez, C. F., Melian, J. A. H., Mendez, J. A. O., Rodriguez, J. M. D., et al. (2008). Combining TiO2-photocatalysis and wetland reactors for the efficient treatment of pesticides. Chemosphere 71 (4), 788–794. doi:10.1016/j.chemosphere.2007.10.008
Arif, M., Qi, Y., Dong, Z., and Wei, H. (2022). Rapid retrieval of cadmium and lead content from urban greenbelt zones using hyperspectral characteristic bands. J. Clean. Prod. 374, 133922. doi:10.1016/j.jclepro.2022.133922
Avila, C., García, J., and Garfí, M. (2016). Influence of hydraulic loading rate, simulated storm events and seasonality on the treatment performance of an experimental three stage hybrid constructed wetland system. Ecol. Eng. 87, 324–332. doi:10.1016/j.ecoleng.2015.11.042
Awad, Y. M., Blagodatskaya, E., Ok, Y. S., and Kuzyakov, Y. (2012). Effects of polyacrylamide, biopolymer, and biochar on decomposition of soil organic matter and plant residues as determined by 14c and enzyme activities. Eur. J. Soil Biol. 48, 1–10. doi:10.1016/j.ejsobi.2011.09.005
AyazAktas¸, S. Ç. O., Akça, L., Fındık, N., and Fındık, N. (2015). Effluent quality and reuse potential of domestic wastewater treated in a pilot-scale hybrid constructed wetland system. J. Environ. Manag. 156, 115–120. doi:10.1016/j.jenvman.2015.03.042
Aziz, O., Hussain, S., Rizwan, M., Riaz, M., Bashir, S., Lin, L., et al. (2018). Increasing water productivity, nitrogen economy, and grain yield of rice by water saving irrigation and fertilizer-N management. Environ. Sci. Pollut. Res. 25, 16601–16615. doi:10.1007/s11356-018-1855-z
Bakhshoodeh, R., Alavi, N., and Paydary, P. (2017). Composting plant leachate treatment by a pilot-scale, three-stage, horizontal flow constructed wetland in central Iran. Environ. Sci. Pollut. Res. 24, 23803–23814. doi:10.1007/s11356-017-0002-6
Barden, C., Mankin, K. R., Ngandu, D. M., Geyer, W. A., Devlin, D. L., and McVay, K. (2003). “Assessing the effectiveness of various riparian buffer vegetation types. SRL-137,” in Kansas state university agricultural experimental station and cooperative extension service (Manhattan, Kansas. http://www.oz-net.ksu.edu/library/forst2/SRL137.pdf (Accessed May 2, 2023).
Barlow, K., Nash, D., Turral, H., and Grayson, R. (2003). Phosphorus uptake and release in surface drains. Agric. Water Manag. 63, 109–123. doi:10.1016/s0378-3774(03)00177-x
Baulch, H. M., Elliott, J. A., Cordeiro, M. R. C., Flaten, D. N., Lobb, D. A., and Wilson, H. F. (2019). Soil and water management: Opportunities to mitigate nutrient losses to surface waters in the northern great Plains. Environ. Rev. 27, 447–477. doi:10.1139/er-2018-0101
Berndtsson, J. C., Emilsson, T., and Bengtsson, L. (2006). The influence of extensive vegetated roofs on runoff water quality. Sci. Total Environ. 355, 48–63. doi:10.1016/j.scitotenv.2005.02.035
Bowmer, K. H., Bales, M., and Roberts, J. (1994). Potential use of irrigation drains as wetlands. Water Sci. Technol. 29, 151–158. doi:10.2166/wst.1994.0179
Brix, H. (1995). “Treatment wetlands: An overview,” in Proceedings conference on constructed wetlands for wastewater treatment. Editors I. Toczylowska, and M. Mierzejewski (Poland: Technical University of Gdansk), 167–176.
Bulc, T. G., Klemencic, A. K., and Razinger, J. (2011). Vegetated ditches for treatment of surface water with highly fluctuating water regime. Water Sci. Technol. 63 (10), 2353–2359. doi:10.2166/wst.2011.588
Cai, S., Shi, H., Pan, X., Liu, F., Cui, Y., and Xie, H. (2017). Integrating ecological restoration of agricultural non-point source pollution in poyang lake basin in China. Water 9, 745. doi:10.3390/w9100745
Calheiros, C. S. C., and Stefanakis, A. I. (2021). Green roofs towards circular and resilient cities. Circ. Econ. Sustain. 1, 395–411. doi:10.1007/s43615-021-00033-0
Cao, W., Arif, M., Cui, Z., and Li, C. (2023). Effects of different trace elements on ecophysiological characteristics of ligustrum obtusifolium saplings. Forests 14 (5), 972. doi:10.3390/f14050972
Carpenter, S. R. (2008). Phosphorus control is critical to mitigating eutrophication. Proc. Natl. Acad. Sci. USA. 105 (32), 11039–11040. doi:10.1073/pnas.0806112105
Cassman, K. G., Dobermann, A., and Walters, D. T. (2002). Agroecosystems, nitrogen-use efficiency, and nitrogen management. Ambio 31, 132–140. doi:10.1579/0044-7447-31.2.132
Castaldelli, G., Aschonitis, V., Vincenzi, F., Fano, E. A., and Soana, E. (2018). The effect of water velocity on nitrate removal in vegetated waterways. J. Environ. Manage 215, 230–238. doi:10.1016/j.jenvman.2018.03.071
Castaldelli, G., Soana, E., Racchetti, E., Vincenzi, F., Fano, E. A., and Bartoli, M. (2015). Vegetated canals mitigate nitrogen surplus in agricultural watersheds. Agric. Ecosyst. Environ. 212, 253–262. doi:10.1016/j.agee.2015.07.009
Chen, P. Y. (2022). Effects of meteorological variables and substrate moisture on evapotranspiration and thermal performance of a green roof in a subtropical climate. Ecol. Eng. 180, 106663. doi:10.1016/j.ecoleng.2022.106663
Chen, Z., Cuervo, D. P., Müller, J. A., Wiessner, A., Koser, H., Vymazal, J., et al. (2016). Hydroponic root mats for wastewater treatment-a review. Environ. Sci. Pollut. Res. 23, 15911–15928. doi:10.1007/s11356-016-6801-3
Chiemchaisri, W., Junsod, J., Threedeach, S., and Wicranarachchi, P. N. (2009). Leachate treatment and greenhouse gas emission in subsurface horizontal flow constructed wetland. Bioresour. Technol. 100 (16), 3808–3814.
Chislock, M. F., Doster, E., Zitomer, R. A., and Wilson, A. E. (2013). Eutrophication: Causes, consequences, and controls in aquatic ecosystems. Nat. Educ. Knowl. 4 (4), 10 Available at: https://www.nature.com/scitable/knowledge/library/eutrophication-causes-consequences-and-controls-in-aquatic-102364466/.
Clausen, J. C., Jokela, W. E., Potter, F. I., and Williams, J. W. (1996). Paired watershed comparison of tillage effects on runoff, sediment, and pesticide losses. J. Environ. Qual. 25, 1000–1007. doi:10.2134/jeq1996.00472425002500050011x
Colares, G. S., Dell’Osbel, N., Wiesel, P. G., Oliveira, G. A., Lemos, P. H. Z., da Silva, F. P., et al. (2020). Floating treatment wetlands: A review and bibliometric analysis. Sci. Total Environ. 714, 136776. doi:10.1016/j.scitotenv.2020.136776
Cooper, C. M., Moore, M. T., Bennett, E. R., Smith, S., Farris, J. L., Milam, C. D., et al. (2004). Innovative uses of vegetated drainage ditches for reducing agricultural runoff. Water Sci. Technol. 49, 117–123. doi:10.2166/wst.2004.0176
Cordell, D., Drangert, J. O., and White, S. (2009). The story of phosphorus: Global food security and food for thought. Glob. Environ. Change 19, 292–305. doi:10.1016/j.gloenvcha.2008.10.009
Coupe, S. J., Smith, H. G., Newman, A. P., and Puehmeier, T. (2003). Biodegradation and microbial diversity within permeable pavements. Eur. J. Protistol. 39 (4), 495–498. doi:10.1078/0932-4739-00027
Dal Ferro, N., Ibrahim, H. M. S., and Borin, M. (2018). Newly-established free water-surface constructed wetland to treat agricultural waters in the low-lying Venetian plain: performance on nitrogen and phosphorus removal. Sci. Total Environ. 639, 852–859.
Daryanto, S., Wang, L., and Jacinthe, P. A. (2017). Meta-analysis of phosphorus loss from no-till soils. J. Environ. Qual. 46, 1028–1037. doi:10.2134/jeq2017.03.0121
Daverede, I. C., Kravchenko, A. N., Hoeft, R. G., Nafziger, E. D., Bullock, D. G., Warren, J. J., et al. (2003). Phosphorus runoff: Impact of tillage and soil phosphorus levels. J. Environ. Qual. 32, 1436–1444. doi:10.2134/jeq2003.1436
Diaz, R. J., and Rosenberg, R. (2008). Spreading dead zones and consequences for marine ecosystems. Science 321 (5891), 926–929. doi:10.1126/science.1156401
Dodd, R. J., and Sharpley, A. N. (2016). Conservation practice effectiveness and adoption: Unintended consequences and implications for sustainable phosphorus management. Nutr. Cycl. Agroecosyst. 104, 373–392. doi:10.1007/s10705-015-9748-8
Dollinger, J., Dagès, C., Bailly, J. S., Lagacherie, P., and Voltz, M. (2015). Managing ditches for agroecological engineering of landscape: A review. Agron. Sustain. Dev. 35, 999–1020. doi:10.1007/s13593-015-0301-6
Drake, J., Bradford, A., and Seters, T. V. (2014). Stormwater quality of spring–summer-fall effluent from three partial-infiltration permeable pavement systems and conventional asphalt pavement. J. Environ. Manag. 139, 69–79. doi:10.1016/j.jenvman.2013.11.056
Du, L., Trinh, X., Chen, Q., Wang, C., Wang, H., Xia, X., et al. (2018). Enhancement of microbial nitrogen removal pathway by vegetation in integrated vertical-flow constructed wetlands (IVCWs) for treating reclaimed water. Bioresour. Technol. 249, 644–651. doi:10.1016/j.biortech.2017.10.074
Eagle, A. J., Bird, J. A., Horwath, W. R., Linquist, B. A., Brouder, S. M., Hill, J. E., et al. (2000). Rice yield and nitrogen utilization efficiency under alternative straw management practices. Agron. J. 92 (6), 1096–1103. doi:10.2134/agronj2000.9261096x
El-Khateeb, M. A., Al-Herrawy, A. Z., Kamel, M. M., and El-Gohary, F. A. (2009). Use of wetlands as post-treatment of anaerobically treated effluent. Desalination 245, 50–59. doi:10.1016/j.desal.2008.01.071
El-Khateeb, M. A., and El-Bahrawy, A. Z. (2013). Extensive post treatment using constructed wetland. Life Sci. J. 10, 560–568. doi:10.1016/j.desal.2008.01.071
El-Sheikh, M. A., Saleh, H. I., El-Quosy, D. E., and Mahmoud, A. A. (2010). Improving water quality in polluated drains with free water surface constructed wetlands. Ecol. Eng. 36, 1478–1484.
Environmental Working Group (2018). New EWG database details $30 billion spent on U.S. Farm conservation programs. https://www.ewg.org/release/new-ewg-database-details-30-billion-spent-us-farmconservationprograms (Accessed April 9, 2018).
FAOSTAT (2021). Faostat. http://www.fao.org/faostat/en/#data/RFN (Accessed January 15, 2021).
Fernandez-Fernandez, M. I., de la Vega, P. T. M., Jaramillo-Moran, M. A., and Garrido, M. (2020). Hybrid constructed wetland to improve organic matter and nutrient removal. Water 12, 2023. doi:10.3390/w12072023
Flora, C., and Kröger, R. (2014). Use of vegetated drainage ditches and low grade weirs for aquaculture effluent mitigation: I nutrients. Aquacult. Eng. 60, 56–62. doi:10.1016/j.aquaeng.2014.04.006
Fu, L., Wei, L., Ma, M., and Ma, M. (2018). Biological floating bed and bio-contact oxidation processes for landscape water treatment: Simultaneous removal of Microcystis aeruginosa, TOC, nitrogen and phosphorus. Environ. Sci. Pollut. Res. 25, 24220–24229. doi:10.1007/s11356-018-2417-0
Gaballah, M. S., Ismail, K., Aboagye, D., Ismail, M. M., Sobhi, M., and Stefanakis, A. I. (2021). Effect of design and operational parameters on nutrients and heavy metal removal in pilot floating treatment wetlands with Eichhornia Crassipes treating polluted lake water. Environ. Sci. Pollut. Res. 28, 25664–25678. doi:10.1007/s11356-021-12442-7
Galloway, J. N., Townsend, A. R., Erisman, J. W., Bekunda, M., Cai, Z., Freney, J. R., et al. (2008). Transformation of the nitrogen cycle: Recent trends, questions, and potential solutions. Science 320 (5878), 889–892. doi:10.1126/science.1136674
Gao, D. W., and Hu, Q. (2012). Bio-contact oxidation and greenhouse-structured wetland system for rural sewage recycling in cold regions: A full-scale study. Ecol. Eng. 49, 249–253. doi:10.1016/j.ecoleng.2012.08.012
Geng, R., and Sharpley, A. N. (2019). A novel spatial optimization model for achieve the trad-offs placement of best management practices for agricultural non-point source pollution control at multi-spatial scales. J. Clean. Pro. 234, 1023–1032. doi:10.1016/j.jclepro.2019.06.277
Gholipour, A., and Stefanakis, A. I. (2021). A full-scale anaerobic baffled reactor and hybrid constructed wetland for University dormitory wastewater treatment and reuse in an arid and warm climate. Ecol. Eng. 170, 106360. doi:10.1016/j.ecoleng.2021.106360
Gikas, G. D., and Tsihrintzis, V. A. (2012). A small-size vertical flow constructed wetland for on-site treatment of household wastewater. Eng. 44, 337–343. doi:10.1016/j.ecoleng.2012.04.016
Gill, L. W., Ring, P., Casey, B., Higgins, N. M. P., and Johnston, P. M. (2017). Long term heavy metal removal by a constructed wetland treating rainfall runoff from a motorway. Sci. Total Environ. 601–602, 32–44. doi:10.1016/j.scitotenv.2017.05.182
Gomes, A. C., Silva, L., Albuquerque, A., Simões, R., and Stefanakis, A. I. (2020). Treatment of cork boiling wastewater using a horizontal subsurface flow constructed wetland combined with ozonation. Chemosphere 260, 127598. doi:10.1016/j.chemosphere.2020.127598
Grant, C. A., and Flaten, D. N. (2019). 4R management of phosphorus fertilizer in the Northern Great Plains. J. Environ. Qual. 48, 1356–1369. doi:10.2134/jeq2019.02.0061
Gruber, N., and Galloway, J. N. (2008). An earth-system perspective of the global nitrogen cycle. Nature 451 (7176), 293–296. doi:10.1038/nature06592
Gunes, K., Tuncsiper, B., Ayaz, S., and Drizo, A. (2012). The ability of free water surface constructed wetland system to treat high strength domestic wastewater: a case study for the Mediterranean. Ecol. Eng. 44 (2), 278–284.
Gupta, S., Srivastava, P., and Yadav, A. K. (2020). Simultaneous removal of organic matters and nutrients from high-strength wastewater in constructed wetlands followed by entrapped algal systems. Environ. Sci. Pollut. Res. 27, 1112–1117. doi:10.1007/s11356-019-06896-z
Haddaway, N. R., Brown, C., Eales, J., Eggers, S., Josefsson, J., Kronvang, B., et al. (2018). The multifunctional roles of vegetated strips around and within agricultural fields. Environ. Evid. 7, 14. doi:10.1186/s13750-018-0126-2
Ham, J. H., Yoon, C. G., Hwang, S. J., and Jung, K. W. (2004). Seasonal performance of constructed wetland and winter storage pond for sewage treatment in Korea. J. Environ. Sci. Heal., A 39 (5), 1329–1343. doi:10.1081/ese-120030335
Hao, Z., Xue, Y. G., Wang, Z. Q., Yang, J. C., and Zhang, J. H. (2009). An alternate wetting and moderate soil drying regime improves root and shoot growth in rice. Crop Sci. 49, 2246–2260. doi:10.2135/cropsci2009.02.0099
Hashemi, S. S., Mahmud, H. B., and Ashraf, M. A. (2015). Performance of green roofs with respect to water quality and reduction of energy consumption in tropics: A review. Renew. Sustain. Energy Rev. 52, 669–679. doi:10.1016/j.rser.2015.07.163
He, X., Zhao, X., Zhang, W., Ren, B., and Zhao, Y. (2022). Developing a novel alum sludge-based floating treatment wetland for natural water restoration. Water 14, 2433. doi:10.3390/w14152433
He, Y., Zhou, X., Zhang, Q., Gu, J. D., Zhang, Y., Liu, Y., et al. (2021b). Highly efficient removal of phosphorus from agricultural runoff by a new akadama clay barrier-vegetated drainage ditch system (VDD) and its mechanism. J. Environ. Manag. 290, 112575. doi:10.1016/j.jenvman.2021.112575
Henny, C., Jasalesmana, T., Kurniawan, R., Melati, I., Suryono, T., Susanti, E., et al. (2020). “The effectiveness of integrated floating treatment wetlands (FTWs) and lake fountain aeration systems (LFAS) in improving the landscape ecology and water quality of a eutrophic lake in Indonesia,” in IOP conference series: Earth and environmental science (Bristol, UK: IOP Publishing), 012018.
Herrera-Melian, J. A., Martin-Rodriguez, A. J., Ortega-Mendez, A., Arana, J., DonaRodriguez, J. M., and Perez-Pena, J. (2012). Degradation and detoxification of 4-nitrophenol by advanced oxidation technologies and bench-scale constructed wetlands. J. Environ. Manage. 105, 53–60. doi:10.1016/j.jenvman.2012.03.044
Howarth, R. W., Sharpley, A., and Walker, D. (2002). Sources of nutrient pollution to coastal waters in the United States: Implications for achieving coastal water quality goals. Estuaries 25 (4), 656–676. doi:10.1007/bf02804898
Hu, Z., Lee, J. W., Chandran, K., Kim, S., and Khanal, S. K. (2012). Nitrous oxide (N2O) emission from aquaculture: A review. Environ. Sci. Technol. 46 (12), 6470–6480. doi:10.1021/es300110x
Huang, F., Huang, Y., Jia, J., Li, Z., Xu, J., Ni, S., et al. (2022). Research and engineering application of bypass combined artificial wetlands system to improve river water quality. J. Water Process Eng. 48, 102905. doi:10.1016/j.jwpe.2022.102905
Huang, J., Xu, C., Ridoutt, B. G., Wang, X., and Ren, P. (2017). Nitrogen and phosphorus losses and eutrophication potential associated with fertilizer application to cropland in China. J. Clean. Prod. 159, 171–179. doi:10.1016/j.jclepro.2017.05.008
Hussein, A., and Scholz, M. (2017). Dye wastewater treatment by vertical-flow constructed wetlands. Ecol. Eng. 101, 28–38.
Ioannidou, V., and Stefanakis, A. I. (2020). “The use of constructed wetlands to mitigate pollution from agricultural runoff,” In Contaminants in Agriculture. Springer, Cham 233–246.
Irfan, S. A., Razali, R., KuShaari, K., Mansor, N., Azeem, B., and Ford Versypt, A. N. (2018). A review of mathematical modeling and simulation of controlled-release fertilizers. J. Control Release 271, 45–54. doi:10.1016/j.jconrel.2017.12.017
Jamshidi, S., Akbarzadeh, A., Woo, K. S., and Valipour, A. (2014). Wastewater treatment using integrated anaerobic baffled reactor and Bio-rack wetland planted with Phragmites sp. and Typha sp. J. Environ. Health. Sci. Eng. 12, 131. doi:10.1186/s40201-014-0131-5
Ji, B., Zhao, Y., Vymazal, J., Qiao, S., Wei, T., Li, J., et al. (2020). Can subsurface flow constructed wetlands be applied in cold climate regions? A review of the current knowledge. Ecol. Eng. 157, 105992. doi:10.1016/j.ecoleng.2020.105992
Ji, M., Hu, Z., Hou, C., Liu, H., Ngo, H. H., Guo, W., et al. (2020). New insights for enhancing the performance of constructed wetlands at low temperatures. Bioresour. Technol. 24, 122722–123601. doi:10.1016/j.biortech.2019.122722
Jia, Z., Tang, S., Luo, W., and Hai, Y. (2016). Water quality improvement through five constructed serial wetland cells and its implications on nonpoint-source pollution control. Hydrol. Sci. J. 61 (16), 2946–2956. doi:10.1080/02626667.2016.1171323
Jian, L., and Wu, Y. (2021). Nitrogen removal by different riparian vegetation buffer strips with different stand densities and widths. Water Supply 21, 3541–3556. doi:10.2166/ws.2021.119
Kamali, M., Delkash, M., and Tajrishy, M. (2017). Evaluation of permeable pavement responses to urban surface runoff. J. Environ. Manag. 187, 43–53. doi:10.1016/j.jenvman.2016.11.027
Kieta, K. A., Owens, P. N., Lobb, D. A., Vanrobaeys, J. A., and Flaten, D. N. (2018). Phosphorus dynamics in vegetated buffer strips in cold climates: A review. Environ. Rev. 26, 255–272. doi:10.1139/er-2017-0077
Kong, Z., Ma, H. Y., Song, Y. Q., Wang, X. Y., Li, L. Q., Yuan, Y. S., et al. (2022). A long term study elucidates the relationship between media amendment and pollutant treatment in the stormwater bioretention system: Stability or efficiency? Water Res. 225, 119124. doi:10.1016/j.watres.2022.119124
Kotti, I. P., Gikas, G. D., and Tsihrintzis, V. A. (2010). Effect of operational and design parameters on removal efficiency of pilot-scale FWS constructed wetlands and comparison with HSF systems. Ecol. Eng. 36 (7), 862–875.
Kröger, R., Holland, M. M., Moore, M. T., and Cooper, C. M. (2008). Agricultural drainage ditches mitigate phosphorus loads as a function of hydrological variability. J. Environ. Qual. 37, 107–113. doi:10.2134/jeq2006.0505
Kröger, R., Holland, M. M., Moore, M. T., and Cooper, C. M. (2007). Hydrological variability and agricultural drainage ditch inorganic nitrogen reduction capacity. J. Environ. Qual. 36, 1646–1652. doi:10.2134/jeq2006.0506
Kujala, K., Karlsson, T., Nieminen, S., and Ronkanen, A. K. (2019). Design parameters for nitrogen removal by constructed wetlands treating mine waters and municipal wastewater under Nordic conditions. Sci. Total Environ. 662, 559–570. doi:10.1016/j.scitotenv.2019.01.124
Kumwimba, M. N., Batool, A., and Li, X. (2020). How to enhance the purification performance of traditional floating treatment wetlands (FTWs) at low temperatures: Strengthening strategies. Sci. Total Environ. 766, 142608. doi:10.1016/j.scitotenv.2020.142608
Kumwimba, M. N., Dong, Z., Zhu, B., Tang, J., and Wang, T. (2017a). Assessing nutrient, biomass and sediment transport of drainage ditches in the Three Gorges Reservoir area. Clean. - Soil Air Water 45, 1863–0669. doi:10.1002/clen.201501012
Kumwimba, M. N., Dzakpasu, M., and Li, X. (2020b). Potential of invasive watermilfoil (Myriophyllum spp) to remediate eutrophic waterbodies with organic and inorganic pollutants. J. Environ. Manag. 270, 110919. doi:10.1016/j.jenvman.2020.110919
Kumwimba, M. N., Dzakpasu, M., Xinzhu, L., Wang, W., Jie, Z., Bao, L., et al. (2021). Sequential detention pond-biogeochemical barrier-free water surface wetland system for effluent purification and river eutrophication control. J. Water Proc. Eng. 41, 102075. doi:10.1016/j.jwpe.2021.102075
Kumwimba, M. N., Dzakpasu, M., Zhu, B., Muyembe, D. K., Ilunga, L., and Kavidia Muyembe, D. (2017b). Nutrient removal in a trapezoidal vegetated drainage ditch used to treat primary domestic sewage in a small catchment of the upper Yangtze River. Water Environ. J. 31, 72–79. doi:10.1111/wej.12225
Kumwimba, M. N., Huang, J. L., Dzakpasu, M., Ajibade, F. O., Li, X. Y., Edmond, S., et al. (2023a). Enhanced nutrient removal in agro-industrial wastes-amended hybrid floating treatment wetlands treating real sewage: Laboratory microcosms to field-scale studies. Chemosphere 330, 138703. doi:10.1016/j.chemosphere.2023.138703
Kumwimba, M. N., Huang, J. L., Dzakpasu, M., Koshila, D. S., Ohore, O. E., Ajibade, F. O., et al. (2023b). An updated review of the efficacy of buffer zones in warm/temperate and cold climates: Insights into processes and drivers of nutrient retention. J. Environ. Manage 336, 117646. doi:10.1016/j.jenvman.2023.117646
Kumwimba, M. N., Li, X. Y., Huang, J. L., Muyembe, D. K., Dzakpasu, M., and Sanganyado, E. (2022). Performance of various fillers in ecological floating beds planted with Myriophyllum aquaticum treating municipal wastewater. Sci. Total Environ. 842, 156827. doi:10.1016/j.scitotenv.2022.156827
Kumwimba, M. N., and Meng, F. (2019). Roles of ammonia-oxidizing bacteria in improving metabolism and cometabolism of trace organic chemicals in biological wastewater treatment processes: A review. Sci. Total Environ. 659, 419–441. doi:10.1016/j.scitotenv.2018.12.236
Kumwimba, M. N., Meng, F., Iseyemi, O., Moore, M. T., Zhu, B., Tao, W., et al. (2018). Removal of non-point source pollutants from domestic sewage and agricultural runoff by vegetated drainage ditches (VDDs): Design, mechanism, management strategies, and future directions. Sci. Total Environ. 639, 742–759. doi:10.1016/j.scitotenv.2018.05.184
Kumwimba, M. N., Zhu, B., and Muyembe, D. K. (2017c). Estimation of the removal efficiency of heavy metals and nutrients from ecological drainage ditches treating town sewage during dry and wet seasons. Environ. Monit. Assess. 189, 434. doi:10.1007/s10661-017-6136-7
Kumwimba, M. N., Zhu, B., Muyembe, D. K., and Dzakpasu, M. (2017d). Growth characteristics and nutrient removal capability of eco-ditch plants in mesocosm sediment receiving primary domestic wastewater. Environ. Sci. Pollut. Res. 24 (30), 23926–23938. doi:10.1007/s11356-017-9992-3
Kumwimba, M. N., Zhu, B., Suanon, F., Muyembe, D. K., and Dzakpasu, M. (2017e). Long-term impact of primary domestic sewage on metal/loid accumulation in drainage ditch sediments, plants and water: Implications for phytoremediation and restoration. Sci. Total Environ. 581–582, 773–781. doi:10.1016/j.scitotenv.2017.01.007
Kumwimba, M. N., Zhu, B., Wang, T., Dzakpasu, M., and Li, X. (2020a). Nutrient dynamics and retention in a vegetated drainage ditch receiving nutrient-rich sewage at low temperatures. Sci. Total Environ. 741, 140268. doi:10.1016/j.scitotenv.2020.140268
Kun, L. I. (2012). Effects of controlled release fertilizer on loss of nitrogen and phosphorus from farmland. J. Anhui Agr. Sci. 40, 12466–12470. Available at: https://caod.oriprobe.com/articles/30652111/Effects_of_Controlled_Release_Fertilizer_on_Loss_of_Nitrogen_and_Phosp.htm
Lacas, J. G., Voltz, M., Gouy, V., Carluer, N., and Gril, J. J. (2005). Using grassed strips to limit pesticide transfer to surface water: A review. Agron. Sustain. Dev. 25, 253–266. doi:10.1051/agro:2005001
Lassaletta, L., Billen, G., Grizzetti, B., Anglade, J., and Garnier, J. (2014). 50 year trends in nitrogen use efficiency of world cropping systems: The relationship between yield and nitrogen input to cropland. Environ. Res. Lett. 9 (10), 105011. doi:10.1088/1748-9326/9/10/105011
Lee, C. H., Wang, C. C., Lin, H. H., Lee, S. S., Tsang, D. C. W., Jien, S. H., et al. (2018). In-situ biochar application conserves nutrients while simultaneously mitigating runoff and erosion of an Fe-oxide-enriched tropical soil. Sci. Total Environ. 619-620, 665–671. doi:10.1016/j.scitotenv.2017.11.023
Lee, K., Isenhart, T., and Schultz, R. (2003). Sediment and nutrient removal in an established multi-species riparian buffer. Soil Water Conserv. 58, 1–8. Available at: https://www.jswconline.org/content/58/1/1
Lee, S. S., Shah, H. S., Awad, Y. M., Kumar, S., and Ok, Y. S. (2015). Synergy effects of biochar and polyacrylamide on plants growth and soil erosion control. Environ. Earth Sci. 74, 2463–2473. doi:10.1007/s12665-015-4262-5
Li, C., Liu, M., Hu, Y., Gong, J., Sun, F., and Xu, Y. (2014). Characterization and first flush analysis in road and roof runoff in Shenyang, China. Water Sci. Technol. 70, 397–406. doi:10.2166/wst.2014.203
Li, G. M., Zhang, X. Q., and Sun, Y. (2019). Regulating effect of concave green land on surface runoff. J. Water Eng. 30 (2), 31–36.
Li, L., and Davis, A. P. (2014). Urban stormwater runoff nitrogen composition and fate in bioretention systems. Environ. Sci. Technol. 48 (6), 3403–3410. doi:10.1021/es4055302
Li, T., Jin, Y., and Huang, Y. (2022). Water quality improvement performance of two urban constructed water quality treatment wetland engineering landscaping in Hangzhou, China. Water Sci. Technol. 85, 1454–1469. doi:10.2166/wst.2022.063
Liang, X. Q., Chen, Y. X., Nie, Z. Y., Ye, Y. S., Liu, J., Tian, G. M., et al. (2013). Mitigation of nutrient losses via surface runoff from rice cropping systems with alternate wetting and drying irrigation and site-specific nutrient management practices. Environ. Sci. Pollut. Res. 20 (10), 6980–6991. doi:10.1007/s11356-012-1391-1
Liang, X., Wang, Z., Zhang, Y., Zhu, C., Lin, L., and Xu, L. (2016). No-tillage effects on N and P exports across a rice-planted watershed. Environ. Sci. Pollut. Res. 23, 8598–8609. doi:10.1007/s11356-016-6112-8
Liao, M., Zhaojin, Y. E., Huang, Y., Ting, L., Shen, J., and Zhang, Y. (2017). Influence of different fertilization management modes on phosphorus loss in run-off from nursery land in the catchment area of Hexi reservoir in Changxing county. Acta Ecol. Sin. 37, 7342–7350. Available at: https://www.ecologica.cn/stxb/article/abstract/stxb201608211712 doi:10.5846/stxb201608211712
Lin, Y. F., Jing, S. R., and Lee, D. Y. (2003). The potential use of constructed wetlands in a recirculating aquaculture system for shrimp culture. Environ. Pollut. 123 (1), 107–113. doi:10.1016/s0269-7491(02)00338-x
Lintern, A., McPhillips, L., Winfrey, B., Duncan, J., and Grady, C. (2020). Best management practices for diffuse nutrient pollution: Wicked problems across urban and agricultural watersheds. Environ. Sci. Technol. 54 (15), 9159–9174. doi:10.1021/acs.est.9b07511
Lintern, A., Webb, J. A., Ryu, D., Liu, S., Waters, D., Leahy, P., et al. (2018). What are the key catchment characteristics affecting spatial differences in riverine water quality? Water Resour. Res. 54 (10), 7252–7272. doi:10.1029/2017wr022172
Liu, B., and Zhao, T. (2011). Research and application of comprehensive prevention and control technology for agricultural non-point source pollution. Beijing: China Agricultural Science and Technology Press.
Liu, H., Wang, Z., Yu, R., Li, F., Li, K., Cao, H., et al. (2016). Optimal nitrogen input for higher efficiency and lower environmental impacts of winter wheat production in China. Agric. Ecosyst. Environ. 224, 1–11. doi:10.1016/j.agee.2016.03.022
Liu, J., Fu, B., Wang, Y., Xu, P., Zhou, G., and Chen, X. (2016a). Sleep disordered breathing symptoms and daytime sleepiness are associated with emotional problems and poor school performance in children. CSAE 32 (24), 218–225. doi:10.1016/j.psychres.2016.05.017
Liu, J., Wang, F., Liu, W., Tang, C., Wu, C., and Wu, Y. (2016c). Nutrient removal by up-scaling a hybrid floating treatment bed (HFTB) using plant and periphyton: From laboratory tank to polluted river. Bioresour. Technol. 207 (0), 142–149. doi:10.1016/j.biortech.2016.02.011
Liu, K., Elliott, J. A., Lobb, D. A., Flaten, D. N., and Yarotski, J. (2014). Conversion of conservation tillage to rotational tillage to reduce phosphorus losses during snowmelt runoff in the Canadian Prairies. J. Environ. Qual. 43, 1679–1689. doi:10.2134/jeq2013.09.0365
Long, H., and Liu, Y. (2016). Rural restructuring in China. J. Rural. Stud. 47, 387–391. doi:10.1016/j.jrurstud.2016.07.028
Long, T., Wellen, C., Arhonditsis, G., and Boyd, D. (2014). Evaluation of stormwater and snowmelt inputs, land use and seasonality on nutrient dynamics in the watersheds of Hamilton harbour, ontario, Canada. J. Gt. Lakes. Res. 40 (4), 964–979. doi:10.1016/j.jglr.2014.09.017
Łopata, M., Augustyniak, R., Grochowska, J., Parszuto, K., Tandyrak, R., and Wi´sniewski, G. (2020). Behavior of aluminum compounds in soft-water lakes subjected to experimental reclamation with polyaluminum chloride. Water Air Soil Pollut. 231, 358. doi:10.1007/s11270-020-04708-6
Maine, M. A., Sanchez, G. C., Hadad, H. R., Caffaratti, S. E., Pedro, M. C., Mufarrege, M. M., et al. (2019). Hybrid constructed wetlands for the treatment of wastewater from a fertilizer manufacturing plant: Microcosms and field scale experiments. Sci. Total Environ. 650, 297–302. doi:10.1016/j.scitotenv.2018.09.044
Mallin, M. A., and McIver, M. R. (2012). Pollutant impacts to cape hatteras national seashore from urban runoff and septic leachate. Mar. Pollut. Bull. 64 (7), 1356–1366. doi:10.1016/j.marpolbul.2012.04.025
Matsuo, N., and Mochizuki, T. (2009). Growth and yield of six rice cultivars under three water-saving cultivations. Plant Prod. Sci. 12, 514–525. doi:10.1626/pps.12.514
Mbuligwe, S. E. (2004). Comparative effectiveness of engineered wetland systems in the treatment of anaerobically pre-treated domestic wastewater. Ecol. Eng. 23, 269–284. doi:10.1016/j.ecoleng.2004.09.009
Mehnaz, K. R., Keitel, C., and Dijkstra, F. A. (2019). Phosphorus availability and plants alter soil nitrogen retention and loss. Sci. Total Environ. 671, 786–794. doi:10.1016/j.scitotenv.2019.03.422
Meier, S., Curaqueo, G., Khan, N., Bolan, N., Cea, M., Eugenia, G. M., et al. (2017). Chicken-manure-derived biochar reduced bioavailability of copper in a contaminated soil. J. Soils Sediments 17, 741–750. doi:10.1007/s11368-015-1256-6
Melbourne Water (2016). Melbourne water. https://www.melbournewater.com.au/planing-and-building/stormwater-management/wsud_treatments/pages/porous-paving.aspx (Accessed May 20, 2016).
Metcalfe, C., Guppy, L., and Qadir, M. (2017). Global barriers to improving water quality: A critical review; UNU-inweh report; 02; UNUinweh. Hamilton: Canada.
Meuleman, A. F. M., and Beltman, B. (1993). The use of vegetated ditches for water quality improvement. Hydrobiologia 253, 375. doi:10.1007/bf00050767
Moore, M. T., and Kröger, R. (2010a). Evaluating plant species-specific contributions to nutrient mitigation in drainage ditch mesocosms. Water Air Soil Pollut. 217, 445–454. doi:10.1007/s11270-010-0599-2
Moran, A., Hunt, B., and Jennings, G. (2004). “A North Carolina field study to evaluate green roof runoff quantity, runoff quality, and plant growth,” in Book section, 1–10.
NCDENR (2005). “Updated draft manual of storm water best management practices,” in Public consultation document doc–, 7–1. Division of water quality (NC, USA: North Carolina Department of Environment and Natural Resources).
Nguyen, X. C., Chang, S. W., Nguyen, T. L., Ngo, H. H., Kumar, G., Banu, J. R., et al. (2018). A hybrid constructed wetland for organic-material and nutrient removal from sewage: Process performance and multi-kinetic models. J. Environ. Manag. 222, 378–384. doi:10.1016/j.jenvman.2018.05.085
Ogata, Y., Ishigaki, T., Ebie, Y., Sutthasil, N., Chiemchaisri, C., and Yamada, M. (2015). Water reduction by constructed wetlands treating waste landfill leachate in a tropical region. Waste Manage 44, 164–171.
Olguín, E. J., Sánchez-Galván, G., Melo, F. J., Hernández, V. J., and González-Portela, R. E. (2017). Long-term assessment at field scale of floating treatment wetlands for improvement of water quality and provision of ecosystem services in a eutrophic urban pond. Sci. Total Environ. 584–585, 561–571. doi:10.1016/j.scitotenv.2017.01.072
Ongley, E. D., Xiaolan, Z., and Tao, Y. (2010). Current status of agricultural and rural non-point source pollution assessment in China. Environ. Pollut. 158 (5), 1159–1168. doi:10.1016/j.envpol.2009.10.047
Oral, H. V., Radinja, M., Rizzo, A., Kearney, K., Andersen, T. R., Krzeminski, P., et al. (2021). Management of urban waters with nature-based solutions in circular cities - exemplified through seven urban circularity challenges. Water 13 (23), 3334. doi:10.3390/w13233334
Osmond, D. L., Shober, A. L., Sharpley, A. N., Duncan, E. W., and Hoag, D. L. K. (2019). Increasing the effectiveness and adoption of agricultural phosphorus management strategies to minimize water quality impairment. J. Environ. Qual. 48, 1204–1217. doi:10.2134/jeq2019.03.0114
Otto, S., Pappalardo, S. E., Cardinali, A., Masin, R., Zanin, G., and Borin, M. (2016). Vegetated ditches for the mitigation of pesticides runoff in the Po Valley. PLoS One 11 (4), e0153287. doi:10.1371/journal.pone.0153287
Pierobon, E., Castaldelli, G., Mantovani, S., Vincenzi, F., and Anna Fano, E. (2013). Nitrogen removal in vegetated and unvegetated drainage ditches impacted by diffuse and point sources of pollution. Clean. Soil Air Water 41, 24–31. doi:10.1002/clen.201100106
Pinardi, M., Soana, E., Bresciani, M., Villa, P., and Bartoli, M. (2020). Upscaling nitrogen removal processes in fluvial wetlands and irrigation canals in a patchy agricultural watershed. Wetl. Ecol. Manag. 28 (2), 297–313. doi:10.1007/s11273-020-09714-3
Plaza-Bonilla, D., Cantero-Martínez, C., Viñas, P., and Álvaro-Fuentes, J. (2013). Soil aggregation and organic carbon protection in a no-tillage chronosequence under Mediterranean conditions. Geoderma 193-194, 76–82. doi:10.1016/j.geoderma.2012.10.022
Prenarathna, K. S. D., Rajapaksha, A. U., Sarkar, B., Kwon, E. E., Bhatnagar, A., Ok, Y. S., et al. (2019). Biochar-based engineered composites for sorptive decontamination of water: A review. Chem. Eng. J. 372, 536–550. doi:10.1016/j.cej.2019.04.097
Prosser, R. S., Hoekstra, P. F., Gene, S., Truman, C., White, M., and Hanson, M. L. (2020). A review of the effectiveness of vegetated buffers to mitigate pesticide and nutrient transport into surface waters from agricultural areas. J. Environ. Manag. 261, 110210. doi:10.1016/j.jenvman.2020.110210
Qian, J., Qu, K., Tian, B., and Zhang, Y. (2021). Water treatment of polluted rivers in cities based on biological filter technology. Environ. Technol. Innov. 23, 101544. doi:10.1016/j.eti.2021.101544
Racchetti, E., Bartoli, M., Soana, E., Longhi, D., Christian, R. R., Pinardi, M., et al. (2011). Influence of hydrological connectivity of riverine wetlands on nitrogen removal via denitrification. Biogeochemistry 103, 335–354. doi:10.1007/s10533-010-9477-7
Rasul, G. (2016). Managing the food, water, and energy nexus for achieving the sustainable development goals in south Asia. Environ. Dev. 18, 14–25. doi:10.1016/j.envdev.2015.12.001
Reyes, N., Geronimo, F., Guerra, H., and Kim, L. (2023). Bibliometric analysis and comprehensive review of stormwater treatment wetlands: Global research trends and existing knowledge gaps. Sustainability 15, 2332. doi:10.3390/su15032332
Ribaudo, M. O., Heimlich, R., Claassen, R., and Peters, M. (2001). Least-cost management of nonpoint source pollution: Source reduction versus interception strategies for controlling nitrogen loss in the Mississippi basin. Ecol. Econ. 37 (2), 183–197. doi:10.1016/s0921-8009(00)00273-1
Rigotti, J. A., Paqualini, J. P., and Rodrigues, L. R. (2021). Root growth and nutrient removal of Typha domingensis and Schoenoplectus californicus over the period of plant establishment in a constructed floating wetland. Environ. Sci. Pollut. Res. 28, 8927–8935. doi:10.1007/s11356-020-11681-4
Rissman, A. R., and Carpenter, S. R. (2015). Progress on nonpoint pollution: Barriers &opportunities. Daedalus 144 (3), 35–47. doi:10.1162/daed_a_00340
Saeed, T., Haque, I., and Khan, T. (2019). Organic matter and nutrients removal in hybrid constructed wetlands: Influence of saturation. Chem. Eng. J. 371, 154–165. doi:10.1016/j.cej.2019.04.030
Satchithanantham, S., English, B., and Wilson, H. (2019). Seasonality of phosphorus and nitrate retention in riparian buffers. J. Environ. Qual. 48, 915–921. doi:10.2134/jeq2018.07.0280
Schierano, M. C., Panigatti, M. C., Maine, M. A., Griffa, C. A., and Boglione, R. (2020). Horizontal subsurface flow constructed wetland for tertiary treatment of dairy wastewater: Removal efficiencies and plant uptake. J. Environ. Manag. 272, 111094. doi:10.1016/j.jenvman.2020.111094
Schmidt, J. H., Junge, S., and Finckh, M. R. (2019). Cover crops and compost prevent weed seed bank buildup in herbicide-free wheat-potato rotations under conservation tillage. Ecol. Evol. 9, 2715–2724. doi:10.1002/ece3.4942
Scholz, M., and Trepel, M. (2004). Water quality characteristics of vegetated groundwater-fed ditches in a riparian peatland. Sci. Total Environ. 332, 109–122. doi:10.1016/j.scitotenv.2004.02.001
Selman, M., Greenhalgh, S., Diaz, R., and Sugg, Z. (2008). Eutrophication and hypoxia in coastal areas: A global assessment of the state of knowledge. Washington, D.C: World Resources Institute, 6.
Šereš, M., Hnátková, T., Vymazal, J., and Vaněk, T. (2017). Removal efficiency of constructed wetland for treatment of agricultural wastewaters. Chem. J. Mold. 12 (1), 45–52. doi:10.19261/cjm.2017.403
Sharma, P., Pandey, A. K., Udayan, A., and Kumar, S. (2021). Role of microbial community and metal-binding proteins in phytoremediation of heavy metals from industrial wastewater. Bioresour. Technol. 326, 124750. doi:10.1016/j.biortech.2021.124750
Sharpley, A. N., Bergström, L., Aronsson, H., Bechmann, M., Bolster, C. H., Börling, K., et al. (2015). Future agriculture with minimized phosphorus losses to waters: Research needs and direction. Ambio 44 (2), 163–179. doi:10.1007/s13280-014-0612-x
Sim, C. H., Quek, B. S., Shutes, R. B., and Goh, K. H. (2013). Management and treatment of landfill leachate by a system of constructed wetlands and ponds in Singapore. Water Sci. Technol 68 (5), 1114–1122.
Simon, M., and Joshi, H. (2021). A review on green technologies for the rejuvenation of polluted surface water bodies: Field-scale feasibility, challenges, and future perspectives. J. Environ. Chem. Eng. 9, 105763. doi:10.1016/j.jece.2021.105763
Singh, S., Haberl, R., Moog, O., Shrestha, R. R., Shrestha, P., and Shrestha, R. (2009). Performance of an anaerobic baffled reactor and hybrid constructed wetland treating high strength wastewater in Nepal-a model for DEWATS. Ecol. Eng. 35, 654–660. doi:10.1016/j.ecoleng.2008.10.019
Smith, V. H. (2003). Eutrophication of freshwater and coastal marine ecosystems a global problem. Environ. Sci. Pollut. Res. 10, 126–139. doi:10.1065/espr2002.12.142
Soana, E., Balestrini, R., Vincenzi, F., Bartoli, M., and Castaldelli, G. (2017). Mitigation of nitrogen pollution in vegetated ditches fed by nitrate-rich spring waters. Agric. Ecosyst. Environ. 243, 74–82. doi:10.1016/j.agee.2017.04.004
Soana, E., Bartoli, M., Milardi, M., Fano, E. A., and Castaldelli, G. (2019). An ounce of prevention is worth a pound of cure: Managing macrophytes for nitrate mitigation in irrigated agricultural watersheds. Sci. Total Environ. 647, 301–312. doi:10.1016/j.scitotenv.2018.07.385
Soana, E., Gavioli, A., Tamburini, E., Fano, E. A., and Castaldelli, G. (2018). To mow or not to mow: Reed biofilms as denitrification hotspots in drainage canals. Ecol. Eng. 113, 1–10. doi:10.1016/j.ecoleng.2017.12.029
Soana, E., Gavioli, A., Vincenzi, F., Fano, E. A., and Castaldelli, G. (2020). Nitrate availability affects denitrification in Phragmites australis sediments. J. Environ. Qual. 49 (1), 194–209. doi:10.1002/jeq2.20000
Song, G. H., Huang, C. J., An, J., Shen, P., Zhang, X. J., Chen, J., et al. (2018). Treatment of rural domestic wastewater using multi-soillayering systems: Performance evaluation, factorial analysis and numerical modeling. Sci. Total Environ. 644, 536–546. doi:10.1016/j.scitotenv.2018.06.331
Stefanakis, A. I. (2020). Constructed wetlands for sustainable wastewater treatment in hot and arid climates: Opportunities, challenges and case studies in the Middle East. Water 12 (6), 1665. doi:10.3390/w12061665
Stefanakis, A. I., Akratos, C. S., and Tsihrintzis, V. A. (2014). Vertical flow constructed wetlands: Eco-engineering systems for wastewater and sludge treatment. first ed. Amsterdam, Netherlands: Elsevier Publishing.
Summerfelt, S. T. (2006). Design and management of conventional fluidized-sand biofilters. Aquacult. Eng. 34 (3), 275–302. doi:10.1016/j.aquaeng.2005.08.010
Sun, B., Zhang, L., Yang, L., Zhang, F., Norse, D., and Zhu, Z. (2012). Agricultural non-point source pollution in China: Causes and mitigation measures. Ambio 41 (4), 370–379. doi:10.1007/s13280-012-0249-6
Sun, L., Liu, Y., and Jin, H. (2009). Nitrogen removal from polluted river by enhanced floating bed grown canna. Ecol. Eng. 35, 135–140. doi:10.1016/j.ecoleng.2008.09.016
Sun, X., and Davis, A. P. (2007). Heavy metal fates in laboratory bioretention systems. Chemosphere 66 (9), 1601–1609. doi:10.1016/j.chemosphere.2006.08.013
Sun, Y. Q., Chen, S. S., Lau, A. Y. T., Tsang, D. C. W., Mohanty, S. K., Bhatnagar, A., et al. (2020). Waste-derived compost and biochar amendments for stormwater treatment in bioretention column: Cotransport of metals and colloids. J. Hazard Mat. 383, 121243. doi:10.1016/j.jhazmat.2019.121243
Tan, D., Jiang, L., Tan, S., Zheng, F., Xu, Y., Cui, R., et al. (2013). An in situ study of inorganic nitrogen flow under different fertilization treatments on a wheat–maize rotation system surrounding Nansi Lake, China. Agr. Water manage. 123, 45–54. doi:10.1016/j.agwat.2013.03.011
Teemusk, A., and Mander, Ü. (2009). Green roof potential to reduce temperature fluctuations of a roof membrane: A case study from Estonia. Build. Environ. 44, 643–650. doi:10.1016/j.buildenv.2008.05.011
Tharp, R., Westhelle, K., and Hurley, S. (2019). Macrophyte performance in floating treatment wetlands on a suburban stormwater pond: Implications for cold climate conditions. Ecol. Eng. 136, 152–159. doi:10.1016/j.ecoleng.2019.06.011
Tiessen, K. H., Elliott, J. A., Yarotski, J., Lobb, D. A., Flaten, D. N., and Glozier, N. E. (2010). Conventional and conservation tillage: Influence on seasonal runoff, sediment, and nutrient losses in the Canadian prairies. J. Environ. Qual. 39, 964–980. doi:10.2134/jeq2009.0219
Toet, S., Logtestijn, R. S. P. V., Kampf, R., Schreijer, M., and Verhoeven, J. T. A. (2005). The effect of hydraulic retention time on the removal of pollutants from sewage treatment plant effluent in a surface-flow wetland system. Wetlands 25, 375–391. doi:10.1672/13
Uusitalo, R., Lemola, R., and Turtola, E. (2018). Surface and subsurface phosphorus discharge from a clay soil in a nine-year study comparing no-till and plowing. J. Environ. Qual. 47, 1478–1486. doi:10.2134/jeq2018.06.0242
Valenca, R., Le, H., Zu, Y., Dittrich, T. M., Tsang, D. C. W., Datta, R., et al. (2021). Nitrate removal uncertainty in stormwater control measures: Is the design or climate a culprit? Water Res. 190, 116781. doi:10.1016/j.watres.2020.116781
Valkama, P., Mäkinen, E., Ojala, A., Vahtera, H., Lahti, K., Rantakokko, K., et al. (2017). Seasonal variation in nutrient removal efficiency of a boreal wetland detected by high-frequency on-line monitoring. Ecol. Eng. 98, 307–317. doi:10.1016/j.ecoleng.2016.10.071
Vanrobaeys, J. A., Owens, P. N., Lobb, D. A., Kieta, K. A., and Campbell, J. M. (2019). Seasonal efficacy of vegetated filter strips for phosphorus reduction in surface runoff. J. Environ. Qual. 48, 880–888. doi:10.2134/jeq2018.12.0452
Varma, M., Gupta, A. K., Ghosal, P. S., and Majumder, A. (2021). A review on performance of constructed wetlands in tropical and cold climate: Insights of mechanism, role of influencing factors, and system modification in low temperature. Sci. Total environ., 755. Part 2, 142540. doi:10.1016/j.scitotenv.2020.142540
Vera, L., Martel, G., and Marquez, M. (2013). Two years monitoring of the natural system for wastewater reclamation in Santa Lucía, Gran Canaria Island. Ecol. Eng. 50, 21–30. doi:10.1016/j.ecoleng.2012.08.001
Vymazal, J. (2007). Removal of nutrients in various types of constructed wetlands. Sci. Total Environ. 380, 48–65. doi:10.1016/j.scitotenv.2006.09.014
Vymazal, J., and Březinová, T. D. (2018). Removal of nutrients, organics and suspended solids in vegetated agricultural drainage ditch. Ecol. Eng. 118, 97–103. doi:10.1016/j.ecoleng.2018.04.013
Walsh, C. J., Roy, A. H., Feminella, J. W., Cottingham, P. D., Groffman, P. M., and Morgan, R. P. (2005). The urban stream syndrome: Current knowledge and the search for a cure. J. North Am. Benthol. Soc. 24 (3), 706–723. doi:10.1899/04-028.1
Wang, G. B., Wang, Y., and Zhang, Y. (2018). Combination effect of sponge iron and calcium nitrate on severely eutrophic urban landscape water: An integrated study from laboratory to fields. Environ. Sci. Pollut. Res. 25, 8350–8363. doi:10.1007/s11356-017-1161-1
Wang, J., Zhang, P., Yang, L., and Huang, T. (2016). Cadmium removal from urban stormwater runoff via bioretention technology and effluent risk assessment for discharge to surface water. J. Contam. Hydrol. 185–186, 42–50. doi:10.1016/j.jconhyd.2016.01.002
Wang, T., Zhu, B., and Kuang, F. (2012). Reducing interflow nitrogen loss from hillslope cropland in a purple soil hilly region in southwestern China. Nutr. Cycl. Agroecosyst. 93, 285–295. doi:10.1007/s10705-012-9515-z
Wang, T., Zhu, B., and Zhou, M. H. (2019). Ecological ditch system for nutrient removal of rural domestic sewage in the hilly area of the central Sichuan Basin, China. China. J. Hydrol. 570, 839–849. doi:10.1016/j.jhydrol.2019.01.034
Wang, W. H., Wang, Y., Sun, L. Q., Zheng, Y. C., and Zhao, J. C. (2020). Research and application status of ecological floating bed in eutrophic landscape water restoration. Sci. Total Environ. 704, 135434. doi:10.1016/j.scitotenv.2019.135434
Wang, W. H., Wang, Y., Wei, H. S., Wang, L. P., and Peng, J. (2020a). Stability and purification efficiency of composite ecological floating bed with suspended inorganic functional filler in a field study. J. Water Process. Eng. 37, 101482. doi:10.1016/j.jwpe.2020.101482
Wang, X., Burns, D. A., Yanai, R. D., Briggs, R. D., and Germain, R. H. (2006). Changes in stream chemistry and nutrient export following a partial harvest in the catskill mountains, New York, USA. For. Ecol. Manage. 223 (1-3), 103–112. doi:10.1016/j.foreco.2005.10.060
Won, C., Shin, M., Lee, S., Park, Y., Lee, Y., Shin, Y., et al. (2016). NPS pollution reduction from Alpine fields using surface cover material and soil amendments. Irrig. Drain. 65, 193–199. doi:10.1002/ird.2068
Wu, B., Zhang, G., and Zhang, S. (2016). Fate and implication of acetylacetone in photochemical processes for water treatment. Water Res. 101, 233–240. doi:10.1016/j.watres.2016.05.083
Wu, H., Hao, B., Zhou, Q., Xu, K., Cai, Y., and Liu, G. (2021). Contribution of various categories of environmental factors to sediment nitrogen-removal in a low C/N ratio river. Ecol. Eng. 159, 106121. doi:10.1016/j.ecoleng.2020.106121
Wu, H. M., Wang, R. G., Yan, P. H., Wu, S. B., Chen, Z. B., Zhao, Y. Q., et al. (2023). Constructed wetlands for pollution control. Earth Environ. 4, 218–234. doi:10.1038/s43017-023-00395-z
Wu, M., Tang, X., Li, Q., Yang, W., Jin, F., Tang, M., et al. (2013). Review of ecological engineering solutions for rural non-point source water pollution control in Hubei Province, China. Water Air Soil Pollut. 224 (5), 1561. doi:10.1007/s11270-013-1561-x
Wu, S., Kuschk, P., Brix, H., Vymazal, J., and Dong, R. (2014). Development of constructed wetlands in performance intensifications for wastewater treatment: A nitrogen and organic matter targeted review. Water Res. 57, 40–55. doi:10.1016/j.watres.2014.03.020
Wu, Y., Hu, Z., and Yang, L. (2011a). Strategies for controlling agricultural non-point source pollution: Reduce-retain-restoration (3R) theory and its practice. Trans. CSAE 27 (5), 1–6. Available at: https://www.ingentaconnect.com/content/tcsae/tcsae/2011/00000027/00000005/art00001#
Wu, Y., Hu, Z., Yang, L., Graham, B., and Kerr, P. G. (2011b). The removal of nutrients from nonpoint source wastewater by a hybrid bioreactor. Bioresour. Technol. 102 (3), 2419–2426. doi:10.1016/j.biortech.2010.10.113
Wu, Y., Liu, J., Shen, R., and Fu, B. (2017). Mitigation of nonpoint source pollution in rural areas: From control to synergies of multi ecosystem services. Sci. Total Environ. 607-608, 1376–1380. doi:10.1016/j.scitotenv.2017.07.105
Xia, L., Hoermann, G., Ma, L., and Yang, L. (2013). Reducing nitrogen and phosphorus losses from arable slope land with contour hedgerows and perennial alfalfa mulching in Three Gorges Area, China. Catena 110, 86–94. doi:10.1016/j.catena.2013.05.009
Xiao, Q., Liu, Z., Hu, Z., Wang, W., Zhang, M., Xiao, W., et al. (2021). Notable changes of carbon dioxide in a eutrophic lake caused by water diversion. J. Hydrol. 603, 127064. doi:10.1016/j.jhydrol.2021.127064
Xu, Y., He, T., Li, Z., Ye, Q., Chen, Y., Xie, E., et al. (2017). Nitrogen removal characteristics of Pseudomonas putida Y-9 capable of heterotrophic nitrification and aerobic denitrification at low temperature. Biomed. Res. Int. 7, 1–7. doi:10.1155/2017/1429018
Xu, Y., Li, H., Li, Y., Zheng, X., Zhang, C., Gao, Y., et al. (2022). Systematically assess the advancing and limiting factors of using the multi-soillayering system for treating rural sewage in China: From the economic, social, and environmental perspectives. J. Environ. Manag. 312, 114912. doi:10.1016/j.jenvman.2022.114912
Xue, L., Hou, P., Zhang, Z., Shen, M., Liu, F., and Yang, L. (2020). Application of systematic strategy for agricultural non-point source pollution control in Yangtze River basin, China. Agric. Ecosyst. Environ. 304, 107148. doi:10.1016/j.agee.2020.107148
Xue, L., Yu, Y., and Yang, L. (2014). Maintaining yields and reducing nitrogen loss in ricewheat rotation system in Taihu Lake region with proper fertilizer management. Environ. Res. Lett. 9, 115010. doi:10.1088/1748-9326/9/11/115010
Yang, H., Wang, J., Li, J., Zhou, H., and Liu, Z. (2021). Modelling impacts of water diversion on water quality in an urban artificial lake. Environ. Pollut. 276, 116694. doi:10.1016/j.envpol.2021.116694
Yang, J., Huang, D., Duan, H., Tan, G., and Zhang, J. (2009). Alternate wetting and moderate soil drying increases grain yield and reduces cadmium accumulation in rice grains. J. Sci. Food Agric. 89 (10), 1728–1736. doi:10.1002/jsfa.3648
Yang, J., Luo, Y., Dai, Y., Yang, B., Wang, J., and Zhang, X. (2019). “Effect of plastic carbon fiber-biofilm technology on landscape water purification,” in IOP conf. Ser. Earth environ. Sci. (Bristol, UK: IOP Publishing), 052127.
Yang, L. Z., Feng, Y. F., Shi, W. M., Xue, L. H., Wang, S. Q., Song, X. F., et al. (2013). Review of the advances and development trends in agricultural non-point source pollution control in China. Chin. J. Eco-Agr. 21 (1), 96–101. doi:10.3724/SP.J.1011.2013.00096
Yang, L. Z., Shi, W. M., and Xue, L. H. (2013a). Reduce-retain-reuse-restore technology for the controlling the agricultural non-point source pollution in countryside in China: General countermeasures and technologies. J. Agro-Environ. Sci. 1, 1–8.
Yang, Y., Zhao, Q., Cui, Y., Wang, Y., Xie, S., and Liu, Y. (2016). Spatio-temporal variation of sediment methanotrophic microorganisms in a large eutrophic lake. Microb. Ecol. 71, 9–17. doi:10.1007/s00248-015-0667-7
Yang, Y., Zhao, Y., Liu, R., and Morgan, D. (2018). Global development of various emerged substrates utilized in constructed wetlands. Bioresour. Technol. 261, 441–452. doi:10.1016/j.biortech.2018.03.085
Yao, Y., Zhang, M., Tian, Y., Zhao, M., Zhang, B., Zeng, K., et al. (2018). Urea deep placement in combination with Azolla for reducing nitrogen loss and improving fertilizer nitrogen recovery in rice field. Field Crop Res. 218, 141–149. doi:10.1016/j.fcr.2018.01.015
Ye, C., Li, L., Zhang, J., and Yang, Y. (2012). Study on ABR stage-constructed wetland integrated system in treatment of rural sewage. Procedia Environ. Sci. 12, 687–692. doi:10.1016/j.proenv.2012.01.335
Ye, Z. J., Liao, M., Huang, Y., Ting, L., Shen, J., and Zhang, Y. (2016). Influence of different management mode of fertilization on nitrogen losses in runoff from nursery land in a catchment area. J. Soil Water Conserv. 30, 30–37.
Yu, C., Huang, X., Chen, H., Godfray, H. C. J., Wright, J. S., Hall, J. W., et al. (2019). Managing nitrogen to restore water quality in China. Nature 567 (7749), 516–520. doi:10.1038/s41586-019-1001-1
Zeng, S. C., Su, Z. Y., Chen, B. G., Wu, Q. T., and Ouyang, Y. (2008). Nitrogen and phosphorus runoff losses from orchard soils in South China as affected by fertilization depths and rates. Pedosphere 18, 45–53. doi:10.1016/s1002-0160(07)60101-5
Zhai, X., Zhang, Y., Wang, X., Xia, J., and Liang, T. (2014). Non-point source pollution modelling using soil and water assessment tool and its parameter sensitivity analysis in Xin'anjiang catchment, China. Hydrol. Process. 28 (4), 1627–1640. doi:10.1002/hyp.9688
Zhang, C. (2019). Control of rainfall runoff and its pollution by typical grassed swale. Journal of Huazhong University of Science and Technology. Master.
Zhang, J., Gilbert, D., Gooday, A. J., Levin, L., Naqvi, S. W. A., Middelburg, J. J., et al. (2010). Natural and human-induced hypoxia and consequences for coastal areas: Synthesis and future development. Biogeosciences 7 (5), 1443–1467. doi:10.5194/bg-7-1443-2010
Zhang, K. X., Garg, A., Mei, G. X., Jiang, M. J., Wang, H., Huang, S., et al. (2022). Thermal performance and energy consumption analysis of eight types of extensive green roofs in subtropical monsoon climate. Build. Environ. 216, 108982. doi:10.1016/j.buildenv.2022.108982
Zhang, L., Zhao, J., Cui, N., Dai, Y., Kong, L., Wu, J., et al. (2016). Enhancing the water purification efficiency of a floating treatment wetland using a biofilm carrier. Environ. Sci. Pollut. Res. 23, 7437–7443. doi:10.1007/s11356-015-5873-9
Zhang, S., Liu, F., Huang, Z., Xiao, R., Zhu, H., and Wu, J. (2020). Are vegetated drainage ditches effective for nitrogen removal under cold temperatures? Bioresour. Technol. 301, 122744. doi:10.1016/j.biortech.2020.122744
Zhang, S., Liu, F., Xiao, R., Li, Y., He, Y., and Wu, J. (2016a). Effects of vegetation on ammonium removal and nitrous oxide emissions from pilot-scale drainage ditches. Aquat. Bot. 130, 37–44. S. Zhang, et al. Bioresour. Technol., 301 (2020) 122744. doi:10.1016/j.aquabot.2016.01.003
Zhang, S., Xiao, R., Liu, F., Zhou, J., Li, H., and Wu, J. (2016b). Effect of vegetation on nitrogen removal and ammonia volatilization from wetland microcosms. Ecol. Eng. 97, 363–369. doi:10.1016/j.ecoleng.2016.10.021
Zhao, X., Zhou, Y., Min, J., Wang, S., Shi, W., and Xing, G. (2012). Nitrogen runoff dominates water nitrogen pollution from rice-wheat rotation in the Taihu Lake region of China. Agr. Ecosyst. Environ. 156, 1–11. doi:10.1016/j.agee.2012.04.024
Zhao, Z., Song, X., Wang, Y., Wang, D., Wang, S., He, Y., et al. (2016). Effects of algal ponds on vertical flow constructed wetlands under different sewage application techniques. Ecol. Eng. 93, 120–128. doi:10.1016/j.ecoleng.2016.05.033
Zhong, L., Ding, J., Wu, T., ZhaoPang, Y. L. J. W., Jiang, J. P., Jiang, J. Q., et al. (2023). Bibliometric overview of research progress, challenges, and prospects of rural domestic sewage: Treatment techniques, resource recovery, and ecological risk. J. Water Process. Eng. 51, 103389. doi:10.1016/j.jwpe.2022.103389
Keywords: nonpoint source pollution, agricultural and urban watersheds, reduce-retain-reuse-restore approach, modification strategies, nitrogen and phosphorus
Citation: Nsenga Kumwimba M, Zhu B, Stefanakis AI, Ajibade FO, Dzakpasu M, Soana E, Wang T, Arif M, Kavidia Muyembe D and Agboola TD (2023) Advances in ecotechnological methods for diffuse nutrient pollution control: wicked issues in agricultural and urban watersheds. Front. Environ. Sci. 11:1199923. doi: 10.3389/fenvs.2023.1199923
Received: 04 April 2023; Accepted: 14 June 2023;
Published: 11 July 2023.
Edited by:
Buddhi Wijesiri, Queensland University of Technology, AustraliaReviewed by:
Hanxi Wang, Harbin Normal University, ChinaAyomi Jayarathne, The University of Queensland, Australia
Copyright © 2023 Nsenga Kumwimba, Zhu, Stefanakis, Ajibade, Dzakpasu, Soana, Wang, Arif, Kavidia Muyembe and Agboola. This is an open-access article distributed under the terms of the Creative Commons Attribution License (CC BY). The use, distribution or reproduction in other forums is permitted, provided the original author(s) and the copyright owner(s) are credited and that the original publication in this journal is cited, in accordance with accepted academic practice. No use, distribution or reproduction is permitted which does not comply with these terms.
*Correspondence: Mathieu Nsenga Kumwimba, bWF0aGlldW5zZW5nYUBnbWFpbC5jb20=