- 1Department of Applied Chemistry, College of Science, China Agricultural University, Beijing, China
- 2Guizhou Academy of Testing and Analysis, Guiyang, China
Introduction: Prothioconazole is a triazolinthione fungicide widely used to control diseases on various crops caused by Ascomycetes, Basidiomycetes, and Imperfecti fungi. However, in view of the rapid transformation of parent prothioconazole to its major metabolite prothioconazole-desthio during application and the fact that the latter is of higher toxicity, prothioconazole poses a potential threat to the health of operators in direct contact with it. Currently, very few studies have been carried out regarding prothioconazole and prothioconazole-desthio exposure.
Methods: Therefore, a typical exposure scenario from wheat-planting provinces in China was chosen, and a motorized backpack-type sprayer was used as the application equipment. The actual exposure doses of prothioconazole and prothioconazole-desthio for mixers/loaders and applicators under different exposure routes were determined, and a tiered risk assessment was conducted considering different conditions.
Results: The results of this study indicated that the exposure risk to operators after spraying with 30% prothioconazole oil dispersion was unacceptable in the primary risk assessment, while the risk was acceptable with integrated risk quotients less than 1 at a better level of protection. Moreover, the upper legs and hands were regarded as the most contaminated sections during the mixing/loading process, and legs were the most contaminated sections during spraying. Good protection equipment, such as hats, long-sleeve shirts, long pants, socks, shoes, and rubber or nitrile gloves, was effective at alleviating prothioconazole and prothioconazole-desthio exposure. This study aims to provide important references for the safe use and risk management of prothioconazole.
1 Introduction
Prothioconazole, developed by Bayer CropScience, is a triazolinthione fungicide that exerts excellent bactericidal properties. It is widely used to control diseases caused by Ascomycetes, Basidiomycetes, and Imperfecti fungi on crops such as grain, soybean, and oilseed (Griffiths et al., 2003; Jautelat et al., 2004; Lin et al., 2017). The mechanism of action for prothioconazole is to interfere with the synthesis of ergosterol by inhibiting the enzymatic activity of CYP51, resulting in changes in cell membrane morphology and function (Zarn et al., 2003; Dutzmann, 2004; Casida and Durkin, 2017). Owing to its good systematicity, long duration, and broad-spectrum bactericidal activity, prothioconazole has been registered in more than 60 countries around the world, with increasing sales year by year since its introduction in 2004 (Jautelat et al., 2004). In 2005, sales of prothioconazole reached $110 million US, and rose to $800 million US in 2015, rapidly ranking among the top 10 varieties of fungicides in the world (Huang and Bai, 2017; Cheng et al., 2018; Zhang et al., 2020). In China, although the domestic registration work for prothioconazole products has just begun, there are already 15 domestic enterprises that have registered for the technical ingredients and six companies that have been approved for pesticide formulations (China Pesticide Information Network, 2023). Overall, prothioconazole has broad prospects in the global fungicide market.
It has been reported that prothioconazole has reproductive and developmental toxicity and a relatively high potential exposure risk to humans (Zhang et al., 2020). The increasing use of prothioconazole products worldwide inevitably brings out the exposure issues for operators. It has been reported that a major metabolite, prothioconazole-desthio, is derived from parent prothioconazole when applied in field spraying or in the metabolic testing of animals and plants (USEPA, 2007; JMPR, 2008). For example, after spraying, on the surfaces of treated seeds, crops, application equipment, clothes, and human skin, prothioconazole tended to be transformed into prothioconazole-desthio (Hellpointner and Borchers, 2004; USEPA, 2007). Moreover, prothioconazole can be completely photolyzed into the major product prothioconazole-desthio, which accounts for up to 56% of the total content with a half-life of 48 h in experimental conditions (Shi et al., 2016). Worse still, prothioconazole-desthio has higher toxicity than parent prothioconazole and might induce symptoms, including increased liver weight, CYP enzyme induction, primary hypertrophy, hepatic steatosis (lipid vacuolation), single cell or focal necrosis, watery degeneration, and increased chromosome ploidy, according to a short-term repeated dose toxicity study (Lin et al., 2017; Xie et al., 2019a; Meng et al., 2021). For prothioconazole-desthio, the incidence of cleft palate increased significantly at 50 and 100 mg/kg bw/d in both rat and rabbit developmental studies. On the contrary, in a developmental study with prothioconazole, no cleft palate was observed in offspring (USEPA, 2007; JMPR, 2008). Conjugation with glucuronic acid is a common pathway for metabolism in animals, which is an effective detoxification reaction (Testa and Krämer, 2008). Prothioconazole is conjugated with glucuronic acid, forming an S-glucuronide, and then excreted quickly to reduce toxicity; however, prothioconazole-desthio does not undergo this process and therefore has a longer metabolism and is more toxic than prothioconazole. Undoubtedly, the rapid conversion and formation of a more toxic metabolite makes prothioconazole a potential threat to the health of operators in direct contact with these two compounds during application. Consequently, a health risk assessment following simultaneous exposure of operators to prothioconazole and its major metabolite prothioconazole-desthio deserves urgent attention.
Given the uncertain health risks posed by prothioconazole and prothioconazole-desthio, the European Food Safety Agency (EFSA) and United States Environmental Protection Agency (EPA) took the lead to conduct a health risk assessment of pesticide operators. The EFSA adopted the UK-POEM model and the German model to assess operator exposure with tractor application and concluded that the exposure is lower than the allowable level only with good personal protection equipment (EFSA, 2007). Additionally, the EPA assessment demonstrated that the exposure with aircraft application is not acceptable to operators even with good personal protection equipment and a closed cap system (USEPA, 2007). The above two existing studies focused mostly on tractor and/or aircraft applications, which are representative of occupational personal protection equipment, respiratory protection equipment, and closed protection systems that strictly follow label recommendations. However, these scenarios seem unsuitable for developing countries, including China, and studies relevant to developing countries are rarely carried out. In China, the cultivation mode is mainly characterized by small-scale production from scattered farmers with the family as a unit, and thus, there are great differences across regions in terms of production conditions, farming mode, etc. Moreover, the application equipment is typically a manual or motorized backpack-type sprayer, and the motorized version is the most frequently used equipment in rural areas of China as it is more labor-saving than the manual one (Gao, 2014). Additionally, there is a lack of systematic management and protection regulations in terms of the safe use of pesticides by farmers in China. Furthermore, the farmers’ awareness of personal protective equipment is poor, and the market supply of personal protective equipment for backpack sprayers is very insufficient (Tao et al., 2019). The only Chinese report showed that when prothioconazole is used in the backpack manual spray scenario with a calculated dosage of 0.2 kg available ingredient (a.i.)/ha and wheat and other medium-height crops as the target crops, the health risk following exposure is unacceptable due to the fact that prothioconazole could be converted to prothioconazole-desthio (Yu, et al., 2017). Thus, operators in developing countries (China included) have higher exposure risks to prothioconazole and prothioconazole-desthio.
Currently, no field data have been generated for the backpack sprayer application of prothioconazole-containing formulations in field conditions. Alarmingly, few studies regarding prothioconazole and prothioconazole-desthio exposure have been undertaken. Therefore, it is imperative to clarify the conversion rule and determine the exposure dose of prothioconazole and prothioconazole-desthio and exactly evaluate their health risk during the application process in combination with the actual application status in China. In this context, considering the potentially high health risk of prothioconazole and prothioconazole-desthio to pesticide operators (including the mixer/loader and applicator), the aims of this study are: 1) to determine the actual exposure dose for active ingredients of prothioconazole and its metabolite prothioconazole-desthio after the spraying of prothioconazole formulation with a backpack sprayer, and 2), to exactly assess the risk of operators in the above operation with the help of a tiered evaluation method.
2 Materials and methods
2.1 Reagents and materials
The standards prothioconazole (98.2% purity) and prothioconazole-desthio (98.98% purity) were provided by Anhui Jiuyi Agriculture Co., Ltd., and Hefei Liheng Chemical Co., Ltd, respectively. The pesticide formulation of 30% prothioconazole oil dispersion (OD) was provided by Anhui Jiuyi Agriculture Co., Ltd. Acetonitrile, methanol, and formic acid of chromatographic grade were purchased from Beihua Fine-chemicals Co., (Beijing, China). Acetonitrile, hydrochloric acid, aerosol, and sodium chloride (NaCl) of analytical grade were purchased from Tianjin Saifurui Technology Co., Ltd. The cotton clothes and gauze were purchased from Beijing Bopute Technology Co., Ltd. UltraPure water was purchased from Beijing Kaimia Technology Co., Ltd.
2.2 Field study
The trials were conducted in Henan province, Hunan province, and Anhui province, China, which are the main wheat planting provinces in China. A motorized power backpack sprayer was used to apply 30% prothioconazole OD at a dose of 675 ml product formulation/ha to the treatment plots once, covering an area of 2 acres (approximately 1,333 m2) for each individual (90 ml formulation per individual in total; the duration of application was 82–113 min). Each mixer/loader prepared the solution for immediate spraying; the total duration for mixing/loading per individual was 20–39 min. There were seven mixers/loaders and seven applicators at each trial site. The whole-body sampling method was used to collect tested samples from the participants for exposure analysis of prothioconazole and its metabolite prothioconazole-desthio. In the survey for this study, the temperature was maintained at 19.0°C–32.2°C; relative humidity was recorded between 20.7% and 50.4%, and wind speed was measured at a range of 0.2–2.2 m/s.
2.2.1 Mixing/loading
The process of mixing/loading began after all the protection suits and test units had been well equipped. The detailed operation was described as follows: add 18 ml of 30% prothioconazole OD into the sprayer tank and mix with 5 L of water to agitate sufficiently; finally, complement the mix with 10 L water and agitate properly. This process was repeated five times, indicating that each mixer/loader would prepare five tanks (equivalent to 0.0277 kg of active ingredient in total) for fresh use, resulting in a content of active ingredient of 0.0208–0.0333 kg. At the end of mixing/loading, tested pieces from different parts of seven mixers/loaders (M01∼M07) per application site were individually collected and recorded. The sectioning process was conducted according to the process described by Gao et al. (2014).
2.2.2 Pesticide spraying
The applicators performed the spraying according to their own habit. The treatment plots were subjected to one spraying with the pesticide formulation at a dose of 675 ml/ha, covering a spraying area of 2 acres (approximately 1,333 m2) for each applicator, which was equivalent to 0.0277 kg of active ingredient, thus the actual contents of active ingredient ranged between 0.0208 and 0.0333 kg. At the end of spraying, tested pieces from different parts of seven applicators (A01∼A07) per application site were individually collected and recorded. The sectioning process was conducted according to the process described by Gao et al. (2014).
2.3 Sample extraction
2.3.1 Cotton cloth (inner and outer layer clothes, gloves, and hats)
Acetonitrile was added at a ratio of 20 ml/1.0–1.5 g of cotton to ensure the samples could be completely submerged. After ultrasonic extraction for 10 min, the supernatant layer was transferred and filtered using a 0.22-μm nylon filter for further injection analysis.
2.3.2 OVS tube
The OVS tubes were divided into two parts, the front end of which was coated with 500 mg of XAD-2 packing (the front and rear glass fiber cotton included) and the back end was coated with 100 mg of XAD-2 packing. The two parts were added with 20 ml of methanol, vortexed vigorously for 2 min, and then the supernatant layer was transferred and filtered using a 0.22-μm nylon filter for further injection analysis.
2.3.3 Gauze
The cotton gauze was transferred into a 100 ml centrifuge tube. Then, 4 ml of pure water, 200 μl of 0.1 mol/l hydrochloric acid solution, and 40 ml of acetonitrile were added; the tubes were then mixed vigorously for 2 min. Next, 3.0 g of NaCl was added and the tubes were mixed again for 2 min and then centrifuged at 5,000 r/min for 3 min. The supernatant layer was transferred and filtered using a 0.22-μm nylon filter for further injection analysis.
2.3.4 Aerosol solution
A volume of 2 ml of 0.01% aerosol solution was added with 100 μl of 0.1 mol/L hydrochloric acid solution and 10 ml of acetonitrile; the tubes were then mixed vigorously for 2 min. Then, 1.0 g of NaCl was added and the tubes were mixed for 1 min and then left to stand for 5 min. The supernatant layer was transferred and filtered using a 0.22-μm nylon filter for further injection analysis.
2.4 Instrument analysis
The exposure measurement for prothioconazole and prothioconazole-desthio was performed using the high-performance liquid chromatograph coupled with a mass spectrometer (HPLC-MS/MS, Thermo, United States). A Shim-pack XR-ODS Ⅱ analytical column (2.0 mmi.d. × 75 mm) with 1 μl of injection volume for each run was used, and the temperature of the column was maintained at 40°C. The run time was set as 5.5 min for each injection. The mobile phase running at 0.3 ml/min flow rate consisted of 5 mmol/L ammonium acetate +0.02% formic acid solution (A) and acetonitrile (B). The elution gradient was performed from 15% B (maintained within 1.5 min), then increased to 95% B at 1.5 min (maintained for 2.0 min), and finally decreased back to 15% B at 3.51 min.
MS/MS detection was operated under positive electrospray ionization (ESI+) for prothioconazole-desthio and negative electrospray ionization (ESI-) for prothioconazole. The main parameters were set as follows: source temperature, 300°C; desolvation temperature, 250°C; heating block temperature, 400°C; atomized gas flow rate, 3 L/min; heating gas flow rate, 10 L/min; and desolvation gas flow rate, 10 L/min. A dwell time of 100 ms per ion pair was used to achieve high sensitivity for the analytes. The optimized ion transitions for prothioconazole were 342.10→100.20 with 20 V collision energy and 342.10→125.30 with 25 V collision energy for quantitative analysis and qualitative analysis, respectively. For prothioconazole-desthio, the quantitative ion transition was 312.20→70.10 with 25 V collision energy and 312.20→125.20 with 30 V collision energy for qualitative ion transition. Under the conditions above, the retention time of prothioconazole and prothioconazole-desthio was approximately 2.82 and 2.77 min, respectively.
2.5 Quality assurance and quality control
The specificity, precision, and accuracy of the analytical method were validated by the blank sample and recovery assays. The limit of quantification (LOQ) for prothioconazole and prothioconazole-desthio was defined as the lowest fortification concentration for each matrix, in which the concentration level could produce a signal to noise (S/N) ratio ≥10. Thus, the method LOQs for prothioconazole in cotton cloth, the OVS tube, gauze, and aerosol solution were estimated at 0.04 mg a.i. kg−1, 0.2 mg a.i. kg−1, 0.02 mg a.i. kg−1, and 0.01 mg·L−1, respectively. For prothioconazole-desthio, the method LOQs were 0.008 mg a.i. kg−1 for cotton cloth, 0.04 mg a.i. kg−1 for the OVS tube, 0.04 mg a.i. kg−1 for gauze, and 0.002 mg·L−1 for aerosol solution.
The standard solutions were analyzed in triplicate, with the concentrations ranging from 0.001 to 0.5 mg·L−1 for prothioconazole and 0.0002–0.1 mg·L−1 for prothioconazole-desthio, and good linearities were observed, with the correlation coefficient of the standard curves (r) higher than 0.99. From the results of the recovery assay, at fortification concentrations of LOQ and 200-fold LOQ for prothioconazole and at fortification concentrations of LOQ and 75-fold LOQ for prothioconazole-desthio, the overall mean recoveries for the two compounds fell within 84.8%–103.6%, with 4.4%–8.6% RSD for cotton cloth; 70.7%–93.6% with 2.1%–12.3% RSD for the OVS tube at LOQ and 100-fold LOQ both for prothioconazole and prothioconazole-desthio; 78.2%–106.0% with 6.4%–10.4% RSD for gauze at LOQ and 200-fold LOQ both for prothioconazole and prothioconazole-desthio; 86.0%–107.5% with 0.0%–6.4% RSD for aerosol solution at LOQ and 200-fold LOQ both for prothioconazole and prothioconazole-desthio.
As for the field site recovery assay, cotton cloth (inner and outer layer clothes, gloves, and hats) was tested at a 10-fold LOQ level with six replicates, gauze with four replicates at 20-fold LOQ, OVS tube with four replicates at 10-fold LOQ, and aerosol solution with four replicates at 10-fold LOQ. The overall recovery values were in the range of 53.4%–102.0% at the Henan site, 55.7%–110.5% at the Hunan site, and 35.0%–114.7% at the Anhui site.
From the recovery results of transport and storage stability, for the samples from the Henan trial site, the overall mean recoveries of prothioconazole and prothioconazole-desthio ranged from 65.9% to 113.9%. The mean recoveries of prothioconazole and prothioconazole-desthio fell within 47.4%–113.0% and 64.5%–109.8% for the Hunan trial site and Anhui trial site samples, respectively.
2.6 Model parameters of COPRisk 2.3
Because of the vast territory of China, the geographical and climatic differences between provinces are obvious, and the applications are also different from other developed countries; therefore, the Ministry of Agriculture and Rural Affairs (ICAMA) of China developed a pesticide risk assessment model COPrisk based on China’s national conditions. A COPrisk model can combine the toxicity test data of different active ingredients and establish exposure scenarios to assess the possible risks of pesticides. Unit exposure (UE) data are obtained after extensive preliminary tests and then established in the model. Now, the only application method used for COPrisk is the manual backpack sprayer. The model can be applied to the products with hundreds of different target crops and pests. However, this model cannot be applied to other pesticide formulation types, such as powder and granules, and some new application methods, such as aircraft control, because of the lack of UE data of these exposure scenarios. In the tier 1 risk assessment, the default UE data in the model is used. If the risk is unacceptable, the UE data for the specific application scenario can be used for tier 2 risk assessment.
Prothioconazole has been registered in China to control scab, powdery mildew, and rust in wheat with various formulations, of which oil-based suspension concentrate, suspension concentrate, and water-dispersible granule make up the majority. The oil-based suspension concentrate composed of 30% prothioconazole became the maximum recommended dosage (202.5 g a.i./ha) among all the formulations. In this study, a knapsack sprayer was used for spraying. Considering the actual situation of the awareness of self-protection and how the farmers dress when they were applying pesticide, several factors related to applicators were considered to create three personal protective equipment levels (a. short-sleeve shirt, short pants, socks, and shoes; b. long-sleeve shirt, long pants, socks, and shoes; c. long-sleeve shirt, long pants, socks, shoes, and gloves). According to the guidance on health risk assessments of pesticide operators in China, the spraying directions were downward (spraying wheat <80 cm in height) and horizontal (spraying wheat 80–130 cm in height) when used to control scab, powdery mildew, and rust in wheat.
Prothioconazole formulation application, with a 7-day retreatment interval, was recommended at the first sign of disease in the life cycle of wheat. Owing to the short spraying duration, the occupational exposure to prothioconazole was assumed as short-term dermal and inhalation exposure (1–30 days).
2.7 Data calculation and analysis
The UE approach was applied to expound all dermal route and inhalation route exposure, following Eqs 1, 2.
where UE represents unit exposure (unit, mg a.i. kg-1 a.i. handled), AR represents application rate dose (unit, kg a.i.), Exposuredermal indicates residue measures from each dermal dosimeter (unit, mg a.i.); exposureinhalation indicates residue measures from the OVS tube (unit, mg a.i.), IR indicates the inhalation rate [unit, L·min−1], with a default of 32.7, V indicates the air sampling volume (unit, L), T indicates the air sampling time (unit, min), and AR represents the application rate dose (unit, kg a.i.).
Exposure and RQ dose via dermal and inhalation routes resulting from mixing/loading and spraying were calculated according to Eqs 3, 4:
(AOEL is shown in Table 1),
Where Rate indicates the application dosage (unit, kg/ha), Area indicates the application area per day (unit, ha) (COPRisk 2.3 default value = 1 ha), and BW represents body weight (unit, kg) (COPRisk 2.3 default value = 60.6 kg) (MOA, 2017; ICAMA, 2018). If RQ ≤ 1, the health risk is acceptable, whereas if RQ > 1, the health risk is unacceptable.
All statistical analyses in this study were carried out using IBM SPSS Statistics 23.0. The Skewness-Kurtosis test was used to examine the normal distribution for the obtained data or not. Two-tailed p < 0.05 was regarded as statistically significant.
3 Results and discussion
3.1 Unit exposure
3.1.1 Mixer/loader unit exposure
The total dermal and inhalation UE for 21 mixers/loaders from three trial sites were measured and summarized in Tables 2, 3. For prothioconazole, the potential dermal UE ranged from 485.66 to 4,689.11 mg a.i. kg−1 a.i. handled; the actual dermal UE ranged from 2.2 to 413.68 mg a.i. kg−1 a.i. handled and the potential inhalation UE ranged from 0.07 to 0.84 mg a.i. kg−1 a.i. handled. As for prothioconazole-desthio, the potential dermal UE was 8.58–117.53 mg a.i. kg−1 a.i. handled; the actual dermal UE was 0.28–8.11 mg a.i. kg−1 a.i. handled, and the potential inhalation UE was 0.01–0.17 mg a.i. kg−1 a.i. handled.
3.1.2 Applicator unit exposure
The total dermal and inhalation UE for 21 applicators from three trial sites is shown in Tables 4, 5. For prothioconazole, the potential dermal UE ranged from 46.05 to 443.11 mg a.i. kg−1 a.i. handled; the actual dermal UE ranged from 2.62 to 39.55 mg a.i. kg−1 a.i. handled, and the potential inhalation UE ranged from 0.08 to 1.01 mg a.i. kg−1 a.i. handled. As for prothioconazole-desthio, the potential dermal UE was 13.79–261.39 mg a.i. kg−1a.i. handled; the actual dermal UE was 0.42–19.87 mg a.i. kg−1 a.i. handled, and the potential inhalation UE was 0.02–0.14 mg a.i. kg−1 a.i. handled.
For prothioconazole, the UE of the mixer/loader is much larger than that of applicators. The reasons for this may be that during mixing and loading, the mixer/loader is in direct contact with the high concentrations of pesticides. This result is similar to that reported by Lee et al. (2023). During mixing/loading and hand-held spraying scenarios, the most exposed body part was the hand (90.4%) of the mixer/loader.
For farmers, especially those with no safe practices and insufficient education about the use of pesticides, dermal exposure is the predominant exposure route due to splashing, spillage, and spray drift of pesticides during the application process (Mahaboonpeeti et al., 2018; Bootsikeaw et al., 2021; Bormann et al., 2021; Tudi et al., 2022). As for the findings from our field tests, for the mixer/loader and applicators, the most contaminated sections were upper legs/hands and legs, respectively. These data are similar to the exposure assessment data of previous studies (Lee et al., 2023). Ren et al. (2019) evaluated the exposure of 60 greenhouse operators to the insecticide clothianidin when applied with knapsack electric sprayers; the total dermal UE was 598.71 mg·kg−1 and the inhalation exposure was only 0.50 mg·kg−1 (Ren et al., 2019). As for the findings from our study, both for the mixer/loader and applicator, the dermal UE was much greater than inhalation UE. Wang et al. (2020) studied the exposure data of occupational handlers using stretcher-type power sprayers in orchards; the results showed that one layer of clothing can reduce dermal exposure by approximately 89% (Wang et al., 2020). Yang et al. (2013) studied the exposure of farmers using a manual sprayer by the whole-body sampling method in corn fields; the exposure of inner layer clothing is lower than that of outer clothing (Yang et al., 2013). Similar to the results of our study, the potential dermal UE is far greater than the actual dermal UE. This also confirms the importance of wearing protective clothing.
3.2 Tiered risk assessment
According to the guidance on health risk assessments of pesticide operators in China (MOA, 2017), the primary health risk assessment was performed using COPRisk 2.3 to predict the potential pesticide exposure. Risk assessment was performed by evaluating the risk quotient (RQ). RQ equals the exposure divided by AOEL. When the RQ is greater than 1, the health risk is unacceptable. In general, the RQ of different exposure routes should be summed, as should the metabolite.
3.2.1 Tier 1 risk assessment
Owing to the lack of data on the chemical transformation of prothioconazole to prothioconazole-desthio, the primary health risk assessment was based on zero (exposure to prothioconazole) and 100% (exposure to prothioconazole-desthio) conversion.
The tier 1 risk assessment was conducted using the default UE data with COPRisk 2.3.When wheat plants were sprayed with 30% prothioconazole OD following the maximum recommended application rate (202.5 g ai/ha), the dermal and inhalation exposure became the main routes. As shown in Table 6, the RQs were all greater than 1 at each PPE level, even with good PPE (RQ = 4.5066) based on zero conversion (exposure to prothioconazole). Then, a 100% conversion (exposure to prothioconazole-desthio) was assumed to modify the health risk assessment so that the maximum recommended application rate of prothioconazole-desthio was 183.7 g ai/ha calculated by molar coefficient conversion (344.1:312.2). Similar to prothioconazole, no RQ was less than 1 for prothioconazole-desthio, even with good PPE (RQ = 33.6679) in this assumed condition (Table 7). Therefore, it could be concluded that the exposure risk to operators after motorized backpack spraying with 30% prothioconazole OD in wheat was unacceptable in the primary risk assessment.
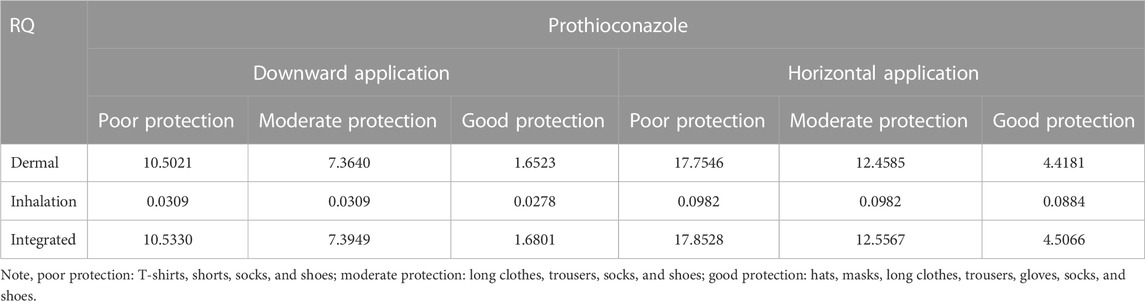
TABLE 6. Respective and integrated risk assessment of 30% prothioconazole OD applied in wheat fields for applicators under dermal/inhalation exposure routes (no conversion).
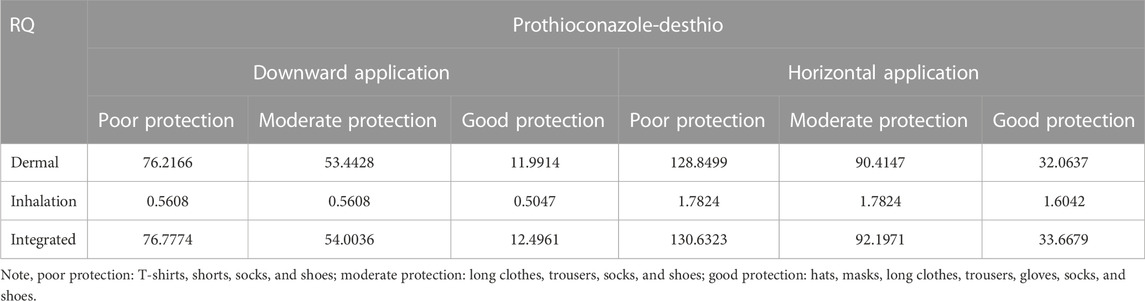
TABLE 7. Respective and integrated risk assessment of 30% prothioconazole OD applied in wheat fields for applicators under dermal/inhalation exposure routes (100% conversion).
In recent years, China has started to conduct occupational exposure assessments of pesticides, and the risk assessment is necessary for pesticide registration. Our exposure assessment was based on the methods used in developed countries, where the application of pesticides is very specialized, serialized, and standardized, and depending on the application, there is a dedicated machine for each scenario (Liu et al., 2010). By contrast, owing to the small-scale farmer household economy in China, large-tonnage mechanical spraying equipment is not popular, and manual pesticide spraying plays a leading role in agricultural production in most areas of China. The pesticide application equipment is aging, and the market share of manual sprayers is 80% in China (National Agro-Tech Center Pesticide and Medicine Division, 2017). It is essential to assess the exposure of Chinese farmers according to their application habits. The COPRisk 2.3 model is established for pesticide health risk assessment according to the fundamental realities of China. The data embedded in the model are only the results of a few trials and may not be a good representation of the actual situation in each specific scenario for each crop.
The UE data varies significantly due to different factors, such as the applicator, crops, equipment, and local environment. The different heights of the crops may lead to different exposures. An et al. (2014) detected the dermal and inhalation exposure at different heights of maize in China; the results indicated that at a plant height of 212 cm, the exposure is much greater than the UE at two other heights (62 and 108 cm). Similar results were found in Ren’s and Li’s studies, which showed that UE increased with increasing crop height (Li et al., 2019; Ren et al., 2019).
The default plant in the COPRisk 2.3 model is maize; however, we applied the pesticide to wheat, and the heights of the two crops are clearly different, and the crop is one of the main factors affecting the result of pesticide exposure (Chen, 2012). Additionally, the application equipment was different; a manual sprayer was used in the COPRisk 2.3 model, whereas we used a motorized backpack sprayer. It is clear that the default data used in the COPRisk 2.3 model overestimate the risk. The different application scenarios are the root cause of fluctuations in pesticide exposure (Cano et al., 2000); therefore, the tier 2 risk assessment using the specific UE of typical use scenarios in China is necessary to evaluate the risks of pesticides.
3.2.2 Tier 2 risk assessment
To overcome the limitation of the primary risk assessment and to obtain the practical UE and exposure data, we performed an exposure monitoring study containing a total of 21 operators (including seven mixers/loaders and seven applicators per site) from three wheat-producing areas.
From the normality test results in Table 8, the data from the mixers/loaders and applicators under the dermal exposure route for prothioconazole and prothioconazole-desthio all demonstrated a normal distribution with p > 0.05. However, the non-normal distribution data were observed for the above groups under the inhalation exposure route with p < 0.05. Therefore, the dermal unit exposure was derived from the arithmetic mean value from the individual’s matrix. The inhalation unit exposure was derived from the geometric mean value from the individual’s matrix. So, we utilized the arithmetic mean value of dermal UE and the geometric mean value of inhalation UE to evaluate the practical risk, and the RQs are listed in Table 9.
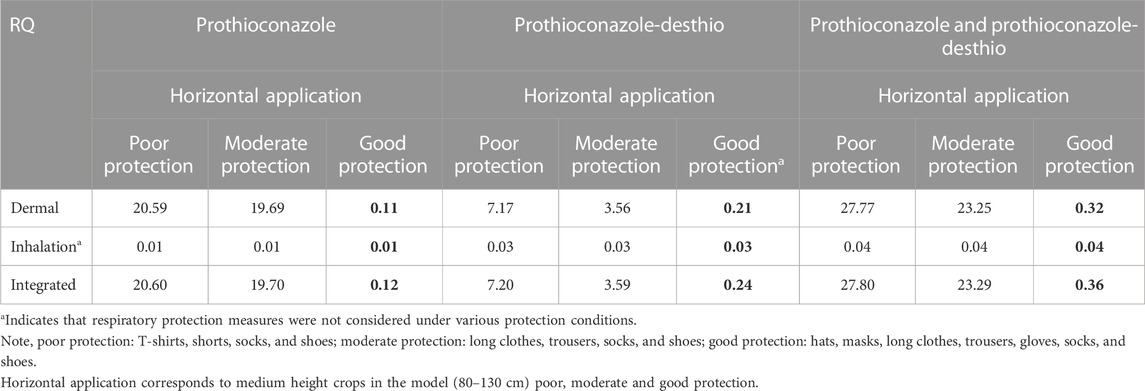
TABLE 9. Respective and integrated risk assessment of 30% prothioconazole OD applied in wheat fields for applicators under dermal/inhalation exposure routes.
The tier 2 risk assessment based on actual exposure data collected by direct measurement indicated that the summed RQ was less than 1 (RQ = 0.36) at a good level of protection. Specifically, the RQs of prothioconazole and prothioconazole-desthio at a better level of protection were 0.12 and 0.24, respectively. It could be concluded that the exposure risk to operators after mixing/loading and motorized power backpack spraying with 30% prothioconazole OD in wheat was acceptable in the tier 2 risk assessment.
As a widely used systemic broad-spectrum triazolinthione fungicide, prothioconazole will continue to be popularly used in agricultural systems (Zhang et al., 2020). Pesticide exposure may adversely affect the health of operators in agricultural production, especially farmers with no protection awareness, poor standard operating procedures for handling and practices, and poorly maintained spraying equipment (Moerman and Steenaard, 2014; Liu et al., 2017; Sharifzadeh et al., 2017; Shammi et al., 2020). However, farmers in China have poor protective habits and usually pay more attention to convenience and therefore seldom wear gloves during mixing/loading and application. Additionally, cotton gloves were used more frequently among operators as they are cheaper and more affordable. Therefore, the PPE conditions of the risk assessment were close to the actual level of protection, and we assumed that the better level of protection represented the current level of protection for farmers. The mixer/loader and applicator are often the same person when backpack spraying. It is necessary to combine the exposure during mixing/loading and application.
It has been suggested that dermal exposure may be greater than inhalation exposure because it is exacerbated by a variety of different risk factors, including crop structure, the maintenance status of the equipment, and the use of personal protective equipment (Wong and Brown, 2021). The main exposure area was the hands, and exposure was relatively uniform for pesticide mixers/loaders (Han et al., 2021; Wang et al., 2021). Wang’s study showed that the hands had the highest exposure, accounting for an average of 59% of total dermal exposure (Wang et al., 2020), which was similar to Ren’s experiment, which showed that the leg was the largest exposure area, accounting for approximately 53% of total dermal exposure (Ren et al., 2019). An et al. found that the major exposure parts of the applicators was different because of the different plant heights; upper leg exposure was greater than other parts of the body when the average plant height was 61.8 cm, which was almost the same height as the wheat in our study (An et al., 2014). As for the findings from our field tests, the most contaminated sections were the upper legs and hands during mixing/loading because these were the major body parts that were directly in contact with pesticides during the unpacking and mixing/loading of pesticides. The degree of direct contact with plants could directly affect the pesticide exposure level (Franklin and Worgan, 2005). This is consistent with the conclusions of Wang et al. (2020), Ren et al. (2017), and An et al. (2014). Therefore, it is critically important to wear rubber or nitrile gloves during mixing and loading (Ren, et al., 2017; Thouvenin et al., 2017; Li et al., 2019; Wang et al., 2020). However, we found that the most contaminated sections were the legs during application, which was directly ascribed to spraying because the legs of the applicators could directly contact the leaves and stems during spraying, resulting in the highest exposure. Similar studies reported that the legs and feet were usually the most exposed body parts because of easy contact with spray droplets (Hughes et al., 2006; Ramos et al., 2010). Therefore, long pants were required during spraying.
4 Conclusion
To exactly evaluate the actual health risk of operators when applying 30% prothioconazole OD in wheat, a monitor study was performed in three wheat-planting provinces in China with the participation of mixers/loaders and applicators from each trial site, and a tiered risk assessment was carried out. The whole-body method and a personal air sampler were used to determine dermal and inhalation exposures, respectively. The exposure data were used to modify a tier 2 health risk assessment. Results demonstrated that the exposure to operators is acceptable when 30% prothioconazole OD is applied following the maximum recommended application rate (202.5 g ai/ha) with a good PPE level. An improved protection level is highly recommended when applying prothioconazole formulation, including wearing a hat, long-sleeve shirt, long pants, socks, and shoes. Additionally, rubber or nitrile gloves are advised to prevent chemical contamination.
Data availability statement
The original contributions presented in the study are included in the article/Supplementary Material, further inquiries can be directed to the corresponding authors.
Author contributions
SL: conceptualization, methodology, data curation, writing—original draft. CM: writing—review and editing. XW: conceptualization, writing—review and editing. XL: conceptualization, writing—review and editing, validation, supervision. SP: conceptualization, writing—review and editing, supervision. All authors contributed to the article and approved the submitted version.
Funding
This study was financially supported by the National Natural Science Foundation of China (32001946) and the Youth Foundation of Guizhou Academy of Sciences (Grant No. Qiankeyuan J [2021]43).
Acknowledgments
We would like to thank our research group colleagues for their constructive suggestions. Additionally, we thank the reviewers and members of the editorial team for their constructive comments.
Conflict of interest
The authors declare that the research was conducted in the absence of any commercial or financial relationships that could be construed as a potential conflict of interest.
Publisher’s note
All claims expressed in this article are solely those of the authors and do not necessarily represent those of their affiliated organizations, or those of the publisher, the editors and the reviewers. Any product that may be evaluated in this article, or claim that may be made by its manufacturer, is not guaranteed or endorsed by the publisher.
Abbreviations
a.i., available ingredient; a.i-handled, application amount of available ingredient; OD, oil dispersion; LOQ, limit of quantitation; RSD, relative standard deviation; UE, unit exposure; RQ, risk quotient.
References
An, X., Ji, X., Wu, M., Hu, X., Yu, R., Zhao, X., et al. (2014). Risk assessment of applicators to chlorpyrifos through dermal contact and inhalation at different maize plant heights in China. J. Agric. Food Chem. 62 (29), 7072–7077. doi:10.1021/jf501027s
Bootsikeaw, S., Kongtip, P., Nankongnab, N., Chantanakul, S., Sujirarat, D., Mahaboonpeeti, R., et al. (2021). Urinary glyphosate biomonitoring of sprayers in vegetable farm in Thailand. Hum. Ecol. Risk Assess. 27 (4), 1019–1036. doi:10.1080/10807039.2020.1797471
Bormann, J. L., Acipayam, A. S. F., and Maibach, H. I. (2021). Percutaneous absorption of chemicals from fabric (textile). J. Appl. Toxicol. 41 (2), 194–202. doi:10.1002/jat.4077
Cano, C. M. L., Vidal, M. J. L., Gonzalez, E. F. J., Martıinez Galera, M., and Cruz Márquez, M. (2000). Gas chromatographic method and whole body dosimetry for assessing dermal exposure of greenhouse applicators to chlorpyrifos-methyl and fenitrothion. Anal. Chim. Acta 423, 127–136. doi:10.1016/S0003-2670(00)01084-9
Casida, J. E., and Durkin, K. A. (2017). Pesticide chemical research in toxicology: Lessons from nature. Chem. Res. Toxicol. 30, 94–104. doi:10.1021/acs.chemrestox.6b00303
Chen, B. (2012). Preliminary evaluation of pesticide exposure of pesticides in wheat field and peanut field spray operations. Taian, China: Shandong Agricultural University.
Cheng, Y., Cui, R., Guo, W., Zuo, W., Zhu, Y., Zhuang, Z., et al. (2018). Membrane protein structural biology in the era of single particle cryo-EM. Shandong Chem. Ind. 47 (6), 58–63. doi:10.1016/j.sbi.2018.08.008
China Pesticide Information Network (2023). Institute Control of Agrochemicals, Ministry of Agriculture and Rural Affairs.
Dutzmann, S. (2004). Prothioconazole: A broad spectrum demethylation-inhibitor (DMI) for arable crops. Pflanzenschutz-Nachrichten Bayer. 57 (2), 249–264.
EFSA (2007). Conclusion regarding the peer review of the pesticide risk assessment of the active substance prothioconazole. EFSA Sci. Rep. 106, 1–98. doi:10.2903/j.efsa.2007.106r
Franklin, C. A., and Worgan, J. P. (2005). Occupational and residential exposure assessment for pesticides. Chichester, UK: Wiley.
Gao, B. (2014). “Pesticide occupational exposure risk assessment for knapsack sprayer scenario,”. Master dissertation. Beijing: Chinese Academy of Agricultural Sciences Dissertation.
Gao, B., Tao, Ch., Ye, J., Ning, J., Mei, X., Jiang, Zh., et al. (2014). Measurement of operator exposure to chlorpyrifos. Pest Manag. Sci. 70 (4), 636–641. doi:10.1002/ps.3601
Griffiths, K. M., Bacic, A., and Howlett, B. J. (2003). Sterol composition of mycelia of the plant pathogenic ascomycete leptosphaeria maculans. Phytochem 62 (2), 147–153. doi:10.1016/S0031-9422(02)00505-8
Han, R., Wu, Z., Huang, Z., Man, X., Teng, L., Wang, T., et al. (2021). Tracking pesticide exposure to operating workers for risk assessment in seed coating with tebuconazole and carbofuran. Pest Manag. Sci. 77 (6), 2820–2825. doi:10.1002/ps.6315
Hellpointner, E., and Borchers, H. (2004). Behaviour of prothioconazole (JAU 6476) in the environment. Pflanzenschutz-Nachrichten Bayer. 57, 163–180.
Huang, H., and Bai, Y. (2017). Global market and application development of prothioconazole. Mod. Agrochem. 16 (96), 45–51.
Hughes, E. A., Zalts, A., Ojeda, J. J., Flores, A. P., Glass, R. C., and Montserrat, J. M. (2006). Analytical method for assessing potential dermal exposure to captan, using whole body dosimetry, in small vegetable production units in Argentina. Pest Manag. Sci. 62 (9), 811–818. doi:10.1002/ps.1232
ICAMA (2018). Manual for unit exposure to pesticide operators with backpack sprayer Released on october 29, 2018, institute control of agrochemicals, Ministry of agriculture, P.R. China. Available at: http://www.chinapesticide.org.cn/jkfxpg/12282.jhtml.
Jautelat, M., Elbe, H. L., Benet-Buchholz, J., and Etzel, W. (2004). Chemistry of prothioconazole (JAU6476). Pflanzenschutz-Nachr. Bayer. 57, 145–162.
JMPR (2008). Pesticide residues in food, joint FAO/WHO meeting on pesticide residues. Québec , Canada: FAO, 265–292.
Lee, J., Lee, J., Jung, M., Shin, Y., Kim, J. W., and Kim, J.-H. (2023). Potential exposure and risk assessment of agricultural workers to the insecticide chlorantraniliprole in rice paddies. Pest Manag. Sci. 79 (2), 678–687. doi:10.1002/ps.7235
Li, Z., Liu, W., Wu, C., and She, D. (2019). Effect of spraying direction on the exposure to handlers with hand-pumped knapsack sprayer in maize field. Ecotoxicol. Environ. Saf. 170, 107–111. doi:10.1016/j.ecoenv.2018.11.121
Lin, H. F., Dong, B., and Hu, J. (2017). Residue and intake risk assessment of prothioconazole and its metabolite prothioconazole-desthio in wheat field. Environ. Monit. Assess. 189 (5), 236. doi:10.1007/s10661-017-5943-1
Liu, F., Zhang, X., Ma, W., and Liu, X. (2010). Transactions of the Chinese society of agricultural machinery. J. Agric.Mech. Res. 32, 246–248.
Liu, H., Yao, G., Liu, X., Liu, C., Zhan, J., Liu, D., et al. (2017). Approach for pesticide residue analysis for metabolite prothioconazole-desthio in animal origin food. J. Agr. Food Chem. 65 (11), 2481–2487. doi:10.1021/acs.jafc.7b00062
Mahaboonpeeti, R., Kongtip, P., Nankongnab, N., Tipayamongkholgul, M., Bunngamchairat, A., Yoosook, W., et al. (2018). Evaluation of dermal exposure to the herbicide alachlor among vegetable farmers in Thailand. Ann. Work. Expo. Health 62 (9), 1147–1158. doi:10.1093/annweh/wxy081
Meng, Z., Tian, S., Sun, W., Liu, L., Yan, S., Huang, S., et al. (2021). Effects of exposure to prothioconazole and its metabolite prothioconazole-desthio on oxidative stress and metabolic profiles of liver and kidney tissues in male mice. Environ. Pollut. 269, 116215. doi:10.1016/j.envpol.2020.116215
MOA (2017). NY/T 3153-2017 Guidance on health risk assessment of pesticide operators. Beijing, China: Ministry of Agriculture, P.R. China.
Moerman, F., and Steenaard, P. (2014). “Hygienic practices for equipment maintenance,” in Woodhead publishing series in Food science, Technology and Nutrition,Hygiene in Food processing. 3rd (Sawston, UK: Woodhead Publishing), 384–407.
National Agro-Tech Center Pesticide and Medicine Division (2017). Overview of pest control in 2017 and analysis of pesticide equipment market in 2018, 30–31.
Ramos, L. M., Querejeta, G. A., Flores, A. P., Hughes, E. A., Zalts, A., and Montserrat, J. M. (2010). Potential dermal exposure in greenhouses for manual sprayers: Analysis of the mix/load, application and re-entry stages. Sci. Total Environ. 408 (19), 4062–4068. doi:10.1016/j.scitotenv.2010.05.020
Ren, J., Tao, C., Zhang, L., Ning, J., Mei, X., and She, D. (2017). Potential exposure to clothianidin and risk assessment of manual users of treated soil. Pest. Manag. Sci. 73, 1798–1803. doi:10.1002/ps.4535
Ren, J. X., Li, Z. K., Tao, C. J., Zhang, L. Y., Zhao, H. F., Wu, C. C., et al. (2019). Exposure assessment of operators to clothianidin when using knapsack electric sprayers in greenhouses. Int. J. Environ. Sci. Technol. 16, 1471–1478. doi:10.1007/s13762-018-1758-z
Shammi, M., Sultana, A., Hasan, N., Mostafizur Rahman, M., Saiful Islam, M., Bodrud-Doza, M., et al. (2020). Pesticide exposures towards health and environmental hazard in Bangladesh: A case study on farmers’ perception. J. Saudi Soc. Agric. Sci. 19 (2), 161–173. doi:10.1016/J.JSSAS.2018.08.005
Sharifzadeh, M. S., Damalas, C. A., and Abdollahzadeh, G. (2017). Retracted: Perceived usefulness of personal protective equipment in pesticide use predicts farmers' willingness to use it. Sci. Total Environ. 609, 517–523. doi:10.1016/j.scitotenv.2017.07.125
Shi, K., Li, L., and Liu, F. (2016). Determination and dissipation of prothioconazole and its metabolite prothioconazole-desthio in soil. Chin. J. Pesticide Sci. 18 (5), 659–663. doi:10.16801/j.issn.1008-7303.2016.0093
Tao, Y., Dung, P., Dong, F., Xu, J., Liu, X., Wu, X., et al. (2019). Urinary monitoring of neonicotinoid imidacloprid exposure to pesticide applicators. Sci. total Environ. 669, 721–728. doi:10.1016/j.scitotenv.2019.03.040
Testa, B., and Krämer, S. D. (2008). The biochemistry of drug metabolism--an introduction: Part 4. Reactions of conjugation and their enzymes. Chem. Biodivers. 5 (11), 2171–2336. doi:10.1002/cbdv.200890199
Thouvenin, I., Bouneb, F., and Mercier, T. (2017). Operator dermal exposure and protection provided by personal protective equipment and working coveralls during mixing/loading, application and sprayer cleaning in vineyards. Int. J. Occup. Saf. Ergon. 23, 229–239. doi:10.1080/10803548.2016.1195130
Tudi, M., Li, H., Li, H., Wang, L., Lyu, J., Yang, L., et al. (2022). Exposure routes and health risks associated with pesticide application. Toxics 10 (6), 335. doi:10.3390/toxics10060335
USEPA (2007). Prothioconazole: Human health risk assessment for proposed uses on Barley,Canola, chickpea, dried shelled peas and beans (except soybean), Lentils,Oilseed crops (except sunflower and safflower), peanut, wheat, and rice. Washington, D.C: United States Environmental Protection Agency, 20460.
Wang, X., Murison, J., Wang, J., Leong, G., Wu, Z. C., and Li, Q. (2021). Dermal exposure assessment to trinexapac-ethyl: A case study of workers in golf course in Hawaii, USA. Environ. Sci. Pollut. Res. 28, 1072–1076. doi:10.1007/s11356-020-10566-w
Wang, Z., Meng, Y., Mei, X., Ning, J., Ma, X., and She, D. (2020). Assessment of handler exposure to pesticides from stretcher-type power sprayers in orchards. Appl. Sci. 10 (23), 8684. doi:10.3390/app10238684
Wong, H. L., and Brown, C. D. (2021). Assessment of occupational exposure to pesticides applied in rice fields in developing countries: A critical review. Int. J. Environ. Sci. Technol. 18 (2021), 499–520. doi:10.1007/s13762-020-02834-6
Xie, Y., Li, L. Y. Z., Hao, W., Chang, J., Xu, P., Guo, B., et al. (2019a). Comparative toxicokinetics and tissue distribution of prothioconazole and prothioconazole-desthio in Chinese lizards (Eremias argus) and transcriptional responses of metabolic-related genes. Environ. Pollut. 247, 524–533. doi:10.1016/j.envpol.2019.01.055
Yang, F., Kai, W., Zhang, W., and Liu, F. (2013). Using the protective clothing to simulate the body dermal to measure the exposure of the operators in corn field. J. Agro-Environ. Sci. 32, 1979–1983. doi:10.11654/jaes.2013.10.011
Yu, X., Xing, L., and Lou, S. (2017). Operators’ health risk assessment of fungicide prothioconazole. Pesticide Sci. Adm. 38 (07), 37–43.
Zarn, J. A., Brüschweiler, B. J., and Schlatter, J. R. (2003). Azole fungicides affect mammalian steroidogenesis by inhibiting sterol 14 alpha-demethylase and aromatase. Environ.Health Perspect. 111 (3), 255–261. doi:10.1289/ehp.5785
Keywords: prothioconazole, prothioconazole-desthio, backpack sprayer, operator exposure, risk assessment
Citation: Li S, Mei C, Wu X, Li X and Pang S (2023) Health risk assessment following exposure of operators to backpack-sprayed prothioconazole and its major metabolite in wheat fields in China. Front. Environ. Sci. 11:1188916. doi: 10.3389/fenvs.2023.1188916
Received: 18 March 2023; Accepted: 15 June 2023;
Published: 07 August 2023.
Edited by:
Anabela Cachada, University of Porto, PortugalReviewed by:
Amir Waseem, Quaid-i-Azam University, PakistanMazhar Iqbal Zafar, Quaid-i-Azam University, Pakistan
Copyright © 2023 Li, Mei, Wu, Li and Pang. This is an open-access article distributed under the terms of the Creative Commons Attribution License (CC BY). The use, distribution or reproduction in other forums is permitted, provided the original author(s) and the copyright owner(s) are credited and that the original publication in this journal is cited, in accordance with accepted academic practice. No use, distribution or reproduction is permitted which does not comply with these terms.
*Correspondence: Xuefeng Li, 91030@cau.edu.cn; Sen Pang, pangsen7812@cau.edu.cn