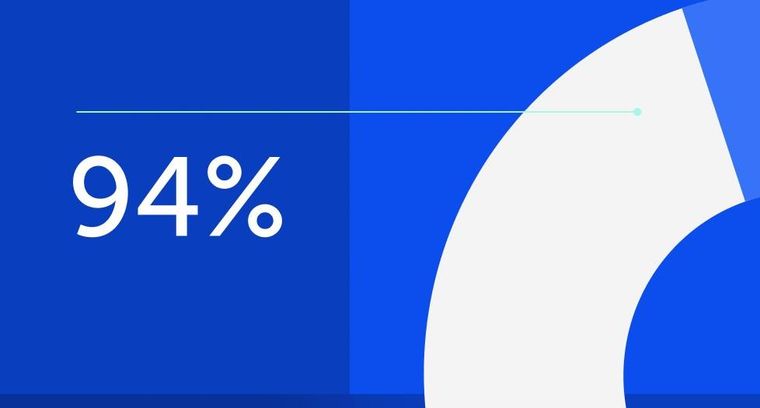
94% of researchers rate our articles as excellent or good
Learn more about the work of our research integrity team to safeguard the quality of each article we publish.
Find out more
ORIGINAL RESEARCH article
Front. Environ. Sci., 09 May 2023
Sec. Soil Processes
Volume 11 - 2023 | https://doi.org/10.3389/fenvs.2023.1173509
This article is part of the Research TopicSoil Health, Functions, and Ecosystem Services: Insights into Soil Parameters and Methods of IntegrationView all 7 articles
It is generally known that soil organic carbon (SOC) stocks tend to increase with an increase in C input, whereas the C sequestration efficiency (CSE), i.e., the conversion ratio of C input to SOC, differs depending on the amount and type of C input. However, there is still a need to better understand the impact of various fertilization practices on CSE. We studied the data from eight long-term experiments located in the main dryland region of China in order to comprehensively assess the key drivers of CSE in the plow layer considering nearly four decades of various fertilizer treatments, i.e., no fertilizer (CK); chemical nitrogen, phosphorus, and potassium (NPK/NP); chemical fertilizers plus manure (NPKM/NPM/NM); and straw (NPKS/NPS/NS). Our results showed that manure amendment had the most significant fertilization effect on SOC sequestration with an average CSE of 14.9%, which was significantly higher than that of chemical fertilization (9.0%) and straw return treatments (7.9%). In addition, manure amendment also had the highest average SOC increase rate of 684 kg C ha-1 yr-1. Variance partitioning analysis (VPA) illustrated that the CSE of the main dryland region of China was mostly controlled by edaphic characteristics (32.2%), especially the soil C/N ratio and clay content. VPA and structural equation modeling revealed that the magnitude and influencing factors driving CSE varied among different fertilizer treatments. Soil total N was the limiting factor for CSE in the CK treatment, whereas the soil C/N ratio and pH were the main explanatory factors for CSE in the long-term chemical NPK fertilizer treatment. The negative impact of C input from straw was the main driver of CSE under straw return treatments, though C input had a positive effect on the improvement of soil physical properties. However, when considering manure amendments, the improvement of soil nutrients and clay content controlled CSE, underlining the main positive direct effect of soil chemical properties. In a nutshell, our results recommend manure plus chemical fertilizers as a sustainable practice for improving the C sequestration rate and efficiency in dryland cropping systems.
Recently, enhancing soil organic carbon (SOC) sequestration in agroecosystems has been considered an important strategy for maintaining soil productivity and mitigating climate change globally (e.g., Amundson and Biardeau, 2019; Sun et al., 2020). It should be noted that considerable SOC losses, taking place continuously across many regions on Earth, are often characterized by either remarkable land use change or intensified agricultural practices, including the unreasonable intensive usage of fertilizers (Pugh et al., 2015; Chen et al., 2021). Generally, manure combined with mineral fertilizer has been considered an effective strategy to improve SOC storage because of the combined effect of i) enhanced residual C input by increasing the biomass production from the mineral fertilizers and ii) higher direct organic C input from the manure (Triberti et al., 2016; Jiang et al., 2018; Gross and Glaser, 2021). However, when considering the incorporation of these sources of C into the soil C pool, it is not only the quantity that matters but also the retention coefficient of those C inputs, which has been defined as carbon sequestration efficiency (i.e., CSE) (Kong et al., 2005; Stewart et al., 2007; Hua et al., 2014; Maillard and Angers, 2014). Recent studies have highlighted that regardless of the application rate, the effects of different types of fertilizers (either mineral or organic types) on SOC sequestration are remarkably different (Aguilera et al., 2013; Li et al., 2020). Consequently, an assessment of region and/or fertilizer treatment-specific CSE could be very useful to identify a better management strategy to enhance the soil’s C sequestration ability (Hua et al., 2014; Liang et al., 2019).
Research indicates that CSE depends on climate conditions, soil types, and fertilizer practices (Triberti et al., 2008; Yan et al., 2013; Zhao et al., 2016; Wang R. et al., 2020). More specifically, faster mineralization of C input, and, hence, lower CSE, usually appears in regions characterized by both higher temperature and precipitation amounts because of the typically more active microbial community in these environments (Liu et al., 2019; Chen et al., 2021). Soils with low initial SOC contents might have greater potential and efficiency to sequester freshly added organic C because these soils are still far from their C saturation levels (Stewart et al., 2008). The CSE of soils with higher clay content could be significantly higher due to the adsorption ability of soil clay particles and, hence, the formation of stronger organic–mineral complexes as compared to soils with more sand and silt (Ekschmitt et al., 2005; Hua et al., 2014). A higher soil bulk density (BD) typically results in a lower SOC turnover and, therefore, a higher CSE because of the generally slower soil–atmosphere–water cycling associated with poor air permeability and restricted hydraulic conductivity (Thomsen et al., 1999; Lu et al., 2018). Soil pH could affect CSE indirectly by controlling SOC solubility and affecting plant nutrient absorption and microorganism growth (Evans et al., 2008; 2012). In addition, CSE might vary significantly depending on the different magnitudes of SOC changes in response to animal manure and crop residue amendments (Liu et al., 2014; Maillard and Angers, 2014), which mainly depends on the quality of the newly added C styles. The soil with composted cattle organic fertilizer, which had a high amount of stabilized component, lignin, could have a higher CSE compared to soil with uncomposted pig material (Hua et al., 2014). However, it was lower than that of soils with dairy manure application (Wang R. et al., 2020). Moreover, the C input amount has been suggested to negatively affect CSE (Stewart et al., 2008). The CSE of soil with a high rate of wheat straw return was lower than that of soil with a half input rate (Hua et al., 2014), and a similar result was also found in soil with manure amendments (Wang R. et al., 2020). The amounts of added substrates as related to microbial biomass C could also affect C sequestration by stimulating microbial growth and altering the composition of the microbial community (Blagodatskaya and Kuzyakov, 2008). However, there is, in particular, a lack of precise knowledge concerning the impact of different fertilizers on CSE. In this context, it is important to note that a comparison of CSEs among regions and/or fertilizer treatments is very difficult due to the existence of complex interactions between climate, edaphic, and fertilization factors (Liang et al., 2016). Hence, a comprehensive study of the individual effects of those factors on CSE under long-term fertilization is urgently needed.
The long-term experiment network of China (from 1979/1989), based on region-specific common soil and fertilizer practices, has effectively monitored the evolution of SOC stocks (Jiang et al., 2014). Hence, this network could be considered a good opportunity to study whether a given fertilizer treatment can sustain or improve C sequestration in the long term. The well-documented and quite detailed records concerning fertilizer management, soil properties, and climates also support a comprehensive assessment of the drivers of CSE. In addition, CSE is a function of fertilization duration (Smith, 2004; Stewart et al., 2008). When considering a period of 30–40 years of fertilizer application, many studies indicate that observed changes in SOC are significantly, positively, and linearly correlated with C input, resulting in a constant CSE with the limited range of C input (Majumder et al., 2008; Bhattacharyya et al., 2010; Zhang et al., 2010; Jiang et al., 2014; Maillard and Angers, 2014; Wang R et al., 2020). The present study aims to 1) quantify CSE in the plow layer (0–20 cm) and 2) identify the main drivers of CSE considering eight long-term experiments in the drylands of China.
For this study, eight classical long-term experiments across a wide range of pedo-climatological conditions were selected, which were based in Gongzhuling (GZL), Changping (CP), Zhengzhou (ZZ), Tianjin (TJ), Xuzhou (XZ), Pingliang (PL), Yangling (YL), and Urumqi (UM) (Figure 1). Detailed information about these particular study sites can be found in the studies by Jiang et al. (2018) and Jiang et al. (2014), which are located in major wheat and maize planting areas. However, key background information, including the locations, initial soil properties, cropping systems, and climates, can be found in Table1 and Supplementary Table S1.
TABLE 1. Initial soil characteristics of plow layer of long-term fertilization experiments at the eight dryland long-term experimental sites across China.
A total of four different fertilizer treatments, present in all of the eight studied sites, were considered, i.e., 1) no fertilizer (CK), 2) balanced application of chemical nitrogen (N), phosphorus (P), and potassium (K) fertilizers (NPK), 3) chemical fertilizers combined with manure (NPKM), and 4) chemical fertilizers combined with straw (NPKS) (except for XZ) (Supplementary Table S2). Especially, CK, NP, NPM, and NPS were used in PL, and no K was applied in PL due to its high soil K content. The 3) and 4) treatments in TJ were NM and NS because of the full supply of P and K from manure and straw.
The total amount of N applied (chemical + organic) was equal for the NPK, NPKM, and NPKS treatments at GZL, ZZ, YL, and UM sites, but this amount was higher in NPKM/NPM/NM and NPKS/NPS/NS treatments at the other sites due to an additional manure application or straw return. The types of manure depended on local availability (Supplementary Table S2). Considering the straw return treatment, crop residues were chopped and put into the soil by rotary tillage. These crop residues were only returned during the corn season in CP, ZZ, XZ, and YL.
Soil samples from the plow layer (0–20 cm) for all experiments were collected approximately 15 days after harvest (the corn season in double-cropping systems) in each long-term experiment (Table 1). The soil was air-dried and then passed through a 2 mm sieve. A part of the sub-sample was dried, crushed, and sieved at 0.25 and 1.0 mm for the determination of total nutrients and available nutrients, respectively. The concentration of SOC was analyzed with dichromate oxidation by a modified Walkley and Black method (i.e., heated for 12 min at 220°C), and a correction factor of 1.1 was applied (Walkley and Black, 1934; Xu H et al., 2016). Total nitrogen (TN), phosphorus (TP), and potassium (TK) were determined by the classical methods of Black (1965), Murphy and Riley (1962), and Kundsen et al. (1982), respectively. Available N was measured following the study by Lu (1999). Available P (Olsen P) was quantified according to the study by Olsen et al. (1954) and available K by Shi (1976). The clay content, soil pH (considering a 1:1 water/soil mixture), and BD were also measured following the study by Lu (1999).
In each site, soil C sequestration efficiency was calculated by using following equations.
The SOC stocks (
where
The C inputs (
where
Considering the shoot biomass, we assumed it was approximately equal to straw dry matter production. When sites had not measured straw production (XZ), it was established as follows:
where
The root biomass could be estimated as follows:
where RSR is the root-to-shoot ratio (Supplementary Table S3).
The soil C sequestration rate (
where
The carbon sequestration efficiency (CSE, %) is the conversion ratio of unit carbon input to SOC stock.
where
To gain insight into factors controlling CSE among the studied dryland soils under different long-term fertilizer treatments, climate variables, edaphic properties, and management factors were selected for each site. Mean annual temperature (MAT) and mean annual precipitation (MAP) as main climate factors influencing crop growth and C decompositions were collected from the National Meteoritical Information Center (http://data.cma.cn/). Considering edaphic factors, soil chemical properties (i.e., measured total N stock (TN), available N (AN), total P (TP), Olsen P (AP), total K (TK), available K (AK), soil C/N ratio, initial SOC content, and pH) and physical properties (i.e., clay content and BD) were selected for further analysis. Regarding the management factors, C inputs from crop residue (stubble, root, and rhizosphere) and organic manure/straw return were calculated for further analysis.
Figure 2 presents the methodological flowchart of this study. One-way analysis of variance (ANOVA) and the least significant difference (LSD; p < 0.05) test were applied to compare the fertilization effects on the SOC sequestration rate, annual C input, and CSE within an individual site. One-way ANOVA was also used to identify the duration when the CSE of studied treatments had no significant difference with the latest record year of the experiment. Paired-sample t-tests were used to compare the difference in CSE between NPK/NP and CK and NPKM/NPM/NM and NPKS/NPS/NS across different sites, respectively. The statistical analyses were performed with SPSS 22.0 (SPSS, IBM, 2015; Chicago, United States). Graphs were prepared using Origin professional version 2019 (OriginLab, Northampton, United States).
Variance partitioning analysis (VPA) was applied to quantify the contributions (%) of i) the total selected edaphic, C input, and climate factors and their interactions, as well as ii) each individual variable within each factor when considering the CSE differences among different fertilizer treatments and sites. Only factors that passed the test of collinearity were considered when conducting the VPA using R-software, version 4.1.2. Subsequently, selected soil variables that significantly (p < 0.05) influenced CSE, following the results of the VPA, were used to build the structural equation modeling (SEM). This SEM was used to investigate the direct and indirect factors regulating CSE under manure amendments and straw return treatments. The chi-square statistic to the respective degrees of freedom ratio (χ2/df < 3 for a good fit and <5 for a reasonable fit), probability level (p > 0.05), comparative fit index (CFI >0.9), root mean square error of approximation (RMSEA <0.08), and low AIC was used to assess the overall performance of the model (Browne and Cudeck, 1992).
Over a time span of 30–40 years of fertilization, chemical fertilizers combined with manure resulted in significantly higher annual SOC sequestration rates (684 kg C ha-1 yr-1) in the top 20 cm of the soil as compared to other treatments (p < 0.05) (Table 2). Furthermore, the average annual SOC sequestration rates of chemical fertilizers with straw return (i.e., 333 kg C ha-1 yr-1) were higher than those of only chemical fertilizers (244 kg C ha-1 yr-1).
TABLE 2. Average SOC change rate (kg C ha-1 yr-1) of typical croplands under different long-term fertilizer treatments.
The annual C input from crop residues was significantly higher in the chemical fertilizers plus manure or straw treatments (i.e., 0.9–2.9 t C ha-1 yr-1) than with CK and chemical treatments (i.e., 0.6–2.3 t C ha-1 yr-1) (Figure 3). The C inputs from manure and straw return were 0.9–5.3 t C ha-1 yr-1 (Figure 3). Generally, the average annual C input under an individual treatment from the highest to the lowest value was as follows: NPKM/NPM/NM (2.6–7.5 t C ha-1 yr-1) > NPKS/NPS/NS (2.8–5.6 t C ha-1 yr-1) > NPK/NP (1.4–3.9 t C ha-1 yr-1) > CK (0.9–1.5 t C ha-1 yr-1) (p < 0.05). However, this trend was slightly different in GZL, ZZ, and PL, where the highest annual C input was found in the NPKS treatment.
FIGURE 3. Average annual C input from different sources under different fertilizer treatments at all long-term experimental sites. Numbers with different lowercase letters indicate significant difference (p < 0.05) for total annual C input under different treatments within an individual site.
After calculating the annual CSE of each fertilizer treatment in each long-term experiment (Supplementary Figure S1), we took an average value of the last 9 years in which CSE had no significant difference with the latest record year for all sites (Table 3, 4, Supplementary Table S4). Generally, the CSE in GZL, UM, PL, and YL, located across Northeast and Northwest China and typically characterized by high clay contents (i.e., 17–34%), was higher (i.e., CSE of 4.6%–27.7%) than those in other sites located across the North China Plain with typically lower clay contents (except TJ) (i.e., CSE of 3.5%–15.2%) (Supplementary Table S1, Table 3). The CSE of chemical NPK fertilizers, with an average value of 9.0%, was significantly lower than that of treatments with manure amendments, with an average of 14.9% (p < 0.01) (Tables 3, 4). However, NPK/NP had a significantly higher CSE as compared to NPKS/NPS/NS (7.9%) (p < 0.01) (Tables 3, 4; Supplementary Table S5).
TABLE 3. Average carbon sequestration efficiency (%) of typical drylands under different long-term fertilizer treatments.
The VPA results indicated that 37.9% of the variations could be explained by edaphic, C input, and climate factors and their interactions across all sites and treatments (Figure 4). However, the edaphic factors contributed the most (32.2%) to the CSE of the studied region, with, in particular, the soil C/N ratio (4.9%) and clay content (3.8%), followed by C input (3.2%) and climate (2.8%). Similarly, the contribution of the edaphic factor (21.4%–50.4%) was much higher than that of the C input (2.1%–21.6%) and climate factors (0.3%–13.0%) under each fertilizer treatment or site (Figure 4). For the contribution of each individual variable within each factor, soil C/N ratio and clay content were important controllers of CSE as indicated by significant relations for most sites (except for GZL and UM for C/N and CP and XZ for clay) (Table 5). Furthermore, the soil C/N ratio only had a significant influence on the CSE of CK and chemical fertilizers (Table 5).
FIGURE 4. Proportional contributions (%) of edaphic, climate, and C input factor and their interactions on CSE under each treatment and each dryland long-term experimental site and across all fertilizer treatments and sites in China based on VPA.
TABLE 5. Proportional contribution (%) of individual edaphic, carbon input, and climate factors on variance of carbon sequestration efficiency based on variance partitioning analysis.
For different treatments, the VPA results showed that TN was the main controller for CSE of CK, with a much higher contribution of 9.6% as compared to other variables that had a significant effect on CSE (1.4%–5.3%, p < 0.05) (Table 5). When considering chemical NPK treatment, soil C/N ratio (5.1%) and pH (1.3%) were the main explanatory factors (Table 5). Furthermore, many edaphic factors had a significant effect on CSE, with contributions ranging between 0.8% and 3.9% and between 1.4% and 5.2% for manure and straw amendments, respectively (Table 5) (p < 0.05). The SEM illustrated that the selected variables were able to predict 66% and 29% of variances in CSE under NPKM/NPM/NM and NPKS/NPS/NS of the studied region, respectively (Figure 5). Soil chemical properties had the largest direct effect on CSE for manure amendments with a positive standardized coefficient of 0.76, whereas C input had the largest indirect effect with a positive standardized coefficient of 0.44 through its association with soil chemical factors and clay content (Figures 5A, C, Supplementary Figure S2A, C). C input had the largest direct effect on CSE with a negative standardized coefficient of −0.69 for straw return treatments, whereas climate and C input had similar indirect effects with a positive standardized coefficient of 0.16 and 0.14, respectively, through their interactions with soil chemical and physical properties (Figures 5B, D, Supplementary Figure S2B, D).
FIGURE 5. Structural equation model showing the effects of climate, carbon (C) input, soil chemical properties, and soil physical properties on soil CSE under NPKM/NPM/NM (A,C) and NPKS/NPS/NS (B,D). The red and blue lines in (A) and (B) represent the positive and negative effects, respectively. The line width and the numbers above the lines (standardized path coefficient) correspond to the strength of the path. Solid and dashed lines represent significant and non-significant paths, respectively (*p < 0.05, **p < 0.01, and ***p < 0.001). Multi-layer rectangles represent the first component from the principal component analysis of climate factors, soil chemical, and physical properties, and C inputs, and the vertical red (/blue) arrows within it represent the positive (/negative) relationships between adjacent variables and the corresponding PC1. MAT: mean annual temperature, MAP: mean annual precipitation, SOCini: initial SOC content, TN: soil total nitrogen content, AN: available N content, TP: total phosphorus content, AP: Olsen P content, TK: total potassium content, AK: available K content, Clay: soil clay content, BD: soil bulk density, pH: soil pH, Cresidual: C input from stubble, root, and exudates, Cmanure: C input from manure, and Cstraw: C input from straw. χ2: chi-square values, d.f.: degree of freedom, GFI: goodness of fit index, and RMSEA: the root mean square error of approximation.
Over nearly 40 years, the combined application of chemical fertilizers and manure significantly (p < 0.05) increased SOC stocks in the top 20 cm of the dryland soils of China under both single-cropping and double-cropping systems (Table 2), which is in agreement with previous studies (Liang et al., 2016; Jiang et al., 2018; Ren et al., 2021). The average SOC sequestration rates (i.e., 148-684 kg C ha-1 yr-1) across our studied sites for all fertilizer treatments were very close to the results of 30–590 kg C ha-1 yr-1 derived by 45 field trials (20–37 years) across Chinese drylands, as reported by Ren et al. (2021). The highest SOC sequestration rate under the NPKM treatment can be explained by the fact that this treatment is characterized by the highest average plant-derived C-input (stubble + root + rhizosphere) of 1.7–4.0 t C ha-1 yr-1 in combination with the additional organic C input from manure (0.85–4.05 t C ha-1 yr-1) (Figure 3). Moreover, an accurate C input estimation is important for the analysis of CSE differences among different treatments. In our study, the annual wheat- and maize-derived C inputs for all treatments ranged between 0.51 and 2.46 t C ha-1 yr-1 and between 0.72 and 2.63 t C ha-1 yr-1, respectively (Supplementary Table S6). Those are comparable to C input values of 0.58–2 .86 t C ha-1 yr-1 for wheat and of 0.90–2.39 t C ha-1 yr-1 for maize reported for China, Belgium, France, and Canada, despite the different methods for C input calculation (Bolinder et al., 2007; Meersmans et al., 2013; Jiang et al., 2018).
Edaphic characteristics, especially soil C/N ratio and clay content, were the main factors driving CSE after 30–40 years of fertilization across the main dryland regions of China (Figure 4; Table 5). The soil C/N ratio is an indication of soil quality as it is typically inversely proportional to the decomposition rate of SOC (Zinn et al., 2007). Agricultural management practices, especially fertilization, tend to affect this ratio in an agroecosystem (Deng et al., 2020). In this study, compared to CK, the long-term intensified N fertilizer application slightly decreased the soil C/N ratio (Supplementary Figure S3). The reduction in the soil C/N ratio usually suggested a potential decrease in SOC and GHG emissions with high mineralization rates (Majumder et al., 2008; Xu X et al., 2016). However, the soil C/N ratio of manure amendments was significantly higher than that of NPK/NP (p < 0.05) and slightly higher than that of chemical fertilizers plus straw return (Supplementary Figure S3). A meta-analysis of globally published studies indicated that the quality of the organic amendment was the main driver determining CSE (Maillard and Angers, 2014), which might be the major reason explaining the difference in soil C/N ratios and their effects on CSE. It seems that the high C/N ratio of straw (i.e., on average 67 for wheat and 50 for maize across the entire study area) contributed to the slow decomposition of organic matter and the deficiency of available mineral nitrogen due to the competition of microbes with crops (Triberti et al., 2016), which, therefore, generally results in increasing SOC stocks (Mahmoodabadi and Heydarpour, 2014). However, organic substances originating from above-ground residues are more resistant to decomposition than those of crop roots (Rasse et al., 2005), which could impede the high CSE. The low C/N ratio of manure (i.e., on average 22 across the entire study area) might result in increased organic matter decomposition, whereas, on the other hand, the input of direct organic C and the associated formation of stable soil organic complexes from the resistant constituents in manure (e.g., lignin and polyphenol) might lead to an accumulation of SOC (Majumder et al., 2008). Furthermore, the application of manure, compared to straw return and chemical NPK applications, was beneficial to the enhancement of the size and functional group diversity of soil microorganisms that contributed to OC sequestration, like Proteobacteria (Wang Q. et al., 2020), and inherent C substance decomposition, like Dothideomycetes (Freedman et al., 2015). Therefore, the mechanisms and efficiencies for the sequestration of C into the SOC pool among the studied treatments were significantly different.
In our study, the high clay content generally contributed to high CSEs. The CSE in GZL, UM, PL, and YL with high clay contents (17%–34%) (except TJ with high contributions of C input and pH to CSE) was higher (4.5%–27.6%) than that of CP, ZZ, and XZ sites (3.5%–15.2%) with relatively low clay contents (6%–13%) (Table 1, Supplementary Table S1). The SEM of NPKM also showed that clay content had a positive effect on CSE (Figures 5A, C). A study focused on CSE in Vertisols with a clay content of around 40% highlighted that soil with a high clay content has typically high CSE irrespective of the type of C input (wheat straw, pig manure, or cattle manure) (Hua et al., 2014). Soil clay influences the sequestration of organic C due to the strong adsorption capacity of the clay particles by organo-mineral bonding reactions (Ekschmitt et al., 2005; Chen et al., 2010). Hence, soils with high clay contents are generally characterized by low SOC decomposition rates, as SOC could be chemically stabilized after being absorbed into clay minerals (Franzluebbers et al., 1996; Mikha and Rice, 2004). This chemical protection of SOC by association with organo-mineral complexes was reinforced with the increase in clay content (Hassink, 1997).
Notably, the main controlling factor of CSE varied considerably among different treatments (Table 5; Figure 5). Based on the results of VPA, soil TN was the most important factor for the CSE of CK. When considering the CK treatment, N supply depended only on the basic soil fertility, whereas N as a limiting factor on crop growth and below-ground C transformations has a significant influence on the amount of C input and, thus, the CSE (Liang et al., 2016). The soil C/N ratio (5.1%) and pH (1.3%) were the main explanatory variables under long-term chemical NPK fertilization (Table 5). The long-term chemical N application caused a decrease in the soil C/N ratio (Supplementary Figure S3), which most probably accelerated soil microorganisms’ mineralization of carbon sources from humus and led to the decomposition of organic carbon (Brown et al., 2014). Liang et al. (2016) showed that pH was an important factor explaining CSE variations in drylands at sites located in Northwest China because pH was significantly inversely related to SOC content. Moreover, the decrease in pH due to the intensified mineral N fertilizer application impeded the abundance and activity of microorganisms, resulting in a reduced SOC quality (e.g., polyphenol content) (Kemmitt et al., 2006) and influencing organic C turnover and accumulation due to reduction of microbial mineralization (Wong et al., 2010).
For treatments combining manure with chemical fertilizers, our results presented that soil chemical properties had the largest direct positive effect on CSE, whereas C input had the largest indirect positive effect, through soil chemical properties and clay content, on CSE (Figure 5, Supplementary Figures S2A, C). Generally, manure application was recognized as a recommended practice for improving soil fertility and soil structure because of the beneficial impacts of the additional organic matter and nutrient inputs (Guo et al., 2019). Previous studies have indicated that manure amendments led to greater retention of total C, N, P, and K in the soil under wheat and maize cropping systems across China (Jiao et al., 2006; Su et al., 2006), which in turn provided favorable conditions for crop growth and microbial activity. A summary of 85 peer-reviewed articles in the last three decades clarified that manure combined with NPK chemical fertilizers could more significantly increase the activity in urease, phosphatase, and invertase, which are strongly associated with soil organic matter sequestration, as compared to CK, NPK, and NPKS (Miao et al., 2019). Additionally, recent research found that manure combined with NPK chemical fertilizers significantly increased the SOC stock and the macro-aggregate component associated with C (Yan et al., 2013; Liu et al., 2019). Moreover, manure amendments seem to promote aggregate stability significantly as compared with long-term chemical fertilizers or no fertilizer treatments (Haynes and Naidu, 1998; Yan et al., 2013; Liu et al., 2019). In this respect, it is important to note that Liu et al. (2019) showed that the CSE of macro-aggregates was greater than that of other physical fractions, and as such, this can be seen as a reason to why the CSE in treatments with manure amendments is higher. The latter can be explained more specifically as follows: First, manure directly increased mycorrhizal hyphae that, as colloids, could be associated with the mineral fraction of the soil in order to bind micro-aggregates into macro-aggregates (Bronick and Lal, 2005). Second, increased microbial biomass and associated activity due to manure amendments might lead to greater production of microbial-derived agents (Six et al., 1999; Pan et al., 2009). Hence, manure applications could result in more stable SOC in both newly formed macro-aggregates and micro-aggregates (Fonte et al., 2009).
For chemical fertilizers plus straw return treatment, the negative effect of C input was the main driver of CSE (Figure 5, Supplementary Figures S2B, D). The negative effect can be explained by the following reasons: 1) the increased mineralization of native soil-origin matter (SOM) was stimulated by fresh OC input following straw return. More specifically, the added residuals could have increased the concentration of easily available compounds (e.g., phytosterols and policosanol), which are essential sources for microorganisms and, thus, stimulate microbial activity, causing a positive priming effect (Kuzyakov et al., 2000; Wu et al., 2019). 2) Most of the added straw-derived C would be mineralized eventually. Studies with 13C-labeled maize straw have estimated that about 42%–79% of the straw-derived C got mineralized, and as such, only c. 10% of this C input was transformed into particulate organic carbon and more stable SOC fractions (De Troyer et al., 2011; Yang et al., 2012; An et al., 2015). 3) Slow biodegradation of crop straw and low stabilization of litter-derived C are both unfavorable to obtain high C sequestration efficiency (Poeplau et al., 2015; Zhao et al., 2016; Berhane et al., 2020). The root-derived C could have more opportunity for physico-chemical interactions with soil particles and, hence, could be much more efficiently incorporated into the stable soil C pools (Rasse et al., 2005), which might explain the significant difference between NPK/NP and NPKS/NPS/NS in the present study. Moreover, our results indicated lower CSE in straw plus chemical fertilizers as compared to manure amendments, highlighting that the type of OC input influences CSE. A 34-year maize–wheat rotation in Italy gave a similar result with more than doubled CSE under manure combined N fertilizer than that of straw returning (Triberti et al., 2008). In addition, another study considering a long-term dryland rotation in Northeast China showed that CSE was 30% higher under NPKM treatment than under NPKS treatment (Zhao et al., 2016). Hence, the type of organic amendment chosen is crucial to increasing soil carbon sequestration.
In our study, the CSE values of CK and chemical fertilizers ranged from 3.5% to 14.0% in the drylands of China, which are close to the results of 2.1%–17.0% derived from the study by Zhao et al. (2016) but lower than those of the study by Wang R. et al. (2020), i.e., 14-32%. The CSE of NPKM/NPM/NM in our study generally (except GZL, Table 3) matched the range of 12% ± 4%, which was a global manure-C retention coefficient established by a meta-analysis based on 130 observations considering an average of 18 years of application (Maillard and Angers, 2014). In addition to the impact of edaphic properties, climate, and C input on CES variations, the uncertainties in our study may also partly intensify the difference. We obtained the CSE of the different treatments by making two assumptions. More precisely, we assumed that i) the short-term effect of the priming effect after exogenous organic matter addition would be negligible for long-term carbon sequestration and ii) the soil respiration rates before and after C addition were similar during long-term fertilization. Moreover, we estimated C input via root and stubble by using a fixed ratio of the above-ground biomass (Supplementary Table S3), and the C contents of manure and crop’s shoot and root were not measured annually. The effect of different sources of manure on CSE was not considered in this study with limited data, even if a three-decade long-term experiment observed that the soil CSE of cattle manure application was higher than that of soil with pig manure (Hua et al., 2014). However, the main reason was that the cattle manure was composted and had a high lignin content of 23.7%, which was considered a stabilized component of SOC, influencing its pool size and turnover (Ghosh et al., 2004). When considering the straw return in different regions, the plant residue chemistry converged after the straw decomposed over nearly a decade despite different climate zones (Wang et al., 2023), and the initial quality did not control the long-term decomposition of SOC (Schmidt et al., 2011). Thus, we should pay more attention to whether the property of manure is one of the determining factors of CSE under long-term fertilization. Although these elements are quite common uncertainties in long-term experiment studies, it should be noted that the estimate of rhizosphere C input may help us reduce the uncertainty.
Additionally, there was a significant linear relation between cumulative C input and SOC stock change for each treatment when combining the data from all sites (Supplementary Figure S4). Based on the fact that there was a curvilinear relationship between SOC change and C input rates obtained by data from 14 long-term experiments with durations of 12–96 years (Stewart et al., 2007), a small range of C input levels (fertilization durations) will not necessarily reflect the full range of linear-to-asymptotic behaviors when soil is subjected to C saturation. Future studies should focus on CSE characteristics and its main controlling factors when a large amount of C has been accumulated.
Our study revealed that nearly four decades of combined chemical NPK fertilizers and manure application significantly promoted SOC sequestration rate and efficiency in the drylands of China. The improvement of soil chemical properties, which was a consequence of the manure application, was the main driver of soil carbon sequestration efficiency in manure amendment. Additionally, the soil C/N ratio and clay content contributed most to CSE across the main dryland regions of China. The controlling factors of CSE varied considerably among the different treatments. Soil total N was the limiting factor for CSE of CK by controlling C input, whereas the soil C/N ratio and pH were the main explanatory factors of the long-term chemical NPK fertilization treatment. The negative impact of C input was the main driver of CSE with straw return due to low humification for crop straw and low stabilization of straw-derived carbon. In conclusion, our findings highlight that different factors are driving SOC sequestration efficiency when considering different fertilization treatments across the main drylands of China. Hence, our study can be considered as a reference to promote soil carbon sequestration under long-term fertilization in wheat and corn production regions.
The original contributions presented in the study are included in the article/Supplementary Material; further inquiries can be directed to the corresponding authors.
SL: conceptualization, methodology, software, writing—original draft, and writing—review and editing. NS: conceptualization, supervision, and funding acquisition. SW: methodology and software. BL and GC: methodology and supervision; JM: methodology and writing—review and editing. LW: methodology. MX: conceptualization, supervision, and funding acquisition. All authors contributed to the article and approved the submitted version.
This study was supported by the National Natural Science Foundation of China (42177341). SL was supported by the China Scholarship Council (No. 202003250099).
The authors acknowledge the Chinese Academy of Agricultural Sciences–Gembloux Agro-Bio Tech joint PhD program and all the colleagues from the long-term fertilization experimental sites for their unremitting assistance.
The authors declare that the research was conducted in the absence of any commercial or financial relationships that could be construed as a potential conflict of interest.
All claims expressed in this article are solely those of the authors and do not necessarily represent those of their affiliated organizations, or those of the publisher, the editors, and the reviewers. Any product that may be evaluated in this article, or claim that may be made by its manufacturer, is not guaranteed or endorsed by the publisher.
The Supplementary Material for this article can be found online at: https://www.frontiersin.org/articles/10.3389/fenvs.2023.1173509/full#supplementary-material
Aguilera, E., Lassaletta, L., Gattinger, A., and Gimeno, B. S. (2013). Managing soil carbon for climate change mitigation and adaptation in mediterranean cropping systems: A meta-analysis. Agric. Ecosyst. Environ. 168, 25–36. doi:10.1016/j.agee.2013.02.003
Amundson, R., and Biardeau, L. (2019). Correction: Opinion: Soil carbon sequestration is an elusive climate mitigation tool. Proc. Natl. Acad. Sci. U. S. A. 116, 13143. doi:10.1073/pnas.1908917116
An, T., Schaeffer, S., Zhuang, J., Radosevich, M., Li, S., Li, H., et al. (2015). Dynamics and distribution of 13C-labeled straw carbon by microorganisms as affected by soil fertility levels in the Black Soil region of Northeast China. Biol. Fertil. Soils 51, 605–613. doi:10.1007/s00374-015-1006-3
Berhane, M., Xu, M., Liang, Z., Shi, J., Wei, G., and Tian, X. (2020). Effects of long-term straw return on soil organic carbon storage and sequestration rate in North China upland crops: A meta-analysis. Glob. Chang. Biol. 26, 2686–2701. doi:10.1111/gcb.15018
Bhattacharyya, R., Prakash, V., Kundu, S., Srivastva, A. K., Gupta, H. S., and Mitra, S. (2010). Long term effects of fertilization on carbon and nitrogen sequestration and aggregate associated carbon and nitrogen in the Indian sub-Himalayas. Nutr. Cycl. Agroecosyst. 86, 1–16. doi:10.1007/s10705-009-9270-y
Black, C. A. (1965). Methods of soil analysis. Part 2, Chemical and microbiological properties. Madison, Wisconsin: American Society of Agronomy: Soil Science Society of America.
Blagodatskaya, E., and Kuzyakov, Y. (2008). Mechanisms of real and apparent priming effects and their dependence on soil microbial biomass and community structure: Critical review. Biol. Fertil. Soils 45, 115–131. doi:10.1007/s00374-008-0334-y
Bolinder, M. A., Angers, D. A., and Dubuc, J. P. (1997). Estimating shoot to root ratios and annual carbon inputs in soils for cereal crops. Agric. Ecosyst. Environ. 63, 61–66. doi:10.1016/S0167-8809(96)01121-8
Bolinder, M. A., Janzen, H. H., Gregorich, E. G., Angers, D. A., and VandenBygaart, A. J. (2007). An approach for estimating net primary productivity and annual carbon inputs to soil for common agricultural crops in Canada. Agric. Ecosyst. Environ. 118, 29–42. doi:10.1016/j.agee.2006.05.013
Bronick, C. J., and Lal, R. (2005). Soil structure and management: A review. Geoderma 124, 3–22. doi:10.1016/j.geoderma.2004.03.005
Brown, K. H., Bach, E. M., Drijber, R. A., Hofmockel, K. S., Jeske, E. S., Sawyer, J. E., et al. (2014). A long-term nitrogen fertilizer gradient has little effect on soil organic matter in a high-intensity maize production system. Glob. Chang. Biol. 20, 1339–1350. doi:10.1111/gcb.12519
Browne, M. W., and Cudeck, R. (1992). Alternative ways of assessing model fit. Sociol. Methods Res. 21, 230–258. doi:10.1177/0049124192021002005
Chen, X., Hu, Y., Xia, Y., Zheng, S., Ma, C., Rui, Y., et al. (2021). Contrasting pathways of carbon sequestration in paddy and upland soils. Glob. Chang. Biol. 27, 2478–2490. doi:10.1111/gcb.15595
Chen, Y., Zhang, X., He, H., Xie, H., Yan, Y., Zhu, P., et al. (2010). Carbon and nitrogen pools in different aggregates of a Chinese Mollisol as influenced by long-term fertilization. J. Soils Sediments 10, 1018–1026. doi:10.1007/s11368-009-0123-8
De Troyer, I., Amery, F., Van Moorleghem, C., Smolders, E., and Merckx, R. (2011). Tracing the source and fate of dissolved organic matter in soil after incorporation of a 13C labelled residue: A batch incubation study. Soil Biol. biochem. 43, 513–519. doi:10.1016/j.soilbio.2010.11.016
Deng, X., Ma, W., Ren, Z., Zhang, M., Grieneisen, M. L., Chen, X., et al. (2020). Spatial and temporal trends of soil total nitrogen and C/N ratio for croplands of East China. Geoderma 361, 114035. doi:10.1016/j.geoderma.2019.114035
Ekschmitt, K., Liu, M., Vetter, S., Fox, O., and Wolters, V. (2005). Strategies used by soil biota to overcome soil organic matter stability - why is dead organic matter left over in the soil? Geoderma 128, 167–176. doi:10.1016/j.geoderma.2004.12.024
Evans, C. D., Goodale, C. L., Caporn, S. J. M., Dise, N. B., Emmett, B. A., Fernandez, I. J., et al. (2008). Does elevated nitrogen deposition or ecosystem recovery from acidification drive increased dissolved organic carbon loss from upland soil? A review of evidence from field nitrogen addition experiments. Biogeochemistry 91, 13–35. doi:10.1007/s10533-008-9256-x
Evans, C. D., Jones, T. G., Burden, A., Ostle, N., Zieliński, P., Cooper, M. D. A., et al. (2012). Acidity controls on dissolved organic carbon mobility in organic soils. Glob. Chang. Biol. 18, 3317–3331. doi:10.1111/j.1365-2486.2012.02794.x
Fonte, S. J., Yeboah, E., Ofori, P., Quansah, G. W., Vanlauwe, B., and Six, J. (2009). Fertilizer and residue quality effects on organic matter stabilization in soil aggregates. Soil Sci. Soc. Am. J. 73, 961–966. doi:10.2136/sssaj2008.0204
Franzluebbers, A. J., Haney, R. L., Hons, F. M., and Zuberer, D. A. (1996). Active fractions of organic matter in soils with different texture. Soil Biol. biochem. 28, 1367–1372. doi:10.1016/S0038-0717(96)00143-5
Freedman, Z. B., Romanowicz, K. J., Upchurch, R. A., and Zak, D. R. (2015). Differential responses of total and active soil microbial communities to long-term experimental N deposition. Soil Biol. biochem. 90, 275–282. doi:10.1016/j.soilbio.2015.08.014
Ghosh, P. K., Ramesh, P., Bandyopadhyay, K. K., Tripathi, A. K., Hati, K. M., Misra, A. K., et al. (2004). Comparative effectiveness of cattle manure, poultry manure, phosphocompost and fertilizer-NPK on three cropping systems in vertisols of semi-arid tropics. I. Crop yields and system performance. Bioresour. Technol. 95, 77–83. doi:10.1016/j.biortech.2004.02.011
Gross, A., and Glaser, B. (2021). Meta-analysis on how manure application changes soil organic carbon storage. Sci. Rep. 11, 5516–5613. doi:10.1038/s41598-021-82739-7
Guo, Z., Zhang, J., Fan, J., Yang, X., Yi, Y., Han, X., et al. (2019). Does animal manure application improve soil aggregation? Insights from nine long-term fertilization experiments. Sci. Total Environ. 660, 1029–1037. doi:10.1016/j.scitotenv.2019.01.051
Hassink, J. (1997). The capacity of soils to preserve organic C and N by their association with clay and silt particles. Plant Soil 191, 77–87. doi:10.1023/A:1004213929699
Haynes, R. J., and Naidu, R. (1998). Influence of lime, fertilizer and manure applications on soil organic matter. Nutr. Cycl. Agroecosyst. 51, 123–137. doi:10.1023/A:1009738307837
Hua, K., Wang, D., Guo, X., and Guo, Z. (2014). Carbon sequestration efficiency of organic amendments in a long-term experiment on a vertisol in huang-huai-hai plain, China. PLoS One 9, e108594. doi:10.1371/journal.pone.0108594
Jiang, G., Xu, M., He, X., Zhang, W., Huang, S., Yang, X., et al. (2014). Soil organic carbon sequestration in upland soils of northern China under variable fertilizer management and climate change scenarios. Glob. Biogeochem. Cycles 28, 319–333. doi:10.1002/2013GB004746
Jiang, G., Zhang, W., Xu, M., Kuzyakov, Y., Zhang, X., Wang, J., et al. (2018). Manure and mineral fertilizer effects on crop yield and soil carbon sequestration: A meta-analysis and modeling across China. Glob. Biogeochem. Cycles 32, 1659–1672. doi:10.1029/2018GB005960
Jiao, Y., Whalen, J. K., and Hendershot, W. H. (2006). No-tillage and manure applications increase aggregation and improve nutrient retention in a sandy-loam soil. Geoderma 134, 24–33. doi:10.1016/j.geoderma.2005.08.012
Kemmitt, S. J., Wright, D., Goulding, K. W. T., and Jones, D. L. (2006). pH regulation of carbon and nitrogen dynamics in two agricultural soils. Soil Biol. biochem. 38, 898–911. doi:10.1016/j.soilbio.2005.08.006
Khan, S. A., Mulvaney, R. L., Ellsworth, T. R., and Boast, C. W. (2007). The myth of nitrogen fertilization for soil carbon sequestration. J. Environ. Qual. 36, 1821–1832. doi:10.2134/jeq2007.0099
Kong, A. Y. Y., Six, J., Bryant, D. C., Denison, R. F., and van Kessel, C. (2005). The relationship between carbon input, aggregation, and soil organic carbon stabilization in sustainable cropping systems. Soil Sci. Soc. Am. J. 69, 1078–1085. doi:10.2136/sssaj2004.0215
Kundsen, D., Peterson, G. A., Pratt, P. F., and Page, A. L. (1982). “Lithium, sodium, and potassium,” in Methods of soil analysis, part 2. Editor A. L. Page (Madison, Wisconsin: American Society of Agronomy: Soil Science Society of America), 225–246.
Kuzyakov, Y., Friedel, J. K., and Stahr, K. (2000). Review of mechanisms and quantification of priming effects. Soil Biol. biochem. 32, 1485–1498. doi:10.1016/S0038-0717(00)00084-5
Li, T., Zhang, Y., Bei, S., Li, X., Reinsch, S., Zhang, H., et al. (2020). Contrasting impacts of manure and inorganic fertilizer applications for nine years on soil organic carbon and its labile fractions in bulk soil and soil aggregates. Catena 194, 104739. doi:10.1016/j.catena.2020.104739
Liang, F., Li, J., Yang, X., Huang, S., Cai, Z., Gao, H., et al. (2016). Three-decade long fertilization-induced soil organic carbon sequestration depends on edaphic characteristics in six typical croplands. Sci. Rep. 6, 30350–30412. doi:10.1038/srep30350
Liang, F., Li, J., Zhang, S., Gao, H., Wang, B., Shi, X., et al. (2019). Two-decade long fertilization induced changes in subsurface soil organic carbon stock vary with indigenous site characteristics. Geoderma 337, 853–862. doi:10.1016/j.geoderma.2018.10.033
Liu, C., Lu, M., Cui, J., Li, B., and Fang, C. (2014). Effects of straw carbon input on carbon dynamics in agricultural soils: A meta-analysis. Glob. Chang. Biol. 20, 1366–1381. doi:10.1111/gcb.12517
Liu, K., Huang, J., Li, D., Yu, X., Ye, H., Hu, H., et al. (2019). Comparison of carbon sequestration efficiency in soil aggregates between upland and paddy soils in a red soil region of China. J. Integr. Agric. 18, 1348–1359. doi:10.1016/S2095-3119(18)62076-3
Lu, Q., Bai, J., Zhang, G., Zhao, Q., and Wu, J. (2018). Spatial and seasonal distribution of carbon, nitrogen, phosphorus, and sulfur and their ecological stoichiometry in wetland soils along a water and salt gradient in the Yellow River Delta, China. Chem. Earth 104, 9–17. doi:10.1016/j.pce.2018.04.001
Lu, R. (1999). in Methods of soil and agrochemical analysis (Beijing: China Agricultural Science and Technology Press). (in Chinese).
Mahmoodabadi, M., and Heydarpour, E. (2014). Sequestration of organic carbon influenced by the application of straw residue and farmyard manure in two different soils. Int. Agrophysics 28, 169–176. doi:10.2478/intag-2014-0005
Maillard, É., and Angers, D. A. (2014). Animal manure application and soil organic carbon stocks: A meta-analysis. Glob. Chang. Biol. 20, 666–679. doi:10.1111/gcb.12438
Majumder, B., Mandal, B., Bandyopadhyay, P. K., Gangopadhyay, A., Mani, P. K., Kundu, A. L., et al. (2008). Organic amendments influence soil organic carbon pools and rice-wheat productivity. Soil Sci. Soc. Am. J. 72, 775–785. doi:10.2136/sssaj2006.0378
Meersmans, J., Martin, M. P., Lacarce, E., Orton, T. G., De Baets, S., Gourrat, M., et al. (2013). Estimation of soil carbon input in France: An inverse modelling approach. Pedosphere 23, 422–436. doi:10.1016/S1002-0160(13)60035-1
Miao, F., Li, Y., Cui, S., Jagadamma, S., Yang, G., and Zhang, Q. (2019). Soil extracellular enzyme activities under long-term fertilization management in the croplands of China: A meta-analysis. Nutr. Cycl. Agroecosyst. 114, 125–138. doi:10.1007/s10705-019-09991-2
Mikha, M. M., and Rice, C. W. (2004). Tillage and manure effects on soil and aggregate-associated carbon and nitrogen. Soil Sci. Soc. Am. J. 68, 809–816. doi:10.2136/sssaj2004.8090
Murphy, J., and Riley, J. P. (1962). A modified single solution method for the determination of phosphate in natural waters. Anal. Chim. Acta. 27, 31–36. doi:10.1016/S0003-2670(00)88444-5
Olsen, S. R., Cole, C. V., and Watanabe, F. S. (1954). Estimation of available phosphorus in soils by extraction with sodium bicarbonate. USDA circular No. 939. Washington DC: US Government Printing Office.
Pan, G., Zhou, P., Li, Z., Smith, P., Li, L., Qiu, D., et al. (2009). Combined inorganic/organic fertilization enhances N efficiency and increases rice productivity through organic carbon accumulation in a rice paddy from the Tai Lake region, China. Agric. Ecosyst. Environ. 131, 274–280. doi:10.1016/j.agee.2009.01.020
Poeplau, C., Kätterer, T., Bolinder, M. A., Börjesson, G., Berti, A., and Lugato, E. (2015). Low stabilization of aboveground crop residue carbon in sandy soils of Swedish long-term experiments. Geoderma 237, 246–255. doi:10.1016/j.geoderma.2014.09.010
Pugh, T. A. M., Arneth, A., Olin, S., Ahlström, A., Bayer, A. D., Klein Goldewijk, K., et al. (2015). Simulated carbon emissions from land-use change are substantially enhanced by accounting for agricultural management. Environ. Res. Lett. 10, 124008. doi:10.1088/1748-9326/10/12/124008
Rasse, D. P., Rumpel, C., and Dignac, M. F. (2005). Is soil carbon mostly root carbon? Mechanisms for a specific stabilisation. Plant Soil 269, 341–356. doi:10.1007/s11104-004-0907-y
Ren, F., Misselbrook, T. H., Sun, N., Zhang, X., Zhang, S., Jiao, J., et al. (2021). Spatial changes and driving variables of topsoil organic carbon stocks in Chinese croplands under different fertilization strategies. Sci. Total Environ. 767, 144350. doi:10.1016/j.scitotenv.2020.144350
Schmidt, M. W. I., Torn, M. S., Abiven, S., Dittmar, T., Guggenberger, G., Janssens, I. A., et al. (2011). Persistence of soil organic matter as an ecosystem property. Nature 478, 49–56. doi:10.1038/nature10386
Shi, R. (1976). Soil and agricultural chemistry analysis. Beijing: China Agriculture Press. (in Chinese).
Six, J., Elliott, E. T., and Paustian, K. (1999). Aggregate and soil organic matter dynamics under conventional and No-tillage systems. Soil Sci. Soc. Am. J. 63, 1350–1358. doi:10.2136/sssaj1999.6351350x
Smith, P. (2004). How long before a change in soil organic carbon can be detected? Glob. Chang. Biol. 10, 1878–1883. doi:10.1111/j.1365-2486.2004.00854.x
Stewart, C. E., Paustian, K., Conant, R. T., Plante, A. F., and Six, J. (2007). Soil carbon saturation: Concept, evidence and evaluation. Biogeochemistry 86, 19–31. doi:10.1007/s10533-007-9140-0
Stewart, C. E., Paustian, K., Conant, R. T., Plante, A. F., and Six, J. (2008). Soil carbon saturation: Evaluation and corroboration by long-term incubations. Soil Biol. biochem. 40, 1741–1750. doi:10.1016/j.soilbio.2008.02.014
Su, Y. Z., Wang, F., Suo, D. R., Zhang, Z. H., and Du, M. W. (2006). Long-term effect of fertilizer and manure application on soil-carbon sequestration and soil fertility under the wheat-wheat-maize cropping system in northwest China. Nutr. Cycl. Agroecosyst. 75, 285–295. doi:10.1007/s10705-006-9034-x
Sun, W., Canadell, J. G., Yu, L., Yu, L., Zhang, W., Smith, P., et al. (2020). Climate drives global soil carbon sequestration and crop yield changes under conservation agriculture. Glob. Chang. Biol. 26, 3325–3335. doi:10.1111/gcb.15001
Thomsen, I. K., Schjønning, P., Jensen, B., Kristensen, K., and Christensen, B. T. (1999). Turnover of organic matter in differently texured soils: II. Microbial activity as influenced by soil water regimes. Geoderma 89, 199–218. doi:10.1016/S0016-7061(98)00084-6
Triberti, L., Nastri, A., and Baldoni, G. (2016). Long-term effects of crop rotation, manure and mineral fertilisation on carbon sequestration and soil fertility. Eur. J. Agron. 74, 47–55. doi:10.1016/j.eja.2015.11.024
Triberti, L., Nastri, A., Giordani, G., Comellini, F., Baldoni, G., and Toderi, G. (2008). Can mineral and organic fertilization help sequestrate carbon dioxide in cropland? Eur. J. Agron. 29, 13–20. doi:10.1016/j.eja.2008.01.009
Walkley, A., and Black, I. A. (1934). An examination of the degtjareff method for determining soil organic matter, and a proposed modification of the chromic acid titration method. Soil Sci. 37, 29–38. doi:10.1097/00010694-193401000-00003
Wang, Q., Liu, L., Li, Y., Qin, S., Wang, C., Cai, A., et al. (2020). Long-term fertilization leads to specific PLFA finger-prints in Chinese Hapludults soil. J. Integr. Agric. 19, 1354–1362. doi:10.1016/S2095-3119(19)62866-2
Wang, R., Zhou, J., Xie, J., Khan, A., Yang, X., Sun, B., et al. (2020). Carbon sequestration in irrigated and rain-fed cropping systems under long-term fertilization regimes. J. Soil Sci. Plant Nutr. 20, 941–952. doi:10.1007/s42729-020-00181-6
Wang, X., Liang, C., Mao, J., Jiang, Y., Bian, Q., Liang, Y., et al. (2023). Microbial keystone taxa drive succession of plant residue chemistry. ISME J. 1. 10, doi:10.1038/s41396-023-01384-2
Wong, V. N. L., Greene, R. S. B., Dalal, R. C., and Murphy, B. W. (2010). Soil carbon dynamics in saline and sodic soils: A review. Soil Use Manag. 26, 2–11. doi:10.1111/j.1475-2743.2009.00251.x
Wu, L., Zhang, W., Wei, W., He, Z., Kuzyakov, Y., Bol, R., et al. (2019). Soil organic matter priming and carbon balance after straw addition is regulated by long-term fertilization. Soil Biol. biochem. 135, 383–391. doi:10.1016/j.soilbio.2019.06.003
Xu, H., Shen, H. P., Zhou, S. W., Wang, X. L., and Sun, N. (2016). Determination of soil organic matter content by heating method with an aluminum module digesting device. Soil fertizer Sci. China 3, 140–144. (in Chinese). doi:10.11838/sfsc.20160323
Xu, X., Shi, Z., Li, D., Rey, A., Ruan, H., Craine, J. M., et al. (2016). Soil properties control decomposition of soil organic carbon: Results from data-assimilation analysis. Geoderma 262, 235–242. doi:10.1016/j.geoderma.2015.08.038
Yan, X., Zhou, H., Zhu, Q. H., Wang, X. F., Zhang, Y. Z., Yu, X. C., et al. (2013). Carbon sequestration efficiency in paddy soil and upland soil under long-term fertilization in southern China. Soil Tillage Res. 130, 42–51. doi:10.1016/j.still.2013.01.013
Yang, X., Ren, W., Sun, B., and Zhang, S. (2012). Effects of contrasting soil management regimes on total and labile soil organic carbon fractions in a loess soil in China. Geoderma 177–178, 49–56. doi:10.1016/j.geoderma.2012.01.033
Zhang, W. J., Wang, X. J., Xu, M. G., Huang, S. M., Liu, H., and Peng, C. (2010). Soil organic carbon dynamics under long-term fertilizations in arable land of northern China. Biogeosciences 7, 409–425. doi:10.5194/bg-7-409-2010
Zhao, Y., Zhang, Y., Liu, X., He, X., and Shi, X. (2016). Carbon sequestration dynamic, trend and efficiency as affected by 22-year fertilization under a rice–wheat cropping system. J. Plant Nutr. Soil Sci. 179, 652–660. doi:10.1002/jpln.201500602
Keywords: soil carbon sequestration efficiency, dryland, long-term fertilization, influence factor, organic amendments
Citation: Liang S, Sun N, Wang S, Colinet G, Longdoz B, Meersmans J, Wu L and Xu M (2023) Manure amendment acts as a recommended fertilization for improving carbon sequestration efficiency in soils of typical drylands of China. Front. Environ. Sci. 11:1173509. doi: 10.3389/fenvs.2023.1173509
Received: 24 February 2023; Accepted: 20 April 2023;
Published: 09 May 2023.
Edited by:
Pascal Boivin, University of Applied Sciences Western Switzerland, SwitzerlandCopyright © 2023 Liang, Sun, Wang, Colinet, Longdoz, Meersmans, Wu and Xu. This is an open-access article distributed under the terms of the Creative Commons Attribution License (CC BY). The use, distribution or reproduction in other forums is permitted, provided the original author(s) and the copyright owner(s) are credited and that the original publication in this journal is cited, in accordance with accepted academic practice. No use, distribution or reproduction is permitted which does not comply with these terms.
*Correspondence: Nan Sun, c3VubmFuQGNhYXMuY24=; Minggang Xu, eHVtaW5nZ2FuZ0BjYWFzLmNu
Disclaimer: All claims expressed in this article are solely those of the authors and do not necessarily represent those of their affiliated organizations, or those of the publisher, the editors and the reviewers. Any product that may be evaluated in this article or claim that may be made by its manufacturer is not guaranteed or endorsed by the publisher.
Research integrity at Frontiers
Learn more about the work of our research integrity team to safeguard the quality of each article we publish.