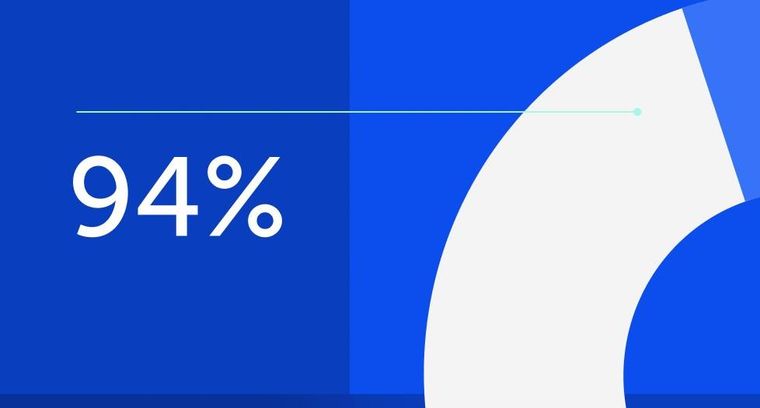
94% of researchers rate our articles as excellent or good
Learn more about the work of our research integrity team to safeguard the quality of each article we publish.
Find out more
ORIGINAL RESEARCH article
Front. Environ. Sci., 01 June 2023
Sec. Freshwater Science
Volume 11 - 2023 | https://doi.org/10.3389/fenvs.2023.1162908
This article is part of the Research TopicRiver Rehabilitation in the AnthropoceneView all 7 articles
Restoration activities commonly aim to reverse the impacts of environmental degradation and return a system back to an original, “pre-disturbance” condition. Is this realistic, achievable, or reflective of an unconscious bias in the Anthropocene, the current geological epoch where human disturbances dominate ecosystems? Billions of dollars are invested into river restoration globally each year, but there are limited empirical data to evaluate river recovery after these activities. Current response models, typically based on concepts of equilibrium and stability, assume rivers return to pre-disturbance conditions by removing or ameliorating a disturbance or stressor. Conceptual frameworks are useful tools to order phenomena and material, and understand patterns and processes in data-limited situations. A framework for the recovery of rivers in the Anthropocene is presented. The framework includes components of resilience thinking, landscape ecology, and river science. It is proposed that rivers in the Anthropocene have metamorphosed to a different basin of attraction (regime/state) displaying alternative functions, structures, and interactions. Resilience thinking suggests that once a river moves beyond the Anthropocene tipping point, recovery to its original state is not possible. If a river system cannot be returned to its original state, it must be repaired to something else. Using principles of landscape ecology for restoring structural and functional heterogeneity the capacity of Anthropocene rivers to withstand current and future disturbances would be enhanced. River science recognizes the significance of physical heterogeneity at multiple scales, resulting in differences in sensitivities to disturbance and associated recovery trajectories. All of these should guide the selection of river restoration activity types at given locations within a river network.
There is substantial and unequivocal evidence that human activities have degraded river ecosystems (Van Cappellen and Maavara, 2016; Best, 2019; Grill et al., 2019) and compromised the ecosystem services rivers provide to society (Vorosmarty et al., 2010; Auerbach et al., 2014). Aspirations to reverse the impact of human activities on river ecosystems drive investment in river restoration and repair, and support the current United Nations’ Decade of Ecosystem Restoration (Palmer and Stewart, 2020). Every year, billions of dollars are spent on restoration (Palmer et al., 2005; Brooks and Lake, 2007), with an estimated USD$ 6–7.3 billion between 1990 and 2003 in the United States (Bernhardt et al., 2005), and AUD$ 750 million in Australia between 1995 and 2007 (Price et al., 2009). River restoration activities aim to improve habitat and water quality, increase biodiversity, enhance fisheries yield, reduce flood severity, manage bank erosion, reinstate channel form and features, reinstate flow regimes, and improve biophysical connectivity and process interactions (Bernhardt et al., 2005; Lake et al., 2007; Wheaton et al., 2008; Palmer and Stewart, 2020; Beechie et al., 2021).
The Anthropocene has been proposed as the current geological epoch, extending back to the mid-1700s to incorporate the industrial revolution, and is characterized by the human domination of ecosystems (Crutzen, 2006; Steffen, 2015). Anthropocene rivers are proposed to have metamorphosed into systems that are less biophysically complex (Thoms and Fuller, 2023), have a reduced capacity to resist disturbances (DeBoer et al., 2020; Wohl, 2020), and display novel traits (Hobbs et al., 2009; DeBoer et al., 2019; Thoms and Fuller, 2023) because of the influence of persistent, pervasive, and multiple human stressors (Ormerod et al., 2010; Sabater et al., 2019). These stressors act as press (persistent and pervasive) and ramp (increase in severity over time) disturbances (Lake et al., 2007). Press and ramp disturbances have become dominant in the Anthropocene (Ellis, 2015; Steffen et al., 2018) and can disrupt natural disturbance regimes and exacerbate pulse (episodic, large, and infrequent events) disturbances (Stanley et al., 2010; Death, 2023). Despite an increased understanding of Anthropocene rivers (Kelly et al., 2018), the science and practice of river restoration remains tied to traditional approaches of linear response, succession, stability, and pulse disturbance that seek to return rivers to a pre-Anthropocene state (Lake et al., 2007; Palmer et al., 2014b). This is reflective of an unconscious bias in river restoration that disconnects restoration planning from what is possible in a metamorphosed Anthropocene river. The characteristics of state change and persistent ramp and press disturbance in the Anthropocene suggest that river restoration cannot, or will not, result in recovery to the pre-disturbance state for many rivers. Thus, there is a need to understand the boundary conditions of river restoration in the Anthropocene, given the level of investment in restoration activities and the value rivers have in society.
Frameworks are heuristic tools useful for proposing new areas of endeavor (Delong and Thoms, 2016). Built on theory, frameworks logically narrate, order, and organize foundational ideas to support the proposed area of endeavor. This paper outlines a conceptual framework for the study and practice of river restoration and repair in the Anthropocene. The framework starts with the idea of river metamorphosis framed by resilience thinking: rivers have change state in the Anthropocene and cannot be returned to their prior state by restoration. Resilience thinking questions the current system state, the potential for alternative states, and the movement between states; it also recognizes that a river cannot return to a prior state once a tipping point has been crossed. Knowing the status of the state change is a fulcrum from which to envisage if river restoration is viable or whether a new approach—which we term river repair—is more appropriate. River repair does not attempt to move, i.e., restore, a system back to a pre-Anthropocene state but rather uses principles of landscape ecology and river science to enhance adaptive capacity to prevent further metamorphosis, thereby reducing the likelihood of compromising ecosystem services associated with a current state. Within the river repair framework, landscape ecology characterizes river systems as multiple biophysical patches, where heterogeneity is associated with increases in biodiversity and the ability to resist and absorb disturbances (Stanley et al., 2010). Finally, river science recognizes the hierarchical and scaled organization of river landscapes and varying sensitivities to disturbance and recovery trajectories of landscape components at different scales. Landscape ecology and river science are the concepts guiding river repair in the Anthropocene and the context from which models and programs can be designed. Integration of these three concepts guides a paradigm shift in the way Anthropocene rivers are studied, managed, and repaired.
This manuscript presents a conceptual framework for planning, implementing, assessing, and evaluating river restoration and repair in the Anthropocene. The framework consists of three key building blocks (Figure 1) that are currently not adequately considered within river restoration activities. It is not the intent of this framework to provide practice guidance for river repair. The framework proposes a new approach to considering what is possible in Anthropocene rivers at the outset of a planned restoration investment or study.
FIGURE 1. Framework for the repair of rivers in the Anthropocene. The framework is based on sequential components of resilience thinking, landscape ecology, and river science. Each component has a series of tenets relevant to river restoration in the Anthropocene.
Resilience thinking has developed as a foundational concept focused on coupled human and natural systems, and is a way to view naturally dynamic systems, such as Anthropocene rivers, that have metamorphosed into a new state. The resilience alliance defines resilience in terms of system change, where “resilience is the amount of change a system can undergo (its capacity to absorb disturbance) and remain within the same regime that essentially retains the same function, structure, and feedback” (Holling, 1973; Carpenter et al., 2001; Walker and Salt, 2006; Cumming, 2011; Hodgson et al., 2015). Characteristics of resilience, including diversity, redundancy, connectivity, feedback, and transformability, enhance the adaptive capacity of systems to absorb disturbance without changing the state (Folke, 2006; Biggs et al., 2012). Resilience has gained traction in various disciplines (Parsons and Thoms, 2018). From an engineering perspective, focus is placed on how quickly a system returns to a steady state, following disturbance, while ecological resilience recognizes alternative stable states, and the potential for systems to breach thresholds and flip into an alternative stable state (Gunderson and Holling, 2002). Resilience is often visualized by the ‘ball and cup model’ (Figure 2), where the range of conditions in which the system (ball) operates is represented by the cup (basin of attraction), and the movement of the ball (by disturbance or other forces) represents the dynamics of the system. Ecosystems can flip into a new basin of attraction upon breaching a major threshold or tipping point.
FIGURE 2. Ball and cup model of river system repair in the Anthropocene. Resilience thinking visualizes the transition of a system (represented by the ball) from one state (cup) to another because of disturbance (arrows). A system operates within a particular state (A) but may cross a tipping point (threshold) to operate within a new state (B) from which recovery back to the original state is difficult. In this new state, the system is governed by different conditions, feedback, functions, and processes. Typically, restoration (represented by the purple arrow) aims to restore the affected system (the white ball) back to its original state. Under resilience thinking, this cannot occur. Instead, there are two potential trajectories: the system remains in the new alternative state, or the system breaches another tipping point. However, given the inherent complexity and unpredictable response trajectories of rivers, it is unclear what a new alternative state may look like and what ecosystem services would be associated, as visualized by (C) and (D).
Disturbance regimes are an inherent aspect of ecosystems. Disturbance can be defined as an event of a particular severity, intensity, and frequency that is uncharacteristic of a system’s predictable range, and which disrupts or changes the structure of ecosystems and their communities or associated resources and environment (Pickett and White, 1985; Resh et al., 1998). Disturbance results in abrupt or slow changes that move systems away from near-equilibrium conditions (Sousa, 1984). Some disturbances result in variable responses within the same basin of attraction; others can result in a breach of threshold conditions, whereby the system is pushed into a new state and is subsequently characterized by different functions, feedback, and structure (Walker et al., 2004). Recovery back to an original state is difficult or potentially unachievable (Walker and Salt, 2006). The precariousness–locality of a system’s state in proximity to a threshold–of a system influences response to disturbance. For example, a basin of attraction may become shallower after repeated disturbances, which implies a reduction in system resilience and an increased likelihood of tipping into an alternative state (Walker and Salt, 2006).
There are significant implications of resilience thinking for rivers; rivers are complex adaptive systems, and resilience thinking recognizes the potential for alternative stable states (basin of attraction) at different scales and the nature of continuous change in river systems (Beisner et al., 2003; Scheffer and Carpenter, 2003; Parsons et al., 2009). Restoration, the dominant paradigm under which rivers are managed, can be defined as returning a system to a pre-disturbance state. Resilience thinking determines if a system is in a natural or anthropogenic state and, in turn, determines if restoration is potentially achievable or if repair activities would be appropriate. Principles of resilience thinking suggest ‘restoration’ is no longer an appropriate aim for many rivers in the Anthropocene—the new regime within which many rivers operate—and that focus should be placed on river repair. Rivers have been extensively modified in the Anthropocene and have often metamorphosed into an alternative stable state (Schumm, 1969; Best, 2019). As such, an Anthropocene river is categorized by a different set of structures, functions, feedback, and identity. Under resilience thinking and the expected continuation of human activity acting as press and ramp disturbances in the Anthropocene (Palmer et al., 2014a; Wohl et al., 2015; Death, 2023), restoration to a previous state is unachievable (Figure 3). Conversely, repair aims to enhance the adaptive capacity of a river ecosystem to prevent further flips and acknowledges the likely continuation of anthropogenic disturbance(s).
FIGURE 3. Types of river disturbance regimes, as distinguished by the strength of the disturbing force over time (after Lake, 2000). (A) Pulse disturbance, (B) press disturbance, (C) ramp disturbance, and (D) anthropogenic disturbance, which are primarily press or ramp disturbances with compounded pulse disturbances. Restoration activities typically have a pulsed disturbance focus (a and b); they are often localized, high-magnitude, and low-frequency events that do not consider the ongoing anthropogenic impact. These repair activities will likely not result in long-term remediation of ecological degradation.
If returning a system to a previous state is not possible in the Anthropocene, then we must ask, ‘what are we repairing our rivers for in the Anthropocene?’ Under resilience thinking, there are two possible trajectories of river response to repair (Figure 2). Repair activities might improve river conditions but keep a system operating in the new basin of attraction, thus retaining and ideally enhancing associated ecosystem services. Alternatively, repair might push the river into another basin of attraction. Regime shifts from one stable state to another can occur at multiple scales (Gordon et al., 2008). Uncontrolled shifts into another state result in the loss of associated ecosystem services upon which society relies (Leuven and Poudevigne, 2002). Valuable ecosystem services include the provision of freshwater, flood control, nutrient cycling, recreation, and spiritual practice. From a social perspective, various stakeholders will place greater or lesser value on different ecosystem services, highlighting that river management is inherently value-laden and trade-offs will always occur. From a biophysical perspective, this framework suggests the repair of Anthropocene rivers should focus on increasing adaptive capacity—the characteristics that enhance the capacity of the system to absorb disturbance—so that the system is better equipped to withstand or respond to current and future stress or disturbance. Increased adaptive capacity would help prevent further threshold breaches, thereby retaining and preventing the loss of valuable ecosystem services associated with the river in its current state.
After using resilience thinking to determine if Anthropocene rivers have metamorphosed, principles of landscape ecology can be used to guide river repair. Landscape ecology is an interdisciplinary approach to the study of entire ecosystems (Wiens, 2002), focusing on how processes, patterns, scale, hierarchy, and connectivity all interact to shape relationships between the system structure and function (Poole, 2002; Wiens, 2002). Landscape ecology can be understood through the hierarchical patch dynamics (HPD) perspective, which views fluvial ecosystems as a discontinuum and as multiscale nested hierarchies of interactive terrestrial and aquatic elements or patches (Poole, 2002). Patches are dynamic as their characteristics change over various temporal and spatial scales naturally and in response to disturbances (Figure 4). Through the lens of HPD, ecological responses to disturbances vary depending on location. Therefore, the nature or heterogeneity of the community of patches plays a role in the response to disturbance.
FIGURE 4. Relevance of landscape ecology to river repair. Important components of landscape ecology highlight the causes and mechanisms of patchiness, both physical and biological, within ecological systems at various temporal and spatial scales. Physical patchiness (heterogeneity) has implications in the context of river repair as a diverse physical template provides the basis on which biological diversity may develop.
Heterogeneity is a foundational tenet of landscape ecology (Turner, 1987) and is significant for river repair activities. A heterogeneous landscape is made up of patches that differ from each other (Art, 1993) or has a non-uniform structure or composition (Lincoln et al., 1998). There are four different types of heterogeneity: patterned, compositional, locational, and spatial variance (Wiens, 1999). Functional heterogeneity is typically evaluated by understanding environmental patterns and the response of organisms to these patterns at multiple scales (Wiens, 1999). However, it also incorporates variations in the time and scale of landscape functions like connectivity, biodiversity, and system fluxes (Hobbs et al., 2009). In river systems, the physical environment provides a template upon which evolution forges characteristic life history strategies (Southwood, 1977; 1988). Accordingly, the physical or structural heterogeneity of a river’s physical template influences the type, abundance, and arrangement of biological assemblages and ecological functions within these landscapes. Interactions between structural and functional components generate distinct patterns within riverine landscapes that have been observed at multiple scales (Thoms et al., 2017).
Heterogeneity is an inherent part of rivers, maintained by natural disturbances (Stewart et al., 1999). The positive association between heterogeneity, redundancy, and the ability to absorb disturbances has been shown across many different ecosystems (Wellnitz and Poff, 2001; Rosenfeld, 2002; Kotschy et al., 2015; Lawson et al., 2015; Van Looy et al., 2019). Heterogeneous ecosystems have been homogenized, simplified, and fragmented as a result of human activities in the Anthropocene (Wiens, 1999; Brierley et al., 2022; Death, 2023). Simplification results in a loss of redundancy through decreases in habitat heterogeneity and associated patterns of species abundance, and community development and assemblage (Lindenmayer and Fischer, 2006; DeBoer et al., 2020). Homogenization decreases biodiversity, reduces resilience, and often increases system sensitivity to disturbance (Oliver et al., 2015; Frainer et al., 2017; Zhang et al., 2021). Moreover, simplification can occur as a result of river management activities like channelization and engineered structures that restrict natural river dynamics. Simplification and fragmentation of river ecosystems can result in more severe and frequent disturbances, which challenges the efficacy of natural river resilience (Death, 2023). As rivers in the Anthropocene have been subject to continuous press or ramp disturbances through human activity, it is important to recognize that heterogeneity enhances the adaptive capacity of ecosystems to withstand current and future disturbances.
There are numerous benefits to enhancing structural and functional heterogeneity across degraded riverscapes in repair efforts. Habitat heterogeneity strengthens the capacity of systems to withstand and recover from disturbance, thus building adaptive capacity. It also increases resource and habitat diversity through varied physical refugia and greater surface area, thereby supporting species diversity (Palmer et al., 2009; Landis, 2017). Increased heterogeneity is associated with greater species redundancy and connectivity, as well as genetic variability and adaptive phenotypic plasticity, all of which increase biodiversity and system resilience (Levin et al., 1998; Lake et al., 2007; Colloff and Baldwin, 2010; Oliver et al., 2015). Resilience is increased through species redundancy as various species within an ecosystem may carry out similar functional roles and allow a system to retain distinctive functions or processes through disturbances despite various responses to disturbance (Walker, 1995). The modification and homogenization of landscapes because of anthropogenic activities have largely ameliorated ‘pristine’ or ‘natural’ conditions, this suggests using ‘original’ reference sites is no longer the most suitable guide for a desired endpoint of successful restoration (Palmer et al., 2005). Instead, this framework proposes that restoration in the Anthropocene should be guided by an increase in heterogeneity.
If an ecosystem is repaired through the re-establishment of heterogeneity, concepts of fluvial landscape ecology can help inform best practice and increase an understanding of potential indicators of success. Heterogeneity must be an “operational term” (Wiens, 1999). It must be measurable or quantifiable and allow comparison among or between sites. Measurable features include mosaic diversity or connectivity at a landscape level. Evidence to support the success of restoring heterogeneity, largely found on the field of dreams approach (“if you build it, they will come”), is limited (Palmer et al., 1997). Restoration activities focused on increasing heterogeneity, such as channel reconfiguration or the construction of additional in-stream geomorphic units, may not always result in a statistically significant increase in biodiversity due to a mismatch between cause and effect at an appropriate scale, or a lack of consideration for factors such as legacy effects, deteriorated water quality, or invasive species (Palmer et al., 2009). These factors may limit an increase in biodiversity if structural heterogeneity is the only focus (Palmer et al., 2009). In addition, heterogeneity imposed by anthropogenic restoration may have severe consequences for the persistence of some species with high habitat specificity or restricted dispersal mechanisms (Stewart et al., 1999). The heterogeneity of riverine landscape incorporates the diversity of aspects such as flow, patches, water quality, floral and faunal biodiversity, connectivity, and functions. This framework suggests that the repair of Anthropocene rivers should focus on increasing physical heterogeneity across riverscapes to enhance species redundancy and biodiversity, and subsequently increase system resilience to current and future disturbances. However, heterogeneity can only be effective when river repair activities are aware of the hierarchy theory, scale, and the nature of various sensitivities across river networks within the concept of river science.
The repair of metamorphosed and homogenized Anthropocene rivers must be cognizant of scale, location in the river network, and varying sensitivities to disturbance and restorative efforts, all of which are understood through the lens of river science. River science has rapidly emerged as an interdisciplinary field of study focusing on interactions between the physical, chemical, and biological components within riverine landscapes (Dollar et al., 2007; Gilvear et al., 2016; Thoms et al., 2016; Pingram et al., 2019) and how they influence and are influenced by human activities. These interactions are studied at multiple scales within both the riverscape (river channels, partially isolated backwaters, and riparian zones) and adjacent floodscape (isolated oxbows, floodplain lakes, wetlands, and periodically inundated flat lands). Its integrative nature facilitates an understanding of complex natural phenomena and their application to the management of riverine landscapes (Thoms, 2006).
With key tenets related to scale, hierarchy theory, and interdisciplinarity, river science allows an understanding of river system organization at multiple scales, and the identification of different sensitivities to disturbance and recovery pathways across a river network. Rivers are complex at multiple scales, and patches are differentiated by particular dominant processes or functions, recognition of which could enhance the success of river repair efforts (Poole, 2002). Different sensitivities of patches within the river network mean that different parts of the river channel, floodplain, or wider network will respond to the same disturbance event in different ways. Rivers operating in the Anthropocene (a new basin of attraction) are typically sensitive in different ways or to different disturbances. The sensitivity of a system can reflect its ability or capacity to adjust or its relationship to a threshold condition (Fryirs and Brierley, 2013). Highly sensitive rivers adjust more readily to perturbations over shorter timeframes when compared with more resilient systems (Fryirs and Brierley, 2013). As restoration is a type of disturbance that aims to “move an ecosystem into a different, preferred state” (Stanley et al., 2010; 77) and rivers are dynamic, disturbance-driven systems (sensu Levin, 1998), disturbance theory must be understood and incorporated into river repair activities.
To enhance an understanding of the implications of different sensitivities to disturbance (therefore repair) and recovery trajectories across river networks, aspects of river science such as interdisciplinarity, hierarchy theory, and scale must be considered. Various disciplines, including ecology, hydrology, geomorphology, and engineering, all aim to understand, describe, explain, and manage rivers (Thoms and Parsons, 2002; Delong and Thoms, 2016). River science recognizes the inherent complexity of river systems and dynamic interactions of various ecological, geological, and hydrological processes at different temporal and spatial scales; interdisciplinarity is, therefore, intrinsically linked with hierarchy theory and scale (Thoms and Parsons, 2002). Rivers are hierarchical systems (O’Neill et al., 1989; Poole, 2002), which have a series of organizational levels (Figure 5) governed by levels above them and represent emergent properties of lower levels (Dollar et al., 2007; Fryirs and Brierley, 2013). Hierarchy influences community development and assemblage as regional species pools dictate dispersal, habitat, and subsequent biotic constraints, which finally affect local assemblage patterns and processes (Lake et al., 2007). Scale, an integral part of hierarchical systems, refers to the physical dimensions or units of measurement of an object (Quinn and Keough, 2002) and determines what units of measurement are most appropriate for each organizational level (Dollar et al., 2007). At the broadest scale, river catchments are systems “containing different sized spatial and temporal scales nested within one another” (Wissmar and Beschta, 1998: 572), where a network of channels link one overarching fluvial system (Fryirs and Brierley, 2013).
FIGURE 5. River characterization scheme that visualizes rivers as hierarchical systems from a river basin, river system, functional process zone, river reach, functional channel set, functional unit, to a macro-habitat. Each organizational level is governed by levels above (top–down constraint) and represents the heterogeneity of emergent properties of lower levels (bottom–up influence). Sensitivity to, and recovery time following, a disturbance varies depending on the scale of an impacted holon or its place within the river network. Different organizational levels of a river will, therefore, respond to the same type of restoration (a disturbance) in different ways.
Understanding rivers as hierarchical systems is essential for effective river repair; activities must be suitable for their location within the river network, and problems driving the need for repair must be matched to processes at the right scale (Thoms and Parsons, 2002). At all hierarchical scales, river characteristics are determined by imposed and flux boundary conditions and connectivity. Valley confinement and slope, bedrock, or low relief topography all dictate imposed boundary conditions, while flux boundary conditions are more dynamic as they are set by changing and fluid interactions between water, vegetation, and sediment (Fryirs and Brierley, 2013). In the context of river repair, it must be understood that spatial scales (e.g., a site, habitat, or landscape) at which environmental heterogeneity can occur and the associated responses of organisms are all scale-dependent (Stewart et al., 1999; Wiens, 1999). River repair activities with a structured focus will only improve the local site or reach scale conditions (Wohl et al., 2015), meaning that repair activities should occur across an entire riverscape and not just within a river channel (Gilvear et al., 2016). Reversing undesirable regime shifts through restoration increases in difficulty and occurs over a longer timeframe with the increase of spatial scale (Figure 5; Parsons et al., 2009). River repair activities must, therefore, be cognizant of hierarchy theory, scale, and the place within a network. Hierarchy theory and landscape sensitivity stress the importance of understanding how heterogeneity and HPD in the river network influence system response to repair activities. Alongside principles of resilience thinking and landscape ecology, the key tenets of river science presented in this paper call for a paradigm shift in the way river repair efforts are viewed and practiced in the Anthropocene. This paradigm shift toward a focus on repair arises from the identification of an unconscious bias in river restoration, where current methods do not often consider the implications of irreversibility in the Anthropocene or alternative stable states and frequently ignore dynamic landscape sensitivities.
Unconscious bias occurs when attitudes, beliefs, and stereotypes unconsciously influence thinking and decision-making (Pinter et al., 2019). This may promote oversimplified and incorrect views on concepts and paradigms that underpin management strategies. In river restoration, unconscious bias may promote certain approaches and strategies being favored over others even when they may not be effective for river ecosystems (cf. Kondolf et al., 2006). This can occur at multiple scales, from sites to river networks. Examples include the preference for engineering solutions to mimic historical conditions rather than working with the river and its unique hydrological, geomorphological, and ecological conditions (Palmer et al., 2005; Brierley et al., 2022). River restoration decision-making needs to be aware of, and minimize, unconscious bias; this can be achieved by expanding interdisciplinary perspectives and engaging in continuous reflection to improve approaches to and strategies for managing river ecosystems in the Anthropocene.
To advance the science and practice of river repair in the Anthropocene, the current state of river restoration must be understood. Stakeholders are driven to improve river conditions because of concerns about water quality, erosion, biodiversity loss, deforestation, alteration of flow or processes, climate change, flood control, or loss of ecosystem services (Bernhardt et al., 2007; Cumming, 2011; Feld et al., 2011; Palmer et al., 2014a). Commonly, restoration aims to remove a stressor so that pre-disturbance conditions can prevail. Ecological degradation is one of the main drivers for restoration projects (Bernhardt et al., 2007; Beechie et al., 2010). Habitat simplification and physical instability are strongly associated with degraded river systems (Chessman et al., 2006); this often results from river modification, pollution, and flow alteration associated with urbanization and agricultural development (Feld et al., 2011).
River restoration was traditionally focused on engineering-based solutions until the mid-1990s when a shift to more holistic process-based restoration emphasized natural ecosystem processes and functionality (Palmer et al., 2005; Kondolf et al., 2006; Wohl et al., 2015). Engineering-based approaches are often active and involve hard structures or physical manipulation of river channels (Raven et al., 2000; Brierley and Fryirs, 2009), such as channel realignment or the construction of log and rock weirs (Brierley and Fryirs, 2009; Palmer et al., 2014a). Conversely, passive approaches to restoration encourage the river to perform the work through actions such as revegetation, implementation of buffer strips, management of nonpoint sources of pollution, or reducing intensive agricultural activities and grazing near waterways (Wissmar and Beschta, 1998; Wheaton et al., 2008; Brierley and Fryirs, 2009; Knouft et al., 2021). In theory, reasons for restoration influence the selection of the most suitable activity type based on the site and associated values. Reasons for river restoration also dictate project objectives and guide selection for appropriate monitoring or measurements of success. In practice, however, monitored variables are often inappropriate for the site or with respect to the project objectives and do not allow for evaluation of restoration success or expected response trajectories (Bernhardt et al., 2005).
Despite increased engagement and investment in river restoration, activities often have a pulse-disturbance focus and ignore the implications of press and ramp disturbances that are dominant in the Anthropocene. Disturbance is a critical component of all three building blocks presented in this framework. As a key aspect of resilience thinking, the duration, magnitude, and frequency of disturbance events influence how a system (the ball, Figure 2) moves within and between states (the cup). A previously highly resilient system subject to a range of anthropogenic disturbances in addition to a natural disturbance regime is likely to become more sensitive to smaller disturbances and more readily tip over into a new state (Walker and Salt, 2006). Landscape ecology suggests that natural disturbance regimes (typically pulse disturbances) generate heterogeneity, while anthropogenic disturbances that predominantly act as press and ramp disturbances homogenize systems. River science considers that varying parts of river networks have differing sensitivities and associated response trajectories to the same disturbance event, stressing the importance of implementing repair activities that are specific to each part of a network. For river repair efforts to be successful in the Anthropocene, human activity and associated stressors on natural ecosystems must be viewed as press or ramp disturbances. However, this is currently not a consideration in the five common river restoration methods.
There are five main groups of river restoration methods: carbon copy, field of dreams, fast-forwarding, cookbook, and command-and-control (Hilderbrand et al., 2005). Building on Clements’ (1936) belief that ecosystems follow a predictable trajectory toward a specific endpoint, the carbon copy method assumes that a replica of a historic or ideal state can be created (Hilderbrand et al., 2005). However, in the current Anthropocene, endpoints that replicate historic conditions may be unachievable due to different climatic regimes, vegetation composition, or land use pressures (Hilderbrand et al., 2005; Brierley and Fryirs, 2009; Nardini and Conte, 2021). Based either on historic photographs and maps or the locality of old river courses (Soar and Thorne, 2001), carbon copy methods frequently involve engineered solutions, where principles of command-and-control dominate. Command-and-control methods involve active, physical manipulation of river systems, which reduces system resilience and adaptive capacity (Gunderson, 2000) and focuses more on the symptoms rather than the causes of ecosystem degradation (Hilderbrand et al., 2005). Field of dreams is based on the assumption that ‘if you build it, they will come’ (Palmer et al., 1997); this ignores natural river complexity, ongoing natural and anthropogenic disturbance regimes, and unpredictable response trajectories (Kondolf, 1995). Despite the lack of evidential success, fast-forwarding is a type of restoration whereby people assume that successional processes may be accelerated to achieve a specific desired outcome or response trajectory (Hilderbrand et al., 2005). The cookbook method assumes systems that have similar physical or ecological characteristics should have similar responses to the same restoration activities. This assumption has led to the continuous use of unsuccessful but published restoration methods common in engineering-based techniques (Hilderbrand et al., 2005). Cookbook methods rarely result in successful outcomes as they oversimplify the natural variability of complex river systems that have diverse traits, sensitivities, and responses (Fryirs and Brierley, 2009).
Aiming to determine the suitability of current restoration methods for the repair of Anthropocene rivers, we evaluated 44 river restoration methods for their alignment with the tenets of resilience thinking, landscape ecology, and river science (Figure 6).
FIGURE 6. Evaluation of river restoration activities. The heat map distinguishes restoration activities that do not (gray) consider or incorporate principles of resilience thinking, landscape ecology, or river science from those that partially (striped) or fully (white) consider the evaluated variables. Principles of resilience thinking are infrequently considered or incorporated within evaluated river restoration activities, particularly those governed by command-and-control, carbon copy, fast-forwarding, or cookbook methodology. Principles of landscape ecology and river science are more adequately incorporated within river restoration activities, yet partial consideration is typically the more dominant outcome. River restoration activities are grouped according to the categories of command-and-control, carbon copy, fast-forwarding, cookbook, and field of dreams.
Principles of resilience thinking are infrequently considered in current river restoration methods. Most (98%) river restoration projects do not fully incorporate irreversibility or the nature of alternative stable states. This implies that restoration activities operate with an unconscious bias under a restoration or ‘natural’ paradigm with the belief that activities can restore rivers to natural or pre-disturbance conditions. In addition, 34% of methods partially or fully considered adaptive capacity, which focuses on building resilience. However, several evaluated studies focused primarily on building adaptive capacity in the context of flood resilience. Overall, 4.5% of all evaluated methods fully incorporated a principle of resilience thinking, and no method fully incorporated all three principles. Only three of the 44 evaluated methods involved partial or full consideration of two or all three principles of resilience thinking.
Principles of landscape ecology are more adequately considered in current river restoration methods, with the dominant outcome of this evaluation across all methods and principles being 50% full incorporation or consideration. Of these methods, 66% fully consider the implications of human activity homogenizing previously heterogeneous landscapes, but only 34% of evaluated activities recognize the relationship between physical heterogeneity, biodiversity, and resilience. A total of 10 of the 44 methods (23%) fully consider all three variables of landscape ecology, nine of which are categorized by a ‘field of dreams’ approach. In contrast to resilience thinking, only three methods do not consider any principles of landscape ecology.
The incorporation of river science was dominated by interdisciplinarity; 91% of methods partially or fully considered the need for an interdisciplinary approach to river restoration. Although principles of hierarchy and scale and landscape sensitivity were often partially considered, this remains a critical gap. A total of 25% of methods fully incorporated principles of hierarchy and scale, while just 7% fully considered landscape sensitivity. The latter principle refers to patch sensitivities and associated differences in the recovery or response following a disturbance across river networks. One type of restoration activity will, therefore, result in variable responses in different parts of the river network. As 61% of methods did not consider landscape sensitivity, a majority of activities are unlikely to be cognizant of place or sympathetic to the natural processes occurring within a given patch. Only 2 of the 44 methods fully consider all three principles of river science, which suggests there is significant scope for growth in this field to increase the suitability of methods in the context of repairing Anthropocene rivers.
Of the five main river restoration categories, activities that have a command-and-control or cookbook approach are the most unsuitable for successful repair of rivers as they lack adequate incorporation of resilience thinking, landscape ecology, and river science. Carbon copy and fast-forwarding activities tend to be aware of natural heterogeneity across landscapes, while command-and-control or cookbook methods often disregard this key tenet of landscape ecology. For both command-and-control and cookbook methods, 67% had no or only partial consideration for principles of landscape ecology. Of the three methods that do not consider any tenets of this framework, two were driven by command-and-control practices, and one was categorized by a cookbook approach. Activities with a reliance on engineered structures often do not recognize the implications of simplification and homogenization through anthropogenic activity. Of all five methods, a disregard for resilience thinking was the dominant outcome of this assessment. Of these methods, 100% that were categorized by a command-and-control, carbon copy, cookbook, or fast-forwarding approach either entirely disregard or only partially consider tenets of resilience thinking. Full consideration of an aspect of resilience thinking was only seen in 8% of activities driven by a field of dreams approach.
Of the 44 evaluated activities, the field of dreams approach was the most dominant method guiding river restoration. The field of dreams approach to river restoration had a greater proportion of activities that fully considered or incorporated principles of landscape ecology (67%) and river science (49%). Nine methods with a field of dreams approach in this evaluation fully consider all three aspects of landscape ecology, while only three methods in this category involve full incorporation of the tenets related to river science. A majority, 83%, of river restoration methods undertaken based on the field of dreams hypothesis are aware of the need to increase habitat heterogeneity in response to anthropogenic homogenization. The potential of successful outcomes from a field of dreams approach is reduced by several key principles: 46% of methods ignore principles of landscape sensitivity, 96% do not consider the implications of alternative stable states, 75% disregard principles of irreversibility, and 50% of evaluated activities do not consider adaptive capacity.
According to this evaluation, current river restoration methods are not fully aware of the key tenets of resilience thinking, landscape ecology, and river science. We argue that this contributes to a low likelihood of successful outcomes, following efforts to improve, restore, or rehabilitate rivers in the Anthropocene. Principles of resilience thinking are frequently absent in current river restoration methods, indicating that many activities operate under the paradigm of an unconscious bias of restoration, with the assumption that a return to an ‘original’ condition may be achievable (Figure 7). Without greater incorporation of the key principles discussed in this framework, unsuitable investment in river restoration will likely continue. This evaluation highlights the need for this framework, which involves a conscious effort and choice toward the development of a new generation of river repair methods more suited to the metamorphosed rivers of the Anthropocene and based on resilience thinking, landscape ecology, and river science.
FIGURE 7. Looking to the future with river repair. Anthropocene rivers operate within a basin of attraction, governed by different interactions, functions, and feedbacks. Restoration activities currently have an unconscious bias, which assumes recovery to “natural.” Resilience thinking implies Anthropocene rivers cannot be returned to an original state, so we must repair rivers in the Anthropocene to something else. This would involve conscious effort or choice. Repair activities must focus on increasing physical heterogeneity while being aware of place in the river network and differing sensitivities to disturbance. Response trajectories of rivers, following restoration as a disturbance, are currently unknown; rivers have complex and unpredictable response patterns, which suggest multiple possible paths forward to a desired state.
Current river assessment and restoration methods look backward as they operate under a ‘natural’ paradigm (Figure 7). Activities are implemented, and the underlying framework or guiding principles are retrofitted. This manuscript argues that restoration is no longer appropriate in the Anthropocene, which is characterized by metamorphosed rivers, and we must look forward to river repair. In response to the current lack of suitable methods, approaches, or guidelines, this framework—built on resilience thinking, landscape ecology, and river science—provides the concepts and context for river repair in the Anthropocene, from which models and subsequent strategies may be developed.
This manuscript presents a framework for the repair of rivers in the Anthropocene with three building blocks. Resilience thinking recognizes the potential for alternative stable states in ecosystems and that the Anthropocene acts as a new regime, within which rivers are metamorphosed. They are characterized by different structures, interactions, processes, functions, and feedback. An outcome of resilience thinking suggests that restoration may not result in recovery back to an original condition when a river has already changed state. For such rivers, the focus should be on repair to enhance adaptive capacity and prevent further uncontrolled flips and associated loss of ecosystem services. Principles of landscape ecology emphasize that riverscapes are naturally heterogeneous and that a focus on building heterogeneity at multiple scales would increase biodiversity, species redundancy, and system resilience and, thereby, adaptive capacity. In river science, it is understood that river landscapes are arranged hierarchically. Thus, different parts of a river network will have various sensitivities to disturbance and associated recovery trajectories. Current river restoration methods based on principles of command-and-control, carbon copy, fast-forwarding, cookbook, or field of dreams are unsuitable and often assume that a return to an original condition is possible. Anthropocene rivers have changed fundamentally, and with this knowledge, the unconscious bias of restoration must also change to one of acknowledging what is possible within an Anthropocene river. Will we continue to aim for an unrealistic goal of ‘natural’, or can we look to the future with river repair?
The original contributions presented in the study are included in the article/supplementary material; further inquiries can be directed to the corresponding author.
RG led the design, analysis, and writing of the manuscript. MT contributed to the design, analysis, and writing of the manuscript. MP contributed to the writing of the manuscript. All authors contributed to the article and approved the submitted version.
The authors thank three reviewers for their feedback and suggestions, which greatly strengthened the quality of this manuscript.
The authors declare that the research was conducted in the absence of any commercial or financial relationships that could be construed as a potential conflict of interest.
All claims expressed in this article are solely those of the authors and do not necessarily represent those of their affiliated organizations, or those of the publisher, the editors, and the reviewers. Any product that may be evaluated in this article, or claim that may be made by its manufacturer, is not guaranteed or endorsed by the publisher.
Abbe, T., and Brooks, A. (2011). “Geomorphic, engineering, and ecological considerations when using wood in river restoration,” in Stream restoration in dynamic fluvial systems: Scientific approaches, analyses, and tools. Editors A. Simon, S. J. Bennett, and J. M. Castro (Washington, DC: American Geophysical Union), 419–451.
Auerbach, D. A., Deisenroth, D. B., McShane, R. R., McCluney, K. E., and Poff, N. L. (2014). Beyond the concrete: Accounting for ecosystem services from free-flowing rivers. Ecosyst. Serv. 10, 1–5. doi:10.1016/j.ecoser.2014.07.005
Beechie, T. J., Fogel, C., Nicol, C., and Timpane-Padgham, B. (2021). A process-based assessment of landscape change and salmon habitat losses in the Chehalis River basin, USA. PLoS ONE 16 (11), e0258251. doi:10.1371/journal.pone.0258251
Beechie, T. J., Richardson, J. S., Gurnell, A. M., and Negishi, J. (2013). “Watershed processes, human impacts, and process-based restoration,” in Stream and watershed restoration: A guide to restoring riverine processes and habitats. Editors P. Roni, and T. Beechie (West Sussex, UK: Wiley-Blackwell), 11–49.
Beechie, T. J., Sear, D. A., Olden, J. D., Pess, G. R., Buffington, J. M., Moir, H., et al. (2010). Process-based principles for restoring river ecosystems. BioScience 60 (3), 209–222. doi:10.1525/bio.2010.60.3.7
Beisner, B. E., Haydon, D. T., and Cuddington, K. (2003). Alternative stable states in ecology. Front. Ecol. Environ. 1 (7), 376–382. doi:10.1890/1540-9295(2003)001[0376:assie]2.0.co;2
Bernhardt, E. S., Palmer, M. A., Alexander, G., Barnas, K., Brooks, S., Carr, J., et al. (2005). Synthesizing the U.S. River restoration efforts. Sci. Supporting Online Material, 1–25.
Bernhardt, E. S., Sudduth, E. B., Palmer, M. A., Allan, J. D., Meyer, J. L., Alexander, G., et al. (2007). Restoring rivers one reach at a time: Results from a survey of U.S. River restoration practitioners. Restor. Ecol. 15 (3), 482–493. doi:10.1111/j.1526-100x.2007.00244.x
Best, J. (2019). Anthropogenic stresses on the world’s big rivers. Nat. Geosci. 12, 7–21. doi:10.1038/s41561-018-0262-x
Beveridge, R., and Moss, T. (2008). “The parrett catchment project: Between rhetoric and reality,” in Restoring floodplains in europe: Policy contexts and project experiences. Editors T. Moss, and J. Monstadt (London, UK: IWA Publishing), 286–316.
Biggs, R., Schulter, M., and Schoon, M. L. (2012). Principles for building resilience: Sustaining ecosystem service in Social-Ecological systems. Cambridge: Cambridge University Press.
Biron, P. M., Carre, D. M., Carver, R. B., Rodrigue-Gervais, K., and Whiteway, S. L. (2011). “Combining field, laboratory, and three-dimensional numerical modeling approaches to improve our understanding of fish habitat restoration schemes,” in Stream restoration in dynamic fluvial systems: Scientific approaches, analyses, and tools. Editors A. Simon, S. J. Bennett, and J. M. Castro (Washington, DC: American Geophysical Union), 209–231.
Bostelmann, R., Braukmann, U., Briem, E., Fleischhacker, T., Humborg, G., Nadolny, I., et al. (1998). “An approach to classification of natural streams and floodplains in south-west Germany,” in Rehabilitation of rivers: Principles and implementation. Editors L. C. deWaal, A. R. G. Large, and P. M. Wade (England, UK: John Wiley & Sons Ltd), 31–55.
Brierley, G., and Fryirs, K. (2009). Don't fight the site: Three geomorphic considerations in catchment-scale river rehabilitation planning. Environ. Manag. 43, 1201–1218. doi:10.1007/s00267-008-9266-4
Brierley, G., Fuller, I., Williams, G., Hikuroa, D., and Tilley, A. (2022). Re-imagining wild rivers in Aotearoa New Zealand. Land 11, 1272. doi:10.3390/land11081272
Briggs, M. K., Hinojosa-Huerta, O., Osterkamp, W. R., Shafroth, P. B., Sifuentes, C., White, L., et al. (2020). “A case for stream corridor restoration,” in Renewing our rivers: Stream corridor restoration in dryland regions. Editors M. K. Briggs, and W. R. Osterkamp (Tucson: The University of Arizona Press), 3–12.
Brooks, S. S., and Lake, P. S. (2007). river restoration in victoria, Australia: Change is in the wind, and none too soon. Restor. Ecol. 15 (3), 584–591. doi:10.1111/j.1526-100x.2007.00253.x
Campbell, D. A., Cole, C. A., and Brooks, R. P. (2002). A comparison of created and natural wetlands in Pennsylvania, USA. Wetl. Ecol. Manag. 10, 41–49. doi:10.1023/a:1014335618914
Carpenter, S., Walker, B., Anderies, J. M., and Abel, N. (2001). From metaphor to measurement: Resilience of what to what? Ecosystems 4, 765–781. doi:10.1007/s10021-001-0045-9
Chessman, B. C., Fryirs, K. A., and Brierley, G. J. (2006). Linking geomorphic character, behaviour and condition to fluvial biodiversity: Implications for river management. Aquatic Conservation Mar. Freshw. Ecosyst. 16, 267–288. doi:10.1002/aqc.724
Clements, F. E. (1936). Nature and structure of the climax. J. Ecol. 24, 252–284. doi:10.2307/2256278
Colloff, M. J., and Baldwin, D. S. (2010). Resilience of floodplain ecosystems in a semi-arid environment. Rangel. J. 32, 305–314. doi:10.1071/rj10015
Crutzen, P. J. (2006). “The “Anthropocene”,” in Earth system science in the Anthropocene. Editors E. Ehlers, and T. Krafft (Berlin, Heidelberg: Springer). doi:10.1007/3-540-26590-2_3
Cumming, G. S. (2011). The resilience of big river basins. Water Int. 36 (1), 63–95. doi:10.1080/02508060.2011.541016
Death, R. G. (2023). “The resilience of riverine ecological communities,” in Resilience and riverine landscapes. Editors M. C. Thoms, and I. Fuller (Netherlands: Elsevier).
DeBoer, J. A., Thoms, M. C., Casper, A. F., and Delong, M. D. (2019). The response of fish diversity in a highly modified large river system to multiple anthropogenic stressors. J. Geophys. Res. Biogeosciences 124, 384–404. doi:10.1029/2018JG004930
DeBoer, J. A., Thoms, M. C., Delong, M. D., Parsons, M. E., and Casper, A. F. (2020). Heterogeneity of ecosystem function in an “Anthropocene” river system. Anthropocene 31, 100252. doi:10.1016/j.ancene.2020.100252
Delong, M. D., and Thoms, M. C. (2016). “An ecosystem framework for river science and management,” in River science: Research and management for the 21stcentury. Editors D. J. Gilvear, M. T. Greenwoods, M. C. Thoms, and P. J. Wood (West Sussex, UK: Wiley Blackwell).
Dollar, E. S. J., James, C. S., Rogers, K. H., and Thoms, M. C. (2007). A framework for interdisciplinary understanding of rivers as ecosystems. Geomorphology 89, 147–162. doi:10.1016/j.geomorph.2006.07.022
Ellis, E. C. (2015). Ecology in an anthropogenic biosphere. Ecol. Monogr. 85, 287–331. doi:10.1890/14-2274.1
Feld, C. K., Birk, S., Bradley, D. C., Hering, D., Kail, J., Marzin, A., et al. (2011). From natural to degraded rivers and back again: A test of restoration ecology theory and practice. Adv. Ecol. Res. 44, 119–209. doi:10.1016/B978-0-12-374794.00003-1
Folke, C. (2006). Resilience: The emergence of a perspective for social-ecological systems analyses. Glob. Environ. Change 16, 253–267. doi:10.1016/j.gloenvcha.2006.04.002
Frainer, A., Polvi, L. E., Jansson, R., and McKie, B. G. (2017). Enhanced ecosystem functioning following stream restoration: The roles of habitat heterogeneity and invertebrate species traits. J. Appl. Ecol. 55, 377–385. doi:10.1111/1365-2664.12932
Fryirs, K. A., and Brierley, G. J. (2013). Geomorphic analysis of river systems: An approach to reading the landscape. Wiley-Blackwell. West Sussex, EK.
Fuglsang, A. (1998). “Rehabilitation of rivers by using wet meadows as nutrient filters,” in Rehabilitation of rivers: Principles and implementation. Editors L. C. deWaal, A. R. G. Large, and P. M. Wade (England, UK: John Wiley & Sons Ltd), 97–111.
Gilvear, D. J., Greenwood, M. T., Thoms, M. C., and Wood, P. J. (2016). River Science: Research and Management for the 21stcentury. West Sussex, EK: Wiley Blackwell.
Gippel, C. J., and Collier, K. J. (1998). “Degradation and rehabilitation of waterways in Australia and New Zealand,” in Rehabilitation of rivers: Principles and implementation. Editors L. C. deWaal, A. R. G. Large, and P. M. Wade (England, UK: John Wiley & Sons Ltd), 269–300.
Gippel, C. J., and Fukutome, S. (1998). “Rehabilitation of Japan’s waterways,” in Rehabilitation of rivers: Principles and implementation. Editors L. C. deWaal, A. R. G. Large, and P. M. Wade (England, UK: John Wiley & Sons Ltd), 301–317.
Gordon, L. J., Peterson, G. D., and Bennett, E. M. (2008). Agricultural modifications of hydrological flows create ecological surprises. Trends Ecol. Evol. 23 (4), 211–219. doi:10.1016/j.tree.2007.11.011
Grill, G., Lehner, B., Thieme, M., Geenen, B., Tickner, D., Antonelli, F., et al. (2019). Mapping the world’s free-flowing rivers. Nature 569 (7755), 215–221. doi:10.1038/s41586-019-1111-9
Gumiero, B., Salmoiraghi, G., Rizzoli, M., and Santini, R. (1998). “Rehabilitation of the acque alte drainage canal on the riverriver Po alluvial plain, Italy,” in Rehabilitation of rivers: Principles and implementation. Editors L. C. deWaal, A. R. G. Large, and P. M. Wade (England, UK: John Wiley & Sons Ltd), 251–267.
Gunderson, L. H. (2000). Ecological resilience – In theory and application. Annu. Rev. Ecol. Syst. 31, 425–439. doi:10.1146/annurev.ecolsys.31.1.425
Gunderson, L. H., and Holling, C. S. (2002). Panarchy: Understanding transformations in human and natural systems. Washington, DC: Island Press.
Hagemeier, M., and Klaphake, A. (2008a). “Restoring an alluvial forest and ancient meander on the garonne: Linking environmental protection to fishing and recreation,” in Restoring floodplains in europe: Policy contexts and project experiences. Editors T. Moss, and J. Monstadt (London, UK: IWA Publishing), 177–200.
Hagemeier, M., and Klaphake, A. (2008b). “Restoring floodplains on the river seine: Combining flood prevention with regional development,” in Restoring floodplains in europe: Policy contexts and project experiences. Editors T. Moss, and J. Monstadt (London, UK: IWA Publishing), 261–285.
Healthy Rivers Commission (Hrc), (1996). Independent inquiry into the williams river, final report. Sydney: Healthy Rivers Commission of New South Wales.
Hester, E. T., and Goosett, M. N. (2011). “Hyporheic restoration in streams and rivers,” in Stream restoration in dynamic fluvial systems: Scientific approaches, analyses, and tools. Editors A. Simon, S. J. Bennett, and J. M. Castro (Washington, DC: American Geophysical Union), 167–187.
Hilderbrand, R. H., Watts, A. C., and Randle, A. M. (2005). The myths of restoration ecology. Ecol. Soc. 10 (1), 19. doi:10.5751/es-01277-100119
Hobbs, R. J., Higgs, E., and Harris, J. A. (2009). Novel ecosystems: Implications for conservation and restoration. Trends Ecol. Evol. 24, 599–605. doi:10.1016/j.tree.2009.05.012
Hodgson, D., McDonald, J. L., and Hosken, D. J. (2015). What do you mean, 'resilient. Trends Ecol. Evol. 30 (9), 503–506. doi:10.1016/j.tree.2015.06.010
Holling, C. S. (1973). Resilience and stability of ecological systems. Annu. Rev. Ecol. Evol. Syst. 4, 1–23. doi:10.1146/annurev.es.04.110173.000245
Jasperse, P. (1998). “Policy networks and the success of lowland stream rehabilitation projects in The Netherlands,” in Rehabilitation of rivers: Principles and implementation. Editors L. C. deWaal, A. R. G. Large, and P. M. Wade (England, UK: John Wiley & Sons Ltd), 13–29.
Johnson, P. A., Hey, R. D., Tessier, M., and Rosgen, D. L. (2001). Use of vanes for control of scour at vertical wall abutments. J. Hydraulic Eng. 127 (9), 772–778. doi:10.1061/(asce)0733-9429(2001)127:9(772)
Kelly, J. M., Scarpino, P., Berry, H., Syvitski, J., and Meybeck, M. (2018). Rivers of the Anthropocene. California: University of California Press.
Knouft, J. H., Botero-Acosta, A., Wu, C-L., Charry, B., Chu, M. L., Dell, A. I., et al. (2021). Forested riparian buffers as climate adaptation tools for management of riverine flow and thermal regimes: A case study in the meramec river basin. Sustainability 12 (4), 1877. doi:10.3390/su13041877
Kondolf, G. M., Boulton, A. J., O’Daniel, S., Poole, G., Rahel, F. J., Stanley, E. H., et al. (2006). Process-based ecological river restoration: Visualizing three-dimensional connectivity and dynamic vectors to recover lost linkages. Ecol. Soc. 11 (2), 5. doi:10.5751/es-01747-110205
Kondolf, G. M. (1995). Five elements for effective evaluation of stream restoration. Restor. Ecol. 3 (2), 133–136. doi:10.1111/j.1526-100x.1995.tb00086.x
Kondolf, G. M. (2011). “Setting goals in river restoration: When and where can the river “heal itself”,” in Stream restoration in dynamic fluvial systems: Scientific approaches, analyses, and tools. Editors A. Simon, S. J. Bennett, and J. M. Castro (Washington, DC: American Geophysical Union), 29–43.
Kotschy, K., Biggs, R., Daw, T., Folke, C., and West, P. (2015). “Principle 1 – maintain diversity and redundancy,” in Principles for building resilience: Sustaining ecosystem services in social-ecological systems. Editors R. Biggs, M. Schluter, and M. Schoon (Cambridge: Cambridge University Press), 50–75.
Kruse, S. (2008). “The restoration of a floodplain on the upper rhine: Managing the interface of large-scale policy and small-scale implementation,” in Restoring floodplains in europe: Policy contexts and project experiences. Editors T. Moss, and J. Monstadt (London, UK: IWA Publishing), 151–176.
Lake, P. S. (2000). Disturbance, patchiness, and diversity in streams. J. North Am. Benthol. Soc. 19 (4), 573–592. doi:10.2307/1468118
Lake, P. S., Bond, N., and Reich, P. (2007). Linking ecological theory with stream restoration. Freshw. Biol. 52, 597–615. doi:10.1111/j.1365-2427.2006.01709.x
Landis, D. A. (2017). Designing agricultural landscapes for biodiversity-based ecosystem services. Basic Appl. Ecol. 18, 1–12. doi:10.1016/j.baae.2016.07.005
Lawson, J. R., Fryirs, K. A., Lenz, T., and Leisham, M. R. (2015). Heterogeneous flows foster heterogeneous assemblages: Relationships between functional diversity and hydrological heterogeneity in riparian plant communities. Freshw. Biol. 60, 2208–2225. doi:10.1111/fwb.12649
Leuven, R. S. E., and Poudevigne, I. (2002). Riverine landscape dynamics and ecological risk assessment. Freshw. Biol. 47, 845–865. doi:10.1046/j.1365-2427.2002.00918.x
Levin, S. A., Barrett, S., Aniyar, S., Baumol, W., Bliss, C., Bolin, B., et al. (1998). Resilience in natural and socio-economic systems. Environ. Dev. Econ. 3, 221–262. doi:10.1017/s1355770x98240125
Levin, S. A. (1998). Ecosystems and the biosphere as complex adaptive systems. Ecosystems 1, 431–436. doi:10.1007/s100219900037
Lincoln, R., Boxshall, D., and Clark, P. (1998). A dictionary of ecology, evolution and systematics. 2nd ed Cambridge: Cambridge University Press.
Lindenmayer, D. B., and Fischer, J. (2006). Habitat fragmentation and landscape change: An ecological and conservation synthesis. Washington, DC: Island Press.
Matheson, A., Thoms, M., and Reid, M. (2017). Does reintroducing large wood influence the hydraulic landscape of a lowland river system? Geomorphology 292, 128–141. doi:10.1016/j.geomorph.2017.03.035
McCoy, A., Shafroth, P. B., Briggs, M. K., Schlatter, K. J., White, L., Zamora, F., et al. (2020). “Quantifying and securing environmental flows,” in Renewing our rivers: Stream corridor restoration in dryland regions. Editors M. K. Briggs, and W. R. Osterkamp (Tucson: The University of Arizona Press), 169–212.
Moerke, A. H., Gerard, K. J., Latimore, J. A., Hellenthal, R. A., and Lamberti, G. A. (2004). Restoration of an Indiana, USA, stream: Bridging the gap between basic and applied lotic ecology. J. North Am. Benthol. Soc. 23 (3), 647–660. doi:10.1899/0887-3593(2004)023<0647:roaius>2.0.co;2
Monstadt, J. (2008). “The relocation of a dyke on the river elbe: Floodplain management as a challenge for intersectoral and multilevel coordination,” in Restoring floodplains in europe: Policy contexts and project experiences. Editors T. Moss, and J. Monstadt (London, UK: IWA Publishing), 229–260.
Moss, T. (2008). “The long eau floodplain restoration project: “flying by the seat of your pants”,” in Restoring floodplains in europe: Policy contexts and project experiences. Editors T. Moss, and J. Monstadt (London, UK: IWA Publishing), 201–228.
Nardini, A. G. C., and Conte, G. (2021). river management & restoration: What river do we wish for. Water 13, 1336. doi:10.3390/w13101336
Newbury, R., Bates, D., and Alex, K. L. (2011). “Restoring habitat hydraulics with constructed riffles,” in Stream restoration in dynamic fluvial systems: Scientific approaches, analyses, and tools. Editors A. Simon, S. J. Bennett, and J. M. Castro (Washington, DC: American Geophysical Union), 353–366.
Ogston, L., Gidora, S., Foy, M., and Rosenfeld, J. (2015). Watershed-scale effectiveness of floodplain habitat restoration for juvenile coho salmon in the Chilliwack River, British Columbia. Can. J. Fish. Aquatic Sci. 72, 479–490. doi:10.1139/cjfas-2014-0189
Oliver, T. H., Heard, M. S., Isaac, N. J. B., Roy, D. B., Procter, D., Eigenbrod, F., et al. (2015). Biodiversity and resilience of ecosystem functions. Trends Ecol. Evol. 30 (11), 673–684. doi:10.1016/j.tree.2015.08.009
O’Neill, R. V., Johnson, A. R., and King, A. W. (1989). A hierarchical framework for the analysis of scale. Landsc. Ecol. 3, 193–205. doi:10.1007/bf00131538
Ormerod, S. J., Dobson, M., Hildrew, A. G., and Townsend, C. R. (2010). Multiple stressors in freshwater ecosystems. Freshw. Biol. 55, 1–4. doi:10.1111/j.1365-2427.2009.02395.x
Palmer, M. A., Ambrose, R. F., and Poff, N. L. (1997). Ecological theory and community restoration ecology. Restor. Ecol. 5 (4), 291–300. doi:10.1046/j.1526-100x.1997.00543.x
Palmer, M. A., Bernhardt, E. S., Allan, J. D., Lake, P. S., Alexander, G., Brooks, S., et al. (2005). Standards for ecologically successful river restoration. J. Appl. Ecol. 42, 208–217. doi:10.1111/j.1365-2664.2005.01004.x
Palmer, M. A., Filoso, S., and Fanelli, R. M. (2014a). From ecosystems to ecosystem services: Stream restoration as ecological engineering. Ecol. Eng. 65, 62–70. doi:10.1016/j.ecoleng.2013.07.059
Palmer, M. A., Hondula, K. L., and Koch, B. J. (2014b). Ecological restoration of streams and rivers: Shifting strategies and shifting goals. Annu. Rev. Ecol. Evol. Syst. 45, 247–269. doi:10.1146/annurev-ecolsys-120213-091935
Palmer, M. A., Menninger, H. L., and Bernhardt, E. (2009). river restoration, habitat heterogeneity and biodiversity: A failure of theory or practice? Freshw. Biol. 55 (1), 205–222. doi:10.1111/j.1365-2427.2009.02372.x
Palmer, M. A., and Stewart, G. A. (2020). Ecosystem restoration is risky … but we can change that, but we can change that. One Earth 3, 661–664. doi:10.1016/j.oneear.2020.11.019
Parsons, M., Thoms, M., Capon, T., Capon, S., and Reid, M. (2009). Resilience and thresholds in river ecosystems (Water Report Series No 21). Turner: Australia Government National Water Commission.
Parsons, M., and Thoms, M. C. (2018). From academic to applied: Operationalising resilience in river systems. Geomorphology 305, 242–251. doi:10.1016/j.geomorph.2017.08.040
Pingram, M., Price, J., and Thoms, M. (2019). Integrating multiple aquatic values: Perspectives and a collaborative future for river science. River Res. Appl. 35, 1607–1614. doi:10.1002/rra.3562
Pickett, S. T. A., and White, P. S. (1985). The ecology of natural disturbance and patch dynamics. Orlando, Florida: Academic Press.
Piegay, H., Darby, S. E., Mosselman, E., and Surian, N. (2005). A review of techniques available for delimiting the erodible river corridor: A sustainable approach to managing bank erosion. River Res. Appl. 21, 773–789. doi:10.1002/rra.881
Pinter, N., Brasington, J., Gurnell, A., Kondolf, G. M., Tockner, K., Wharton, G., et al. (2019). River research and applications across borders. River Res. Appl. 35, 3430–3775. doi:10.1002/rra.3430
Pollock, M. M., Beechie, T. J., Wheaton, J. M., Jordan, C. E., Bouwes, N., Weber, N., et al. (2014). Using beaver dams to restore incised stream ecosystems. BioScience 64 (4), 279–290. doi:10.1093/biosci/biu036
Poole, G. C. (2002). Fluvial landscape ecology: Addressing uniqueness within the river discontinuum. Freshw. Biol. 47, 641–660. doi:10.1046/j.1365-2427.2002.00922.x
Price, P., Lovett, S., and Davies, P. (2009). A national synthesis of river restoration projects. (Canberra: National Water Commission, Australian Government). Waterlines report series.
Quinn, G. P., and Keough, M. J. (2002). Experimental design and data analysis for biologists. Cambridge, UK: Cambridge University Press.
Raven, P. J., Holmes, N. T. H., Naura, M., and Dawson, F. H. (2000). Using river habitat survey for environmental assessment and catchment planning in the U.K. Hydrobiologia 422/423, 359–367. doi:10.1023/a:1017026417664
Resh, V. H., Brown, A. V., Covich, A. P., Gurtz, M. E., Li, H. W., Minshall, G. W., et al. (1998). The role of disturbance in stream ecology. J. North Am. Benthol. Soc. 7, 433–455. doi:10.2307/1467300
Rhoads, B. L., Engel, F. L., and Abad, J. D. (2011). “Pool-riffle design based on geomorphological principles for naturalizing straight channels,” in Stream restoration in dynamic fluvial systems: Scientific approaches, analyses, and tools. Editors A. Simon, S. J. Bennett, and J. M. Castro (Washington, DC: American Geophysical Union), 367–384.
Rinaldi, M., Piegay, H., and Surian, N. (2011). “Geomorphological approaches for river management and restoration in Italian and French rivers,” in Stream restoration in dynamic fluvial systems: Scientific approaches, analyses, and tools. Editors A. Simon, S. J. Bennett, and J. M. Castro (Washington, DC: American Geophysical Union), 95–113.
Rosenfeld, J. S. (2002). Functional redundancy in ecology and conservation. Oikos 98, 156–162. doi:10.1034/j.1600-0706.2002.980116.x
Rosgen, D. L. (1994). A classification of natural rivers. Catena 22, 169–199. doi:10.1016/0341-8162(94)90001-9
Rosgen, D. L. (2011). “Natural Channel design: Fundamental concepts, assumptions, and methods,” in Stream restoration in dynamic fluvial systems: Scientific approaches, analyses, and tools. Editors A. Simon, S. J. Bennett, and J. M. Castro (Washington, DC: American Geophysical Union), 69–93.
Rosi-Marshall, E. J., Moerke, A. H., and Lamberti, G. A. (2006). Ecological responses to trout habitat rehabilitation in a Northern Michigan stream. Environ. Manag. 38 (1), 99–107. doi:10.1007/s00267-005-0177-3
Sabater, S., Elosegi, A., and Ludwig, R. (2019). Multiple stressors in river ecosystems: Status, impacts and prospects for the future. Netherlands: Elsevier, 392pp.
Scheffer, M., and Carpenter, S. R. (2003). Catastrophic regime shifts in ecosystems: Linking theory to observation. Trends Ecol. Evol. 18 (12), 648–656. doi:10.1016/j.tree.2003.09.002
Schumm, S. A. (1969). River metamorphosis. J. Hydraulics Div. ASCE 95, 255–274. doi:10.1061/jyceaj.0001938
Shields, F. D., Morin, N., and Cooper, C. M. (2004). Large woody debris structures for sand-bed channels. J. Hydraulic Eng. 130 (3), 208–217. doi:10.1061/(asce)0733-9429(2004)130:3(208)
Simons, J., and Boeters, R. (1998). “A systematic approach to ecologically sound river bank management,” in Rehabilitation of rivers: Principles and implementation. Editors L. C. deWaal, A. R. G. Large, and P. M. Wade (England, UK: John Wiley & Sons Ltd), 57–85.
Soar, P. J., and Thorne, C. R. (2001). Channel restoration design for meandering rivers. Fort Collins: Colorado state universityt.
Sousa, W. P. (1984). The role of disturbance in natural communities. Annu. Rev. Ecol. Syst. 15, 353–391. doi:10.1146/annurev.es.15.110184.002033
Southwood, T. R. E. (1977). Habitat, the template for ecological strategies? J. Animal Ecol. 46, 337–365.
Stanley, E. H., Powers, S. M., and Lottig, N. R. (2010). The evolving legacy of disturbance in stream ecology: Concepts, contributions, and coming challenges. J. or North Am. Benthol. Soc. 29 (1), 67–83. doi:10.1899/08-027.1
Steffen, W., Broadgate, W., Deutsch, L., Gaffney, O., and Ludwig, C. (2015). The trajectory of the Anthropocene: The great acceleration. Anthropocene Rev. 2 (1), 81–98. doi:10.1177/2053019614564785
Steffen, W., Rockstrom, J., Richardson, K., Schellnhuber, H. J., Folke, C., Liverman, D., et al. (2018). Trajectories of the Earth system in the Anthropocene. Proc. Natl. Acad. Sci. 115, 8252–8259. doi:10.1073/pnas.1810141115
Stewart, A. J. A., John, E. A., and Hutchings, M. J. (1999). “The world is heterogeneous: Ecological consequences of living in a patchy environment,” in The ecological consequences of environmental heterogeneity. Editors M. J. Hutchings, E. A. John, and A. J. A. Stewart (Hoboken: Blackwell Science).
Thoms, M. C., Delong, M. D., Flotemersch, J. E., and Collins, S. E. (2017). Physical heterogeneity and aquatic community function in river networks: A case study from the kanawha river basin, USA. Geomorphology 290, 277–287.
Thoms, M. C., Gilvear, D. J., Greenwood, M. T., and Wood, P. J. (2016). “An introduction to river science: Research and applications,” in River Science. Research and Management for the 21stcentury. Editors D. J. Gilvear, M. T. Greenwood, M. C. Thoms, and P. J. Wood (Chichester: Wiley Blackwell).
Thoms, M. C., and Parsons, M. (2002). Ecogeomorphology: An interdisciplinary approach to river science. Int. Assoc. Hydrological Sci. 276, 113–120.
Thoms, M. C. (2006). Variability in riverine ecosystems. River Res. Appl. 22, 115–121. doi:10.1002/rra.900
van Cappellen, P., and Maavara, T. (2016). Rivers in the Anthropocene: Global scale modifications of riverine nutrient fluxes by damming. Ecohydrol. Hydrobiology 16 (2), 106–111. doi:10.1016/j.ecohyd.2016.04.001
Van Looy, K., Tonkin, J. D., Floury, M., Leigh, C., Soininen, J., Larsen, S., et al. (2019). The three Rs of river ecosystem resilience: Resources, recruitment, and refugia. River Res. Appl. 35, 107–120. doi:10.1002/rra.3396
van Rijen, J. P. M. (1998). “Practical approaches for nature development: Let nature do its own thing again,” in Rehabilitation of rivers: Principles and implementation. Editors L. C. deWaal, A. R. G. Large, and P. M. Wade (England, UK: John Wiley & Sons Ltd), 113–130.
Vorosmarty, C. J., McIntyre, P. B., Gessner, M. O., Dudgeon, D., Pruservich, A., Green, P., et al. (2010). Global threats to human water security and river biodiversity. Nature 467, 555–561. doi:10.1038/nature09440
Walker, B., Carpenter, S. R., Holling, C. S., and Kinzig, A. (2004). Resilience, adaptability and transformability in social-ecological systems. Ecol. Soc. 9 (2), 5. doi:10.5751/es-00650-090205
Walker, B. (1995). Conserving biological diversity through ecosystem resilience. Conserv. Biol. 9 (4), 747–752. doi:10.1046/j.1523-1739.1995.09040747.x
Walker, B., and Salt, D. (2006). Resilience thinking: Sustaining ecosystems and people in a changing world. Washington, DC: Island Press.
Water Resources Commission (New South Wales), (1976). Namoi River investigations. Water resources commission. River Improvement Branch: Department of Land and Water Conservation.
Webb, A. A., and Erskine, W. D. (2003). A practical scientific approach to riparian vegetation rehabilitation in Australia. J. Environ. Manag. 68, 329–341. doi:10.1016/s0301-4797(03)00071-9
Wellnitz, T., and Poff, N. L. (2001). Functional redundancy in heterogeneous environments: Implications for conservation. Ecol. Lett. 4, 177–179. doi:10.1046/j.1461-0248.2001.00221.x
Wheaton, J. M., Darby, S. E., and Sear, D. A. (2008). “The scope of uncertainties in river restoration,” in river restoration: Managing the uncertainty in restoring physical habitat. Editors S. Darby, and D. Sear (West Sussex, England: John Wiley & Sons Ltd), 21–39.
Wiens, J. A. (1999). “Ecological heterogeneity: An ontogeny of concepts and approaches,” in The ecological consequences of environmental heterogeneity. Editors M. J. Hutchings, E. A. John, and A. J. A. Stewart (Hoboken: Blackwell Science).
Wiens, J. A. (2002). Riverine landscapes: Taking landscape ecology into the water. Freshw. Biol. 47, 501–515. doi:10.1046/j.1365-2427.2002.00887.x
Wissmar, R. C., and Beschta, R. L. (1998). Restoration and management of riparian ecosystems: A catchment perspective. Freshw. Biol. 40, 571–585. doi:10.1046/j.1365-2427.1998.00383.x
Wohl, E., Lane, S. N., and Wilcox, A. C. (2015). The science and practice of river restoration. Water Resour. Res. 51, 5974–5997. doi:10.1002/2014WR016874
Wohl, E. (2020). Rivers in the Anthropocene: The U.S. Perspective. Geomorphology 266, 106600. doi:10.1016/j.geomorph.2018.12.001
Wohl, E. (2011). “Seeing the forest and the trees: Wood in stream restoration in the Colorado front range, United States,” in Stream restoration in dynamic fluvial systems: Scientific approaches, analyses, and tools. Editors A. Simon, S. J. Bennett, and J. M. Castro (Washington, DC: American Geophysical Union), 399–418.
Wyzga, B., Oglecki, P., Radecki-Pawlik, A., and Zawiejska, J. (2011). “Diversity of macroinvertebrate communities as a reflection of habitat heterogeneity in a mountain river subjected to variable human impacts,” in Stream restoration in dynamic fluvial systems: Scientific approaches, analyses, and tools. Editors A. Simon, S. J. Bennett, and J. M. Castro (Washington, DC: American Geophysical Union), 189–207.
Yrjana, T. (1998). “Efforts for in-stream fish habitat restoration within the river iijoki, Finland – goals, methods and test results,” in Rehabilitation of rivers: Principles and implementation. Editors L. C. deWaal, A. R. G. Large, and P. M. Wade (England, UK: John Wiley & Sons Ltd), 239–250.
Zalewski, M., and Frankiewicz, P. (1998). “The influence of riparian ecotones on the dynamics of riverine fish communities,” in Rehabilitation of rivers: Principles and implementation. Editors L. C. deWaal, A. R. G. Large, and P. M. Wade (England, UK: John Wiley & Sons Ltd), 87–96.
Zedler, J. B. (1996). Ecological issues in wetland mitigation: An introduction to the forum. Ecol. Appl. 6 (1), 33–37. doi:10.2307/2269550
Keywords: resilience, landscape ecology, river science, disturbance, unconscious bias
Citation: Greene RH, Thoms MC and Parsons M (2023) We cannot turn back time: a framework for restoring and repairing rivers in the Anthropocene. Front. Environ. Sci. 11:1162908. doi: 10.3389/fenvs.2023.1162908
Received: 10 February 2023; Accepted: 17 May 2023;
Published: 01 June 2023.
Edited by:
Gordon O'Brien, University of Mpumalanga, South AfricaReviewed by:
Guangde Jiang, University of Illinois at Urbana-Champaign, United StatesCopyright © 2023 Greene, Thoms and Parsons. This is an open-access article distributed under the terms of the Creative Commons Attribution License (CC BY). The use, distribution or reproduction in other forums is permitted, provided the original author(s) and the copyright owner(s) are credited and that the original publication in this journal is cited, in accordance with accepted academic practice. No use, distribution or reproduction is permitted which does not comply with these terms.
*Correspondence: Rachel H. Greene, cmdyZWVuZTJAbXl1bmUuZWR1LmF1
†ORCID: Rachel H. Greene, https://orcid.org/0000-0002-3432-5220; Martin C. Thoms, https://orcid.org/0000-0002-8074-0476; Melissa Parsons, https://orcid.org/0000-0002-3918-7306
Disclaimer: All claims expressed in this article are solely those of the authors and do not necessarily represent those of their affiliated organizations, or those of the publisher, the editors and the reviewers. Any product that may be evaluated in this article or claim that may be made by its manufacturer is not guaranteed or endorsed by the publisher.
Research integrity at Frontiers
Learn more about the work of our research integrity team to safeguard the quality of each article we publish.