- 1Department of Biology, Faculty of Science, Akdeniz University, Antalya, Türkiye
- 2Department of Environmental Protection Technologies, Vocational School of Technical Sciences, Recep Tayyip Erdoğan University, Rize, Türkiye
- 3Department of Marine Biology, Faculty of Fisheries, Recep Tayyip Erdoğan University, Rize, Türkiye
Understanding the biogeochemical processes of particulate organic matter occurring in the river under anthropogenic disturbances and its transport to the coastal system is important for environmental resource management. In this study, we investigated the sources and distribution of particulate organic matter (POM) from the upper reaches of the Iyidere River, Türkiye, to the coastal water of the Black Sea during the fall and spring seasons using the elemental (POC and PON (%), C/N), isotopic (δ13C and δ15N), and Bayesian mixing model (MixSIAR) analysis. The POC (%), PON (%), and C/N of POM varied seasonally, indicating that the composition of POM varied with river hydrology, which varies depending on the climate of the region. Both the mixing model and the isotopic and elemental ratios of POM have revealed that the organic matter sources contributing to the riverine of POM, during the fall season, when the precipitation is severe, exhibited a uniform distribution. Heavy rain increased soil erosion along the high-slope land, and as a result, soil and bacteria were identified as the main contributor of POM along the Iyidere River. The results showed that the organic matter sources contributing to POM in the spring season showed significant spatial variation. Terrestrial vegetation, soil OM, and bacteria were the main contributors of POM depending on sites, and these contributions did not show a regular trend along the river. δ15N of POM had significant spatial variation in both seasons that was likely caused by nitrogen inputs derived from anthropogenic activities along the river. The anthropogenic activities and cascade dams causing variations in the contribution of organic matter to the POM are the likely important driving factors in this river-coastal system.
1 Introduction
Freshwater is critical in transporting terrestrial organic matter (OM) from land to sea because it forms an essential part of the global carbon cycle in marine systems (Blair and Aller, 2012). A large amount of transported terrestrial OM accumulates in the coastal zone (Ni et al., 2008) and is eventually buried in marine sediments (a source-to-sink process), participating in the global carbon cycle (Zhang et al., 2021). Therefore, these processes (export and sinking of organic matter and nutrients) significantly affect the biochemical cycle, productivity, and food web dynamics of coastal and marine ecosystems (Caddy, 2000; Wang et al., 2012; Xuan et al., 2020a).
A component of OM in freshwater ecosystems is suspended particulate organic matter (POM), typically measured as particulate organic carbon (POC) and particulate organic nitrogen (PON). Riverine POM usually consists of different sources such as soil OM, terrestrial plants, freshwater phytoplankton, large bacteria, benthic algae, and anthropogenic sources (agricultural fertilizer, industrial and domestic sewages, etc.) (Liu et al., 2019a; Lee et al., 2019). Several studies concluded that POM transported by rivers consists of refractory soil OM, terrestrial vegetation, and detritus and, therefore, plays an important role in the biogeochemistry of coastal areas (Raymond and Bauer, 2001; Wu et al., 2007; Holmes et al., 2008; Bouchez et al., 2014), whereas other studies concluded it to be insignificant (Lobbes et al., 2000; Amon et al., 2003). Seasonal hydrological variation of a river also strongly affects the quantity and quality of POM such as the higher contribution of soil and terrestrial plant in the wet and phytoplankton in the dry season (Lu et al., 2016; Mbaye et al., 2016; Ke et al., 2020).
Globally, river drainage basins have been greatly influenced by anthropogenic disturbances such as urbanization, industrial activity, agriculture fertilization, and dam construction (Butman et al., 2015; Park et al., 2018). These disturbances have substantially changed the POC delivery to the freshwaters and its export to the coastal zone (Wang et al., 2022), hence POM sources (Zhang et al., 2022). For example, fluxes of organic carbon increased in response to deforestation (Moore and Jackson, 1989; Kao and Liu, 1996) and decreased in response to the construction of dams (Ittekkot and Arain, 1986; Wu et al., 2007). Dams may have an important impact on POM composition by increasing the travel time of water, promoting sedimentation, and autochthonous production which is easily decomposable and labile and, therefore, may alter the trophic ecology of coastal waters (Panwar and Yang, 2022). Furthermore, urbanization and agriculture activities changed the quality of POM delivered to the marine system, aggravating soil erosion (Cui et al., 2021; Xu et al., 2021; Liu et al., 2022; Lv et al., 2022) and increasing phytoplankton (Yi et al., 2017; Xuan et al., 2020b; Wang H et al., 2020) and fecal bacteria (Surbeck et al., 2010) contribution to the POM pool caused by nutrient pollution.
The rivers in Northeast Anatolia of Türkiye carried a high amount of sediment to the Black Sea (24 million tons of sediment and 40 km3/year of water discharged per year) due to the highly sloping hydrogeomorphology of the rivers and the high precipitation (Algan, 2006). However, the high slope and river discharges have made this region attractive for dam construction. Today, there are cascade dams on all the rivers in this region and these dams hold 83% (>17 million m3) of the suspended sediments carried along the rivers (Berkun, 2010; Berkun et al., 2015). The Iyidere River is located in Northeast Anatolia which is under the influence of not only cascade dams (river-type cascade hydroelectric power plants (HPP)) but also various anthropogenic disturbances. The Iyidere River Basin is exposed to excessive use of fertilizers for tea agriculture, livestock activities, domestic sewage (do not have sewage systems and wastewater treatment facilities), and industrial activities (such as copper factory, cement, and fishmeal, and fish oil factories) (Dokap, 2013). There is currently a lack of data on the sources and distribution of POM along the river under an anthropogenic disturbance from land to sea in Northeast Anatolia. Previous studies have mostly focused on the nutrient concentrations, quality, and stoichiometry of sediment and POM from the coastal zone (Akbal et al., 2011; Bayram et al., 2011) and open water of the Black Sea (Coban-Yıldız et al., 2000; Çoban-Yıldız, 2006; Ergül and Topcuoğlu, 2009; Akçay and Yücel, 2023).
Based on the aforementioned information, the importance of POM inputs to the Iyidere River and the transport of these OM to the coastal zone of the Black Sea are of concern. Therefore, identifying the POM sources of this basin will provide a theoretical reference for environmental management and ecological assessments for this and other river basins under anthropogenic disturbance. For this study, the elemental (the weight percentages of POC (%) and PON (%), and C/N ratio) and isotopic (δ13C and δ15N) compositions of POM in suspended particulate matter from the Iyidere River mainstream and the coastal zone of the Black Sea were investigated during two seasons (high- and low-rainfall period). The purpose of this study was to i) determine the spatiotemporal variation of the elemental and isotopic composition of POM from the Iyidere River to the Black Sea coast, ii) identify the mechanisms controlling this spatiotemporal variability, and iii) quantify the proportion of the potential OM source’s contribution to the riverine and coastal POM using the MixSIAR mixing model. With these combined techniques (elemental, isotopic, and mixing model analysis), the results can provide comprehensive information on the changes in POM and clarify the impacts of human activities on POM.
2 Materials and methods
2.1 Study sites
This study was carried out in the Iyidere River Basin located in the northeast of Türkiye (Figure 1). It originates within the Soganlı Mountains and flows into the Black Sea. The catchment of this river covers an area of 1047.4 km2 and is 78.4 km long. The average discharge of this stream is 28.35 m³/s. The slope of the stream is 38% (https://tr.wikipedia.org/wiki/%C4%B0yidere_Deresi). The source of the river is about 3000 m above sea level and flows vertically predominantly in the north–northwest direction into the sea in narrow and deep valleys (Ayaz et al., 2013). The eastern Black Sea region of Türkiye is typically characterized by a humid temperate climate (marine climate) sub-type in Köppen classification (Öztürk et al., 2017). Precipitation is plentiful (annual precipitation is 2000–2,500 mm), all seasons are rainy, and there is no dry period. Most precipitation falls in the fall season (from September to November, 804.6 mm) and the least in the spring season (November to April, 334.6 mm) (Dokap, 2013).
In this study, suspended POM samples were collected from eight sampling sites between 2020 and 2021 during the fall (high rainfall, October 2020) and spring (low rainfall, March 2020) seasons (Figure 1). Sampling sites 1 and 2 are located in the upper channel of the river and at an altitude of 643–715 m. The landscape of the upper basin is dominated by natural forests and partly agricultural areas. The most important agricultural product in this basin is the tea plant (Camellia sinensis L.). Sites 3 and 4 were in the middle basin of the river with an altitude of 275–502 m. Sites 5, 6, and 7 were in the lower basin with elevations ranging from 6 to 110 m. The middle basin is under the influence of intensive agricultural activities (tea plantations), while the lower basin is under the influence of agricultural activities, uncontrolled and irregular industrialization, and urbanization (Verep et al., 2012). Since there is no sewerage system in many villages within the basin, wastewater is directly poured into the stream system and discharged into the Black Sea without treatment. In addition, the natural flow of the Iyidere River is interrupted by three (river-type cascade hydroelectric power plants (HPP)) between site 3 and 4, site 4 and 5, and site 5 and 6. Only site 8 is located in the coastal zone of the Black Sea.
2.2 Sampling and stable isotope analysis of particulate organic matter
Surface water samples for the analysis of POM at each riverine site during two seasons were collected about 0.3 m below the water surface using 5-L precleaned plastic and sunproof bottles. Seawater surface samples were collected with a Van Dorn water sampling bottle about 1 m below water surface and placed in 5 L sunproof bottles. The water samples brought to the laboratory in cold storage were filtered through precombusted (550 °C, 5 h) a Whatman GF/F filter (47 mm diameter, and 0.7 μm pore size) (Merck, Darmstadt, Germany) on the same day. Sea salt was removed by using 10 ml of 33% salt solution (Milli-Q water and NaCl-Panreac PA-ACS-ISO) only in filtered seawater samples.
Stable δ13C and δ15N analyses were conducted on two sub-samples of POM surface water for each site and season. One sub-sample was acidified by rinsing with 10 ml 1N HCl to remove inorganic carbonate for δ13C analysis, whereas the other was analyzed without any prior treatment for δ15Ν analysis. The samples treated with acid were then rinsed with Milli-Q water. All samples dried at 60 °C for 48 h in the oven and then were ground to a fine powder and stored in glass vials. About 2–5 mg of carbonate-free samples for δ13C and about 15–30 mg for δ15N analyses were weighed into ultra-pure tin capsules (Elemental Microanalysis Ltd, Okehampton, UK). Samples were analyzed for carbon and nitrogen isotope ratios, and the POC (%) and PN (%) ratios using the Isotope Ratio Mass Spectrometer at the Akdeniz University, Food Safety and Agricultural Research Center (Antalya, Türkiye). Ratios (R) of the heavy to light isotopes (i.e., 13C/12C, 15N/14N) are expressed in parts per thousand, relative to the standards in delta notation according to the following formula:
in which the standards are Pee Dee Belemnite limestone and atmospheric molecular nitrogen for C and N, respectively. Atmospheric nitrogen (for δ15N) and Pee Dee Belemnite (PDB) (for δ13C) were used as the standards. The analytical precision was within 0.2‰ and 0.3‰ for carbon and nitrogen isotope measurements, respectively. The C/N atom ratios of the samples were determined by multiplying the ratio of carbon and nitrogen weight percentages by 1.167 (Leng and Lewis, 2017).
2.3 Data analyses
The proportional contributions of different OM sources to the suspended POM of the Iyidere River were determined using a Bayesian stable isotope mixing model MixSIAR in R (ver. 4.2.1., R Foundation for Statistical Computing, Vienna, Austria). A detailed description of the MixSIAR model was described by Stock et al. (2018). The five main sources of OM of the stream ecosystem are as follows: bacteria, algae, soil, leaf detritus, and macrophytes (Finlay and ve Kendall, 2007). We did not sample any of these OM sources in this study, and therefore, we derived three end-member sources (terrestrial plants, riverine phytoplankton, and soil OM) and their mean and range values of δ13C and δ15N and C/N from previous studies (McKinney et al., 2001; Lamb et al., 2006; Wu et al., 2007; Kaymak et al., 2015; Chen and Jia, 2009; Lammers et al., 2017, Table 1) for this model. We did not include macrophytes because they did not show significant dispersal in this system. We also did not include bacteria because they largely overlapped with the stable C and N isotope ratios of the other three sources because the δ13C of heterotrophic bacteria growing on organic carbon substrates are typically the same as the δ13C of the OM used (Finlay and ve Kendall, 2007). We added another end-member (marine phytoplankton, −22.43 ± 1.7‰ for δ13C and 6.8 ± 3.07‰ for δ15N, Çoban-Yıldız et al., 2006; Banaru et al., 2007; Mutlu, 2019) for site 8 on the Black Sea coast and ran this analysis for two seasons again. Since C/N ratios seem to be a pretty good indicator in separating all these OM sources, the classical C/N vs δ13C and δ15N vs C/N plots were also used to appear in the POM distribution within the ranges of the four end-member sources. For these plots, we also added the bacteria as an end-member and obtained the isotope ratios from the studies of McCallister et al. (2004), Finlay and ve Kendall (2007), Bao et al. (2012), and Lammers et al. (2017).
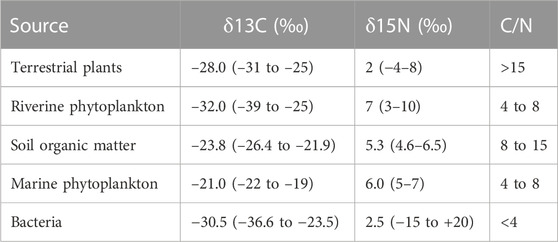
TABLE 1. Mean and range of the δ13C, δ15N, and C/N ratios of potential organic matter sources used by MixSIAR analyses and the scatter plot of δ13C vs C/N ratios and δ15N vs C/N ratios.
Paired t-tests were used to compare the variations in isotopic parameters (POC (%), PN (%), C/N ratios, δ13C, and δ15N) of POM between the fall and spring seasons. After testing for normality (Shapiro–Wilk test) and homogeneity of variance (Levene’s test), one-way ANOVA was performed to assess spatial variation in each season. Relationships between the parameters were investigated with the Pearson correlation coefficient (significant when p < 0.05). All analyses were conducted using R statistical software (version 4.2.1, R Project for Statistical Computing). Principal component analysis (PCA) was performed using PAST 4.0.4 statistics in order to evaluate the relationships between the elemental and isotopic composition of the POM samples and sites.
3 Results
3.1 Spatiotemporal variation of particulate organic matter
The δ13C and δ15N values of POM did not differ among seasons (paired t-test, p > 0.05) (Table 2). There were significant differences in POC (%) and PN (%) of POM among seasons, with the higher ratio in the fall relative to the spring season (paired t-test, p < 0.01 for POC (%) and p < 0.05 for PN (%)). In contrast, the C/N of POM was significantly higher during the spring season than in the fall season (paired t-test, p < 0.05) (Table 2).
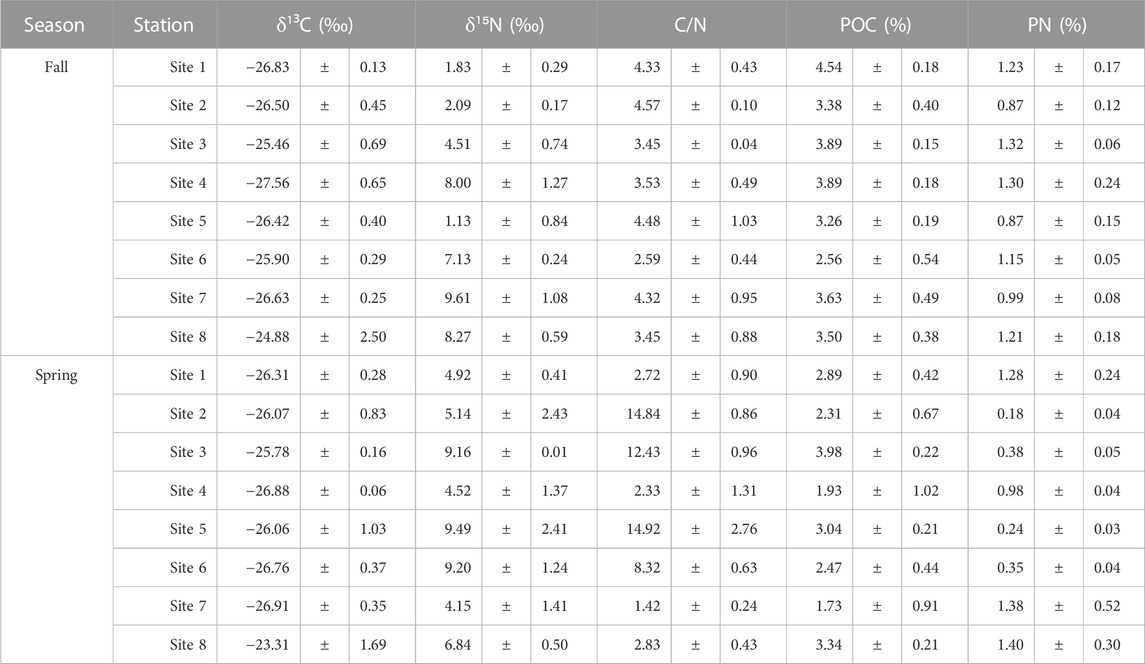
TABLE 2. Elemental and isotopic composition of POM from the Iyidere River to the Black Sea coast during the fall and spring seasons.
During the fall season, although the δ13C values of the POM did not differ among sites (r2 = 0.112, p = 0.205), the δ15N values of sites 1, 2, 3, and 5 were considerably lower than those of sites 4, 6, 7, and 8 (r2 = 0.517, p < 0.001) (Table 2; Figure 2). PN (%) and C/N ratios of POM did not differ significantly among sites (r2 = 0.005, p = 0.804 and r2 = 0.096, p = 0.242, respectively). The POC (%) of POM was the highest at site 1 and the lowest at site 6 (r2 = 0.298, p < 0.05). During the spring season, the δ13C value of site 8 was significantly higher than that of sites 4, 6, and 7 (r2 = 0.286, p < 0.05), while the δ15N values of POM from sites 3, 5, and 6 were higher than those from the other sites (r2 = ,0.291, p < 0.05) (Table 2; Figure 2). PN (%) and C/N of POM varied significantly among sites (r2 = 0.623, p < 0.001 and r2 = 0.556, p < 0.001, respectively), except for POC (%) ratios (r2 = 0.011, p = 0.245). Based on the PN (%) of POM, sites were statistically divided into three groups: sites with low (sites 2, 5, and 6), medium (site 1), and high ratios (sites 7 and 8). The C/N of POM increased at sites 2, 3, and 5 (Table 2; Figure 2).
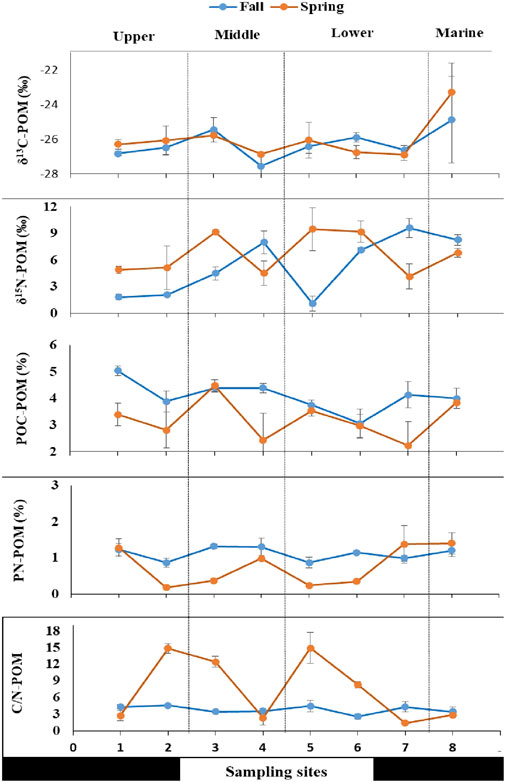
FIGURE 2. Spatiotemporal variation in the elemental and isotopic composition of POM along the Iyidere River. Blue dots represent samples collected in the fall season, and orange dots represent samples collected in the spring season. Error bars indicate standard deviation for each sample.
The two PC axes, PC1 and PC2, explained 67.68% and 25.85%, respectively, of the total variance of POM. The PC 1 axis, which was positively related to C/N (0.90) and separated sites 2, 3, 5, and 6 during the spring season dominated by these features from other sites and seasons (Figure 3). The PC 2 axis has high factor loadings of δ15N (0.90) and clearly distinguished site 6 and 8 for both seasons and site 4 and 7 in the fall season from other sites (Figure 3). The Pearson correlation analysis (Table 3) showed that the PN (%) correlated strongly with the C/N of POM in the fall season, whereas both δ13C and δ15N were found to be positively correlated with POC (%) of POM during the spring season. The C/N ratio of POM correlated positively with δ15N and negatively with PN (%) (Table 3).
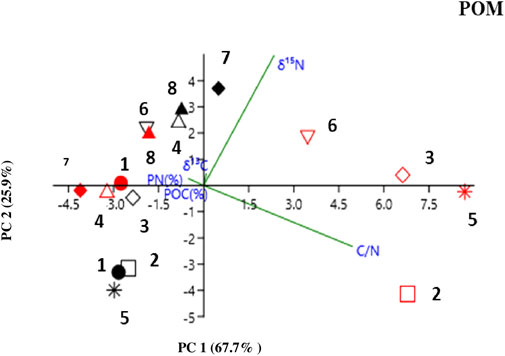
FIGURE 3. PCA results using the elemental and isotopic composition from the fall and spring season samples. Individual sampling sites are indicated by their site number. Black color represents the fall season, and red color represents the spring season (the shapes reflect the site numbers: dot: site 1, square: site 2, diamond: site 3, triangle: site 4, star: site 5, inverted triangle: site 6, filled diamond: site 7, and filled triangle: site 8).
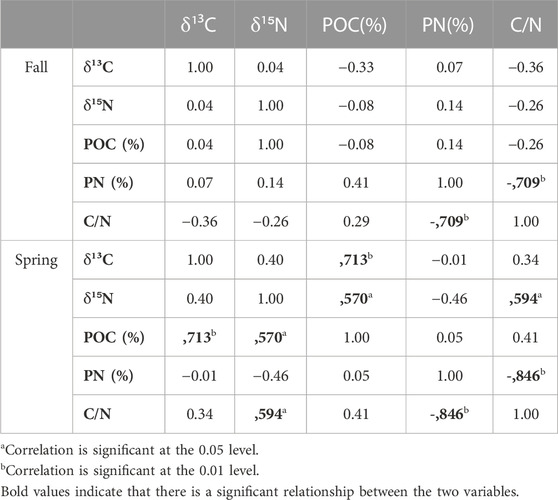
TABLE 3. Pearson correlation between the elemental and isotopic composition of POM during the fall and spring seasons.
3.2 Spatiotemporal variation of potential organic matter sources of particulate organic matter
The MixSIAR mixing model results indicated that POM was supported by terrestrial plant sources, but the proportional contributions of this source differed along the longitudinal fluvial gradient and seasons (Table 4). During the high-rainfall fall season, terrestrial plants contributed most to POM at sites 1, 2, 3, and 5, whereas terrestrial plants and riverine phytoplankton supported a large fraction at sites 4, 6, and 7. POM from site 8 on the coastal zone originated mainly from soil OM to a lesser extent from terrestrial plants and marine phytoplankton. In the spring season, terrestrial plants accounted for more than 65% of all sites along the river. However, riverine phytoplankton contribution somewhat increased to the POM, especially sites located upstream (site 3) of the first dam. The contribution of soil OM to the POM at site 8 decreased while marine phytoplankton increased (Table 4).
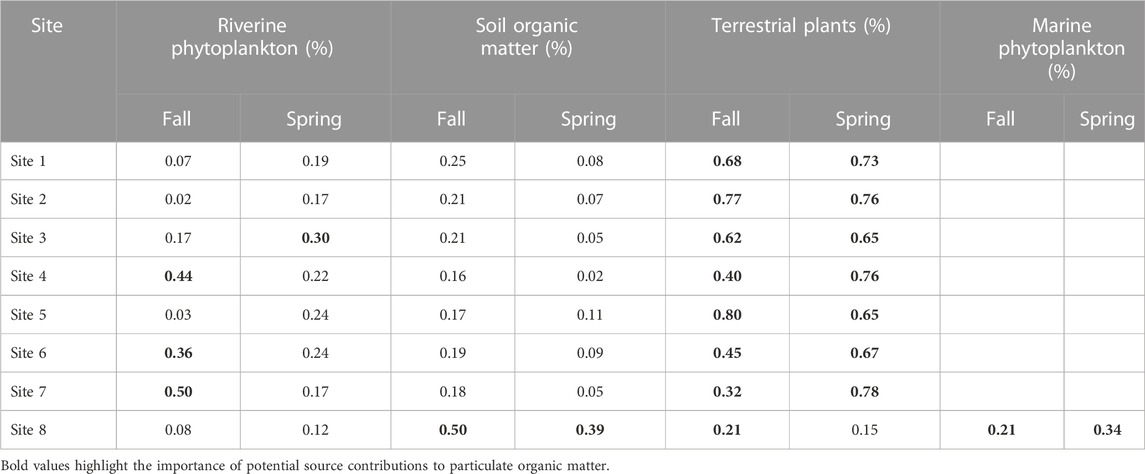
TABLE 4. Mean of the MixSIAR model-estimated proportions of potential source contributions to the particulate organic matter during both seasons.
The plot δ13C vs. C/N and δ15N vs. C/N clearly separated these four end-member sources: terrestrial plants, soil OM, riverine phytoplankton, and bacteria (Figures 4A, B). Some POM samples (sites 2, 3, 5, and 6) that fell between soil OM and terrestrial plants and others (sites 1, 4, 7, and 8) located within the isotopic range of bacteria during the spring season suggested contributing these sources to POM. While some samples (sites 1, 2, 5, and 7) were located close to riverine phytoplankton and bacteria, some POM samples (sites 3, 4, 6, and 8) also exhibited bacterial C/N values during the fall season (Figures 4A, B).
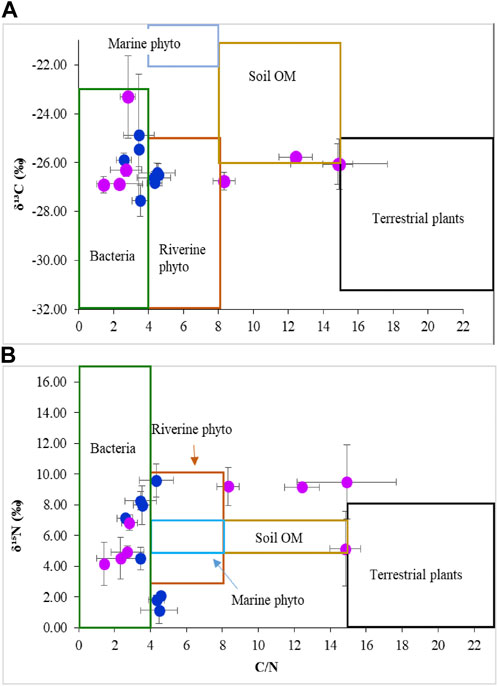
FIGURE 4. Scatter plot of δ13C vs. C/N ratios (A) and δ15N vs C/N ratios (B) for POM samples collected in the Iyidere River during the fall (the blue color) and spring seasons (the purple color). The data in Table 1 were used for the isotope ranges of potential organic matter sources in this figure (phyto: phytoplankton).
4 Discussion
4.1 Seasonal and spatial variation of riverine particulate organic matter
Although there was no clear seasonal trend from upstream to downstream of the river in terms of δ13C and δ15N values of POM (Figure 2), POC (%) and PN (%) ratios increased in the fall (3.58% and 1.11%, respectively) and decreased in the spring season (2.71% and 0.77%, respectively) (Figure 2). Exports of PN and POC (%) have increased, as the intensity of storms and associated soil erosion is the highest (Correll et al., 2001; Wang Y et al., 2020) in this region during fall rainy season. Moreover, the C/N ratio of POM was elevated (mean 7.48) during the spring (less rainy period) and decreased (mean 3.84) in the fall season. The low C/N ratio indicates that soil OM contribution is high due to erosion during heavy rainy periods, whereas a high C/N ratio in low-rainy periods indicates increasing autochthonous OM derived from benthic and pelagic algae (Kaymak et al., 2015). In contrast, many studies showed that the C/N ratios of POM samples from many freshwater systems increased during the wet season and decreased in the dry season (Jha and Masao, 2013; Mbaye et al., 2016; Liu et al., 2021) and indicated that the sources of OM contributing to POM vary with fluvial hydrology.
4.1.1 Fall season
The large amount of precipitation in this region during the fall season increases the average freshwater discharge in the Iyidere River Basin (Dokap, 2013; Altınışık, 2015). In this season, fairly uniform distributions of the δ13C, PN (%), and C/N ratio of POM were observed along the Iyidere River, indicating higher discharge throughout the mainstream channel caused soil erosion by flooding events in this basin. The low C/N ratio of POM (2.59–4.57) from all sites in this season indicated increased mixing of soil bacteria (C/N < 4, Bao et al., 2012; Lv et al., 2022). The δ13C of POM ranged between −26.83‰ and −25.46‰ along the river (except for site 4) which could indicate that allochthonous OM (terrestrial and soil OM) (Chen and Jia, 2009) dominates the POM. The Iyidere River is covered by agricultural areas, especially for tea plant cultivation. Due to the conversion of forest and meadow lands into tea lands, after heavy rain, a large amount of water passes into the surface flow and causes erosion and increasing soil (Yüksek, 2017) as well as POC (%) and PN (%) toward the main channel of the river (Wang H et al., 2020; Gao et al., 2021). This situation may also increase the bacterial density of the river using POC as a source (Caillon and Schelker, 2020).
However, POC (%) and δ15N values of POM showed spatial variations along the river. POC (%) values tend to decrease from the upstream to the downstream of the river, with the highest value at site 1 and lowest at site 6 (Table 1; Figure 2). In the natural forest area, the top 1 cm of the soil contains 4.3% organic carbon because it covers too much terrestrial plant leaf litter; on the contrary, less plant litter in agricultural areas causes less organic carbon, 0.87% (Correll et al., 2001). In this case, we can state that the POM is composed of a mixture of terrestrial plants and soil OM due to increased precipitation and soil erosion along the Iyidere River.
Furthermore, PCA and ANOVA results suggested that the δ15N of POM had high spatial variation during this season (Table 1; Figures 2, 3) with depleted 15N values at sites 1, 2, 3, and 5 and enriched 15N at sites 4, 6, and 7. δ15N can be used as an indicator of anthropogenic pollution such as agricultural synthetic fertilizers and manure (5.9–6.6‰, and 3.5–16.2‰, respectively Bateman and Kelly, 2007), industrial sewage (1.5 ± 1.54‰, Xuan et al., 2020a), and municipal and domestic wastewater (10–20‰, Amir et al., 2019; 7–14‰, Duan et al., 2014; Xiao and Liu, 2010; Liu et al., 2021) sources that have distinct δ15N values. TN concentrated non-point sources such as fertilizer usage, livestock activities, and domestic sewage reaching the Iyidere River Basin were 1484, 213, and 216 kg/km2 per year, respectively, and 20.6, 16, and 34 kg/km2 per year, respectively, for TP concentrations (Erdoğan, 2017). NO2–N concentrations of 1.57–12.83 μg/l (Serdar and Verep, 2018) and NO3–N of 3.45–8.94 mg/l (Mutlu et al., 2017) were observed in the Iyidere River. Previous studies have reported that constant precipitation, together with the hydrogeomorphology of the Iyidere River, collected pollutants throughout the basin into the main channel of the river and transports them to the Black Sea (Dokap, 2013; Environment and urban ministry of Türkiye, 2015; Levent et al., 2018). Overall, in addition to the results from the previous studies, the δ15N of POM that is induced by excessive anthropogenic activities also has the ability to alter the sources of the POM in the Iyidere River.
4.1.2 Spring season
Generally, the Iyidere River Basin experiences low freshwater discharge and precipitation during the early spring sampling month (October) relative to the fall season. The C/N ratios of POM from the upper reach of the river dominated by natural forest were greater than those in the lower reach of the river (Bracken, L. J. and Croke, 2007; Gao et al., 2021). Because of the slope and high discharge across the upper basin, terrestrial allochthonous POM from upland soil causes high C/N and POC (%) (Raymond and Spencer, 2015; McClelland et al., 2016). The proportion of terrestrial contribution gradually decreases with autochthonous POC (Wang et al., 2022) and PN (von Schiller et al., 2016) increasing toward the lower basin of the river. In this study, during the spring season, POC (%) was high in the upper basin (except site 1) and low at the end of the lower basin, but showed significant fluctuations in the middle and head of the lower basin where cascade dams are located. The POC (%) and C/N increased at sites 3 (site above first dam) and 5 (site between dams) above each dam and then decreased from the lower basin toward the Black Sea coast. This indicated that dams may trap the transport of terrestrial OM along the stream and deposit it in the reservoir, thus increasing the contribution of terrestrial OM to POM from these sites. However, the low POC (%) and C/N of POM at sites 4 (site between dam), 6, and 7 (sites below dam) below the dams can be associated with soil OM derived from agricultural land and urbanization (Correll et al., 2001). Cascade dams have altered the POM composition in the Iyidere River and may change the POM export to the coastal system as in other dammed rivers (Liu et al., 2019b; Fang et al., 2019; Xu et al., 2020; Zhao et al., 2021; Wang et al., 2022). Furthermore, high microbial activity by N2-fixating cyanobacteria results in low δ15N and δ13C (Lesutienė et al., 2014). The low δ15N values (4.15–4.92) with a low C/N ratio (1.73–2.47) of POM at sites below dams may be mediated by bacteria, which may also be associated with sewage wastewater (C/N: 2.7, Ogrinc et al., 2008).
The δ13C of POM was similar to that of soil OM and terrestrial plants (Figure 2) (–26.4 to –22‰ (Chen and Jia, 2009) and −31 to −25‰ (Lamb et al., 2006; Kaymak et al., 2015, respectively)), showing that POM mainly comes from mixing of eroded soil and terrestrial plants along the Iyidere River during this season. According to the PCA analysis, sites 2, 3, 5, and 6 differed from other sites with their very high C/N values (8.32–14.92). This was likely to indicate the POM contains a higher fraction of soil OM [C/N = 8–15, (Kendall et al., 2001)], while higher δ15N values (9.16–9.49) at the same sites may imply strong anthropogenic pollution derived from different sources. Unlike the fall season, sites 3, 5, and 6 with high δ15N were located above and between the dams. As dams create barriers along river flow, the accumulation of anthropogenic inputs may vary spatially between cascade dams, causing different isotopic and elemental ratios of POM between sites depending on the season. We also found a significant (p < 0.01) negative correlation between C/N and PN and a positive correlation between C/N and δ15N (Table 2; Figure 3), indicating the disturbance from anthropogenic nitrogen inputs to the middle and lower basin of the Iyidere River and changes the composition of POM. Similar δ15N values (>8‰) were also reported for the eutrophication of rivers with high nutrient and municipal wastewater loads that enter the Baltic Sea (Lesutienė et al., 2014; Remeikaitė-Nikienė et al., 2017; Amir et al., 2019).
4.2 Source composition of riverine particulate organic matter
The source of POM in the river was determined by comparing C and N isotopes and C/N ratios for four different OM sources (Figure 4). The C/N ratio is largely used as a proxy to explain the source and fate of OM in the aquatic environment because four OM sources in stream ecosystem have a unique ratio (Table 1). The δ13C versus C/N plots made a good distinction between the sources of OM (end-member) contributing to POM and revealed that bacterial OM contributed to the POM, especially along the Iyidere River in the fall season. OM sources contributing to POM did not vary spatially during the fall season. Plots of δ15N versus C/N showed that the fall season samples of POM along the Iyidere River reflected enriched and depleted 15N bacterial OM. It may be suggested that different OM inputs (anthropogenic sources such as fertilizer, wastewater, and sewage) originating from anthropogenic activities defined throughout the basin be used as substrates by bacteria in different sites. POM from human-influenced watersheds was more labile and supported higher microbial activity when compared to POM from natural forested basins (Pagano et al., 2014). Interestingly, the MixSIAR results indicated that the contribution of riverine phytoplankton to the POM samples from sites 4, 6, and 7 increased in the fall season. The δ13C and δ15N ratios of the bacteria coincide with the riverine phytoplankton and, therefore, were not included as an end-member in the mixing model. Actually, riverine phytoplankton could be an indicator of bacteria. The stable isotope values of potential sources can affect the resolution of the mixing model results (Liu et al., 2021), so it is necessary to have a different number of sources sampling in future studies to reduce the uncertainty of the model output.
The δ13C vs C/N plots showed the OM sources contributing to POM had spatial variation, with terrestrial plant and soil OM in some sites, but bacteria in some sites during the spring season. These results were consistent with those from the MixSIAR analysis. The contribution of terrestrial plants to the POM along the İyidere River was found to be quite high; in addition, riverine phytoplankton contributed to the POM in some sites. High rainfall transported plant litter to streams throughout the basin during fall season. This leaf debris accumulated at riverbank since the winter seasons (low-rainy season) and then may become important for POM when rainfall decreases in the spring season. Furthermore, the contribution of riverine phytoplankton increased in the spring season at the upstream sites above the dam (site 3). In fact, this is to be expected because these sites are affected by reservoirs, lower water velocity, greater light penetration, and water residence time likely promoted phytoplankton growth (Chen and Jia, 2009). Interestingly, the contribution of riverine phytoplankton to the POM at sites 4, 6, and 7 below the dams increased in the rainy fall season, but decreased in the spring season. While the opposite situation was observed in other streams (Vivien et al., 2010; Kaymak et al., 2015), Liu et al. (2021)’s results were found to be consistent with our results. Overall, anthropogenic activities and cascade dams during the spring season were the dominant factors influencing the sources of POM along the Iyidere River.
4.3 Seasonal and spatial variation of coastal particulate organic matter
High slope and river discharges have made this basin attractive for dams; there are three dams across the basin and these dams are reported to hold 83% of the suspended sediment carried along the river (Berkun, 2010; Berkun et al., 2015). Therefore, the annual sediment transport of the Iyidere catchment is 58,632 tons/year and consists of mostly sand, clay, and silt (Ayaz et al., 2013). In addition, it has been reported that the amount of large particles reaching the sea has decreased significantly due to the fact that the sediments are completely retained in the reservoirs (Berkun, 2010). Here, we demonstrated that both δ15N and δ13C of POM showed different values during the fall and spring seasons at coastal site 8, with a relatively enriched 13C and depleted 15N in the latter (Table 1; Figures 2, 3). The POC (%), δ13C, and δ15N values in the fall season have been attributed to contributions from both soil OM and terrestrial plants to the coastal POM pool. This was also evidenced by MixSIAR analysis results, which showed a high contribution of soil OM (50%) and low terrestrial plants (21%) and marine phytoplankton (21%) to the coastal POM. The elemental and isotopic compositions of POM in the coastal site 8 indicate a high contribution of soil OM from severe soil erosion along the river that is induced by excessive anthropogenic activities such as agricultural and mining activities.
When the stream flow and consequently its input decreased during the spring season, the marine phytoplankton contribution increased and reached 34% of coastal POM content according to the MixSIAR results. This result was also confirmed by the increasing δ13C and δ15N of POM from coastal site 8 because δ13C and δ15N values, average −20.5 ± 2.8‰ and 6 ± 4.2‰, respectively, were representative of marine phytoplankton. (Lamb et al., 2006; Gao et al., 2012). However, the C/N of coastal POM (2.83 ± 0.43) was considerably lower than that of marine plankton (6–8, Ho et al., 2021). When the freshwater influence was weak in this season, terrestrial nutrient input decreased (39% for soil and 15% for terrestrial plants); therefore, high δ15N value of POM with low C/N ratio of this site could indicate the development of microbial food chains (Ho et al., 2021). The C/N ratio of a small size fraction of POM (0.7–50 μm) may decrease with the increase of phagotrophic protists because protists have a lower C/N ratio than phytoplankton (5.9–12 for dinoflagellates and 5 to 5.5 for ciliates; Meunier et al., 2012; Golz et al., 2015). These results are quite plausible considering that dams trap large sizes of POM and leave small ones. Another explanation for this could be the presence of bacteria in POM samples from coastal zone 8. A similar situation, namely, the contribution of bacteria to the POM, was also found at sites (6 and 7) close to the river estuary. Hereby, anthropogenic activities (urbanization, agriculture activities, etc.) changed the quality of POM delivered to the Black Sea coast by aggravating soil erosion, increasing the presence of different types of phytoplankton and bacteria (Surbeck et al., 2010; Xuan et al., 2020b; Lv et al., 2022).
A high positive correlation was obtained between POC (%) and δ15N and δ13C, when POM parameters were examined by combining all sites. This high correlation likely indicated that the contribution of soil OM resulting from anthropogenic activities was higher compared to terrestrial OM to the POM from the upstream of the Iyidere River to the Black Sea coast. Similarly, several studies (Barros et al., 2010; Ke et al., 2020; Zhang et al., 2022) have reported that anthropogenically affected river inputs can alter the POM content of estuarine or coastal areas.
5 Conclusion
In this study, we investigated stable δ13C and δ15N isotopes and POC (%), PON (%), and C/N ratios of POM in suspended particulate matter from the Iyidere River mainstream in Northeast Anatolia of Türkiye and the coastal zone of the Black Sea during two seasons. In the spring, OM sources contributing to POM differed significantly between sites, with terrestrial vegetation, soil OM, and bacteria being the main contributors of POM depending on the site, due to urbanization, agricultural activities, and cascade dams. The composition of POM exhibited a uniform distribution among sites during the fall season. Urbanization and agricultural activities might have a greater impact on the change in POM composition along the river than with cascading dams in this season. Interestingly, the δ15N of POM significantly varied along the Iyidere River during the fall and spring seasons, suggesting that different sources or intensities of anthropogenic nutrient loading could enrich or deplete 15N in POM. Existing anthropogenic activities, such as urbanization, industrial and agricultural activities, and dam construction, changed the compositions of OM of the Iyidere River, which in turn might affect the regional carbon cycle and coastal ecosystems of the Black Sea. The combined application of elemental and isotopic analytical techniques in this study has revealed changes in the composition and transport of the POM from the Iyidere River mainstream to the coastal zone of the Black Sea under anthropogenic disturbances. Future studies should also consider climate changes and sample all potential OM sources in the stream ecosystem.
Data availability statement
The raw data supporting the conclusions of this article will be made available by the authors, without undue reservation.
Author contributions
NK performed data analysis and wrote the manuscript. TM designed the study and performed the sample collection and analysis. BV designed and supervised the study and manuscript. All authors read and approved the final manuscript.
Funding
This study was supported by the Recep Tayyip Erdogan University Research Fund (RTEUR Project Number FBA-2019-1034). However, this research fund only provided the payments needed for field studies and isotope analysis. Neither our university/institution nor this research fund paid/will pay any fee for open-access publication.
Conflict of interest
The authors declare that the research was conducted in the absence of any commercial or financial relationships that could be construed as a potential conflict of interest.
Publisher’s note
All claims expressed in this article are solely those of the authors and do not necessarily represent those of their affiliated organizations, or those of the publisher, the editors, and the reviewers. Any product that may be evaluated in this article, or claim that may be made by its manufacturer, is not guaranteed or endorsed by the publisher.
References
Akbal, F., Gürel, L., Bahadır, T., Güler, I., Bakan, G., and Büyükgüngör, H. (2011). Water and sediment quality assessment in the mid-Black Sea coast of Turkey using multivariate statistical techniques. Environ. Earth Sci. 64, 1387–1395. doi:10.1007/s12665-011-0963-6
Akçay, İ., and Yücel, M. (2023). Distinct patterns of sedimentary phosphorus fractionation and mobilization in the seafloor of the Black Sea, marmara sea, and the mediterranean Sea. Sci. Total Environ. 863, 160936. doi:10.1016/j.scitotenv.2022.160936
Algan, O. (2006). Riverine fluxes into the black and marmara seas. In fluxes of small and medium-size mediterranean rivers: Impact on coastal areas. CIESM Workshop Monogr. 30, 47–53.
Altınışık, U. (2015). Doğu karadeniz havzası akımlarının incelenmesi. [dissertation/Phd thesis]. Istanbul: Istanbul Teknik University.
Amir, M., Paul, D., and Samal, R. N. (2019). Sources of organic matter in Chilika lagoon, India inferred from stable C and N isotopic compositions of particulates and sediments. J. Mar. Syst. 194, 81–90. doi:10.1016/j.jmarsys.2019.03.001
Amon, R. M., Budéus, G., and Meon, B. (2003). Dissolved organic carbon distribution and origin in the nordic seas: Exchanges with the arctic ocean and the north atlantic. J. Geophys. Res. Oceans. 108, 1594. doi:10.1029/2002JC001594
Ayaz, S., Erdoğan, N., Beşiktaş, M., Aytış, E. A., Dereli, E. M., Aynur, S., et al. (2013). Havza koruma eylem planlarinin hazirlanmasi projesi doğu karadeniz havzasi nihai raporu. Sci. Technol. Res. Institution Turk. Marmara Res. Cent. Available at: https://www.tarimorman.gov.tr/SYGM/Belgeler/havza%20koruma%20eylem%20planlar%C4%B1/Dogu_Karadeniz_web.pdf (Accessed December 12, 2022).
Bănaru, D., Harmelin-Vivien, M., Gomoiu, M. T., and Onciu, T. M. (2007). Influence of the Danube River inputs on C and N stable isotope ratios of the Romanian coastal waters and sediment (Black Sea). Mar. Pollut. Bull. 54 (9), 1385–1394. doi:10.1016/j.marpolbul.2007.05.022
Bao, H., Wu, Y., Zhang, J., Deng, B., and He, Q. (2012). Composition and flux of suspended organic matter in the middle and lower reaches of the changjiang (yangtze river) - impact of the three gorges dam and the role of tributaries and channel erosion: Organic matter along middle and lower changjiang. Hydrol. 28 (3), 1137–1147. doi:10.1002/hyp.9651
Barros, G. V., Martinelli, L. A., Novais, T. M. O., Ometto, J. P. H., and Zuppi, G. M. (2010). Stable isotopes of bulk organic matter to trace carbon and nitrogen dynamics in an estuarine ecosystem in Babitonga Bay (Santa Catarina, Brazil). Sci. Total Environ. 408 (10), 2226–2232. doi:10.1016/j.scitotenv.2010.01.060
Bateman, A. S., and Kelly, S. D. (2007). Fertilizer nitrogen isotope signatures. Isot. Environ. Health Stud. 43 (3), 237–247. doi:10.1080/10256010701550732
Bayram, A., Önsoy, H., Akinci, G., and Bulut, V. N. (2011). Variation of total organic carbon content along the stream harsit, eastern Black Sea basin, Turkey. Environ. Monit. Assess. 182, 85–95. doi:10.1007/s10661-010-1860-2
Berkun, M., Aras, E., and Akdemir, U. O. (2015). Water runoff, sediment transport and related impacts in the Southeastern Black Sea Rivers. Environ. Eng. Manag. J. 14, 781–791. doi:10.30638/eemj.2015.087
Berkun, M. (2010). Environmental evaluation of Turkey’s transboundary rivers' hydropower systems. Can. J. Civ. Eng. 37, 684–694. doi:10.1139/l10-003
Blair, N. E., and Aller, R. C. (2012). The fate of terrestrial organic carbon in the marine environment. Ann. Rev. Mar. Sci. 4, 401–423. doi:10.1146/annurev-marine-120709-142717
Bouchez, J., Galy, V., Hilton, R. G., Gaillardet, J., Moreira-Turcq, P., Pérez, M. A., et al. (2014). Source, transport and fluxes of Amazon River particulate organic carbon: Insights from river sediment depth-profiles. GCA 133, 280–298. doi:10.1016/j.gca.2014.02.032
Bracken, L. J., and Croke, J. (2007). The concept of hydrological connectivity and its contribution to understanding runoff-dominated geomorphic systems. Hydrol. 21, 1749–1763. doi:10.1002/hyp.6313
Butman, D. E., Wilson, H. F., Barnes, R. T., Xenopoulos, M. A., and Raymond, P. A. (2015). Increased mobilization of aged carbon to rivers by human disturbance. Nat. Geosci. 8, 112–116. doi:10.1038/ngeo2322
Caddy, J. F. (2000). Marine catchment basin effects versus impacts of fisheries on semi-enclosed seas. ICES Mar. Sci. Symp. 57, 628–640. doi:10.1006/jmsc.2000.0739
Caillon, F., and Schelker, J. (2020). Dynamic transfer of soil bacteria and dissolved organic carbon into small streams during hydrological events. Aquat. Sci. 82, 41–11. doi:10.1007/s00027-020-0714-4
Chen, F., and Jia, G. (2009). Spatial and seasonal variations in δ13C AND δ15N of particulate organic matter in a dam-controlled subtropical river. River Res. Appl. 25 (9), 1169–1176. doi:10.1002/rra.1225
Coban-Yildiz, Y., Tugrul, S., Ediger, D., Yilmaz, A., and Polat, C. (2000). A comparative study on the abundance and elemental composition of POM in three interconnected basins: The black, the marmara and the mediterranean seas. Mediterr. Mar. Sci. 1, 51–64. doi:10.12681/mms.5
Çoban-Yıldız, Y., Altabet, M. A., Yılmaz, A., and Tuğrul, S. (2006). Carbon and nitrogen isotopic ratios of suspended particulate organic matter (SPOM) in the Black Sea water column. Deep Sea Res. Part II Top. Stud. Oceanogr. 53, 1875–1892. doi:10.1016/j.dsr2.2006.03.021
Correll, D. L., Jordan, T. E., and Weller, D. E. (2001). Effects of precipitation, air temperature, and land use on organic carbon discharges from Rhode River watersheds. Water, Air, Soil Pollut. 128, 139–159. doi:10.1023/a:1010337623092
Cui, J., Kong, X., Chen, J., Sun, J., and Zhu, Y. (2021). Spatially explicit evaluation and driving factor identification of land use conflict in Yangtze River economic belt. Land 10 (1), 43. doi:10.3390/land10010043
Dokap, T. C. (2013). Kalkinma bakanliği bölge kalkinma idaresi başkanliği. Turkiye: Rize Il Raporu. Available at: https://www.rtb.org.tr/uploads/files/113-DOKAPRizeilRaporu.pdf (Accessed January 15, 2023).
Duan, S., Amon, R. M., and Brinkmeyer, R. L. (2014). Tracing sources of organic matter in adjacent urban streams having different degrees of channel modification. Sci. Total Environ. 485, 252–262. doi:10.1016/j.scitotenv.2014.03.066
Environment and urban ministry of Turkey (2015). Atıksu artımı eylem planı 2015-2023. Turkey: Environment and urban ministry of Turkey. Available at: https://webdosya.csb.gov.tr/db/destek/icerikler/aat_eylem_plan–20191127122144.pdf (Accessed November 01, 2022).
Erdoğan, N. (2017). Doğu Karadeniz Havzası’nda kirlilik yüklerinin değerlendirilmesi. [dissertation/Phd thesis]. Sakarya: Sakarya University.
Ergül, H. A., and Topcuoğlu, S. (2009). Seasonal changes in settling the particulate matter on the eastern Turkish coast of the Black Sea. Fresenius Environ. Bull. 18, 1312–1318.
Fang, Y., Chen, Y., Hu, L., Tian, C., Luo, Y., Li, J., et al. (2019). Large-river dominated black carbon flux and budget: A case study of the estuarine-inner shelf of east China sea, China. Sci. Total Environ. 651, 2489–2496. doi:10.1016/j.scitotenv.2018.10.156
Finlay, J. C., and ve Kendall, C. (2007). “Stable isotope tracing of organic matter sources and food web interactions in watersheds,” in Stable isotopes in ecology and environmental science. Editors K. Lajtha, and R. Michener (Blackwell, Oxford, U.K: Ecological Methods and Concepts), 283–333.
Gao, X., Yang, Y., and Wang, C. (2012). Geochemistry of organic carbon and nitrogen in surface sediments of coastal Bohai Bay inferred from their ratios and stable isotopic signatures. Mar. Pollut. Bull. 64, 1148–1155. doi:10.1016/j.marpolbul.2012.03.028
Gao, C., Li, W., Cui, L., Ma, Q., and Cai, J. (2021). Catchment landscape components alter relationships between discharge and stream water nutrient ratios in the Xitiao River Basin China. Sci. Rep. 11 (1), 1–15. doi:10.1038/s41598-021-89804-1
Golz, A. L., Burian, A., and Winder, M. (2015). Stoichiometric regulation in micro-and mesozooplankton. J. Plankton Res. 37 (2), 293–305. doi:10.1093/plankt/fbu109
Ho, P. C., Okuda, N., Yeh, C. F., Wang, P. L., Gong, G. C., and Hsieh, C. H. (2021). Carbon and nitrogen isoscape of particulate organic matter in the East China Sea. Prog. Oceanogr. 197, 102667. doi:10.1016/j.pocean.2021.102667
Holmes, R. M., McClelland, J. W., Raymond, P. A., Frazer, B. B., Peterson, B. J., and Stieglitz, M. (2008). Lability of DOC transported by alaskan rivers to the arctic ocean. Geophys. Res. Lett. 35, 837. doi:10.1029/2007GL032837
Ittekkot, V., and Arain, R. (1986). Nature of particulate organic matter in the river Indus, Pakistan. GCA. 50 (8), 1643–1653. doi:10.1016/0016-7037(86)90127-4
Jha, P. K., and Masao, M. (2013). Factors affecting nutrient concentration and stable carbon and nitrogen isotope ratio of particulate organic matter in the Ishikari River System, Japan. Wat. Air. Soil. Poll. 224, 1–23. doi:10.1007/s11270-013-1551-z
Kao, S. J., and Liu, K. K. (1996). Particulate organic carbon export from a subtropical mountainous river (Lanyang Hsi) in Taiwan. Limnol. Oceanogr. 41 (8), 1749–1757. doi:10.4319/lo.1996.41.8.1749
Kaymak, N., Winemiller, K. O., Akin, S., Altuner, Z., Polat, F., and Dal, T. (2015). Stable isotope analysis reveals relative influences of seasonal hydrologic variation and impoundment on assimilation of primary production sources by fish in the Upper Yesilırmak River, Turkey. Hydrobiologia. 753, 131–147. doi:10.1007/s10750-015-2201-9
Ke, Z., Chen, D., Liu, J., and Tan, Y. (2020). The effects of anthropogenic nutrient inputs on stable carbon and nitrogen isotopes in suspended particulate organic matter in Jiaozhou Bay, China. Cont. Shelf Res. 208, 104244. doi:10.1016/j.csr.2020.104244
Kendall, C., Silva, S. R., and Kelly, V. J. (2001). Carbon and nitrogen isotopic compositions of particulate organic matter in four large river systems across the United States. Hydrol. Process. 15, 1301–1346. doi:10.1002/hyp.216
Lamb, A. L., Wilson, G. P., and Leng, M. J. (2006). A review of coastal palaeoclimate and relative sea-level reconstructions using δ13C and C/N ratios in organic material. Earth Sci. Rev. 75 (1–4), 29–57. doi:10.1016/j.earscirev.2005.10.003
Lammers, J. M., Reichart, G. J., and Middelburg, J. J. (2017). Seasonal variability in phytoplankton stable carbon isotope ratios and bacterial carbon sources in a shallow Dutch lake. Limnol. Oceanogr. 62, 2773–2787. doi:10.1002/lno.10605
Lee, B. J., Kim, J., Hur, J., Choi, I. H., Toorman, E. A., Fettweis, M., et al. (2019). Seasonal dynamics of organic matter composition and its effects on suspended sediment flocculation in river water. Water Resour. Res. 55 (8), 6968–6985. doi:10.1029/2018wr024486
Leng, M. J., and Lewis, J. P. (2017). “C/N ratios and carbon isotope composition of organic matter in estuarine environments,” in Applications of paleoenvironmental techniques in estuarine studies. Developments in paleoenvironmental research. Editors K. Weckström, K. Saunders, P. Gell, and C. Skilbeck (Dordrecht: Springer), 20. doi:10.1007/978-94-024-0990-1_9
Lesutienė, J., Bukaveckas, P. A., Gasiūnaitė, Z. R., Pilkaitytė, R., and Razinkovas-Baziukas, A. (2014). Tracing the isotopic signal of a cyanobacteria bloom through the food web of a Baltic Sea coastal lagoon. Estuar. Coast. Shelf Sci. 138, 47–56. doi:10.1016/j.ecss.2013.12.017
Levent, B. A. T., Öztekin, A., Şahin, F., Arici, E., and Özsandikçi, U. (2018). An overview of the Black Sea pollution in Turkey. MedFAR. 1 (2), 66–86. Available at: https://dergipark.org.tr/en/pub/medfar/issue/37150/397536.
Liu, Y., Deng, B., Du, J., Zhang, G., and Hou, L. (2019a). Nutrient burial and environmental changes in the Yangtze Delta in response to recent river basin human activities. Environ. Pollut. 249, 225–235. doi:10.1016/j.envpol.2019.03.030
Liu, Y., Wang, X., Wen, Q., and Zhu, N. (2019b). Identifying sources and variations of organic matter in an urban river in Beijing, China using stable isotope analysis. Ecol. Indic. 102, 783–790. doi:10.1016/j.ecolind.2019.03.023
Liu, J., Yan, T., and Shen, Z. (2021). Sources, transformations of suspended particulate organic matter and their linkage with landscape patterns in the urbanized Beiyun River Watershed of Beijing, China. Sci. Total Environ. 791, 148309. doi:10.1016/j.scitotenv.2021.148309
Liu, C., Wu, Z., He, C., Zhang, Y., Huang, W., and Wang, D. (2022). Source identification and chemical compositions of particulate and mineral-associated organic matter in the deposited sediments of a dam-controlled watershed. Catena 219, 106618. doi:10.1016/j.catena.2022.106618
Lobbes, J. M., Fitznar, H. P., and Kattner, G. (2000). Biogeochemical characteristics of dissolved and particulate organic matter in Russian rivers entering the Arctic Ocean. GCA 64, 2973–2983. doi:10.1016/S0016-7037(00)00409-9
Lu, L., Cheng, H., Pu, X., Wang, J., Cheng, Q., and Liu, X. (2016). Identifying organic matter sources using isotopic ratios in a watershed impacted by intensive agricultural activities in Northeast China. Agric. Ecosyst. Environ. 222, 48–59. doi:10.1016/j.agee.2015.12.033
Lv, Y., Ren, Y., and Li, X. (2022). Influence of reservoir management on the source and transport of particulate organic carbon in surface waters of the lower Yellow River. J. Soils Sediments 22, 2548–2556. doi:10.1007/s11368-022-03268-y
Mbaye, M. L., Gaye, A. T., Spitzy, A., Dähnke, K., Afouda, A., and Gaye, B. (2016). Seasonal and spatial variation in suspended matter, organic carbon, nitrogen, and nutrient concentrations of the Senegal River in West Africa. Limnologica. 57, 1–13. doi:10.1016/j.limno.2015.12.003
McCallister, S. L., Bauer, J. E., Cherrier, J. E., and Ducklow, H. W. (2004). Assessing sources and ages of organic matter supporting river and estuarine bacterial production: A multiple-isotope (Δ14C, δ13C, and δ15N) approach. Limnol. Oceanogr. 49 (5), 1687–1702. doi:10.4319/lo.2004.49.5.1687
McClelland, J. W., Holmes, R. M., Peterson, B. J., Raymond, P. A., Striegl, R. G., Zhulidov, A. V., et al. (2016). Particulate organic carbon and nitrogen export from major Arctic rivers. Glob. Biogeochem. Cycles. 30 (5), 629–643. doi:10.1002/2015gb005351
McKinney, R. A., Nelson, W. G., Charpentier, M. A., and Wigand, C. (2001). Ribbed mussel nitrogen isotope signatures reflect nitrogen sources in coastal salt marshes. Ecol. Appl. 11 (1), 203–214. doi:10.1890/1051-0761(2001)011[0203:rmnisr]2.0.co;2
Meunier, C. L., Haafke, J., Oppermann, B., Boersma, M., and Malzahn, A. M. (2012). Dynamic stoichiometric response to food quality fluctuations in the heterotrophic dinoflagellate Oxyrrhis marina. Mar. Biol. 159, 2241–2248. doi:10.1007/s00227-012-2009-3
Moore, T. R., and Jackson, R. J. (1989). Dynamics of dissolved organic carbon in forested and disturbed catchments, westland, New Zealand: 2. Larry river. Water Resour. Res. 25 (6), 1331–1339.
Mutlu, T., Verep, B., Onay, D., and Kırlıoğlu, K. (2017). Güneydoğu karadeniz rize kıyılarına dökülen bazı akarsuların fiziko-kimyasal su kalitesi. J. Anatol. Environ. Anim. Sci. 2, 67–71. doi:10.35229/jaes.349879
Mutlu, T. (2019). Doğu Karadenı̇z Havzasında Bazı Akarsuların Eser Element Düzeylerı̇ Ve Karadenı̇z’e Gı̇rdı̇lerı̇nı̇n Duraylı Karbon Ve Azot İzotop Oranlarıyla Belı̇rlenmesı̇. [dissertation/Phd thesis]. Rize: Recep Tayyip Erdoğan University.
Ni, H. G., Lu, F. H., Luo, X. L., Tian, H. Y., and Zeng, E. Y. (2008). Riverine inputs of total organic carbon and suspended particulate matter from the Pearl River Delta to the coastal ocean off South China. Mar. Pollut. Bull. 56 (6), 1150–1157. doi:10.1016/j.marpolbul.2008.02.030
Ogrinc, N., Markovics, R., Kanduč, T., Walter, L. M., and Hamilton, S. K. (2008). Sources and transport of carbon and nitrogen in the River Sava watershed, a major tributary of the River Danube. Geochemistry 23, 3685–3698. doi:10.1016/j.apgeochem.2008.09.003
Öztürk, M. Z., Çetinkaya, G., and Aydin, S. (2017). Köppen-Geiger iklim sınıflandırmasına göre Türkiye’nin iklim tipleri. Coğrafya Derg. 35, 17–27. doi:10.26650/jgeog295515
Pagano, T., Bida, M., and Kenny, J. E. (2014). Trends in levels of allochthonous dissolved organic carbon in natural water: A review of potential mechanisms under a changing climate. Water 6, 2862–2897. doi:10.3390/w6102862
Panwar, S., and Yang, S. (2022). Influence of Three Gorges Dam and drought on particulate organic carbon flux and its source in the lower Yangtze River. Biogeochemistry 158, 269–284. doi:10.1007/s10533-022-00889-w
Park, J. H., Nayna, O. K., Begum, M. S., Chea, E., Hartmann, J., Keil, R. G., et al. (2018). Reviews and syntheses: Anthropogenic perturbations to carbon fluxes in Asian river systems–concepts, emerging trends, and research challenges. Biogeosciences 15, 3049–3069. doi:10.5194/bg-15-3049-2018
Raymond, P. A., and Bauer, J. E. (2001). Riverine export of aged terrestrial organic matter to the North Atlantic Ocean. Nature 409, 497–500. doi:10.1038/35054034
Raymond, P. A., and Spencer, R. G. (2015). “Riverine DOM,” in Biogeochemistry of marine dissolved organic matter (Academic Press), 509–533.
Remeikaitė-Nikienė, N., Lujanienė, G., Malejevas, V., Barisevičiūtė, R., Zilius, M., Vybernaitė-Lubienė, I., et al. (2017). Assessing nature and dynamics of POM in transitional environment (the Curonian Lagoon, SE Baltic Sea) using a stable isotope approach. Ecol. Indic. 82, 217–226. doi:10.1016/j.ecolind.2017.06.035
Serdar, O., and Verep, B. (2018). The investigation of water quality of İyidere and Çiftekavak Streams using physico-chemical and biotic indexes. Int. J. pure Appl. Sci. 4 (1), 61–71. doi:10.29132/ijpas.398725
Stock, B. C., Jackson, A. L., Ward, E. J., Parnell, A. C., Phillips, D. L., and Semmens, B. X. (2018). Analyzing mixing systems using a new generation of bayesian tracer mixing models. PeerJ 6, e5096. doi:10.7717/peerj.5096
Surbeck, C. Q., Jiang, S. C., and Grant, S. B. (2010). Ecological control of fecal indicator bacteria in an urban stream. Environ. Sci. Technol. 44 (2), 631–637. doi:10.1021/es903496m
Verep, B., Mutlu, C., Apaydin, G., and Cevik, U. (2012). The trace element analysis in freshwater fish species, water and sediment in Iyidere stream (Rize-Turkey). PJBS. 15 (14), 658–665. doi:10.3923/pjbs.2012.658.665
Vivien, M. H., Dierking, J., Banaru, D., Fontaine, M. F., and Arlhac, D. (2010). Seasonal variation in stable C and N isotope ratios of the rhone river inputs to the mediterranean sea (2004–2005). Biogeochemistry 100, 139–150. doi:10.1007/s10533-010-9411-z
von Schiller, D., Aristi, I., Ponsatí, L., Arroita, M., Acuña, V., Elosegi, A., et al. (2016). Regulation causes nitrogen cycling discontinuities in Mediterranean rivers. Sci. Total Environ. 540, 168–177. doi:10.1016/j.scitotenv.2015.07.017
Wang, X., Ma, H., Li, R., Song, Z., and Wu, J. (2012). Seasonal fluxes and source variation of organic carbon transported by two major Chinese Rivers: The Yellow River and Changjiang (Yangtze) River. Glob. Biogeochem. Cycles. 26. doi:10.1029/2011gb0041302
Wang, H., Ran, X., Bouwman, A. F., Wang, J., Xu, B., Song, Z., et al. (2022). Damming alters the particulate organic carbon sources, burial, export and estuarine biogeochemistry of rivers. J. Hydrol. 607, 127525. doi:10.1016/j.jhydrol.2022.127525
Wang H, H., Li, Z., Zhuang, W. E., Hur, J., Yang, L., and Wang, Y. (2020). Spectral and isotopic characteristics of particulate organic matter in a subtropical estuary under the influences of human disturbance. J. Mar. Syst. 203, 103264. doi:10.1016/j.jmarsys.2019.103264
Wang Y, Y., Peng, J., Cao, X., Xu, Y., Yu, H., Duan, G., et al. (2020). Isotopic and chemical evidence for nitrate sources and transformation processes in a plateau lake basin in Southwest China. Sci. Total Environ. 711, 134856. doi:10.1016/j.scitotenv.2019.134856
Wu, Y., Zhang, J., Liu, S. M., Zhang, Z. F., Yao, Q. Z., Hong, G. H., et al. (2007). Sources and distribution of carbon within the Yangtze River system. Estuar. Coast. Shelf Sci. 71, 13–25. doi:10.1016/j.ecss.2006.08.016
Xiao, H. Y., and Liu, C. Q. (2010). Identifying organic matter provenance in sediments using isotopic ratios in an urban river. Geochem. J. 44 (3), 181–187. doi:10.2343/geochemj.1.0059
Xu, Z., Wan, S., Colin, C., Li, T., Clift, P. D., Chang, F., et al. (2020). Enhanced terrigenous organic matter input and productivity on the Western margin of the Western Pacific Warm Pool during the Quaternary sea-level lowstands: Forcing mechanisms and implications for the global carbon cycle. Quat. Sci. Rev. 232, 106211. doi:10.1016/j.quascirev.2020.106211
Xu, S., Yue, F. J., Li, S. L., Ding, H., Xu, S., Lang, Y. C., et al. (2021). Carbon and nitrogen isotope constraints on source and variation of particulate organic matter in high-latitude agricultural rivers, Northeast China. J. Clean. Prod. 321, 128974. doi:10.1016/j.jclepro.2021.128974
Xuan, Y., Tang, C., Liu, G., and Cao, Y. (2020a). Carbon and nitrogen isotopic records of effects of urbanization and hydrology on particulate and sedimentary organic matter in the highly urbanized Pearl River Delta, China. J. Hydrol. 591, 125565. doi:10.1016/j.jhydrol.2020.125565
Xuan, Y., Tang, C., and Cao, Y. (2020b). Mechanisms of nitrate accumulation in highly urbanized rivers: Evidence from multi-isotopes in the pearl river delta, China. J. Hydrol. X. 587, 124924. doi:10.1016/j.jhydrol.2020.124924
Yi, Q., Chen, Q., Hu, L., and Shi, W. (2017). Tracking nitrogen sources, transformation, and transport at a basin scale with complex plain river networks. Environ. Sci. Technol. 51 (10), 5396–5403. doi:10.1021/acs.est.6b06278
Yüksek, T. (2017). Land use, some forestry studies and a general evaluation of the temporal distribution of precipitation in rize. J. Anatol. Environ. Anim. Sci. 2 (3), 59–66. doi:10.35229/jaes.359157
Zhang, Y., Meng, X., Bai, Y., Wang, X., Xia, P., Yang, G., et al. (2021). Sources and features of particulate organic matter in tropical small mountainous rivers (SW China) under the effects of anthropogenic activities. Ecol. Indic. 125, 107471. doi:10.1016/j.ecolind.2021.107471
Zhang, S., Yager, P. L., Liang, C., Shen, Z., and Xian, W. (2022). Distribution and spatial-temporal variation of organic matter along the Yangtze River-ocean continuum. Elem. Sci. Anthropocene 10, 00034. doi:10.1525/elementa.2021.00034
Keywords: particulate organic matter, stable isotope, river under anthropogenic disturbance, Bayesian mixing model, seasonal variation, sources
Citation: Kaymak N, Mutlu T and Verep B (2023) Distribution and sources of particulate organic matter from the anthropogenically disturbed Iyidere River to the Black Sea coast. Front. Environ. Sci. 11:1162601. doi: 10.3389/fenvs.2023.1162601
Received: 09 February 2023; Accepted: 04 April 2023;
Published: 17 April 2023.
Edited by:
Holly Michael, University of Delaware, United StatesReviewed by:
Peng Zhang, Guangdong Ocean University, ChinaSelvaraj Kandasamy, Xiamen University, China
Copyright © 2023 Kaymak, Mutlu and Verep. This is an open-access article distributed under the terms of the Creative Commons Attribution License (CC BY). The use, distribution or reproduction in other forums is permitted, provided the original author(s) and the copyright owner(s) are credited and that the original publication in this journal is cited, in accordance with accepted academic practice. No use, distribution or reproduction is permitted which does not comply with these terms.
*Correspondence: Nehir Kaymak, bmVoaXJib3prdXJ0QGhvdG1haWwuY29t