- 1Department of Chemistry, College of Science, Princess Nourah bint Abdulrahman University, Riyadh, Saudi Arabia
- 2Department of Chemistry, Forman Christian College (A Chartered University), Lahore, Pakistan
- 3Biology Department, College of Science, Taibah University, Al Madinah Al Munawarah, Saudi Arabia
- 4Department of Chemistry, School of Natural Sciences (SNS), National University of Science and Technology (NUST), Islamabad, Pakistan
- 5Department of Chemistry, Turabah University College, Taif University, Taif, Saudi Arabia
- 6Centre of Excellence in Environmental Studies (CEES), King Abdulaziz University, Jeddah, Saudi Arabia
- 7Department of Chemistry, Division of Science and Technology, University of Education, Lahore, Pakistan
- 8Department of Chemistry, College of Science and Technology, Wenzhou-Kean University, Wenzhou, China
- 9Chemistry Department, Faculty of Science, King Khalid University, Abha, Saudi Arabia
- 10Faculty of Engineering, King Khalid University, Abha, Saudi Arabia
- 11Department of Pharmaceutical Sciences, College of Pharmacy, AlMaarefa University, Riyadh, Saudi Arabia
Metal oxide-based adsorbents are quite in for wastewater treatment because of their selectivity, stable structure and very low solubility in aqueous systems. To explore the adsorption of methylene blue (MB), Mn3O4-Bi2O3 adsorbents were made using a wet-impregnation technique with various concentrations of Mn3O4. The presence of Mn3O4 contents on the surface of monoclinic Bi2O3 was confirmed through representative scanning electron micrographs. The diffractions pertaining to cubic Mn3O4 and monoclinic Bi2O3 were noticed in the XRD pattern of 5% Mn3O4-Bi2O3 which confirm the composite nature of the adsorbent. XPS analysis revealed the existance of Bi 4f, Bi 4d, Bi 4p, Bi4s, and Mn 2p core levels in Mn3O4-Bi2O3. The adsorption study divulged highest efficiency (∼95% and qe = ∼1.4 mgg-1) of 5% Mn3O4-Bi2O3 composite among other contestants in removing 30 ppm MB at 28
Introduction
It is well-established that the textile industries around the world are utilizing over 1,000 tons of approximately 10,000 commercially available dyes in a year, wherein 10%–15% of these dyes are being released into waterbodies as effluent [ (El-Zahhar and Awwad, 2016; Homaeigohar et al., 2016; Hu and Xu, 2020)]. Textile dyes are complex organic toxic compounds that can cause serious threats to the environment if they are not handled properly [ (Ayub et al., 2020; Palansooriya et al., 2020; Rahman et al., 2020)]. Moreover, the long-term presence of these dyes in aqueous systems due to their stable chemical structure reduces oxygen contents in water which disturbs aquatic life and produces carcinogenic and mutagenic compounds which pose toxic effects [ (Zaharia et al., 2009; Yagub et al., 2014; Hu et al., 2018; Alshorifi et al., 2022)].
Among textile dyes, methylene blue (MB) is an aromatic, cationic and heterocyclic dye. At room temperature, it is an odourless solid dark green powder that turns dark blue when dissolved in water. Due to its exceptional resistance to the stomach’s acidic environment and the body’s natural hydrolytic enzymes, it is extremely stable in the human body. The liver fails to metabolize the dye and is filtered out through the kidneys. Continuous human exposure to MB can cause serious health issues such as eyes to burn, irritation in the skin and gastrointestinal tract, nausea, profuse sweating, mental confusion, methemoglobinemia, vomiting and diarrhea [(Laurent et al., 2008; Dada et al., 2016; Li et al., 2018)]. Therefore, it is highly desirable to treat MB-contaminated water and finding an effective method to treat contaminated water without producing secondary waste is the prime concern of the day. In this context, studies are underway in the exploration of cost-effective and facile techniques for the cleaning of polluted water without even leaving trivial traces of dyes.
The removal of dyes and high-risk pollutants from water using flexible techniques such adsorption, membrane separation, photocatalysis, electrosorption, electro-flocculation, and nanofiltration has been practised to reduce environmental concerns over the past few decades [(Bakry et al., 2022)]. Among these removal techniques, Adsorption is found to be the best approach that has greater potential to remove toxic dyes, due to its adaptability, economical affordability, low-energy requirements, and easy maintenance mechanism [(Allen and Koumanova, 2005; Li et al., 2020; Ibrahim et al., 2021)]. This technique is mainly based on the forces between the adsorbate and adsorbent. Moreover, being a key component of adsorption the exploration of adsorbents for the whole elimination of organic contaminants from polluted water is highly desirable because there is a lack of actual progress in wastewater treatment due to the inaccessibility of high-capacity adsorbents.
Metal oxide-based adsorbents, owing to high capacity, selectivity, specific surface energy and affinity, chemically stable structure and low solubility are declared efficient contestants for the adsorption of contaminants such as dyes, phenols, pesticides, etc. [(Faraji et al., 2010; De Jesús Ruíz-Baltazar et al., 2019; Mittal et al., 2020)]. Previously, iron oxides, titanium dioxide, cobalt oxide, zinc oxide, aluminum oxide, magnesium oxide and mixed metal oxides in their pure and modified forms were used for the treatment of dye-containing water [(Krishnan et al., 2022)]. A couple of years ago, Das and Sharma synthesized Gd2O3/Bi2O3@rGO adsorbent for the elimination of methyl orange dye and optimized the parameters for efficient adsorption of adsorbate. [(Das and Sharma, 2020)]. Recently, Adam et. al described an increase in the adsorption efficiency of MgO with the increasing content of Bi2O3 for the removal of indigo carmine [(Adam et al., 2022)]. While taking the advantage of metal oxide-based adsorbents, in this study an effort has been made to prepare novel composites of surface altered monoclinic Bi2O3 with varying amounts of Mn3O4 using wet-impregnation methodology. The synthesized nanocomposites were further utilized to remove 30 ppm MB from the contaminated water. Moreover, different kinetic models and adsorption isotherms were also studied to investigate the phenomenon of adsorption of MB on newly synthesized composites. As this study was not done before while taking into account the removal of MB by Mn3O4-Bi2O3 composites through the adsorption process, however, effecincy of a few metal oxide-based adsorbents were reported for the adosprion of MB [(Gómez-Pastora et al., 2014; Yang et al., 2014; Li et al., 2019; Naseri and Allahverdi, 2019; Alomar et al., 2021)]. Morover, this study provides a sufficient insight about the synthesis and characterization of novel Mn3O4-Bi2O3 adsorbent having excellent adsorption efficiency prepared through surface modification technique for the removal of MB dye.
Experimental
Materials
Manganese (II) nitrate tetrahydrate [Mn(NO3)2.4H2O, Sigma-Aldrich, ≥ 97%], Ethanol (C2H5OH, Sigma-Aldrich, ≥ 99.8%), Bismuth (III) nitrate pentahydrate [Bi(NO3)3.5H2O, Sigma-Aldrich, ≥ 98%], Acetone [CH3COCH3, Sigma-Aldrich], Nitric acid [HNO3, Sigma-Aldrich, 70%], potassium hydroxide [KOH, Sigma-Aldrich, 99.5%], Distilled water and Triton X-100 [Tx-100, Sigma-Aldrich] were used without further purification for the synthesis of Bi2O3-based adsorbents. These synthesized composites were used to remove MB [C16H18CIN3S, Sigma-Aldrich, ≥ 95%], the target pollutant, from the contaminated water.
Surfactant-assisted synthesis of α-Bi2O3 and Mn3O4 adsorbents
α-Bi2O3 and Mn3O4 were synthesized as per the reported method [ (Hameed et al., 2015; Qamar et al., 2017a)]. In a typical synthesis of α-Bi2O3, 25 g Bi (NO3).5H2O was dissolved in a solvent containing 85 mL distilled water and 15 mL nitric acid at 50
Mn3O4 was synthesized by dissolving 10 g Manganese (II) nitrate tetrahydrate in distilled water. After the complete dissolution of the precursor in distilled water, 3 mL surfactant TX -100 was added. The surfactant-containing solution was hydrolyzed with the slow addition of 0.5 M KOH till pH 8 with stirring at 50
Synthesis of Mn3O4-Bi2O3 adsorbents using wet-impregnation
Mn (NO3)2.4H2O was used to prepare 1, 3, 5, and 10 wt% composites of Mn3O4-Bi2O3 using the wet-impregnation method by varying the amount of Mn concerning the fixed weight of Bi2O3. In a typical synthesis of 1% Mn3O4-Bi2O3 composite, 0.23 g Mn (NO3)2.4H2O was disbanded in 50 mL distilled water at 50
Characterization of the synthesized material
By using an X’pert X-ray powder diffractometer (Philips PW1398) with a Cu K radiation source at 40 mA electric current and 40 kV operating voltage, XRD patterns were acquired from 10o to 80o with 0.01o step size. The PHI 5000 Versaprobe II X-ray photoelectron spectrometer captured the XPS survey scan of the 5 percent Mn3O4-Bi2O3 sample in the binding energy range of 0–1,350.08 eV. The morphology of the synthesized adsorbents was evaluated by scanning electron microscope (TESCAN LYRA3). Thermal stability of 5% Mn3O4-Bi2O3 before and after adsorption was inspected using TA instruments SDT Q600 TGA/DSC analyzer in N2 environment from 0°C to 700°C at 20°C/min rate. FTIR spectra of MB and 5% Mn3O4-Bi2O3 were recorded before and after adsorption ranging from 550 to 4,000 cm-1 using Bruker Alpha II FTIR spectrometer.
Adsorption study
Synthetic wastewater composed of MB was used as an adsorbate and its four various contents, i.e., 5, 10, 20, and 30 ppm were prepared in distilled water by dissolving 5, 10, 20, and 30 mg MB, respectively. In the first phase, 6 different conical flasks containing 50 mL 30 ppm MB were taken and then 1 g of each adsorbent, i.e., Bi2O3, Mn3O4, 1, 3, 5, and 10 wt% Mn3O4-Bi2O3 was added in the flasks, respectively. The flask having a heterogeneous mixture of adsorbate and adsorbent were shaken in an orbital mechanical shaker at 250 rpm, 28 ᵒC, 7 pH for 5 h. The adsorption of MB on each adsorbent was monitored by collecting samples from each flask after 30, 60, 120, 180, 240, and 300 min (contact time) shaking time. The filtered samples were measured for percent adsorption and adsorption capacity (qt) using the following equations in a UV-Visible spectrophotometer after being filtered with a syringe filter [(Mahmoud and Mahmoud, 2021; Kasbaji et al., 2022)]:
Where Co is the initial concentration of the adsorbate; Ct is the concentration of the adsorbate at any time (t); V is the volume of adsorbate in (l) and W is the amount of adsorbent in grams (g). The MB concentration was calculated using UV-Visible spectrophotometer at 665 nm (λmax). In the second phase, a similar adsorption procedure as stated above was followed to study the adsorption of 5, 10, 20 and 30 ppm MB on 5% Mn3O4-Bi2O3 adsorbent for 480 min shaking time. After an hour, the samples were collected and put through a syringe filter. Using Eqs 1, 2, the obtained samples were then exposed to a UV-Visible spectrophotometer for the purpose of determining the percent adsorption and adsorption capacity.
Study of kinetic models and adsorption isotherms
The adsorption process of MB on Bi2O3- based adsorbents at 28
Recycling of 5% Mn3O4-Bi2O3 adsorbent
Initially, the regeneration study was performed in a 250 mL beaker using 90% ethanol as per reported method (Li et al., 2020). Secondly, the conical flask containing suspension composed of 1.0 g regenerated 5% Mn3O4-Bi2O3 adsorbent and 50 mL 30 ppm MB adsorbate was placed in a n orbital mechanical shaker at 28
Results and discussion
Characterization of the synthesized material
XRD patterns of the designed Bi2O3 and 5% Mn3O4-Bi2O3 adsorbents are presented in Figure 1A. A moboclinic phase of α-Bi2O3 with lattice parameters (
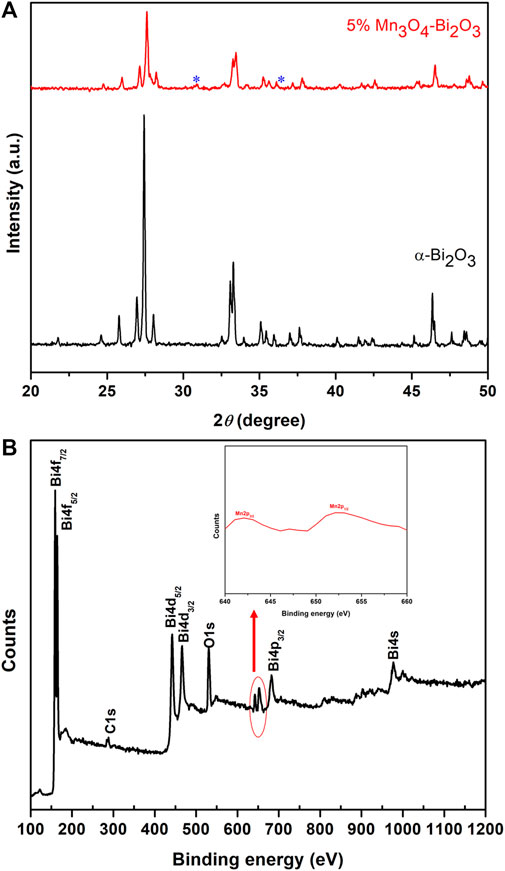
FIGURE 1. (A) The comparison of XRD patterns of Bi2O3 and 5% Mn3O4-Bi2O3 in 2θ range of 20° −50° and (B) the wide angle XPS survey scan of 5% Mn3O4-Bi2O3 composite.
The FTIR spectra of 5% Mn3O4-Bi2O3 composite (before and after adsorption) and MB dye are presented in Figure 2A, where the bands due to Bi-O-Bi and Mn-O vibrations at 846 cm−1 and 574 cm−1 are observable in 5% Mn3O4-Bi2O3. The appearance of these bands is in close accordance with the literature, which further confirms the composite nature of the adsorbent [(Zulkifli et al., 2018; Khan and Wahab, 2022)]. A borad band at 3,299 cm−1 is attributed to OH groups in aqueous solution of MB whereas a peak appeared at 1,636 cm-1 is mainly due to vibrations of C=N and C=C groups in aromatic rings of MB [(Raiyaan et al., 2021; Birniwa et al., 2022)].
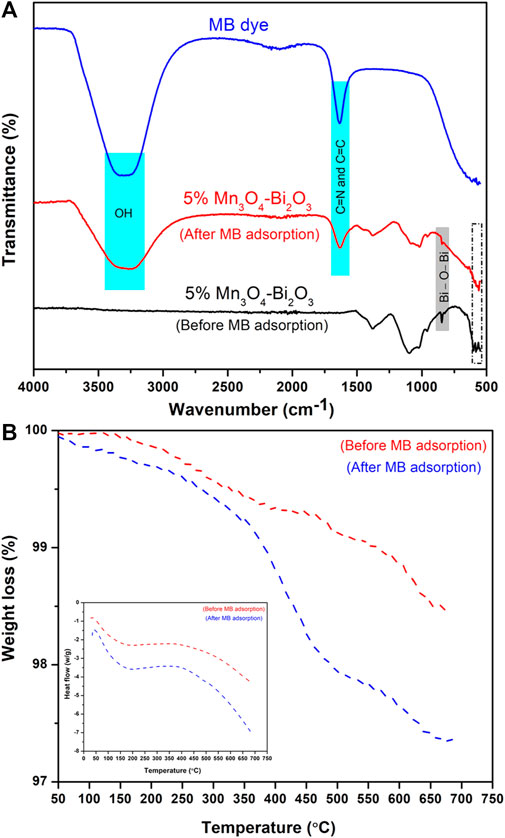
FIGURE 2. (A) The comparison of FTIR spectra of MB and 5% Mn3O4-Bi2O3 adsorbent and (B) the TGA/DSC curves of 5% Mn3O4-Bi2O3 composite.
FTIR spectrum of 5% Mn3O4-Bi2O3 composite shows a decrease in Bi-O-Bi and Mn-O bands after the adsorption of MB. In addition, the peaks due to OH and aromatic rings related to MB solution with minor shift are also observed in FTIR spectrum of adsorbent after adsorption. The reduction in peak intensity and shifts in band positions as shown in Figure 2A further authorise the binding of MB dye with adsorbent. The thermal stability of the 5% Mn3O4-Bi2O3 composite was investigated through TGA/DSC as shown in Figure 2B, where the composite experienced a weight loss of about 1.5%; however, after the MB dye was adsorbed, this loss increased to a maximum of 2.6%. The main cause of this comparatively larger weight loss is the desorption of water and MB molecules from the adsorbent’s surface (Naeem et al., 2016). Moreover, the higher stability and lower content degradation rate of 5% Mn3O4-Bi2O3 composite was noticed before adsorption in comparison to after MB adsorption as presented in DSC curves given in inset of Figure 2B.
Dye adsorption onto synthesized adsorbents
Using a UV-visible spectrophotometer, the adsorption of 30 ppm MB on the unaltered and modified Bi2O3 was observed. The spectra captured after various shaking periods are shown in Figure 3. The comparison of MB removal shows that the maximum adsorption of the pollutant was noticed by 5% Mn3O4-Bi2O3 composite among all synthesized adsorbents whereas its comparion with few previously studied metal oxide-based adsorbents is given in Table 1. Moreover, the continuous decrease in MB concentration with increasing contact time for all adsorbents can be seen in Figure 3d. The efficient adsorbent 5% Mn3O4-Bi2O3 was able to remove ∼95% MB (qe = ∼ 1.4 mgg-1) in 4 h at 28
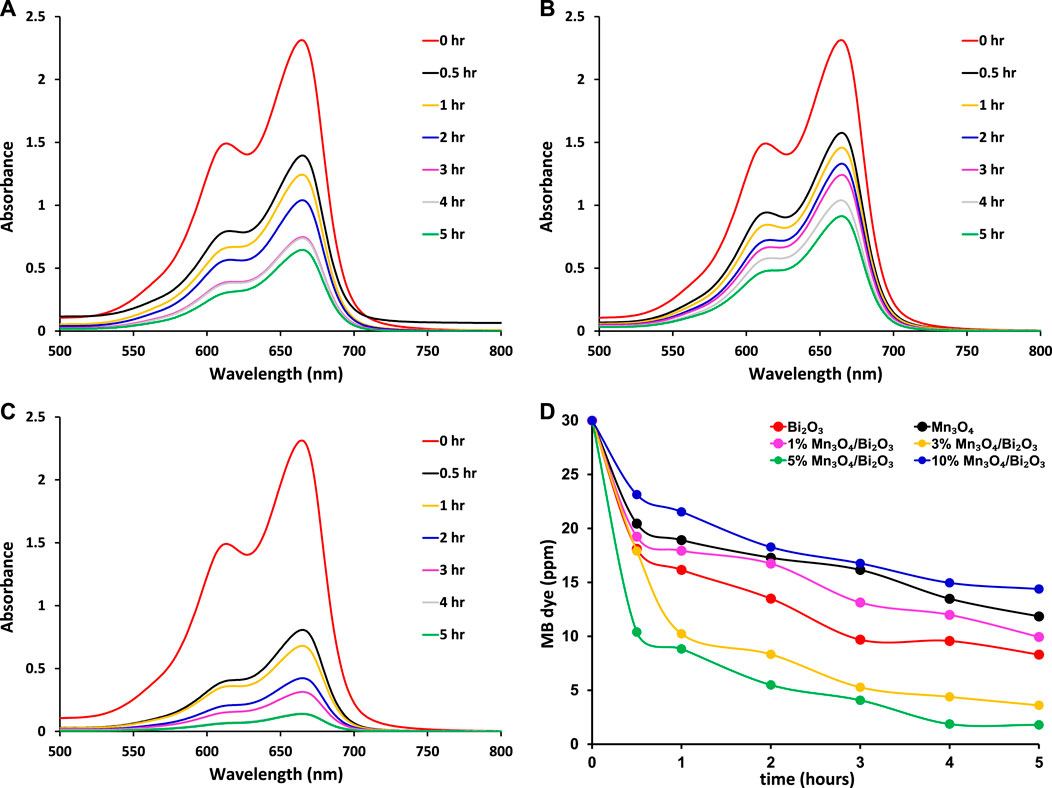
FIGURE 3. The comparison of UV-Vis. absorption of unadsorbed MB at 665 nm after adsorption on prepared (A) Bi2O3 (B) Mn3O4 and (C) 5% Mn3O4-Bi2O3 adsorbents at 28
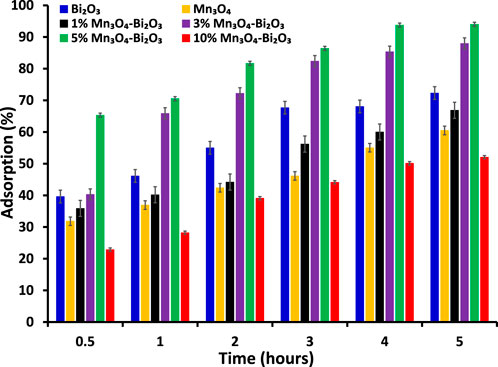
FIGURE 4. The comparison of percentage adsorption of 30 ppm MB on different adsorbents at 28
The possible greater adsorption of MB on 5% Mn3O4-Bi2O3 as compared to Bi2O3 was attributed to the increase in capturing sites than pure Bi2O3. Whereas, the lower dye removal by 10% Mn3O4-Bi2O3 may be due to the greater content of Mn3O4 which showed lower adsorption of MB when it was used alone. Therefore, a proper balance between the quantities of both components in a composite is an important factor to consider while synthesing adsorbents for dye removal from polluted water. Due to the existence of more captacuring sites on the surface of the composite adsorbent than on the surface of unmodified Bi2O3, as seen in Figure 5D, the enhanced removal effectiveness of 5% Mn3O4-Bi2O3 was further strengthened. Additionally, the surface of Bi2O3 in composites was found to have smaller Mn3O4 aggregates; however, the unmodified surface of monoclinic Bi2O3 without aggregates is clearly visible in the scanning electron micrographs shown in Figure 5.
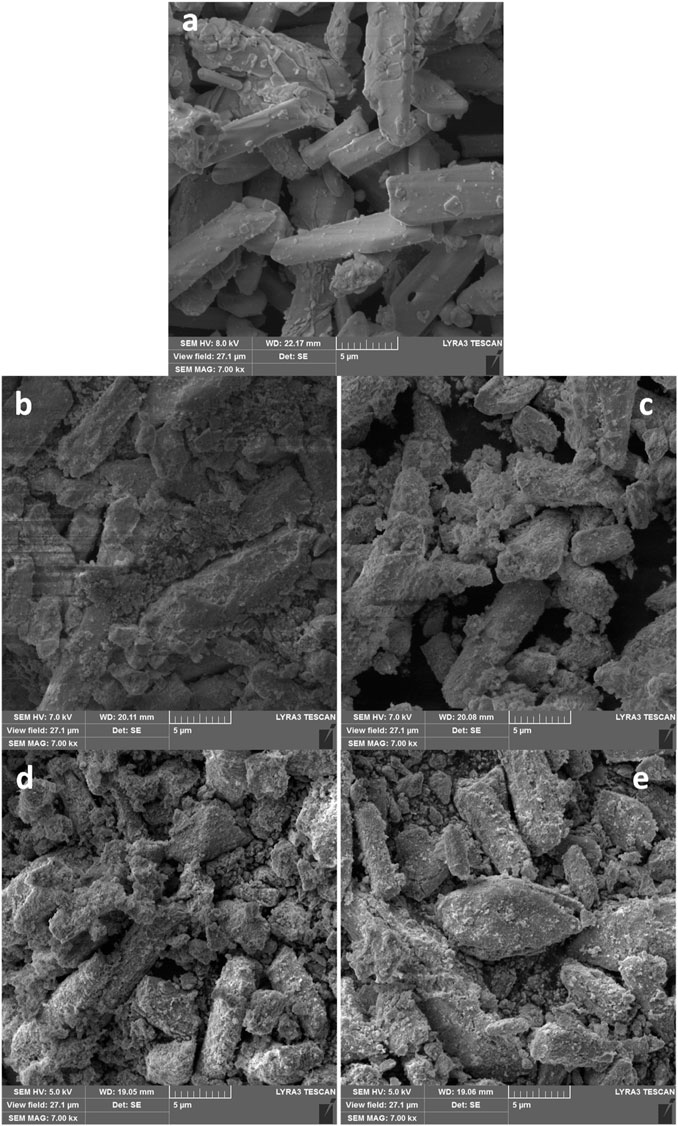
FIGURE 5. The scanning electron micrographs of (A) Bi2O3 (B) 1 wt% (C) 3 wt% (D) 5 wt% and (E) 10 wt% Mn3O4-Bi2O3 adsorbents at 7.00 kx magnifications.
Effect of initial concentration of adsorbate and contact time
The effect of different concentrations of MB on the adsorption efficiency of 5% Mn3O4-Bi2O3 was investigated with four different concentrations of MB dye such as 5, 10, 20, and 30 ppm at different contact times. Figure 6 shows the graphs that show how varied initial MB concentrations affect adsorption capacity. As the initial concentration of MB rises, the contact time has a substantial impact on the adsorption mechanism because there are more active sites accessible. However, after some time, the equilibrium is reached, and the adsorption becomes constant as illustrated in Figure 6. Moreover, a similar trend was also noticed in few previously reported adsoprtion studies [(Al-Ghouti et al., 2009; Shi et al., 2014; Basu et al., 2018)]. It is observed that in initial 30 min, the fastest adsorption occurs at all concentrations. The plot (qt vs. t) clearly shows that a condition of dynamic equilibrium is reached after a certain contact time and the value of qt becomes constant. This equilibrium is a state where the rate of adsorption and desorption becomes equal [(Tan et al., 2008; Ishak and Kumar, 2022)]. It is also evident from the Figure 6 that the process of MB adsorption does not substantially change after equilibrium time. Moreover, The curves show that the equilibrium condition of the 30 ppm MB dye took 4 h to reach, whereas the equilibrium states of the other concentrations (5, 10, and 20 ppm) were almost reached after 2 h of contact time.
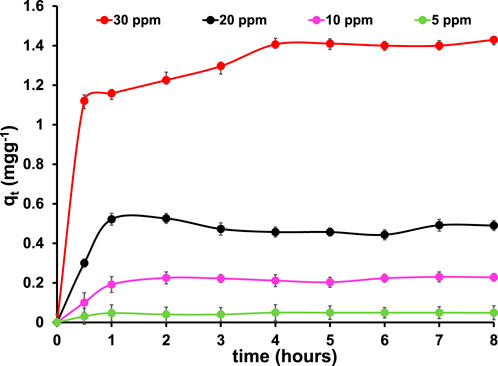
FIGURE 6. Effect of preliminary MB concentration on adsorption capacity of 5, 10, 20 and 30 ppm MB dye at 28
As the process of adsorption involves, firstly the mass transfer of dye molecules to the adsorbent’s surface, i.e., external diffusion. Secondly, the dye molecules starts attaching and forming a thin film onto the surface of adsorbent, i.e., actual adsorpton and finally the diffusion of adsorbed dye molecules in the pores of adsorbent, i.e., interior diffusion [(Abou-Gamra and Ahmed, 2015)]. It is observed that at a lower concentration of MB, adsorption equilibrium is achieved earlier than higher MB concentration as shown in Figure 4. Which suggests that MB dye removal process mainly follows actual adsorption phenomenon instead of internal and external diffusion phenomena at lower MB concentration whereas the removal of 30 ppm MB is found to be controlled by both actual adsorption and internal diffusion processes [(Abou-Gamra and Ahmed, 2015; Amadi et al., 2017)].
Kinetic models
The adsorption rate of 30 ppm MB on all synthesized adsorbents is evaluated through pseudo 1st and pseudo 2nd-order kinetic models. The models were used to predict the nature of adsorption and determination of adsorption rate constants. Moreover, R2 values were used to deveolp a correlation between experimental data and kinetic models. The kinetics of adsorption are assumed to be inversely related to dye concentration and directly proportional to the availability of sites on the adsorbent’s surface in the pseudo-first order kinetic model. The rate constant (k1) was determined using following pseudo 1st-order kinetic model equation [(Kasbaji et al., 2022)]:
where k1 (min-1) is the rate constant and qe and qt are the amounts of MB dye adsorbed (mg/g) at equilibrium and a time t (minutes), respectively. Additionally, as shown in Figure 7A, the values of k1 and qe are determined from the slope and intercept of the plot, respectively.
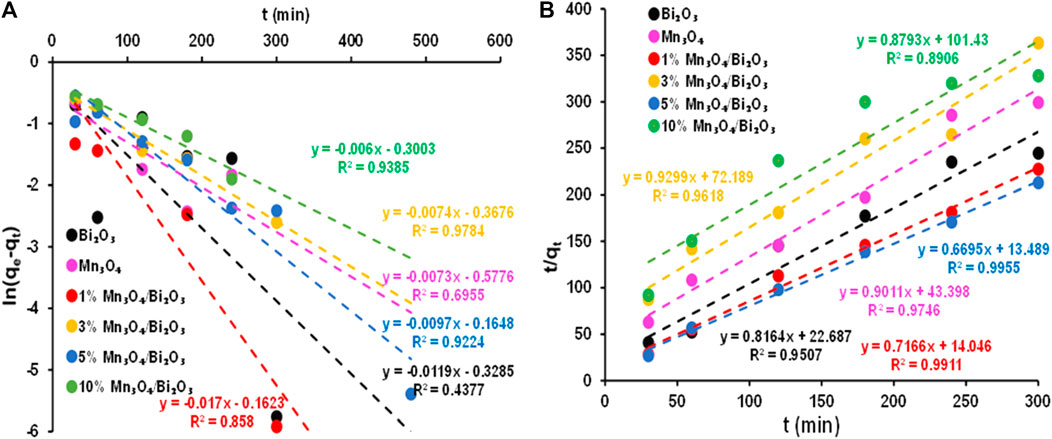
FIGURE 7. Linearized plots of (A) pseudo 1st order and (B) pseudo 2nd order for the adsorption of 30 ppm MB at 28
Contrary to pseudo 1st-order, pseudo 2nd-order presumes that the rate constant is directly proportional to available active sites of adsorbents. This kinetic model is applied to examine the chemisorption kinetics of dye and adsorbent. The linear equation of Pseudo 2nd-order is given below [34]:
All the parameters are well described above. The plot of t/qt against t shown in Figure 7B can be used to determine the value of constant k2 (g/mg min).
Pseudo 1st-order and 2nd-order plots for Bi2O3, Mn3O4, 1% Mn3O4-Bi2O3, 3% Mn3O4-Bi2O3, 5% Mn3O4-Bi2O3 and 10% Mn3O4-Bi2O3 are shown in Figure 7. These graphs and the kinetic parameters in Table 2 make it clear that practically all adsorbents have greater linear regression coefficients in pseudo 2nd-order kinetic models than in 1st-order kinetic models. Accordingly, pseudo first order provides a poor description of the kinetics of all the adsorbents stated above, while pseudo second order provides the best fit for the kinetics of the adsorption of 30 ppm MB on all the adsorbents. This kinetic study suggests that the adsorption of 30 ppm MB on synthesized adsorbents follows chemical adsorption mechanism wherin the adsorbent surface is playing a rate-limiting role [(Ramutshatsha-Makhwedzha et al., 2022; Nayl et al., 2023)].
The adsorption of 5, 10, 20, and 30 ppm MB onto a 5 percent Mn3O4-Bi2O3 adsorbent was also examined using pseudo 1st order and pseudo 2nd order kinetics, and the corresponding plots and kinetic parameters are shown in Figure 8 and Table 3, respectively. The favorability of the kinetic models was decided based on R2 values and adsorption data was found to agree with the pseudo 2nd order model. Which was adequate proof that MB was chemisorption on a 5 percent Mn3O4-Bi2O3 composite, which revealed the necessity of electron sharing or exchange between MB and the surface of the adsorbent to complete the adsorption process.[(Li et al., 2015; Ahmadi et al., 2020)]. Additionally, it is probable that a decrease in adsoptive sites at the surface of the adsorbent is to blame for the observed decrease in k2 with an increase in dye concentration. [(Rápó and Tonk, 2021; Tejada-Tovar et al., 2021; Al-Gorair et al., 2022)]
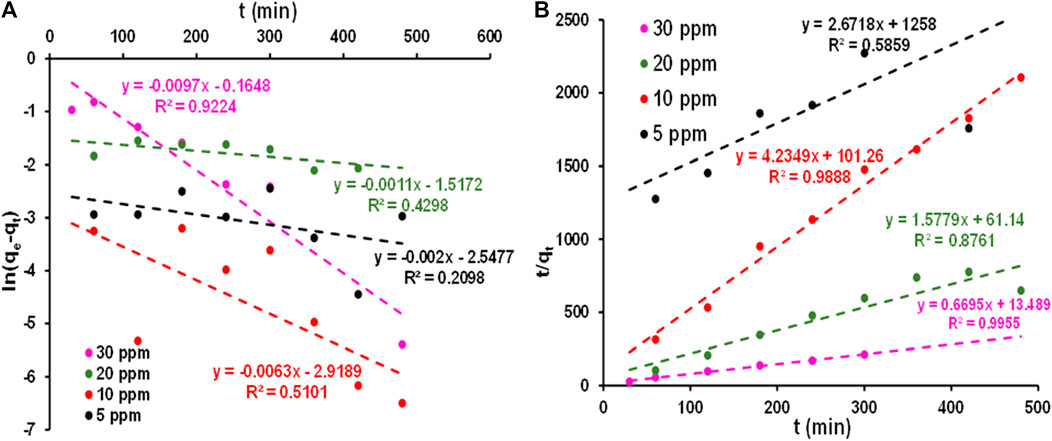
FIGURE 8. Linearized plots of (A) pseudo 1st order and (B) pseudo 2nd order for the adsorption of 5, 10, 20 and 30 ppm MB at 28
Adsorption isotherms
The adsorption isotherms evaluate the relationship between unadsorbed and adsorbed adsorbate at an equilibrium state. According to the literature, it is vital for adsorption related studies to analyse the data after fitting it into different adsorption isotherms. Additionally, the interpretation of isotherms is a critical procedure but necessary to design an effective adsorption process. The results obtained from isotherms are used to optimize the adsorbent properties and further help to understand the adsorption mechanism because each isotherm model evaluates certain physical and chemical properties needed for the adsorption process [(Das and Sharma, 2020; Adam et al., 2022)]. In order to get a detail insight about the adsorption of methylene blue onto 5% Mn3O4-Bi2O3 the following adsorption isotherms have been applied in this study. Moreover, the applicability of these isotherms is evaluated by comparing respective correlation coefficient (R2) values.
According to the Langmuir adsorption isotherm, the development of a homogenous monolayer of dye molecules on the adsorbent surface triggers the adsorption of the adsorbate. Additionally, it is claimed that only certain active sites underwent adsorption and that no interactions between adsorbed molecules and phase transitions exist. Additionally, the Langmuir adsorption isotherm is described by the following equation [33, 34]:
where Ce denotes the adsorbate concentration at equilibrium (MB) and qe is the amount of dye adsorbed in (mg/g) at equilibrium. While KL (l/mg) is the Langmuir constant, which is connected to the adsorption energy, and qmax (mg/g) is the maximum adsorption capacity. The Ce/qe and Ce representative graph is presented in Figure 9A, and Table 3 contains the evaluated values. The crucial component of the Langmuir isotherm, generally referred to as the separation factor, is another dimensionless quantity, RL. The following equation is used to calculate this parameter [(Kasbaji et al., 2022)].
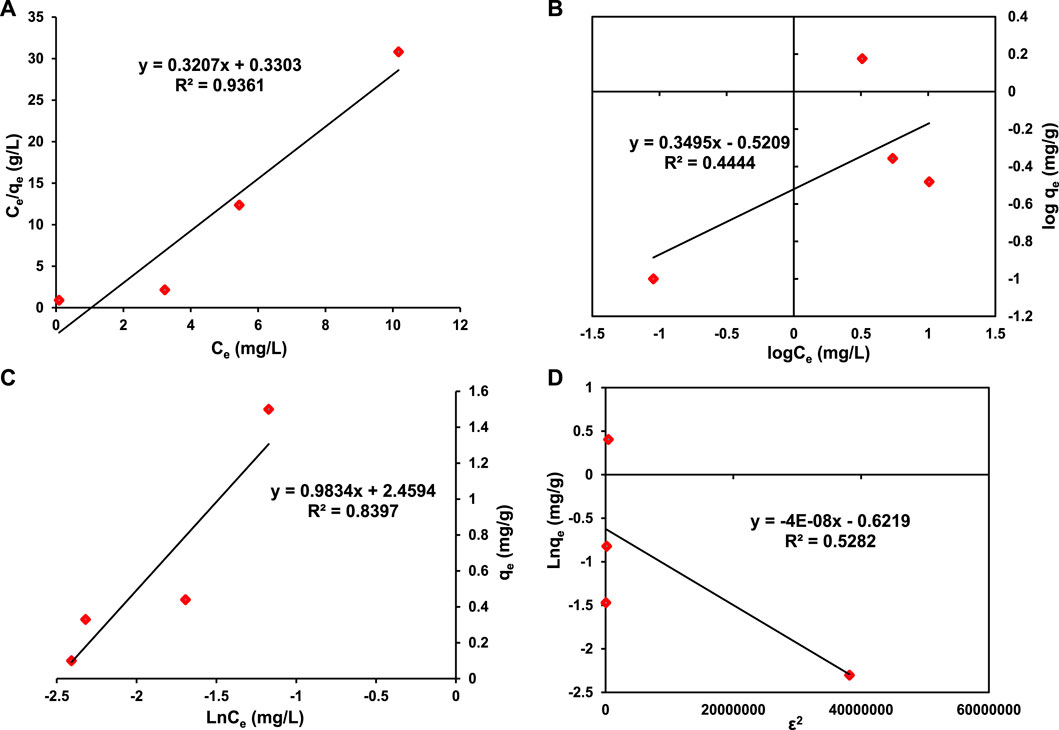
FIGURE 9. Adsorption isotherms (A) Langmuir (B) Freundlich (C) Temkin (D) Dubinin-Raduskavich-Kaganer (DRK).
The RL value indicates whether it is linear (RL = 1), irreversible (RL = 0), favorable (0< RL <1) or unfavorable (RL >1). RL value investigated for this study is 0.0004 which depicts that the adsorption of MB dye on 5% Mn3O4/Bi2O3 is favorable.
The heterogeneous surface of the adsorbent is studied via the Freundlich adsorption isotherm for both monolayer and multilayer adsorption. It also explains why the molecules that have been adsorbed repel one another. Additionally, it assesses the active spots’ exponential distribution and potential energies. The following equation presents the Freundlich isotherm’s linear representation [(Kasbaji et al., 2022)]:
where the Freundlich constants KF (l/mg) and 1/n, which characterise the adsorption capacity and intensity, respectively, are used. The value of n, which ranges from 1 to 10, indicates how well the adsorption process works, and KF, for the unit equilibrium concentration, is the amount of MB adsorbed on the adsorbent, 5% Mn3O4/Bi2O3. Moreover, the value of 1/n below one and closer to 0 indicates the more hetrogenisty of surface [(Bello et al., 2010; Ameta, 2020; Bindhu et al., 2021)]. In the present study, the value of 1/n is 0.3495 which suggests the adsorption of MB on the prepared adsorbent is a favorable and heterogeneous [(Ayawei et al., 2017)]. The adsorption parameters obtained in this study are presented in Table 4 whereas the representative plots (log Ce vs. log qe) are shown in Figure.9B. Additionally, the low R2 value (0.4444) for the Freundlich model in this study as compared to other isotherms indicates the absence of multilayer physisorption.
The Temkin model explains how the adsorbate and adsorbent interactions affect the adsorption mechanism. It implies that when the number of adsorbate molecules increases on the adsorbent surface, the heat of adsorption (ΔHads) decreases. The Temkin isotherm is expressed according to the following equations [(Kasbaji et al., 2022)]:
where,
The Temkin constant for the heat of adsorption is bT (J/mol), while the equilibrium binding constant is AT (l/g). The values of the constants are calculated from the slope and intercept of the plot in Figure 9C, which is qe vs. ln Ce. The obtained values of bT and AT are presented in Table 4 which are 2565 J/mol and 12.2 l/g, respectively with 0.8397 R2 value in this investigation.
Dubinin-Raduskavich-Kaganer (DRK) is a semiempirical adsorption isotherm, which is primarily designed to express the Gaussian Energy distribution onto the heterogeneous surface of an adsorbent. It assumes that adsorption occurs through pore filling multilayer physical mechanism. DRK is a temperature-dependent model. The model is expressed by the following equation [(Kasbaji et al., 2022)]:
where
KDRK (mol2/kJ2) is the Dubinin-Raduskavich-Kaganer constant which is relates to free adsorption energy, whereas qd (mg/g) is the theoretical adsorption ability. The values of these constants KDRK and qd are mentioned in Table 4 which are 4 × 10−8 mol2/kJ2 and 0.5369 mg/g, respectively. These values are estimated by interpreting the slope and intercept of the plot presented in Figure 9D. It distinguishes between physical and chemical adsorption with its mean free adsorption energy, E calculated as [ (Kasbaji et al., 2022)]:
The value of E greater than 7 kJ/mol indicates the nature of chemical adsorption. The R2 value for DRK model obtained from the plot in Figure 9D is 0.5282, which is not very ideal, and this shows the following mechanism does not follow the DRK model.
It is evident from the adsorption isotherms that the adsorption of MB on 5% Mn3O4-Bi2O3 follows the Langmuir isotherm the best. The decreasing trend of validity of adsorption isotherms based on R2 value is; Langmuir isotherm > Temkin isotherm > Dubinin-Radushkevich isotherm > Freundlich isotherm.
The Langmuir model has a higher R2 correlation coefficient than the other isotherms, at 0.9361. This validates the monolayer methylene blue adsorption on the surface of the 5 percent Mn3O4-Bi2O3 adsorbent. Additionally, it is stated that the value of “n" indicates if the adsorption process is appropriate; in this study, the value, 2.86, falls between 1 and 10 and favours the phenomena of sorption [(Bello et al., 2010; Ameta, 2020)]. The adsorption process uses chemisorption, and there are barely any interactions between the methylene blue molecules that have been adsorbed on the surface of the adsorbent. The adsorption technique was more advantageous since the separation factor’s RL value was less than 1.
The Temkin isotherm’s R2 value, which is 0.8397, indicates that the adsorption process somewhat resembles the Temkin model. From this information, it is inferred that the interaction between MB molecules and adsorbent increases due to the increase in surface coverage of 5% Mn3O4-Bi2O3 adsorbent. Moreover, the validity of Temkin model confirms the chemisorption process of MB onto 5% Mn3O4-Bi2O3 [(Ayawei et al., 2017)].
Recycling of 5% Mn3O4-Bi2O3 adsorbent
The 5% Mn3O4-Bi2O3 adsorbent was used in four cycles for the adsorption of 30 ppm MB and increase in concentration of unadsorbed MB dye was noticed as shown in the Figure 10. It is observed from the plot that ∼95, ∼90, ∼83, and ∼76% MB dye was removed by 5% Mn3O4-Bi2O3 in 1st, 2nd, 3rd, and 4th cycle, respectively. It is inferred from this recycling study that the adsorbent material can be successfully recycled and reused for the removal of MB even after four cycles.
BET analysis
The surface areas of Bi2O3, Mn3O4, and Mn3O4-Bi2O3 have been calculated using isotherms of nitrogen absorption and desorption. The observed isotherms correlate to mesoporous structures when compared to the Mn3O4-Bi2O3 composites isotherm pattern and the IUPAC standard isotherm pattern. The Mn3O4-Bi2O3 has a higher surface area of 73.81 m2g−1 than that of Bi2O3 (18.46 m2g−1) and Mn3O4 (31.29 m2g−1), according to the N2 adsorption-desorption isotherm findings (Figure 11). The potential for adsorption of nanocomposites with large surface areas is thought to be increased by the synergism effect of individual nanoparticles. The mesoporous Mn3O4-Bi2O3 composites, however, can successfully boost surface active sites, improving the adsorbent’s adsorption properties.
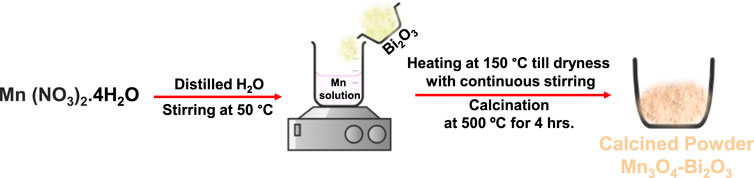
SCHEME 1. Schematic diagram for the synthesis of Mn3O4-Bi2O3 composite using wet-impregnation method.
Antifungal activity
Bi2O3, Mn3O4, and 5% Mn3O4-Bi2O3 were further tested for their antifungal effectiveness against A. solani and M. fructicola using the agar well-diffusion method and amphotericin B as a reference. With zone inhibition values of 58.6 and 53.9 mm, 5 percent 5% Mn3O4-Bi2O3display stronger antifungal capability than Bi2O3 and Mn3O4, according to the antifungal activity data (Table 5). The 5% Mn3O4-Bi2O3 composites interface with the fungal cell membrane, cling to functional protein groups, and ultimately cause fungal cell death due to their high surface area. The synergistic interaction of 5% Mn3O4-Bi2O3 composites and high surface area led to the increased antifungal impact.
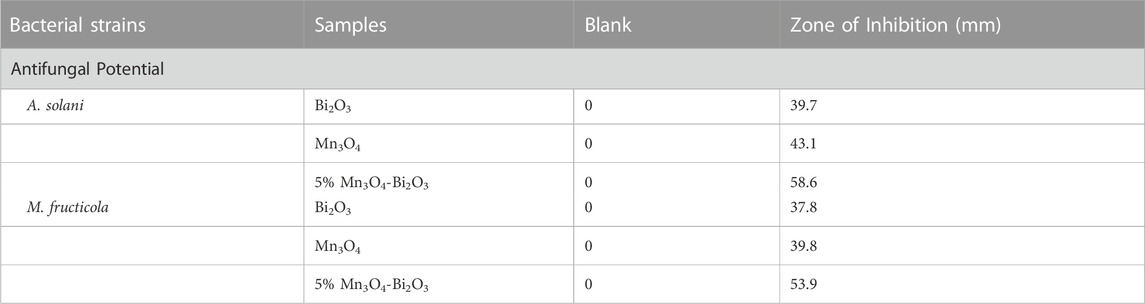
TABLE 5. Zone of inhibition for the antifungal activity of Bi2O3, Mn3O4, and 5% Mn3O4-Bi2O3 using the Agar Well diffusion method.
Conclusion
Mn3O4-Bi2O3 adsorbents were successfully prepared through wet-impregnation method and smaller aggregates of Mn3O4 were noticed on monoclinic α-Bi2O3 surface. The composite nature of the adsorbent was verified through FTIR, XRD, SEM and XPS analyses. Among the prepared adsorbents, 5% Mn3O4-Bi2O3 was found efficient to remove 30 ppm MB at 28
Data availability statement
The original contributions presented in the study are included in the article/supplementary material, further inquiries can be directed to the corresponding authors.
Author contributions
SIA: Conception, reviewed original manuscript and critical revision, acquisition of data and funding acquisition. AsA: She wrote the antifungal analysis part in the manuscript, interpreted the data and critical revision. MTQ: Conception, design of study, writing-original draft preparation. AhA: He wrote the UV-vis application part in the manuscript, interpreted the data and critical revision. SI: Interpret the data, performed major experimental works, writing-original draft preparation and editing. MTA: Performed UV-vis analysis, reviewed original manuscript and critical revision. MA: He wrote the BET analysis part in the manuscript, interpreted the data and critical revision. MAQ: Reviewed original manuscript, and critical revision. AB: He performed synthesis methodology. NSA: Visualization of data, XRD analysis, funding acquisition, writing reviewing, and editing. YJ: Conception, visualization of data, performed bandgap analysis, funding acquisition. EBE: Visualization of data, reviewed original manuscript and critical revision. All authors listed have made a substantial, direct, and intellectual contribution to the work and approved it for publication.
Funding
The author thankfully acknowledge the funding provided by Scientific Research Deanship, King Khalid University, Abaha, Kingdom of Saudi Arabia, under the grant number R.G.P.1/267/43. The researchers would like to acknowledge Deanship of Scientific Research, Taif University for funding this work. This research was funded by Princess Nourah bint Abdulrahman University Researchers Supporting Project number (PNURSP2023R58), Princess Nourah bint Abdulrahman University, Riyadh, Saudi Arabia. The authors thank Department of Chemistry, Forman Christian College (A Chartered University) Lahore Pakistan for supporting this work. The authors extend their appreciation to the Research Center at AlMaarefa University for funding this work.
Acknowledgments
The author thankfully acknowledge the funding provided by Scientific Research Deanship, King Khalid University, Abaha, Kingdom of Saudi Arabia, under the grant number R.G.P.1/267/43. The researchers would like to acknowledge Deanship of Scientific Research, Taif University for funding this work. This research was funded by Princess Nourah bint Abdulrahman University Researchers Supporting Project number (PNURSP2023R58), Princess Nourah bint Abdulrahman University, Riyadh, Saudi Arabia. The authors thank Department of Chemistry, Forman Christian College (A Chartered University) Lahore Pakistan for supporting this work. The authors extend their appreciation to the Research Center at AlMaarefa University for funding this work.
Conflict of interest
The authors declare that the research was conducted in the absence of any commercial or financial relationships that could be construed as a potential conflict of interest.
Publisher’s note
All claims expressed in this article are solely those of the authors and do not necessarily represent those of their affiliated organizations, or those of the publisher, the editors and the reviewers. Any product that may be evaluated in this article, or claim that may be made by its manufacturer, is not guaranteed or endorsed by the publisher.
References
Abou-Gamra, Z. M., and Ahmed, M. A. (2015). TiO2 nanoparticles for removal of malachite green dye from waste water. Adv. Chem. Eng. Sci. 5 (03), 373–388. doi:10.4236/aces.2015.53039
Adam, F. A., Ghoniem, M., Diawara, M., Rahali, S., Abdulkhair, B. Y., Elamin, M., et al. (2022). Enhanced adsorptive removal of indigo carmine dye by bismuth oxide doped MgO based adsorbents from aqueous solution: Equilibrium, kinetic and computational studies. RSC Adv. 12, 24786–24803. doi:10.1039/d2ra02636h
Ahmadi, S., Mohammadi, L., Rahdar, A., Rahdar, S., Dehghani, R., Adaobi Igwegbe, C., et al. (2020). Acid dye removal from aqueous solution by using neodymium (III) oxide nanoadsorbents. Nanomaterials 10, 556. doi:10.3390/nano10030556
Al-Ghouti, M. A., Khraisheh, M. A., Ahmad, M. N., and Allen, S. (2009). Adsorption behaviour of methylene blue onto Jordanian diatomite: A kinetic study. J. Hazard. Mater. 165 (1-3), 589–598. doi:10.1016/j.jhazmat.2008.10.018
Al-Gorair, A. S., Sayed, A., and Mahmoud, G. A. (2022). Engineered superabsorbent nanocomposite reinforced with cellulose nanocrystals for remediation of basic dyes: Isotherm, kinetic, and thermodynamic studies. Polymers 14 (3), 567. doi:10.3390/polym14030567
Alhogbi, B. G., Aslam, M., Hameed, A., and Qamar, M. T. (2020). The efficacy of Co3O4 loaded WO3 sheets for the enhanced photocatalytic removal of 2, 4, 6-trichlorophenol in natural sunlight exposure. J. Hazard. Mater. 397, 122835. doi:10.1016/j.jhazmat.2020.122835
Allen, S. J., and Koumanova, B. (2005). Decolourisation of water/wastewater using adsorption. J. Univ. Chem. Technol. Metallurgy 40 (3), 175–192.
Alomar, T. S., AlMasoud, N., Sharma, G., Alothman, Z. A., and Naushad, M. (2021). Incorporation of trimetallic nanoparticles to the SiO2 matrix for the removal of methylene blue dye from aqueous medium. J. Mol. Liq. 336, 116274. doi:10.1016/j.molliq.2021.116274
Alshorifi, F. T., Ali, S. L., and Salama, R. S. (2022). Promotional synergistic effect of Cs–Au NPs on the performance of Cs–Au/MgFe2O4 catalysts in catalysis 3, 4-Dihydropyrimidin-2 (1H)-Ones and degradation of RhB Dye. J. Inorg. Organomet. Polym. Mater. 32 (10), 3765–3776. doi:10.1007/s10904-022-02389-8
Amadi, O., Odidiozor, C., and Okoro, I. (2017). Sorption kinetic and intraparticle diffusivities of As3+ and Hg2+ detoxification from aqueous solution using cellulosic biosorbent derived from okra (abelmoschus esculentus) stems. Int. J. Eng. Inf. Syst. 1 (8), 72–85.
Ameta, R. K. (2020). [Fe (CN)5NO]2–-Based MOIFs for adsorption of organic pollutants and as a self-rotatory motor. ACS omega 6 (1), 456–464. doi:10.1021/acsomega.0c04896
Astuti, Y., Fauziyah, A., Nurhayati, S., Wulansari, A., Andianingrum, R., Hakim, A., et al. (2016). in Synthesis of α-Bismuth oxide using solution combustion method and its photocatalytic properties, IOP Conference Series: Materials Science and Engineering (Bristol, United Kingdom: IOP Publishing), 012006.
Ayawei, N., Ebelegi, A. N., and Wankasi, D. (2017). Modelling and interpretation of adsorption isotherms. J. Chem., 2017, 1–11. doi:10.1155/2017/3039817
Ayub, A., Raza, Z. A., Majeed, M. I., Tariq, M. R., and Irfan, A. (2020). Development of sustainable magnetic chitosan biosorbent beads for kinetic remediation of arsenic contaminated water. Int. J. Biol. Macromol. 163, 603–617. doi:10.1016/j.ijbiomac.2020.06.287
Bakry, A. M., Alamier, W. M., Salama, R. S., El-Shall, M. S., and Awad, F. S. (2022). Remediation of water containing phosphate using ceria nanoparticles decorated partially reduced graphene oxide (CeO2-PRGO) composite. Surfaces Interfaces 31, 102006. doi:10.1016/j.surfin.2022.102006
Basu, S., Ghosh, G., and Saha, S. (2018). Adsorption characteristics of phosphoric acid induced activation of bio-carbon: Equilibrium, kinetics, thermodynamics and batch adsorber design. Process Saf. Environ. Prot. 117, 125–142. doi:10.1016/j.psep.2018.04.015
Bello, O. S., Adelaide, O. M., Hammed, M. A., and Popoola, O. A. M. (2010). Kinetic and equilibrium studies of methylene blue removal from aqueous solution by adsorption on treated sawdust. Macedonian J. Chem. Chem. Eng. 29 (1), 77–85. doi:10.20450/mjcce.2010.181
Bindhu, B., Shaji, H., Kuruvila, K., Nazerine, M., and Shaji, S. (2021). in Removal of total hardness using low cost adsorbents, IOP Conference Series: Materials Science and Engineering (United States: IOP Publishing), 012089.
Birniwa, A. H., Mahmud, H. N. M. E., Abdullahi, S. S. a., Habibu, S., Jagaba, A. H., Ibrahim, M. N. M., et al. (2022). Adsorption behavior of methylene blue cationic dye in aqueous solution using polypyrrole-polyethylenimine nano-adsorbent. Polymers 14 (16), 3362. doi:10.3390/polym14163362
Dada, A. O., Latona, D. F., Ojediran, O. J., and Nath, O. O. (2016). Adsorption of Cu (II) onto bamboo supported manganese (BS-Mn) nanocomposite: Effect of operational parameters, kinetic, isotherms, and thermodynamic studies. J. Appl. Sci. Environ. Manag. 20 (2), 409–422. doi:10.4314/jasem.v20i2.24
Das, T. R., and Sharma, P. K. (2020). Bimetal oxide decorated graphene oxide (Gd2O3/Bi2O3@ GO) nanocomposite as an excellent adsorbent in the removal of methyl orange dye. Mater. Sci. Semicond. Process. 105, 104721. doi:10.1016/j.mssp.2019.104721
De Jesús Ruíz-Baltazar, Á., Reyes-López, S. Y., de Lourdes Mondragón-Sánchez, M., Robles-Cortés, A. I., and Pérez, R. (2019). Eco-friendly synthesis of Fe3O4 nanoparticles: Evaluation of their catalytic activity in methylene blue degradation by kinetic adsorption models. Results Phys. 12, 989–995. doi:10.1016/j.rinp.2018.12.037
El-Zahhar, A. A., and Awwad, N. S. (2016). Removal of malachite green dye from aqueous solutions using organically modified hydroxyapatite. J. Environ. Chem. Eng. 4 (1), 633–638. doi:10.1016/j.jece.2015.12.014
Faraji, M., Yamini, Y., and Rezaee, M. (2010). Magnetic nanoparticles: Synthesis, stabilization, functionalization, characterization, and applications. J. Iran. Chem. Soc. 7 (1), 1–37. doi:10.1007/bf03245856
Gómez-Pastora, J., Bringas, E., and Ortiz, I. (2014). Recent progress and future challenges on the use of high performance magnetic nano-adsorbents in environmental applications. Chem. Eng. J. 256, 187–204. doi:10.1016/j.cej.2014.06.119
Hameed, A., Aslam, M., Ismail, I. M., Salah, N., and Fornasiero, P. (2015). Sunlight induced formation of surface Bi2O4− x–Bi2O3 nanocomposite during the photocatalytic mineralization of 2-chloro and 2-nitrophenol. Appl. Catal. B Environ. 163, 444–451. doi:10.1016/j.apcatb.2014.08.029
Homaeigohar, S., Zillohu, A. U., Abdelaziz, R., Hedayati, M. K., and Elbahri, M. (2016). A novel nanohybrid nanofibrous adsorbent for water purification from dye pollutants. Materials 9 (10), 848. doi:10.3390/ma9100848
Hu, H., and Xu, K. (2020). “Physicochemical technologies for HRPs and risk control,” in High-risk pollutants in wastewater (Amsterdam, Netherlands: Elsevier), 169–207.
Hu, X. S., Liang, R., and Sun, G. (2018). Super-adsorbent hydrogel for removal of methylene blue dye from aqueous solution. J. Mater. Chem. A 6 (36), 17612–17624. doi:10.1039/c8ta04722g
Ibrahim, A. A., Salama, R. S., El-Hakam, S. A., Khder, A. S., and Ahmed, A. I. (2021). Synthesis of 12-tungestophosphoric acid supported on Zr/MCM-41 composite with excellent heterogeneous catalyst and promising adsorbent of methylene blue. Colloids Surfaces A Physicochem. Eng. Aspects 631, 127753. doi:10.1016/j.colsurfa.2021.127753
Ishak, Z., and Kumar, D. (2022). Adsorption of methylene blue and reactive black 5 by activated carbon derived from tamarind seeds. Trop. Aquatic Soil Pollut. 2 (1), 1–12. doi:10.53623/tasp.v2i1.26
Kasbaji, M., Mennani, M., Grimi, N., Barba, F. J., Oubenali, M., Simirgiotis, M. J., et al. (2022). Implementation and physico-chemical characterization of new alkali-modified bio-sorbents for cadmium removal from industrial discharges: Adsorption isotherms and kinetic approaches. Process Biochem. 120, 213–226. doi:10.1016/j.procbio.2022.06.010
Khan, F., and Wahab, R. (2022). Utilization of solution grown manganese oxide nanocrystallite to microstructure against bacteria’s inhibition. J. Inorg. Organomet. Polym. Mater. 32 (5), 1650–1667. doi:10.1007/s10904-021-02206-8
Krishnan, A., Ravindran, B., Balasubramanian, B., Swart, H. C., Panchu, S. J., and Prasad, R. (2022). Emerging nanomaterials for advanced technologies. Berlin, Germany: Springer.
Laurent, S., Forge, D., Port, M., Roch, A., Robic, C., Vander Elst, L., et al. (2008). Magnetic iron oxide nanoparticles: Synthesis, stabilization, vectorization, physicochemical characterizations, and biological applications. Chem. Rev. 108 (6), 2064–2110. doi:10.1021/cr068445e
Li, C., Liu, H., Jiang, X., Waterhouse, G. I., Zhang, Z., and Yu, L. (2018). Hierarchical Fe3O4/C with a flower-like morphology: A highly efficient and reusable dye adsorbent. Synth. Met. 246, 45–56. doi:10.1016/j.synthmet.2018.09.010
Li, H., Liu, L., Cui, J., Cui, J., Wang, F., and Zhang, F. (2020). High-efficiency adsorption and regeneration of methylene blue and aniline onto activated carbon from waste edible fungus residue and its possible mechanism. RSC Adv. 10 (24), 14262–14273. doi:10.1039/d0ra01245a
Li, L., Xiao, J., Liu, P., and Yang, G. (2015). Super adsorption capability from amorphousization of metal oxide nanoparticles for dye removal. Sci. Rep. 5, 9028–9036. doi:10.1038/srep09028
Li, N., Wang, J.-Y., Liu, Z.-Q., Guo, Y.-P., Wang, D.-Y., Su, Y.-Z., et al. (2014). One-dimensional ZnO/Mn 3 O 4 core/shell nanorod and nanotube arrays with high supercapacitive performance for electrochemical energy storage. Rsc Adv. 4 (33), 17274–17281. doi:10.1039/c4ra00588k
Li, Y., Lu, H., Wang, Y., Zhao, Y., and Li, X. (2019). Efficient removal of methyl blue from aqueous solution by using poly (4-vinylpyridine)–graphene oxide–Fe3O4 magnetic nanocomposites. J. Mater. Sci. 54, 7603–7616. doi:10.1007/s10853-019-03441-8
Mahmoud, M., and Mahmoud, A. S. (2021). Wastewater treatment using nano bimetallic iron/copper, adsorption isotherm, kinetic studies, and artificial intelligence neural networks. Emergent Mater. 4 (5), 1455–1463. doi:10.1007/s42247-021-00253-y
Mittal, H., Morajkar, P. P., Al Alili, A., and Alhassan, S. M. (2020). In-situ synthesis of ZnO nanoparticles using gum Arabic based hydrogels as a self-template for effective malachite green dye adsorption. J. Polym. Environ. 28 (6), 1637–1653. doi:10.1007/s10924-020-01713-y
Naeem, S., Baheti, V., Militky, J., Wiener, J., Behera, P., and Ashraf, A. (2016). Sorption properties of iron impregnated activated carbon web for removal of methylene blue from aqueous media. Fibers Polym. 17, 1245–1255. doi:10.1007/s12221-016-6423-x
Naseri, K., and Allahverdi, A. (2019). Methylene blue adsorption by TiO2-based nano-adsorbents: Performance evaluation and kinetic study. Res. Chem. Intermed. 45, 4863–4883. doi:10.1007/s11164-019-03866-5
Nayl, A. A., Abd-Elhamid, A. I., Arafa, W. A., Ahmed, I. M., AbdEl-Rahman, A. M., Soliman, H., et al. (2023). A novel P@ SiO2 nano-composite as effective adsorbent to remove methylene blue dye from aqueous media. Materials 16 (2), 514. doi:10.3390/ma16020514
Palansooriya, K. N., Yang, Y., Tsang, Y. F., Sarkar, B., Hou, D., Cao, X., et al. (2020). Occurrence of contaminants in drinking water sources and the potential of biochar for water quality improvement: A review. Crit. Rev. Environ. Sci. Technol. 50 (6), 549–611. doi:10.1080/10643389.2019.1629803
Qamar, M. T., Aslam, M., Rehan, Z., Soomro, M. T., Ahmad, I., Ishaq, M., et al. (2017). MoO3 altered ZnO: A suitable choice for the photocatalytic removal of chloro-acetic acids in natural sunlight exposure. Chem. Eng. J. 330, 322–336. doi:10.1016/j.cej.2017.07.168
Qamar, M. T., Aslam, M., Rehan, Z., Soomro, M. T., Basahi, J. M., Ismail, I. M., et al. (2017). The influence of p-type Mn3O4 nanostructures on the photocatalytic activity of ZnO for the removal of bromo and chlorophenol in natural sunlight exposure. Appl. Catal. B Environ. 201, 105–118. doi:10.1016/j.apcatb.2016.08.004
Rahman, H. L., Erdem, H., Sahin, M., and Erdem, M. (2020). Iron-incorporated activated carbon synthesis from biomass mixture for enhanced arsenic adsorption. Water, Air, & Soil Pollut. 231 (1), 6–17. doi:10.1007/s11270-019-4378-4
Raiyaan, G., Khalith, S., and Sheriff, M. (2021). Bio-adsorption of methylene blue dye using chitosan-extracted from Fenneropenaeus indicus shrimp shell waste. Journal. Aquac. Mar. Biol. 10 (4), 146–150.
Ramutshatsha-Makhwedzha, D., Mavhungu, A., Moropeng, M. L., and Mbaya, R. (2022). Activated carbon derived from waste orange and lemon peels for the adsorption of methyl orange and methylene blue dyes from wastewater. Heliyon 8 (8), e09930. doi:10.1016/j.heliyon.2022.e09930
Rápó, E., and Tonk, S. (2021). Factors affecting synthetic dye adsorption; desorption studies: A review of results from the last five years (2017–2021). Molecules 26 (17), 5419. doi:10.3390/molecules26175419
Shi, H., Li, W., Zhong, L., and Xu, C. (2014). Methylene blue adsorption from aqueous solution by magnetic cellulose/graphene oxide composite: Equilibrium, kinetics, and thermodynamics. Industrial Eng. Chem. Res. 53 (3), 1108–1118. doi:10.1021/ie4027154
Sood, S., Umar, A., Mehta, S. K., and Kansal, S. K. (2015). α-Bi2O3 nanorods: An efficient sunlight active photocatalyst for degradation of Rhodamine B and 2, 4, 6-trichlorophenol. Ceram. Int. 41 (3), 3355–3364. doi:10.1016/j.ceramint.2014.10.038
Tan, I., Ahmad, A. L., and Hameed, B. H. (2008). Adsorption of basic dye using activated carbon prepared from oil palm shell: Batch and fixed bed studies. Desalination 225 (1-3), 13–28. doi:10.1016/j.desal.2007.07.005
Tejada-Tovar, C., Villabona-Ortíz, Á., and Gonzalez-Delgado, Á. D. (2021). Adsorption of azo-anionic dyes in a solution using modified coconut (Cocos nucifera) mesocarp: Kinetic and equilibrium study. Water 13 (10), 1382. doi:10.3390/w13101382
Yagub, M. T., Sen, T. K., Afroze, S., and Ang, H. M. (2014). Dye and its removal from aqueous solution by adsorption: A review. Adv. colloid interface Sci. 209, 172–184. doi:10.1016/j.cis.2014.04.002
Yang, L., Zhang, Y., Liu, X., Jiang, X., Zhang, Z., Zhang, T., et al. (2014). The investigation of synergistic and competitive interaction between dye Congo red and methyl blue on magnetic MnFe2O4. Chem. Eng. J. 246, 88–96. doi:10.1016/j.cej.2014.02.044
Zaharia, C., Suteu, D., Muresan, A., Muresan, R., and Popescu, A. (2009). Textile wastewater treatment by homogenous oxidation with hydrogen peroxide. Environ. Eng. Manag. J. 8 (6), 1359–1369. doi:10.30638/eemj.2009.199
Keywords: adsorption, kinetic models, isotherms, Mn3O4-Bi2O3 composite, methylene blue
Citation: Al-Saeedi SI, Areej A, Qamar MT, Alhujaily A, Iqbal S, Alotaibi MT, Aslam M, Qayyum MA, Bahadur A, Awwad NS, Jazaa Y and Elkaeed EB (2023) Isotherm and kinetic studies for the adsorption of methylene blue onto a novel Mn3O4-Bi2O3 composite and their antifungal performance. Front. Environ. Sci. 11:1156475. doi: 10.3389/fenvs.2023.1156475
Received: 02 February 2023; Accepted: 05 April 2023;
Published: 26 May 2023.
Edited by:
Mohamed Ashour, National Institute of Oceanography and Fisheries (NIOF), EgyptReviewed by:
Ahmed E. Alprol, National Institute of Oceanography and Fisheries (NIOF), EgyptReda S. Salama, Delta University for Science and Technology, Egypt
Copyright © 2023 Al-Saeedi, Areej, Qamar, Alhujaily, Iqbal, Alotaibi, Aslam, Qayyum, Bahadur, Awwad, Jazaa and Elkaeed. This is an open-access article distributed under the terms of the Creative Commons Attribution License (CC BY). The use, distribution or reproduction in other forums is permitted, provided the original author(s) and the copyright owner(s) are credited and that the original publication in this journal is cited, in accordance with accepted academic practice. No use, distribution or reproduction is permitted which does not comply with these terms.
*Correspondence: Shahid Iqbal, c2hhaGlkLmlxYmFsNzA0QHlhaG9vLmNvbQ==; Ali Bahadur, aGRlcmc3NTYxQGdtYWlsLmNvbQ==; Eslam B. Elkaeed, aWthZWVkQG1jc3QuZWR1LnNh