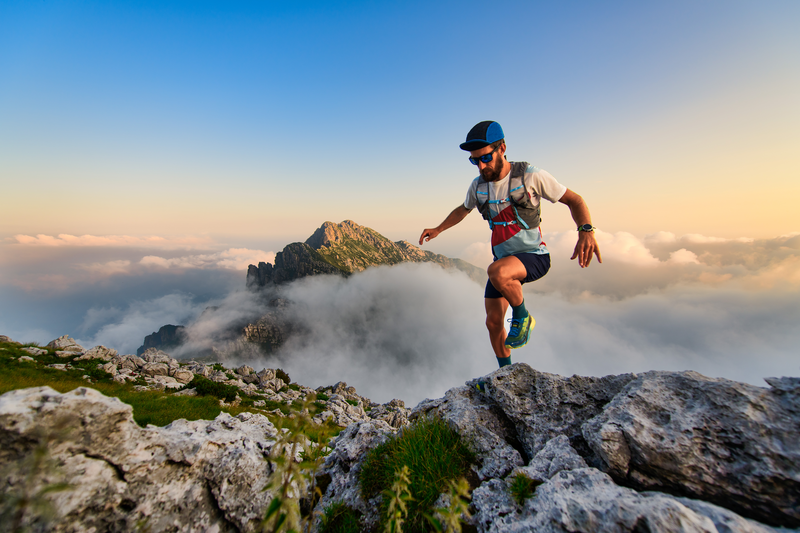
94% of researchers rate our articles as excellent or good
Learn more about the work of our research integrity team to safeguard the quality of each article we publish.
Find out more
REVIEW article
Front. Environ. Sci. , 28 March 2023
Sec. Freshwater Science
Volume 11 - 2023 | https://doi.org/10.3389/fenvs.2023.1151315
This article is part of the Research Topic River Rehabilitation in the Anthropocene View all 7 articles
Rehabilitation of large Anthropocene rivers requires engagement of diverse stakeholders across a broad range of sociopolitical boundaries. Competing objectives often constrain options for ecological restoration of large rivers whereas fewer competing objectives may exist in a subset of tributaries. Further, tributaries contribute toward building a “portfolio” of river ecosystem assets through physical and biological processes that may present opportunities to enhance the resilience of large river fishes. Our goal is to review roles of tributaries in enhancing mainstem large river fish populations. We present case histories from two greatly altered and distinct large-river tributary systems that highlight how tributaries contribute four portfolio assets to support large-river fish populations: 1) habitat diversity, 2) connectivity, 3) ecological asynchrony, and 4) density-dependent processes. Finally, we identify future research directions to advance our understanding of tributary roles and inform conservation actions. In the Missouri River United States, we focus on conservation efforts for the state endangered lake sturgeon, which inhabits large rivers and tributaries in the Midwest and Eastern United States. In the Colorado River, Grand Canyon United States, we focus on conservation efforts for recovery of the federally threatened humpback chub. In the Missouri River, habitat diversity focused on physical habitats such as substrate for reproduction, and deep-water habitats for refuge, whereas augmenting habitat diversity for Colorado River fishes focused on managing populations in tributaries with minimally impaired thermal and flow regimes. Connectivity enhancements in the Missouri River focused on increasing habitat accessibility that may require removal of physical structures like low-head dams; whereas in the Colorado River, the lack of connectivity may benefit native fishes as the disconnection provides refuge from non-native fish predation. Hydrologic variability among tributaries was present in both systems, likely underscoring ecological asynchrony. These case studies also described density dependent processes that could influence success of restoration actions. Although actions to restore populations varied by river system, these examples show that these four portfolio assets can help guide restoration activities across a diverse range of mainstem rivers and their tributaries. Using these assets as a guide, we suggest these can be transferable to other large river-tributary systems.
Over the past few decades, evidence underscoring the roles of biodiversity and complexity in stabilizing ecosystem processes in river networks has grown. For example, the asynchronous dynamics of interconnected populations of sockeye salmon (Oncorhynchus nerka) have led to stable interannual catch rates within Bristol Bay, Alaska (Schindler et al., 2010). Foundational to such “portfolio effects” is environmental heterogeneity across a river network, which supports processes such as fish growth and recruitment by providing habitat mosaics that shift over time and space (Brennan et al., 2019; Humphries et al., 2019). In free-flowing rivers, upstream dynamics are aggregated as they converge in downstream reaches; thus, diversity, asynchrony, and connectivity among low-order streams contribute to the stability of mainstem rivers (Horwitz, 1978; Moore et al., 2015). Further, the size and diversity of networks comprising sub-watersheds may act in concert to enhance biodiversity of riverine fishes at larger spatial and temporal scales (Terui et al., 2021). Such knowledge has underscored calls to consider the broader riverscape in research and management of stream and river fishes (Fausch et al., 2002). These and other research efforts have advanced our understanding of ecological processes and resilience of riverine ecosystems and suggest a link between heterogeneity and stability pertinent to highly modified rivers.
The majority of today’s large rivers have been simplified and fragmented (Grill et al., 2019). Many large rivers now resemble single-threaded deep channels that are disconnected from their floodplain, while other rivers have been stabilized, segmented, and restructured by large dams (Nilsson et al., 2005). As a result, the ecological structure and function of large rivers have become constrained (Peipoch et al., 2015) and increasingly homogenized (Rahel, 2002). Many large rivers have also experienced declines of fluvial specialist or rheophilic species and an increasing dominance of generalist, often non-native, species (Galat and Zweimüller, 2001). In the case of the Central Valley, California, analyses spanning 5 decades indicate the loss of biocomplexity has weakened the buffering capacity of Chinook salmon (O. tshawytscha) in this system from effects of changing conditions (Carlson and Satterthwaite, 2011; Sturrock et al., 2020). High interannual recruitment variability can also occur where portfolio effects are weakened following isolation or degradation of previously interconnected riverine and lake spawning habitats in multi-stock fisheries (DuFour et al., 2015). While restoration in large rivers typically aims to reintroduce lost or degraded habitat conditions and diversify local habitat mosaics within the mainstem river (Gore and Shields, 1995; Stoffers et al., 2022), consideration of portfolio effects may necessitate thinking beyond the mainstem river and the roles of tributaries in maintaining portfolio assets needed to conserve large river fishes.
Multiple and varied definitions exist regarding what constitutes a mainstem and tributary system and much of the consternation depends on spatial scale. For instance, the Missouri River is the longest river in North America and is, for all intents and purposes, a large river. However, the Missouri River is a tributary to the Mississippi River. Paukert and Galat (2010) suggest large rivers are those with a mean annual discharge >100 m3/s, a basin area >217 km2, and a stream-order (Strahler) delineation ≥3 and nearly ubiquitously >5. For the purposes of this manuscript, we focus on basin-defining rivers (e.g., Arkansas, Colorado, Columbia, Mississippi, Missouri, Ohio, Tennessee) as mainstem rivers. These systems are greatly modified to meet multiple objectives such as hydroelectric generation, flood control, irrigation, and navigation (and thus are considered working rivers) with much socio-political resistance to active change in channel form and flow patterns. We consider tributaries to these large systems where hardened infrastructure and socio-political pressures are generally lessened, as possible opportunities for ensuring that a full complement of environmental heterogeneity and ecological processes exist both spatially and temporally along mainstem rivers to support sustainability of fish biodiversity. Admittedly, such a definition of tributaries results in an expansive array of differences in stream size and complexity. The array of tributaries of different sizes and those sustained by varied ecological processes (e.g., groundwater dominated perennial versus runoff-dominated intermittent systems) is an important consideration when evaluating their relative roles in sustaining large river fish populations. Therefore, our goal is to review the roles of tributaries in providing four portfolio assets for large river fish populations using case histories from two distinct large river-tributary systems. Specifically, we highlight the contributions of tributaries to provisioning of 1) habitat diversity, 2) connectivity, 3) ecological asynchrony, and 4) density-dependent processes that may facilitate completion of life stages for fishes inhabiting greatly modified large river systems.
Tributaries may support populations of large river fishes through maintenance and creation of multiple unique habitat conditions. Tributaries provide a suite of supplemental, complementary, and vestige large river habitats to support multiple life stages of fishes (Dunn and Paukert, 2021). Sections of large tributaries can have similar physical structure to mainstem rivers and have relatively high contributing discharge, thus, representing supplemental large river habitats (Pracheil et al., 2013). For example, the federally endangered pallid sturgeon (Scaphirhynchus albus) uses lower reaches of large tributaries (e.g., Platte River and Yellowstone River) of the Missouri River (Bramblett and White, 2001; Hamel et al., 2016). For pelagophilic-spawning fishes that require long distances of free-flowing river for eggs and larvae to develop while in drift (Perkin and Gido, 2011), tributaries with supplemental large river habitats may extend dispersal distance needed by these fishes for recruitment. Tributaries also can provide ecologically unique, complementary habitats to mainstem fish populations. For instance, lithophilic-spawning fishes utilize gravel beds that supply oxygen to developing embryos (Kondolf, 2000), yet coarse substrates are relatively rare in low gradient, sand-dominated mainstem rivers. Tributaries with gravel bars have been associated with greater richness of lithophilic large river specialists (Dunn and Paukert, 2021), indicative that tributaries contribute to mainstem populations of these species. Tributaries also can provide habitat conditions that have been lost in contemporary mainstem rivers (i.e., vestige large river habitats) due to regulation or channelization. For example, alligator gar (Atractosteus spatula) in the regulated Brazos and Arkansas rivers make predictable seasonal movements into free-flowing tributaries to access inundated floodplains as discharge and water temperatures rise (Naus and Adams, 2018; Roberts et al., 2022). In the lower Missouri River, channelization and levees preclude low velocity, shallow habitat conditions within the mainstem; however, the lower reaches of tributaries often exhibit reduced velocities and shallower depths compared to the mainstem (Brown and Coon, 1994). Catch rates of fish near tributary confluences of the Missouri River indicated seasonal use by fishes during early spring and summer, likely related to foraging, refugia, and spawning uses of tributaries (Braaten and Guy, 1999). Similarly, tributaries to the Meuse River serve as recruitment sources for limnophilic species that no longer have suitable spawning habitats within the mainstem (Pollux et al., 2006). Thus, even when mainstem rivers are fragmented or homogenized, free-flowing tributaries can be critical to maintaining overall species diversity (Vasconcelos et al., 2021) and genetic diversity (Ferreira et al., 2022).
Connectivity through active and passive movement among supplemental, complementary, and vestige large river habitats inextricably links mainstem and tributary systems at multiple spatial and temporal scales. Further, such connections may sustain populations of some large river fishes. From a life history perspective, large rivers disproportionally support periodic strategists (Winemiller and Rose, 1992; Winemiller, 2005), which tend to be impacted by fragmentation of river networks by dams, changes in flow regimes, and loss of habitats required for different life history needs more so than species exhibiting equilibrium and opportunistic strategies (Olden et al., 2006) as a result of their migratory behaviors and adaptation to seasonal flow regimes. Thus, we expect many large river species to exhibit population connectivity among mainstems and tributaries. Emerging research indicates widespread use of tributaries by large river fishes across a broad spectrum of life history strategies and a range of large rivers. Specifically, analyses of natal origins indicate both mainstem and tributary recruitment and rearing—and movement between the systems—contribute to mainstem populations of a wide range of species including channel catfish (Ictalurus punctatus; Spurgeon et al., 2018), western silvery minnow (Hybognathus argyritis), flathead chub (Platygobio gracilis; Duncan et al., 2021), humpback chub (Gila cypha; Hayden et al., 2013), and winter-run Chinook salmon (Phillis et al., 2018). Therefore, consideration of migratory behaviors and specific requirements for different life stages, such as spawning substrate, nursery habitats, and overwintering conditions (Galat and Zweimüller, 2001) in addition to life history strategy may be informative of tributary use and the importance of connectivity between mainstem and tributary systems.
Asynchrony leading to stability of population dynamics and maintenance of population abundances through space and time may be an emergent property attributable to differences among groups of tributaries along a mainstem (McCluney et al., 2014). Asynchrony may arise from inherent differences and similarities in the geomorphic, landscape, and latitudinal positioning of tributaries intersecting large rivers. Further, groups of tributaries may provide unique temperature or river discharge regimes that are distinct from the main channel. The spatial configuration of tributaries may aid in stabilizing mainstem fish abundance by offering contrasting thermal regimes that provide optimal temperatures for juvenile rearing (Phillis et al., 2018), serve as refuge for cool-coldwater fluvial species during warm periods (Barrett and Armstrong, 2022; Tingley et al., 2022), offer thermal refugia habitats necessary for overwintering (Moore et al., 2022a), or support reproduction when suitable temperatures are absent downstream of hypolimnetic-release dams (Clarkson and Childs, 2000). Tributaries can also provide refuge from interspecific competition with invasive species that reside in the mainstem (Pfauserova et al., 2021; Yackulic et al., 2021). The spatial configuration of such complementary habitats provided by tributaries along altered mainstem rivers may be especially important in maintaining biodiversity of fishes along riverine networks. Further, localized disturbances such as floods or droughts give rise to temporal asynchrony in flow and temperature among tributaries, which contribute to an increased probability that the necessary environmental conditions for recruitment and survival will occur and maintain population stability. Such asynchronous effects on population stability have been documented among tributaries in headwater watersheds for salmonid populations but may also be present in larger alluvial systems (e.g., Schroeder et al., 2016; Tsuboi et al., 2020).
The size, productivity, and fish assemblage of tributary systems may dictate their capacity to promote portfolio assets for large river fish populations. Tributaries provide energy resources to mainstem environments with the relative influence being driven by a combination of size and density of tributaries as well as the state of alteration in the mainstem. The relative contributions of tributaries to large river fishes in terms of provisioning of habitat as well as energy resources likely fluctuates and is directly linked to the asynchronous dynamics previously discussed. Tributaries could mediate density dependence at specific life stages. For example, numerically rare large river fishes may disperse from mainstems to congregate around key spawning habitats in tributaries, thereby increasing reproduction (potamodromy) or spur movements if densities are too great and resources are limited. Density-dependence in growth, survival, and recruitment can be important in regulating populations of stream fishes, and thus density may be a critical consideration when managing tributary populations of fishes (Grossman and Simon, 2020; Healy et al., 2022a). For example, concentration of fish within a thermal refuge may result in density-dependent food limitations that require individuals to disperse into suboptimal temperatures to find sufficient prey (Brewitt et al., 2017). Food availability and frequent disturbance events may shape tributary fish communities and reduce the strength of density-dependent population regulation. However, the degree to which intraspecific density-dependent processes act on native fish populations in tributaries may also depend on densities of invasive predators that can limit recruitment (Jellyman and McIntosh, 2010).
As our awareness of the role of tributaries in supporting mainstem fish populations and biodiversity across the river network has grown, we assert that enhancing the resilience of native fishes of mainstem rivers in an uncertain future requires the inclusion of tributaries in large river conservation and management efforts. Here we highlight two case studies–lake sturgeon (Acipenser fulvescens) in the lower Missouri River and humpback chub in the lower Colorado River–where ongoing research has advanced our understanding of the role of tributaries in large river fish conservation. Within each case study, we emphasize how tributaries contribute four portfolio assets that help sustain large river fish populations.
The lower Missouri River (Figure 1) has been altered through channelization for commercial navigation and flood control and by basin-wide land conversion for agricultural and urban development (Paukert and Galat, 2010). Restoration of mainstem Missouri River habitats through floodplain reconnection, construction of side-channel chutes, and dike notching have yet to result in the recovery of the full suite of ecological processes and native species (Jacobson and Galat, 2006; Schloesser et al., 2012; Erwin et al., 2017). A growing body of research illustrates the importance of Missouri River tributaries to large river specialists (Dunn et al., 2018) including shovelnose sturgeon (Scaphirhynchus platorynchus; Latka, 1994), and upstream reproduction for American paddlefish (Polyodon spathula) and blue sucker (Cycleptus elongatus; Morey and Berry, 2003; Lallaman, 2012).
FIGURE 1. Study area map for the lake sturgeon case study in lower Missouri River, highlighting the state of Missouri and major tributaries used by lake sturgeon. A diversity of ecoregions (different colored regions) provides unique supplementary and complementary habitats. Specific types of habitats used by lake sturgeon are illustrated by the accompanying photos including (A) low-velocity resting habitat at the Missouri River-Osage River confluence during winter, (B) deep, gravel-dredge pool in Osage River, (C) side channel/backwater in Gasconade River used as stopover habitat on spring migrations, and (D) spawning shoal used by large river fishes for reproduction and for which lake sturgeon were present at time of photo. Photographs by Michael Moore, Missouri Cooperative Fish and Wildlife Unit, University of Missouri.
With its complex lifecycle, perhaps no species better exemplifies the connections between tributaries and mainstem habitats in the lower Missouri River than the lake sturgeon. A reintroduction program for the Missouri State endangered lake sturgeon began in Missouri in 1984 (Todd, 2007), and the species is currently being evaluated for federal listing under the U.S. Endangered Species Act (Fink, 2018). Anglers anecdotally reported encountering lake sturgeon in the Osage and Gasconade rivers dating back to the 1930s. Some biologists began to surmise that remnant lake sturgeon populations may have sought refuge in these large tributaries to the lower Missouri River that provided shelter from commercial fishing pressure and rocky habitats suitable for spawning (Pflieger, 1997; Figure 1D). In 2009, stocking efforts were relocated to tributaries, a shift that emphasized a growing appreciation of the role of these tributaries in lake sturgeon recovery in this portion of the basin. Research on the spatial ecology of lake sturgeon has supported the role tributaries play for lake sturgeon population maintenance. In contrast to the Great Lakes watersheds where several projects have been completed to reconstruct spawning reefs (Fischer et al., 2018), little habitat restoration targeted specifically for lake sturgeon has occurred in lower Missouri River tributaries. The recently acquired knowledge of the Lake Sturgeon’s habitat requirements may present future opportunities for conservation and habitat restoration in this system that we outline below.
Lake sturgeon depend on multiple habitat types including rocky substrates for reproduction, productive feeding habitats, and deep lentic resting refuge habitats during periods of thermal extremes. There exists increasing awareness in the ecological variability among populations and life stages (Kerr et al., 2010; Baril et al., 2018). In Great Lakes populations, spawning or nursery habitat is provided by tributaries and often considered to be the primary bottleneck for lake sturgeon recovery (Daugherty et al., 2009; Collier et al., 2022). However, in the Missouri River drainage, tributaries appear to provide habitat throughout all seasons and throughout the species’ lifecycle. For example, adult and subadult lake sturgeon spent >78% of their time in tributaries (Moore et al., 2022a) and <18% of tributary-stocked juveniles left tributaries to overwinter in the mainstem Missouri River (Moore et al., 2022b). Habitat suitability models indicated that 32% of the area in the lower Osage and 78% of the area in the lower Gasconade rivers provided suitable lake sturgeon spawning habitat (Moore et al., 2021b). However, 74% of tagged adult and subadult lake sturgeon were confined to just a few deep pools that comprised just 3% of the area in the Osage and 2% of the area in the Gasconade rivers in the winter and summer (Moore et al., 2021b). In the Osage and Gasconade rivers, these deep low-velocity refuge habitats could be additional bottlenecks to consider. Historically, these habitats may have occurred in the Missouri River’s floodplain and other off-channel habitats, but these deep, low-velocity habitats now occur mainly in the forms of tributary confluences (Figure 1A), tributary backwater sloughs (Gasconade River during the winter only; Figure 1C), and dredged pools (Osage River only; Figure 1B).
Large in size and possessing poor burst swimming abilities, lake sturgeon are perhaps one of the most challenging species of fish to restore movement past barriers (Thiem et al., 2011). In 1906, Lock and Dam #1 was constructed in the Osage River 19 km upstream of its confluence with the Missouri River in a failed attempt to establish commercial navigation on the river. Ever since, this structure has blocked passage at low river flows for many large river species. Previous telemetry studies suggested that a threshold of the combined discharge from the Missouri River and Osage River gages can be used as an approximate indicator for passability of the structure by paddlefish and lake sturgeon (Lallaman, 2012). Although highest flows typically occur during spring, lake sturgeon have also migrated upstream over the dam during rarer high flow-events during the fall, suggesting that dam passage to access tributary habitats is important during multiple seasons (Moore et al., 2022a). Management options to improve connectivity of mainstem and tributary habitats include managing discharges within the Osage River to overtop Lock and Dam #1 via upstream water releases from Bagnell Dam on the Osage River during important times of the year or through removal of obsolete barriers such as Lock and Dam #1.
Asynchronous life history strategies and environmental conditions may act to stabilize production of large river fishes (Moore et al., 2010). In the case of the Missouri River, a single tributary may not always be enough for lake sturgeon. In the Erie-Huron connector system, Fischer et al. (2018) recommended restoring a diversity of spawning sites within the St. Clair River to buffer lake sturgeon from localized disturbances; however, this principle can extend across multiple tributaries as well. Tributary migrations of lake sturgeon in the Missouri River during the reproductive season are strongly associated with temperature and flow regimes that are affected by hydrologic regulation and climatic patterns (Moore et al., 2022a). Comparison of multivariate indicators of annual hydrologic regimes of five major tributaries to the lower Missouri River (i.e., mean, CV, skewness, kurtosis, AR1 correlation, amplitude, and phase; Archfield et al., 2014) illustrates how among-tributary differences in annual hydrology may increase the value of targeted restoration across tributaries (Figure 2). For example, tributaries fluctuate in their relative similarity to the selected reference hydrograph (i.e., Gasconade River) on an interannual basis, which would suggest potential interannual variability in where the optimal conditions for upstream migration and spawning within tributaries might occur. Telemetry tagged lake sturgeon were detected using multiple tributaries during the same spring season in a repeatable order based on spring warming rates of individual rivers, which may be a way to hedge bets against a single tributary being unsuitable for reproduction in a given year (Moore et al., 2022a).
FIGURE 2. Multivariate indicators of annual hydrologic regime (Archfield et al., 2014) corrected for watershed area of five major tributaries to the lower Missouri River (i.e., mean, CV, skewness, kurtosis, AR1 correlation, amplitude, and phase). The plot illustrates how interannual variability in relatively fine-scale environmental processes can determine which tributaries are most hydrologically similar to the unregulated Gasconade River, that serves as the reference line in this plot.
Replication of habitat assets within and among tributaries is important for positive or negative density dependent effects. In terms of positive density dependence (i.e., overcoming Allee effects or depensation; Courchamp et al., 1999), enhancement efforts must focus on areas that will be utilized by enough individuals for an ecological process to be completed. For example, with regards to lake sturgeon spawning habitats, McAdam et al. (2018) asked the question “If you build it, will they come?” and discovered that spawning had not been documented in nearly half of the restored spawning sites that were assessed (Fischer et al., 2018; McAdam et al., 2018). Additional knowledge of the movement ecology of these fish can be used to inform restoration site selection (Brooks et al., 2017), thereby reframing the question as a management strategy: “they come, so let’s build”. By focusing habitat restoration on improving areas already used by fish, managers can increase the value of existing portfolio assets.
The abundance of seemingly suitable spawning habitat and variable spring migration distances among individuals and across years present challenges for the identification of likely spawning sites in the Gasconade River. Therefore, efforts to add additional spawning habitat in this tributary may be ineffective without a greater understanding of the factors driving migration distance and spawning site choice in this river. Alternatively, restoration in the Osage River may be needed due to its higher and more consistent use by lake sturgeon and greater hydrologic and habitat alteration to prevent it from being a population sink to large migratory fishes. The high densities of lake sturgeon in limited deep pool habitats during periods of thermal maxima may increase their vulnerability to climate change and future angling when physiological stress may be high.
Draining 629,000 km2 of land area in seven western States, the Colorado River is one of the most regulated and over-appropriated of major river systems. More than 15 dams constructed in the last century have the capacity to store approximately seven times the mean annual flow of the Colorado River to meet the agricultural and municipal water needs for more than 30 million people [reviewed in Schmidt, (2007); Dibble et al. (2021)]. Prominent changes in Colorado River hydrology, sediment and thermal regimes are attributable to trans-basin diversions for irrigation and municipal use, reservoir water storage, and hydropower generation (Schmidt, 2010). Since the late 1800s, over 60 invasive fishes have become established. Collectively, these abiotic and biotic stressors led to imperilment and listing of four of the endemic fishes of the Colorado River basin under the ESA (Minckley et al., 2003; Olden et al., 2006).
Our second case study focuses on the population of ESA-listed humpback chub inhabiting the segment of the Colorado River within the Grand Canyon, Arizona, United States (Figure 3). This is the largest remaining population of this long-lived and large-bodied endemic minnow as well as the sole population downstream of Lake Powell (U.S. Fish and Wildlife Service, 2010). Dramatic ecological changes following Glen Canyon Dam construction on the Colorado River in 1964 led to declines in the humpback chub population (Webb et al., 2002; Coggins et al., 2006). The regulated discharge is characterized by elevated baseflow, higher daily fluctuations for hydropower generation, and dampened seasonal and annual peak flows (Figure 4). Hypolimnetic releases from Lake Powell through Glen Canyon Dam transformed this formerly turbid and seasonally-warm river to a clearer and colder river with less seasonality (Figure 5). Invasive salmonids thriving in the post-dam mainstem and several perennial groundwater-fed tributaries remain a primary threat to humpback chub and other native fishes (Yard et al., 2011; Yackulic et al., 2018; Healy et al., 2022a). Warmwater predators such as smallmouth bass (Micropterus dolomieu; Udall and Overpeck, 2017; Dibble et al., 2021; Bruckerhoff et al., 2022) inhabiting adjacent reservoirs are also a concern as they have been responsible for decimating humpback chub and other native fishes in other areas of the Colorado River Basin (e.g., Johnson et al., 2008; U.S. Fish and Wildlife Service, 2010).
FIGURE 3. Study area map for our case study highlighting humpback chub in the lower Colorado River, Grand Canyon National Park, Arizona, United States. Photos show typical (A) mainstem Colorado River habitat, (B) Havasu Creek, (C) the Little Colorado River, and (D) Shinumo Creek. Photographs A, C, D by Brian Healy, National Park Service (NPS), photograph B, Amy Martin, NPS.
FIGURE 4. One hundred years of annual hydrology (daily mean discharge, m3/second) of the Colorado River at Lees Ferry prior to and after Glen Canyon Dam construction in 1964. Data source: U.S. Geological Survey (USGS) gaging station 09380000 (US Geological Survey, 2022a).
FIGURE 5. Daily maximum discharge (m3/s) and daily mean water temperature (°C) from U.S. Geological Survey (USGS) gaging stations on the Colorado River at Lees Ferry row (A), and major Grand Canyon tributaries including the Little Colorado River (B), Havasu Creek (C), and Bright Angel Creek (D; gauge Number 09403000). Horizontal dashed lines on temperature plots indicate a minimum threshold for successful reproduction for humpback chub (Gila cypha) for reference. Data sources: Colorado River, USGS gaging station 09380000 (US Geological Survey, 2022a); Little Colorado River, USGS gaging station 09402300 (US Geological Survey, 2022b); Havasu Creek, USGS gaging station 09404115 (US Geological Survey, 2022c); Bright Angel Creek, USGS gaging station number 09403000 (US Geological Survey, 2022d).
Until recently (after ∼2014), the Grand Canyon humpback chub population has been primarily sustained through reproduction and recruitment in a single tributary with suitable temperatures for reproduction–the Little Colorado River (Coggins et al., 2006). Recovery actions for humpback chub have focused on increasing population redundancy through translocations to additional tributaries outside of the Little Colorado River (Spurgeon et al., 2015a; Healy et al., 2020a), expansion of the population within Little Colorado River (Stone et al., 2020; Yackulic et al., 2021), suppression of invasive fishes to enhance juvenile humpback chub recruitment (Coggins et al., 2011; Healy et al., 2020b), and flow experiments to enhance backwater habitats using Glen Canyon Dam discharge (Dodrill et al., 2015; Healy et al., 2022c). Through evaluations of the efficacy of these actions and extensive research and monitoring conducted in tributaries and the mainstem, broad insights into the population ecology of humpback chub and knowledge of the species’ life history requirements have been gained. We examine the potential role of Grand Canyon tributaries in maintaining portfolio assets for long-term population maintenance of humpback chub.
Humpback chub evolved under temporally and spatially diverse habitat conditions. Reproduction and recruitment in both the recently warming, flow-regulated mainstem (Van Haverbeke et al., 2017; Gilbert et al., 2022), and in tributaries with diverse substrate types, flow regimes, and water chemistries (e.g., high CO2 content; Gorman and Stone, 1999; Healy et al., 2020a; Supplementary Table S1) suggests flexibility in habitat use. For successful egg incubation and somatic growth, spring to summer temperatures exceeding 16°C and 12°C, respectively, are required (Hamman, 1982). Cold temperatures have impeded humpback chub reproduction in the mainstem (Clarkson and Childs, 2000; Yackulic et al., 2014; Yackulic et al., 2018), but recent warming of dam discharge due to declining reservoir surface elevation has led to mainstem reproduction and expansion of humpback chub to western reaches of the Colorado River in the Grand Canyon (Van Haverbeke et al., 2017; Gilbert et al., 2022). Cold and invariable temperatures limiting reproduction (Figure 5) also prolong age at maturity and vulnerability to predation by gape-limited introduced trout (Yard et al., 2011; Yackulic et al., 2014).
Dynamic habitats in tributaries with less-altered flow, temperature, and sediment regimes have been critical to sustaining humpback chub in the Grand Canyon. Tributary flooding is an important driver of population dynamics in the Little Colorado River and other tributaries where translocations have occurred (Van Haverbeke et al., 2013; Spurgeon et al., 2015a; Spurgeon et al., 2015b; Dzul et al., 2016; Healy et al., 2022a). Fine sediment accumulation in years lacking floods in the Little Colorado River (Dean and Topping, 2019) may degrade spawning habitats (clean gravels and cobbles, Gorman and Stone, 1999). For example, minimal young-of-year humpback chub production occurred in the Little Colorado River in the years since 2016, which corresponded to reduced late winter flooding (Dzul, 2021; see Figure 5B). Further, invasive predators of eggs or larval humpback chub may thrive with a lack of extreme flooding (Rogosch et al., 2019). Evidence suggests enhanced feeding opportunities (Behn and Baxter, 2019), resulting in higher growth and survival rates of adult/juvenile humpback chub occur with more frequent summer monsoon-driven floods (Healy et al., 2022a; e.g., summer of 2015; Figure 5C).
Isolated and connected tributaries have sustained the Grand Canyon population of humpback chub in the Anthropocene–future establishment and augmentation of isolated populations through active management may enhance portfolio assets for humpback chub. Given evidence that management of tributary populations can benefit the overall population (Yackulic et al., 2021; Healy et al., 2022a) and the disparity in efficacy of invasive species suppression in tributaries and the mainstem, we underscore the importance of tributaries as potential refuges to maintain humpback chub populations. Under the threat of smallmouth bass invasion of the Colorado River mainstem (Dibble et al., 2021), management of several isolated tributary populations through translocations and invasive species suppression could become a necessary option for long-term sustainability of the Grand Canyon humpback chub population.
In contrast to Missouri River tributaries, connectivity of tributaries to the Colorado River is selectively managed to sustain native fish populations. For example, humpback chub possess life history diversity in that some populations complete their life cycle in short, isolated tributaries (Healy et al., 2022a), whereas other populations (Little Colorado River) rely on both tributary-resident spawners and migrations of adults between the mainstem and tributaries (Yackulic et al., 2014; Dzul et al., 2021). Under Colorado River flow regulation, Havasu and Shinumo creeks are generally isolated from upstream movements of fishes from the mainstem. A reproducing humpback chub population was established in the lower 5.6 km of Havasu Creek (Healy et al., 2022a) and expanded in the upper Little Colorado River through translocations (Yackulic et al., 2021). Translocations to Havasu Creek ceased in 2016 (Healy et al., 2020a), resulting in a population that has persisted despite frequent floods (see Figure 5; Melis et al., 1996) that are associated with increased adult humpback chub growth and survival demonstrating their resilience to catastrophic disturbances (Healy et al., 2022a). Given that gene flow can only occur in a downstream direction from populations isolated above barriers (e.g., Whiteley et al., 2010), occasional augmentation to maintain genetic variation is an important consideration for long-term persistence of tributary humpback chub populations (USFWS 2010).
Translocations were initiated, in part, to establish humpback chub in tributaries isolated from introduced piscivores inhabiting the mainstem (Spurgeon et al., 2015a; Spurgeon et al., 2015b; Healy et al., 2020a). Introduced fishes were represented solely by rainbow trout (O. mykiss) in translocation sites. Rainbow trout are considered less piscivorous and are more effectively suppressed in tributaries (e.g., Healy et al., 2020b) than other invasive predators (i.e., Ictalurids, Centrarchids) inhabiting the mainstem and connected reservoirs that serve as sources for invasions of the Colorado River in Grand Canyon (Mueller, 2005; Coggins et al., 2011; Yackulic et al., 2021).
Longevity and high fecundity are important life history traits of humpback chub, for long-term population persistence in semi-arid or desert streams and rivers subject to stochastic disturbance events (Olden et al., 2006). These traits allow for population stability through periods of poor and optimum conditions for reproduction and recruitment. The contemporary Colorado River and tributaries experience extreme temporal and spatial asynchrony in flow and temperature regimes (Figure 5), and both factors have important influences on humpback chub demographic rates (Yackulic et al., 2014; Spurgeon et al., 2015b; Healy et al., 2022a). Flow variability also regulates populations of important invasive predators and competitors, thereby causing potential indirect effects to humpback chub. For instance, elevated spring snowmelt runoff suppresses recruitment of invasive brown trout (Salmo trutta) in Bright Angel Creek (Healy et al., 2022b), and extreme monsoon flooding appears to disadvantage invasive green sunfish (Lepomis cyanellus) in tributaries (Rogosch et al., 2019).
Monsoon precipitation intensity can be spatially variable, and localized catastrophic events may impact a single tributary during a given season (Griffiths et al., 2004). Tributaries deemed potentially important to humpback chub with minimally altered flow and thermal regimes (relative to the mainstem) include Little Colorado River, and Bright Angel, Shinumo, and Havasu creeks, which all have perennial groundwater-driven baseflow discharge (Tobin et al., 2018) experiencing variable timing, frequencies, and magnitudes of floods (Figure 5). Thus, we expect asynchronized population dynamics among tributaries to stabilize the greater Grand Canyon population, leading to long-term sustainability.
Evidence suggests humpback chub populations are subject to intraspecific density-dependence in demographic rates (Pine et al., 2013; Yackulic et al., 2018; Healy et al., 2022a) – an unexpected finding for a periodic strategist inhabiting streams with frequent disturbance events (Winemiller, 2005; reviewed in Healy et al., 2022a). Carrying capacities of small tributaries slated for translocations outside the Little Colorado River were estimated to be ∼100–2,500 individuals (Pine et al., 2013; see Supplementary Table S1), and low prey density due to travertine deposition (Oberlin et al., 1999) may be a significant factor limiting humpback chub abundance in Havasu Creek and the Little Colorado River. While humpback chub can inhabit relatively small tributaries (Laub et al., 2018), the small population sizes in small tributaries (Pine et al., 2013) may be at higher risk of genetic bottlenecks and stochastic demographic processes leading to extirpation. Thus, potential for intraspecific density dependence within very small tributaries indicates mainstem and tributary populations may need to be jointly managed. Protecting the natural flow regime in tributaries may be particularly important for allochthonous food delivery to alleviate density-dependent constraints on growth and survival in these canyon-bound systems (Behn and Baxter, 2019; Healy et al., 2022a).
Important actions may be needed to maintain long-term tributary-mainstem assets for humpback chub and may include mitigation of factors that limit recruitment (predation by invasive species), management of upland forests to limit the probability of intense ash-laden flooding, consideration of carrying capacities in each tributary if augmentation were to occur (Healy et al., 2022a), and maintenance of population redundancy. For instance, the humpback chub was reclassified from endangered to threatened under the ESA in 2021 following a status review (U.S. Fish and Wildlife Service, 2018; US Department of the Interior, 2021) that recognized the importance of alleviating predation pressures through invasive species suppression, expansion in the range of humpback chub and enhanced population redundancy in Grand Canyon through translocations, as discussed above.
Our two case studies highlight the importance of tributaries to large river fish populations and underscore how tributaries can be integrated into the management of large river fishes. While the lower Missouri River and lower Colorado River have distinct climatic, geologic, hydrologic, biogeographic, and anthropogenic stressors, our review indicates the four portfolio assets are relevant and transferable among river systems. Each asset was represented when considering imperiled-species recovery in both rivers and took on context-dependent meanings due to unique management challenges of each river and the biology of each focal species.
Both the lake sturgeon and humpback chub share similar traits representative of the periodic life history strategy, and therefore, both species face similar threats. For example, dams regulate seasonal environmental cues, interfere with large-scale movements for critical life cycle functions, and alter the distribution and abundance of habitat conditions. Both systems harbor invasive species; however, the greatest concern is for piscivorous invaders in the lower Colorado River that can predate on native leucisids and catostomids, whereas in the Missouri River planktivorous bigheaded carps may have less negative impact on benthic-invertivores like the lake sturgeon than migratory planktivores such as paddlefish and bigmouth buffalo (Ictiobus cyprinellus; Pendleton et al., 2017). Future hydroclimate projections in both of these rivers (Heidari et al., 2020) emphasize the need to understand how the diversity of habitats provided by tributaries can provide population refugia. Water temperatures can approach the upper edge of lake sturgeon thermal tolerance and are believed to be a factor driving their use of deep lentic habitats that are rare in the channelized mainstem river but present in some lower Missouri River tributaries (Moore et al., 2021b). Similarly, drought and warming temperatures are forecasted to amplify the effects of invasive species on humpback chub by increasing interactions between humpback chub and warmwater piscivores such as smallmouth bass in dam tailwater reaches of the lower Colorado River (Dibble et al., 2021). Lake sturgeon are more migratory than humpback chub (Paukert et al., 2006; Moore et al., 2021a), meaning that habitat diversity at broader spatial extents may be needed to sustain lake sturgeon populations. Increasing connectivity of mainstem and tributary habitats is a primary way to meet the habitat diversity requirements for restoration for species like lake sturgeon. In contrast, we illustrated that for species like humpback chub, barriers may help maintain invader-free habitats in isolated tributaries.
Spatiotemporal asynchrony in occurrence of these conditions is likely important to both species. By providing multiple tributaries with suitable habitats for reproduction, lake sturgeon may increase the number of successful spawning events in this recovering population. We noted in both systems that hydrologic conditions can vary greatly on an annual basis during key periods of each species’ life history because of patchy precipitation patterns and differential processes that generate surface flow such as snowmelt in the mainstem Missouri River to intense spates of precipitation such as monsoon rains in the tributaries of the Colorado River. The longevity and high fecundity of both fishes will allow them to take advantage of spatially- and temporarily rare environmental conditions for reproduction or other processes that may have been further reduced due to flow regulation in mainstems. For example, flow pulses in tributaries support lateral connectivity, which is critical to provide pulses of allochthonous resources for humpback chub growth, promote habitat complexity by creating gravel and sand bars in the Colorado River, and ensure access to off-channel backwaters for lake sturgeon to rest and feed.
Finally, density dependent processes were important for recovery of both species. We envision scenarios where habitat restoration actions would be ineffective if they were completed in areas of low-use or low-density especially when there are gaps in our understanding of the factors driving habitat use across river networks. For example, previous research suggests that lake sturgeon may bypass seemingly suitable rocky spawning habitat on upstream tributary migrations and select spawning habitats from multiple tributaries to exploit this spatial variation in habitat suitability within a single season. On the other hand, both species potentially face habitat-induced bottlenecks if solely reliant on individual tributaries. Many Missouri River tributaries are too shallow for year-round use by lake sturgeon and some have just a few suitable deep pool habitats where a majority of fish congregate. Similarly, research on humpback chub indicated that individual tributaries had finite carrying capacities indicating a need for spatial replication among multiple tributaries.
Wholistic, functional flow and habitat restoration in large mainstem rivers often poses difficult challenges. For example, experimental dam operations for ecological restoration on large mainstem rivers may compete with socio-economic or even ecological values and may be prohibitively expensive in terms of changes in water deliveries and lost hydropower generation (Schmidt et al., 1998; reviewed in Olden et al., 2014). Further, options to rehabilitate mainstem river habitat mosaics can be constrained by floodplain land uses and channelization (Kondolf, 2011). In contrast, slight increases and redistribution of seasonal dam discharge volumes to mimic a natural flow regime have been effective in reestablishing native fish communities in a small tributary to the Sacramento River (Kiernan et al., 2012). Tributaries are often less-constrained than mainstem rivers and offer options to enhance portfolio assets through restoring connectivity with mainstem habitats by tributary dam operations, barrier removal or fish passage installation, suppression or eradication of invasive fishes reliant on tributary habitats, protection of natural flow regimes and riparian zones, reintroductions or managed relocations, and spawning or rearing habitat improvements, among others. By observing the portfolio assets that were represented in these case studies, we have summarized several challenges and opportunities for incorporating tributaries into restoration and management of large river ecosystems (Table 1).
TABLE 1. List of opportunities and challenges to including tributaries in conservation and management of large rivers.
Tributaries may offer opportunities to enhance habitat diversity and connectivity of asynchronized environmental conditions in support of large river fish populations. Tributaries may have a lower degree of alteration or have barriers that are smaller and easier to remove to restore fish populations. The intensity of jurisdictional or socio-political conflicts may be lessened in some tributary watersheds relative to larger mainstem segments. There may be fewer competing stakeholders and fewer jurisdictional constraints on restoration options, including those on western state and federally managed lands (e.g., Lawrence et al., 2011; Buktenica et al., 2018). However, fewer stakeholders may mean fewer restoration funding resources or opportunities to leverage resources across agencies. Furthermore, because large river fish populations generally operate at broad spatial extents it may be challenging to gain consensus on a tributary-focused strategy across a river network.
There are some existing programs and policy mechanisms already in place that can be used to advance tributary restoration, particularly where flow regimes have been altered. Conservation measures mandated in Section 7 of the ESA allows biological opinions issued by regulatory agencies to offset dam operational impacts to also include components that may enhance portfolio assets (e.g., translocations, U.S. Department of the Interior, 2016). Further, there are 1,039 active licenses for hydropower facilities that are granted by the Federal Energy Regulatory Commission. Terms in licenses are typical renegotiated every 30–50 years, which presents opportunities to implement minimum releases and seasonal releases to benefit aquatic life (reviewed in Olden et al., 2014). Coordinated operations across dams could help reduce negative ecological effects in regulated river basins (Roy et al., 2018). Many dams are operated by the United States Army Corps of Engineers (USACE) or Bureau of Reclamation for hydropower, water supply, and flood control. The Sustainable Rivers Program (SRP) is a collaborative program between USACE and The Nature Conservancy, founded in 2002, to implement environmental flows that better approximate the magnitude, timing, and duration of natural flow regimes. This program is rapidly growing and as of 2019 there were active projects composed of environmental research and experimental flow modifications at 66 USACE dams in 16 rivers. In 2022, an additional 28 rivers are being examined for project implementation including several large tributaries (e.g., Willamette, Pecos, Bill Williams, Kaskaskia, White/Black/Little, Kansas, Osage, Kanawha, Green, Des Moines, and Iowa rivers).
Other management actions, such as the removal and suppression of invasive competitors and predators, may be more effective in tributaries as compared to mainstem rivers. Invasive suppression may relieve density-dependent effects and allow for native fish population expansion into tributaries that are otherwise limited by biotic interactions with invasive species, thus enhancing connectivity and diversity of habitats accessible to native fishes. Tributary suppression programs for invasive species may effectively target tributaries important for vulnerable life stages (e.g., spawning, rearing) while also reducing predation or competition pressures on resident tributary native fish communities (Rytwinski et al., 2019; Healy et al., 2020b; Altenritter et al., 2022), and potentially creating refuges for native species. Suppression or eradication operations in tributaries are often more feasible and logistically less expensive than in large mainstem habitats where suppression efficiency may be lower, connectivity and reinvasion are more likely (Healy et al., 2022b). The probability of detecting population-level responses to suppression in both native and invasive fish populations while controlling for confounding effects of environmental variation and immigration is higher relative to larger rivers (Coggins et al., 2011; Franssen et al., 2014). For instance, electrofishing capture probability (removal efficiency) for spawning invasive brown trout in a small tributary was found to be 2–3 times that of the capture probability of brown trout in the Colorado River (Healy et al., 2022b).
There may also be barriers for tributary restoration that are not present in mainstems. Few large tributaries in North America are themselves un-impacted by human alterations, and conditions within tributaries can depend on those in mainstems. For example, within the context of dam removal to restore anadromous fishes within tributaries, Roy et al. (2018) found that 90% of river lengths were inaccessible to anadromous species mainly due to mainstem dams farther downstream in the watershed that must be passed before fish could access tributaries. Recognizing interdependencies of tributaries and mainstems, structured decision-making approaches could be useful when choosing where to allocate limited resources to river restoration in mainstems or tributaries (Peterson and Duarte, 2020). For example, consideration of all opportunities for barrier removal across the larger watershed in multi-objective algorithms may reduce the opportunity costs of recovering fishes (Roy et al., 2018).
In some cases, tributaries may fall outside regulatory or authorized boundaries, which may restrict or reduce the priority of tributary actions despite potential benefits. For example, successful translocations of humpback chub have occurred in tributaries; however, these tributaries are outside of critical habitat designation for the species under the ESA (U.S. Fish and Wildlife Service, 2018, 5-year review). Similarly, in the Central Valley of California, some tributaries historically used for spawning and rearing are excluded from critical habitat designations for green sturgeon (Acipenser medirostris; reviewed in Moser et al., 2016; Anderson et al., 2018) and winter-run Chinook salmon (Phillis et al., 2018), respectively. These tributaries may not be afforded the same protections as others designated as critical habitat and thus, may represent lost opportunities to contribute toward species recovery. Other federal programs may also exclude opportunities to restore or enhance tributaries. For example, under the Upper Mississippi River Restoration Program, restoration actions are limited to mainstem and tributary reaches that are considered commercial navigation channels (Bouska et al., 2018), which means tributaries that have not been altered for navigation are outside of the program’s authority.
The smaller and sometimes isolated nature of tributaries may also require active augmentation and management to maintain populations of native fishes. Spatial factors including the distance from a source population and connectivity, or inherent population characteristics (e.g., intrinsic population growth rate) may regulate populations in tributaries following disturbance events that result in high mortality (Gido et al., 2019). To protect vulnerable native fishes from invasion and the effects of predation and competition, tradeoffs between extinction risk and isolation above barriers may need consideration (cf. Fausch et al., 2009). Translocations or managed reintroductions can be used to overcome issues related to reduced dispersal and population isolation due to losses of connectivity in fragmented and dam-regulated systems (Olden et al., 2011). Specifically targeting tributaries to enhance redundancy (e.g., Spurgeon et al., 2015b; Hickerson and Walters, 2019; Healy et al., 2020a) with the four portfolio assets in mind may benefit such selection processes. While tributary augmentation may be less costly than maintenance of ecosystem function and native fish populations through large-scale operations of dams or mainstem invasive species suppression for instance, long-term management of isolated tributary populations may be a difficult commitment for management entities operating on limited budgets and with limited jurisdictional authority.
With an increased understanding of which species use tributaries, why they use them, and what types of tributaries they prefer, we may be able to tackle more complex questions including quantifying the strength of portfolio effects from tributaries in maintaining stability in large river fish populations. Applying portfolio theory as a management tool can improve predictions of fish population responses to rehabilitation actions aimed at enhancing portfolio assets of tributaries. For example, Dufour et al. (2015) applied portfolio theory to identify tributaries of Lake Erie in which conservation and restoration actions would have greatest impact to population stability. Documentation of portfolio effects in large and open mainstem-tributary (riverscape) networks is appreciably difficult given hardships in reliably quantifying population changes using traditional fisheries management assessment strategies. Understanding the potentially spatially and temporally asynchronous reproduction and recruitment dynamics of large river fishes is greatly hindered due—in part—to limited understanding of reproduction and recruitment at the riverscape extent. For example, drivers of mainstem recruitment of endangered fishes in the Grand Canyon, including contributions of tributary emigrants from recently established reproducing populations, is uncertain (Gilbert et al., 2022). Pracheil et al. (2009) found that tributary and mainstem flow combined explained annual paddlefish young of year density in a long-term dataset; however, it is unknown whether tributaries provided complementary reproductive habitats or restorative ecological flows to the heavily regulated Missouri River. Thus, a greater understanding regarding variation in recruitment dynamics across the riverscape and quantification of connectivity within and among habitat patches in tributary and mainstem environments will be critical for informing basic understanding (e.g., pushing forward meta-population theory in linear networks), identifying tributaries that meet important habitat and life history requirements, and informing applied management decisions (e.g., habitat restoration, stocking, and translocation strategies).
Determining which tributaries are most valuable for large river fishes and why is also an area of active research. Large river fishes have been documented using tributaries of varying sizes (Pracheil et al., 2013; Hamel et al., 2016; Laub et al., 2018; Cathcart et al., 2019). Discharge has been suggested as a critical threshold whereby tributaries with greater discharges may support large river assemblages through provisioning of supplemental large river habitats (Pracheil et al., 2013; Laub et al., 2018; Dunn and Paukert, 2021). Alternatively, a competing hypothesis is that tributaries may be increasingly valuable where they are most distinct (i.e., greater available complementary habitats) from their recipient rivers. For example, gravel and cobble substrate dominated tributaries in the Ozark Highland physiographic province within the Missouri River basin may make them more attractive for spawning and year-round residency than sandy and silty prairie tributaries of the Central Plains province (Figure 1). Dunn and Paukert, (2021) found average site-level richness of large-river fishes was higher in a prairie tributary, but an Ozark tributary possessing both supplemental and complementary habitats supported higher river-wide richness of large-river fishes due to additional lithophilic fishes within river sections having complementary habitats in the form of coarse substrates. Thus, the value of a tributary likely depends on the ability of tributaries to provide habitats that fulfill specific life-history functions of mainstem fishes. Small tributaries within floodplain rivers can provide off-channel, low-velocity habitats, Brown and Coon, 1994; Bottcher et al., 2013; Naus and Adams, 2018; Phillis et al., 2018) and vital conduits to backwater habitats (Roberts et al., 2022). Further, small tributaries may contribute to invertebrate and allochthonous inputs to mainstem regulated rivers (Whiting et al., 2014; Sabo et al., 2018; Milner et al., 2019), which can be food limited given extensive alteration (Cross et al., 2013). Identification of tributaries across a gradient of stream size that contribute unique habitat conditions and whether such conditions are supplemental, complementary, or vestige large-river habitats could further refine prioritization of tributaries for rehabilitation.
To date, portfolio effects have been quantified using metrics of stability in population abundance of salmonid populations with distinct stock structure (Moore et al., 2010). Such population structure has not been reliably detected in many large river populations leaving its existence in doubt. However, several alternative assessment methods exist including greater use of mark-recapture (acoustic arrays, centralized information-sharing tools for reporting recaptures or acoustic signals), microchemistry (Rude and Whitledge, 2019), and genetic (Bessert and Orti, 2008; Shi et al., 2021; Ferreira et al., 2022) approaches. For example, Price et al. (2021) applied genetic tools to historical fish scales to detect temporal change in diversity of sockeye salmon populations. Further, as described below, greater coordination between tributary and mainstem monitoring efforts within and among connected basins may improve understanding regarding intra-annual and inter-annual synchronization as well as asynchrony in population dynamics at different spatial and temporal scales.
Active tributary restoration, offers opportunities to learn how large river fish populations respond to enhancing the four portfolio assets. Effects of local restoration actions on ecological communities are likely more detectable in tributaries where rapid dispersal from regional species pools is less likely to mask fish-habitat relationships (Swan and Brown, 2017). Further, tributaries may offer rare opportunities to study the population dynamics of native fishes in the absence of or following removal of invasive species. When there is an intact connection between mainstems and tributaries, detecting post-restoration responses of fish populations may require accounting for confounding effects of environmental variation and coordinated monitoring of both tributary and mainstem that could be coupled with mark-recapture studies (e.g., telemetry, fish tagging). Comprehensive network-wide monitoring such as this may be necessary to demonstrate movement and metapopulation connectivity between mainstem and tributary subpopulations, to understand how tributaries may support stability in fish populations (Jager et al., 2016; Schroeder et al., 2016). For example, juvenile life history diversification was found to stabilize river basin-wide Chinook salmon smolt production through tagging thousands of fish throughout tributaries and the mainstem (Schroeder et al., 2016). Further, Yackulic et al. (2021) assessed the value of contributions of tributary translocations toward offsetting losses to invasive species predation through demographic analyses. By incorporating recaptures and resights of both tributary translocated or in-situ produced individuals from interagency network-wide monitoring, mark-recapture models allowed for tributary fidelity and survival estimates to be calculated, which provided insights into the population-level benefits of translocations (Healy et al., 2022a). Measuring progress of tributary restoration actions toward defined objectives related to portfolio assets may be vital to ensuring cost-effective network-wide restoration efforts.
Finally, a key line of inquiry will be to better unite with other scientific fields to understand the relative roles of tributaries in setting habitat templates at riverscape scales. For example, does the distribution of local habitat diversity at tributary-mainstem confluences along riverscapes influence population and assemblage structure of large river fishes at regional scales (Jones and Schmidt, 2017)? Further, tributaries provide unique habitats for mainstem fishes and affect fish habitats within mainstems. Therefore, tributaries could influence the distribution of refuge habitats that could mitigate impacts of climate change on discharge and temperature (Troia et al., 2019). Alternatively, tributaries may be vulnerable to changes in hydrologic regimes and temperature (Dettinger et al., 2015; reviewed in Lynch et al., 2016) with implications for their relative roles in the portfolio. Thus, understanding interactions between physical and biological processes among tributaries and mainstems could help us anticipate net effects of changing climates on large-river ecosystems.
We contend that tributaries are integral to many large river fish populations by providing habitat diversity, connectivity, asynchronous environmental conditions, and by regulating density-dependence. Conservation actions solely focused on mainstem habitats and fish populations in large river systems have resulted in limited success (Gore and Shields, 1995). A contributing factor to such limited success may be associated with the numerous logistical, infrastructural, bureaucratic/jurisdictional, and societal constraints placed on mainstems. However, another contributing factor could be overlooking contributions of tributaries within large river ecosystems. Successes of two tributary-focused management efforts across distinct river systems demonstrate how tributaries could factor into a broader river restoration strategy. Thus, other efforts to restore fish populations in large mainstem rivers might benefit from active management in tributaries. Recognizing that tributaries contribute a portfolio of assets at varying spatial and temporal scales may be increasingly necessary as further demands are placed on watersheds to meet societal needs.
All authors listed have made a substantial, direct, and intellectual contribution to the work and approved it for publication.
Any use of trade, firm, or product names is for descriptive purposes only and does not imply endorsement by the U.S. Government. KB was supported by the U.S. Army Corps of Engineers Upper Mississippi River Restoration Program. The Iowa Cooperative Fish and Wildlife Research Unit is jointly supported by a cooperative agreement among U.S. Geological Survey, the Iowa Department of Natural Resources, Iowa State University, the U.S. Fish and Wildlife Service, and the Wildlife Management Institute. The Mississippi Cooperative Fish and Wildlife Research Unit is jointly supported by a cooperative agreement among U.S. Geological Survey, the Mississippi Department of Wildlife, Fisheries, and Parks, Mississippi State University, the U.S. Fish and Wildlife Service, and the Wildlife Management Institute. The Nebraska Cooperative Fish and Wildlife Research Unit is jointly supported by a cooperative agreement among U.S. Geological Survey, the Nebraska Game and Parks Commission, the University of Nebraska, the U.S. Fish and Wildlife Service, and the Wildlife Management Institute. The Missouri Cooperative Fish and Wildlife Research Unit is jointly supported by a cooperative agreement among U.S. Geological Survey, Missouri Department of Conservation, the University of Missouri, the U.S. Fish and Wildlife Service, and the Wildlife Management Institute.
The authors appreciate the review and constructive comments by D. Gibson-Reinemer and two anonymous peer-reviewers that improved the manuscript.
The authors declare that the research was conducted in the absence of any commercial or financial relationships that could be construed as a potential conflict of interest.
The handling editor JD declared a past co-authorship with the author KB.
All claims expressed in this article are solely those of the authors and do not necessarily represent those of their affiliated organizations, or those of the publisher, the editors and the reviewers. Any product that may be evaluated in this article, or claim that may be made by its manufacturer, is not guaranteed or endorsed by the publisher.
The Supplementary Material for this article can be found online at: https://www.frontiersin.org/articles/10.3389/fenvs.2023.1151315/full#supplementary-material
Altenritter, M. E., DeBoer, J. A., Maxson, K. A., Casper, A. F., and Lamer, J. T. (2022). Ecosystem responses to aquatic invasive species management: A synthesis of two decades of bigheaded carp suppression in a large river. J. Environ. Manag. 305, 114354. doi:10.1016/j.jenvman.2021.114354
Anderson, J. T., Schumer, G., Anders, P. J., Horvath, K., and Merz, J. E. (2018). Confirmed observation: A North American green sturgeon Acipenser medirostris recorded in the stanislaus river, California. J. Fish Wildl. Manag. 9, 624–630. doi:10.3996/012018-JFWM-006
Archfield, S. A., Kennen, J. G., Carlisle, D. M., and Wolock, D. M. (2014). An objective and parsimonious approach for classifying natural flow regimes at a continental scale. River Res. Appl. 30 (9), 1166–1183. doi:10.1002/rra.2710
Baril, A. M., Buszkiewicz, J. T., Biron, P. M., Phelps, Q. E., and Grant, J. W. (2018). Lake sturgeon (Acipenser fulvescens) spawning habitat: A quantitative review. Can. J. Fish. Aquatic Sci. 75 (6), 925–933. doi:10.1139/cjfas-2017-0100
Barrett, H. S., and Armstrong, J. B. (2022). Move, migrate, or tolerate: Quantifying three tactics for cold-water fish coping with warm summers in a large river. Ecosphere 13, e4095. doi:10.1002/ecs2.4095
Behn, K. E., and Baxter, C. V. (2019). The trophic ecology of a desert river fish assemblage: Influence of season and hydrologic variability. Ecosphere 10 (1), e02583. doi:10.1002/ecs2.2583
Bessert, M. L., and Orti, G. (2008). Genetic effects of habitat fragmentation on blue sucker populations in the upper Missouri River (Cycleptus elongatus Lesueur, 1918). Conserv. Genet. 9, 821–832. doi:10.1007/s10592-007-9401-4
Bottcher, J. L., Walsworth, T. E., Thiede, G. P., Budy, P., and Speas, D. W. (2013). Frequent usage of tributaries by the endangered fishes of the upper Colorado River basin: Observations from the san rafael river, Utah. North Am. J. Fish. Manag. 33 (3), 585–594. doi:10.1080/02755947.2013.785993
Braaten, P. J., and Guy, C. S. (1999). Relations between physicochemical factors and abundance of fishes in tributary confluences of the lower channelized Missouri River. Trans. Am. Fish. Soc. 128, 1213–1221. doi:10.1577/15488659(1999)128<1213:RBPFAA>2.0.CO;2
Bramblett, R. G., and White, R. G. (2001). Habitat use and movement of pallid and shovelnose sturgeon in the Yellowstone and Missouri rivers in Montana and North Dakota. Trans. Am. Fish. Soc. 130, 1006–1025. doi:10.1577/1548-8659(2001)130<1006:HUAMOP>2.0.CO;2
Brennan, S. R., Schindler, D. E., Cline, T. J., Walsworth, T. E., Buck, G., and Fernandez, D. P. (2019). Shifting habitat mosaics and fish production across river basins. Science 364, 783–786. doi:10.1126/science.aav4313
Brewitt, K. S., Danner, E. M., and Moore, J. W. (2017). Hot eats and cool creeks: Juvenile pacific salmonids use mainstem prey while in thermal refuges. Can. J. Fish. Aquatic Sci. 74, 1588–1602. doi:10.1139/cjfas-2016-0395
Brooks, J. L., Boston, C., Doka, S., Gorsky, D., Gustavson, K., Hondorp, D., et al. (2017). Use of fish telemetry in rehabilitation planning, management, and monitoring in areas of concern in the Laurentian Great Lakes. Environ. Manag. 60 (6), 1139–1154. doi:10.1007/s00267-017-0937-x
Brown, D. J., and Coon, T. G. (1994). Abundance and assemblage structure of fish larvae in the lower Missouri River and its tributaries. Trans. Am. Fish. Soc. 123, 718–732. doi:10.1577/1548-8659(1994)123<0718:AAASOF>2.3.CO;2
Bruckerhoff, L. A., Wheeler, K., Dibble, K. L., Mihalevich, B. A., Neilson, B. T., Wang, J., et al. (2022). Water storage decisions and consumptive use may constrain ecosystem management under severe sustained drought. J. Am. Water Resour. Assoc. 58, 654–672. doi:10.1111/1752-1688.13020
Buktenica, M. W., Hering, D. K., Scott, N., Lambert, C., McKee, J., Maletis, E., et al. (2018). A long-term watershed-scale partnership to restore bull trout across federal, state, private, and historic tribal land near Crater Lake National Park, Oregon. Fisheries 43, 183–193. doi:10.1002/fsh.10047
Carlson, S. M., and Satterthwaite, W. H. (2011). Weakened portfolio effect in a collapsed salmon population complex. Can. J. Fish. Aquatic Sci. 68, 1579–1589. doi:10.1139/f2011-084
Cathcart, C. N., McKinstry, M. C., MacKinnon, P. D., and Ruffing, C. M. (2019). A tribute to tributaries: Endangered fish distributions within critical habitat of the san juan river, USA. North Am. J. Fish. Manag. 39 (5), 1015–1025. doi:10.1002/nafm.10335
Clarkson, R. W., and Childs, M. R. (2000). Temperature effects of hypolimnial-release dams on early life stages of Colorado River basin big-river fishes. Copeia 2000 (2), 402–412. doi:10.1643/0045-8511(2000)000[0402:TEOHRD]2.0.CO;2
Coggins, L. G., Pine, W. E., Walters, C. J., van Haverbeke, D. R., Ward, D., and Johnstone, H. C. (2006). Abundance trends and status of the Little Colorado River population of humpback chub. North Am. J. Fish. Manag. 26 (1), 233–245. doi:10.1577/m05-075.1
Coggins, L. G., Yard, M. D., and Pine, W. E. (2011). Nonnative fish control in the Colorado River in Grand canyon, Arizona: An effective program or serendipitous timing? Trans. Am. Fish. Soc. 140, 456–470. doi:10.1080/00028487.2011.572009
Collier, J. J., Chiotti, J. A., Boase, J., Mayer, C. M., Vandergoot, C. S., and Bossenbroek, J. M. (2022). Assessing habitat for lake sturgeon (Acipenser fulvescens) reintroduction to the Maumee River, Ohio using habitat suitability index models. J. Gt. Lakes. Res. 48 (1), 219–228. doi:10.1016/j.jglr.2021.11.006
Courchamp, F., Clutton-Brock, T., and Grenfell, B. (1999). Inverse density dependence and the Allee effect. Trends Ecol. Evol. 14, 405–410. doi:10.1016/S0169-5347(99)01683-3
Cross, W. F., Baxter, C. V., Donner, K. C., Rosi-Marshall, E. J., Kennedy, T. A., Hall, R. O., et al. (2011). Ecosystem ecology meets adaptive management: Food web response to a controlled flood on the Colorado river, glen canyon. Ecol. Appl. 21 (6), 2016–2033. doi:10.1890/10-1719.1
Cross, W. F., Baxter, C. V., Rosi-Marshall, E. J., Hall, R. O., Kennedy, T. A., Donner, K. C., et al. (2013). Food-web dynamics in a large river discontinuum. Ecol. Monogr. 83 (3), 311–337. doi:10.1890/12-1727.1
Daugherty, D. J., Sutton, T. M., and Elliott, R. F. (2009). Suitability modeling of lake sturgeon habitat in five northern lake Michigan tributaries: Implications for population rehabilitation. Restor. Ecol. 17 (2), 245–257. doi:10.1111/j.1526-100X.2008.00368.x
Dean, D. J., and Topping, D. J. (2019). Geomorphic change and biogeomorphic feedbacks in a dryland river: The Little Colorado River, Arizona, USA. Bull. Geol. Soc. Am. 131 (11–12), 1920–1942. doi:10.1130/B35047.1
Dettinger, M. D., Udall, B., and Georgakakos, A. (2015). Western water and climate change. Ecol. Appl. 25, 2069–2093. doi:10.1890/15-0938.1
Dibble, K. L., Yackulic, C. B., Kennedy, T. A., Bestgen, K. R., and Schmidt, J. C. (2021). Water storage decisions will determine the distribution and persistence of imperiled river fishes. Ecol. Appl. 31 (2), e02279. doi:10.1002/eap.2279
Dodrill, M. J., Yackulic, C. B., Gerig, B., Pine, W. E., Korman, J., and Finch, C. (2015). Do management actions to restore rare habitat benefit native fish conservation? Distribution of juvenile native fish among shoreline habitats of the Colorado river. River Res. Appl. 31, 1203–1217. doi:10.1002/rra.2842
DuFour, M. R., May, C. J., Roseman, E. F., Ludsin, S. A., Vandergoot, C. S., Pritt, J. J., et al. (2015). Portfolio theory as a management tool to guide conservation and restoration of multi-stock fish populations. Ecosphere 6, art296–21. doi:10.1890/ES15-00237.1
Duncan, M. B., Bramblett, R. G., and Zale, A. V. (2021). Movements of selected minnows between the lower Yellowstone River and its tributaries. Can. J. Zoology 99, 45–56. doi:10.1139/cjz-2020-0040
Dunn, C. G., Brooke, B. L., Hrabik, R. A., and Paukert, C. P. (2018). Intensive sampling reveals underreported use of great-river tributaries by large-river fishes in Missouri. Southeast. Nat. 17 (3), 512–520. doi:10.1656/058.017.0316
Dunn, C. G., and Paukert, C. P. (2021). Accounting for dispersal and local habitat when evaluating tributary use by riverine fishes. Ecosphere 12 (8), e03711. doi:10.1002/ecs2.3711
Dzul, M. C., Kendall, W. L., Yackulic, C. B., Winkelman, D. L., van Haverbeke, D. R., and Yard, M. (2021). Partial migration and spawning movements of humpback chub in the Little Colorado River are better understood using data from autonomous PIT tag antennas. Can. J. Fish. Aquatic Sci. 78 (8), 1057–1072. doi:10.1139/cjfas-2020-0291
Dzul, M. C. (2021). Life history and population dynamics of humpback chub in the Grand Canyon. Doctoral dissertation (Fort Collins, Colorado: Department of Fish, Wildlife, and Conservation Biology, Colorado State University), 269.
Dzul, M. C., Yackulic, C. B., Stone, D. M., and van Haverbeke, D. R. (2016). Survival, growth, and movement of subadult humpback chub, Gila Cypha, in the Little Colorado River, Arizona. River Res. Appl. 32 (3), 373–382. doi:10.1002/rra.2864
Erwin, S. O., Jacobson, R. B., and Elliott, C. M. (2017). Quantifying habitat benefits of channel reconfigurations on a highly regulated river system, Lower Missouri River, USA. Ecol. Eng. 103, 59–75. doi:10.1016/j.ecoleng.2017.03.004
Fausch, K. D., Rieman, B. E., Dunham, J. B., Young, M. K., and Peterson, D. P. (2009). Invasion versus isolation: Trade-offs in managing native salmonids with barriers to upstream movement. Conserv. Biol. 23, 859–870. doi:10.1111/j.1523-1739.2008.01159.x
Fausch, K. D., Torgersen, C. E., Baxter, C. V., and Li, H. W. (2002). Landscapes to riverscapes: Bridging the gap between research and conservation of stream fishes. BioScience 52, 483–498. doi:10.1641/0006-3568(2002)052[0483:LTRBTG]2.0.CO;2
Ferreira, D. G., Carlsson, J., Galindo, B. A., Frantine-Silva, W., Apolinário-Silva, C., Meschini, J. S., et al. (2022). The role of free-flowing tributary rivers in the maintenance of genetic diversity of a migratory fish species living in a river fragmented by dams. Hydrobiologia 849, 1221–1237. doi:10.1007/s10750-021-04782-9
Fink, M. (2018). Petition to list U.S. populations of lake sturgeon (Acipenser fulvescens) as endangered or threatened under the endangered species act. Center for Biological Diversity. Available at: https://www.biologicaldiversity.org/species/fish/pdfs/Lake-Sturgeon-petition-5-14-18.pdf.
Fischer, J. L., Pritt, J. J., Roseman, E. F., Prichard, C. G., Craig, J. M., Kennedy, G. W., et al. (2018). Lake Sturgeon, Lake Whitefish, and Walleye egg deposition patterns with response to fish spawning substrate restoration in the St. Clair–Detroit River system. Trans. Am. Fish. Soc. 147 (1), 79–93. doi:10.1002/tafs.10016
Franssen, N. R., Davis, J. E., Ryden, D. W., and Gido, K. B. (2014). Fish community responses to mechanical removal of nonnative fishes in a large southwestern river. Fisheries 39, 352–363. doi:10.1080/03632415.2014.924409
Galat, D. L., and Zweimüller, I. (2001). Conserving large-river fishes: Is the highway analogy an appropriate paradigm? J. North Am. Benthol. Soc. 20, 266–279. doi:10.2307/1468321
Gido, K. B., Propst, D. L., Whitney, J. E., Hedden, S. C., Turner, T. F., and Pilger, T. J. (2019). Pockets of resistance: Response of arid-land fish communities to climate, hydrology, and wildfire. Freshw. Biol. 64, 761–777. doi:10.1111/fwb.13260
Gilbert, E. I., Brandenburg, W. H., Barkalow, A. L., Albrecht, B. C., Healy, B. D., Smith, E. C. O., et al. (2022). Systematic larval fish surveys and abiotic correlates characterize extant native fish assemblages reproductive success in the Colorado River Western Arizona. Southwest. Assoc. Nat. 66, 67–76. doi:10.1894/0038-4909-66.1.67
Gore, J. A., and Shields, F. D. (1995). Can large rivers be restored? BioScience 45, 142–152. doi:10.2307/1312553
Gorman, O. T., and Stone, D. M. (1999). Ecology of spawning humpback chub, Gila cypha, in the little Colorado river near Grand canyon, Arizona. Environ. Biol. Fishes 55 (1–2), 115–133. doi:10.1023/A:1007450826743
Griffiths, P. G., Webb, R. H., and Melis, T. S. (2004). Frequency and initiation of debris flows in Grand Canyon, Arizona. J. Geophys. Res. 109 (F4), F04002–F04014. doi:10.1029/2003JF000077
Grill, G., Lehner, B., Thieme, M., Geenen, B., Tickner, D., Antonelli, F., et al. (2019). Mapping the world's free-flowing rivers. Nature 569, 215–221. doi:10.1038/s41586-019-1111-9
Grossman, G. D., and Simon, T. N. (2020). Density-dependent effects on salmonid populations: A review. Ecol. Freshw. Fish 29, 400–418. doi:10.1111/eff.12523
Hamel, M. J., Spurgeon, J. J., Pegg, M. A., Hammen, J. J., and Rugg, M. L. (2016). Hydrologic variability influences local probability of Pallid Sturgeon occurrence in a Missouri River tributary. River Res. Appl. 32, 320–329. doi:10.1002/rra.2850
Hamman, R. L. (1982). Spawning and culture of humpback chub. Progressive Fish Cult. 44 (4), 213–216. doi:10.1577/1548-8659(1982)44[213:SACOHC]2.0.CO
Hayden, T. A., Limburg, K. E., and Pine, W. E. (2013). Using otolith chemistry tags and growth patterns to distinguish movements and provenance of native fish in the Grand Canyon. River Res. Appl. 29, 1318–1329. doi:10.1002/rra.2627
Healy, B. D., Budy, P., Conner, M. M., and Omana Smith, E. C. (2022a). Life and death in a dynamic environment: Invasive trout, floods, and intra-specific drivers of translocated populations. Ecol. Appl. 32, e2635. doi:10.1002/eap.2635
Healy, B. D., Budy, P., Yackulic, C. B., Murphy, B. P., Schelly, R. C., and McKinstry, M. C. (2022b). Exploring metapopulation-scale suppression alternatives for a global invader in a river network experiencing climate change. Conserv. Biol. 37, e13993. doi:10.1111/cobi.13993
Healy, B. D., Omana Smith, E. C., Schelly, R. C., Trammell, M. A., and Nelson, C. B. (2020a). Establishment of a reproducing population of endangered humpback chub through translocations to a Colorado river tributary in Grand canyon, Arizona. North Am. J. Fish. Manag. 40 (1), 278–292. doi:10.1002/nafm.10408
Healy, B. D., Schelly, R. C., Yackulic, C. B., Smith, E. C. O., and Budy, P. (2020b). Remarkable response of native fishes to invasive trout suppression varies with trout density, temperature, and annual hydrology. Can. J. Fish. Aquatic Sci. 77 (9), 1446–1462. doi:10.1139/cjfas-2020-0028
Healy, B. D., Yackulic, C. B., and Schelly, R. C. (2022c). Impeding access to tributary spawning habitat and releasing experimental fall-timed floods increase Brown trout immigration into a dam’s tailwater. Can. J. Fish. Aquatic Sci. 80, 614–627. doi:10.1139/cjfas-2022-0231
Heidari, H., Arabi, M., Warziniack, T., and Kao, S. (2020). Assessing shifts in regional hydroclimatic conditions of U.S. river basins in response to climate change over the 21st century. Earth’s Future 8, e2020EF001657. doi:10.1029/2020EF001657
Hickerson, B. T., and Walters, A. W. (2019). Evaluation of potential translocation sites for an imperiled cyprinid, the hornyhead chub. North Am. J. Fish. Manag. 39, 205–218. doi:10.1002/nafm.10261
Horwitz, R. J. (1978). Temporal variability patterns and the distributional patterns of stream fishes. Ecol. Monogr. 48, 307–321. doi:10.2307/2937233
Humphries, P., King, A., McCasker, N., Keller Koph, R., Stoffels, R., Zampatti, B., et al. (2019). Riverscape recruitment: A conceptual synthesis of drivers of fish recruitment in rivers. Can. J. Fish. Aquatic Sci. 77, 213–225. doi:10.1139/cjfas-2018-0138
Jacobson, R. B., and Galat, D. L. (2006). Flow and form in rehabilitation of large-river ecosystems: An example from the lower Missouri river. Geomorphology 77 (3-4), 249–269. doi:10.1016/j.geomorph.2006.01.014
Jager, H. I., Parsley, M. J., Cech, J. J., McLaughlin, R. L., Forsythe, P. S., Elliott, R. F., et al. (2016). Reconnecting fragmented sturgeon populations in North American rivers. Fisheries 41, 140–148. doi:10.1080/03632415.2015.1132705
Jellyman, P. G., and Mcintosh, A. R. (2010). Recruitment variation in a stream galaxiid fish: Multiple influences on fry dynamics in a heterogeneous environment. Freshw. Biol. 55, 1930–1944. doi:10.1111/j.1365-2427.2010.02427.x
Johnson, B. M., Martinez, P. J., Hawkins, J. A., and Bestgen, K. R. (2008). Ranking predatory threats by nonnative fishes in the Yampa River, Colorado, via bioenergetics modeling. North Am. J. Fish. Manag. 28 (6), 1941–1953. doi:10.1577/M07-199.1
Jones, N. E., and Schmidt, B. J. (2017). Tributary effects in rivers: Interactions of spatial scale, network structure, and landscape characteristics. Can. J. Fish. Aquatic Sci. 74 (4), 503–510. doi:10.1139/cjfas-2015-0493
Kerr, S. J., Davison, M. J., and Funnell, E. (2010). A review of lake sturgeon habitat requirements and strategies to protect and enhance sturgeon habitat. Peterborough, Ontario: Fisheries Policy Section, Biodiversity Branch. Ontario Ministry of Natural Resources, 58.
Kiernan, J. D., Moyle, P. B., and Crain, P. K. (2012). Restoring native fish assemblages to a regulated California stream using the natural flow regime concept. Ecol. Appl. 22, 1472–1482. doi:10.1890/11-0480.1
Kondolf, G. M. (2000). Assessing salmonid spawning gravel quality. Trans. Am. Fish. Soc. 129, 262–281. doi:10.1577/1548-8659(2000)129<0262:ASSGQ>2.0.CO;2
Kondolf, G. M. (2011). “Setting goals in river restoration: When and where can the river “heal itself”,” in Stream restoration in dynamic fluvial systems: Scientific approaches, analyses, and tools, geophysical monograph series 194 (Washington, D.C., United States: American Geophysical Union). doi:10.1029/2010GM00102029
Lallaman, J. (2012). Factors affecting paddlefish reproductive success in the lower Osage River. PhD Dissertation. Columbia, United States: University of Missouri-Columbia.
Latka, D. (1994). Habitat use by shovelnose sturgeon in the channelized Missouri River and selected tributary confluences PhD Dissertation. Ames, United States: Iowa State University.
Laub, B., Thiede, G. P., Macfarlane, W. W., and Budy, P. (2018). Evaluating the conservation potential of tributaries for native fishes in the Upper Colorado River Basin. Fisheries 43 (4), 194–206. doi:10.1002/fsh.10054
Lawrence, D. J., Larson, E. R., Liermann, C. A. R., Mims, M. C., Pool, T. K., and Olden, J. D. (2011). National parks as protected areas for U.S. freshwater fish diversity. Conserv. Lett. 4 (5), 364–371. doi:10.1111/j.1755-263X.2011.00185.x
Lynch, A. J., Myers, B. J. E., Chu, C., Eby, L. A., Falke, J. A., Kovach, R. P., et al. (2016). Climate change effects on North American inland fish populations and assemblages. Fisheries 41, 346–361. doi:10.1080/03632415.2016.1186016
McAdam, S. O., Crossman, J. A., Williamson, C., St-Onge, I., Dion, R., Manny, B. A., et al. (2018). If you build it, will they come? Spawning habitat remediation for sturgeon. J. Appl. Ichthyology 34 (2), 258–278. doi:10.1111/jai.13566
McCluney, K. E., Poff, N. L., Palmer, M. A., Thorp, J. H., Poole, G. C., Williams, B. S., et al. (2014). Riverine macrosystems ecology: Sensitivity, resistance, and resilience of whole river basins with human alterations. Front. Ecol. Environ. 42, 48–58. doi:10.1890/120367
Melis, T. S., Phillips, W. M., Webb, R. H., and Bills, D. J. (1996). When the blue-green waters turn red: Historical flooding in Havasu creek, Arizona. In Water-resources investigations report 96-4059 (Virginia, United States: U.S. Geological Survey). Water-Resources Investigations Report 96–4059.
Melis, T. S., Walter, C. J., and Korman, J. (2015). Surprise and opportunity for learning in Grand canyon: The glen canyon dam adaptive management program. Ecol. Soc. 20 (3), 22. doi:10.5751/es-07621-200322
Melis, T. S. (Editor) (2011). Effects of three high-flow experiments on the Colorado river ecosystem downstream from glen canyon dam, Arizona (Virginia, United States: U.S. Geological Survey Circular), 147.
Milner, V. S., Yarnell, S. M., and Peek, R. A. (2019). The ecological importance of unregulated tributaries to macroinvertebrate diversity and community composition in a regulated river. Hydrobiologia 829, 291–305. doi:10.1007/s10750-018-3840-4
Minckley, W. L., Marsh, P. C., Deacon, J. E., Dowling, T. E., Hedrick, P. W., Matthews, W. J., et al. (2003). A conservation plan for native fishes of the lower Colorado River. BioScience 53 (3), 219–232. doi:10.1641/0006-3568(2003)053[0219:ACPFNF]2.0.CO;2
Moore, J. W., Beakes, M. P., Nesbitt, H. K., Yeakel, J. D., Patterson, D. A., Thompson, L. A., et al. (2015). Emergent stability in a large, free-flowing watershed. Ecology 96, 340–347. doi:10.1890/14-0326.1
Moore, J. W., McClure, M., Rogers, L. A., and Schindler, D. E. (2010). Synchronization and portfolio performance of threatened salmon. Conserv. Lett. 3 (5), 340–348. doi:10.1111/j.1755-263X.2010.00119.x
Moore, M. J., Paukert, C. P., Bonnot, T. W., Brooke, B. L., and Moore, T. L. (2022b). Does where they start affect where they finish? A multimethod investigation of the role of stocking location on survival and dispersal of hatchery-reared Lake Sturgeon in Missouri River tributaries. River Res. Appl. 38 (4), 627–638. doi:10.1002/rra.3925
Moore, M. J., Paukert, C. P., Brooke, B. L., and Moore, T. L. (2022a). Lake sturgeon seasonal movements in regulated and unregulated Missouri River tributaries. Ecohydrology 15, e2362. doi:10.1002/eco.2362
Moore, M. J., Paukert, C. P., and Moore, T. L. (2021a). Effects of latitude, season, and temperature on lake sturgeon movement. North Am. J. Fish. Manag. 41 (4), 916–928. doi:10.1002/nafm.10416
Moore, M. J., Paukert, C. P., Owens, S. P., and Moore, T. L. (2021b). Habitat selection in a southern Lake Sturgeon population: Implications of temporal, spatial, and ontogenetic variation for restoration. Restor. Ecol. 30, e13602. doi:10.1111/rec.13602
Morey, N. M., and Berry, C. R. (2003). Biological characteristics of the blue sucker in the james River and the big sioux river, south Dakota. J. Freshw. Ecol. 18 (1), 33–41. doi:10.1080/02705060.2003.9663949
Moser, M. L., Israel, J. A., Neuman, M., Lindley, S. T., Erickson, D. L., McCovey, B. W., et al. (2016). Biology and life history of green sturgeon (Acipenser medirostris ayres, 1854): State of the science. J. Appl. Ichthyology 32, 67–86. doi:10.1111/jai.13238
Mueller, G. A. (2005). Predatory fish removal and native fish recovery in the Colorado River mainstem: What have we learned? Fisheries 30 (9), 10–19. doi:10.1577/1548-8446(2005)30[10:PFRANF]2.0.CO;2
Naus, C. J., and Adams, S. R. (2018). Fish nursery habitat function of the main channel, floodplain tributaries and oxbow lakes of a medium-sized river. Ecol. Freshw. Fish 27, 4–18. doi:10.1111/eff.12319
Nilsson, C., Reidy, C. A., Dynesius, M., and Revenga, C. (2005). Fragmentation and flow regulation of the world's large river systems. Science 308, 405–408. doi:10.1126/science.1107887
Oberlin, G. E., Shannon, J. P., and Blinn, D. W. (1999). Watershed influence on the macroinvertebrate fauna of ten major tributaries of the Colorado River through Grand Canyon, Arizona. Southwest Nat. 44, 17–30.
Olden, J. D., Kennard, M. J., Lawler, J. J., and Poff, N. L. (2011). Challenges and opportunities in implementing managed relocation for conservation of freshwater species. Conserv. Biol. 25, 40–47. doi:10.1111/j.1523-1739.2010.01557.x
Olden, J. D., Konrad, C. P., Melis, T. S., Kennard, M. J., Freeman, M. C., Mims, M. C., et al. (2014). Are large-scale flow experiments informing the science and management of freshwater ecosystems? Front. Ecol. Environ. 12, 176–185. doi:10.1890/130076
Olden, J. D., Poff, N. L., and Bestgen, K. R. (2006). Life-history strategies predict fish invasions and extirpations in the Colorado River Basin. Ecol. Monogr. 76, 25–40. doi:10.1890/05-0330
Paukert, C. P., Coggins, L. G., and Flaccus, C. E. (2006). Distribution and movement of humpback chub in the Colorado River, Grand Canyon, based on recaptures. Trans. Am. Fish. Soc. 135 (2), 539–544. doi:10.1577/T05-204.1
Paukert, C. P., and Galat, D. L. (2010). “Warmwater rivers,” in Inland fisheries management in North America. Editors Hubert, W. A., and Quist, M. C. (Bethesda, MD: American Fisheries Society), 699–730.
Peipoch, M., Brauns, M., Hauer, F. R., Weitere, M., and Valett, H. M. (2015). Ecological simplification: Human influences on riverscape complexity. BioScience 65, 1057–1065. doi:10.1093/biosci/biv120
Pendleton, R. M., Schwinghamer, C., Solomon, L. E., and Casper, A. (2017). Competition among river planktivores: Are native planktivores still fewer and skinnier in response to the silver carp invasion? Environ. Biol. Fishes 100, 1213–1222. doi:10.1007/s10641-017-0637-7
Perkin, J. S., and Gido, K. B. (2011). Stream fragmentation thresholds for a reproductive guild of Great Plains fishes. Fisheries 36, 371–383. doi:10.1080/03632415.2011.597666
Peterson, J. T., and Duarte, A. (2020). Decision analysis for greater insights into the development and evaluation of Chinook salmon restoration strategies in California’s Central Valley. Restor. Ecol. 28, 1596–1609. doi:10.1111/rec.13244
Pfauserova, N., Slavik, O., Horky, P., Turek, J., and Randak, T. (2021). Spatial distribution of native fish species in tributaries is altered by the dispersal of non-native species from reservoirs. Sci. Total Environ. 755, e143108. doi:10.1016/j.scitotenv.2020.143108
Phillis, C. C., Sturrock, A. M., Johnson, R. C., and Weber, P. K. (2018). Endangered winter-run Chinook salmon rely on diverse rearing habitats in a highly altered landscape. Biol. Conserv. 217, 358–362. doi:10.1016/j.biocon.2017.10.023
Pine, W. E., Healy, B., Smith, E. O., Trammell, M., Speas, D., Valdez, R., et al. (2013). An individual-based model for population viability analysis of humpback chub in Grand Canyon. North Am. J. Fish. Manag. 33 (3), 626–641. doi:10.1080/02755947.2013.788587
Pollux, B. J. A., Korosi, A., Verberk, W. C. E. P., Pollux, P. M. J., and van der Velde, G. (2006). Reproduction, growth, and migration of fishes in a regulated lowland tributary: Potential recruitment to the river Meuse. Hydrobiologia 565, 105–120. doi:10.1007/s10750-005-1908-4
Pracheil, B. M., McIntyre, P. B., and Lyons, J. D. (2013). Enhancing conservation of large-river biodiversity by accounting for tributaries. Front. Ecol. Environ. 11, 124–128. doi:10.1890/120179
Pracheil, B. M., Pegg, M. A., and Mestl, G. E. (2009). Tributaries influence recruitment of fish in large rivers. Ecol. Freshw. Fish 18 (4), 603–609. doi:10.1111/j.1600-0633.2009.00376.x
Price, M. H. H., Moore, J. W., Connors, B. M., Wilson, K. L., and Reynolds, J. D. (2021). Portfolio simplification arising from a century of change in salmon population diversity and artificial production. J. Appl. Ecol. 58, 1365–2664. doi:10.1111/1365-2664.13835
Rahel, F. J. (2002). Homogenization of freshwater faunas. Annu. Rev. Ecol. Syst. 33, 291–315. doi:10.1146/annurev.ecolsys.33.010802.150429
Roberts, H. C., Acre, M. R., Claus, M. P. A., Kappen, F. J., Winemiller, K. O., Daugherty, D. J., et al. (2022). Tributary streams provide migratory fish with access to floodplain habitats in a regulated river: Evidence from alligator gar, Atractosteus spatula. Can. J. Fish. Aquatic Sci. 80, 393–407. doi:10.1139/cjfas-2022-0076
Rogosch, J. S., Tonkin, J. D., Lytle, D. A., Merritt, D. M., Reynolds, L. V., and Olden, J. D. (2019). Increasing drought favors nonnative fishes in a dryland river: Evidence from a multispecies demographic model. Ecosphere 10 (4), 1–19. doi:10.1002/ecs2.2681
Roy, S. G., Uchida, E., de Souza, S. P., Blachly, B., Fox, E., Gardner, K., et al. (2018). A multiscale approach to balance trade-offs among dam infrastructure, river restoration, and cost. Proc. Natl. Acad. Sci. 115 (47), 12069–12074. doi:10.1073/pnas.1807437115
Rude, N. P., and Whitledge, G. W. (2019). Natal environments of age-0 paddlefish in the middle Mississippi River inferred from dentary microchemistry. River Res. Appl. 35, 1554–1562. doi:10.1002/rra.3526
Rytwinski, T., Taylor, J. J., Donaldson, L. A., Britton, J. R., Browne, D. R., Gresswell, R. E., et al. (2019). The effectiveness of non-native fish removal techniques in freshwater ecosystems: A systematic review. Environ. Rev. 27, 71–94. doi:10.1139/er-2018-0049
Sabo, J. L., Caron, M., Doucett, R., Dibble, K. L., Ruhi, A., Marks, J. C., et al. (2018). Pulsed flows, tributary inputs and food-web structure in a highly regulated river. J. Appl. Ecol. 55 (4), 1884–1895. doi:10.1111/1365-2664.13109
Schindler, D. E., Hilborn, R., Chasco, B., Boatright, C. P., Quinn, T. P., Rogers, L. A., et al. (2010). Population diversity and the portfolio effect in an exploited species. Nature 465, 609–612. doi:10.1038/nature09060
Schloesser, J. T., Paukert, C. P., Doyle, W. J., Hill, T. D., Steffensen, K. D., and Travnichek, V. H. (2012). Fish assemblages at engineered and natural channel structures in the lower Missouri river: Implications for modified dike structures. River Res. Appl. 28 (10), 1695–1707. doi:10.1002/rra.1578
Schmidt, J. C. (2007). The Colorado river” in large rivers: Geomorphology and management. Editor A. Gupta (New Jersey, United States: John Wiley & Sons), 183–233.
Schmidt, J. C. (2010). “A watershed perspective of changes in streamflow, sediment supply, and geomorphology of the Colorado River,” in Proceedings of the Colorado River Basin Science and Resource Management Symposium, Scottsdale, AZ. Editors T. S. Melis, J. F. Hamill, G. E. Benett, L. G. Coggins, P. E. Grams, T. A. Kennedyet al. (Reston, VA: U.S. Geological Survey Scientific Investigations Report 2010-5135. U.S. Geological Survey), 51–75.
Schmidt, J. C., Webb, R. H., Valdez, R. A., Marzolf, G. R., and Stevens, L. (1998). Science and values in river restoration in the Grand Canyon. BioScience 48, 735–747. doi:10.2307/1313336
Schroeder, R. K., Whitman, L. D., Cannon, B., and Olmsted, P. (2016). Juvenile life-history diversity and population stability of spring Chinook salmon in the Willamette River basin, Oregon. Can. J. Fish. Aquatic Sci. 73 (6), 921–934. doi:10.1139/cjfas-2015-0314
Shi, Y., Bouska, K. L., McKinney, G. J., Dokai, W., Bartels, A., McPhee, M. V., et al. (2021). Gene flow influences the genomic architecture of local adaptation in six riverine fish species. Mol. Ecol. doi:10.1111/mec.16317
Spurgeon, J. J., Paukert, C. P., Healy, B. D., Kelley, C. A., and Whiting, D. P. (2015a). Can translocated native fishes retain their trophic niche when confronted with a resident invasive? Ecol. Freshw. Fish 24 (3), 456–466. doi:10.1111/eff.12160
Spurgeon, J. J., Paukert, C. P., Healy, B. D., Trammell, M., Speas, D., and Omana Smith, E. (2015b). Translocation of humpback chub into tributary streams of the Colorado river: Implications for conservation of large-river fishes. Trans. Am. Fish. Soc. 144, 502–514. doi:10.1080/00028487.2015.1007165
Spurgeon, J. J., Pegg, M. A., and Halden, N. M. (2018). Mixed-origins of channel catfish in a large-river tributary. Fish. Res. 198, 195–202. doi:10.1016/j.fishres.2017.09.001
Stoffers, T., Buijse, A. D., Geerling, G. W., Jans, L. H., Schoor, M. M., Poos, J. J., et al. (2022). Freshwater fish biodiversity restoration in floodplain rivers requires connectivity and habitat heterogeneity at multiple spatial scales. Sci. Total Environ. 838, e156509. doi:10.1016/j.scitotenv.2022.156509
Stone, D. M., Pillow, M. J., Young, K. L., Van Haverbeke, D. R., and Walters, J. D. (2020). Effects of disparate water temperatures and food bases on humpback chub growth rates within the Little Colorado River, Arizona. North Am. J. Fish. Manag. 40, 475–487. doi:10.1002/nafm.10425
Sturrock, A. M., Carlson, S. M., Wikert, J. D., Heyne, T., Nussle, S., Merz, J. E., et al. (2020). Unnatural selection of salmon life histories in a modified riverscape. Glob. Change Biol. 26, 1235–1247. doi:10.1111/gcb.14896
Swan, C. M., and Brown, B. L. (2017). Metacommunity theory meets restoration: Isolation may mediate how ecological communities respond to stream restoration. Ecol. Appl. 27 (7), 2209–2219. doi:10.1002/eap.1602
Terui, A., Kim, S., Dolph, C. L., Kadoya, T., and Miyazaki, Y. (2021). Emergent dual scaling of riverine biodiversity. Proc. Natl. Acad. Sci. 118, e2105574118. doi:10.1073/pnas.2105574118
Thiem, J. D., Binder, T. R., Dawson, J. W., Dumont, P., Hatin, D., Katopodis, C., et al. (2011). Behaviour and passage success of upriver-migrating lake sturgeon Acipenser fulvescens in a vertical slot fishway on the Richelieu River, Quebec, Canada. Endanger. Species Res. 15 (1), 1–11. doi:10.3354/esr00360
Tingley, R. W., Infante, D. M., Dean, E. M., Schemske, D. W., Cooper, A. R., Ross, J. A., et al. (2022). A landscape approach for identifying potential reestablishment sites for extirpated stream fishes: An example with arctic grayling (Thymallus arcticus) in Michigan. Hydrobiologia 849, 1397–1415. doi:10.1007/s10750-021-04791-8
Tobin, B. W., Springer, A. E., Kreamer, D. K., and Schenk, E. (2018). Review: The distribution, flow, and quality of Grand canyon springs, Arizona (USA). Hydrogeology J. 26 (3), 721–732. doi:10.1007/s10040-017-1688-8
Todd, B. (2007). A plan for recovery of the Lake Sturgeon in Missouri. Jefferson, MO: Missouri Department of Conservation.
Tripp, S. J., Phelps, Q. E., Hupfeld, R. N., Herzog, D. P., Ostendorf, D. E., Moore, T. L., et al. (2019). Sturgeon and paddlefish migration: Evidence to support the need for interjurisdictional management. Fisheries 44 (4), 183–193. doi:10.1002/fsh.10215
Troia, M. J., Kaz, A. L., Niemeyer, J. C., and Giam, X. (2019). Species traits and reduced habitat suitability limit efficacy of climate change refugia in streams. Nat. Ecol. Evol. 3 (9), 1321–1330. doi:10.1038/s41559-019-0970-7
Tsuboi, J., Morita, K., Koseki, Y., Endo, S., Sahashi, G., Kishi, D., et al. (2020). Spatial covariation of fish population vital rates in a stream network. Oikos 129 (6), 924–937. doi:10.1111/oik.07169
Udall, B., and Overpeck, J. (2017). The twenty-first century Colorado River hot drought and implications for the future. Water Resour. Res. 53, 2404–2418. doi:10.1002/2016WR019638
US Geological Survey (2022a). Inventory for the site 09380000 - Colorado River at Lees Ferry, Arizona, USA. US geological Survey - Grand canyon monitoring and research web page. Available from: https://www.gcmrc.gov/discharge_qw_sediment/station/GCDAMP/09380000 (Accessed December 1, 2022).
US Geological Survey (2022b). Inventory for the site 09402300 – little Colorado river near desert view, Arizona, USA. US geological Survey - Grand canyon monitoring and research web page. Available from: https://www.gcmrc.gov/discharge_qw_sediment/station/GCDAMP/09402300 (Accessed December 1, 2022).
US Geological Survey (2022d). Inventory for the site 09403000 – Bright Angel creek near Grand canyon, Arizona, USA. US geological Survey - Grand canyon monitoring and research web page. Available from: https://www.gcmrc.gov/discharge_qw_sediment/station/GCDAMP/09403000 (accessed December 1, 2022).
US Geological Survey (2022c). Inventory for the site 09404115 – Havasu creek above the mouth near supai, Arizona, USA. US geological Survey - Grand canyon monitoring and research web page. Available from: https://www.gcmrc.gov/discharge_qw_sediment/station/GCDAMP/09404115 (accessed December 1, 2022).
U.S. Department of the Interior (2021). Endangered and threatened wildlife and plants; reclassification of the humpback chub from endangered to threatened with a section 4(d) rule. Federal Register. Available at: https://www.federalregister.gov/documents/2021/10/18/2021-20964/endangered-and-threatened-wildlife-and-plants-reclassification-of-the-humpback-chub-from-endangered (accessed December 29, 2022).
U.S. Department of the Interior (2016). Long term and experimental management plan for the Glen Canyon Dam, environmental impact statement and decision notice. Washington D.C: U.S. Department of the Interior, Bureau of Reclamation.
U.S. Fish and Wildlife Service (2010). A genetic management plan for captive and translocated endangered Humpback Chub in the Lower Colorado River Basin. Dexter, New Mexico: Dexter National Fish Hatchery and Technology Center, 43.
U.S. Fish and Wildlife Service (2018). Humpback chub (Gila cypha) 5-year review: Summary and evaluation. Lakewood, Colorado: Mountain-Prairie Region.
Van Haverbeke, D. R., Stone, D. M., Coggins, L. G., and Pillow, M. J. (2013). Long-term monitoring of an endangered desert fish and factors influencing population dynamics. J. Fish Wildl. Manag. 4 (1), 163–177. doi:10.3996/082012-JFWM-071
Van Haverbeke, D. R., Stone, D. M., Dodrill, M. J., Young, K. L., and Michael, J. (2017). Population expansion of humpback chub in Western Grand Canyon and hypothesized mechanisms. Southwest. Nat. 62 (4), 285–292. doi:10.1894/0038-4909-62.4.285
Vasconcelos, L. P., Alves, D. C., da Câmara, L. F., and Hahn, L. (2021). Dams in the Amazon: The importance of maintaining free-flowing tributaries for fish reproduction. Aquatic Conservation-Marine Freshw. Ecosyst. 31, 1106–1116. doi:10.1002/aqc.3465
Webb, R. H., Melis, T. S., and Valdez, R. A. (2002). “Observations of environmental change in Grand canyon, Arizona, tucson, Arizona,” in U.S. geological Survey water-resources investigations report 02-4080 (Reston, VA: U.S. Geological Survey), 41.
Whiteley, A. R., Hastings, K., Wenburg, J. K., Frissell, C. A., Martin, J. C., and Allendorf, F. W. (2010). Genetic variation and effective population size in isolated populations of coastal cutthroat trout. Conserv. Genet. 11, 1929–1943. doi:10.1007/s10592-010-0083-y
Whiting, D. P., Paukert, C. P., Healy, B. D., and Spurgeon, J. J. (2014). Macroinvertebrate prey availability and food web dynamics of nonnative trout in a Colorado River tributary, Grand Canyon. Freshw. Sci. 33 (3), 872–884. doi:10.1086/676915
Winemiller, K. O. (2005). Life history strategies, population regulation, and implications for fisheries management. Can. J. Fish. Aquatic Sci. 62, 872–885. doi:10.1139/f05-040
Winemiller, K. O., and Rose, K. A. (1992). Patterns of life-history diversification in North American fishes: Implications for population regulation. Can. J. Fish. Aquatic Sci. 49, 2196–2218. doi:10.1139/f92-242
Yackulic, C. B., Korman, J., Yard, M. D., and Dzul, M. (2018). Inferring species interactions through joint mark–recapture analysis. Ecology 99 (4), 812–821. doi:10.1002/ecy.2166
Yackulic, C. B., van Haverbeke, D. R., Dzul, M., Bair, L., and Young, K. L. (2021). Assessing the population impacts and cost-effectiveness of a conservation translocation. J. Appl. Ecol. 58 (8), 1602–1612. doi:10.1111/1365-2664.13908
Yackulic, C. B., Yard, M. D., Korman, J., and Van Haverbeke, D. R. (2014). A quantitative life history of endangered humpback chub that spawn in the Little Colorado River: Variation in movement, growth, and survival. Ecol. Evol. 4 (7), 1006–1018. doi:10.1002/ece3.990
Yard, M. D., Coggins, L. G., Baxter, C. V., Bennett, G. E., and Korman, J. (2011). Trout piscivory in the Colorado river, Grand canyon: Effects of turbidity, temperature, and fish prey availability. Trans. Am. Fish. Soc. 140 (2), 471–486. doi:10.1080/00028487.2011.572011
Keywords: populations, habitat diversity, portfolio theory, fish management, river rehabilitation
Citation: Bouska KL, Healy BD, Moore MJ, Dunn CG, Spurgeon JJ and Paukert CP (2023) Diverse portfolios: Investing in tributaries for restoration of large river fishes in the Anthropocene. Front. Environ. Sci. 11:1151315. doi: 10.3389/fenvs.2023.1151315
Received: 25 January 2023; Accepted: 20 March 2023;
Published: 28 March 2023.
Edited by:
Jason DeBoer, Illinois Natural History Survey, United StatesReviewed by:
Sarah Yarnell, University of California, Davis, United StatesCopyright © 2023 Bouska, Healy, Moore, Dunn, Spurgeon and Paukert. This is an open-access article distributed under the terms of the Creative Commons Attribution License (CC BY). The use, distribution or reproduction in other forums is permitted, provided the original author(s) and the copyright owner(s) are credited and that the original publication in this journal is cited, in accordance with accepted academic practice. No use, distribution or reproduction is permitted which does not comply with these terms.
*Correspondence: Craig P. Paukert, cGF1a2VydGNAbWlzc291cmkuZWR1
Disclaimer: All claims expressed in this article are solely those of the authors and do not necessarily represent those of their affiliated organizations, or those of the publisher, the editors and the reviewers. Any product that may be evaluated in this article or claim that may be made by its manufacturer is not guaranteed or endorsed by the publisher.
Research integrity at Frontiers
Learn more about the work of our research integrity team to safeguard the quality of each article we publish.