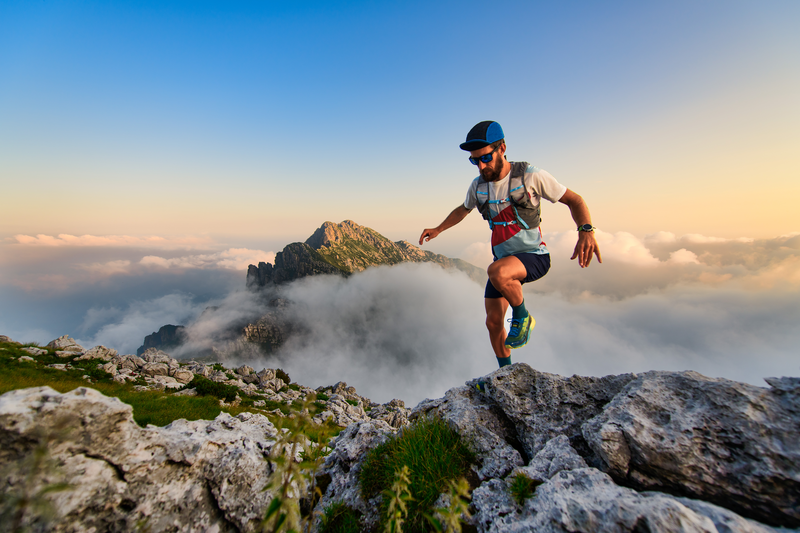
94% of researchers rate our articles as excellent or good
Learn more about the work of our research integrity team to safeguard the quality of each article we publish.
Find out more
ORIGINAL RESEARCH article
Front. Environ. Sci. , 05 May 2023
Sec. Toxicology, Pollution and the Environment
Volume 11 - 2023 | https://doi.org/10.3389/fenvs.2023.1148360
This article is part of the Research Topic Emerging Pollutants in the Environment: Impact and Challenges for Agriculture and Nutritional Security View all 8 articles
Heavy metal contamination in aquaculture farm sediment may create a major risk to ecosystem and human health via the food web as a result of poor handling, incorrect waste management, and extensive use of artificial feed to increase yield and make profit. In this study, heavy metals (e.g., Cd, Cr, Pb, Cu, Zn, Mn, and Ni) were analyzed to examine the accumulation, sources, and contamination levels from the bottom sediment samples in 12 different commercial aquaculture farms from a South Asian coastal area using atomic absorption spectrometer (AAS). The findings revealed that the mean concentrations of heavy metals (µg/g) followed the decreasing order of Mn (48.75 ± 6.75) > Zn (7.09 ± 2.61) > Ni (6.85 ± 2.08) > Cr (5.01 ± 1.04) > Cu (3.00 ± 0.54) > Pb (2.77 ± 0.80) > Cd (0.22 ± 0.07). Contamination factor (CF < 1) and geo-accumulation index (Igeo < 0) for all metals implying the unpolluted status of fish farms by heavy metals. The mean pollution load index (PLI) was found 0.11 refereeing to very low level of contamination. Positive significant correlations (p < 0.05) between Cu-Cr (r = 0.65), Mn-Cd (r = 0.594), Zn-Pb (r = 0.591), Zn-Cu (r = 0.586) and Zn-Cr (r = 0.757) indicated their similar sources of origin. Multivariate analysis like cluster analyses (CA) and principal component analysis (PCA) specified that Cr, Cu, and Zn might have anthropogenic sources. Therefore, future research is recommended to determine element mobility from the feed to pond sediment and fish, and thereby reduce environmental risk to ecosystem and human health.
The need for animal source protein has increased significantly over the past few decades, and aquaculture plays a vital part in meeting that demand in a sustainable way by generating 62.5% of the world’s total fish production (FAO, 2020). With an annual production of 2.58 million metric tons in 2019–20, aquaculture in Bangladesh plays a significant role in maintaining food security for a large population (Hernandez et al., 2018). As a result, this emerging nation is the fifth-largest producer of inland aquaculture in the world (Department of Fisheries, 2020). Over the past few decades, aquaculture in Bangladesh has expanded, become more diverse, and achieved technological excellence, contributing 3.52% of the country’s overall and agricultural GDP (Gross Domestic Product). Commercial feed, agrochemicals, and other products are frequently used to improve natural productivity, manage aquatic creature health, prepare ponds, regulate water and soil quality, and stimulate growth (Faruk et al., 2008). Although feed and medications aid in boosting aquaculture production, contaminated ingredients have a negative impact on the fish, its environment, and even the consumers.
In the environment, heavy metals contamination becomes concentrated as a consequence of human caused activities. Many undesirable elements such as heavy metals, microplastics can come from the manufacturing process, agrochemicals, medical and domestic wastes (Khan et al., 2019). In addition, the sediments of aquatic ecosystems are contaminated by the buildup of heavy metals from a variety of sources, such as the disposal of high-metal wastes, land application of fertilizers, animal manures, sewage sludge, pesticides, and waste water irrigation (Zhang et al., 2010). Further, mining and industrial waste, lead acid batteries, vehicle emission, fertilizers, paints and treated woods are most known anthropogenic sources of heavy metals. The well-known possible natural sources of heavy metals are volcanic activity, forest fires, rock erosion, etc. A number of heavy metals like Fe, Co, Cu, Mn, Zn, etc. are essential for humans. However, extreme levels can be harmful to the organism. Hg, Ni, Cd, Cr, and Pb are highly toxic heavy metals. When these heavy metals build up in an animal’s body over time, it can result in serious health problems (Singh et al., 2011).
Previous research has shown that fish and shellfish from commercial aquaculture farms are contaminated with various levels of heavy metals (Hossain et al., 2022; Sultana et al., 2022; Mohiuddin et al., 2022). Metals can concentrate into fish organs through bioaccumulation and transfer to the consumer through food chains. Bio-magnification of highly toxic metals can inversely affect any essential biological functions (López-Alonso, 2012; NRC, 2005). Animal wastes such as pig, chicken, and cow excrement are used as food in aquaculture ponds. These wastes, which are delivered to fish ponds either as solids or semi-solids, contain higher levels of Cu, As, and Zn, and if they are frequently used as fish feed in the pond, they can result in a reasonable buildup of these metals over a longer period of time in the sediment (Basta et al., 2005). Due to changes in the surrounding water’s environmental factors (temperature, pH, redox potential, and salinity), these toxic metals are released back into the aqueous stage (Soares et al., 1999). Additionally, increased concentrations of heavy metals, particularly in sediments, may be a symptom of perturbations brought on by humans (Eja et al., 2003). Sediments that enter water bodies trap hydrophobic chemical contaminants and slowly release the toxin back into the water column (Chapman, 1996; McCready et al., 2006). Since sediments serve as a vital link between chemical and biological processes and safeguard human health, it is crucial to maintain a healthy aquatic ecosystem in order to guarantee good sediment quality.
Noakhali and Lakshmipur districts are located in the central coastal region of Bangladesh along the northern Bay of Bengal. The area consists of extensive flat, coastal and deltaic land of the Meghna river delta. In that region, there are about 5 million people, most of whom involved in agriculture, aquaculture, and fishing. Aquaculture is promoted in coastal regions due to greater productivity and a salinity intrusion issue in the soil. Due to this, extensive production systems for coastal aquaculture in the region began to emerge in the early 1970s. By the end of 2000, this mode of culture had steadily evolved into a semi-intensive one. However, changing the culture system necessitated a lot of human involvement, such as the use of artificial feed, fertilizers, and chemicals. Once more, rapid industrial development and population growth have increased waste discharge into aquatic environments, which has significantly increased pollution level, including metal contamination. According to several studies (Hossain et al., 2022; Sultana et al., 2022; Mohiuddin et al., 2022), excessive metals were present in commercial fish from Bangladesh. These studies also suggested that the distribution of metal in various fish tissues was largely influenced by the metal load in water, sediment, and feed. As per a recent study by Sarker et al. (2016), fish feed, which is made from contaminated ingredients, is one of the sources of metal contamination in aquaculture farms. Despite the fact that fish is one of the major sources of animal protein in coastal areas (Saha et al., 2016) higher consumption rates of metal-contaminated fish may result in higher health risks for people. Bangladesh has a number of laws in place (such as the Bangladesh Constitution) to make sure that health and food safety guidelines are adhered to. Sediments play a part in the transfer of pollutants and nutrients to living or cultured organisms in aquatic habitats. In order to evaluate the danger to aquatic ecosystems and animals, it is essential to quantify the concentration of heavy metals in sediment. To the best of our knowledge, only one comprehensive investigation has been conducted in the central coastal area of Bangladesh regarding metal pollution in the sediment of aquaculture ponds (Das et al., 2017). Therefore, releasing the above fact, this study was conducted in the central coastal area to estimate heavy metal (Cd, Cr, Pb, Cu, Zn, Mn, and Ni) concentration, and to evaluate their pollution level along with their potential ecological risk and sources in surface sediments in the aquaculture ponds of central coastal area of Bangladesh.
Present study was conducted in two central coastal districts of Bangladesh: Noakhali and Lakshmipur (Figure 1) which are the catchment area of the Meghna River estuary, Bangladesh’s biggest estuarine ecosystem. The studied area contains upper and lower tidal channels of Meghna River. The Bay of Bengal is exposed to the Noakhali districts’ southern portion and Meghna River is situated on the western side of Lakshmipur. The studied area have an average annual rainfall of 2,980 mm, whereas mean temperatures range from 12°C (December–February) to 34°C (April–June) (Bangladesh Meteorogical Department, 2016). Based on the Köppen-Geiger climate classification, this sort of environment is referred to as Am (tropical monsoon climate). The monsoon (June–October) is characterized by southeast monsoon winds with cloud cover, humidity, heavy rainfall, thunderstorms, cyclones, as well as periodic storm surges. This area is inundated occasionally by the incoming wave from the Bay of Bengal. Meghna River, and Dakatia River are the main riverine system on the studied areas.
Since Noakhali and Lakshmipur districts of Bangladesh contribute significantly to the output of fresh water aquaculture, 12 aquaculture ponds in these districts were chosen for assessing heavy metal contamination (Figure 1). Based on a preliminary survey to identify and locate low, medium, and severely impacted sites, these sites were selected (known to be polluted from domestic, agricultural, and industrial effluents including fish and cattle farming, erosion, sewage, landfills, fertilizer factories, etc.). A total of 36 surficial sediment (0–10 cm) samples were collected from the selected commercial fish farms where commercial feed were extensively used (Table 1). An Ekman dredge (stainless steel grab sampler) was used to collect sediment samples during September to November 2018 using. 5% of HNO3 was added to the samples immediately after collecting it to avoid the decay of heavy metals.
For sample preparation, processing, digestion and elemental analyses, the protocol described in Hossain et al. (2021) was followed. Briefly, samples were ground up in an agar mortar and dried at 50°C (for 24 h) prior to laboratory examination. The preferred amount of 10–20 g was used to weigh the samples in a silica dish, and then dried at 120°C in an oven. Then, after placing all of the measured samples in a muffle furnace and gradually raising the temperature from 450°C, 1 g of ground sediment was removed, digested with 10 mL of nitric acid, and filtered through Whatman No. 44 filter paper in a 50 mL chemical flask. Atomic absorption spectroscopy was used to identify the metal concentration after the residue had been removed. A metal standard solution was also prepared for calibrating the system. However, to do the analysis, initially the AAS (Model-iCE 3,300, Thermo Scientific, Designed in United Kingdom, Made in China) was settled along with flame condition. Thereafter, sample blank, sediment samples, standards and deionized water were aspirated into the flame in AAS. Least square method of fitting a straight line was used to analyze the metals statistically. To explore the data more reliable and accurate, a blank reading was also emerged. During the calculation, all necessary corrections were made of individual elements. For avoiding any contamination, all precautions have been taken. Limit of detection and certified reference values mentioned in the user manual were used.
In order to show the variations in concentrations of heavy metals, One-way analysis of variance (ANOVA) was implied using PAST (version 3.0) statistical software. Data normality test was conducted before ANOVA. The graphical presentation of heavy metals and various risk indices were performed using Microsoft Excel 2016, PAST, SPSS (version 25.0), and Origin. Cluster analysis (CA), principal component analysis (PCA), and Pearson rank correlation matrix were used on the original data set to identify the potential sources using PAST software. Moreover, ArcGIS (version 10.3) was used to create the study area map.
Several indices, e.g., contamination factor (CF), pollution load index (PLI), geo-accumulation index (Igeo), enrichment factor (EF), and potential ecological risk index (PERI) were measured to evaluate the current contamination status of sediment of farms (Table 2).
The mean concentrations of the studied heavy metals in the surficial sediment from 12 aquaculture farms of two coastal districts followed the decreasing order of Mn (48.75 ± 6.75 μg/g) > Zn (7.09 ± 2.61 μg/g) > Ni (6.85 ± 2.08 μg/g) > Cr (5.01 ± 1.04 μg/g) > Cu (3.00 ± 0.54 μg/g) > Pb (2.77 ± 0.80 μg/g) > Cd (0.22 ± 0.07 μg/g) (Figure 2A). In comparison, farms in Noakhali had a greater mean load of Mn (51.27 ± 5.10), Ni (6.90 ± 2.37), Cr (5.20 ± 1.08), Cu (3.08 ± 0.69), Pb (2.96 ± 1.09), and Cd (0.27 ± 0.03) but farms in Lakshmipur had a higher load of Zn (7.32 ± 2.67) (Figure 2B). No significant difference (p > 0.05) was found in metal concentration between Noakhali and Lakshmipur district except Cd (F = 10.91, p = 0.00797), but the concentration of essential metals (Cu, Mn, Zn) and non-essential metals (Pb, Cd, Cr and Ni) differed highly and significantly (F = 26.12, p < 0.01).
FIGURE 2. Metal concentrations in surficial sediments in fish farms of Noakhali and Lakshmipur districts; (A) mean metal concentration; (B) metal load variations; (C) metal contents in different sampling sites.
In Noakhali region, sampling site S1 had the highest concentrations of Mn (58.49 μg/g), Ni (9.58 μg/g) and Cd (0.31 μg/g), whereas S4 and S5 had maximum concentration of Pb (4.68 μg/g), Zn (11.7 μg/g), and Cr (6.6 μg/g), Cu (4 μg/g), respectively (Figure 2C). S1 and S4 sampling sites were very close to the mouth of Meghna River estuary. Hence, the metals in those sites might be attributed from the river discharge. S5 site, on the other hand, is located in close proximity to the city; thus, increased concentrations of Cr and Cu in that site could be caused by surface runoff from residential waste and small-scale industry effluents. In Lakshmipur, maximum concentrations of Zn (10.35 μg/g), Cu (3.15 μg/g), and Pb (2.97 μg/g) were documented in S11 which could be originated mainly from industrial activities. The highest concentrations of Mn and Cd were at S7 (56.59 μg/g for Mn and 0.3 μg/g for Cd), whereas S8 and S12 had the abundant concentrations of Cr (6.17 μg/g), and Ni (10.11 μg/g), respectively (Figure 2C). As S7 and S8 sampling sites were near the Meghna River, thus, these sites may receive noticeable amount of metals from river discharges. However, the sampling sites did not differ statistically in both Noakhali (p < 0.05) and Lakshmipur (p < 0.05), respectively.
The findings of the present study were compared with the other scientific studies conducted on the surficial metal values from Bangladesh and the recommended values (Table 3; Table 4). Except for Cr and Mn, the concentration of metal in surficial sediments was found to be lower in this study than in the other investigated sites (Sarker et al., 2016; Das et al., 2017; Rahman et al., 2019; Dietrich and Ayers, 2021). Rahman et al. (2021) recorded Cr as 2.7 μg/g in the sediment of mangrove forest, the Sundarban and Islam et al. (2018) revealed higher Mn (37.85 μg/g) in the surficial sediment of Feni River than this study. Further, all the documented values in this study were far below the shale value (Turekian and Wedepohl, 1961) and other sediment quality standards (MacDonald et al., 2000; NOAA, 2012). Nevertheless, save for embankment collapses or tidal river management, aquaculture ponds get negligible silt deposition upon excavation, which may account for reduced metal concentrations in the aquaculture ponds (Bomer et al., 2020). The concentration of Cd and Cu in aquaculture pond sediments may be partly introduced to anthropogenic inputs into ponds, e.g., commercial fish feed, application of fertilizers, pesticides, and agrochemicals (Paul and Vogl, 2011). Dietrich and Ayers, (2021) analyzed fish feed from Bangladesh and found Cr, Cu, Se, Ni, and Sb whereas Islam et al. (2017) reported Cd and Co in commercial fish feed. However, if fish feed is to blame for the metal loading in pond sediment, it could be due to leaching into the water column and subsequent sorption to sediment, as farmers in Bangladesh meticulously measure out their fish meal to avoid excessive solid waste. As a result, additional research on metal leaching from fish diet is required to evaluate element mobility from feed to pond sediment.
TABLE 4. Comparison of heavy metal concentrations (µg/g) in sediments samples of the aquaculture ponds in central coastal region with other surficial sediments in Bangladesh and standard values (BDL means below detection level and NA means not assessed).
In this study, by using Eq. (2), CF value was calculated, and found that the mean CF values for all examined metals (Cd, Cr, Pb, Cu, Zn, Mn, and Ni) were below 1, indicating a low contamination rate (Figure 3). The average CF values of all heavy metals followed the decreasing order as Cd > Pb > Ni > Zn > Cu > Mn > Cr.
FIGURE 3. Contamination factor (CF) of selected metals in the sediment of Noakhali and Lakshmipur fish farms.
Generally, an assessment of metal concentration in sediments along with background reference values is used to evaluate metal enrichment (Tuna et al., 2007). Mean enrichment factor (EF) values are presented in Figure 4. Further, mean EF values Cd, Pb, Ni, Zn, Cu, Mn, and Cr were refereed in the respective regions. In Noakhali, the highest and lowest EF values ranged from 27.60 to 20.74 and 32.51 and 20.83 were in Lakshmipur. According to EF standards, the results of Cd emerged from moderate (7.25) at S12 to severe (18.93) at S6. Anthropogenic actions play vital role in order to accumulation and enrichment of Mn. This investigation showed that total EF values followed the order of Cd (12.94) > Pb (4.08) > Ni (1.76) > Zn (1.34) > Cu (1.18) > Mn (1.00) > Cr (0.99). The large EF values at the site S3 for Cd (12.94) clarify the severe enrichment of the site for Cd. Moreover, Pb (4.08) represents the moderate enrichment for Pb. In addition, Ni (1.76), Zn (1.34), Cu (1.18), Mn (1.00) expressed the minor enrichment for Ni, Zn, Cu and Mn, respectively. Thereafter, Cr (0.99) shows no enrichment for Cr.
FIGURE 4. Regional variation in enrichment factor (EF) of selected metals on sediment in Noakhali and Lakshmipur region.
Sediment quality is explained using Igeo values (Karbassi et al., 2008). The Igeo of the current investigation found that all of the sediment samples from the sampling sites were almost unpolluted (Igeo < 0). However, Igeo values of all metals were in the decreasing order of Cd (−0.32) > Pb (−1.05) > Ni (−1.19) > Zn (−1.33) > Cu (−1.36) > Mn (−1.42) > Cr (−1.44) (Figure 5).
The PLI values for all sampling sites were ranged from 0.22 to 0.63 and mean PLI value was 0.47. It was found that PLI values in all sampling stations were below 1, implying nil to very low level of contamination with heavy metals in sediments of commercial aquaculture farms from South Asian coastal area. As per PLI values, the sampling sites followed the decreasing order of S4 (0.138) >S1 (0.121) > S8 (0.117) > S5 (0.114) > S3 (0.107) > S6 (0.106) > S11 (0.105) > S12 (0.103) >S7 (0.096) >S9 (0.092) >S10 (0.084)> S2 (0.078) (Figure 6).
The genesis and transport of metals are indicated by correlations between heavy metals (Suresh et al., 2011). If there is no association between the elements, then no one factor controls the composition of metals (Kükrer et al., 2014). In this study, Cu-Cr (r = 0.65), Mn-Cd (r = 0.594), Zn-Pb (r = 0.591), and Zn-Cu (r = 0.586) were all found to have positive significant correlations (p < 0.05), and Zn-Cr (r = 0.757) exhibited a highly significant positive correlation (p < 0.01). None of the metal concentrations and Ni concentration were significantly associated (Table 5). However, the relationships between the investigated metals pointed to a single or shared source of origin (Singh et al., 2005). Despite the fact that metals are deposited in sediments, the majority of them end up in the aquatic environment (Grabowski et al., 2001). The quantity of heavy metals in sediments has been significantly influenced by human activity and some environmental conditions, including temperature, salinity, the amount of organic matter in sediments, and sediment grain size (Bentivegna et al., 2004).
CA stands for the spatially variable, similar and different groupings of the sampling site that can be used to detect specific locations of contamination (Sundaray et al., 2011). In this work, significant clusters were defined as (Dlink/Dmax) < 15 and dendrograms were created using Ward’s approach with Euclidean distance as a measure of similarity and dissimilarity. Cluster 1 consisted of five sites (S5, S12, S4, S1, and S7) with comparatively higher metal loads in sediment and cluster 2 consisted of seven sites (S11, S2, S9, S10, S3, S6, and S8) with lower metal loads (Figure 7A). A relationship among the heavy metals and their potential sources can also be identified through CA (Chung et al., 2011). Further analysis revealed a dendrogram with three groups of significant clusters at Dlink/Dmax <20 (Figure 7B), where group 1 had Cd, Cr, Pb, and Cu, group 2 consist of Zn and Ni, and group 3 had only Mn. The findings indicated that Cd, Cr, Pb, and Cu in the studied area were mainly originated naturally whereas Zn, Ni, and Mn originated due to anthropogenic activities, e.g., feeding to fish, surface runoff, dumping household and industrial waste near fish farming area, etc.
FIGURE 7. Cluster analysis for (A) Euclidean distance for the sampling sites, and (B) Euclidean distance for the studied metals.
The extraction approach was used in PCA analysis to identify the principal components (PC), where Eigen values (>1) were taken into consideration. PCA reduces the original dimension of the data set to seven principal components (PCs), which account for all of the variation in the sample (Figure 8). With loadings of 0.515 and 0.5356, respectively, Cr and Zn dominated PC 1, which had an eigenvalue of 2.95 and explained 42.2% of the total variances. The relative importance of Pb in relation to component one reveals the metals’ particular place of origin. Due to their high loading scores in the respective components, Zn, Mn, and Cr were the prominent metals in the PCA analysis. PC 2 accounted for about 27.08% of the overall variation, and Mn contained the scores with the highest loadings (0.6423). PC 3 explained 15.9% of the total variance and displayed positive factor loadings on Cu (0.6023) (Table 6). The results of the sediment samples show that several sources, either anthropogenic or natural, may be responsible for the heavy metals found in commercial aquaculture farms. The necessary trace elements (Cu, Zn, and Cr) for the metals can be obtained from commercial fish feed (Hossain et al., 2022); as a result, they are grouped together in the same cluster (Figure 7B). While Pb is widely used in the production of batteries, metal products, ammunition, and X-ray shielding equipment, it can be introduced through anthropogenic sources (Sharma et al., 2014). Other non-essential elements, such Cd, Cr, and Hg, may enter the environment through the marketing of aquaculture systems, the use of medications and chemicals, improper aquaculture handling, and subpar waste disposal management, all of which are in agreement with Sultana et al. (2022). These metals can be related to house dissipates, fossil fuel burning, and agricultural overflow, according to correct analysis after the main sources of these metals were discovered during the study. Almost all locations in the Noakhali and Lakshmipur region have shown significant Cd accumulation. The analysis further clarifies that human activities are the primary cause of heavy metal concentrations on sediment, which has a significant negative impact.
FIGURE 8. PCA plot displaying the loading of two factors affecting the fluctuation of heavy metals in fish farm sediments.
The results of this study offer details on the characteristics of heavy metal pollution in sediments from several commercial aquaculture farms in the coastal region of South Asia. The findings demonstrated that the levels of pollution from seven heavy metals were identical in both regions and decreased in the following order: Mn > Zn > Ni > Cr > Cu > Pb > Cd. This analysis also demonstrates that, with the exception of Ni, sediment samples from the Noakhali region had higher concentrations of all heavy metals than those from the Lakshmipur region. The average CF and Igeo values were found higher in Cd followed by Pb, Ni, Zn, Cu, Mn, and Cr. Average enrichment factor (EF) demonstrated moderate to extremely high enrichment of heavy metals in the study areas. However, PCA result implies that Cr, Cu and Zn primarily originated from anthropogenic sources. The findings of the present study can be used to highlight measures for assuring the sediment of commercial aquaculture farms from South Asian coastal area against long-term heavy metal deposition as well as future investigation is recommended to determine element mobility from the feed to pond sediment and fish, accurate assessment of heavy metals and thereby reduce environmental risk.
The original contributions presented in the study are included in the article/supplementary material, further inquiries can be directed to the corresponding author.
Conceptualization—MH; Methodology—JS, MB, MM, and AA-U; Software—A-AN and FP; Validation—MH, TA, and BP; Investigation—JS and MM.; Writing-original draft preparation—JS, FP, and A-AN; Review draft—MH, BP, JY, and TA; Funding—BP and TA All authors have read and agreed to the published this version of the manuscript.
This study was financially supported by the Research Cell, NSTU, Bangladesh, Universiti Brunei Darussalam under the Faculty/Institute/Center Research Grant (No. UBD/RSCH/1.4/FICBF(b)/2020/029), (No. UBD/RSCH/1.4/FICBF(b)/2021/037) and the FOS Allied Fund (UBD/RSCH/1.4/FICBF(a)/2023). Also supported by Researchers Supporting Project Number (RSP 2023R144), King Saud University, Riyadh, Saudi Arabia.
The authors would like to extend their sincere appreciation to the Researchers Supporting Project Number (RSP 2023R144), King Saud University, Riyadh, Saudi Arabia.
The authors declare that the research was conducted in the absence of any commercial or financial relationships that could be construed as a potential conflict of interest.
All claims expressed in this article are solely those of the authors and do not necessarily represent those of their affiliated organizations, or those of the publisher, the editors and the reviewers. Any product that may be evaluated in this article, or claim that may be made by its manufacturer, is not guaranteed or endorsed by the publisher.
Ab Manan, W. N. A., Sulaiman, F. R., Alias, R., and Laiman, R. (2018). Determination of selected heavy metal concentrations in an oil palm plantation soil. J. Phys. Sci. 29, 63–70. doi:10.21315/jps2018.29.s3.8
Ahmed, M. K., Baki, M. A., Kundu, G. K., Islam, S., Islam, M., and Hossain, M. (2016). Human health risks from heavy metals in fish of Buriganga river, Bangladesh. Springerplus 5 (1), 1697. doi:10.1186/s40064-016-3357-0
Bangladesh Meteorogical Department (2016). Monthly maximum and minimum temperature, normal monthly rainfall.
Basta, N. T., Ryan, J. A., and Chaney, R. L. (2005). Trace element chemistry in residualtreated soil, key concepts and metal bioavailability. J. Environ. Qual. 34, 49–63. doi:10.2134/jeq2005.0049dup
Begum, A., Mustafa, A. I., Amin, M. N., Chowdhury, T. R., Quraishi, S. B., and Banu, N. (2013). Levels of heavy metals in tissues of shingi fish (Heteropneustes fossilis) from Buriganga River, Bangladesh. Environ. Monit. Assess. 185, 5461–5469. doi:10.1007/s10661-012-2959-4
Bentivegna, C. S., Alfano, J. E., Bugel, S. M., and Czechowicz, K. (2004). Influence of sediment characteristics on heavy metal toxicity in an urban marsh. Urban Habitats 2 (1), 91–111.
Birch, G. F., and Olmos, M. A. (2008). Sediment-bound heavy metals as indicators of human influence and biological risk in coastal water bodies. ICES J. Mar. Sci. 65 (8), 1407–1413. doi:10.1093/icesjms/fsn139
Bomer, E. J., Wilson, C. A., Hale, R. P., Hossain, A. N. M., and Rahman, F. A. (2020). Surface elevation and sedimentation dynamics in the Ganges-Brahmaputra tidal delta plain, Bangladesh: Evidence for mangrove adaptation to human-induced tidal amplification. Catena 187, 104312. doi:10.1016/j.catena.2019.104312
Chapman, D. V. (1996). Water quality assessments: A guide to the use of biota, sediments and water in environmental monitoring. Boca Raton: CRC Press.
Chowdhury, A., and Maiti, S. K. (2016). Assessing the ecological health risk in a conserved mangrove ecosystem due to heavy metal pollution: A case study from sundarbans biosphere reserve, India. J. Hum. Ecol. Risk Assess. 22 (7), 1519–1541. doi:10.1080/10807039.2016.1190636
Chung, C. Y., Chen, J. J., Lee, C. G., Chiu, C. Y., Lai, W. L., and Liao, S. W. (2011). Integrated estuary management for diffused sediment pollution in Dapeng Bay and neighboring rivers (Taiwan). Environ. Monit. Assess. 173, 499–517. doi:10.1007/s10661-010-1401-z
Das, P. R., Hossain, M. K., Sarker, B. S., Parvin, A., Das, S. S., Moniruzzaman, M., et al. (2017). Heavy metals in farm sediments, feeds and bioaccumulation of some selected heavy metals in various tissues of farmed Pangasius hypophthalmus in Bangladesh. Int. J. Fish. Aquac. 8 (3), 1–9. doi:10.4172/2150-3508.1000218
Department of Fisheries. (2020). Yearbook of fisheries statistics of Bangladesh 2019-20. Fisheries Resources Survey System (FRSS), Department of Fisheries, Ministry of Fisheries and Livestock, 138.
Dietrich, M., and Ayers, J. (2021). Geochemical partitioning and possible heavy metal (loid) bioaccumulation within aquaculture shrimp ponds. Sci. Total Environ. 788, 147777. doi:10.1016/j.scitotenv.2021.147777
Eja, C. E., Ogri, O. R., and Arikpo, G. E. (2003). Bioconcentration of heavy metals in surface sediments from the Great Kwa river estuary, Calabar, Southeast Nigeria. J. Nig. Environ. Soci. 1, 247–256.
FAO (1990). Food standards programme, Guideline levels for cadmium and lead in food. Codex committee of food additives and contamination, 22nd session. the Netherlands: Haugue.
Faruk, M. A. R., Ali, M. M., and Patwary, Z. P. (2008). Evaluation of the status of use of chemicals and antibiotics in freshwater aquaculture activities with special emphasis to fish health management. J. Bangladesh Agric. Univ. 6 (2), 381–390. doi:10.3329/jbau.v6i2.4838
Grabowski, L. A., Houpis, J. L., Woods, W. I., and Johnson, K. A. (2001). Seasonal bioavailability of sediment-associated heavy metals along the Mississippi river floodplain. Chemosphere 45 (4-5), 643–651. doi:10.1016/s0045-6535(01)00037-6
Hakanson, L. (1980). An ecological risk index for aquatic pollution control. A sedimentological approach. Water Res. 14 (8), 975–1001. doi:10.1016/0043-1354(80)90143-8
Hernandez, R., Belton, B., Reardon, T., Hu, C., Zhang, X., and Ahmed, A. (2018). The “quiet revolution” in the aquaculture value chain in Bangladesh. Aquaculture 493, 456–468. doi:10.1016/j.aquaculture.2017.06.006
Hossain, M. B., Miazie, M. R., Nur, A. A. U., Paul, S. K., Bakar, M. A., Paray, B. A., et al. (2022). Assessment of metal contamination in water of freshwater aquaculture farms from a South asian tropical coastal area. Toxics. 10 (9), 536. doi:10.3390/toxics10090536
Hossain, M., Karmakar, D., Begum, S. N., Ali, S. Y., and Patra, P. K. (2021). Recent trends in the analysis of trace elements in the field of environmental research: A review. Microchem. J. 165, 106086. doi:10.1016/j.microc.2021.106086
Islam, G. M., Habib, M. R., Waid, J. L., Rahman, M. S., Kabir, J., Akter, S., et al. (2017). Heavy metal contamination of freshwater prawn (Macrobrachium rosenbergii) and prawn feed in Bangladesh: A market-based study to highlight probable health risks. Chemosphere 170, 282–289. doi:10.1016/j.chemosphere.2016.11.163
Islam, M. S., Ahmed, M. K., Raknuzzaman, M., Habibullah-Al-Mamun, M., and Islam, M. K. (2015). Heavy metal pollution in surface water and sediment: A preliminary assessment of an urban river in a developing country. Ecol. Indic. 48, 282–291. doi:10.1016/j.ecolind.2014.08.016
Islam, M. S., Hossain, M. B., Matin, A., and Sarker, M. S. I. (2018). Assessment of heavy metal pollution, distribution and source apportionment in the sediment from Feni River estuary, Bangladesh. Chemosphere 202, 25–32. doi:10.1016/j.chemosphere.2018.03.077
Islam, S. M. D., Bhuiyan, M. A. H., Rume, T., and Mohinuzzaman, M. (2016). Assessing heavy metal contamination in the bottom sediments of shitalakhya river, Bangladesh; using pollution evaluation indices and geo-spatial analysis. Pollution 2 (3), 299–312. doi:10.7508/PJ.2016.03.005
Karbassi, A. R., Monavari, S. M., Nabi Bidhendi, G. R., Nouri, J., and Nematpour, K. (2008). Metal pollution assessment of sediment and water in the Shur River. Environ. Monit. Assess. 147, 107–116. doi:10.1007/s10661-007-0102-8
Ke, X., Gui, S., Huang, H., Zhang, H., Wang, C., and Guo, W. (2017). Ecological risk assessment and source identification for heavy metals in surface sediment from the Liaohe River protected area, China. Chemosphere 175, 473–481. doi:10.1016/j.chemosphere.2017.02.029
Khan, R., Parvez, M. S., Jolly, Y. N., Haydar, M. A., Alam, M. F., Khatun, M. A., et al. (2019). Elemental abundances, natural radioactivity and physicochemical records of a southern part of Bangladesh: Implication for assessing the environmental geochemistry. Environ. Nanotechnol. Monit. Manag. 12, 100225. doi:10.1016/j.enmm.2019.100225
Kükrer, S., Şeker, S., Abacı, Z. T., and Kutlu, B. (2014). Ecological risk assessment of heavy metals in surface sediments of northern littoral zone of Lake Çıldır, Ardahan, Turkey. Environ. Monit. Assess. 186, 3847–3857. doi:10.1007/s10661-014-3662-4
López-Alonso, M. (2012). “Animal feed contamination by toxic metals,” in Animal feed contamination (New Delhi: Woodhead Publ), 183–204.
MacDonald, D. D., Ingersoll, C. G., and Berger, T. A. (2000). Development and evaluation of consensus-based sediment quality guidelines for freshwater ecosystems. Arch. Environ. Contam. Toxicol. 39, 20–31. doi:10.1007/s002440010075
McCready, S., Birch, G. F., and Long, E. R. (2006). Metallic and organic contaminants in sediments of sydney harbour, Australia and vicinity—A chemical dataset for evaluating sediment quality guidelines. Environ. Int. 32 (4), 455–465. doi:10.1016/j.envint.2005.10.006
Mohiuddin, M., Hossain, M. B., Ali, M. M., Hossain, M. K., Habib, A., Semme, S. A., et al. (2022). Human health risk assessment for exposure to heavy metals in finfish and shellfish from a tropical estuary. J. King Saud. Univ. Sci. 34 (4), 102035. doi:10.1016/j.jksus.2022.102035
Muller, G. (1979). Schwermetalle in den sedimenten des Rheins-Veranderungen seit 1971. Umschau 79, 778–783.
NOAA (2012). Screening quick reference tables. Washington, D.C: National Oceanic and Atmospheric Administration.
NRC (2005). Mineral tolerance of animals. 2nd revised edn. Washington (DC): National Research Council of the national academies, The National Academies Press.
Paul, B. G., and Vogl, C. R. (2011). Impacts of shrimp farming in Bangladesh: Challenges and alternatives. Ocean. Coast. Manag. 54 (3), 201–211. doi:10.1016/j.ocecoaman.2010.12.001
Rahman, M. S., Hossain, M. S., Ahmed, M. K., Akther, S., Jolly, Y. N., Akhter, S., et al. (2019). Assessment of heavy metals contamination in selected tropical marine fish species in Bangladesh and their impact on human health. Environ. Nanotechnol. Monit. Manag. 11, 100210. doi:10.1016/j.enmm.2019.100210
Rahman, M. S., Saha, N., Ahmed, A. S., Babu, S. O. F., Islam, A. R. M. T., Begum, B. A., et al. (2021). Depth-related dynamics of physicochemical characteristics and heavy metal accumulation in mangrove sediment and plant: Acanthus ilicifolius as a potential phytoextractor. Mar. Pollut. Bull. 173, 113160. doi:10.1016/j.marpolbul.2021.113160
Saha, N., Mollah, M. Z. I., Alam, M. F., and Rahman, M. S. (2016). Seasonal investigation of heavy metals in marine fishes captured from the Bay of Bengal and the implications for human health risk assessment. Food control. 70, 110–118. doi:10.1016/j.foodcont.2016.05.040
Sarker, M. J., Kanungo, I., Tanmay, M. H., and Patwary, M. S. A. (2016). A study on the determination of heavy metals in sediment of fish farms in Bangladesh. Fish. Aquac. J. 7 (159), 2.
Sayadi, M. H. (2017). Grain size fraction of heavy metals in soil and their relationship with land use. Proc. Int. Acad. Ecol. Environ. Sci. 7 (1), 1.
Sharma, G., Pathania, D., Naushad, M., and Kothiyal, N. C. (2014). Fabrication, characterization and antimicrobial activity of polyaniline Th (IV) tungstomolybdophosphate nanocomposite material: Efficient removal of toxic metal ions from water. J. Chem. Eng. 251, 413–421. doi:10.1016/j.cej.2014.04.074
Singh, R., Gautam, N., Mishra, A., and Gupta, R. (2011). Heavy metals and living systems, an overview. Indian J. Pharmacol. 43 (3), 246–253. doi:10.4103/0253-7613.81505
Singh, V. K., Singh, K. P., and Mohan, D. (2005). Status of heavy metals in water and bed sediments of river Gomti–A tributary of the Ganga river, India. Environ. Monit. Assess. 105 (1), 43–67. doi:10.1007/s10661-005-2816-9
Soares, H. M. V. M., Boaventura, R. A. R., Machado, A. A. S. C., and Da Silva, J. E. (1999). Sediments as monitors of heavy metal contamination in the Ave river basin (Portugal), multivariate analysis of data. Environ. Pollut. 105 (3), 311–323. doi:10.1016/s0269-7491(99)00048-2
Sultana, S., Hossain, M. B., Choudhury, T. R., Yu, J., Rana, M. S., Noman, M. A., et al. (2022). Ecological and human health risk assessment of heavy metals in cultured shrimp and aquaculture sludge. Toxics 10 (4), 175. doi:10.3390/toxics10040175
Sundaray, S. K., Nayak, B. B., Lin, S., and Bhatta, D. (2011). Geochemical speciation and risk assessment of heavy metals in the river estuarine sediments—A case study: Mahanadi basin, India. J. Hazard. Mater. 186 (2-3), 1837–1846. doi:10.1016/j.jhazmat.2010.12.081
Suresh, G., Ramasamy, V., Meenakshisundaram, V., Venkatachalapathy, R., and Ponnusamy, V. (2011). Influence of mineralogical and heavy metal composition on natural radionuclide concentrations in the river sediments. Appl. Radiat. Isot. 69 (10), 1466–1474. doi:10.1016/j.apradiso.2011.05.020
Tomlinson, D. L., Wilson, J. G., Harris, C. R., and Jeffrey, D. W. (1980). Problems in the assessment of heavy-metal levels in estuaries and the formation of a pollution index. Helgoländer Meeresunters. 33, 566–575. doi:10.1007/bf02414780
Tuna, A. L., Yilmaz, F., Demirak, A., and Ozdemir, N. (2007). Sources and distribution of trace metals in the Saricay stream basin of southwestern Turkey. Environ. Monit. Assess. 125, 47–57. doi:10.1007/s10661-006-9238-1
Turekian, K. K., and Wedepohl, K. H. (1961). Distribution of the elements in some major units of the earth's crust. Geol. Soc. Am. Bull. 72 (2), 175–192. doi:10.1130/0016-7606(1961)72[175:doteis]2.0.co;2
WHO/FAO (1984). List of maximum levels recommended for contaminants by the Joint FAO/WHO. Codex Alimentarius Commission. Rome: CAC/FAL.
WHO/FAO (2020). The state of world fisheries and aquaculture 2020. Sustainability in action. Rome: Food and Agriculture Organization of the United Nations. doi:10.4060/ca9229en
Varol, M. (2011). Assessment of heavy metal contamination in sediments of the Tigris River (Turkey) using pollution indices and multivariate statistical techniques. J. Hazard. Mater. 195, 355–364. doi:10.1016/j.jhazmat.2011.08.051
Keywords: heavy metals, aquaculture farms, coastal area, Bangladesh, risk
Citation: Hossain MB, Sultana J, Pingki FH, Nur A-AU, Mia MS, Bakar MA, Yu J, Paray BA and Arai T (2023) Accumulation and contamination assessment of heavy metals in sediments of commercial aquaculture farms from a coastal area along the northern Bay of Bengal. Front. Environ. Sci. 11:1148360. doi: 10.3389/fenvs.2023.1148360
Received: 20 January 2023; Accepted: 07 April 2023;
Published: 05 May 2023.
Edited by:
Saurabh Pandey, Guru Nanak Dev University, IndiaReviewed by:
Akbar Hossain, Bangladesh Wheat and Maize Research Institute, BangladeshCopyright © 2023 Hossain, Sultana, Pingki, Nur, Mia, Bakar, Yu, Paray and Arai. This is an open-access article distributed under the terms of the Creative Commons Attribution License (CC BY). The use, distribution or reproduction in other forums is permitted, provided the original author(s) and the copyright owner(s) are credited and that the original publication in this journal is cited, in accordance with accepted academic practice. No use, distribution or reproduction is permitted which does not comply with these terms.
*Correspondence: Mohammad Belal Hossain, YmVsYWwuaG9zc2FpbkBuc3R1LmVkdS5iZA==
Disclaimer: All claims expressed in this article are solely those of the authors and do not necessarily represent those of their affiliated organizations, or those of the publisher, the editors and the reviewers. Any product that may be evaluated in this article or claim that may be made by its manufacturer is not guaranteed or endorsed by the publisher.
Research integrity at Frontiers
Learn more about the work of our research integrity team to safeguard the quality of each article we publish.