- 1Department of Entomology, Post Graduate College of Agriculture, Dr. Rajendra Prasad Central Agricultural University, Pusa(Samastipur), Bihar, India
- 2Department of Entomology, College of Agriculture, Punjab Agricultural University (Ludhiana), Punjab, India
- 3Department of Entomology, College of Agriculture, Assam Agricultural University (Jorhat), Assam, India
- 4Department of Entomology, College of Agriculture, Govind Ballabh Pant University of Agriculture and Technology (Pantnagar), Uttarakhand, India
- 5Department of Entomology, College of Horticulture, Dr. YSR Horticultural University, Parvathipuram(Vizianagaram), Andhra Pradesh, India
- 6Sugarcane Research Institute, Department of Entomology, Dr. Rajendra Prasad Central Agricultural University, Pusa(Samastipur), Bihar, India
- 7Department of Entomology, College of Horticulture, Dr. Yashwant Singh Parmar University of Horticulture and Forestry, Nauni(Solan), Himachal Pradesh, India
- 8Department of Entomology, Sri Karan Narendra College of Agriculture (Jobner), Rajasthan, India
Environmental contamination research has been quite interesting in bioindicators recently. The basic objective of bioindicator research is to find species that can reliably detect environmental disturbances and demonstrate how those disturbances affect other species or biodiversity as a whole. Since they frequently come into contact with the harmful substances found in soil, water, and air, insects are particularly valuable for evaluating how human activities affect the terrestrial ecosystem, the aquatic system, and the atmosphere. In this review article, we’ve emphasized the use of insects as a resource for assessing contaminants and monitoring environmental contamination. Insects have been our main focus since they are key indicators of changes in soil, water, and air quality. The majority of insects, including beetles, ants, honey bees, and butterflies are employed in this study as biological indicators since they are sensitive to even the slightest environmental changes and are also used to monitor different environmental toxins.
1 Introduction
Global food production is in grave danger as a result of rising temperatures, climatic extremities, increased CO2 and other greenhouse gas concentrations (GHGs), as well as changed precipitation patterns (Shrestha, 2019). Today’s world is dealing with the significant issue of global warming. It has reached unprecedented levels, as indicated by the incredible rates of increase in the air temperature and sea level (Field et al., 2014). According to the World Meteorological Organization (WMO), the world is currently about one degree warmer than it was before heavy industrialization. According to the Intergovernmental Panel on Climate Change (Field et al., 2014; IPCC, 2022), each of the past three decades has gotten warmer, with the 2000s being the warmest. The Earth may warm by 1.4°C–5.8°C over the next century, according to a variety of global climate models and development scenarios (Pachauari and Reisinger, 2007). Greenhouse gas concentrations have dramatically increased during the past two centuries when compared to the pre-industrial era, which is a primary contributor to global warming (Rogelj et al., 2018). Carbon dioxide (CO2), Methane (CH4), and Nitrous oxide (N2O) are produced in the atmosphere as a result of the most prevalent anthropogenic activities, such as burning fossil fuels and altering land use (Yoro and Daramola, 2020). Over the past 50 years, one of the atmosphere’s well-observed changes has been the rise in atmospheric CO2 (Prentice et al., 2001). Its atmospheric concentration has drastically increased to 416 ppm from 280 ppm reported for the pre-industrial period and is expected to double in 2,100 (Pachauari and Reisinger, 2007; Anonymous, 2023). Heat infrared radiation from the Earth’s surface is significantly absorbed by the atmosphere; therefore CO2 is classified as a greenhouse gas. In proportion to the quantity of atmospheric gasses that absorb thermal infrared radiation from the Earth’s surface, more radiation is discharged from the atmosphere towards the surface of the planet (Mahlman, 1997). Since there is more energy available for sensible and latent heat flow, the long-wave balance at the Earth’s surface is less negative. The amount of energy available for heat flux increases, which raises the temperature of the air (Streck, 2005). Extreme weather and climatic phenomena have been observed to shift since the middle of the 20th century. There have been other changes that have been connected to anthropogenic impacts, such as a decrease in cold temperature extremes, increased warm temperature extremes, faster rates of sea level rise, and more frequent heavy precipitation events have all been observed in several areas. Weather extremities, such as heat waves and prolonged periods of extreme precipitation, are predicted to rise in frequency and severity in some areas (Field et al., 2014). Even though the speed and complexity of global change pose a significant challenge for ecology, this fact does not exclude taking action to address the fundamental causes of global change (Yang et al., 2021).
Detrimental environmental change across the world has received much more attention in recent decades. The two main factors influencing the current state of global biodiversity are habitat change and overexploitation. Finding practical methods for averting or minimizing potentially catastrophic consequences on biodiversity became necessary. An agricultural environmental scheme is a complementary tool that which could reverse the negative trends of declining biodiversity (Pereira et al., 2012). Ecosystems are directly challenged by environmental contamination, making environmental monitoring a necessary component of their management and prediction. The concept of bioindication is not a conventional one; it has been turned into an emerging issue related to conservation assessment. The term “bioindicator” refers to a species or a group of species that represents the abiotic or biotic state of the environment. It illustrates how environmental change affects a habitat, community, or ecosystem and indicates whether that change has a positive or negative influence (Parmar et al., 2016). Various living organisms are extremely sensitive to environmental changes that disrupt their core functions, such as metabolism, growth, and reproduction (David, 1989). Owens et al. (2020) concluded how artificial lights can be tuned to reduce their impact on susceptible populations. Artificial light at night is unique among anthropogenic habitat disturbances in that it is fairly easy to upgrade, and leaves behind no residual effects. Moreover, recognition of the ways in which artificial light at night affects insects can help conservationists to reduce or eliminate one of the major drivers of insect declines. Contrary to other putative causes of insect decreases, such as habitat loss, chemical and light pollution, and nutrient dilution, these factors may be common in surviving natural areas (Welti et al., 2020). Sánchez-Bayo and Wyckhuys (2019) demonstrates significant rates of decline that within the next several decades could result in the extinction of 40% of all insect species worldwide. Bioindicators are frequently utilized as markers for biological parameters, taxonomic variety, or natural change.
Arthropods turn out the most successful among all invertebrates despite their survival during the Cretaceous and Permian mass extinctions. They are the principle players functioning in the ecosystem processes (Kim, 1993). The most prevalent animal in all types of ecosystems, insects can be utilized to detect the impact of alterations in the environment. Taxonomic, behavioural, and environmental studies eventually are necessary to monitor the changes in biodiversity and its inevitable implications. To assess environmental change, biomonitors and bioindicators are essential components. When evaluating the environment, bioindicators can be used to estimate the quality of changes occurring while biomonitors are employed to gather quantitative data on the environment’s quality. Therefore, it is necessary to use insects as a bioindicator to assess the quality of environmental changes. This review paper aims to provide a summary of the major insect bioindicator groups of different ecosystems and their role.
2 Criteria for the selection of bioindicator
To preserve biodiversity, healthy ecosystems must be maintained. Since ecosystems are so complex, using indicator taxa to assess ecosystem health is highly imperative. Ecosystem health, biodiversity levels, target taxa status, endemism levels, and resource availability have all been evaluated using indicator taxa (Faith and Walker, 1996). The prerequisites for the indicator’s selection are flexible and heavily influenced by the investigation’s targets, such as the indicator for a study of an area’s biological variety, the indicator for habitat loss, the indicator for climate change, and the indicator for a polluted area. To choose a decent indicator, it must satisfy a wide range of requirements. The use of bioindicators is a common and economical method for identifying and monitoring environmental changes. A good bioindicator should have well-established taxonomy and ecology, be widespread across a large geographic area, be specialized to certain habitat requirements, provide early warning of change, be simple and cost-effective to survey, be largely independent of sample size, have a response that reflects that of other species, and be able to differentiate between trends or cycles caused by anthropogenic stress and those triggered by natural cycles or trends (Noss, 1990). The major criteria for the selection of bioindicators are shown in Figure 1.
Based on domestic and global studies on the selection of bioindicators, eleven selection standards have been established (Han et al., 2015) viz.
i. Species (or species groups) with clear classification and ecology
ii. Species (or species groups), those are distributed in a geographically widespread area.
iii. Species (or species groups), those show clear habitat characteristics
iv. Species (or species groups), those can provide early warning for a change
v. Species (or species groups), those can benefit promptly and economically from the investigation
vi. Species (or species groups), those are not adversely affected by the size of individual groups and have numerous independent individual groups
vii. Species (or species groups), those are thought to represent the response of other species.
viii. Species (or species groups), those are representative of the ecology change caused by the pressure of human influence
ix. Species (or species groups) for which research on climate change have been done
x. Species (or species groups), those are easy to observe, appear for a long time and form a large group of individuals
xi. Species (or species groups), those are significant in terms of culture, economy, and society
Moreover, there are 19 selection criteria can be categorized into four main groups: baseline information, location information, niche and life history attributes, and others (Hilty and Merenlender, 2000).
3 Classification of bioindicator
A bioindicator, which in certain contexts focuses on a living entity, monitors the progress of biological or non-biological factors in an environment. It serves as a representation to understand and assess the ecosystem’s health status. However, it also refers more broadly or specifically to the impacts of environmental change on a species or group of species that represent the state of living forms or inanimate objects in the environment. Likewise, it could be used to describe living entities or group of living things that illustrate the diversity of a taxonomic group in a specific area or the whole diversity (Gerhardt, 2002). Bioindicators can be categorized in a variety of ways (Mc Geoch, 1998). classified them into three categories: environmental, ecological, and biodiversity indicators based on diverse background and application. The meta-analysis of global data sets reveals that pesticide resistance levels are linked to the species overwintering range. By facilitating local persistence all year round, climate change can promote and expand pesticide resistance of this destructive species globally (Ma et al., 2021).
3.1 Environmental indicator
A species or group of species that respond well to environmental disturbances or a change in the status of the environment in predictable ways that can be easily observed and quantified is known as an environmental indicator. It is used to detect changes in the environment.
3.2 Ecological indicator
The species or group of species demonstrates the impact of a stressor on a biotic system and is used to monitor long-term stressor-induced change on biota (including habitat alteration, fragmentation and climate change).
3.3 Biodiversity indicator
A group of taxa (such as a genus, tribe, family, or order, or a particular group of species from a range of higher taxa) or a functional group that exhibits diversity (character and species richness, or level of endemism) that is reflective of the diversity of other taxa in a habitat is referred to as a biodiversity indicator (Mc Geoch, 1998; Stewart et al., 2007).
Also, the term bioindicators can mean different types of indicators, including plant, animal, and microbial indicators according to their taxonomic status (Figure 2).
3.4 Plant indicator
In the era of urbanization, pollution continues to threaten health and welfare. The Plant shows high sensitivity to environmental stress recognition and prediction. Biomonitoring with sensitive plant species performs an effective means of attenuating this problem. Increased levels of pollutants like sulphur and nitrogen are responsible for the disappearance of lichens from forest areas (Holt and Miller, 2010). Marine ecosystem contamination is indicated by alterations in the diversity of phytoplankton species, such as Euglena clastica, Phacus tortus, and Trachelon anas (Jain et al., 2010). Cyanophyta is a biological indicator of powerful plankton that shows the rapid eutrophication of aquatic habitats through the production of bloom patterns (Thakur et al., 2013). The impact of air pollutants can be interpreted by calculating Air Pollution Tolerance Index (APTI) using biochemical parameters of plants like total chlorophyll content, ascorbic acid content, relative water content, and pH value (Singare and Talpade, 2013). The Guava cultivar “Paluma” can potentially be used as a bioindicator of phytotoxic ozone levels in the tropical region (Furlan et al., 2007). Auto fluorescence analyses of the secretory cells of vegetative tissues or microspores are suitable objects for ozone (O3) monitoring and could early indicate damage by stress factors (Roshchina and Roshchina, 2003).
3.5 Animal indicator
Changes in a number of an organism’s characteristics have been successfully employed as indicators. Animal indicators assist in determining the level of toxins present in an animal’s tissues (Khatri and Tyagi, 2015). Zooplanktons that are zone-based indicators of pollution include Alona guttata, Mesocyclops edax, Cyclops, and Aheyella (Jain et al., 2010; Hosmani, 2014). They are used to indicate water quality, eutrophication, and contaminated water. Amphibians, notably anurans, which include frogs and toads, are frequently used as biological indicators of contaminant accumulation in a specific ecosystem (Zaghloul et al., 2020). Earthworms, a key soil system species, can play a vital role in the formation and decomposition of soil aggregates, according to the kind of organic residues (Al-Maliki et al., 2021). Earthworms are more sensitive to temperature changes as a bioindicator of soil quality than the effects of moisture content. Invertebrates are also reliable bioindicators; insects play vital roles as indicators in ecosystems. Environmental stress can be assessed at several scales, from the individual animal to the entire invertebrate community.
3.6 Microbial indicator
Microorganisms are potentially used as indicator species to detect environmental pressures when exposed to environmental perturbations. They exhibit high sensitivity towards environmental change. Changes in the physiological system of microbes like the development of stress protein (Khatri and Tyagi, 2015) or alterations in the digestive system help to detect the presence of toxins in water (Uttah et al., 2008). A group of eukaryotic microbes, Foraminifera is used for coral reef monitoring (Hallock et al., 2003). Assessment of coral reef health through biofilms coupled with Autonomous Reef Monitoring Structures (ARMS) tools are also employed (Roitman et al., 2018). Bacterial orders such as the opitulales, chitinophagales, cytophagales and saccharimonadales may act as bioindicators of high P or nutrient-rich locations (Mason et al., 2020). Molds, including Trichoderma sp., Exophiala sp., Stachybotrys sp., Aspergillus fumigatus, A. versicolor, A. niger, Phialophora sp., Fusarium sp., Ulocladium sp., Penicillium sp., Candida albicans, and certain yeasts are frequently used as biological indicators for contaminants. According to (Dokulil, 2003) blue-green algae may be used as a biological indicator for detecting pH value variations in various ecosystems.
4 Insects used as a bioindicator of climate change
One of the most important contemporary ecological issues is how the consequences of climate change on living things affect them. These consequences are pervasive and frequently negative (Wilson et al., 2007; Bellard et al., 2012; Grimm et al., 2013; Menéndez et al., 2014; Sheldon, 2019). Various other studies have demonstrated that climate can have both direct and indirect effects on species interactions, range, abundance, and phenology. It also affects on the structure and dynamics of ecosystems, biodiversity, and the constitution of biological communities. According to numerous reports, animals may migrate northward in response to climate change based on their physiological tolerances to temperature (Parmesan, 1996; Root et al., 2003; Menéndez, 2007; Menéndez et al., 2014; Sheldon, 2019). Different ecological processes, as well as the biology and ecology of species, are being impacted by climate change. As a result, choosing a bioindicator to track these consequences becomes essential, even if choosing the right species is quite challenging. In this regard, some researchers have suggested criteria for choosing species (Groot et al., 1995). Also, they have been cited as effective bioindicators of climate change (Menéndez, 2007; Gerlach et al., 2013) as the climate has a direct impact on their survival, reproduction, and development Figure 3 (Bale et al., 2002).
Contrary to other living things, insects frequently have shortened life cycles, and they are represented at many different trophic levels, where they play significant roles in ecosystem function levels. However, it is crucial to comprehend the current elevation distribution and other ecological characteristics of the local insect populations to monitor the consequences of climate change in mountains. Among insects, beetles (Coleoptera) stand out as the most diversified known living species among the 300,000–450,000 recognized species (Bouchard et al., 2017). They may be easily sampled using a variety of approaches and have already been suggested as bioindicators in various contexts (Gerlach et al., 2013; Oliveira et al., 2014). The most vulnerable species to extinction due to climate change are those found in mountains and those confined to high latitudes. As a result of global warming, the cold-adapted species will be compelled to ascend to higher latitudes. Some of the species might someday reach the end of their range and go extinct. The decline of a species can happen over many hundreds of years and is difficult to identify. Between 1970 and 1999, there was a significant shift in the diversity of butterfly species in Britain (Menéndez et al., 2006). As an example, during two seasons of artificial warming, the isotomid Cryptopygus antarcticus experienced a population loss, which led to a decrease in the overall abundance of the order Collembola in the lichen community. The effects of temperature increases of 1°C–2°C will have effects comparable in magnitude to the climate change currently observed in the Antarctic region. Gamasellus racovitzai (Trouessart), a mesostigmatid predatory mite, significantly decreased, and the overall number of prostigmata significantly increased (Bokhorst et al., 2008). In the United Kingdom, a lot of beetles, butterflies, dragonflies, grasshoppers, and aquatic bugs have migrated north and to higher altitudes (Hickling et al., 2006). Studied by (Colares et al., 2021) five Coleopteran species from the family Cerambycidae, Eumolpinae (Chrysomelidae), Lampyridae, and Phengodidae families were chosen as ideal bioindicators because of their heat tolerance, narrow elevational ranges, and abundance. The smallest elevation range belongs to the family Eumolpinae and Lampyridae. Utilizing abundant species (or groups of species) with constricted elevation ranges as bioindicators can help to monitor the effects of climate change on the biota and determine how species are adapting through time. According to their findings, imidacloprid residues may be extremely hazardous to non-target insects in the environment, even in areas far from the agro ecosystem where the herbicides were used (Wang et al., 2022). Their findings showed that the Scarabaeinae fauna of Itatiaia is incredibly diverse, with wide fluctuations in richness and abundance along the elevation gradient and notable phonological shifts at the community level related to seasonal climate variations (Araújo et al., 2022).
The class Insecta includes many potential representatives that can be utilized as environmental bioindicators since they possess a higher structural and functional organization level, highly complex morphology, physiology, well-developed sense organs, complex behavior, and are characterized by a greater diversity of species. As a result, they can be more precise, quicker, and more flexible to reflect disturbances in their environment, as xenobiotics may reach the organisms via air, water, or soil. Utilizing bioindicators is a cutting-edge approach for detecting several forms of environmental mismanagement, such as contamination, high-input farming that degrades soil health, improper waste disposal, and pollution. The significance of insects as bioindicators in supporting human policy decisions for conservation and assessing the functional effects of human disturbance on ecosystems is acknowledged globally, but we ignore this in India, hence it is highlighted here (Table 1). Coleoptera, Diptera, Hymenoptera, Isoptera, Lepidoptera, and Hemiptera are examples of orders that may serve as indicators of changes in microclimate, foraging behavior and nesting places, reduced food availability due to the use of agrochemicals, and interactions with other species (Figure 4).
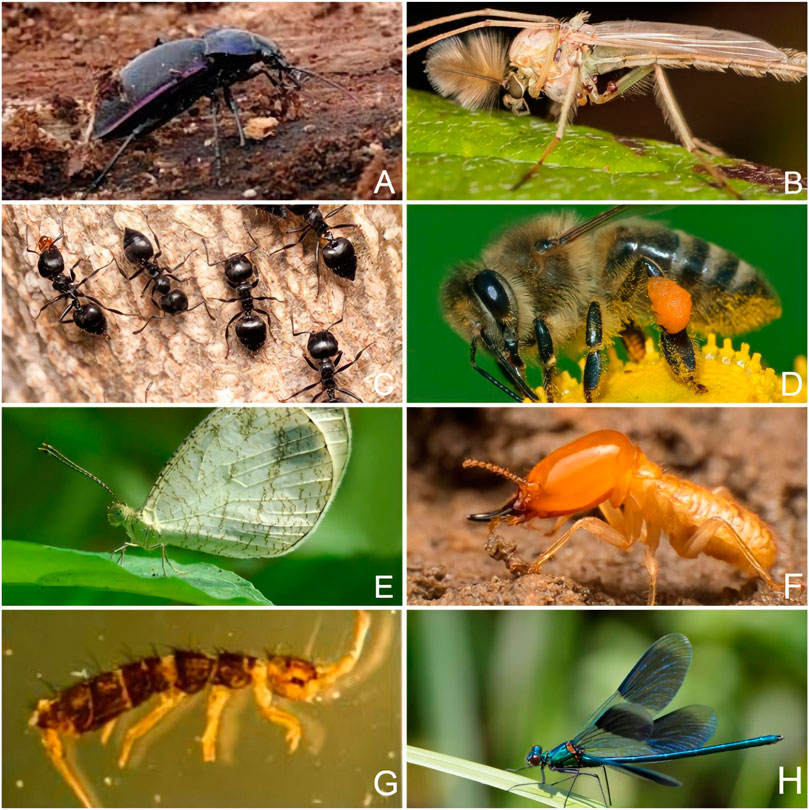
FIGURE 4. Major bioindicator insects. (A) Beetle, (B) Chironomid, (C) Ant, (D) Bee, (E) Butterfly, (F) Termite, (G) Springtail, (H) Dragonfly.
To determine how climate change will affect IPM programs necessitates an understanding of how changes in relevant abiotic factors will affect insect pheromonal communication is described in Table 2. Minor alteration in temperature, relative humidity, CO2 and O3 concentrations, wind currents, and average annual precipitation could change in insect behavior which will create consequences for agricultural productivity (Sharma and Singla, 2021). Insect pheromone production could be impacted by rising atmospheric CO2 levels. The production of secondary metabolites is impacted by variations in CO2 concentrations in plants (Ode et al., 2014). Escape behavior of aphids could be affected due to increasing CO2 concentration by limiting the acetylcholinesterase enzymatic activity, which is related to neural transmission of alarm pheromone perception (Boullis et al., 2016).
There are three ways that ectotherms might adapt to climate change: plasticity, local adaptations, or both. They can modify their phenology to adapt to new seasonal climate patterns or change their geographic distribution to match their current thermal preferences (Walther et al., 2002; Parmesan and Yohe, 2003). Each of these actions has an impact on the population and community levels as well as the evolution of species (Blanckenhorn, 2018). If insects can intermittently boost their cold tolerance, such as by fast cold-hardening, quiescence, a immediate behavioral response may enable them to deal with warmer and more fluctuating winter temperatures (Owen et al., 2013). The parasitoid Anaphes nitens (Hymenoptera: Mymaridae) undergoes winter quiescence in mild-winter regions of N-E Spain instead of displaying genuine diapause to adapt the duration of dormancy to the winter time temperatures (Santolamazza-Carbone et al., 2009).
5 Bioindicator insect groups to monitor environmental changes
5.1 Beetles as bioindicator
Coleopterans are considered as potential bioindicators and mainly belong to the group Carabidae, Staphylinidae, and Curculionidae. The most popular insect utilized in environmental biomonitoring is the ground beetle, especially for evaluating environmental pollutants and forest management (Ghannem et al., 2018). They are collected by using pitfall traps from the region of chronic heavy metal pollution, which has resulted in an accumulation of various heavy metals in the soil. Multiple morphological traits are assessed to monitor heavy metal pollution like elytra length, body size, etc. (Lagisz, 2008). The toxic effect of copper has resulted in changes in the locomotory behaviour of adult carabids and a high degree of larval mortality. It is believed to be that behavioural changes are associated with copper exposure during larval development (Bayley et al., 1995). With research on Pterostichus oblongopunctatus (Simon et al., 2016), observed high BAF (Bioaccumulation factor) values for Copper (Cu) and Zinc (Zn) indicating that this species is preferable in metal contamination assessment. Additionally, it has been demonstrated by (Conti et al., 2017) that the carabid sp. Parallelomorphus laevigatus is a reliable indicator of toxic substances. In forests or on farms, scarabaeid beetles have a tremendous amount of potential as environmental indicators (Niemela et al., 1993). Dung beetles are likewise affected by forest fragmentation, and their abundance and species richness are positively correlated with the size of the fragments (Rainio and Niemela, 2003). To evaluate the genotoxic effects of herbicides on non-target soil-dwelling species, Harpalus rufipes provides early warning signs (Cavaliere et al., 2019).
5.2 Lepidopterans as bioindicator
Butterflies are significant bioindicators on account of their sensitivity towards the slightest alteration in environmental factors. They have been extensively used as heavy metal and environmental pollution bioindicators that are close to industrial states and even inside metropolitan regions (Da Renato et al., 2010). Butterflies are very sensitive to climatic variables. The temperature has a significant impact on butterfly range shifts, oviposition sites, egg-laying rates, larval development, and survival rates (Sharma and Sharma, 2017). Pupal size of Noctuidae and Geometridae species changes with levels of industrial air pollutants (Heliövaara et al., 1989). According to (Kyerematen et al., 2018), different environmental stressors in Sierra Leone’s wetlands affect butterfly communities, and the higher the stress, the lesser the diversity of butterflies in a specified place. According to the estimates, the above observation is the consequence of significant environmental stresses in the Sierra Leone River Estuary (SLRE), including mining, agricultural, and factory pollution. The results further show that Mamunta Mayosso Wildlife Sanctuary (MMWS) is made up of a mosaic of several vegetation patches which supports higher diversity of butterfly species. This study also reveals that anthropogenic activities have a deleterious impact on butterfly diversity. Moths have been utilized as bioindicators to monitor the recovery of vegetation following environmental stress (Kumar et al., 2015). Arctiinae, Catocalinae, Heliothinae, Noctuinae, Hermeniidae, and Phycitinae are some moth families/subfamilies that respond positively to disturbances, while Ennominae, Geometrinae, Lymantriidae, Epipaschiinae, and Anthelidae respond negatively (David, 1989). Kozlov et al. (2022) also noted that the overall abundance of Lepidoptera (moths and butterflies) did not alter along the pollution gradient. The life history characteristics of each species were linked to how it responded to pollution. Insect ability to withstand heavy metals varies widely. Despite the global decline in pollinators, little is understood about how heavy metals affect insects. According to Skaldina and Sorvari (2019), the heavy metal pollution alters the morphology and physiology of insect pests including aphids and butterfly larvae.
5.3 Ants as bioindicator
Ants are ubiquitous in almost all the trophic levels of the web, food chains, and other ecological functions. They are used as a good bioindicator group for various types of pollution (Kaspari and Majer, 2000; Andersen et al., 2002) including soil-heavy metal contamination (Gramigni et al., 2013; Khan et al., 2017), aerial phthalate pollution (Lenoir et al., 2014) and land rehabilitation (Khan et al., 2017). Red wood ant Formica lugubris accumulates heavy metals such as Aluminum (Al), Cadmium (Cd), Cobalt (Co), Copper (Cu), Iron (Fe), Nickel (Ni), Lead (Pb), and Zinc (Zn) both in worker ant bodies and nest material (Skaldina et al., 2018). Ants are increasingly being used as bioindicators to monitor ecosystem health conditions (Akhila and Keshamma, 2022). The ant fauna also somewhat reflects the other invertebrate taxa found in a region (Majer, 1983). Ants are frequently employed as efficient disturbance bioindicators for restoring biodiversity and managing ecosystems (Underwood and Fisher, 2006) because of their ecological relevance (Tibcherani et al., 2018) and sensitivity to ecosystem disruption brought on by species invasion, grazing, thinning of the forest, conversion of the forest, fragmentation of the forest, and other disturbances (Da Renato et al., 2010).
5.4 Bees as bioindicator
Social insects can consume pollutants while foraging and then pass them on to the larvae or mix them into the materials used to build their nests. Pollutants in bees can also wind up in food that has been kept, such as honey or bee bread (Feldhaar and Otti, 2020). Honey bees are reliable and efficient biological indicators because they exhibit environmental chemical impairment due to a high rate of mortality and intercept particles suspended in air or flowers. They were incredibly effective in ground surveys due to their high mobility. Honey bees are used to monitor pesticides, heavy metals, and radionuclides (Porrini et al., 2003). Studied by (Di Fiore et al., 2022) the concentrations of different elements viz., Beryllium (Be), Cadmium (Cd), Cobalt (Co), Chromium (Cr), Nickel (Ni), Lead (Pb), Copper (Cu), and Vanadium (V) in urban and rural areas. Later, they concluded that the honeybee can accumulate environmental pollutants and provide data on the progression of environmental pollution across time and space. Honey bees use two signals to indicate the chemical disruption of the environment, i.e., mortality (mostly due to pesticide residues) and residues detected in their bodies or bee hive products (pesticides and other contaminants like heavy metals and radionuclides), that can be determined employing appropriate laboratory analysis (Barganska et al., 2016). There is a possibility that the honey bee colony will absorb pesticides in layers which represents the yearly treatment strategy throughout the colony’s territory because of its diverse range of feeding preferences and extended foraging season. Careful pesticide content monitoring can reveal variations in the persistence of agrochemicals in particular hive compartments (Quigley et al., 2019). When compared to colonies that were taken in less disturbed habitats, those that were obtained in heavily anthropogenic areas show more asymmetry. Indeed, morphological characteristics can also reveal the habitat quality (Nunes et al., 2015). Cunningham et al. (2022) stated that the pollinator European honey bee, Apis mellifera exposed to pollutants and diseases while foraging they bring these things back to their hives where they may be identified and used as biomonitoring. Polluted substance is found in bees and their hive components such as honey, wax, and stored pollen, as well as heavy metals, air pollution, pesticides, and plant diseases. Honey bees are also used as indicators on new dangers like climate change and antibiotic resistance.
5.5 Parasitic wasps as bioindicator
Recently, parasitic wasps have been employed as bioindicators for habitats in woodlands (Hilszczanski et al., 2005). On a landscape scale, the parasitoids were more prevalent in habitats of mixed woodlands with a variety of species than in coniferous woodlands. The parasitoid assemblage was more strongly influenced by forest type and deadwood attributes, indicating that it is essential to maintain the diversity of deadwood habitats for parasitoid conservation. Due to their high trophic position, complex biology, and restricted host ranges, parasitic wasps are intricate and highly specialized (Maleque et al., 2009). Aculeate wasps can accurately quantify the variety of all arthropod species in an ecosystem, both social and solitary wasp species, and some social wasp species have also been found to be reliable indicators of heavy metal contamination (Brock et al., 2021).
5.6 Chironomids as bioindicator
Chironomids have been used as a biomonitoring model for ecotoxicological tests. Antennal deformities serve as great early warning indicators for the detection of toxic contaminants in the environment (Warwick, 1990). Both somatic aberrations (deletions, inversions, and deficiencies) and functional abnormalities (reduction in the amount of BRc, BRb, and the NOR activity) caused by trace-metal contamination can be easily recognized and utilized as reliable markers of genotoxicity (Michailova et al., 2012). Mouthparts deformities of chironomids are also observed under elevated metal concentrations (Arimoro et al., 2018). The family Chironomidae has the potential to be a bioindicator of changes in aquatic ecosystems on a global scale due to their species richness, including the indirect effects of drought (Cañedo-Argüelles et al., 2016).
5.7 Syrphid flies as bioindicator
Syrphid flies are a strong bioindicator of landscape-level forest management practices due to their widespread geographic distribution (Maleque et al., 2009). Eristalis spp. and Sphaerophoria spp. accumulate heavy metals like Mn, Pb, and Cd of the industrial region in their body (Markova and Alexiev, 2002). Additionally, due to their high adult mobility, flies are the best tool to assess the biodiversity of landscapes (Sommaggio, 1999).
5.8 EPT taxa as bioindicator
Ephemeroptera, Plecoptera, and Trichoptera (EPT), more commonly referred to as mayflies, stoneflies, and caddisflies are great bioindicators of good water quality. Mayflies are sensitive to oxygen depletion in flowing water. Stoneflies indicate highly oxygenated water and caddisflies are sensitive to water pollution and used as bioindicators of water purity (Parikh et al., 2020). Caddisflies and mayflies being abundant in waters subject to little toxic stress, it has been hypothesized that the relative numbers of caddisflies, and mayflies may indicate various degrees of heavy-metal stress (Winner et al., 1980).
5.9 Dragonflies as bioindicator
The most reliable ecological indicator in riparian and aquatic environments is thought to be dragonflies. They respond sensitively to habitat disturbance and heavy metal deposition, particularly in lakes and flooded drainage areas (Shafie et al., 2017). Their presence in any aquatic body indicates that it is synthetic pollution-free (Azam et al., 2015).
5.10 Termites as bioindicator
Termites are “ecosystem engineers”, which act as bioindicators of soil fertility through the determination of soil physico chemical parameters of termite mound soil in comparison with chemically fertilized soil (Nithyatharani and Kavitha, 2018). Termites also show indicator capacity for soil ecosystem services such as chemical fertility, hydrological functions, macro-aggregation, and biodiversity (Duran-Bautista et al., 2020). Termites accumulated high amounts of heavy metals such as Ca, Mg, Al, Fe, Zn, Cu, Mn, Be, Ba, Pb, Cr, V, Ni, and Cd. Alajmi et al. (2019) investigated and recorded a significant direct relationship between the presence of termites on the concentrations of Al, Cu, Zn, Be, Cd, Mn, Ca, Mg, Pb, V, and Mo, while, a significant indirect correlation existed for Ba, Cr, Ni, Co, and Fe.
5.11 Springtails as bioindicator
Springtails are abundant soil arthropods which are very sensitive to changes in the soil properties and the impact of anthropogenic activities. The decline in springtail species richness reflects soil water acidity brought on by organic pollutants and wastes, pesticide use in agricultural soils, and heavy metal pollution (Rusek, 1998). The QBS-adapt index, which measures the soil’s biological quality in response to seasonal changes, is a sensitive tool for distinguishing between various land use patterns for different seasons. The QBS-adapt index can be determined by the morphological traits of springtails (Machado et al., 2019).
5.12 Minor insect groups as bioindicator
Aphids are indications of pollution even though surge in population density while feeding on hosts that are exposed to environments with high CO2 concentrations (Zaghloul et al., 2020). Also, Nepidae, Corixidae, Notonectidae, Gerridae, Belostomatidae, Veliidae, and Mesoveliidae are hemipteran insect families that serve as bioindicators used to assess the water quality (John and Polhemus, 2008). Gerridae family members were used to monitor different iron and manganese concentrations, however, it seems that they behaved less well enough for nickel and lead (Nummelin et al., 2007).
6 Insect as bioindicators of different ecosystems
The major bioindicator insects in different ecosystems are depicted in Figure 5.
6.1 Insect as bioindicators of agricultural ecosystem
Changing consumer demand, the need for higher production, and climate change are all exerting strain on agricultural landscapes. Environmentally significant creatures that inhabit agricultural land and its surroundings will be impacted by decisions made about land management. Agricultural landscape quality changes can be detected and followed over time and space using honey bees as bioindicators (Quigley et al., 2019). Agro-ecosystems are primarily performed as both providers and consumers of ecological services including carbon sequestration, soil conditioning, decomposition of organic matter, nutrient recycling, predation, pollination, support biodiversity, and cultural services. Intensive chemical input has subjected to serious degradation of these services. Monoculture provides an optimum environment for the growing population of herbivorous insects. Insect pollinators include hundreds of solitary bee species, bumble bees, flies, beetles, and butterflies, mainly hymenoptera communities that are common for pollination in agricultural areas. Furthermore, predatory insects such as some beetles, true bugs, lacewings, flies, midges, and wasps contribute to crucial ecosystem functions by acting as biocontrol agents. Moreover, through interacting with the soil, insects play a crucial role in enhancing agricultural soil. Dung beetle increases nitrogen, phosphorus, potassium, calcium, and magnesium or total protein content in the soil which elevates the crop yield in relation to chemicals (De Groot et al., 2002). Bioturbation agents, like ants, play a major role in bioindication due to their greater diversity in agro-ecosystem. The activity of terrestrial decomposer termites boosts infiltration potential, which results in water retention and improved productivity.
6.2 Insect bioindicators of forest ecosystem
Bioindicators are a potentially useful tool for Sustainable Forest Management (SFM). An increase or decrease in the population of insect species may indicate significant changes in the ecosystem. The predominance of houseflies and mosquitoes indicates unhygienic environmental conditions. Butterflies are regarded as reliable ecological indicators and are tolerant to changes in topography and moisture. Butterflies are a sign of healthy environmental conditions (Weiss et al., 1988). Due to the intensive competition for resources, which prevents the predominance of a few dominating species, areas that are either cultivated or heavily forested with a diverse range of plant species demonstrated significant insect species diversity and higher ecological stability (Da Rocha et al., 2010). An extensive study of the consequences of forest fragmentation has been conducted utilizing a variety of arthropod indicator species. For example, (Fujita et al., 2008) revealed that urban forest remnants’ richness in carabid species increased with the rise in fragment area but remained largely constant with the increase in isolation distance from major forests. Dung beetles are likewise more affected by forest fragmentation, and their number and species richness were positively associated with the extent of the fragments (Feer and Hingrat, 2005). Syrphid flies are the best tool for assessing landscape-level biodiversity due to their great adult mobility and species richness (Maleque et al., 2009). Furthermore, climate change will have a significant impact on increasing frequency, and severity of non-indigenous invasive insect species in forest ecosystem. Invasive species are introduced beyond their native regions to a completely new region of less or no natural enemies and capable of causing vast invasion owing to their great dispersal and adaptation ability. Invasion of insect species is a serious threat like emerald ash borer (Agrilus planipennis) causes severe destruction of ash trees (Herms and Mc Cullough, 2014), balsam wooly adegid (Adelges tsuga) infestation resulting in death of hemlock tree species (Havill et al., 2011), spread of gypsy moth (Lymantria dispar) causes Quercus sp. mortality (Davidson et al., 1999), and Asian long horned beetle (Anoplophora glabripennis) is responsible for the death of hardwood species of Acer, Salix, Aesculus, etc. (Dodds and Orwig, 2011) across the forests of eastern N. America.
6.3 Insect bioindicators of aquatic ecosystem
Ephemeroptera, Plecoptera, Trichoptera, and Diptera (EPT&D) are aquatic invertebrate insect species that are extremely sensitive to changes in water quality and are only able to survive in streams with very little pollution, cool temperatures, and well-oxygenated waters. Baetis and Paraleptophlebia (Ephemeroptera), and Chironomidae (Diptera) exhibit a considerable rise in abundance, and therefore density, immediately after an anthropogenic disturbance (Relyea et al., 2000). Insects are less typically utilized as biological indicators of metal contamination, despite species of the genus Halobates being suited to detect Cd and Hg (Nummelin et al., 2007). There are several benefits of using aquatic insects to biomonitor the health of aquatic ecosystems. One of these is the potential for detecting changes in water quality, both during sampling and changes that have occurred, as a result of these species’ largely sedentary lifestyles and extended life spans (Wahizatul et al., 2018).
6.4 Insect bioindicators of mountain ecosystem
In alpine regions, anthropogenic environmental degradation is still prevalent. According to their specific environmental tolerances, invertebrate communities are dispersed along with these sharp altitudinal gradients, and they respond accordingly when environmental conditions change. Only a few reliable invertebrate biomonitoring systems, particularly those which focus on terrestrial invertebrates, are regularly and extensively employed to track the changes in the mountain environment. Neophilaenus lineatus, a species of spittlebug, responds to shifting mean temperatures by exhibiting fast, often yearly changes in their upper altitudinal limit. Craspedolepta schwarzi, a species found at high elevations, may become extinct if suitable alpine habitats disappear. C. nebulosa and C. subpunctata, two lower-altitude species, may be anticipated to demonstrate an upward extension of their overlapping ranges, however, C. nebulosa always inhabits an elevation that is slightly higher than C. subpunctata (Hodkinson and Bird, 1998).
7 Conclusion
For environmental monitoring, indicator species are crucial as ecological indicators. The primary attributes and traits of a bioindicator include dependability, ecological faithfulness and fragility to tiny environmental changes, ease of handling, cost-effectiveness, species richness and variety, and ease of assessing environmental changes. The class Insecta has all of them. Insect serves as a crucial indication of changes in the quality of the soil, the air, and the water. Although it can be difficult to choose a particular indication and then recognize it, as well as the relationship between the indicators and their specialized uses, these modifications have an impact on many species’ physiological characteristics and abundance. This insect will be highly useful in the future as an indicator species because we can use it to detect pollution in the soil, water, and air. By doing so, we will be able to prevent habitat loss and reduce pollution in the future.
Author contributions
SC and VKD initiated and wrote the manuscript. All the authors reviewed the manuscript, contributed to consulting references, and agreed for publication.
Conflict of interest
The authors declare that the research was conducted in the absence of any commercial or financial relationships that could be construed as a potential conflict of interest.
Publisher’s note
All claims expressed in this article are solely those of the authors and do not necessarily represent those of their affiliated organizations, or those of the publisher, the editors and the reviewers. Any product that may be evaluated in this article, or claim that may be made by its manufacturer, is not guaranteed or endorsed by the publisher.
References
Aizawa, S., Kakuta, K., Akatsuka, M., Inoue, S., and Akaiwa, H. (1994). Special Articles: Environmental Sciences and Analytical Chemistry. Evaluation of Trichoptera as an indicator organism for environmental pollution by heavy metals. Jpn. Analy 43 (11), 865–871. doi:10.2116/bunsekikagaku.43.865
Akhila, A., and Keshamma, E. (2022). Recent perspectives on ants as bioindicators: A review. J. Entomol. Zool. Stud. 10 (3), 11–14. doi:10.22271/j.ento.2022.v10.i3a.9005
Al-Maliki, S., Al-Taey, D. K., and Al-Mammori, H. Z. (2021). Earthworms and eco-consequences: Considerations to soil biological indicators and plant function: A review. Acta Ecol. Sin. 41 (6), 512–523. doi:10.1016/j.chnaes.2021.02.003
Alajmi, R., Abdel-Gaber, R., and AlOtaibi, N. (2019). Characterization of the 12S rRNA gene sequences of the harvester termiteAnacanthotermes ochraceus (blattodea: Hodotermitidae) and its role as A bioindicator of heavy metal accumulation risks in Saudi arabia. Insects 10 (2), 51. doi:10.3390/insects10020051
Andersen, A. N., Hoffmann, B. D., Muller, W. J., and Griffiths, A. D. (2002). Using ants as bioindicators in land management: Simplifying assessment of ant community responses. J. Appl. Ecol. 39, 8–17. doi:10.1046/j.1365-2664.2002.00704.x
Anna, M., Łukasz, M., and Elżbieta, W. Ż. (2021). Hoverflies (syrphidae: Diptera) in areas contaminated with heavy metals (Cd, Zn, Pb). Folia Hortic. 33 (2), 325–342. doi:10.2478/fhort-2021-0025
Anonymous, (2023). Global monitioring division, Earth system research laboratory. Trends in Atmospheric Carbon Dioxide. Available at: http://www.esrl.noaa.gov/gmd/ccgg/trends/ (Accessed on December 31, 2022).
Araújo, C. D. O., Hortal, J., de Macedo, M. V., and Monteiro, R. F. (2022). Elevational and seasonal distribution of Scarabaeinae dung beetles (scarabaeidae: Coleoptera) at Itatiaia national park (Brazil). Int. J. Trop. Insect Sci. 42 (2), 1579–1592. doi:10.1007/s42690-021-00680-8
Arimoro, F. O., Auta, Y. I., Odume, O. N., Keke, U. N., and Mohammed, A. Z. (2018). Mouthpart deformities in Chironomidae (Diptera) as bioindicators of heavy metals pollution in Shiroro Lake, Niger State, Nigeria. Ecotoxicol. Environ. Saf. 149, 96–100. doi:10.1016/j.ecoenv.2017.10.074
Arndt, U. (1995). Air pollutants and pheromones—a problem? Chemosphere 30 (6), 1023–1031. doi:10.1016/0045-6535(95)00013.x
Azam, I., Afsheen, S., Zia, M., Javed, R., Saeed, M., Kaleem, S., et al. (2015). Evaluating insects as bioindicators of heavy metal contamination and accumulation near industrial area of Gujrat, Pakistan. Biomed. Res. Int. 2015, 1–11. doi:10.1155/2015/942751
Bale, J. S., Masters, G. J., Hodkinson, I. D., Awmack, C., Bezemer, T. M., Brown, V. K., et al. (2002). Herbivory in global climate change research: Direct effects of rising temperature on insect herbivores. Glob. Change Biol. 8, 1–16. doi:10.1046/j.1365-2486.2002.00451.x
Barganska, Z., Slebioda, M., and Namiessnik, J. (2016). Honey bees and their products Bioindicators of environmental contamination. Crit. Rev. Environ. Sci. Tech. 46 (3), 235–248. doi:10.1080/10643389.2015.1078220
Bartosova, M., Glovinova, E., and Povolny, D. (1997). Use of flesh-flies (Diptera, Sarcophagidae) for eco toxicological bioindication. Ekol. Bratisl. 16 (3), 319–322.
Bayley, M., Baatrup, E., Heimbach, U., and Bjerregaard, P. (1995). Elevated copper levels during larval development cause Altered locomotor behaviour in the adult carabid beetle Pterostichus Cupreus L. (copleoptera:carabidae). Ecotoxicol. Environ. Saf. 32, 166–170. doi:10.1006/eesa.1995.1098
Bellard, C., Bertelsmeier, C., Leadley, P., Thuiller, W., and Courchamp, F. (2012). Impacts of climate change on the future of biodiversity. Ecol. Lett. 15, 365–377. doi:10.1111/j.1461-0248.2011.01736.x
Blanckenhorn, W. U. (2018). “Behavioral, plastic, and evolutionary responses to a changing world,” in Insect behavior: From mechanisms to ecological and evolutionary consequences. Editors A. Córdoba-Aguilar, D. González-Tokman, and I. González-Santoyo (Oxford, U.K: Oxford University Press). doi:10.1093/oso/9780198797500.001.0001
Bohac, J. (1999). Staphylinid beetles as bioindicators. Agric. Ecosyst. Environ. 74, 357–372. doi:10.1016/S0167-8809(99)00043-2
Bokhorst, S., Huiskes, A., Convey, P., Van Bodegom, P. M., and Aerts, R. (2008). Climate change effects on soil arthropod communities from the Falkland Islands and the Maritime Antarctic. Soil Biol. biochem. 40, 1547–1556. doi:10.1016/j.soilbio.2008.01.017
Bouchard, P., Smith, A. B. T., Douglas, H., Gimmel, M. L., Brunke, A. J., and Kanda, K. (2017). Biodiversity of coleoptera. Insect Biodivers. 1, 337–417. doi:10.1002/9781118945568.ch11
Boullis, A., Detrain, C., Francis, F., and Verheggen, F. J. (2016). Will climate change affect insect pheromonal communication? Curr Opi Insect Sci 17, 87–91. doi:10.1016/j.cois.2016.08.006
Braby, M. F., and Williams, M. R. (2015). Biosystematics and conservation biology: Critical scientific disciplines for the management of insect biological diversity. Austr. Entomol. 55 (1), 1–17. doi:10.1111/aen.12158
Brock, R. E., Cini, A., and Sumner, S. (2021). Ecosystem services provided by aculeate wasps. Biol. Rev. 96 (4), 1645–1675. doi:10.1111/brv.12719
Brodersen, K. P., and Lindegaard, C. (1997). Significance of subfossil chironomid remains in classification of shallow lakes. Hydrobiologia 342, 125–132. doi:10.1023/a:1017089912582
Burgio, G., Sommaggio, D., Marini, M., Puppi, G., Chiarucci, A., Landi, S., et al. (2015). The influence of vegetation and land scape structural connectivity on butterflies (Lepidoptera: Papilionoidea and Hesperiidae), carabids (Coleoptera: Carabidae), syrphids (Diptera: Syrphidae), and sawflies (Hymenoptera: Symphyta) in Northern Italy farmland. Environ. Entomol. 44 (5), 1299–1307. doi:10.1093/ee/nvv105
Cañedo-Argüelles, M., Bogan, M. T., Lytle, D. A., and Prat, N. (2016). Are Chironomidae (Diptera) good indicators of water scarcity? Dryland streams as a case study. Ecol. Indic. 71, 155–162. doi:10.1016/j.ecolind.2016.07.002
Cavaliere, F., Brandmayr, P., and Giglio, A. (2019). DNA damage in haemocytes of Harpalus (Pseudophonus) rufipes (De Geer, 1774) (Coleoptera, Carabidae) as an indicator of sublethal effects of exposure to herbicides. Ecol. Indic. 98, 88–91. doi:10.1016/j.ecolind.2018.10.055
Colares, C., Roza, A. S., Mermudes, J. R., Silveira, L. F., Khattar, G., Mayhew, P. J., et al. (2021). Elevational specialization and the monitoring of the effects of climate change in insects: Beetles in a Brazilian rainforest mountain. Ecol. Indic. 120, 106888. doi:10.1016/j.ecolind.2020.106888
Conti, E., Dattilo, S., Costa, G., and Concetto, P. (2017). The ground beetle Parallelomorphus laevigatus is a potential indicator of trace metal contamination on the eastern coast of Sicily. Ecotoxicol. Environ. Saf. 135, 183–190. doi:10.1016/j.ecoenv.2016.09.029
Cunningham, M. M., Tran, L., Mc Kee, C. G., Polo, R. O., Newman, T., Lansing, L., et al. (2022). Honey bees as biomonitors of environmental contaminants, pathogens, and climate change. Ecol. Indic. 134, 108457. doi:10.1016/j.ecolind.2021.108457
Da Rocha, J. R. M., De Almeida, J. R., Lins, G. A., and Durval, A. (2010). Insects as indicators of environmental changing and pollution: A review of appropriate species and their monitoring. Holos Environ. 10 (2), 250–262. doi:10.14295/holos.v10i2.2996
David, T. (1989). Bio-indicator in air pollution research. Application and Constraints biologic markers of air pollution stress and damage in forests. Washington, DC: Nation Academics Press, 73–80.
Davidson, C. B., Gottschalk, K. W., and Johnson, J. E. (1999). Tree mortality following defoliation by the European gypsy moth (Lymantrian dipar L.) in the United States: A review. For. Sci. 45 (1), 74–84. doi:10.1093/forestscience/45.1.74
De Groot, R. S., Wilson, M. A., and Boumans, R. M. J. (2002). A typology for the classification, description and valuation of ecosystem functions, goods and services. Ecol. Econ. 41, 393–408. doi:10.1016/S0921-8009(02)00089-7
Di Fiore, C., Nuzzo, A., Torino, V., De Cristofaro, A., Notardonato, I., Passarella, S., et al. (2022). Honey bees as bioindicators of heavy metal pollution in urban and rural areas in the south of Italy. Atmosphere 13 (4), 624. doi:10.3390/atmos13040624
Dodds, K. J., and Orwig, D. A. (2011). An invasive urban forest pest invades natural environments-Asian long horned beetle in northeastern US hardwood forests. Canad. J. For. Res. 41 (9), 1729–1742. doi:10.1139/x11-097
Dokulil, M. T. (2003). in Algae as biological bioindicator. Bioindicators and Biomonitors. Editors B. A. Markert, A. M. Breure, and H. G. Zechmeister (Elsevier Sci. LTD). The Boulevard, Langford Lane Kidlington, Oxford PX5 1GB, UKISBN: 9780080527970.
Duran-Bautista, E. H., Armbrecht, I., Acioli, A. N. S., Suárez, J. C., Romero, M., Quintero, M., et al. (2020). Termites as indicators of soil ecosystem services in transformed amazon landscapes. Ecol. Indic. 117, 106550. doi:10.1016/j.ecolind.2020.106550
Faith, D. P., and Walker, P. A. (1996). Environmental diversity: On the best-possible use of surrogate data for assessing the relative biodiversity of sets of areas. Biodivers. Conserv. 5, 399–415. doi:10.1007/bf00056387
Feer, F., and Hingrat, Y. (2005). Effects of forest fragmentation on a dung beetle community in French Guiana. Conserv. Biol. 19, 1103–1112. doi:10.1111/j.1523-1739.2005.00087.x
Feldhaar, H., and Otti, O. (2020). Pollutants and their interaction with diseases of social Hymenoptera. Insects 11 (3), 153. doi:10.3390/insects11030153
Fernandez-alaez, C., Soto, J., Fernandez-alaez, M., and Garcia-criado, F. (2002). Spatial structure of the caddisfly (Insecta, Trichoptera) communities in a river basin in NW Spain affected by coal mining. Hydrobiologia 487, 193–205. doi:10.1023/A:1022932515761
Field, C. B., Barros, V. R., Dokken, D. J., Mach, K. J., Mastrandrea, M. D., Bilir, T. E., et al. (2014). “IPCC Summary for policymakers,” in Climate change 2014: Impacts, adaptation, and vulnerability, Part A: Global and sectoral aspects; contribution of working group II to the fifth assessment report of the intergovernmental Panel on climate change (Cambridge, UK; New York, NY, USA: Cambridge University Press), 1–32. ISBN 978-92-9169-143-2.
Frampton, G. K., and Van den Brink, P. J. (2007). Collembola and macroarthropod community responses to carbamate, organophosphate and synthetic pyrethroid insecticides: Direct and indirect effects. Environ. Pollut. 147 (1), 14–25. doi:10.1016/j.envpol.2006.08.038
Fujita, A., Maeto, K., Kagawa, Y., and Ito, N. (2008). Effects of forest fragmentation on species richness and composition of ground beetles (Coleoptera: Carabidae and Brachinidae) in urban landscapes. Entomol. Sci. 11, 39–48. doi:10.1111/j.1479-8298.2007.00243.x
Furlan, C. M., Moraes, R. M., Bulbovas, P., Domingos, M., Salatino, A., and Sanz, M. J. (2007). Psidium guajava ‘Paluma’ (the guava plant) as a new bio-indicator of ozone in the tropics. Environ. Pollut. 147 (3), 691–695. doi:10.1016/j.envpol.2006.09.014
Gerhardt, A. (2002). Bioindicator species and their use in biomonitoring. Environ. Monit. 1, 77–123.
Gerlach, J., Samways, M., and Pryke, J. (2013). Terrestrial invertebrates as bioindicators: An overview of available taxonomic groups. J. Insect Conserv. 17 (4), 831–850. doi:10.1007/s10841-013-9565-9
Ghannem, S., Touaylia, S., and Boumaiza, M. (2018). Beetles (Insecta: Coleoptera) as bioindicators of the assessment of environmental pollution. Hum. Ecol. Risk Assess. 24 (2), 456–464. doi:10.1080/10807039.2017.1385387
Gramigni, E., Calusi, S., Gelli, N., Giuntini, L., Massi, M., Delfino, G., et al. (2013). Ants as bioaccumulators of metals from soil: Body content and tissue-specific distribution of metals in the ant, Crematogaster scutellaris. Eur. J. Soil Biol. 58, 24–31. doi:10.1016/j.ejsobi.2013.05.006
Grimm, N. B., Chapin, F. S., Bierwagen, B., Gonzalez, P., Groffman, P. M., Luo, Y., et al. (2013). The impacts of climate change on ecosystem structure and function. Front. Ecol. Environ. 11 (9), 474–482. doi:10.1890/120282
Groot, R. S., Ketner, P., and Ovaah, A. H. (1995). Selection and use of bio-indicators to assess the possible effects of climate change in Europe. J. Biogeo. 22, 935–943. doi:10.2307/2845994
Hallock, P., Lidz, B. H., Cockey-Burkhard, E. M., and Donnelly, K. B. (2003). “Foraminifera as bioindicators in coral reef assessment and monitoring: The FORAM index,” in Coastal monitoring through partnerships (Dordrecht: Springer), 38–221.
Han, Y. G., Kwon, O., and Cho, Y. (2015). A study of bioindicator selection for long-term ecological monitoring. J. Ecol. Environ. 38 (1), 119–122. doi:10.5141/ecoenv.2015.013
Havill, N. P., Montgomery, M. E., Keena, M., Onken, B., and Reardon, R. (2011). Hemlock woolly adelgid and its hemlock hosts: A global perspective. Implementation and status of biological control of the hemlock woolly adelgid. Morgantown, WV: US Forest Service, 3–14.
Heliövaara, K., and Väisänen, R. (1990). Heavy-metal contents in pupae of Bupalus piniarus (Lepidoptera: Geometridae) and Panolis flammea (Lepidoptera: Noctuidae) near an industrial source. Environ. Entomol. 19, 481–485. doi:10.1093/ee/19.3.481
Heliövaara, K., Väisänen, R., and Kemppi, E. (1989). Change of pupal size of Panolis flammea (Lepidoptera; Noctuidae) and Bupalus piniarius (Geometridae) in response to concentration of industrial pollutants in their food plant. Oecologia 79 (2), 179–183. doi:10.1007/bf00388475
Hendrickx, F., Van Winggerden, W., Maelfait, J.- P., Bugter, R. J. F., Speelmans, M., Aviron, S., et al. (2007). How landscape structure, land-use intensity and habitat diversity affect components of total arthropod diversity in agricultural landscapes. J. Appl. Ecol. 44 (2), 340–351. doi:10.1111/j.1365-2664.2006.01270.x
Herms, D. A., and Mc Cullough, D. G. (2014). Emerald ash borer invasion of north America: History, biology, ecology, impacts, and management. Ann. Rev. Entomol. 59, 13–30. doi:10.1146/annurev-ento-011613-162051
Hickling, R., Roy, D. B., Hill, J. K., Fox, R., and Thomas, C. D. (2006). The distributions of a wide range of taxonomic groups are expanding polewards. Glob. Chang. Boil. 12 (3), 450–455. doi:10.1111/j.1365-2486.2006.01116.x
Hilszczanski, J., Gibb, H., €alten, J., Atlegrim, O., Johansson, T., Pettersson, R. B., et al. (2005). Parasitoids (Hymenoptera, Ichneumonoidea) of saproxylic beetles are affected by forest successional stage and deadwood characteristics in boreal spruce forest. Biol. Conserv. 126 (4), 456–464. doi:10.1016/j.biocon.2005.06.026
Hilty, J., and Merenlender, A. (2000). Faunal indicator taxa selection for monitoring ecosystem health. Biol. Conserve. 92 (2), 185–197. doi:10.1016/S0006-3207(99)00052-X
Hodkinson, I. D., and Bird, J. M. (1998). Host-specific insect herbivores as sensors of climate change in Arctic and alpine environments. Arc. Alp. Res. 30, 78–83. doi:10.2307/1551747
Holt, E. A., and Miller, S. W. (2010). Bioindicators: Using organisms to measure environmental impacts. Nature 3 (10), 8–13.
IPCC (2022). IPCC. Available at: https://www.ipcc.ch/.
Jain, A., Singh, B. N., Singh, S. P., Singh, H. B., and Singh, S. (2010). “Exploring biodiversity as bioindicators for water pollution,” in National Conference on Biodiversity, Development and Poverty Alleviation, Uttar Pradesh. Lucknow (India), 2010 May 22 (Uttar Pradesh State Biodiversity Board).
Jardine, T. D., Al, T. A., MacQuarrie, K. T. B., Ritchie, C. D., Arp, P. A., Maprani, A., et al. (2005). Water striders (family Gerridae): Mercury sentinels in small freshwater ecosystems. Environ. Pollut. 134, 165–171. doi:10.1016/j.envpol.2004.07.006
John, T. P., and Polhemus, D. A. (2008). Global diversity of true bugs (Heteroptera; Insecta) in freshwater. Hydrobiologia 595 (1), 379–391. doi:10.1007/s10750-007-9033-1
Kaspari, M., and Majer, J. D. (2000). “Using ants to monitor environmental changes,” in Ants: Standard Methods for Measuring and Monitoring Biodiversity. Biological Diversity Handbook Series.Editor D. Agosti, J. Majer, E. Alonso, and T. Schultz(Washington, DC: Smithsonian Institution Press), 20+280. Available at: http://hdl.handle.net/20.500.11937/32656.
Khan, S. R., Singh, S. K., and Rastogi, N. (2017). Heavy metal accumulation and ecosystem engineering by two common mine site-nesting ant species: Implications for pollution-level assessment and bioremediation of coal mine soil. Environ. Monit. Assess. 189 (195), 195–219. doi:10.1007/s10661-017-5865-y
Khatri, N., and Tyagi, S. (2015). Influences of natural and anthropogenic factors on surface and groundwater quality in rural and urban areas. Front. Life Sci. 8 (1), 23–39. doi:10.1080/21553769.2014.933716
Kim, K. C. (1993). Biodiversity, conservation and inventory: Why insects matter. Biodivers. Conserv. 2 (3), 191–214. doi:10.1007/bf00056668
Kopeszki, H. (1997). An active bioindication method for the diagnosis of soil properties using Collembola. Pedobiologia 41, 159–166. Available at: https://www.webofscience.com/wos/WOSCC/full-record/A1997XH07200027.
Kozlov, M. V., Castagneyrol, B., Zverev, V., and Zvereva, E. L. (2022). Recovery of moth and butterfly (Lepidoptera) communities in a polluted region following emission decline. Sci. Total Environ. 838, 155800. doi:10.1016/j.scitotenv.2022.155800
Kumar, S., Joshi, P. C., Nath, P., Awasthi, S., Kumar Singh, V., and Dalip, K. M. (2015). Insects as bio-indicators of environmental pollution. Int. J. Environ. Sci. 1 (1), 19–21.
Kyerematen, R., Kaiwa, F., Acquah-Lamptey, D., Adu-Acheampong, S., and Andersen, R. S. (2018). Butterfly assemblages of two wetlands: Response of biodiversity to different environmental stressors in Sierra Leone. Open J. Ecol. 8, 379–395. doi:10.4236/oje.2018.87023
Lagisz, M. (2008). Changes in morphology of the Ground beetle Pterostichus oblongopunctatus Fab. (coleoptera; carabidae) from vicinities of a zinc and lead smelter. Environ. Toxicol. Chem. 27 (8), 1744–1747. doi:10.1897/07-661.1
Lemmen, J., and Evenden, M. (2015). Environmental conditions terminate reproductive diapause and influence pheromone perception in the long-lived moth C. aloptilia fraxinella. Physiol. Entomol. 40 (1), 30–42. doi:10.1111/phen.12086
Lenoir, A., Touchard, A., Devers, S., Christides, J. P., Boulay, R., and Cuvillier Hot, V. (2014). Ant cuticular response to phthalate pollution. Environ. Sci. Pollut. Res. 21 (23), 13446–13451. doi:10.1007/s11356-014-3272-2
Lindenmayer, D. B., Margules, C. R., and Botkin, D. B. (2000). Indicators of biodiversity for ecologically sustainable forest management. Conserv. Biol. 14 (4), 941–950. doi:10.1046/j.1523-1739.2000.98533.x
Liu, M., Xu, J., Krogh, P. H., Song, J., Wu, L., Luo, Y., et al. (2018). Assessment of toxicity of heavy metal-contaminated soils toward Collembola in the paddy fields supported by laboratory tests. Environ. Sci. Pollut. Res. 25, 16969–16978. doi:10.1007/s11356-018-1864-y
Ma, C. S., Zhang, W., Peng, Y., Zhao, F., Chang, X. Q., Xing, K., et al. (2021). Climate warming promotes pesticide resistance through expanding overwintering range of a global pest. Nat. Comm. 12 (1), 5351. doi:10.1038/s41467-021-25505-7
Machado, J. S., Oliveira, F. L., Sants, J. C., Paulino, A. T., and Baretta, D. (2019). Morphological diversity of springtails (Hexapoda: Collembola) as soil quality bioindicators in land use systems. Biota Neo Trop. 19 (1), e20180610. doi:10.1590/1676-0611-BN-2018-0618
Mahlman, J. D. (1997). Uncertainties in projections of human-caused climate warming. Sci 278 (5342), 1416–1417. doi:10.1126/science.278.5342.1416
Majer, J. D. (1983). Ants: Bio-indicators of minesite rehabilitation, land-use, and land conservation. Environ. Manag. 7 (4), 375–383. doi:10.1007/bf01866920
Maleque, M. A., Maeto, K., and Ishii, H. T. (2009). Arthropods as bioindicators of sustainable forest management, with a focus on plantation forests. Appl. Entomol. Zoolo. 44 (1), 1–11. doi:10.1303/aez.2009.1
Markova, E., and Alexiev, A. (2002). “Bioaccumulation of heavy metals in syrphid flies (Syrphidae, Diptera). In Study, conservation and utilisation of forest resources,” in Proceedings of the Third Balkan Scientific Conference, Sofia, Bulgaria, 2-6 October 2001 (Forest Research Institute) Volume III, 151–157.
Mason, L. M., Eagar, A., Patel, P., Blackwood, C. B., and DeForest, J. L. (2020). Potential microbial bioindicators of phosphorus mining in a temperate deciduous forest. J. Appl. Microbiol. 130, 109–122. doi:10.1111/jam.14761
Mc Geoch, M. (1998). The selection, testing and application of terrestrial insects as bioindicators. Biol. Rev. 73, 181–201. doi:10.1111/j.1469-185X.1997.tb00029.x
Menéndez, R., González-Megías, A. G., Hill, J. K., Braschler, B., Willis, S. G., Collingham, Y., et al. (2006). Species richness changes lag behind climate change. Proc. R. Soc. Lond. B 273, 1465–1470. doi:10.1098/rspb.2006.3484
Menéndez, R., González-Megías, A., Jay-Robert, P., and Marquéz-Ferrando, R. (2014). Climate change and elevational range shifts: Evidence from dung beetles in two European mountain ranges. Glob. Ecol. Biogeogr. 23, 646–657. doi:10.1111/geb.12142
Menéndez, R. (2007). How are insects responding to global warming? Tijdschr. Entomol. 150, 355–365. Available at: https://www.nev.nl/tve/pdf/te0150355 (Accessed December 28, 2022).
Michailova, P., Sella, G., and Petrova, N. (2012). Chironomids (Diptera) and their salivary gland chromosomes as indicators of trace-metal genotoxicity. Ital. J. Zool. 79 (2), 218–230. doi:10.1080/11250003.2011.622084
Mousavi, S. K. (2002). Boreal chironomid communities and their relations to environmental factors- the impact of lake depth, size and acidity. Boreal Environ. Res. 7, 63–75.
Mozūraitis, R., and Būda, V. (2006). Pheromone release behaviour in females of Phyllonorycter junoniella (Z.) (Lepidoptera, Gracillariidae) under constant and cycling temperatures. J. Insect Behav. 19, 129–142. doi:10.1007/s10905-005-9000-5
Niemela, J., Langor, D., and Spence, J. R. (1993). Effects of clear-cut harvesting on boreal groundbeetle assemblages (Coleoptera, Carabidae) in Western Canada. Conserv. Biol. 7 (3), 551–561. doi:10.1046/j.1523-1739.1993.07030551.x
Nithyatharani, R., and Kavitha, U. S. (2018). Termite soil as bio-indicator of soil fertility. Int. J. Res. Appl. Sci. Eng. Technol. 6 (1), 659–661. doi:10.22214/ijraset.2018.1099
Noss, R. F. (1990). Indicators for monitoring biodiversity: A hier-archical approach. Conserv. Biol. 4, 355–364. doi:10.1111/j.1523-1739.1990.tb00309.x
Nummelin, M., Lodenius, M., Tulisali, E., Hirvonen, H., and Alanko, T. (2007). Predatory insects as bioindicators of heavy metal pollution. Environ. Pollut. 145, 339–347. doi:10.1016/j.envpol.2006.03.002
Nunes, L. A., Edilson Divino de Araújo, E. D., and Marchini, L. C. (2015). Fluctuating asymmetry in Apis mellifera (Hymenoptera: Apidae) as bioindicator of anthropogenic environments. Rev. Biol. Trop. Int. J. Trop. Biol.) 63 (3), 673–682. doi:10.15517/rbt.v63i3.15869
Ode, P. J., Johnson, S. N., and Moore, B. D. (2014). Atmospheric change and induced plant secondary metabolites—Are we reshaping the building blocks of multi-trophic interactions? Curr. Opi. Insect Sci. 5, 57–65. doi:10.1016/j.cois.2014.09.006
Oliveira, M. A., Gomes, C. F. F., Pires, E. M., Marinho, C. G. S., and Della-Lucia, T. M. C. (2014). Bioindicadores ambientais: Insetos como um instrumento desta avaliação. Rev. Ceres 61, 800–807. doi:10.1590/0034-737X201461000005
Owen, E. L., Bale, J. S., and Hayward, S. A. L. (2013). Can winter-active bumblebees survive the cold? Assessing the cold tolerance of bombus terrestris audax and the effects of pollen feeding. PLoS One 8, e80061. doi:10.1371/journal.pone.0080061
Owens, A. C., Cochard, P., Durrant, J., Farnworth, B., Perkin, E. K., and Seymoure, B. (2020). Light pollution is a driver of insect declines. Biol. Cons. 241, 108259. doi:10.1016/j.biocon.2019.108259
Pachauari, R. K., and Reisinger, A. (2007). Climate change: Synthesis report. Contribution of working groups I, II and III to the fourth assessment report on intergovernmental Panel on climate change. Geneva, Switzerland: Intergovernmental Panel on Climate Change. ISBN 92-9169-122-4.
Parikh, G., Rawtani, D., and Khatri, N. (2020). Insects as an indicator for environmental pollution. Environ. Claims J. 33 (2), 161–181. doi:10.1080/10406026.2020.1780698
Parmar, T. K., Rawtani, D., and Agrawal, Y. K. (2016). Bioindicators: The natural indicator of environmental pollution. Front. Life Sci. 9 (2), 110–118. doi:10.1080/21553769.2016.1162753
Parmesan, C., and Yohe, G. (2003). A globally coherent fingerprint of climate change impacts across natural systems. Nature 521, 37–42. doi:10.1038/nature01286
Pereira, L. R., Cabette, H. S., and Juen, L. (2012). Trichoptera as bioindicators of habitat integrity in the Pindaíba river basin, Mato Grosso (Central Brazil). Ann. Limnologie-Int. Limnol. 48 (3), 295–302. doi:10.1051/limn/2012018
Porrini, C., Sabatini, A. G., Girotti, S., Ghini, S., Medrzycki, P., Grillenzoni, F., et al. (2003). Honey bees and bee products as monitors of the environmental contamination. Apiacta 38, 63–70.
Prentice, I. C., Farquhar, G. D., Fasham, M. J. R., Goulden, M. L., Heimann, M., Jaramillo, V. J., et al. (2001). “The carbon cycle and atmospheric carbon dioxide,” in Climate change 2001: The scientific basis. Editors J. T. Houghton, Y. Ding, D. J. Griggs, M. Noguer, P. J. van der Linden, X. Daiet al. (New York, NY, USA: Cambridge University Press), 185–237. ISBN 0521807670.
Quigley, T. P., Amdam, G. V., and Harwood, G. H. (2019). Honey bees as bioindicators of changing global agricultural landscapes. Curr. Opi. Insect Sci. 35, 132–137. doi:10.1016/j.cois.2019.08.012
Rainio, J., and Niemela, J. (2003). Ground beetles (Coleoptera: Carabidae) as bioindicators. Biodiv. Cons. 12 (3), 487–506. doi:10.1023/a:1022412617568
Relyea, C. D., Minshall, G. W., and Danehy, R. J. (2000). Stream insects as bioindicators of fine sediment. Proc. Water Environ. Fed. 2000 (6), 663–686. doi:10.2175/193864700785150123
Roeser-Mueller, K., Strohm, E., and Kaltenpoth, M. (2010). Larval rearing temperature influences amount and composition of the marking pheromone of the male beewolf, Philanthus triangulum. J. Insect Sci. 10 (1), 1–16. doi:10.1673/031.010.7401
Rogelj, J. D., Shindell, K., Jiang, S., Fifita, P., Forster, V., Ginzburg, C., et al. (2018). “Mitigation pathways compatible with 1.5 ◦C in the context of sustainable development global warming of 1.5 ◦C. An IPCC special report on the impacts of global warming of 1.5 ◦C above pre-industrial levels and related global greenhouse gas emission pathways,” in The context of strengthening the global response to the threat of climate change, sustainable de-velopment, and efforts to eradicate poverty. Editors V. P. Masson-Delmotte, H.-O. Zhai, D. Pörtner, J. Roberts, P. R. Skea, A. Shuklaet al. (Geneva, Switzerland: WMO). ISBN 978-92-9169-151-7.
Roitman, S., Joseph Pollock, F., and Medina, M. (2018). “Coral microbiomes as bioindicators of reef health,” in Population genomics: Marine organisms (Cham: Springer), 39–57. doi:10.1007/13836_2018_29
Root, T. L., Price, J. T., Hall, K. R., Schneider, C. R., and Pounds, J. A. (2003). Fingerprints of global warming on wild animals and plants. Nature 421, 57–60. doi:10.1038/nature01333
Roshchina, V. V., and Roshchina, V. (2003). Ozone in plant cell: Indication of Damages and sensitivity of model system. ISBN: 978-90-481-6340-3. doi:10.1007/978-94-017-2523-1
Rusek, J. (1998). Biodiversity of Collembola and their functional role in the ecosystem. Biodivers. Conserv. 7, 1207–1219. doi:10.1023/a:1008887817883
Sánchez-Bayo, F., and Wyckhuys, K. A. (2019). Worldwide decline of the entomofauna: A review of its drivers. Biol. Cons. 232, 8–27. doi:10.1016/j.biocon.2019.01.020
Santolamazza-Carbone, S., Pestaña Nieto, M., Pérez Otero, R., Mansilla Vázquez, P., and Cordero Rivera, A. (2009). Winter and spring ecology of Anaphes nitens, a solitary egg-parasitoid of the Eucalyptus snout-beetle Gonipterus scutellatus. Bio Cont. 54, 195–209. doi:10.1007/s10526-008-9184-7
Schweiger, O., Maelfait, J. P., Van Wingerden, W., Hendrickx, F., Billeter, R., Speelmans, M., et al. (2005). Quantifying the impact of environmental factors on arthropod communities in agricultural landscapes across organizational levels and spatial scales. J. Appl. Ecol. 42 (6), 1129–1139. doi:10.1111/j.1365-2664.2005.01085.x
Sentis, A., Ramon-Portugal, F., Brodeur, J., and Hemptinne, J. L. (2015). The smell of change: Warming affects species interactions mediated by chemical information. Glob. Change Biol. 21 (10), 3586–3594. doi:10.1111/gcb.12932
Shafie, M., Wong, A., Harun, S., and Fikri, A. H. (2017). The use of aquatic insects as bioindicator to monitor freshwater stream health of Liwagu River, Sabah, Malaysia. J. Entomol. Zool. Stud. 5 (4), 1662–1666.
Sharma, M., and Sharma, N. (2017). Suitability of butterflies as indicators of ecosystem condition: A comparison of butterfly diversity across four habitats in gir wildlife sanctuary. Int. J. Advan. Res. Biol. Sci. 4, 2348–8069. doi:10.22192/ijarbs.2017.04.03.005
Sharma, T., and Singla, A. (2021). Potential impacts of climate change on insect behavior. Research & reviews. A. J. Life Sci. 11 (3), 29–35. doi:10.37591/RRJoLS
Sheldon, K. S. (2019). Climate change in the tropics: Ecological and evolutionary responses at low latitudes. Ann. Rev. Ecol. Evol. Syst. 50, 303–333. doi:10.1146/annurev-ecolsys-110218-025005
Shrestha, S. (2019). Effects of climate change in agricultural insect pest. Acta Sci. Agric. 3 (12), 74–80. doi:10.31080/ASAG.2019.03.0727
Simon, E., Harangi, S., Baranyai, E., Braun, M., Fabian, I., Mizser, S., et al. (2016). Distribution of toxic elements between biotic and abiotic components of terrestrial ecosystem along an urbanization gradient: Soil, leaf litter and ground beetles. Ecol. Indic. 60, 258–264. doi:10.1016/j.ecolind.2015.06.045
Singare, P. U., and Talpade, M. S. (2013). Physiological responses of some plant species as a bio-indicator of roadside automobile pollution stress using the air pollution tolerance index approach. Int. J. Plant Res. 3 (2), 9–16. doi:10.5923/j.plant.20130302.01
Skaldina, O., and Sorvari, J. (2019). “Ecotoxicological effects of heavy metal pollution on economically important terrestrial insects,” in Environmental science and engineering (Netherlands: Springer). doi:10.1007/978-3-319-96511-6_7
Skaldina, O., Peraniemi, S., and Sorvari, J. (2018). Ants and their nests as indicators for industrial heavy metal contamination. Environ. Pollut. 240, 574–581. doi:10.1016/j.envpol.2018.04.134
Sommaggio, D. (1999). Syrphidae: Can they be used as environmental bioindicators? Agricul. Ecosys. Environ. 74 (1–3), 343–356. doi:10.1016/S0167-8809(99)00042-0
Srivastava, S., Dash, H. R., and Das, S. (2017). Assessment of the biological quality of riverine water using pathogenicity islands (PAIs) of coliform bacteria as pollution indicator. Water Resou 44 (1), 150–157. doi:10.1134/s0097807817010146
Steiner, W. A. (1995). Influence of air-pollution on moss dwelling animals. Terrestrial fauna, with emphasis on Oribatida and Collembola. Acarologia 36, 149–173.
Stewart, A. J., New, T. R., and Lewis, O. T. (Editors) (2007). Insect conservation biology: Proceedings of the royal entomological society's 23nd symposium (CABI), 1–473.
Streck, N. A. (2005). Climate change and agro ecosystems: The effect of elevated atmospheric CO2 and temperature on crop growth, development, and yield. Ciênc. Rural. 35, 730–740. doi:10.1590/S0103-84782005000300041
Thakur, R. K., Jindal, R., Singh, U. B., and Ahluwalia, A. S. (2013). Plankton diversity and water quality assessment of three freshwater lakes of Mandi (Himachal Pradesh, India) with special reference to planktonic indicators. Environ. Monit. Assess. 185, 8355–8373. doi:10.1007/s10661-013-3178-3
Tibcherani, M., Nacagava, V., Aranda, R., and Mello, R. L. (2018). Review of ants (hymenoptera: Formicidae) as bioindicators in the Brazilian savanna. Sociobio 65 (2), 112–129. doi:10.13102/sociobiology.v65i2.2048
Underwood, E. C., and Fisher, B. L. (2006). The role of ants in conservation monitoring: If, when, and how. Biol. Conserv. 132, 166–182. doi:10.1016/j.biocon.2006.03.022
Uttah, E. C., Uttah, C., Akpan, P. A., Ikpeme, E. M., Ogbeche, J., Usip, J. O., et al. (2008). Bio-survey of plankton as indicators of water quality for recreational activities in Calabar River, Nigeria. J. Appl. Sci. Environ. Manag. 12 (2), 35–42. doi:10.4314/jasem.v12i2.55525
Wahizatul, A. A., Nur, H. H., and Nakisah, M. A. (2018). Monitoring of water quality using aquatic insects as biological indicators in three streams of Terengganu. J. Sustain. Sci. Manag. 13, 67–76.
Walther, G. R., Post, E., Convey, P., Menzel, A., Parmesan, C., Beebee, T. J. C., et al. (2002). Ecological responses to recent climate change. Nature 416, 389–395. doi:10.1038/416389a
Wang, Y. Z., Cao, C. Q., and Wang, D. (2022). Physiological responses of the firefly Pyrocoelia analis (Coleoptera: Lampyridae) to an environmental residue from chemical pesticide imidacloprid. Front. Physiol. 13, 879216. doi:10.3389/fphys.2022.879216
Warwick, W. F. (1990). The use of morphological deformities in Chironomid larvae for biological effects monitoring. Inland Waters Dir. Sci. Publ. Ser. 173, 1–34.
Weiss, S. B., Murphy, D. D., and White, R. R. (1988). Sun, slope, and butterflies: Topographic determinants of habitat quality for euphydryas editha. Ecol 69, 1486–1496. doi:10.2307/1941646
Welti, E. A., Roeder, K. A., de Beurs, K. M., Joern, A., and Kaspari, M. (2020). Nutrient dilution and climate cycles underlie declines in a dominant insect herbivore. Proc. Nat. Acad. Sci. 117 (13), 7271–7275. doi:10.1073/pnas.1920012117
Wilson, R., Davies, Z., and Thomas, C. (2007). “Insects and climate change: Processes, patterns and implications for conservation,” in Insect conservation biology. Proceedings of the royal entomological society's 23rd symposium. Editors A. J. A. Stewart, T. R. New, and O. T. Lewis (Wallingford: CABI Publishing), 245–279.
Winner, R. W., Boesel, M. W., and Farrell, M. P. (1980). Insect community structure as an index of heavy-metal pollution in lotic ecosystems. Can. J. Fish. Aqu. Sci. 37 (4), 647–655. doi:10.1139/f80-081
Yang, L. H., Postema, E. G., Hayes, T. E., Lippey, M. K., and MacArthur-Waltz, D. J. (2021). The complexity of global change and its effects on insects. Curr. Opi. Insect Sci. 47, 90–102. doi:10.1016/j.cois.2021.05.001
Yoro, K. O., and Daramola, M. O. (2020). “CO2 emission sources, greenhouse gases, and the global warming effect,” in Advances in carbon capture. Editors M. R. Rahimpour, M. Farsi, and M. A. Makarem (Sawston, UK: Woodhead Publishing), 3–28. ISBN 9780128196571.
Zaghloul, A., Saber, M., Gadow, S., and Awad, F. (2020). Biological indicators for pollution detection in terrestrial and aquatic ecosystems. Bulletin of the National Research Centre, 44–127.
Keywords: insects, monitoring, climate change, environmental pollutants, ecosystem, bioindicator
Citation: Chowdhury S, Dubey VK, Choudhury S, Das A, Jeengar D, Sujatha B, Kumar A, Kumar N, Semwal A and Kumar V (2023) Insects as bioindicator: A hidden gem for environmental monitoring. Front. Environ. Sci. 11:1146052. doi: 10.3389/fenvs.2023.1146052
Received: 16 January 2023; Accepted: 20 February 2023;
Published: 02 March 2023.
Edited by:
Sudhir Kumar Pandey, Guru Ghasidas Vishwavidyalaya, IndiaReviewed by:
Jose-Luis Martinez-Guitarte, National University of Distance Education (UNED), SpainConstantin Nechita, National Institute for research and Development in Forestry Marin Dracea (INCDS), Romania
Copyright © 2023 Chowdhury, Dubey, Choudhury, Das, Jeengar, Sujatha, Kumar, Kumar, Semwal and Kumar. This is an open-access article distributed under the terms of the Creative Commons Attribution License (CC BY). The use, distribution or reproduction in other forums is permitted, provided the original author(s) and the copyright owner(s) are credited and that the original publication in this journal is cited, in accordance with accepted academic practice. No use, distribution or reproduction is permitted which does not comply with these terms.
*Correspondence: Vinod Kumar Dubey, dmlub2RrdW1hcmR1YmV5NDJAZ21haWwuY29t