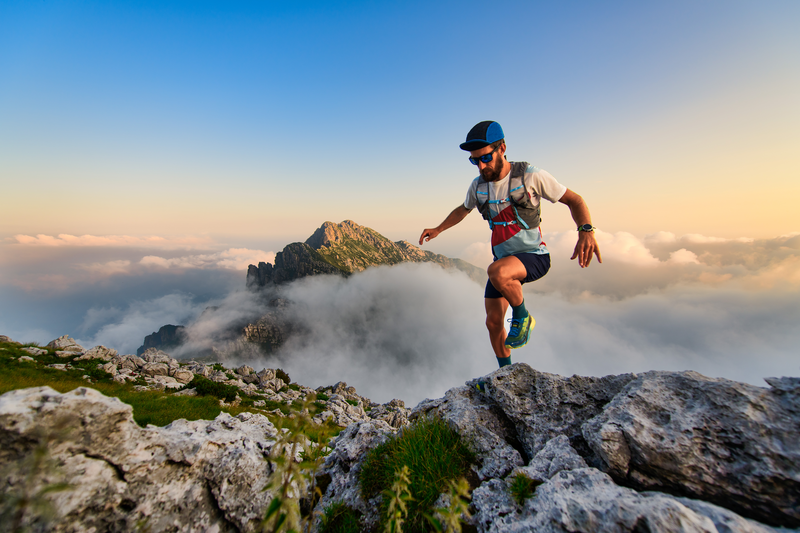
94% of researchers rate our articles as excellent or good
Learn more about the work of our research integrity team to safeguard the quality of each article we publish.
Find out more
REVIEW article
Front. Environ. Sci. , 07 April 2023
Sec. Toxicology, Pollution and the Environment
Volume 11 - 2023 | https://doi.org/10.3389/fenvs.2023.1142071
This article is part of the Research Topic Recent Research Advances on Heavy Metals, Microplastics, Persistent Organic Pollutants, and Solid Waste in Aquatic and Terrestrial Ecosystems View all 11 articles
Plastic pollution on land and in oceans is currently a pressing environmental issue. The accumulation of waste has caused severe, irreversible impacts and consequences on marine life, ecosystems, and the environment due to the lack of good waste collection, treatment, and management systems. Limited resources and infrastructure constantly challenge waste management in Southeast Asia. Therefore, we will examine the current plastic situation and issues in Southeast Asia and gain an understanding of the issues of the existing waste management systems in those countries. Then, we will examine the current practices applied in tackling plastic pollution and review the collective commitment and actions of governments, private sectors, social organizations, stakeholders, and consumers, as the key players in ending plastic pollution.
Plastic material is a convenient and versatile commodity used on a global scale with diverse applications such as in electronics, healthcare, agriculture, transportation, construction, and most significantly, packaging (Huo et al., 2020; Kunwar et al., 2016). With the massive growing population and rapid urbanization, global plastic production reached a cumulative total of 360 million tons in 2018, demonstrating substantial leaps of 1.2-fold from 299 million tons in the short time frame of 5 years (Anuar Sharuddin et al., 2016; Yao et al., 2021). While the use of plastic is swiftly expanding, the accumulation of municipal plastic waste entering the solid waste stream is a major cause of severe environmental issues. The issue is exacerbated as these plastic materials are highly durable due to their unique molecular structures composed of hydrogen, carbon, and other elements that take years to decompose fully. Thus, effective plastic waste management and treatment approaches are urgently needed to solve the environmental problem. Incineration and landfilling are the two most common ways of dealing with plastics disposal, and only 9% of plastic waste is being recycled globally, resulting in an estimated 4–12 million metric tons of plastic waste pilling up in the ocean annually (Geyer et al., 2017a; Jambeck et al., 2015; Wen et al., 2021). The mismanaged plastic waste that enters the ocean forms microplastics, which are tiny plastic particles that originate from primary and secondary sources with a size of <5 mm in an infinite shape (pellets, fibers, etc.) (Akdogan and Guven, 2019; Horton et al., 2017). When the plastic materials are exposed to ultraviolet radiation, the plastics become brittle and subsequently fragment into microplastics due to the photo-oxidation process (Figure 1). Under the influence of heat, sunlight, and well-aerated conditions, plastic waste undergoes iterative fragmentation processes, and the anoxic conditions of aquatic environments result in the slow degradation of plastics (Zhang, 2017). The primary microplastics originate from synthetic fibers and textiles derived from the abrasion of clothes, in which 1900 fibers per item are estimated to be leached during washing (Napper and Thompson, 2016). Another source of primary microplastics is sedimented microplastics in cosmetic and medical products. On the other hand, secondary microplastics are derived from the fragmentation/degradation of macroplastics into plastic debris due to physical, chemical, and biological processes (Akdogan and Guven, 2019). Light macro- and microplastics can be transported across the land by wind, and the dense ones will be buried deeper in soil layers. The piling up of microplastics poses an ecotoxicological risk, and this hydrophobic debris in water serves as a good absorb heavy metals that affect the water quality (Avio et al., 2017; Wang et al., 2017). Incineration is a common practice in developed countries to resolve domestic plastic accumulation by burning plastic waste at high temperatures (Gupta et al., 2022). However, incineration involves energy-intensive pre-treatment that engenders severe environmental impact, whereas the presence of additives and blends within the plastic lattice may complicate the recycling process (Ragaert et al., 2017; Cao et al., 2020).
FIGURE 1. Overview of factors influencing the weathering of plastics (Jahnke et al., 2017).
As an alternative, global plastic trade flow was triggered in the late 1990s, whereby plastic waste was transferred from developed to developing countries. China, as the primary importer of plastic waste, found that these materials are profitable for goods production; however, the low quality/grade of the plastic waste (contaminated) is the cause of environmental issues. In 2013, China introduced a temporary plastic waste import restriction, which is also known as the “Green Fence” campaign, to combat poor quality and contaminated plastic waste and to reduce illegal foreign smuggling and trading (Brooks et al., 2018). However, this temporary campaign did not entirely halt the illegal transfer of plastic waste, resulting in an annual plastic waste import of 8.88 million tons in China, which triggered a series of environmental problems (Chen et al., 2019). In 2017, China issued a new import policy banning the import of 24 types of solid waste, including plastic waste, which has globally challenged and disrupted the flow pattern of the global plastic waste trade. Following the China ban, the global plastic waste trade volume clearly decreased, as compared to the trade volume prior to the China ban. At the same time, a surge of plastic waste entered developing countries, especially Southeast Asia, making it a major contributor to plastic pollution. Perhaps, these actions could have helped the developed countries in partly managing their domestic waste (waste trading); nevertheless, it poses threats to developing countries. The Southeast Asian countries Indonesia, Thailand, Vietnam, the Philippines, and Malaysia are the top five countries for the production of large municipal solid waste, at 1.14 kg/capita/day worldwide (Arumdani et al., 2021). Mismanaged contaminated and unprocessable waste, poor domestic waste disposal management and facilities and the lack thereof, and insufficient land for proper waste disposal are the main causes of the threat to Southeast Asia’s environment (Jain, 2020). As plastic debris is blown by the wind or washed by rain into waterways, plastic materials pile up in the sea, leading to an estimated 14 million tons of waste entering the ocean every year (Bello, 2022). Figure 2 displays the annual flow of river plastic inputs into oceans in tons (Lebreton et al., 2017). This phenomenon is worsening with the illegal waste dumping by developed countries and the smuggling process, which has ensued from the Southeast Asian governments imposing restrictive measures on plastic waste imports. Southeast Asian nations have taken up the challenge in recent years and pledged to curtail the pollution issue. In 2018, Malaysia implemented a roadmap toward zero single-use plastics with the aim of addressing single-use plastic pollution for a cleaner and healthier environment in Malaysia by 2030. In addition, Malaysia has returned 4,120 tons of plastic waste to 13 countries and has officially shut down 200 illegal plastic recycling centers since 2019 (New Strait Times, 2019). Meanwhile, Thailand restricted electronic waste imports and pledged to end plastic waste imports by 2021 (Sasaki, 2021).
FIGURE 2. Mass of river plastic flowing into oceans in tons per year. River contributions are derived from individual watershed characteristics such as population density (in inhabkm−2), mismanaged plastic waste (MPW) production per country (in kginhab−1d−1), and monthly averaged runoff (in mmd−1). The model is calibrated against river plastic concentration measurements from Europe, Asia, and North and South America (Lebreton et al., 2017).
Here, we review the issues of plastic waste and microplastics in Southeast Asia by first understanding the current situation surrounding the plastic waste issue in Southeast Asia, followed by identifying the current waste management systems (landfilling, incineration, and recycling) in Southeast Asia. Then, we will identify the potential solutions for tackling the plastic waste crisis in Southeast Asia. In addition, we quantify the cascading impacts of China’s import ban and discuss how the ban affects the global trade flow of plastic waste and quantify the magnitude of the environmental impact of trade flow changes and eco-costs of five midpoint indications, namely global warming (GW), fine particulate matter formation (FPMF), freshwater ecotoxicity (FEW), human carcinogenic toxicity (HCT), and water consumption (WC) resulting from the China ban.
Plastic waste is a prevalent issue worldwide. In recent years, due to the COVID-19 pandemic, there has been an alarming increase in the use of single-use plastics throughout Southeast Asia. Due to the lockdown periods, Malaysia, Thailand, and Singapore recorded a spike in plastics such as single-use plastic packaging, bags, and containers (The Japan Times, 2020). Other impacts include the increased plastic medical equipment required due to the pandemic. In Malaysia, there were instances of waste spillage (Yuen et al., 2020) and an increase in household plastic waste (Teoh, 2020). Similar instances were recorded in Singapore, Thailand, Myanmar, the Philippines, and Vietnam (Praveena and Aris, 2021). The total waste generation and management in Southeast Asian regions are tabulated in Table 1.
TABLE 1. Demographic context, waste generation, and waste management in Southeast Asian countries. Data extracted are based on 2015, unless specified Tun et al. (2020).
Southeast Asia is a wealthy and biodiverse region, with almost 150,000 km of coastline and over 25,000 islands including approximately 34% of the world’s coral reefs and 25%–33% of the global mangrove forests, which are diverse with tropical marine species (Omeyer et al., 2022). Countries with higher populous density along the coastlines show a higher potential for polluting the ocean with plastics (Ritchie and Roser, 2018). With multiple countries, including Indonesia, the Philippines, and Vietnam, having large coastal populations, it is highly likely that this would lead to a more significant possibility of polluting the ocean with plastics. These Southeast Asian countries produce over 1.5 million metric tons of mismanaged plastics annually. Malaysia, Thailand, Vietnam, the Philippines, and Indonesia all ranked in the top 10 countries with the highest generation of mismanaged plastics, whereas Indonesia and the Philippines ranked second and third, respectively (Jambeck et al., 2015). These extensive populous coastlines may contribute mainly to the large amount of plastic waste entering marine areas in Southeast Asia. Despite this, many other countries within Asia have a high number of populous coastal regions but do not have a similar issue to the aforementioned countries. These countries have well-established, robust plastic waste management systems despite their proximity to the ocean (Loh, 2020).
It was estimated that 99.5 million metric tons of plastic waste were generated in coastal regions in 2010, and of this amount, around 4.8 to 12.7 million metric tons of plastics ended up in the ocean, which accounts for between 1.7% and 4.6% of the total plastic waste generated by the countries involved (Jambeck et al., 2015). These numbers are already alarming; detrimental effects have already been seen in marine life, in which microplastics have been detected within their bloodstream. Such microplastics have also been detected in humans (Leslie et al., 2022).
Another perspective showed that these large waste-generating countries within Southeast Asia, including Indonesia, the Philippines, and Vietnam, have undergone rapid economic growth over the last 3 decades, which also explains why food waste makes up a significant proportion of waste in Southeast Asia (UN environment, 2017). Considering that ASEAN’s urbanization rate is expected to surpass 70% by 2050, waste management issues are likely to worsen in the coming years (Loh, 2020).
The waste management system preferred in Southeast Asia is open landfill due to the ease of construction and low processing cost. Open landfill, as the name states, is a large land mass area sacrificed to accommodate the large amount of waste produced daily by citizens. Bantar Gebang, Jakarta’s largest landfill at around 120 ha, receives nearly 7,000 tons of waste daily. It is estimated to already hold 39 million tons of waste and should reach its capacity of 49 million tons (Raslan, 2019). It has been shown that Southeast Asian countries have produced between 0.21–640 million tons of municipal solid waste, the largest being Indonesia and the smallest being Brunei Darussalam (UN environment, 2017). Reliance on plastics, especially during the pandemic, has increased plastic usage and single-use plastics (Chen et al., 2021).
The prospect of landfills is not sustainable as land mass use would increase daily and would eventually lead to the depletion of usable land mass. Aside from the apparent leachate issues that would be detrimental to the land mass and water sources surrounding the landfill, plastics are a challenging issue since they have a long lifespan, and the issue of microplastics has become more prevalent in recent years. Plastic pollution’s impact is visible on land and in the ocean. Landfills are favored, but due to their detrimental effect on the environment, which includes air, water, and land pollution, as well as the change in climate caused by greenhouse gases, they are not sustainable (Arumdani et al., 2021). In Malaysia, approximately 85% of municipal solid waste material goes to landfill sites, and because plastic is not biodegradable, with the current rate at which landfills are being filled, they will soon reach capacity (Chen et al., 2021).
Currently, there is a multitude of ways to manage plastic waste where the Southeast Asian countries use landfills, sanitary landfills, incineration, and composting. Southeast Asian countries use landfills, sanitary landfills, incineration, and composting. Landfills, as mentioned previously are not sustainable in the long run. Only a small percentage of landfills in Southeast Asian countries are sanitary landfills (Arumdani et al., 2021), which provide low-cost waste management compared to other alternatives. Incineration involves higher capital and a management cost of around 80–102 USD per ton, while for sanitary landfills, it is around 10–45 USD per ton (Tun et al., 2020). Despite that, due to the limitations of capacity, this option is not considered sustainable given the amount of waste coming into the site, which is leading to sanitary landfills becoming unsanitary landfills (Loh, 2020).
The current recycling rate for most Southeast Asian countries is below 50% due to the limitations of infrastructure and logistics to provide the necessary operations for it to be profitable (UN environment, 2017). Private companies run most recycling facilities in Southeast Asian countries, and profitability is the main driving factor. In most cases, the waste produced is too dirty to qualify for mechanical recycling (Chen et al., 2021). The current infrastructure is not viable because most recycling practices require sorting and separation processes beforehand. It would be difficult to develop the necessary infrastructure to keep up with the increase in plastic usage, making it a race against time. Most of the recycling infrastructure is located in urban areas; hence, people from outside cities do not have alternatives for recycling their plastics. Regarding plastics, there are limitations to what types of plastics can be recycled. Post-industrial plastics are the easiest to recycle due to their purity. Contrary to that, post-consumer plastics pose many challenges not only due to a lack of waste separation during the initial stages of the waste generation process but also due to the mixture of plastics when producing consumer plastics (Antelava et al., 2019). Due to the ever-increasing load of the recycling process, most plastics that go to recycling plants would be deemed unrecyclable and be directed to landfills (Geyer et al., 2017b).
In addition, the backbone of the recycling process in Southeast Asian countries is often underprivileged citizens. There is no specific unified system to homogenize the retrieval process, which subsequently affects the entire supply chain process of the recycling route. This unreliable route further reduces the profitability of the recycling process, which further decreases the chances of plastics being recycled. In some instances, companies would instead import plastic waste from overseas for recycling purposes (Chen et al., 2021).
Another waste management process in Southeast Asian countries is the waste-to-energy process, or incineration, which focuses on burning waste to create energy. Plastic waste is considered a good source of fuel. A similar problem can be seen in the implementation of incineration plants in Southeast Asian countries, which is due to the lack of infrastructure and cooperation between governments, municipalities, and private companies regarding the supply of waste. Consistency and quality of waste are crucial for the incineration process to create good quality and reliable energy. Since the majority of waste in Southeast Asian countries is primarily organic waste, this leads to the creation of wet waste, reducing the overall efficiency in producing energy (Tun et al., 2020). The mixture of wastes can produce toxic by-products such as noxious gas emissions and ash by-products that require more advanced after-treatment processes, pushing the cost of incineration plants (Energy, 2020). Hence, similar to the recycling process, sorting is important in order to produce good-quality energy, and hydrocarbon sources such as plastics are more favorable than wet organic waste, which produces lower calorific value energy ranging from 5–11 MJ/kg (Tun et al., 2020).
The problem of plastic waste closely relates to the problem of waste management, as plastic causes further damage due to its long lifespan. Hence, waste management systems are vital to improving the situation around plastic waste destroying land and ocean ecosystems. Major stakeholders, including government bodies, private companies, and international bodies, need to work together internally and externally to create an integrated system to help better manage plastic waste.
Southeast Asia accounts for a significant proportion of global microplastic pollution, ascribed to the abundance of mangrove, seagrass, and coral habitats in coastal and shallow waters that lead to plastic accumulation by snagging. Microplastics are found in beach sediments, water columns, benthic sediments, and marine biota (Figure 3A) and are accumulated along the high-strand vegetation lines and trapped between plants, according to investigations conducted in Thailand and Singapore (Curren and Leong, 2019).
FIGURE 3. (A) Schematic diagram representing the presence of microplastics in the marine environment: in beach sediments, water column, benthic sediments, and marine biota. (B) Overall composition of microplastic types found across beach sediments, seawater, benthic sediments, and marine organisms. A total of six main types of microplastic were identified (Curren et al., 2021).
Microplastics are classified into primary and secondary forms. Primary microplastics are derived from sources such as resin beads, microbeads for facewash or toothpaste, and other products. Secondary microplastics are fragmented macroplastics that originate from coastal and domestic sources and international ocean flows. The fragmentation of macroplastics occurs through environmental weathering, which alters polymer properties due to abiotic factors (light, temperature, air, water, and mechanical forces). Light microplastic debris floats on the water’s surface. Over a certain period, the microplastic surfaces can be colonized by microorganisms, which results in denser microplastic particles that eventually sink to form benthic sediment (Riani and Cordova, 2022).
Domestic sources of plastics, such as due to marine litter and fishing activities have caused a substantial environmental impact in the coral reef localities of Darvel Bay, East Sabah Malaysia, where plastic bags (10%), plastic bottles (13%), and fishing nets/lines (21%) have been found in the reef (Figure 4) (Santodomingo et al., 2021). As well as in Malaysia, the presence of microplastics in freshwater ecosystems has also been detected in the river streams of Indonesia, Thailand, and Vietnam. The concentration of microplastics in the seawater in Southeast Asian regions ranges from 0.13–11,100 pieces/L, which is comparable to the figures recorded in the Arctic Ocean and Santa Monica Bay (Curren et al., 2021). Reviewing the case in Thailand, Thailand receives several hundreds of thousands of tons of plastic waste from developed countries every year. At the same time, they have a poor management system, and plastic waste leaks into canals and beaches during heavy flooding. Johansson and Ericsson reported that hard/soft microplastics in the form of foams and beads were found on the water surface of the Chao Phraya River (Ericsson and Johansson, 2018). In contrast, 0.04–0.30 particles/L microplastics were discovered on the water surface of the Dungun River in Malaysia (Tee et al., 2020). Microplastics from 50–5000 μm were observed on the water surface and in the sediment of the Citarum River, Ciwalengke River, and Surabaya River in Indonesia. Microplastics can exist in various forms, such as filament, fiber, granule, fragment, film, and foam. Among these forms, Curren and co-workers discovered fragment-type microplastics were dominantly found across beach sediments, seawater, and benthic sediments, whereas the fiber type was discovered in marine organisms (Curren et al., 2021). The overall compositions of microplastic types found across the beach sediments, seawater, benthic sediments, and marine organisms are depicted in Figure 3B.
FIGURE 4. Examples of marine litter found in the Darvel Bay reefs: (A) Abandoned fishing net in the Triangle Reef at 10 m depth, (B) plastic bag (BAG), plastic bottles (BOT), and aluminum can (CAN) in Baik at 5 m depth, (C) other food wrap (OFW) and textiles (TEX) in Sakar at 5 m depth, and (D) plastic bag (BAG) in Sakar at 10 m depth (Santodomingo et al., 2021).
These microplastics have a negative impact on oceanic carbon cycles, altering the composition of microbial and planktonic communities. In addition, tiny pieces of microplastics can escape from wastewater treatment plants and enter the water stream as domestic effluents (Carr et al., 2016). From there, they can be mistakenly ingested by marine organisms such as sea turtles, whales, and sharks, causing digestive tract injury (Abreo et al., 2019; Coram et al., 2021). The development of coastal and marine pollution, overfishing, aquaculture, etc., endangers 80% of the region’s reef species. Hence, it is crucial to identify microplastic pollution hotspots and standardize protocols to better quantify, assess, and monitor microplastic contamination levels.
An integrated waste management system to combat plastic pollution includes efficient collection, processing, and treatment processes. However, these processes still need improvement in most of Southeast Asia. Despite the deployment of ‘Interceptors’ and ‘River Trash Booms’ in Indonesia (Jakarta and Bali) and Malaysia (Klang River) to prevent the flow of marine debris into the waterways, they are not a comprehensive solution to marine pollution. In Southeast Asia, the use of microbeads in cosmetic production has been officially banned in Thailand since 2020, as a supportive, collective effort to reduce primary microplastics. We should bear in mind that the fragmentation of macroplastics causes the formation of secondary microplastics; thus, a call to reduce single-use plastics is necessitated in Southeast Asia. Cambodia has banned the import and consumption of single-use plastics. Likewise, Malaysia adopted “The Malaysian Roadmap to Zero Single-Use Plastics” in 2018 and follows the 3R initiative (reduction, reuse, and recycle) (Fauziah et al., 2021) in daily life. Nevertheless, achieving zero single-use plastics is demanding and challenging at this stage because a total ban on plastic bag usage has yet to be implemented throughout Southeast Asia; for example, in Singapore and Malaysia, some supermarkets still provide plastic bags but with certain charges. Hence, educating and changing the public’s mindset on plastic use and waste is crucial to realizing a zero single-use plastic nation.
Following the current trends, the plastics within our oceans are projected to double by 2030 and triple by 2040. Southeast Asian countries are considered significant contributors to the leakage of land-based plastic waste into the seas, with a generation of 31 million tons of plastic waste annually (Julius and Trajano, 2022). It was stipulated that 80% of marine plastic debris originated from the land, which is why it is essential to create or enhance the current waste management system, especially in coastal areas.
The approach used by Southeast Asian countries to tackle waste is regionally blocked and only focuses on specific areas, resulting in significant oversights of an issue affecting the region on a large scale. For example, managing waste through incineration is only available and accessible in some regions, such as Myanmar, Singapore, Thailand, and Vietnam (Table 1). Collaboration between major stakeholders, including government, non-government, and international bodies, is needed in order to tackle this issue. China’s ban on plastic imports has resulted in more than double the amount of plastic waste entering Southeast Asia in countries such as the Philippines, Malaysia, and Indonesia (Yoshida, 2022). Countries including Malaysia and the Philippines are returning the plastics to Western countries, while Thailand and Vietnam have restricted the further import of plastic waste. Despite that, Southeast Asian countries are still struggling with the influx of plastic waste generation within their regions. Out of 27.8 million tons of plastic waste generated in Thailand, 27% is improperly disposed of, and similar situations have been seen in bordering countries, including Malaysia (Chen et al., 2021). More than half of Indonesia’s landfill is made up of open dumpsites without proper safety measures; these places increase the risk of floods, fires, and refuse avalanches, which have already claimed many lives in places including the Philippines and India (Marks, 2019).
The current plastic waste problem is not just a plastic issue, it is a climate problem. The 2021 UNEP report showed that in 2015, the greenhouse emissions from the production, usage, and disposal of fossil fuel-derived plastic emitted approximately 1.7 gigatons of CO2 equivalent, which will only rise to 6.5 gigatons by 2050, which is approximately 15% of the whole carbon budget (Julius and Trajano, 2022). Some argue that making a large systemic change may not be fast enough in order to deal with this issue; hence, in conjunction with large infrastructure changes, a holistic and community-based approach can be implemented in conjunction with the improvement of waste management infrastructure, especially for places outside of the city center.
In Indonesia, a waste-bank program was introduced in 2012 that encourages households to sort their waste into specific categories, which is then deposited in a central waste bank that provides them with monetary returns (Loh, 2020). There is also the ocean cleanup project under a non-profit organization, which aims to get rid of the plastics in the ocean using innovative solutions such as the interceptor unit utilized along rivers and oceans surrounding Southeast Asian countries (Omeyer et al., 2022). The prospect of utilizing plastic waste in developing communities and converting it into liquid fuel has been explored by Joshi and Seay (2016) and Owusu et al. (2018) in India and Uganda, respectively. Similar environments exist in Southeast Asian regions; therefore, this approach can be helpful in reducing plastic waste, especially in rural areas where waste infrastructure is severely lacking.
Other approaches to reducing plastic waste include banning specific items, deposit return schemes, and biodegradable packaging replacing plastic, which work well in the short term, but long-term systemic changes should be the focus of solutions to plastic waste and waste management in general (Omeyer et al., 2022). Integrated collaboration between the countries and help from international bodies would help implement an integrated waste management system in Southeast Asian countries.
Multiple collaborations and policies have been enacted among Southeast Asian members to solve the issue of plastic waste. This includes the ASEAN Regional Action Plan for Combatting Marine Debris in The ASEAN Member States (2021–2025), which directly addresses the issues of marine plastic waste. The members recognized that there is a lack of capacity with regard to plastic waste management both in the public and private sectors; hence, one of the goals of this process is to help bridge that gap and help improve the overall waste management system (ASEAN Secretariat, 2021).
Additionally, there is the ASEAN-Norway Cooperation project on Local Capacity Building for Reducing Plastic Pollution, which commenced in 2019. This initiative focuses on local municipality-/city-level sustainability and sets of science-based and feasible measures to reduce plastic pollution in crucial sectors. This would help improve the capacity of local actors, including regional governments, non-governmental organizations, and academic institutions. Other initiatives include the ASEAN+ 3 Marine Plastic Debris Cooperative Active Initiative and the Japan Funded Promotion of action against marine plastic litter in Asia and the Pacific (CounterMEASURE Project), both of which focus on the reduction of marine plastic waste. In addition, there are National recycling associations set up by the companies in Singapore, Malaysia, Vietnam, and the Philippines; although these are exclusively voluntary and don’t involve government enactment, so there is a bias (UN environment, 2017).
These initiatives are a step in the right direction, but further development of plastic waste management needs to follow the cradle-to-cradle approach and not just focus on the end-life stage of plastic waste. As opposed to climate change, no global plastic agreement has the power to help push for regional action development to keep up with the increase in waste, and most agreements currently focus on the ocean rather than land-based sources of marine litter (Omeyer et al., 2022). This is an approach that treats the symptoms rather than the source. Moving forward, as mentioned previously, crucial stakeholders, including governmental and non-governmental organizations, need to combine forces and tackle the source of the problem, not just mitigate the after-effects of a larger plastic issue.
Plastic waste is a “dirty” material that thrives in the trading of plastic waste worldwide and in the recycling industry. This material is also a significant source of severe environmental issues when it is not adequately treated (Lau et al., 2020). Europe (the EU) is at a crossroads in plastic and plastic waste management and trading plastic waste owing to insufficient recycling capacity. The exportation of plastic waste to Asia has led to substantial growth in international trade from 0.29 million tons (Mt hereafter) in 1988 to 15.99 Mt in 2014. Since 1993, Hong Kong, the USA, Japan, Germany, and the UK have been the largest plastic exporters, and this waste is transported to developing countries for recycling (Brooks et al., 2018; Wen et al., 2021; Tan et al., 2022). China was the world’s foremost player in plastic importing, where the Chinese contributed to an annual plastic waste import of up to 8.88 million tons; due to the price of the imported plastics are cheaper for production, rather than using domestic plastic waste (Velis, 2014; Brooks et al., 2018). Among the plastics exporters, Hong Kong exported approximately 3,184,176 tons of plastic waste per annum (approximately 22% of the global trade) to China. Among the types of plastic waste, polyethylene (PE) is top of the list in plastic waste trade flow, which recorded approximately 37% or 11,404,697 tons in total, followed by polypropylene (PP), polyethylene terephthalate (PET), polystyrene (PS), and polyvinyl chloride (PVC) at a lower rate of 23%, 12%, 14%, and <8%, respectively (Figure 5A). The flow of PE from Hong Kong to China is particularly prominent, with a record of 46.2%, while the USA, Japan, and European countries exported 77.9%, 87.6%, and 57.5% of plastic waste to China (Wen et al., 2021). Unexpectedly, China imposed a ban named Prohibition of Foreign Garbage Imports: The Reform Plan on Solid Waste Import Management on 27 July 2017 to ban the import of particular wastes due to the low quality and contamination level of the importer plastics, which triggered severe environmental problems (Qu et al., 2019). China’s imports have substantially plummeted by 95.4% (relative to baseline levels) and the world’s total plastic waste trade flow declined by 45.5% in 2018 after the ban was imposed, as opposed to the scenario prior to the ban (Baseline Scenario). The China ban has greatly affected all major exporters with total export rates having reduced in Japan, the USA, and Europe by 39.2%, 54.1%, and 29.9%, respectively. This phenomenon has consequently resulted in a surging import of 362% to Southeast Asia, as illustrated in Figure 5B (China Dialogue, 2021).
FIGURE 5. Trade flows of six types of plastic waste under two scenarios. (A) Global trade flows of six types of plastic waste prior to the ban (Baseline Scenario); (B) flows subsequent to the ban (2018 Scenario). The unit of the flows is tons (Wen et al., 2021).
The proportion of exports from developed countries to Southeast Asia, for instance, Japan, has substantially skyrocketed by approximately 50%, from 4.34% to 55.9%. In contrast, the United States saw an increment of 41.26% (5.24% to 46.5%) in export rates, and Europe saw an increment of 6.1% to 33.0%. At the same time, the import rates of Southeast Asia were increased by 3-fold, approximately 3.62 times higher than the Baseline Scenario, attributed to the contributions from Japan (25.8%), the United States (19.4%), Germany (11.7%), Hong Kong (10.3%), and the UK (9.8%), respectively. The calculated import lg (TF2018/TFbaseline) of Southeast Asian countries, especially Thailand, the Philippines, and Malaysia, is relatively higher, as illustrated in Figure 6. Upon the Chinese ban, Malaysia grew as the largest plastic waste importer after China by importing a high volume (105 thousand tons) of plastic waste in 2017, achieving an increasing rate of 68% as of 2016. However, the imported plastic wastes, including illegal imports, are of lower grades (contaminated), resulting in severe environmental issues. As a solution, the Malaysian Government has introduced policies such as issuing plastic waste import permits and close monitoring of permit holders to address the issue mentioned above. Evidently, 62 current permit holders in Malaysia have been monitored closely as of June 2019, and 148 illegal plastic recycling plants were shut down in the same year (Hassan et al., 2000; New Strait Times, 2019; Chen et al., 2021). According to the export data available in Comtrade June 2020, the total exports of plastic waste trade from all countries to Southeast Asia dropped by 32% in 2019, with a total plastic waste trade of 1,331,851 tons, as compared to 1,948,554 tons in 2018. The ban’s impact has further intensified the plunging export volumes of the United States, the United Kingdom, and the Republic of Korea to 60%, 37%, and 46% of 2018’s volumes, respectively.
FIGURE 6. Changes in import and export flows after the ban (Wen et al., 2021).
In brief, the circumstances of the China ban are expected to result in waste accumulation, or these wastes will be transferred to other low-income countries such as Southeast Asia, consequently leading to undesirable environmental impacts. Figure 7 portrays the environmental impact of trade flow changes (EIT) by considering the environmental indicators, including GW, FPMF, FEW, HCT, and WC, upon the China ban (2018 Scenario). Thanks to the initiative of the China ban, the changes in trade flow have contributed to an improved indicator of FPMF, FEW, HCT, and WC after promoting global environmental sustainability. At the same time, the plummeting export rates resulted in temporal environmental impacts on GW owing to the higher incineration rates of developed countries compared to developing countries because landfilling is the primary waste treatment. In summary, strengthening local management and waste treatment in all countries is essential and is expected to mitigate the environmental issues of the plastic waste trade (Wen et al., 2021).
FIGURE 7. EIT and eco-cost of the China ban for the 2018 Scenario. Note that an item has a beneficial environmental impact when its value is negative. To enhance the visibility of midpoint indicator values on the ordinate axis, the unit of each indicator was adjusted as shown in the brackets at the bottom of the figure (Wen et al., 2021).
Conventional plastics derived from crude oil are the major contributor to environmental pollution and global warming, attributed to their non-biodegradable properties where these materials require decades for degradation. The non-biodegradability of plastic refers to the plastic’s chemical structure that could not be degraded or broken down by naturally occurring microorganisms, water, carbon dioxide, etc. (Babu et al., 2013). In contrast, biodegradable plastics are compostable to form biomass, water, and carbon dioxide or methane via microorganisms under specific conditions (Atiwesh et al., 2021).
As per the European Bioplastics Association, bioplastics are composed of materials with partially bio-based renewable raw materials such as biomass and are biodegradable depending on the monomer’s characteristics and polymerization processes. Biodegradability implies the conversion of material into natural substances by microorganisms such as bacteria, fungi, and algae. Bioplastics can be bio-based or biodegradable or feature both properties. Bioplastics can be produced using three types of generation feedstock. The first-generation feedstock includes carbohydrate resources based on edible food crops such as sugarcane, potato, and corn, raising concerns over sustainability. In comparison, the second-generation raw materials are derived from lignocellulose-rich feedstock such as wood and non-edible by-products of food crops. Although second-generation raw materials are more eco-friendly than first-generation raw materials, lignocellulose conversion is energy intensive (Singhvi and Gokhale, 2019). Meanwhile, algae and municipal waste are third-generation feedstock for bioplastic production (Singh et al., 2022). Bioplastic is an alternative plastic material derived from all kinds of whole or partial renewable biomass, thus giving rise to bioplastics with different properties (Nandakumar et al., 2021). For instance, PLA, bio-PET, etc., are suitable for packaging, while bio-based succinic acid is used in the automotive and textile industries. There are three ways to prepare bioplastics: (a) thermochemical and catalytic processes, converting biomass feedstock into monomers and then polymerizing them; (b) fermentation processes, fermenting biomass to produce monomers followed by conversion into polymers; and (c) modifying naturally occurring polymers (Singh et al., 2022). In 2019, among the 2.05 million tons of bioplastics produced, merely 54% of them were biodegradable, while 46% were non-biodegradable (IFBB, 2022). The degradability of bioplastics relies on their composition, degree of crystallinity, and environmental factors that result in a degradation time frame that varies from days to years. For example, PLA is the most commercially developed biodegradable plastic, and the biodegradation of PLA bioplastics contributed to a zero net increase in CO2 and 70% fewer greenhouse gases during the biodegradation in landfills, implying bioplastics are more environmentally friendly than conventional plastics (Elsawy et al., 2017).
Although biodegradable plastics offer significant momentum to end plastic pollution, there are still great uncertainties waiting to be explored, including the complexity of waste management and the presence of contaminants that may trade off the compost quality and the emancipation of toxic chemicals to the environment. Although biodegradable plastics can be degraded under the action of bacteria, fungi, or algae, in some circumstances, degradation can be initiated under the influence of temperature. However, biodegradable bioplastic waste is still processable through mechanical and chemical recycling, thus offering viable waste recovery options that reduce reliance on primary resources, leading to a definite shift of the plastic chain towards sustainability (Fredi and Dorigato, 2021).
Waste-to-wealth refers to upcycling and valorizing waste by turning it into valuable/useful products, including refinery feedstock, fuel, and monomers (Jiang et al., 2022). Upcycling plastic waste to make fuel is promising because plastic-derived fuel has a high calorific value comparable to gasoline and diesel in the market. While most countries are still practicing incineration in dealing with plastic wastes to save landfill space, this method merely offers low energy recovery efficiency with the emission of hazardous and greenhouse gases. Another versatile approach worth mentioning is chemical recycling, where plastic waste can undergo gasification and pyrolysis to convert it into valuable products.
Pyrolysis refers to the thermal degrading of complex molecules into smaller molecules at a high temperature (300°C–800°C) in an inert condition, producing liquid oil, char, and gases as value-added products (Fivga and Dimitriou, 2018). It can be performed either with (catalytic) or without the assistance of the catalyst named thermal pyrolysis. The catalysts, such as zeolite and silica-alumina, are often used during the pyrolysis process as the catalyst reduces pyrolysis temperatures, narrows product distribution, and increases product selectivity (Singh et al., 2018; Chen et al., 2021). The actual application of the pyrolysis process has been conducted by Muang Sa Ad Co., Ltd., a company that collects, cleans, and converts plastic waste into oil through the pyrolysis process. The pyrolysis oil is refined and used as fuel in refuse trucks in Thailand (MSA, 2018).
Unlike the pyrolysis process, gasification converts the solid fuel to gaseous fuel such as syngas (hydrogen and carbon monoxide) production at high temperatures (usually higher than 800°C) in an oxygen-limited condition. The gasification of plastic waste has caught considerable attention because the produced syngas is an excellent raw feedstock in a fuel cell to generate electricity (Salaudeen et al., 2019; Saebea et al., 2020). On another occasion, researchers from Nanyang Technological University (NTU Singapore) found a way to convert plastic waste to hydrogen based on high-temperature chemical processes. The produced hydrogen, as an alternative clean energy source, helps generate electricity and power fuel cells in electric vehicles (NTU Singapore, 2022).
The establishment of plastic waste upcycling technologies is still in its embryonic stage, but it is an attractive approach to converting municipal plastic into its original monomers, chemicals, and fuel products. In addition, these technologies are promising as a replacement for high-cost plastic waste incineration.
In establishing a circular economy for plastics, it is first essential for society to reconsider plastic as a renewable resource instead of as a waste. Nevertheless, according to the Ellen MacArthur Foundation, merely 14% of plastic packaging is recycled, 40% is left in landfills, 32% is left in ecosystems, and 14% is incinerated for energy recovery. A thriving circular economy would mean the constant flow of plastic around a closed-loop system; looping the used plastic back into the value chain rather than being used once and discarded. This will involve (i) redesigning products for recyclability using new or renewable materials and (ii) closing the loop with chemical recycling. The first case is achievable by substituting fossil-based feedstocks with renewable feedstocks, such as the development of biodegradable plastics (discussed in Section 5.1) that can be degraded in a shorter time frame without contaminating the environment. In addition, the plastic industries should prohibit single-use materials during plastic production and reduce the use of colorants and additives to simplify the recycling process. For instance, Unilever unveiled their new recycling technology, called CreaSolv Process, to recover the plastic from sachets and use it to create new sachets for Unilever products (Unilever, 2017).
Second, chemical recycling (Section 5.2) should be promoted as this process transforms plastic material and additives into their original monomer, which can be the feedstock for a new product. Recently, new and modified pyrolysis pilots have been emerging. For instance, the United Kingdom start-up Recycling Technologies uses a fluidized bed reactor for pyrolysis and found that this reactor could evenly distribute the temperature and modularize, which is more adaptable to a dispersed collection and plastic recycling system (Recycling Technologies, 2018). Moreover, pyrolysis provides high flexibility in terms of feedstock. This thermal process disintegrates polymers, other organic materials, and vulcanized polymers, including automotive rubber tires, which could not be recycled using other methods. In brief, chemical recycling technologies are the alternative methods for processing materials that are hard to be treated with mechanical recycling while producing higher-quality recycled materials (Jahnke et al., 2017). For example, PETRONAS Chemical Group and Plastic Energy Ltd. have successfully developed a new technology to convert non-recyclable and low-quality plastic waste into naphthalene in Malaysia, which can produce virgin-quality polymers (KASA, 2021).
Since 2021, Malaysia adopted the Malaysia Plastics Sustainability Roadmap to set Malaysia on a pathway to plastics sustainability for 2030 and beyond. The first approach to achieving plastic sustainability is improving product design using recycled resin as the raw material to assure environmental friendliness and to be kept in the loop for long without compromising the product’s quality and performance. In Malaysia, PP, PET, HDPE, and LDPE are the most common resins used for single-use packaging, which should be phased out and replaced with a new recyclable product with a longer shelf-life and value in the chain. For instance, Thong Guan Industries Berhad, Malaysia’s most extensive stretch film manufacturer, has produced nano stretch film to wrap pallets and goods. This new type of film has excellent grip, load stability, and durability that resist wear and puncture compared to the single-use conventional multi-layered stretch film (KASA, 2021). On another occasion, to ensure material circulation, KLEAN Malaysia worked with Shell Malaysia by placing Reverse Vending Machines at certain Shell stations in Kuala Lumpur for the public to deposit/recycle used plastic containers in exchange for a reward (KLEAN, 2021). In Thailand, the Dow Thailand Group and Siam Cement Group collaborated in surfacing roads using recycled plastic waste. They found that asphalt derived from plastic wastes gave rise to enhanced strength and superior erosion resistance of roads (SCG, 2019).
A circular economy is an effective approach to addressing environmental issues such as global greenhouse gas (GHG) emissions and post-consumer waste pollution. Due to the fact that most plastic products (>90%) are produced from virgin petroleum-based feedstock, it is expected that this phenomenon will contribute to 15% GHG emissions by 2050. The Swedish Environmental Protection Agency revealed that recycled plastic saves approximately 1–1.5 kg CO2/kg resin, and each kg of recycled plastic saves approximately 130,000 kJ of energy (Rahimi and García, 2017). Figure 8A depicts that the total reduction of GHG emissions was achieved by the reuse and recycling of plastic waste between China and trading countries, with a subtle 76-fold increment from 1992 (0.11 million metric tons CO2e) to 2012 (8.71 million metric tons CO2e). This phenomenon is credited to the Chinese stakeholders who have imported massive plastic waste from developed countries (EU, US, and Japan) to fulfill the domestic market’s needs. The skyrocketing GPWT between China and trading countries through ship transportation from 1992 to 2017 (Figure 8B) led to severe GHG emissions from 0.015 MMT CO2e to 0.229 MMT CO2e in 2017, especially during the peak periods between 2009 and 2012. The GHG emissions generated by shipping plunged due to the launch of the Chinese Green Fence campaign by the Chinese government to combat waste smuggling activities in China, in addition to the restrictions on allowing low-quality plastic waste to enter China. In 2017, a substantial reduction in GHG emissions was observed due to the ban on 24 categories of recyclables and solid waste by the Chinese government. Multiple efforts to address the issues of GHG emissions and plastic pollution include the execution of Horizon 2020 by the EU, where Canada banned harmful single-use plastic in a bid to reduce ocean waste, and China’s ban on non-biodegradable plastic bags that are <25 μm (Walker and Xanthos, 2018; Fraccascia et al., 2019; Liu et al., 2021). Despite CE being proven to be effective in alleviating environmental issues, there are still many obstacles in countries with different standards and operational systems. Thus, the implementation of a robust after-use system for post-consumer plastic materials is vital.
FIGURE 8. (A) GHG reductions achieved by Chinese plastic waste recycling industries (CPWRI) associated with global plastic waste trade (GPWT) between China and trading countries from 1992 to 2017. The import and export of plastic waste are indicated by orange and green bars, respectively. (B) GHG emissions generated by shipment transportation associated with GPWT between China and trading countries from 1992 to 2017 (Liu et al., 2021).
The lack of sophisticated plastic waste management systems in Southeast Asia is the prime cause of severe environmental impacts. Southeast Asia is a hotspot for receiving plastic waste from developed countries, yet most of the countries in Southeast Asia lack the infrastructure for sound waste management. Since 2017, Southeast Asian countries such as Thailand, Malaysia, and Vietnam have restricted plastic waste imported from Western countries and imposed various bans to curb the over-usage of single-use plastics and non-biodegradable plastic bags. Moreover, the turning point in winning this battle (ending plastic pollution) is dependent on the individual and collective choices of the people per se, as well as the collective efforts and commitments of all interested parties, including the government and NGOs. The Southeast Asian region should raise awareness of the potential environmental risks of waste disposal and, at the same time, formulate related policies to hamper undesirable consequences, which can be done by restricting the production and use of particular plastic products via regulations and raising the plastic recycling rate through the construction and improvement of recycling facilities.
In an effort to secure global waste trade, establishing a global extended producer responsibility system is essential to ensure fair and responsible waste trade. This system is aimed toward not only developing nations but also developed countries, who should work hand-in-hand to reshape and rebalance the global CE for plastics to reduce environmental pollution and GHG emissions globally. In addition, it is vital to establish a global standard for the reuse and recycling of plastic waste, such as standardizing the treatment methods and operational systems (mechanical, chemical, and organic recycling) for plastic waste of different kinds to ensure these wastes are properly recycled in other countries. In addition, the transfer of knowledge and technology from developed countries to developing countries helps mitigate potential environmental issues. For example, developed countries could invest in research and development (R&D) and train local employees (employees in the developing countries) in dealing with waste management and recycling technologies.
CN and MM wrote the first draft of the manuscript. CN, AG, AI, and JF contributed to the conceptualization and finding resources for the review paper. CS and JS contributed to visualization. ST and JS contributed to the review and editing of the paper. YT-Y and JJ supervised the progression of the writing and preparation of the manuscript. All authors contributed to the manuscript revision and read and approved the submitted version.
This work was funded by the Ministry of Higher Education Malaysia Translational Research (TR@M) and Universiti Malaysia Sabah Special Grant Scheme (SDK0321-2021).
The authors declare that the research was conducted in the absence of any commercial or financial relationships that could be construed as a potential conflict of interest.
All claims expressed in this article are solely those of the authors and do not necessarily represent those of their affiliated organizations, or those of the publisher, the editors, and the reviewers. Any product that may be evaluated in this article, or claim that may be made by its manufacturer, is not guaranteed or endorsed by the publisher.
Abreo, N. A. S., Blatchley, D., and Superio, M. D. (2019). Stranded whale shark (Rhincodon typus) reveals vulnerability of filter-feeding elasmobranchs to marine litter in the Philippines. Mar. Pollut. Bull. 141, 79–83. doi:10.1016/j.marpolbul.2019.02.030
Akdogan, Z., and Guven, B. (2019). Microplastics in the environment: A critical review of current understanding and identifi cation of future research needs. Environ. Pollut. 254, 113011. doi:10.1016/j.envpol.2019.113011
Antelava, A., Damilos, S., Hafeez, S., Manos, G., Al-Salem, S. M., Sharma, B. K., et al. (2019). Plastic solid waste (PSW) in the context of life cycle assessment (LCA) and sustainable management. Environ. Manag. 64, 230–244. doi:10.1007/s00267-019-01178-3
Anuar Sharuddin, S. D., Abnisa, F., Wan Daud, W. M. A., and Aroua, M. K. (2016). A review on pyrolysis of plastic wastes. Energy Convers. Manag. 115, 308–326. doi:10.1016/j.enconman.2016.02.037
Arumdani, I. S., Puspita, A. S., and Budihardjo, M. A. (2021). MSW handling of top 5 leading waste-producing countries in Southeast Asia. IOP Conf. Ser. Earth Environ. Sci. 896, 012003. doi:10.1088/1755-1315/896/1/012003
ASEAN Secretariat (2021). ASEAN regional action plan for combatting marine debris in the ASEAN member states (2021–2015). The ASEAN Secretariat Jakarta.
Atiwesh, G., Mikhael, A., Parrish, C. C., Banoub, J., and Le, T. A. T. (2021). Environmental impact of bioplastic use: A review. Heliyon 7, e07918. doi:10.1016/j.heliyon.2021.e07918
Avio, C. G., Gorbi, S., and Regoli, F. (2017). Plastics and microplastics in the oceans: From emerging pollutants to emerged threat. Mar. Environ. Res. 128, 2–11. doi:10.1016/j.marenvres.2016.05.012
Babu, R. P., O’Connor, K., and Seeram, R. (2013). Current progress on bio-based polymers and their future trends. Prog. Biomaterials 2, 8. doi:10.1186/2194-0517-2-8
Bello, L. D. (2022). How plastic is fuelling a hidden climate crisis in Southeast Asia. China Dialogue Ocean. Available at: https://chinadialogueocean.net/en/pollution/how-plastic-is-fuelling-a-hidden-climate-crisis-in-southeast-asia/ (Accessed May 19, 2022).
Brooks, A. L., Wang, S., and Jambeck, J. R. (2018). The Chinese import ban and its impact on global plastic waste trade. Sci. Adv. 4, eaat0131–8. doi:10.1126/sciadv.aat0131
Cao, C., Bian, C., Wang, G., Bai, B., Xie, Y., and Jin, H. (2020). Co-gasification of plastic wastes and soda lignin in supercritical water. Chem. Eng. J. 388, 124277. doi:10.1016/j.cej.2020.124277
Carr, S. A., Liu, J., and Tesoro, A. G. (2016). Transport and fate of microplastic particles in wastewater treatment plants. Water Res. 91, 174–182. doi:10.1016/j.watres.2016.01.002
Chen, H. L., Nath, T. K., Chong, S., Foo, V., Gibbins, C., and Lechner, A. M. (2021a). The plastic waste problem in Malaysia: Management, recycling and disposal of local and global plastic waste. SN Appl. Sci. 3, 437–515. doi:10.1007/s42452-021-04234-y
Chen, H., Wan, K., Zhang, Y., and Wang, Y. (2021b). Waste to wealth: Chemical recycling and chemical upcycling of waste plastics for a great future. ChemSusChem 14, 4123–4136. doi:10.1002/cssc.202100652
Chen, Y., Cui, Z., Cui, X., Liu, W., Wang, X., Li, X. X., et al. (2019). Life cycle assessment of end-of-life treatments of waste plastics in China. Resour. Conservation Recycl. 146, 348–357. doi:10.1016/j.resconrec.2019.03.011
China Dialogue (2021). Reduce plastic production to help Southeast Asia. Southeast Asia: The Third Pole.
Coram, A., Abreo, N. A. S., Ellis, R. P., and Thompson, K. F. (2021). Contribution of social media to cetacean research in SoutheastSoutheast Asia: Illuminating populations vulnerable to litter. Biodivers. Conservation 30, 2341–2359. doi:10.1007/s10531-021-02196-6
Curren, E., Kuwahara, V. S., Yoshida, T., and Leong, S. C. Y. (2021). Marine microplastics in the ASEAN region: A review of the current state of knowledge. Environ. Pollut. 288, 117776. doi:10.1016/j.envpol.2021.117776
Curren, E., and Leong, S. C. Y. (2019). Profiles of bacterial assemblages from microplastics of tropical coastal environments. Sci. Total Environ. 655, 313–320. doi:10.1016/j.scitotenv.2018.11.250
Elsawy, M. A., Kim, K. H., Park, J. W., and Deep, A. (2017). Hydrolytic degradation of polylactic acid (PLA) and its composites. Renew. Sustain. Energy Rev. 79, 1346–1352. doi:10.1016/j.rser.2017.05.143
Energy (2020). Gaining Traction: Southeast Asian countries look at WtE plants to solve garbage woes. Southeast Asia: Southeast Asia Infrastructure.
Ericsson, E., and Johansson, E. (2018). “Quantification for the flow of microplastic particles in urban environment,” in A case of the Chao Phraya River (Bangkok Thailand: KTH Royal Institute Of Technology).
Fauziah, S. H., Rizman-Idid, M., Cheah, W., Loh, K. H., and Sharma, S. (2021). Marine debris in Malaysia: A review on the pollution intensity and mitigating measures. Mar. Pollut. Bull. 167, 112258. doi:10.1016/j.marpolbul.2021.112258
Fivga, A., and Dimitriou, I. (2018). Pyrolysis of plastic waste for production of heavy fuel substitute: A techno-economic assessment. Energy 149, 865–874. doi:10.1016/j.energy.2018.02.094
Fraccascia, L., Giannoccaro, I., and Albino, V. (2019). Business models for industrial symbiosis: A taxonomy focused on the form of governance. Resour. Conservation Recycl. 146, 114–126. doi:10.1016/j.resconrec.2019.03.016
Fredi, G., and Dorigato, A. (2021). Recycling of bioplastic waste: A review. Adv. Industrial Eng. Polym. Res. 4, 159–177. doi:10.1016/j.aiepr.2021.06.006
Geyer, R., Jambeck, J. R., and Law, K. L. (2017b). Production, use, and fate of all plastics ever made - supplementary Information. Sci. Adv. 3, 19–24.
Geyer, R., Jambeck, J. R., and Law, K. L. (2017a). Production, use, and fate of all plastics ever made. Sci. Adv. 3, 17007822–e1700829. doi:10.1126/sciadv.1700782
Gupta, P., Kumar, A., and Sinhamahapatra, A. (2022). Liquid fuel from plastic waste. Oxford: Elsevier, 904–916. doi:10.1016/B978-0-12-820352-1.00128-0
Hassan, M. N., Rahman, R. A., Chong, T. L., Zakaria, Z., Awang, M., and Hassan, M. N. (2000). Waste recycling in Malaysia: Problems and prospects. Waste Manag. Res. 18, 320–328. doi:10.1034/j.1399-3070.2000.00133.x
Horton, A. A., Walton, A., Spurgeon, D. J., Lahive, E., and Svendsen, C. (2017). Microplastics in freshwater and terrestrial environments: Evaluating the current understanding to identify the knowledge gaps and future research priorities. Sci. Total Environ. 586, 127–141. doi:10.1016/j.scitotenv.2017.01.190
Huo, E., Lei, H., Liu, C., Zhang, Y., Xin, L., Zhao, Y., et al. (2020). Jet fuel and hydrogen produced from waste plastics catalytic pyrolysis with activated carbon and MgO. Sci. Total Environ. 727, 138411. doi:10.1016/j.scitotenv.2020.138411
IFBB (2022). Biopolymers facts and statistics: Production capacities, processing routes, feedstock, land and water use. Hannover, Germany: Institute for Bioplastics and Biocomposites.
Jahnke, A., Arp, H. P. H., Escher, B. I., Gewert, B., Gorokhova, E., Kühnel, D., et al. (2017). Reducing uncertainty and confronting ignorance about the possible impacts of weathering plastic in the marine environment. Environ. Sci. Technol. Lett. 4, 85–90. doi:10.1021/acs.estlett.7b00008
Jain, A. (2020). Trash trade wars: Southeast Asia’s problem with the world’s waste. Council on Foreign Relations. Available at: https://www.cfr.org/in-brief/trash-trade-wars-southeast-asias-problem-worlds-waste (Accessed May 8, 2020).
Jambeck, J. R., Geyer, R., Wilcox, C., Siegler, T. R., Perryman, M., Andrady, A., et al. (2015). Plastic waste inputs from land into the ocean. Science 347, 768–771. doi:10.1126/science.1260352
Jiang, J., Shi, K., Zhang, X., Yu, K., Zhang, H., He, J., et al. (2022). From plastic waste to wealth using chemical recycling: A review. J. Environ. Chem. Eng. 10, 106867. doi:10.1016/j.jece.2021.106867
Joshi, C. A., and Seay, J. R. (2016). An appropriate technology based solution to convert waste plastic into fuel oil in underdeveloped regions. J. Sustain. Dev. 9, 133–143. doi:10.5539/jsd.v9n4p133
Julius, B., and Trajano, C. (2022). Plastic pollution in Southeast Asia: Wasted opportunity ? Singapore: RSIS.
KASA (2021). Malaysia plastics sustainability roadmap 2021-2030. Available at: www.kasa.gov.my.
KLEAN (2021). Klean turning trash into cash. Available at: www.klean.asia.
Kunwar, B., Cheng, H. N., Chandrashekaran, S. R., and Sharma, B. K. (2016). Plastics to fuel: A review. Renew. Sustain. Energy Rev. 54, 421–428. doi:10.1016/j.rser.2015.10.015
Lau, W. W. Y., Shiran, Y., Bailey, R. M., Cook, E., Stuchtey, M. R., Koskella, J., et al. (2020). Evaluating scenarios toward zero plastic pollution. Science 369, 1455–1461. doi:10.1126/science.aba9475
Lebreton, L. C. M., Van Der Zwet, J., Damsteeg, J. W., Slat, B., Andrady, A., and Reisser, J. (2017). River plastic emissions to the world’s oceans. Nat. Commun. 8, 15611. doi:10.1038/ncomms15611
Leslie, H. A., van Velzen, M. J. M., Brandsma, S. H., Vethaak, A. D., Garcia-Vallejo, J. J., and Lamoree, M. H. (2022). Discovery and quantification of plastic particle pollution in human blood. Environ. Int. 163, 107199. doi:10.1016/j.envint.2022.107199
Liu, Z., Liu, W., Walker, T. R., Adams, M., and Zhao, J. (2021). How does the global plastic waste trade contribute to environmental benefits: Implication for reductions of greenhouse gas emissions? J. Environ. Manag. 287, 112283. doi:10.1016/j.jenvman.2021.112283
Loh, P. Y. (2020). Why is Southeast Asia so bad at managing its waste. kontinentalist. Available at: https://kontinentalist.com/stories/southeast-asia-ocean-plastics-pollution-waste-management (Accessed April 13, 2020).
Marks, D. (2019). Southeast Asia’s plastic waste problem. East Asia Forum. Available at: https://www.eastasiaforum.org/2019/06/26/southeast-asias-plastic-waste-problem/ (Accessed June 26, 2019).
MSA (2018). Your trusted partner in waste management. Available at: http://msa.co.th/en/products-services.html.
Nandakumar, A., Chuah, J. A., and Sudesh, K. (2021). Bioplastics: A boon or bane? Renew. Sustain. Energy Rev. 147, 111237. doi:10.1016/j.rser.2021.111237
Napper, I. E., and Thompson, R. C. (2016). Release of synthetic microplastic plastic fibres from domestic washing machines: Effects of fabric type and washing conditions. Mar. Pollut. Bull. 112, 39–45. doi:10.1016/j.marpolbul.2016.09.025
New Strait Times (2019). 62 Malaysian companies hold permits to import plastic waste. New Strait Times. Available at: https://www.nst.com.my/news/nation/2019/06/494782/62-malaysian-companies-hold-permits-import-plastic-waste (Accessed June 8, 2019).
NTU Singapore (2022). Turning plastic trash into clean hydrogen fuel. Available at: https://www.ntu.edu.sg/news/detail/turning-plastic-trash-into-clean-hydrogen-fuel.
Omeyer, L. C. M., Duncan, E. M., Aiemsomboon, K., Beaumont, N., Bureekul, S., Cao, B., et al. (2022). Priorities to inform research on marine plastic pollution in Southeast Asia. Sci. Total Environ. 841, 156704. doi:10.1016/j.scitotenv.2022.156704
Owusu, P. A., Banadda, N., Seay, J., Kiggundu, N., Banadda, N., Zziwa, A., et al. (2018). Reverse engineering of plastic waste into useful fuel products. J. Anal. Appl. Pyrolysis 130, 285–293. doi:10.1016/j.jaap.2017.12.020
Praveena, S. M., and Aris, A. Z. (2021). The impacts of COVID-19 on the environmental sustainability: A perspective from the SoutheastSoutheast Asian region. Environ. Sci. Pollut. Res. 28, 63829–63836. doi:10.1007/s11356-020-11774-0
Qu, S., Guo, Y., Ma, Z., Chen, W. Q., Liu, J., Liu, G., et al. (2019). Implications of China’s foreign waste ban on the global circular economy. Resour. Conservation Recycl. 144, 252–255. doi:10.1016/j.resconrec.2019.01.004
Ragaert, K., Delva, L., and Van Geem, K. (2017). Mechanical and chemical recycling of solid plastic waste. Waste Manag. 69, 24–58. doi:10.1016/j.wasman.2017.07.044
Rahimi, A., and García, J. M. (2017). Chemical recycling of waste plastics for new materials production. Nat. Rev. Chem. 1, 0046. doi:10.1038/s41570-017-0046
Recycling Technologies (2018). Recycling technologies. Available at: https://recyclingtechnologies.co.uk.
Riani, E., and Cordova, M. R. (2022). Microplastic ingestion by the sandfish Holothuria scabra in Lampung and Sumbawa, Indonesia. Mar. Pollut. Bull. 175, 113134. doi:10.1016/j.marpolbul.2021.113134
Ritchie, H., and Roser, M. (2018). Plastic pollution. Our World in Data. Available at: https://ourworldindata.org/plastic-pollution (Accessed April, 2022).
Saebea, D., Ruengrit, P., Arpornwichanop, A., and Patcharavorachot, Y. (2020). Gasification of plastic waste for synthesis gas production. Energy Rep. 6, 202–207. doi:10.1016/j.egyr.2019.08.043
Salaudeen, S. A., Arku, P., and Dutta, A. (2019). “10 - gasification of plastic solid waste and competitive technologies,” in Plastics design library, 269–293. doi:10.1016/B978-0-12-813140-4.00010-8
Santodomingo, N., Perry, C., Waheed, Z., Syed Hussein, M. A. B., Rosedy, A., and Johnson, K. G. (2021). Marine litter pollution on coral reefs of Darvel Bay (East Sabah, Malaysia). Mar. Pollut. Bull. 173, 112998. doi:10.1016/j.marpolbul.2021.112998
Sasaki, S. (2021). The effects on Thailand of China’s import restrictions on waste: Measures and challenges related to the international recycling of waste plastic and e-waste. J. Material Cycles Waste Manag. 23, 77–83. doi:10.1007/s10163-020-01113-3
SCG (2019). Recycled plastic road. Available at: https://www.scg.com/sustainability/circular-economy/en/collaboration-projects/recycled-plastic-road/.
Singh, M. V., Kumar, S., and Sarker, M. (2018). Waste HD-PE plastic, deformation into liquid hydrocarbon fuel using pyrolysis-catalytic cracking with a CuCO3 catalyst. Sustain. Energy & Fuels 2, 1057–1068. doi:10.1039/C8SE00040A
Singh, N., Ogunseitan, O. A., Wong, M. H., and Tang, Y. (2022). Sustainable materials alternative to petrochemical plastics pollution: A review analysis. Sustain. Horizons 2, 100016. doi:10.1016/j.horiz.2022.100016
Singhvi, M. S., and Gokhale, D. V. (2019). Lignocellulosic biomass: Hurdles and challenges in its valorization. Appl. Microbiol. Biotechnol. 103, 9305–9320. doi:10.1007/s00253-019-10212-7
Tan, E., Jaafar, N. F., Aileen Tan, S. H., and Mohd Zanuri, N. B. (2022). A review of plastic and microplastic pollution towards the Malaysian marine environment. IOP Conf. Ser. Earth Environ. Sci. 1013, 012012. doi:10.1088/1755-1315/1013/1/012012
Tee, Y. H., Ibrahim, Y. S., and Khalik, W. M. A. W. M. (2020). Microplastic abundance, distribution, and composition in sungai Dungun, terengganu, Malaysia. Sains Malays. 49, 1479–1490. doi:10.17576/jsm-2020-4907-01
Teoh, M. (2020). Blue skies, less waste: Covid-19 and the MCO’s effects on the environment. TheStar. Available at: https://www.thestar.com.my/lifestyle/living/2020/04/22/earth-day-a-wake-up-call-to-lead-more-environmentally-sustainable-lives (Accessed April 22, 2020).
The Japan Times (2020). Plastic piles up in Thailand as virus fight sidelines pollution battle. Available at: https://www.japantimes.co.jp/news/2020/05/12/asia-pacific/plastic-thailand-coronavirus-pollution/ (Accessed May 12, 2020).
Tun, M. M., Palacky, P., Juchelkova, D., and Síťař, V. (2020). Renewable waste-to-energy in southeast Asia: Status, challenges, opportunities, and selection of waste-to-energy technologies. Appl. Sci. Switz. 10, 7312–7328. doi:10.3390/app10207312
UN environment (2017). Waste management in ASEAN countries: Summary report. Nairobi, Kenya: UN Environment Programme.
Unilever (2017). Unilever develops new technology to tackle the global issue of plastic sachet waste. HUL.
Velis, C. (2014). Global recycling markets plastic waste. A story for one player-China. Int. Solid Waste Assoc. 2014, 1–66. doi:10.13140/RG.2.1.4018.4802
Walker, T. R., and Xanthos, D. (2018). A call for Canada to move toward zero plastic waste by reducing and recycling single-use plastics. Resour. Conservation Recycl. 133, 99–100. doi:10.1016/j.resconrec.2018.02.014
Wang, J., Peng, J., Tan, Z., Gao, Y., Zhan, Z., Chen, Q., et al. (2017). Microplastics in the surface sediments from the Beijiang River littoral zone: Composition, abundance, surface textures and interaction with heavy metals. Chemosphere 171, 248–258. doi:10.1016/j.chemosphere.2016.12.074
Wen, Z., Xie, Y., Chen, M., and Dinga, C. D. (2021). China’s plastic import ban increases prospects of environmental impact mitigation of plastic waste trade flow worldwide. Nat. Commun. 12, 425. doi:10.1038/s41467-020-20741-9
Yao, D., Li, H., Dai, Y., and Wang, C. H. (2021). Impact of temperature on the activity of Fe-Ni catalysts for pyrolysis and decomposition processing of plastic waste. Chem. Eng. J. 408, 127268. doi:10.1016/j.cej.2020.127268
Yoshida, A. (2022). China’s ban of imported recyclable waste and its impact on the waste plastic recycling industry in China and Taiwan. J. Material Cycles Waste Manag. 24, 73–82. doi:10.1007/s10163-021-01297-2
Yuen, M., Koh, W., and Pfordten, D. (2020). An mco “side-effect”: A breath of fresh air. MCO. TheStar.
Keywords: plastic waste, microplastics, Southeast Asia, waste management, global trade
Citation: Ng CH, Mistoh MA, Teo SH, Galassi A, Ibrahim A, Sipaut CS, Foo J, Seay J, Taufiq-Yap YH and Janaun J (2023) Plastic waste and microplastic issues in Southeast Asia. Front. Environ. Sci. 11:1142071. doi: 10.3389/fenvs.2023.1142071
Received: 11 January 2023; Accepted: 20 March 2023;
Published: 07 April 2023.
Edited by:
Xiaoguang Duan, University of Adelaide, AustraliaReviewed by:
Rakesh Kumar, Independent researcher, Rajgir, IndiaCopyright © 2023 Ng, Mistoh, Teo, Galassi, Ibrahim, Sipaut, Foo, Seay, Taufiq-Yap and Janaun. This is an open-access article distributed under the terms of the Creative Commons Attribution License (CC BY). The use, distribution or reproduction in other forums is permitted, provided the original author(s) and the copyright owner(s) are credited and that the original publication in this journal is cited, in accordance with accepted academic practice. No use, distribution or reproduction is permitted which does not comply with these terms.
*Correspondence: Chi Huey Ng, chihueyng@ums.edu.my; Yun Hin Taufiq-Yap, taufiq@upm.edu.my; Jidon Janaun, jidon@ums.edu.my
Disclaimer: All claims expressed in this article are solely those of the authors and do not necessarily represent those of their affiliated organizations, or those of the publisher, the editors and the reviewers. Any product that may be evaluated in this article or claim that may be made by its manufacturer is not guaranteed or endorsed by the publisher.
Research integrity at Frontiers
Learn more about the work of our research integrity team to safeguard the quality of each article we publish.