- 1Department of Chemical Engineering, College of Engineering, King Khalid University, Abha, Saudi Arabia
- 2Research Laboratory of Processes, Energetics, Environment and Electrical Systems, National School of Engineers of Gabes, Gabes University, Gabes, Tunisia
- 3Department of Social Environmental Sciences, Faculty of Language History and Geography, Ankara University, Ankara, Turkey
- 4Department of Civil Engineering, College of Engineering, University of Diyala, Diyala, Iraq
- 5Civil, Environmental and Natural Resources Engineering, Lulea University of Technology, Lulea, Sweden
- 6Department of Computer Science, Al-Turath University College Al Mansour, Baghdad, Iraq
A wide range of studies has been carried out to describe the equilibrium data of adsorption for the surface adsorption process. However, no extensive investigation has been carried out to evaluate the oil sludge based activated carbon surface adsorption. Therefore, the possibility of carbon active production using different oil sludges and consequently the adsorption mechanism of these kind of adsorbents is still unknown. In this study, a novel low-cost approach was introduced to synthesize the activated carbon using oil sludge applying a two-step process including carbonization and chemical activation. In this way, four different types of oil sludges were characterized and then applied to synthesize different carbon actives and their performance were investigated as an adsorbent. The results showed that all synthesized activated carbons, with about 6% ash and pH = 7 and the specific surface area of 110 m2/gr, have the ability to treatment of oily wastewater; which can be referred to the high carbon content (>80%). The iodine number and the efficiency of prepared activated carbon were obtained as 406.8 mg/g and 94%, respectively. The adsorption process was also studied at different process conditions such as temperature (308–338 K), pH value (3–9) and adsorbent amount (50–200 mg/L) to find the optimum condition for wastewater treatment. The results show that the pH value has an optimum in the adsorption rate (the maximum adsorption was measured at pH = 5) and the adsorption capacity can be reduced by increasing the temperature or decreasing the adsorbent amount. Moreover, three different adsorption isotherm models were applied, i.e., Langmuir, Temkin, and Freundlich isotherms; which the Langmuir equation was more suitable than others investigated isotherm models with R2 ≈ 0.999.
1 Introduction
Oil removal methods in wastewater treatment are the most important processes in many industries; because the oil contaminated wastewater brings a high organic load to the downstream process units. Oily wastewaters are produced in industries such as oil, petrochemical and food industries. These kinds of wastewaters are mainly in the form of oil/water emulsions. Oil separation requires an understanding of the physical and chemical properties of oil/water emulsions. In order to choose the appropriate separation process, several properties that play a key role in the stability of oil/water emulsions should be measured (Birkland et al., 2003; Cetin, 2020).
Considering the position of the oil industry in the world and its functional diversity, the management of industrial waste, including oily effluents (Saba, 2001), is one of the problems caused by the incompatibility of consumables with the environment (Hamta and Dehghani, 2017; Yadav et al., 2020).
In oil industries, wastewater is produced in different units; which makes the wastewater treatment process difficult (El-Gendy and Nassar, 2021). The separation of petroleum compounds is one of the most difficult types of wastewater treatment which its entry into the environment has serious negative effects on the health of human beings (Aron et al., 2021; Zhao et al., 2021). The petroleum substances and their derivatives are stable and hard to degrade in natural conditions and as a result cause sensitivity and other irreparable problems (Hamta et al., 2017). Therefore, removing these compounds from wastewater is one of the most research priorities (Eryılmaz and Genc, 2021).
On the other hand, due to the increasing in energy consumption, the use of fossil resources has increased subsequently, followed by the release of oil pollution in the environment (Munoz-Cupa et al., 2021). With the growth of offshore oil industries and the need to transport oil by sea, oil spill and the release of liquid petroleum hydrocarbon into the environment became a serious threat to marine ecosystems (Hamta et al., 2018; Zhao et al., 2021).
Another problem in the oil refinery industries is the production of high-viscosity wastes named oil sludge (Nie et al., 2020). Generally, oil sludge contains various amounts of solids, water, petroleum hydrocarbon and heavy compounds. According to research conducted by the US Environmental Protection Agency, each refinery in the United States produces an average of 30,000 tons of oil sludge per year. It should be noted that the improper burial or insufficient treatment of the oil sludge can be a serious threat to the environment and human health (Liu et al., 2018).
There are different ways to reduce the amount of oil sludge (Atallah et al., 2019; Solé-Bundó et al., 2020). One of these methods is the incineration process, which is used for energy recovery, but is becoming obsolete due to the low thermal energy, and the emission of polluting gases (Hamta et al., 2020). The landfill is another way which is not cost-effective due to the high level of consumed land (Awa and Hadibarata, 2020; Urionabarrenetxea et al., 2021). Another option to reduce the oil sludge pollution is thermal treatment or pyrolysis. The pyrolysis is a type of thermal treatment in which materials are carbonized in the atmosphere without the presence of oxygen (Gao et al., 2020; Hamta et al., 2022). In this method, in addition to reducing the volume of oil sludge, the leaching of metals is also reduced (Leng et al., 2018).
In the recent years, porous materials have attracted a lot of attention due to their potential application in various fields, from chemistry to biotechnology (Gong et al., 2020; Zhang et al., 2022). In this regard, nano-sized porous materials, due to their high surface area and large length-to-diameter ratio, have been specifically used as an adsorbent (Xue et al., 2019; Liu et al., 2020).
Activated carbon is a solid neutral adsorbent material that is generally can be used to separate various pollutants from water and gases (Shukla, 2021). Activated carbon is one of the most applicable porous materials, which has received much attention in the adsorption and wastewater purification processes (Hamta et al., 2021; Zhang et al., 2021). The advantages of the activated carbon, including high flexibility, and high adsorption and desorption rate, have caused a lot of research in the field of process optimization and industrialization (Mariana et al., 2021).
For the production of activated carbon, different methods such as electric arc discharge and laser ablation have been already applied, which are complex and expensive processes with low-efficiency. Usually, the electrospinning activated carbon production is preferred due to its simplicity and low cost. In the electrospinning procedure, a power source with high voltage is applied to produce the charge of electric in the polymer solution (Kurtan, 2021).
In order to produce activated carbon, a power source with high voltage is applied to the obtained solution of the polymer. As the solution passes through the capillary tube, due to the generated electric field between the collector and the capillary tube, the solution is charged and drawn from the capillary tube to the collector (Yang et al., 2022). Because of the movement of the fluid, the solvent is evaporated and consequently the filaments are generated on the collector with the sub-micron diameter. Therefore, the spiral motion is induced into the charged system, because of the electric force interaction, viscoelastic force, the flow surface charge, and surface tension and subsequently, the activated carbon materials are produced in the form of interconnected layers (Li et al., 2023).
Recently, lots of researchers all around the world have conducted numerous researches to convert various types of oil sludge into activated carbon (Li et al., 2019; Mojoudi et al., 2019). However, no comprehensive study has been conducted in the field of comparing the types of obtained activated carbon with different sources of oil sludge. Also, the effect of different parameters on the adsorption of the activated carbon has not been fully investigated, and the adsorption mechanism in this type of adsorbent is still unknown (Xu et al., 2022).
As mentioned, the existence of the oil sludge is one of the biggest environmental problems worldwide, and on the other hand, the production of high-performance adsorbents is mandatory to wastewater management and removing the oil pollutants from the environment. Considering the high cost of commercial activated carbon and the limitation of primary resources for their production, the use of wastes such as sludge from oil industries as raw material for the production of activated carbon is important from several aspects, including the fact that its production is reliable economically and technically; which can solve the environmental concerns such as the accumulation of oil sludge, reduce soil and water pollution (Padaki et al., 2015; Zhao et al., 2021). Therefore, in this study, oil sludge has been applied as a carbon source in the production of high-performance adsorbents, and its performance in removing the petroleum substances (diesel and motor oil) has been investigated.
In this work, several activated carbons were synthesized using four different types of oil sludges and their properties were characterized as a novel approach to convert the hazardous pollutant to a high-performance adsorbent. After that, the equilibrium adsorption of oily wastewater was studied on each of the synthesized activated carbons. Furthermore, different adsorption isotherms were applied to correlate the process of surface adsorption of different synthesized carbon actives.
2 Materials and methods
2.1 Characterization
One of the most important characteristics of activated carbon is its specific surface, which was determined by the BET method (Huang, 1978). This method is based on the physical adsorption of nitrogen gas at a temperature of 350°C. In this method, the samples were first heated to 200°C for degassing. Then, at a constant temperature, the amount of nitrogen adsorbed at different pressures was checked using the nitrogen adsorption and removal system. These isotherms are used to determine the specific surface area, the volume of micropores, mesopores, the total volume of pores, and the distribution of particle size.
In order to measure the amount of moisture, ash and pH of the oil sludge samples, ASTM D2216-80, ASTM D482-87 and ASTM D4980-89 standards were applied, respectively (Mahzoonieh et al., 2017). Moreover, the elemental analysis was performed in order to determine the chemical composition of the oil sludge samples. In this way, a small amount of carbon, hydrogen, nitrogen and sulfur elements of the raw material were determined using the Vario EL III CHNS equipment. To determine the weight loss of the oil sludge samples, 80 mg of each sample was placed in the furnace (the model Rheometric Scientific), in the presence of nitrogen gas and its temperature was increased from 25 to 1200°C with a heating rate of 10°C/min (Zhou et al., 2023).
To determine the iodine number of the prepared activated carbon, according to the ASTM D4607-94 standard, after preparing 0.1 normal solutions of sodium thiosulfate, iodine and potassium iodate were standardized and the amount of iodine adsorbed by the sodium thiosulfate solution was determined (Patnukao and Pavasant, 2008). The iodine milligrams that can be adsorbed using 1 g of adsorbent is defined as iodine number. In such a way that a certain amount of the adsorbent, whose moisture has already been removed, was gradually mixed with 10 mL of 5% hydrochloric acid solution in a 250 mL Erlenmeyer flask. Then the mixture was placed on the hotplate and boiled for 30 s. After the Erlenmeyer flask was cooled, 100 mL of standard iodine solution was immediately added to the solution and was shaken vigorously for 30 s. After this period of time, the solution was filtered quickly and 50 mL of the solution was separated for titration with 0.1 normal sodium thiosulfate. Finally, the amount of adsorbed iodine can be determined by measuring the amount of consumed thiosulfate (Bai et al., 2023).
2.2 Activated carbon production
To produce an adsorbent from oil sludge, the oil sludge must first be produced in the form of powder and then the activation process should be applied. These two processes, which are called carbonization and activation are explained in detail, respectively.
2.2.1 Carbonization
To carry out the carbonization process and produce oil sludge in the form of solid powder, first, a special SS-316 container containing a certain amount of oil sludge was placed inside the electric vertical furnace tank. Then, for 20 min, nitrogen gas with a flow rate of 200 mL/min was passed in the inner space of the furnace, which was established in order to neutralize the environment (Hu et al., 2022).
After creating an oxygen-free environment, the temperature was increased from ambient temperature to 700°C at a rate of 10°C/min, and the sample was placed at 700°C for 1 h. Then the furnace temperature was cooled down to the ambient temperature at a rate of 10°C/min. Finally, the sample was removed from the furnace and crushed using a porcelain mortar. The carbonization stage causes fundamental changes in the physical properties, structure and chemical composition of the activated carbon (Bai et al., 2019).
2.2.2 Chemical activation
Activation is a process that should be applied to improve the surface porosity of the obtained carbon powder. Activated carbon, which have a porous surface, can be used in various fields such as the production of electrode materials, nanofiltration, and adsorbents to remove toxic pollutants. During the activation process, the surface structure of carbon powder is modified, which leads to the creation of new micropores and a large specific surface area (Hu et al., 2022).
In this manner, according to the saturation ratio, 40 g of zinc chloride powder (ZnCl2) was dissolved in 200 mL of distilled water and 20 g of carbonized materials were added to it. The obtained mixture was placed on a magnetic stirrer at a temperature of 70°C for 7 h. Next, the saturated system was placed in an oven with a temperature of 110°C for 24 h to completely remove the moisture. Then, the dried saturated material was placed in a vertical furnace with a temperature of 800°C for 1 h. The prepared adsorbent was washed 4 times with distilled water until its pH reached about 6.5. Finally, the obtained neutral adsorbent was again placed in an oven with a temperature of 110°C for 24 h and the dried sample was kept in a closed container for adsorption tests (Boustila et al., 2022).
2.3 Adsorption test
Due to the exothermic nature of the adsorption processes, the high pressure and low temperature are thermodynamically favorable. It should be noted that there is no chemical reaction in the adsorption test between species and there are just van der Waals forces between the adsorbent and adsorbing molecules without any electron transfer (Lin et al., 2021).
As the activation energy of the adsorption process is close to zero and the heat of reaction is low, the adsorption is a fast process. In order to perform the adsorption test, a certain amount of adsorbent material was placed in a solution with a certain pollution using an adsorption reactor. The amount of adsorption was measured under different operating conditions using a scale with an accuracy of ±0.0001 g.
The adsorption study of the oily wastewater by the synthesized activated carbon was carried out in batch mode. In order to carry out the adsorption test, the oily wastewater consist of a stable emulsion of oil in water, spiked at four different concentrations from 50 to 200 mg/L using an amber glass bottle to mix 1,000 mL water with 15 mL tween80 as surfactant and different concentrations of gasoil at ambient temperature and 10,000 rpm for 30 min. As the Higher oil concentrations are not applicable the maximum concentration of 200 mg/L was applied.
To measure the maximum adsorption, a plug of salinized glass wool was inserted at the bottom of a glass column. The synthesized oily wastewater was added to the batch and was placed for 30 min the oil contaminated wastewater was analyzed for remaining oil concentrations and the experiments were repeated three times and the average data were reported. The effect of pH was checked on adsorption test by manipulating the pH value in the range of 3–9. In order to adjust the pH value NaOH or HCl was added to the solution. The experiments were carried out in a water bath to adjust the temperature in the range of 308–338 K (Guan et al., 2021).
2.4 Adsorption isotherm models
The adsorption mechanism can be determined using isotherm models to optimize the amount of adsorbent required in a process. In order to investigate the relationship between the solution concentration at equilibrium condition and the adsorbed concentration, different isotherm models can be applied.
When an adsorbent is placed in contact with a pollutant solution, the pollutant concentration on the adsorbent surface increases until reaching the thermodynamic equilibrium. This equilibrium state, known as the adsorption isotherm, is the primary basis for the design of adsorption systems. These data can be used to design an optimized chemical adsorption process. In order to study the adsorbents behavior in treatment process, the experimental data should be correlated using Langmuir, Freundlich and Temkin isotherm adsorption models.
The Langmuir model, is a presented model for the surface adsorption process on a flat surface. This model considers the adsorption process as a continuous process in which the rate of adsorption and desorption is equal. In this model, the adsorption energy is considered as a constant value in all the free sites, and the adsorbent surface is assumed as a homogeneous surface (Chatla et al., 2022). This model assumes that each adsorption site can adsorb only one molecule. the linear Langmuir equation is presented in Eq. 1.
In Eq. 1, Kl is the Langmuir constant, Ce is the equilibrium concentration, and qm is the maximum capacity of the adsorption process; so, the validity of the Langmuir model can be obtained using a linear plot of Ce/qe versus Ce.
The Freundlich isotherm can also be used to describe the experimental equilibrium data, as Eq. 2; where KF and n are temperature-dependent constants of Freundlich isotherm. As it is obvious the constants of the Freundlich equation can be calculated plotting lnqe in terms of lnCe.
In Eq. 2, KF represents the irreversibility of the process, and the larger the value of n, the more the isotherm deviates from the linear state and if n = 1, the Freundlich equation will be linear (Misran et al., 2022). One of the assumptions in the Freundlich model is that the surface is non-uniform in terms of distribution of energy, and sites, with the equal energy, are placed next to each other.
The linearized Temkin isotherm is shown in Eq. 3, where T is the temperature in kelvin, R is the universal gas constant, and KT and b are adjustable parameters. As it can be seen, the constants of the Temkin equation can be presented by plotting qe in terms of lnCe.
3 Results and discussion
The properties of the different oil sludges, including moisture, ash percentage and pH, can be found in Table 1. As can be seen from Table 1, it can be understood that the moisture, ash percentage and the pH of all oil sludge samples are about 5.5%, 6% and 7, respectively.

TABLE 1. The elemental characteristics and properties of the different types of investigated industrial oil sludges.
The carbon, hydrogen, nitrogen and sulfur content of the different types of investigated industrial oil sludge is also shown in Table 1. Due to the high amount of carbon in the oil sludge of all samples, it can be concluded that these kinds of oil sludges can be applied to prepare a high-performance activated carbon. Also, the low amount of oxygen (about 3%) in all the oil sludge samples indicates the presence of small amounts of oxygenated surface groups in the samples.
The thermal analysis of the samples showed that in all the investigated samples, with the increase in temperature up to 300°C, a small percentage of the weight decreases due to the loss of moisture. But in the range of 300–420°C, about 35% of the raw materials weights are reduced. Decomposition and evaporation of hydrocarbons with lower molecular weight can be the cause of weight loss in this stage. It should be noted that in the range of 420–630°C, heavy hydrocarbons and organic substances are decomposed and weight loss in this stage is obtained around 40% by weight. After that, the weight loss gradually decreases due to the depletion of moisture and volatile substances in the samples. Finally, in the last stage from 630°C to 810°C, the oil sludge is almost stable and around 10% weight loss is probably due to the decomposition of minerals. According to the results, the temperature of 700°C was chosen as the carbonization temperature due to the approximately constant weight of the sample (Ge et al., 2019).
The surface characteristics and the pore structure of the prepared activated carbons are shown in Table 2. According to the results, the specific surface of the produced activated carbon is upper than 109.23 m2/g, which mostly includes pores in the range of micro-pores and meso-pores.
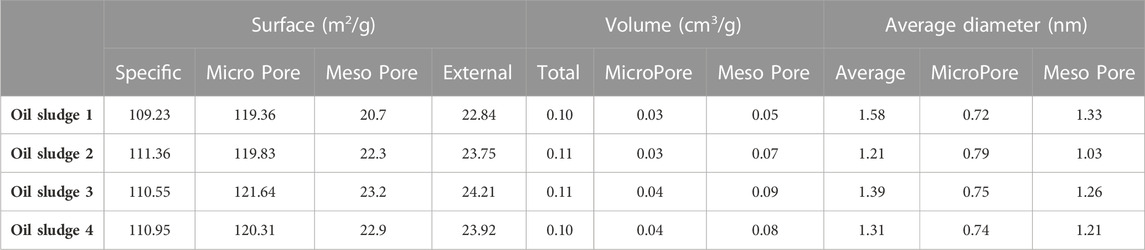
TABLE 2. The surface characteristics and the pore structure of the prepared activated carbon using different types of oil sludge.
The iodine number and efficiency of activated carbon prepared from different sources of oil sludge are listed in Table 3. The observed mass reduction in carbonization process is due to the presence of high minerals; and the mass reduction during activation process can be related to the loss of some material during the washing and the decomposition reactions between the carbonized material and the activating agent (Pan and Chen, 2021).
The effects of temperature on the oil adsorption of the wastewater on the obtained carbon actives is shown in Figure 1. As can be seen, with an increase in temperature, all the presented results in Figure 1 show a decrease in adsorbent amount which can be related to the exothermic reaction of the adsorption process. The temperature effects on the treatment of oily wastewater using oil sludge one and oil sludge 4 carbon actives through the linearized Langmuir isotherm are shown in Figure 2.
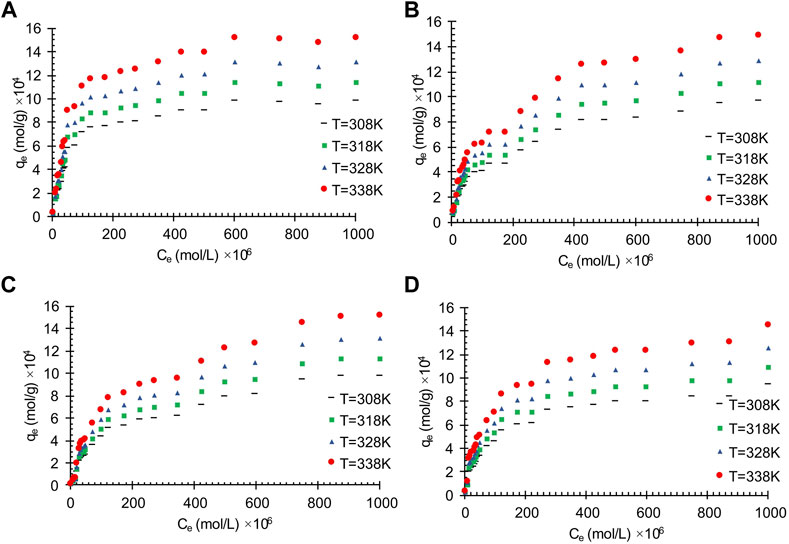
FIGURE 1. The effects of temperature on the wastewater treatment by applying oil adsorption on the obtained carbon actives from (A) Oil sludge 1; (B) Oil sludge 2; (C) Oil sludge 3; (D) Oil sludge 4 at pH = 5 and adsorbent concentration of 100 mg/L.
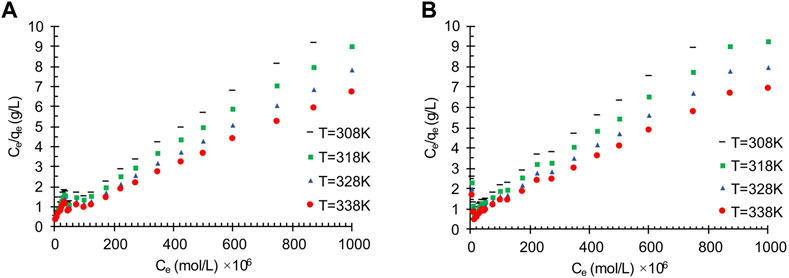
FIGURE 2. The effects of temperature on the wastewater treatment by using oil adsorption applying linearized Langmuir isotherm on the obtained carbon actives from (A) Oil sludge 1; (B) Oil sludge 4 at pH = 5 and adsorbent concentration of 100 mg/L.
The adsorption experimental data were analyzed using three different isotherms, Langmuir, Temkin, and Freundlich models, to check the compatibility of adsorption isotherms and to predict the adsorption process. In this way, the adsorption process experimental data for the carbon active obtained from oil sludge one were correlated and the isotherm parameters were determined, which are listed in Table 4.
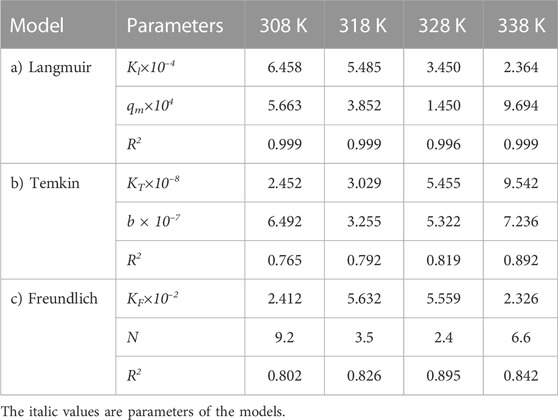
TABLE 4. Parameters of a) Langmuir, b) Temkin, and c) Freundlich isotherm models at different temperatures using Oil sludge 1 as carbon active at pH = 5 and adsorbent concentration of 100 mg/L.
The same adsorption process experimental data for the carbon active obtained from oil sludge 2, oil sludge 3, and oil sludge 4 were correlated and the isotherm parameters were reported in Tables 5–7, respectively.
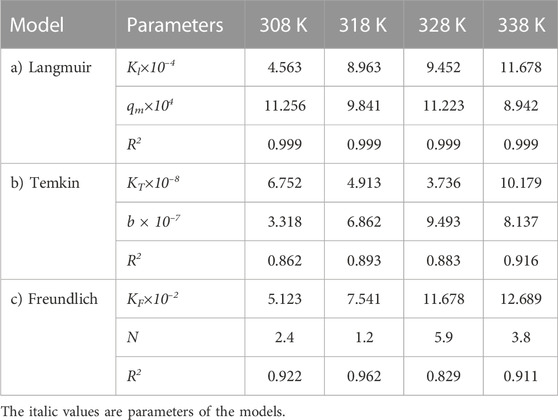
TABLE 5. Parameters of a) Langmuir, b) Temkin, and c) Freundlich isotherm models at different temperatures using Oil sludge 2 as caron active at pH = 5 and adsorbent concentration of 100 mg/L.
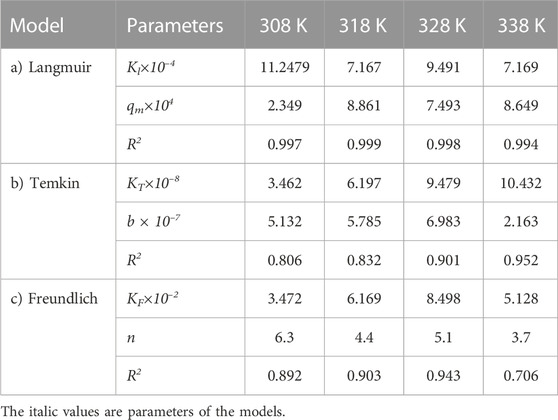
TABLE 6. Parameters of a) Langmuir, b) Temkin, and c) Freundlich isotherm models at different temperatures using Oil sludge 3 as carbon active at pH = 5 and adsorbent concentration of 100 mg/L.
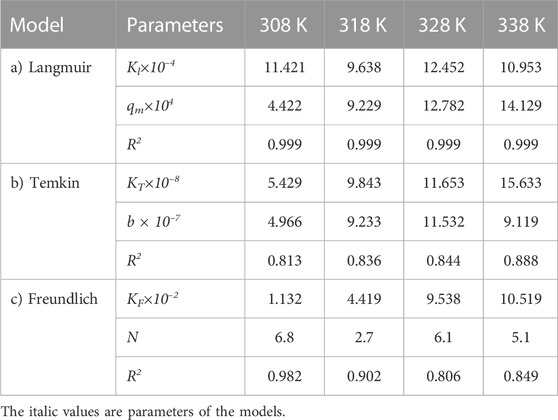
TABLE 7. Parameters of a) Langmuir, b) Temkin, and c) Freundlich isotherm models at different temperatures using Oil sludge 4 as carbon active at pH = 5 and adsorbent concentration of 100 mg/L.
The adsorption capacity of the synthesized activated carbon depends not only on its physical properties, such as distribution pore size and specific surface area, but also on the chemical composition (Bai et al., 2022). Therefore, manipulating the pH values plays an important role in the adsorption performance which could lead to the transformation of chemical characteristics in adsorption pilot system on the surface of activated carbon. In this work the adsorption tests were carried out in the initial pH range of 3–9. The effects of pH value on the oil adsorption of the oil contaminated wastewater on the different obtained carbon actives is shown in Figure 3. The results show that the decreasing the acidity increases the amount of adsorption (Qu et al., 2022). Moreover, the pH effects on the linearized Langmuir isotherm of the oily wastewater adsorption on different synthesized carbon actives are shown Figure 4.
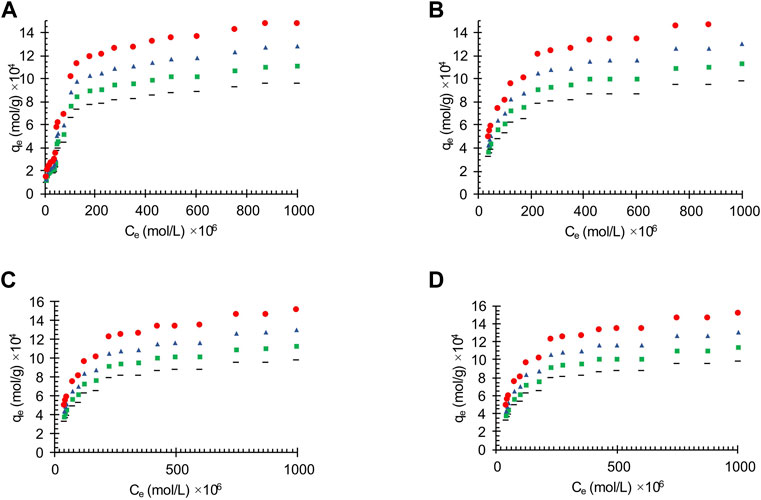
FIGURE 3. The effects of pH value on the oil adsorption of the wastewater on the different synthesized carbon actives obtained from (A) Oil sludge 1; (B) Oil sludge 2; (C) Oil sludge 3; (D) Oil sludge 4 at T = 318 K and adsorbent concentration of 100 mg/L.
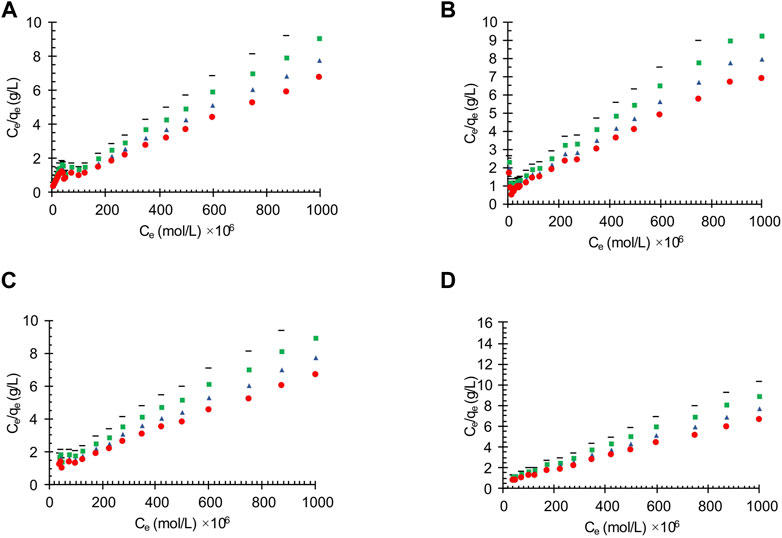
FIGURE 4. The effects of pH value on the oil adsorption of the wastewater applying linearized Langmuir isotherm on the different synthesized carbon actives obtained from (A) Oil sludge 1; (B) Oil sludge 2; (C) Oil sludge 3; (D) Oil sludge 4 at T = 318 K and adsorbent concentration of 100 mg/L.
The results showed that in acidic conditions, pH values below 6, hydrogen ions are likely to compete with species. As can be seen from Figure 3 the adsorption is highly pH dependent and similar results have been reported in previous studies (Yahya et al., 2015).
The experimental data of the adsorption process were analyzed by three different isotherms, Langmuir, Temkin, and Freundlich models, to check the compatibility of adsorption isotherms and predict the adsorption process at different pH values. In this way, the adsorption process experimental data for the carbon active obtained from the oil sludge one were correlated at different pH values and the isotherm parameters were determined which are listed in Table 8.
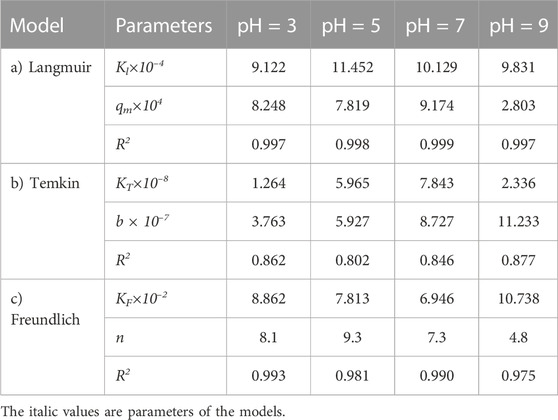
TABLE 8. Parameters of a) Langmuir, b) Temkin, and c) Freundlich isotherm models at different pH values using Oil sludge 1 as carbon active at T = 318 K and adsorbent concentration of 100 mg/L.
The same adsorption process experimental data at different pH values, for the carbon active obtained from oil sludge 2, oil sludge 3, and oil sludge 4 were correlated and the isotherm parameters were reported in Tables 9–11, respectively.
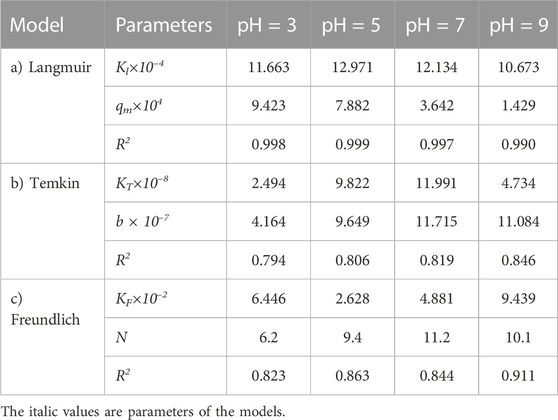
TABLE 9. Parameters of a) Langmuir, b) Temkin, and c) Freundlich isotherm models at different pH values using Oil sludge 2 as carbon active at T = 318 K and adsorbent concentration of 100 mg/L.
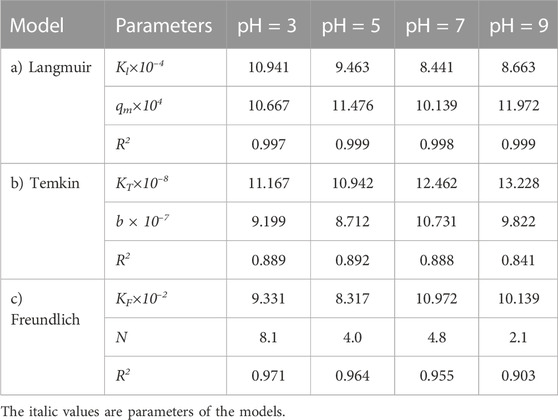
TABLE 10. Parameters of a) Langmuir, b) Temkin, and c) Freundlich isotherm models at different pH values using Oil sludge 3 as carbon active at T = 318 K and adsorbent concentration of 100 mg/L.
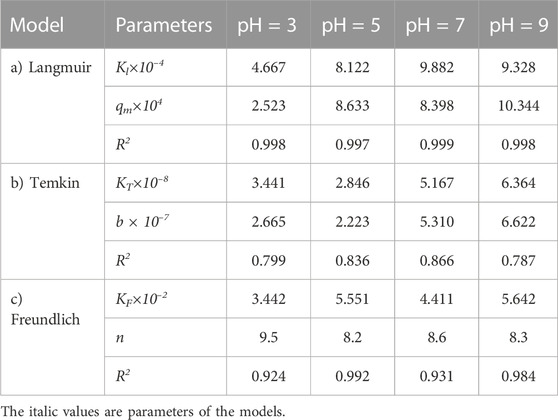
TABLE 11. Parameters of a) Langmuir, b) Temkin, and c) Freundlich isotherm models at different pH values using Oil sludge 4 as carbon active at T = 318 K and adsorbent concentration of 100 mg/L.
Figure 5 shows the effect of synthesized adsorbent amount on removal percentage. The result shows that increasing the amount of adsorbent increases the removal percentage. Based on the obtained results, it is possible to achieve 100% removal using a sufficient amount of adsorbent. Nevertheless, the very low amount of adsorbent in the solution has caused a very high adsorption of pollution.
The adsorption process experimental data at different adsorbent amount, for the synthesized carbon active from oil sludge1, oil sludge 2, oil sludge 3, and oil sludge 4 were correlated. The effect of adsorbent amount on the oil adsorption of the wastewater by using the obtained carbon actives is shown in Figure 6.
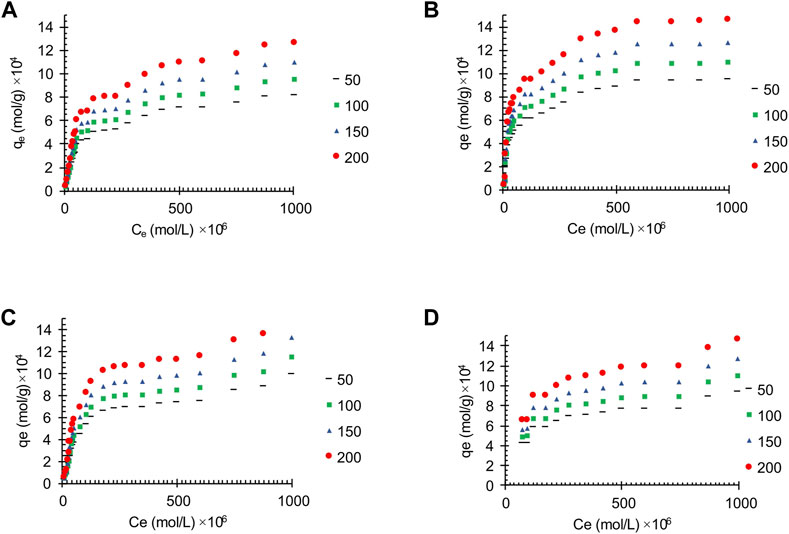
FIGURE 6. The effect of adsorbent amount on the oil adsorption of the synthesized wastewater on the activated carbon obtained from (A) Oil sludge 1; (B) Oil sludge 2; (C) Oil sludge 3; (D) Oil sludge 4 at T = 318 K and pH = 5.
Figure 7 shows the effect of adsorbent amount on the adsorption of the oily wastewater on the synthesized carbon actives on linearized Langmuir isotherm model. Furthermore, the correlated parameters of Langmuir, Temkin, and Freundlich isotherm models using different adsorbent amount for the synthesized carbon active by oil sludge 1, oil sludge 2, oil sludge 3, and oil sludge 4 are listed in Table 12, Table 13, Table 14, and Table 15, respectively.
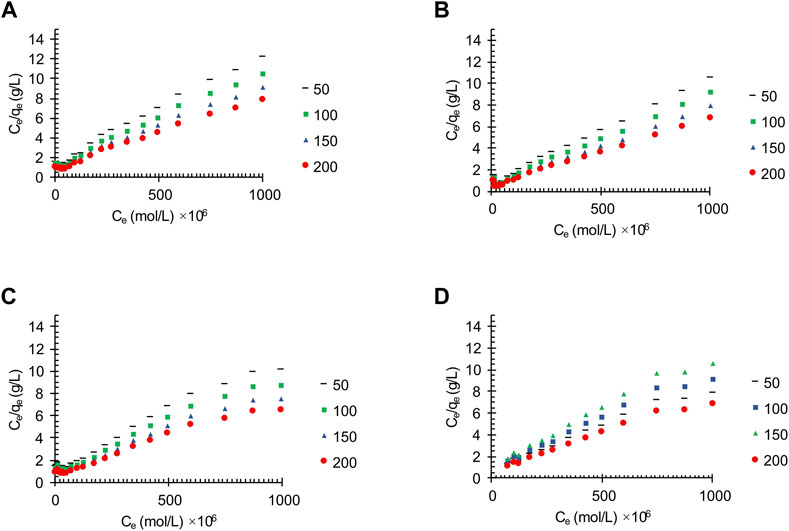
FIGURE 7. The effect of adsorbent amount on the oil adsorption of the synthesized wastewater applying linear Langmuir isotherm on the activated carbon obtained from (A) Oil sludge 1; (B) Oil sludge 2; (C) Oil sludge 3; (D) Oil sludge 4 at T = 318 K and pH = 5.
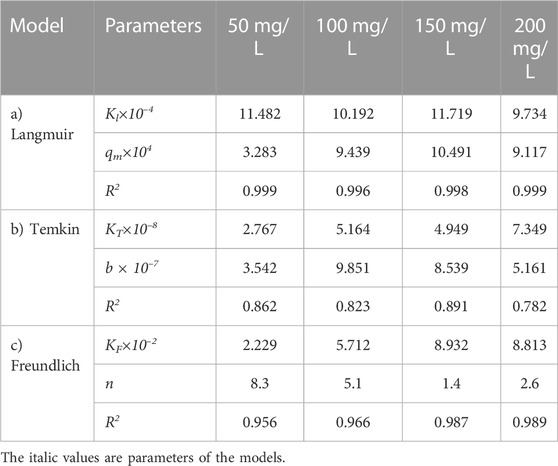
TABLE 12. Parameters of a) Langmuir, b) Temkin, and c) Freundlich isotherm models at different adsorbent amount using Oil sludge 1 as carbon active at T = 318 K and pH = 5.
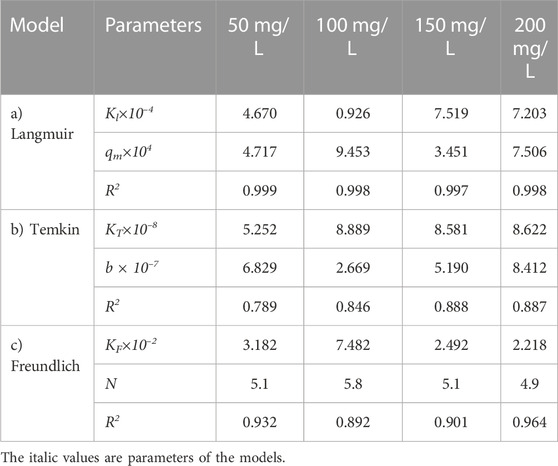
TABLE 13. Parameters of a) Langmuir, b) Temkin, and c) Freundlich isotherm models at different adsorbent amount using Oil sludge 2 as carbon active at T = 318 K and pH = 5.
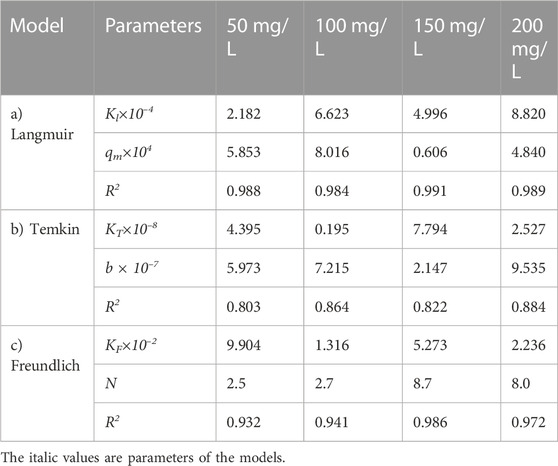
TABLE 14. Parameters of a) Langmuir, b) Temkin, and c) Freundlich isotherm models at different adsorbent amount using Oil sludge 3 as carbon active at T = 318 K and pH = 5.
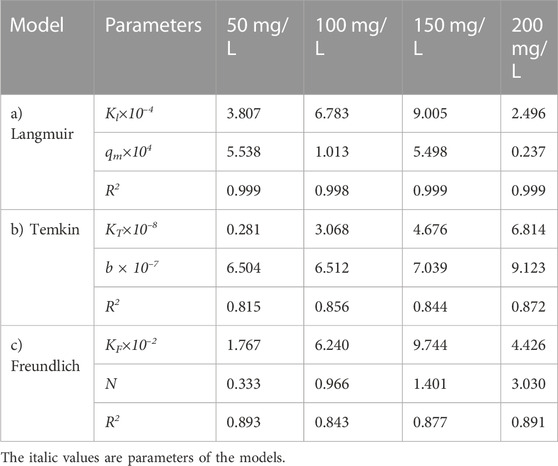
TABLE 15. Parameters of a) Langmuir, b) Temkin, and c) Freundlich isotherm models at different adsorbent amount using Oil sludge 4 as carbon active at T = 318 K and pH = 5.
The obtained results showed that the synthesized oil sludge based activated carbon was mainly microporous and mesoporous in character with specific surface area up to 120 and 23.2 m2/g, respectively; and the total pore volume and the micropore volume of 0.1 and 0.04 cm3/g, respectively. Over the range of initial concentration investigated, the efficiency of oil removal was above 98.5%, which is referred to the large adsorption capacity of the synthesized oil sludge based activated carbon.
4 Conclusion
Producing activated carbon from different oil sludges can not only significantly eliminate the need for further treatment of sludge, reduce the cost of hauling, landfilling and transporting the sludge, but can also produce a valuable adsorbent with lower cost than commercial activated carbons. For the surface adsorption process a wide range of studies has been carried out to describe the adsorption equilibrium data; however, no extensive investigation has been carried out to evaluate the oil sludge based activated carbon surface adsorption. Therefore, the possibility of carbon active production using different oil sludges and consequently the adsorption mechanism of these kind of adsorbents is still unknown. Moreover, no comprehensive study has been carried out in the field of comparing the obtained activated carbons from different oil sludge sources. Also, the effect of different parameters on the adsorption of the activated carbon from oil sludge has not been fully investigated. In this work in order to synthesize the activated carbon by using oil sludge, a novel two-step process was introduced to convert the hazardous oil sludge pollutant to a high-performance adsorbent. Within this research, two benefits can be achieved simultaneously; the first, is to remove the oil sludge that causes many environmental problems and turn it into a valuable adsorbent material, and the second is to use the produced activated carbon to purify the oily wastewater. In this way, four different types of oil sludges were characterized and the analysis showed that all of the oil sludge samples can be used as the adsorbent according to their carbon content. Moreover, the adsorption performance of the synthesized adsorbents was investigated in wastewater treatment application. The results showed that all synthesized activated carbons have the ability to remove the oily substances and purify the wastewater; which can be referred to their high adsorption capacity. Furthermore, the adsorption process was studied at different process conditions, e.g., temperature, pH value and adsorbent amount to find the optimum condition for oily wastewater treatment. In this way, four different types of oil sludges were characterized and then applied to synthesize different carbon actives and their performance were investigated as an adsorbent. The results showed that all synthesized activated carbons, with about 6% ash and pH = 7 and the specific surface area of 110 m2/gr, have the ability to treatment of oily wastewater; which can be referred to the high carbon content (>80%). The iodine number and the efficiency of prepared activated carbon were obtained as 406.8 mg/g and 94%, respectively. The adsorption process was also studied at different process conditions such as temperature (308–338 K), pH value (3–9) and adsorbent amount (50–200 mg/L) to find the optimum condition for wastewater treatment. The results show that the pH value has an optimum in the adsorption rate and the adsorption capacity can be reduced by increasing the temperature. Finally, three different adsorption isotherm models were applied, i.e., Langmuir, Temkin, and Freundlich isotherms, to correlate the adsorption process. Briefly, the highlights of this paper are introducing a novel low-cost two-step process to convert the hazardous oil sludges to a high-performance adsorbent; Studying the equilibrium adsorption experiments to analysis the wastewater treatment efficiency; Investigation of equilibrium adsorption isotherm models, i.e., Langmuir, Temkin, and Freundlich; and Comparison of different types of obtained activated carbons with different sources of oil sludge in the adsorption of oily wastewater; which were carried out for the first time.
Data availability statement
The raw data supporting the conclusion of this article will be made available by the authors, without undue reservation.
Author contributions
For Conceptualization, AA and HO; methodology, AA and HS; software, HO; validation, AA, NA-A, and HO; investigation, AA; visualization: SS and NA-A; resources, HO; data curation, AA; writing—original draft preparation, AN, AA, SS, NA-A, and HO; supervision, AA and NA-A; project administration, AA; All authors have read and agreed to the published version of the manuscript.
Acknowledgments
The authors extend their appreciation to the Deanship of Scientific Research at King Khalid University for funding this work through Research Groups Program under grant number RGP.2/182/43.
Conflict of interest
The authors declare that the research was conducted in the absence of any commercial or financial relationships that could be construed as a potential conflict of interest.
Publisher’s note
All claims expressed in this article are solely those of the authors and do not necessarily represent those of their affiliated organizations, or those of the publisher, the editors and the reviewers. Any product that may be evaluated in this article, or claim that may be made by its manufacturer, is not guaranteed or endorsed by the publisher.
References
Aron, N. S. M., Khoo, K. S., Chew, K. W., Veeramuthu, A., Chang, J.-S., and Show, P. L. (2021). Microalgae cultivation in wastewater and potential processing strategies using solvent and membrane separation technologies. J. Water Process Eng. 39, 101701. doi:10.1016/j.jwpe.2020.101701
Atallah, E., Kwapinski, W., Ahmad, M. N., Leahy, J. J., and Zeaiter, J. (2019). Effect of water-sludge ratio and reaction time on the hydrothermal carbonization of olive oil mill wastewater treatment: Hydrochar characterization. J. Water Process Eng. 31, 100813. doi:10.1016/j.jwpe.2019.100813
Awa, S. H., and Hadibarata, T. (2020). Removal of heavy metals in contaminated soil by phytoremediation mechanism: A review. Water Air Soil Pollut. 231, 47–15. doi:10.1007/s11270-020-4426-0
Bai, B., Bai, F., Li, X., Nie, Q., Jia, X., and Wu, H. (2022). The remediation efficiency of heavy metal pollutants in water by industrial red mud particle waste. Environ. Technol. Innov. 28, 102944. doi:10.1016/j.eti.2022.102944
Bai, B., Bai, F., Sun, C., Nie, Q., and Sun, S. (2023). Adsorption mechanism of shell powders on heavy metal ions Pb2+/Cd2+ and the purification efficiency for contaminated soils. Front. Earth Sci. (Lausanne) 10, 1071228. doi:10.3389/feart.2022.1071228
Bai, B., Rao, D., Chang, T., and Guo, Z. (2019). A nonlinear attachment-detachment model with adsorption hysteresis for suspension-colloidal transport in porous media. J. Hydrol. (Amst) 578, 124080. doi:10.1016/j.jhydrol.2019.124080
Birkland, T. A., Burby, R. J., Conrad, D., Cortner, H., and Michener, W. K. (2003). River ecology and flood hazard mitigation. Nat. Hazards Rev. 4, 46–54. doi:10.1061/(asce)1527-6988(2003)4:1(46)
Boustila, H., Boutillara, Y., Velasco, L. F., Djellali, A., and Tazibet, S. (2022). Tailoring activated carbon properties for Pb (II) and Cr (VI) removal from water in continuous mode. Chem. Eng. Technol. 45, 258–265. doi:10.1002/ceat.202100331
Cetin, M. (2020). Climate comfort depending on different altitudes and land use in the urban areas in Kahramanmaras City. Air Qual. Atmos. Health 13, 991–999. doi:10.1007/s11869-020-00858-y
Chatla, A., Almanassra, I. W., Jaber, L., Kochkodan, V., Laoui, T., Alawadhi, H., et al. (2022). Influence of calcination atmosphere on Fe doped activated carbon for the application of lead removal from water. Colloids Surf. A Physicochem Eng. Asp. 652, 129928. doi:10.1016/j.colsurfa.2022.129928
El-Gendy, N. S., and Nassar, H. N. (2021). Biosynthesized magnetite nanoparticles as an environmental opulence and sustainable wastewater treatment. Sci. Total Environ. 774, 145610. doi:10.1016/j.scitotenv.2021.145610
Eryılmaz, C., and Genc, A. (2021). Review of treatment technologies for the removal of phenol from wastewaters. J. Water Chem. Technol. 43, 145–154. doi:10.3103/s1063455x21020065
Gao, N., Li, J., Quan, C., and Tan, H. (2020). Product property and environmental risk assessment of heavy metals during pyrolysis of oily sludge with fly ash additive. Fuel 266, 117090. doi:10.1016/j.fuel.2020.117090
Ge, D., Yuan, H., Xiao, J., and Zhu, N. (2019). Insight into the enhanced sludge dewaterability by tannic acid conditioning and pH regulation. Sci. Total Environ. 679, 298–306. doi:10.1016/j.scitotenv.2019.05.060
Gong, Z., Liu, C., Wang, M., Wang, Z., and Li, X. (2020). Experimental study on catalytic pyrolysis of oil sludge under mild temperature. Sci. total Environ. 708, 135039. doi:10.1016/j.scitotenv.2019.135039
Guan, Q., Zeng, G., Song, J., Liu, C., Wang, Z., and Wu, S. (2021). Ultrasonic power combined with seed materials for recovery of phosphorus from swine wastewater via struvite crystallization process. J. Environ. Manage 293, 112961. doi:10.1016/j.jenvman.2021.112961
Hamta, A., Ashtiani, F. Z., Karimi, M., and Moayedfard, S. (2022). Asymmetric block copolymer membrane fabrication mechanism through self-assembly and non-solvent induced phase separation (SNIPS) process. Sci. Rep. 12, 771–810. doi:10.1038/s41598-021-04759-7
Hamta, A., Ashtiani, F. Z., Karimi, M., Sadeghi, Y., MoayedFard, S., and Ghorabi, S. (2021). Copolymer membrane fabrication for highly efficient oil-in-water emulsion separation. Chem. Eng. Technol. 44, 1321–1326. doi:10.1002/ceat.202000610
Hamta, A., and Dehghani, M. R. (2017). Application of polyethylene glycol based aqueous two-phase systems for extraction of heavy metals. J. Mol. Liq. 231, 20–24. doi:10.1016/j.molliq.2017.01.084
Hamta, A., Dehghani, M. R., and Gholami, M. (2017). Novel experimental data on aqueous two–phase system containing PEG–6000 and Na2CO3 at T=(293.15, 303.15 and 313.15) K. J. Mol. Liq. 241, 144–149. doi:10.1016/j.molliq.2017.05.149
Hamta, A., Mohammadi, A., Dehghani, M. R., and Feyzi, F. (2018). Liquid–liquid equilibrium and thermodynamic modeling of aqueous two-phase system containing polypropylene glycol and NaClO 4 at T=(288.15 and 298.15) K. J. Solut. Chem. 47, 1–25. doi:10.1007/s10953-017-0704-x
Hamta, A., Zokaee Ashtiani, F., Karimi, M., and Safikhani, A. (2020). Manipulating of polyacrylonitrile membrane porosity via SiO2 and TiO2 nanoparticles: Thermodynamic and experimental point of view. Polym. Adv. Technol. 32, 872–885. doi:10.1002/pat.5138
Hu, R., Liu, Y., Zhu, G., Chen, C., Hantoko, D., and Yan, M. (2022). COD removal of wastewater from hydrothermal carbonization of food waste: Using coagulation combined activated carbon adsorption. J. Water Process Eng. 45, 102462. doi:10.1016/j.jwpe.2021.102462
Huang, C. P. (1978). Chemical interactions between inorganics and activated carbon. Carbon Adsorpt. Handb. 8, 281–329.
Kurtan, Ü. (2021). Carbon nanofibers fabricated from electrospun nano-sized boron oxide/polyacrylonitrile nanofibers as electrode for supercapacitors. Sakarya Univ. J. Sci. 25, 1180–1188. doi:10.16984/saufenbilder.877089
Leng, L., Leng, S., Chen, J., Yuan, X., Li, J., Li, K., et al. (2018). The migration and transformation behavior of heavy metals during co-liquefaction of municipal sewage sludge and lignocellulosic biomass. Bioresour. Technol. 259, 156–163. doi:10.1016/j.biortech.2018.03.019
Li, T., Su, T., Wang, J., Zhu, S., Zhang, Y., Geng, Z., et al. (2023). Simultaneous removal of sulfate and nitrate from real high-salt flue gas wastewater concentrate via a waste heat crystallization route. J. Clean. Prod. 382, 135262. doi:10.1016/j.jclepro.2022.135262
Li, X., Han, D., Zhang, M., Li, B., Wang, Z., Gong, Z., et al. (2019). Removal of toxic dyes from aqueous solution using new activated carbon materials developed from oil sludge waste. Colloids Surf. A Physicochem Eng. Asp. 578, 123505. doi:10.1016/j.colsurfa.2019.05.066
Lin, X., Lu, K., Hardison, A. K., Liu, Z., Xu, X., Gao, D., et al. (2021). Membrane inlet mass spectrometry method (REOX/MIMS) to measure 15N-nitrate in isotope-enrichment experiments. Ecol. Indic. 126, 107639. doi:10.1016/j.ecolind.2021.107639
Liu, W., Zheng, J., Ou, X., Liu, X., Song, Y., Tian, C., et al. (2018). Effective extraction of Cr (VI) from hazardous gypsum sludge via controlling the phase transformation and chromium species. Environ. Sci. Technol. 52, 13336–13342. doi:10.1021/acs.est.8b02213
Liu, Y., Ran, C., Siyal, A. A., Song, Y., Jiang, Z., Dai, J., et al. (2020). Comparative study for fluidized bed pyrolysis of textile dyeing sludge and municipal sewage sludge. J. Hazard Mater 396, 122619. doi:10.1016/j.jhazmat.2020.122619
Mahzoonieh, S., Mirghaffari, N., and Soleimani, M. (2017). “Efficiency of activated carbon prepared from mixed oily sludge and lignocellulosic waste for furfural adsorption from aqueous solutions,” in 2017 International Conference on Environmental Impacts of the Oil and Gas Industries: Kurdistan Region of Iraq as a Case Study (EIOGI), Koya-Erbil, Iraq, 17-19 April 2017 (IEEE), 48–53.
Mariana, M., Hps, A. K., Mistar, E. M., Yahya, E. B., Alfatah, T., Danish, M., et al. (2021). Recent advances in activated carbon modification techniques for enhanced heavy metal adsorption. J. Water Process Eng. 43, 102221. doi:10.1016/j.jwpe.2021.102221
Misran, E., Bani, O., Situmeang, E. M., and Purba, A. S. (2022). Banana stem based activated carbon as a low-cost adsorbent for methylene blue removal: Isotherm, kinetics, and reusability. Alexandria Eng. J. 61, 1946–1955. doi:10.1016/j.aej.2021.07.022
Mojoudi, N., Mirghaffari, N., Soleimani, M., Shariatmadari, H., Belver, C., and Bedia, J. (2019). Phenol adsorption on high microporous activated carbons prepared from oily sludge: Equilibrium, kinetic and thermodynamic studies. Sci. Rep. 9, 19352–19412. doi:10.1038/s41598-019-55794-4
Munoz-Cupa, C., Hu, Y., Xu, C., and Bassi, A. (2021). An overview of microbial fuel cell usage in wastewater treatment, resource recovery and energy production. Sci. Total Environ. 754, 142429. doi:10.1016/j.scitotenv.2020.142429
Nie, F., Li, Y., Tong, K., Wu, B., Zhang, M., Ren, W., et al. (2020). Volatile evolution during thermal treatment of oily sludge from a petroleum refinery wastewater treatment plant: TGA-MS, py-GC (EGA)/MS and kinetics study. Fuel 278, 118332. doi:10.1016/j.fuel.2020.118332
Padaki, M., Surya Murali, R., Abdullah, M. S., Misdan, N., Moslehyani, A., Kassim, M. A., et al. (2015). Membrane technology enhancement in oil-water separation. A review. Desalination 357, 197–207. doi:10.1016/j.desal.2014.11.023
Pan, D., and Chen, H. (2021). Border pollution reduction in China: The role of livestock environmental regulations. China Econ. Rev. 69, 101681. doi:10.1016/j.chieco.2021.101681
Patnukao, P., and Pavasant, P. (2008). Activated carbon from Eucalyptus camaldulensis Dehn bark using phosphoric acid activation. Bioresour. Technol. 99, 8540–8543. doi:10.1016/j.biortech.2006.10.049
Qu, M., Liang, T., Hou, J., Liu, Z., Yang, E., and Liu, X. (2022). Laboratory study and field application of amphiphilic molybdenum disulfide nanosheets for enhanced oil recovery. J. Pet. Sci. Eng. 208, 109695. doi:10.1016/j.petrol.2021.109695
Saba, D. S. (2001). Afghanistan: Environmental degradation in a fragile ecological setting. Int. J. Sustain. Dev. World Ecol. 8, 279–289. doi:10.1080/13504500109470086
Shukla, J. (2021). “Carbon nanofiber to remove heavy metals from aqueous medium,” in Carbon nanofibers: Fundamentals and applications (Scrivener publishing: Wiley), 251–289. doi:10.1002/9781119769149
Solé-Bundó, M., Garfí, M., and Ferrer, I. (2020). Pretreatment and co-digestion of microalgae, sludge and fat oil and grease (FOG) from microalgae-based wastewater treatment plants. Bioresour. Technol. 298, 122563. doi:10.1016/j.biortech.2019.122563
Urionabarrenetxea, E., Garcia-Velasco, N., Anza, M., Artetxe, U., Lacalle, R., Garbisu, C., et al. (2021). Application of in situ bioremediation strategies in soils amended with sewage sludges. Sci. Total Environ. 766, 144099. doi:10.1016/j.scitotenv.2020.144099
Xu, D., Li, J., Liu, J., Qu, X., and Ma, H. (2022). Advances in continuous flow aerobic granular sludge: A review. Process Saf. Environ. Prot. 163, 27–35. doi:10.1016/j.psep.2022.05.018
Xue, Y., Wang, C., Hu, Z., Zhou, Y., Xiao, Y., and Wang, T. (2019). Pyrolysis of sewage sludge by electromagnetic induction: Biochar properties and application in adsorption removal of Pb (II), Cd (II) from aqueous solution. Waste Manag. 89, 48–56. doi:10.1016/j.wasman.2019.03.047
Yadav, B., Pandey, A., Kumar, L. R., and Tyagi, R. D. (2020). Bioconversion of waste (water)/residues to bioplastics-A circular bioeconomy approach. Bioresour. Technol. 298, 122584. doi:10.1016/j.biortech.2019.122584
Yahya, M. A., Zanariah, C. W., Ngah, C. W., Hashim, M. A., and Al-Qodah, Zjj. (2015). Preparation of activated carbon from desiccated coconut residue by chemical activation with NaOH. J. Mater. Sci. Res. 5, 24. doi:10.5539/jmsr.v5n1p24
Yang, Y., Fu, W., Chen, X., Chen, L., Hou, C., Tang, T., et al. (2022). Ceramic nanofiber membrane anchoring nanosized Mn2O3 catalytic ozonation of sulfamethoxazole in water. J. Hazard Mater 436, 129168. doi:10.1016/j.jhazmat.2022.129168
Zhang, L., Li, X., and Antonietti, M. (2021). General, metal-free synthesis of carbon nanofiber assemblies from plant oils. Angew. Chem. Int. Ed. 60, 24459–24467. doi:10.1002/ange.202110725
Zhang, Q., Yang, H., Guo, S., Sun, Q., Zhang, G., and Li, X. (2022). Removal of arsenic in the leachate by a porous carbonaceous solid waste generated from pyrolysis of oily sludge. Groundw. Sustain Dev. 18, 100799. doi:10.1016/j.gsd.2022.100799
Zhao, C., Zhou, J., Yan, Y., Yang, L., Xing, G., Li, H., et al. (2021). Application of coagulation/flocculation in oily wastewater treatment: A review. Sci. Total Environ. 765, 142795. doi:10.1016/j.scitotenv.2020.142795
Keywords: oily wastewater treatment, oil sludge, adsorption, activated carbon, thermodynamic investigation
Citation: Amari A, Noreen A, Osman H, Sammen SS, Al-Ansari N and Salman HM (2023) Investigation of the viable role of oil sludge-derived activated carbon for oily wastewater remediation. Front. Environ. Sci. 11:1138308. doi: 10.3389/fenvs.2023.1138308
Received: 05 January 2023; Accepted: 10 February 2023;
Published: 24 February 2023.
Edited by:
Muhammad Usman, Sultan Qaboos University, OmanReviewed by:
Lavania Baloo, University of Technology Petronas, MalaysiaZakaria Al-Qodah, Al-Balqa Applied University, Jordan
Copyright © 2023 Amari, Noreen, Osman, Sammen, Al-Ansari and Salman. This is an open-access article distributed under the terms of the Creative Commons Attribution License (CC BY). The use, distribution or reproduction in other forums is permitted, provided the original author(s) and the copyright owner(s) are credited and that the original publication in this journal is cited, in accordance with accepted academic practice. No use, distribution or reproduction is permitted which does not comply with these terms.
*Correspondence: Nadhir Al-Ansari, nadhir.alansari@ltu.se