- 1State Key Laboratory of Environmental Criteria and Risk Assessment, Chinese Research Academy of Environmental Sciences, Beijing, China
- 2College of Water Sciences, Beijing Normal University, Beijing, China
In order to realize the efficient removal of sulfamethazine (SMZ) and overcome the recycling difficulty of catalysts in a heterogeneous catalytic ozonation process, low-cost natural zeolite loaded with CeO2 and Fe3O4 (FC/HZ) was synthesized by the co-precipitation method. The FC/HZ catalyst could completely degrade SMZ and realize 39.6% removal of TOC through catalytic ozonation. Moreover, the FC/HZ catalyst exhibited high catalytic ozonation activity under a wide pH range and possessed excellent recyclability (>90%) and stability with a magnet. Based on experiment and material characterization, the possible catalytic mechanism and degradation pathway of SMZ was revealed. In brief, this study offered an effective strategy for preparing recyclable catalysts and eliminating SMZ in aqueous solution.
1 Introduction
Antibiotics are widely used in pharmaceutical and aquaculture industries for the treatment of various non-viral infections (Zhou et al., 2012; Wang et al., 2021; Wu et al., 2022). However, the abuse of antibiotics causes serious threats to ecological environment and human health, due to their persistence and drug resistance (Zhang et al., 2015; Zsirka et al., 2016; Jallouli et al., 2017; Wu et al., 2021). Sulfamethazine (SMZ) is a typical antibiotic, which has been widely used to treat Staphylococcus, lytic Streptococcus, and other infections. Recently, SMZ was detected in some kinds of water. Its threats cannot be ignored (Wehrhan et al., 2010; Xu et al., 2011; Cui et al., 2021; Fu et al., 2022). Owing to the complex intermediates and poor biodegradability of SMZ, it is difficult to effectively remove SMZ with traditional treatment technologies. The heterogeneous catalytic ozonation technology, a representative of advanced oxidation technology, can overcome the selectivity of ozone and improve the mineralization efficiency of organic pollutants, especially the removal of toxic and non-biodegradable substances (Baciogliu and Otker, 2003; Slomczynska et al., 2004; Andreozzi et al., 2005; Dantas et al., 2008). However, the complex preparation methods, high cost, and low recycling of O3 catalysts have restricted the development and application of the technology (Xie et al., 2020; Chen et al., 2021).
Zeolite is a porous aluminosilicate with a framework structure. It has excellent ion exchange, surface activity, adsorption, and chemical stability, which are widely used in the field of adsorption and catalysis (Cheng et al., 2000), wherein most research studies are based on the artificial zeolite due to its controllable material. However, the enormous production cost has become the obstacle for further application. Natural zeolite possesses extremely low production cost, in addition to the similar structure and performance with artificial zeolite (Lu et al., 2007; Sivaguru and Lalitha, 2014). It can promote the adsorption of organic pollutants and the exposure of active sites in the catalytic ozonation process. Moreover, high-purity natural zeolite can be developed into high-efficiency catalysts via appropriate modifications. Therefore, using natural zeolite is a potential and feasible synthesis strategy of O3 catalysts.
In addition, magnetic carrier technology has been applied to the separation and fixation of biological cells, wastewater treatment, and mineral processing (Takafuji et al., 2004; Galindo-González et al., 2005). It can improve the recycling of catalysts via loading the magnetic materials on natural zeolite. Researchers have prepared magnetic adsorbents with different characteristics by combining zeolite and magnetic iron oxide (Bourlinos et al., 2003). However, the prepared catalysts had limited ozone catalytic activity. Also, the complicated process of preparation increased the cost. According to previous studies, catalysts loaded with CeO2 (labeled as Ce/HZ) exhibited high catalytic ozonation activity (Zhang et al., 2020). Therefore, the combination of natural zeolite, magnetic materials, and CeO2 was likely to simultaneously solve the complexity of the complex preparation methods, high cost, and low recycling of O3 catalysts.
To address the problem that powder catalysts were difficult to recover and kept high catalytic ozonation activity, magnetic catalysts based on natural zeolite were prepared. The magnetic component, Fe3O4, and the active substance, CeO2, were loaded on the natural zeolite by the co-precipitation method. The morphology, specific surface area, and element valence changes of the prepared catalyst were systematically studied. The degradation performance of SMZ was evaluated in the catalytic ozonation process with prepared catalysts. The degradation mechanism and pathway of the target pollutant SMZ in the heterogeneous catalytic ozonation system were discussed.
2 Methods
2.1 Experimental material
Natural zeolite (clinoptilolite, 200 mesh), Ce(NO3)3•6H2O, Fe(NO3)3•9H2O, nitric acid (HNO3), and ammonia water were purchased from Sinopharm Group.
2.2 Catalyst preparation
The natural zeolite was added into HNO3 solution (1 mol/L) and stirred at room temperature for 4 h, and then the precipitate was washed with deionized water and dried in an oven at 60°C for 12 h. The prepared material was labeled as HZ. Then, the HZ was added to the Ce(NO3)3 solution (0.029 mol/L) and stirred at room temperature for 6 h. The pH was adjusted to 7–8 with NH3•H2O (2.0 mol/L). Then, the obtained cerium loading catalyst (Ce/HZ) was dried at 12°C for 12 h and calcined at 450°C for 4 h.
FeSO4•7H2O and Fe(NO3)3•9H2O with a molar ratio of 1:2 were added. Then, the mixed solution was stirred, and the NH3•H2O was added until the solution became black. The solution was aged for 2 h at 70°C. The obtained magnetic material (FC/HZ) was dried in an oven at 80°C for 4 h.
2.3 Characterization
The morphologies of the catalyst were analyzed using a cold-field emission scanning electron microscope (SEM, Jeol JSM-7500F). The chemical states of catalysts were obtained by X-ray photoelectron spectroscopy (XPS, AXIS Ultra DLD, Kratos Co., United Kingdom). The specific surface and pore distribution of the catalysts were measured by nitrogen adsorption–desorption on Brunauer–Emmett–Teller (BET, Micromeritics ASAP2460, United States). An X-ray diffractometer (XRD, PANalytical B.V., Holland) was used to analyze the composition of the catalysts.
2.4 Experimental method
All the ozonation experiments were conducted in a 1-L glass reactor. The concentrations of SMZ and catalysts were 50 mg/L and 2 g/L, respectively. The gas flow rate and concentration were 600 mL/min and 6 mg/L, respectively. In degradation experiments, the samples were withdrawn from the reactor at predetermined intervals and filtered through a 0.22-μm PTFE filter into a plastic bottle.
The SMZ concentration of the samples was determined by high-performance liquid chromatography (HPLC, Agilent 1260 Infinity, Agilent Technologies Co., Ltd.). Detection conditions: The chromatographic column was an Eclipse XDB-C18 column. The ratio of pure water and methanol was 50:50, with a liquid flow rate of 1.0 mL/min. Detection was performed at 275 nm. TOC of the samples were measured using a TOC analyzer (TOC-L-CPH-CN200, Shimadzu, Japan). An ultrahigh performance liquid chromatograph (Agilent, 1290 Infinity II) equipped with the Eclipse plus-C18 column and an AgilentG6545 Q-TOF mass spectrometer (UPLC-QTOF-MS/MS) were used to analyze the degradation intermediates of SMZ. The mobile phase A was the mixing solution of acetonitrile and water (V/V 55%/45%). The mobile phase B was the mixing solution of 0.1% formic acid and 5 μM ammonium formate aqueous solution. The injection volume was 20 μL, and the pump flow was 1.0 mL/min.
3 Results and discussion
3.1 Characterization of the catalysts
The morphology of the FC/HZ catalyst was shown in Figure 1A. The fine particles uniformly covered the surface of the modified zeolite, indicating the introduction of CeO2 and Fe3O4. The crystalline structures of FC/HZ, NZ, and HZ catalysts were analyzed by XRD. Figure1B shows that the diffraction peaks at 35.5°, 57.0°, 62.4°, and 64.8° of the FC/HZ catalyst were ascribed to (212), (232), (400), and (308) of Fe3O4 (PDF-# 97-009-2356), respectively. Moreover, the characteristic diffraction peaks of CeO2 could be observed in the XRD pattern of FC/HZ, according to the standard card of CeO2 (PDF-#44-1086). These results further confirmed that CeO2 and Fe3O4 were loaded, and the designed catalyst was successfully prepared by the method of ultrasonic impregnation and heat-treatment.
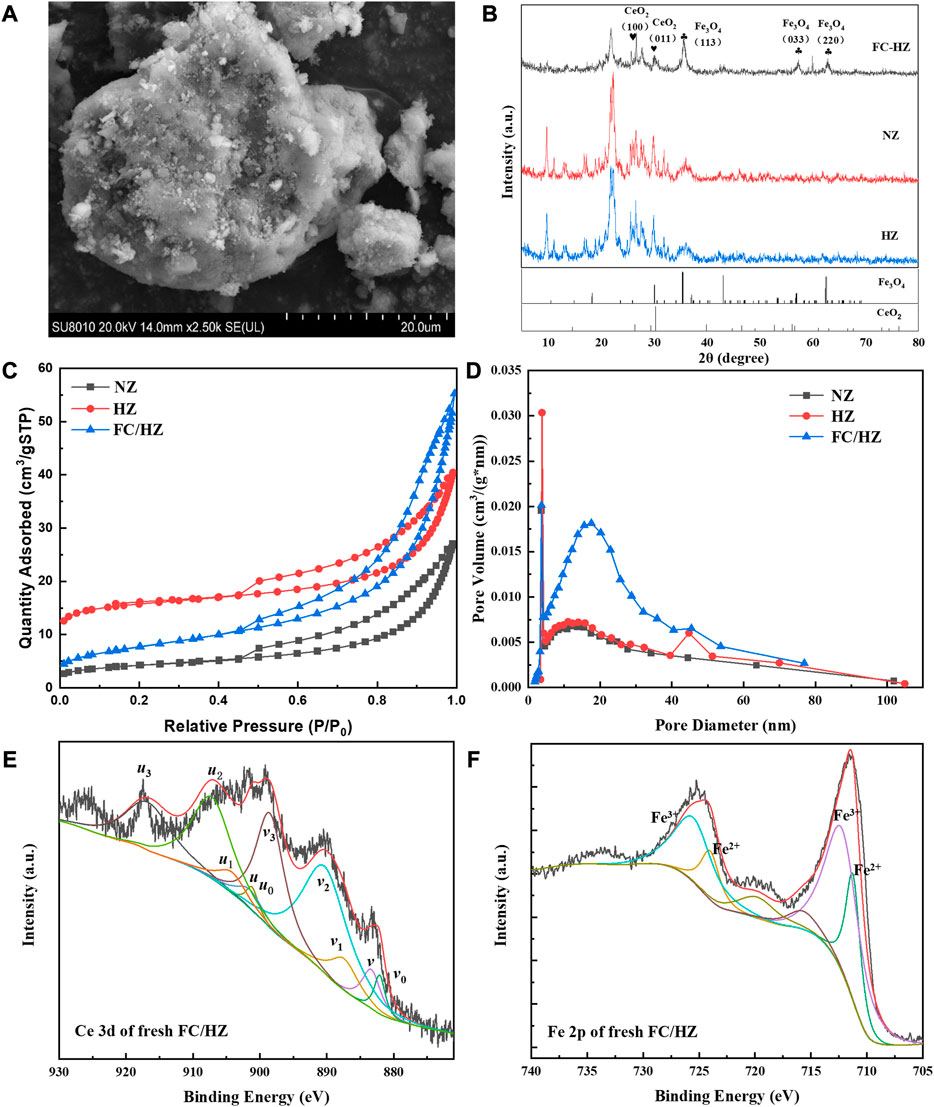
FIGURE 1. Morphologies, crystalline structures, pore size distribution, and chemical states of the FC/HZ catalyst. (A) SEM image. (B) XRD spectra. (C) and (D) show BET data. (E) and (F) show the spectrum of Ce 3d and Fe 2p.
The isotherm of the FC/HZ catalyst showed type IV characteristics with hysteresis loops of type H3 (Figure 1C). The type IV isotherm indicated the FC/HZ catalyst was mesoporous. The specific surface area of the FC/HZ catalyst was 27.78 m2/g. In comparison with HZ, the specific surface area decreased after loading CeO2 and Fe3O4. This might be attributed to CeO2 and Fe3O4 entering the slits and the pore structure of the zeolite. According to Figure 1D, the pore structure was a double-pore distribution, and the main structure was the mesoporous channel with the pore size ranging from 2.0 to 50.0 nm. The other was a microporous channel with the pore size ranging from 1.8 to 2.0 nm. Compared with NZ and HZ, the FC/HZ catalyst showed notable mesoporous distribution, indicating that the FC/HZ catalyst might be favorable to the adsorption of contaminants and catalysis of O3.
XPS was used to analyze the chemical states of the FC/HZ catalyst. Figure 1E was the spectrum of Ce 3d, which was composed of 10 peaks, and the peaks of 3d3/2 and 3d5/2 were represented by
3.2 Catalytic performance
The degradation efficiencies of SMZ were exhibited in Figure 2A. SMZ was hardly degraded during the FC/HZ catalysis alone, indicating negligible adsorption between the catalyst and SMZ due to the limited specific surface area of the FC/HZ catalyst. In comparison with ozonation alone, the degradation efficiency of SMZ improved from 65% to 90% within 15 min for the FC/HZ catalytic ozonation process. The FC/HZ catalyst exhibited better catalytic activity. Moreover, TOC result showed the similar removal order of SMZ for ozonation alone and the FC/HZ catalytic ozonation process (Figure 2B), further confirming the catalytic ozonation activity of the FC/HZ catalyst. These results indicated that the FC/HZ catalyst could effectively convert O3 to •OH and improve the mineralization removal efficiency of pollutants in water.
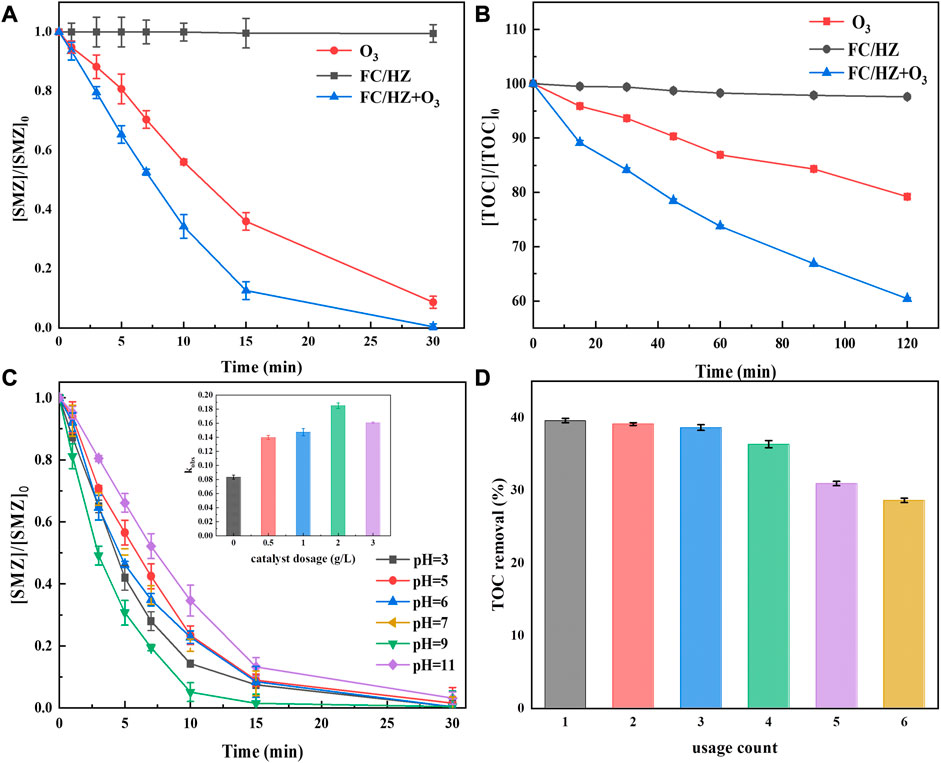
FIGURE 2. (A) and (B) show the degradation efficiencies of SMZ and removal efficiencies of TOC in various processes. (C) Degradation efficiencies of SMZ under various pH in the catalytic ozonation process with the FC/HZ catalyst (inset: the effect of catalyst dosage). (D) Stability and recyclability of the FC/HZ catalyst.
The degradation efficiencies of SMZ under different solution pH levels were evaluated in the FC/HZ catalytic ozonation process. Figure 2C showed that all the experimental groups achieved a removal rate of 97–99%, indicating that FC/HZ catalyzed O3 under a wide pH range. No discernible differences between pH 3 and pH 7 were found. The highest efficiency was achieved at pH 9, and the lowest was at pH 11. When pH reached 11, the degradation efficiency decreased significantly. It was deemed that O3 would react with OH− in solution. When pH reached 11, it might have caused a significant competition between the FC/HZ catalytic ozonation process and O3 reaction with OH−. This would cause the consumption of radicals which was used to generate •OH by chain reactions, further affecting the generation of •OH.
The effect of catalyst dosage was explored (Figure 2C inset). With the catalyst dosage from 0 to 2 g/L, the efficiency of SMZ removal increased significantly with the kobs from 0.083 to 0.185 min-1. It was mainly found that the raised catalyst dosage increased the active sites and generated more •OH. It improved the reaction efficiency of O3, SMZ, and the catalyst (Zhao et al., 2008). However, the degradation efficiencies decreased when catalyst dosage increased to 3 g/L. It might be attributed to the gas quickly turning into large bubbles after colliding with the catalyst, thus hindering the mass transfer of O3 from the gas phase to the liquid phase (Lin and Gurol, 1998).
The recyclability and stability of the FC/HZ catalyst was investigated by repeating SMZ degradation tests (Figure 2D). After degradation, the catalyst was separated by an external magnet and washed with deionized water and ethanol. The collected catalyst was reused in the second reaction under optimum reaction conditions. This same process was repeated six times. The TOC removal efficiencies during the four cyclic experiments did not decrease significantly, confirming the catalytic stability of the FC/HZ catalyst. However, the efficiencies gradually decreased in the fifth and sixth cycles. This might be attributed to the loss of catalysts during the separation process of the catalyst and the analyzed samples in every cyclic experiment. After six cyclic experiments, the recovery efficiency of the suspension FC/HZ by magnetic force was still higher than 90%, indicating the excellent recyclability of the FC/HZ catalyst.
3.3 Possible reaction mechanisms
Tert-butanol (TBA) is a strong •OH radical scavenger that has a reaction rate constant of
Figure 3A showed that the degradation efficiencies did not decrease with the addition of TBA, H2PO4−, and HCO3−. However, this phenomenon could not confirm the absence of •OH because SMZ was easily degraded by O3 alone. The increased degradation efficiencies might be attributed to the activation and improvement of the O3 oxidation path due to the inhibition of free-radical paths. In order to confirm the •OH, the TOC was detected because some intermediates were refractory substances of O3. Figure 3B shows that the removal of TOC was decreased evidently, indicating the generation of •OH in the FC/HZ catalytic ozonation process.
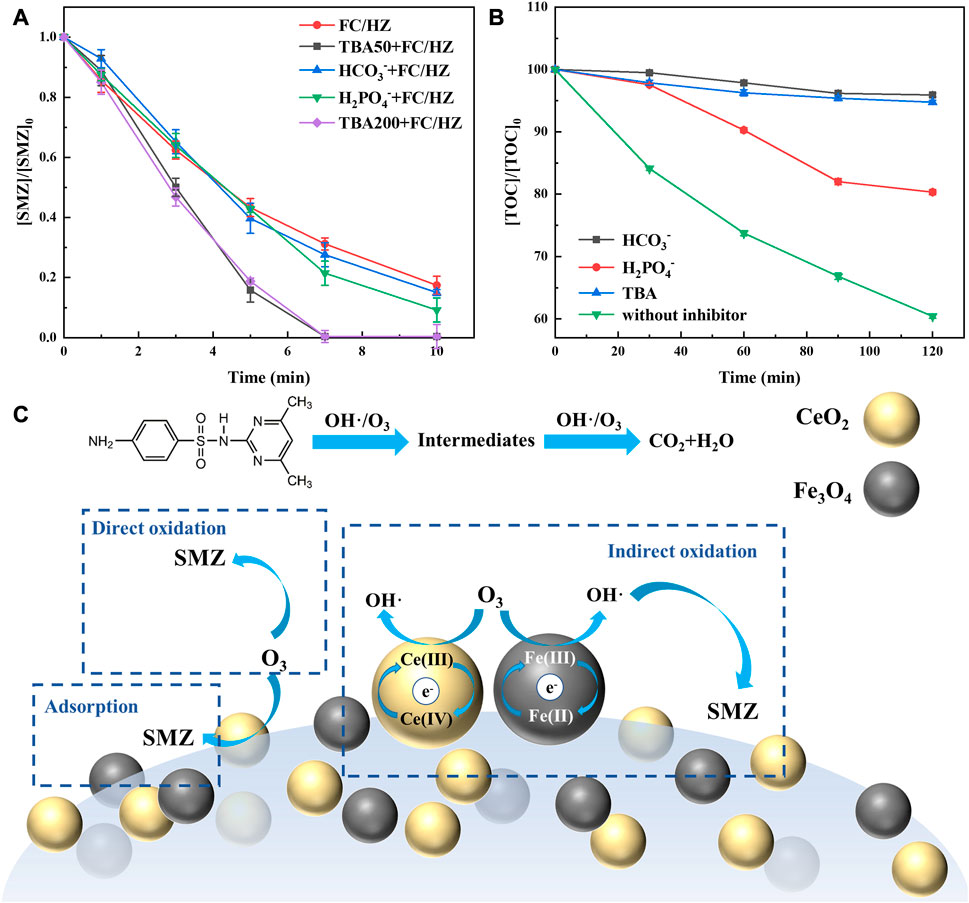
FIGURE 3. (A) and (B) show the effect of scavengers on SMZ degradation efficiency and TOC removal efficiency. (C) Proposed mechanism of the FC/HZ catalyst in the catalytic ozonation process.
Based on the abovementioned analysis and experimental results, a probable catalytic ozonation mechanism was put forward, which included the radical process and direct ozonation (Figure 3C).
(1) Radical process
CeO2 and Fe3O4 as the active sites catalyzed by O3 to generate •OH according to the valence state change of Ce3+/Ce4+ and Fe2+/Fe3+ by the electron transfer process, respectively. The generated •OH diffused into the bulk solution to attack organic pollutants. More O3 was adsorbed on the catalyst surface and converted into •OH. The entire process was continuously circulated, and the entire oxidative degradation process was finally completed.
(2) Direct ozonation
As we all know, ozone has a strong oxidizing property and its redox potential was 2.07 V. According to the control experiment, SMZ could be removed by O3 without a catalyst, indicating that O3 attacked the degradable substances including SMZ and some intermediates. Therefore, the direct ozonation process existed during the removal of SMZ.
3.4 Degradation pathway
The degradation products of SMZ were analyzed by HPLC-MS/MS. Based on the mass spectrum fragment information, the possible pathway of SMZ was shown in Figure 4.
In pathway Ⅰ, substitution reactions occurred after •OH attacked SMZ molecules. There were three ways to replace the hydroxyl group: replacing the hydrogen in the amino group to form a hydroxylamine product, replacing the hydrogen in the benzene ring, and replacing the hydrogen in the pyrimidine ring (Seddigi et al., 2014). The hydroxylated SMZ (P4–P6) was formed by the continuous attack of the active substance. P7 was the product in which the N atom in the hydroxylamine product was further oxidized for nitrosation. P5 and P6 lost the amino to form P8 and P9, respectively.
Pathway Ⅱ showed that P3 might be the product of the cleavage of the sulfonamide bonds [S–N] and [C–S] in SMZ. Several possible pathways for the formation of extrusion products with P3 have been proposed (Guo et al., 2013; Periša et al., 2013). Subsequently, •OH attacked the carbon–nitrogen bond between the benzene and pyrimidine ring in compound P3.
Pathway III was initiated by the breaking of the sulfonamide bond [S–N]. Under the action of ozone and hydroxyl radicals, the [S–N] bond could be broken directly to form P2.
4 Conclusion
In this study, CeO2 and Fe3O4 were loaded on the acid-washed natural zeolite by the co-precipitation method to form the FC/HZ catalyst. The FC/HZ catalyst could improve the degradation and mineralization rate of SMZ. CeO2 and Fe3O4 as the active sites contributed to the rapid generation of •OH in the catalytic ozonation process. The FC/HZ catalyst exhibited high catalytic ozonation activity under a wide pH range and possessed excellent recyclability and stability. This work proposed a feasible path to prepare efficient, stable, and recyclable catalysts based on high-purity natural zeolite and realized the efficient removal of antibiotic in aqueous solution. It provided guidance for the advanced treatment of antibiotic wastewater.
Data availability statement
The original contributions presented in the study are included in the article/Supplementary Material; further inquiries can be directed to the corresponding author.
Author contributions
ZG and YL contributed to the preparation of materials, evaluation of performance, and analysis of data. JZ contributed to the analysis of data. JW and YS contributed to the supervision and modification of the manuscript. All authors contributed to manuscript revision, read, and approved the submitted version.
Acknowledgments
The authors would like to acknowledge the financial support from the National Natural Science Foundation of China (NO. 22206177).
Conflict of interest
The authors declare that the research was conducted in the absence of any commercial or financial relationships that could be construed as a potential conflict of interest.
Publisher’s note
All claims expressed in this article are solely those of the authors and do not necessarily represent those of their affiliated organizations, or those of the publisher, the editors, and the reviewers. Any product that may be evaluated in this article, or claim that may be made by its manufacturer, is not guaranteed or endorsed by the publisher.
References
Andreozzi, R., Canterino, M., Marotta, R., and Paxeus, N. (2005). Antibiotic removal from wastewaters: The ozonation of amoxicillin. J. Hazard. Mater 122 (3), 243–250. doi:10.1016/j.jhazmat.2005.03.004
Baciogliu, I. A., and Otker, M. (2003). Treatment of pharmaceutical wastewater containing antibiotics by O3 and O3/H2O2 processes. Chemophere 50 (1), 85–95. doi:10.1016/s0045-6535(02)00534-9
Bing, J., Li, L., Lan, B., Liao, G., Zeng, J., Zhang, Q., et al. (2012). Synthesis of cerium-doped MCM-41 for ozonation of p-chlorobenzoic acid in aqueous solution. Appl. Catal. B 115-116, 16–24. doi:10.1016/j.apcatb.2011.12.017
Bourlinos, A. B., Zboril, R., and Petridis, D. (2003). A simple route towards magnetically modified zeolites. Microporous Mesoporous Mater 58 (2), 155–162. doi:10.1016/S1387-1811(02)00613-3
Chen, H., Zhang, Z., Hu, D., Chen, C., Zhang, Y., He, S., et al. (2021). Catalytic ozonation of norfloxacin using Co3O4/C composite derived from ZIF-67 as catalyst. Chemosphere 265, 129047. doi:10.1016/j.chemosphere.2020.129047
Cheng, X., He, Q., Guo, J., Yan, H., He, H., and Long, Y. c. (2000). Studies on the hydrothermal stability of natural and modified STI zeolite. Microporous Mesoporous Mater 149 (1), 10–15. doi:10.1016/j.micromeso.2011.09.009
Cui, H., Chang, H., Zheng, H., and Wan, Y. (2021). Determination and occurrence of sulfonamide transformation products in surface waters. Sci. Total Environ. 779, 146562. doi:10.1016/j.scitotenv.2021.146562
Dantas, R. F., Contreras, S., Sans, C., and Esplugas, S. (2008). Sulfamethoxazole abatement by means of ozonation. J. Hazard. Mater 150 (3), 790–794. doi:10.1016/j.jhazmat.2007.05.034
Fu, X., Lin, Y., Yang, C., Wu, S., Wang, Y., and Li, X. (2022). Peroxymonosulfate activation via CoP nanoparticles confined in nitrogen-doped porous carbon for enhanced degradation of sulfamethoxazole in wastewater with high salinity. J. Environ. Chem. Eng. 10 (3), 107734. doi:10.1016/j.jece.2022.107734
Galindo-González, C., Vicente, J. D., Ramos-Tejada, M. M., López-López, M. T., González-Caballero, F., and Duran, J. D. G. (2005). Preparation and sedimentation behavior in magnetic fields of magnetite-covered clay particles. Langmuir 21 (10), 4410–4419. doi:10.1021/la047393q
Guo, C., Xu, J., Wang, S., Zhang, Y., He, Y., and Li, X. (2013). Photodegradation of sulfamethazine in an aqueous solution by a bismuth molybdate photocatalyst. Catal. Sci. Technol. 3 (6), 1603–1611. doi:10.1039/C3CY20811G
Jallouli, N., Pastrana-Martínez, L. M., Ribeiro, A. R., Moreira, N. F., Faria, J. L., Hentati, O., et al. (2017). Heterogeneous photocatalytic degradation of ibuprofen in ultrapure water, municipal and pharmaceutical industry wastewaters using a TiO2/UV-LED system. Chem. Eng. J. 334, 976–984. doi:10.1016/j.cej.2017.10.045
Li, W., Lu, X., Xu, K., Qu, J., and Qiang, Z. (2015). Cerium incorporated MCM-48 (Ce-MCM-48) as a catalyst to inhibit bromate formation during ozonation of bromide-containing water: Efficacy and mechanism. Water Res. 86, 2–8. doi:10.1016/j.watres.2015.05.052
Lin, S. S., and Gurol, M. D. (1998). Catalytic decomposition of hydrogen peroxide on iron oxide: Kinetics, mechanism, and implications. Environ. Sci. Technol. 32 (10), 1417–1423. doi:10.1021/es970648k
Lu, A. H., Salabas, E. L., and Schüth, F. (2007). Magnetic nanoparticles: Synthesis, protection, functionalization, and application. Angew. Chem. Int. Ed. 46 (8), 1222–1244. doi:10.1002/anie.200602866
Periša, M., Babić, S., Škorić, I., Frömel, T., and Knepper, T. P. (2013). Photodegradation of sulfonamides and their N(4)-acetylated metabolites in water by simulated sunlight irradiation: Kinetics and identification of photoproducts. Environ. Sci. Pollut. Res. 20 (12), 8934–8946. doi:10.1007/s11356-013-1836-1
Qi, F., Chen, Z., Xu, B., Shen, J., Ma, J., Joll, C., et al. (2008). Influence of surface texture and acid–base properties on ozone decomposition catalyzed by aluminum (hydroxyl) oxides. Appl. Catal. B 84 (3-4), 684–690. doi:10.1016/j.apcatb.2008.05.027
Seddigi, Z. S., Bumajdad, A., Ansari, S. P., Ahmed, S. A., Danish, E. Y., Yarkandi, N. H., et al. (2014). Preparation and characterization of Pd doped ceria–ZnO nanocomposite catalyst for methyl tert-butyl ether (MTBE) photodegradation. J. Hazard. Mater 264, 71–78. doi:10.1016/j.jhazmat.2013.10.070
Sivaguru, P., and Lalitha, A. (2014). Ceric ammonium nitrate supported HY-Zeolite: An efficient catalyst for the synthesis of 1,8-dioxo-octahydroxanthenes. Chin. Chem. Lett. 25 (2), 321–323. doi:10.1016/j.cclet.2013.11.043
Slomczynska, B., Wasowski, J., and Slomczynski, T. (2004). Effect of advanced oxidation processes on the toxicity of municipal landfill leachates. Water Sci. Technol. 49 (4), 273–277. doi:10.2166/wst.2004.0282
Takafuji, M., Ide, S., Ihara, H., and Xu, Z. (2004). Preparation of Poly(1-vinylimidazole)-grafted magnetic nanoparticles and their application for removal of metal ions. Chem. Mater 16 (10), 1977–1983. doi:10.1021/cm030334y
Wang, K., Zhuang, T., Su, Z., Chi, M., and Wang, H. (2021). Antibiotic residues in wastewaters from sewage treatment plants and pharmaceutical industries: Occurrence, removal and environmental impacts. Sci. Total Environ. 788, 147811. doi:10.1016/j.scitotenv.2021.147811
Wehrhan, A., Streck, T., Groeneweg, J., Vereecken, H., and Kasteel, R. (2010). Long-term sorption and desorption of sulfadiazine in soil: Experiments and modeling. J. Environ. Qual. 39 (2), 654–666. doi:10.2134/jeq2009.0001
Wu, S., Shen, L., Lin, Y., Yin, K., and Yang, C. (2021). Sulfite-based advanced oxidation and reduction processes for water treatment. Chem. Eng. J. 414, 128872. doi:10.1016/j.cej.2021.128872
Wu, S., Yang, C., Lin, Y., and Cheng, J. (2022). Efficient degradation of tetracycline by singlet oxygen-dominated peroxymonosulfate activation with magnetic nitrogen-doped porous carbon. J. Environ. Sci. 115, 330–340. doi:10.1016/j.jes.2021.08.002
Xie, Y., Peng, S., Feng, Y., and Wu, D. (2020). Enhanced mineralization of oxalate by highly active and Stable Ce(III)-Doped g-C3N4 catalyzed ozonation. Chemosphere 239, 124612. doi:10.1016/j.chemosphere.2019.124612
Xu, B., Mao, D., Luo, Y., and Xu, L. (2011). Sulfamethoxazole biodegradation and biotransformation in the water–sediment system of a natural river. Bioresour. Technol. 102 (14), 7069–7076. doi:10.1016/j.biortech.2011.04.086
Zhang, J., Xiong, Z., Wei, J., Song, Y., Ren, Y., Xu, D., et al. (2020). Catalytic ozonation of penicillin G using cerium-loaded natural zeolite (CZ): Efficacy, mechanisms, pathways and toxicity assessment. Chem. Eng. J. 383, 123144. doi:10.1016/j.cej.2019.123144
Zhang, Q. Q., Ying, G. G., Pan, C. G., Liu, Y. S., and Zhao, J. L. (2015). Comprehensive evaluation of antibiotics emission and fate in the river basins of China: Source analysis, multimedia modeling, and linkage to bacterial resistance. Environ. Sci. Technol. 49 (11), 6772–6782. doi:10.1021/acs.est.5b00729
Zhao, L., Ma, J., Sun, Z., and Zhai, X. (2008). Catalytic ozonation for the degradation of nitrobenzene in aqueous solution by ceramic honeycomb-supported manganese. Appl. Catal. B 83 (3-4), 256–264. doi:10.1016/j.apcatb.2008.02.009
Zhou, L. J., Ying, G. G., Liu, S., Zhao, J. L., Chen, F., Zhang, R. Q., et al. (2012). Simultaneous determination of human and veterinary antibiotics in various environmental matrices by rapid resolution liquid chromatography–electrospray ionization tandem mass spectrometry. J. Chromatogr. A 1244, 123–138. doi:10.1016/j.chroma.2012.04.076
Keywords: catalytic ozonation, cerium oxides, natural zeolite, magnetic, sulfamethazine
Citation: Guo Z, Lv Y, Wei J, Zhang J and Song Y (2023) Efficient removal of sulfamethazine by a magnetic recoverable CeO2/Fe3O4/natural zeolite catalyst in catalytic ozonation process. Front. Environ. Sci. 11:1137137. doi: 10.3389/fenvs.2023.1137137
Received: 04 January 2023; Accepted: 24 February 2023;
Published: 10 March 2023.
Edited by:
Hang Yu, Dalian Maritime University, ChinaReviewed by:
Shaohua Wu, Guangdong University of Petrochemical Technology, ChinaJing Zou, Huaqiao University, China
Shan Qiu, Harbin Institute of Technology, China
Chunquan Li, China University of mining and Technology (Beijing), China
Copyright © 2023 Guo, Lv, Wei, Zhang and Song. This is an open-access article distributed under the terms of the Creative Commons Attribution License (CC BY). The use, distribution or reproduction in other forums is permitted, provided the original author(s) and the copyright owner(s) are credited and that the original publication in this journal is cited, in accordance with accepted academic practice. No use, distribution or reproduction is permitted which does not comply with these terms.
*Correspondence: Jian Wei, d2VpamlhbjA5MTFAMTYzLmNvbQ==