- 1Key Laboratory of Urban Security and Disaster Engineering, Ministry of Education, Beijing University of Technology, Beijing, China
- 2Faculty of Science, Department of Statistics and Date Science, Beijing University of Technology, Beijing, China
The physical and mechanical behavior of alpine meadow in Tibetan Plateau is now attracting widespread attention. In this paper, a series of experiments were conducted to study the influence of roots on hydrothermal behavior of alpine meadow located at Nangqian district, in the east of Tibetan Plateau, China. Root biomass, physical properties and hydrothermal properties were measured at a soil depth of 0–25 cm. The results showed that the root biomass within the depth of 0–15 cm accounts for 86% of the total root biomass. With the increase of soil depth, the root biomass shows a decreasing trend. The hydraulic conductivities and thermal conductivities of alpine meadow soil increase with the decrease of root biomass. There are lower hydraulic conductivity and thermal conductivity of swallow soil (0–5 cm) than that of deep layer soil. For the bottom soil layer, swallow alpine meadow acts as a “cap” and plays a function of ecological barrier of reducing water infiltration and heat transfer. Under the background of global warming, this insight is helpful to understand the relationship between soil hydrothermal properties and natural disasters in Tibetan Plateau.
1 Introduction
The Tibetan Plateau, due to high altitude and unique climate condition, has formed widespread alpine meadow covering an area of about 7.0 × 105 km2 (Chen et al., 2018). As an important bridge combined the external climate environment with internal soil environment, the water-heat exchange properties of alpine meadow soil are the key factors controlling water flow and heat transport in soil.
It is well known that the warming and humidifying of global climate has brought great influence to the Tibetan Plateau, such as melting of glaciers, intensifying of debris flow, landslide, collapse and other derivative disasters. Therefore, a further insight into hydrothermal properties of alpine meadow and the response of internal soil environment to climate change in Tibetan Plateau are needed.
The hydrothermal properties include hydraulic conductivity and thermal conductivity, which are the critical factors dominating the magnitude and distribution of soil temperature and moisture (Wang et al., 2012). For alpine meadow soil in Tibetan Plateau, plant root is the significant component that is different with conventional soil. A lot of studies about the influence of roots-induced alterations on soil hydrothermal properties have been reported in the previous literatures (Abu-hamdeh et al., 2000; Chen et al., 2018; He et al., 2018; Su et al., 2021; Doneva et al., 2022), yet conflicting results were found. For instance, within a certain roots content, the permeability coefficient of roots-soil mixture decreases with the increase of roots contents. Furthermore, the permeability coefficient is less than that of bare soil (Jiang et al., 2022). The dense roots in the alpine meadow soil have a strong blocking effect on the movement of soil water and reduce the infiltration performance of the soil (Wang et al., 2021). However, according to the study of Zhang, the permeability coefficients of the vegetated soil are two to three orders of magnitude higher than that of the bare soil with the same saturation within a range from 25% to 70% (Zhang et al., 2017). Therefore, some factors, i.e., grass/vegetation type, roots architecture, vegetation growth stage and soil type, have an important impact on hydraulic conductivity of alpine meadow soil (Jotisankasa et al., 2017; Wang et al., 2018; Li et al., 2021). As for the influence of roots on the thermal conductivity of alpine meadow soil, some qualitative conclusions have been obtained. The existence of roots in the alpine meadow can significantly reduce the thermal conductivity (Yang et al., 2005; Su et al., 2021). Based on the work of Fu et al. (2020) the roots-induced changes in soil thermal should not be ignored. However, fewer studies have investigated the quantitative understandings on hydrothermal behaviors change induced by roots of alpine meadow in Tibetan Plateau.
The aim of this research is to study the influence of roots on hydrothermal properties of alpine meadow in Tibetan Plateau. The detailed objectives were to 1) determine the changes of physical properties, hydraulic conductivity and thermal conductivity of alpine meadow soil with different root contents at different vertical depths; 2) analyze the relationship between root content and hydrothermal properties of alpine meadow qualitatively and quantitatively; 3) evaluate the ecological barrier function of alpine meadow on water-heat exchange in deep soil. This research will provide accurate parameters support for the hydrothermal process model of Tibetan Plateau under the condition of global warming and extreme climate.
2 Materials and methods
2.1 Study site description
This study was conducted in Nangqian district (31°32′–32°43′N, 95°21′N—97°07′E, 3,600–4,300 m above sea level), in the east of Tibetan Plateau, China (Figure 1). The climate of this study region belongs to continental monsoon climate, which is divided into cold and warm seasons. According to the meteorological monitoring data, the annual average precipitation increases with time ranging from 300 mm to 600 mm, meanwhile, the minimum temperature in freezing period is −25°C and the maximum temperature in melting period is 29°C in recent 60 years (Wei et al., 2015; Chen et al., 2018). In this region, alpine meadow is the most widespread soil type with abundant roots. The dominant vegetation is Kobresia humilis, Kobresia capillifolia, Kobresia tibetica Maxim, Kobresia kansuensis Kukenth, Carex scabrirostris and Polygonum viviparum.
2.2 Alpine meadow samples
Samples were collected at the actual site in September, 2021. A field site with uniform vegetation growth and no human or animal activities was selected as samples spot, in which three adjacent 50 cm × 50 cm quadrats were randomly chosen. Previous investigations showed that the roots of alpine meadow in Tibetan Plateau were distributed most densely in the top 0–10 cm soil (Yang et al., 2009; Song et al., 2018; Su et al., 2018), so in each quadrat, undisturbed soil samples were excavated and collected by cutting rings at each 5 cm within 0–25 cm soil depth after removing the surface vegetation of soil layer (Figure 2). Five samples were gathered at different depths along soil profile, 0–5 cm, 5–10 cm, 10–15 cm, 15–20 cm and 20–25 cm. For preventing water loss, all collected samples were wrapped with plastic film.
2.3 Measurement methods
2.3.1 Root biomass
In the laboratory, the samples collected in field site were placed in a nylon mesh bag with a 425 µm sieve as Figure 3A and washed for several times as Figure 3B. Next, the living roots were separated according to roots flexibility and cross section color (Figure 3C). Finally, the separated roots were dehydrated in an oven at 65°C for 72 h and weighed after cooling.
2.3.2 Physical properties
For alpine meadow soils, the measured physical properties main include particle size distribution, natural water content, liquid limit, plastic limit, bulk density and organic matter content. The physical property tests have been carried out according to the Standard for Soil Test Method (GB/T 50123-2019). Corresponding test methods (i.e., densitometer method, drying method, rolling strip method, fall cone method and loss-on-ignition method) were applied to measure particle size distribution, natural water content, bulk density, plastic limit, liquid limit and organic matter content, respectively.
2.3.3 Hydraulic conductivity
The hydraulic conductivities of alpine meadow soil were measured using a ST55-2 permeability device (Figure 4). The diameter and altitude of test sample is 61.8 mm × 40 mm. For the whole profile, there are five samples collected at different depth, 0–5 cm, 5–10 cm, 10–15 cm, 15–20 cm and 20–25 cm. In order to eliminate the test error as far as possible, three adjective samples at same depth were used to conduct permeability test.
2.3.4 Thermal conductivity
The thermal conductivity λ was measured by TC3100 thermal conductivity device (Figure 5) using the transient line heat source method. A sandwich structure included soil sample, sheet-shaped probe and soil sample can be observed in Figure 5B. When measuring, voltage was applied to the probe in the middle of sandwich structure which led to rise in temperature of probe and the surrounding soil sample, then the thermal conductivity can be obtained by measuring heat dissipation and temperature of the probe. For each soil depth, 0–5 cm, 5–10 cm, 10–15 cm, 15–20 cm and 20–25 cm, three adjective samples were used to conduct thermal conductivity test and the average value of three results was used as the value of thermal conductivity. To minimize the experimental error, the time interval of each group of tests is set as 15 min to keep the probe cool before test.
3 Results analysis and discussions
3.1 Measurement results
3.1.1 Root biomass
The results of root biomass at different soil depth and its proportion are shown in Figures 6A, B. It can be seen from Figure 6A that the root biomass decreases with the increase of soil depth. The total root biomass at soil depth 0–25 cm is 9116.4 g/m2. From the top to bottom along soil depth, the values of root biomass are 3663.4 g/m2, 2284.7 g/m2, 1928.6 g/m2, 702.1 g/m2 and 537.6 g/m2, respectively. Furthermore, the root biomass was mainly concentrated in shallow soil (0–15 cm) according to proportion result in Figure 6B, it accounts for 86.4% of the total root biomass. It is worth noting that the top 0–5 cm of soil with the highest root biomass accounts for 40.18% of the total root biomass.
3.1.2 Physical properties
The measured data of physical properties of alpine meadow soil are shown in Table 1; Figure 7. For the soil particle size distribution, there are no obvious differences among the soil depths 0–25 cm (Table 1), the five curves of soil samples with different depths are close to coincidence (Figure 7). Because all values of plastic index are between 11.6 and 12.7, soil type is silty clay. With the increase of soil depth, the values of bulk density (BD) of soil samples increases. From the top to bottom of soil layer, the values of BD vary from 0.78 g/cm3 to 1.48 g/cm3. Natural water content (NWC) and organic matter content (OMC) in each soil layer decreases with increasing soil depth. The highest values of NWC and OMC at top 0–5 cm of soil are 44.1% and 156 g/kg, respectively.
3.1.3 Hydraulic conductivity
The measured results of hydraulic conductivity on alpine meadow soils are given in Table 2. It can be observed that the hydraulic conductivity values increased with the increase of soil depth. From the top (0–5 cm) to the bottom (20–25 cm) of soil depth, the hydraulic conductivity values are 0.68 × 10−4 cm/s and 5.1 × 10−4 cm/s, respectively. Compared with 0–5 cm, the permeability of alpine meadow soils at 20–25 cm has increased 7.5 times.
3.1.4 Thermal conductivity
The measured results of thermal conductivity on alpine meadow soils are given in Table 3. It can be known that the thermal conductivity values increased with the increase of soil depth. From the top (0–5 cm) to the bottom (20–25 cm) of soil depth, the thermal conductivity values are 0.577 W/(mK) and 0.919 W/(mK), respectively. It is worth to note that there exists similar thermal conductivity at soil depth 5–25 cm, yet the thermal conductivity value of surface layer 0–5 cm is much smaller that of 5–25 cm, which maybe due to the high content of roots.
3.2 Analysis and discussion
3.2.1 Relationship between hydraulic conductivity and physical properties of alpine meadow
Based on the measured experimental data, the relationship between hydraulic conductivity and physical properties of alpine meadow are plotted in Figure 8. It can be seen from Figure 8A that hydraulic conductivity shown by the red line has an obvious negative correlation with root biomass, that means with the increase of soil depth, hydraulic conductivity increases and root biomass decreases. Especially at the position of soil layer surface (0–5 cm), the value of hydraulic conductivity is the minimum with a maximum root biomass, which shows that the surface of soil layer has low permeability due to the high root biomass. Compared with the hydraulic conductivity of soil depth 20–25 cm, the values at depth 0–5 cm is reduced by 87%. Hydraulic conductivity, organic matter content (OMC), bulk density (BD) and porosity are plotted on the graph in Figures 8B–D, which shows that hydraulic conductivity has an obvious negative correlation with OMC and porosity and has a positive correlation with BD. With the increase of soil depth, hydraulic conductivity and BD increase, yet OMC and porosity decrease. For the surface soil (0–5 cm), there exists lower permeability and BD, higher OMC and porosity, which relates to root biomass. Because high content of root biomass can lead to high content of OMC, furthermore, the roots and OMC will fill the pores of soil that causes seepage passage decrease, thus the permeability is lower than that of no root and OMC filled in pores. In general, the greater the porosity, the stronger the permeability and the greater the hydraulic conductivity, which is contrary to the experimental results obtained in this study. Here the negative correlation between hydraulic conductivity and porosity can be explained by the roots. Compared with traditional soil, plant roots filled in pores play two roles, absorbing water and retarding water movement, which can both decrease the water flux per unit time and section. Thus, the results in Figure 8D show that the influence of root system on permeability of alpine meadow soil is very significant and should be paid enough attention for stability evaluation of site.
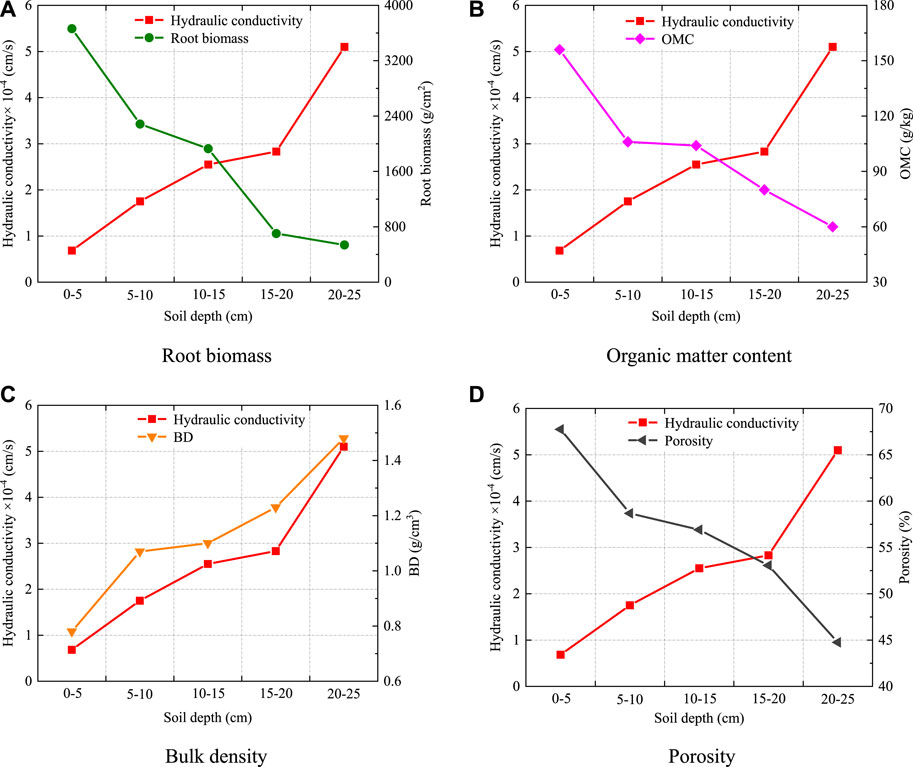
FIGURE 8. Relationship between hydraulic conductivity and (A) root biomass, (B) OMC, (C) BD, (D) porosity.
In addition, according to the research of scholars (Wu et al., 2014; Yang et al., 2016; Fan et al., 2019), the dense roots in the shallow soil of alpine meadow can absorb a lot of water, enhance the water holding capacity of soil and reduce the transportation of water to the lower soil. Meanwhile, combining with the effect of low permeability, it can be concluded that the shallow soil of alpine meadow may reduce the impact of external precipitation on the underlying deep soil and play an important role of protective layer.
3.2.2 Relationship between thermal conductivity and physical properties of alpine meadow
Based on the measured experimental data, the relationship between thermal conductivity and physical properties of alpine meadow are plotted in Figure 9. From the result of thermal conductivity shown by the blue line, it can be found that with the increase of soil depth, the thermal conductivities of alpine meadow gradually increase, and the increase process can be divided into two stages. 1) Rapid increase stage: when the soil depth increases from 0–5 cm to 5–10 cm, the thermal conductivity of alpine meadow soil increases rapidly from 0.577 W/(m∙K) to 0.824 W/(m∙K), and the increase amplitude reaches 42.7%. 2) Slow increase stage. While the soil depth increases from 5–10 cm to 20–25 cm, the thermal conductivity of alpine meadow soil increases slowly from 0.824 W/(m∙K) to 0.919 W/(m∙K). In this case, the increase amplitude is only 11.6%.
The thermal conductivity of alpine meadow is affected by several factors, such as bulk density, natural water content, organic matter content and root biomass. Compared with deep depth soil, high root biomass is the outstanding feature of shallow soil. It can be seen from Figures 9A, B that with the increase of soil depth, root biomass and OMC decrease, but thermal conductivities increase. The experimental results indicate the root biomass and organic matter play a role of weakening the thermal conductivity of soil, there are three main reasons for this. Firstly, in the shallow layer of soil, partial pores space among the soil particles are filled with roots system and organic matter. In essence, the thermal conductivities of plant root 0.4 W/(m∙K) and organic matter 0.25 W/(m∙K) are far less than that of mineral particles of soil, such as 7.7 W/(m∙K) for quartz and 2.5 W/(m∙K) for kaolinite. That means the higher the content of roots and organic matter, the lower the thermal conductivity of alpine meadow soil. Secondly, the presence of roots and organic matter in soil pores makes the number of contact points between soil particles decrease, and the heat transfer path between solid particles and solid particles reduce, then the thermal conductivity is decreased. Thirdly, the results in Figure 9C show that the bulk density increases with the increase of soil depth. An increase in bulk density means that the soil layer is denser. Meanwhile, the amount of mineral particles in soil per unit volume increase, the contact points among soil particles also increase, and there are more heat transfer path between solid particles and solid particles, which can inevitably lead to improve the thermal conductivity.
In the shallow of soil, especially 0–5 cm, the hydraulic conductivity is much smaller than that of 5–25 cm (Figure 8D). The lower permeability results in higher natural water content in the shallow of soil layer (Figure 9D). Because the thermal conductivity of water (0.59 W/(mK)) is far less than the thermal conductivity of clay minerals, the thermal conductivity of shallow soil is lower than that of deep layer soil.
Through comparing the thermal conductivities of shallow soil (0–5 cm) with that of deep soil (20–25 cm), it can be found that the thermal conductivity of shallow soil decreases by 59%. The results indicate that the more the content of plant roots in soil, the smaller the effect of external environmental temperature on bottom layer soil of alpine meadow soil. This study result proves that the swallow soil of alpine meadow acts as an ecological barrier for heat insulation. More precisely, the low hydraulic conductivity and thermal conductivity make the swallow alpine meadow acts as a “cap,” which can effectively reduce water infiltration and heat transfer and is very beneficial to the stability of the bottom soil layer.
4 Summary and conclusion
This study has conducted an insight into the influence of root on hydrothermal properties of alpine meadow in Tibetan Plateau and obtained the following significant results.
(1) The roots of alpine meadow soil are mainly distributed in the shallow soil, the root biomass within the depth of 0–15 cm accounts for 86% of the total root biomass. With the increase of soil depth, the root biomass shows a decreasing trend.
(2) Roots have a critical influence on permeability of alpine meadow soil. The hydraulic conductivities of alpine meadow soil increase with the decrease of root biomass. There is a lower hydraulic conductivity of swallow soil than that of deep layer soil.
(3) The thermal conductivities of alpine meadow soil increase with the increase of soil depth and decrease of root biomass. There is a lower thermal conductivity of swallow soil than that of deep layer soil. The whole change process of thermal conductivity can be divided into rapid increase stage and slow increase stage.
(4) For the bottom soil layer, swallow alpine meadow acts as a “cap” and plays a function of ecological barrier of reducing water infiltration and heat transfer. Under the background of global warming, this insight is helpful to understand the relationship between soil hydrothermal properties and natural disasters in Tibetan Plateau.
Data availability statement
The original contributions presented in the study are included in the article/Supplementary Material, further inquiries can be directed to the corresponding author.
Author contributions
MH: writing—data curation, methodology. ZZ: writing—original draft, editing. XW: corresponding author, writing—review and editing. All authors contributed to the article and approved the submitted version.
Funding
This study is financially supported by the Second Tibetan Plateau Scientific Expedition and Research (STEP) Program (No. 2019QZKK0905).
Conflict of interest
The authors declare that the research was conducted in the absence of any commercial or financial relationships that could be construed as a potential conflict of interest.
Publisher’s note
All claims expressed in this article are solely those of the authors and do not necessarily represent those of their affiliated organizations, or those of the publisher, the editors and the reviewers. Any product that may be evaluated in this article, or claim that may be made by its manufacturer, is not guaranteed or endorsed by the publisher.
References
Abu-hamdeh, N. H., and Reeder, R. C. (2000). Soil thermal conductivity effects of density, moisture, salt concentration, and organic matter. Soil Sci. Soc. Am. J. 64, 1285–1290. doi:10.2136/sssaj2000.6441285x
Chen, J. L., Li, J. H., Cheng, P., Song, L., and Zhou, T. (2018a). Field test on seepage performance of soil cover with different types of vegetation. Rock Soil Mech. 39, 222–228. doi:10.16285/j.rsm.2016.0093
Chen, N., Zhang, Y. J., Zhu, J. T., Li, J. X., Liu, Y. J., Zu, J. X., et al. (2018b). Nonlinear responses of productivity and diversity of alpine meadow communities to degradation. Chin. J. Plant Ecol. 42, 50–65. doi:10.17521/cjpe.2017.0252
Chen, X. Y. (2018c). Characteristics of climate change in Nangqian county in recent 60 years. Reg. Gov. 25, 268. doi:10.3969/j.issn.2096-4595.2018.25.244
Doneva, K., Kercheva, M., Dimitrov, E., Velizarova, E., and Glushkova, M. (2022). Thermal properties of cambisols in mountain regions under different vegetation covers. Soil Water Res. 17, 113–122. doi:10.17221/94/2021-SWR
Fan, Y. J., Hou, X. Y., Zhao, D. P., Shi, H. X., Zhou, H. K., and Li, X. (2019). Preliminary study on soil water-holding capacity along degradation gradients of alpine meadow. J. Southwest Minzu Univ. Nat. Sci. Ed. . 45, 10–15. doi:10.11920/xnmdzk.2019.01.002
Fu, Y. W., Lu, Y. L., Heitman, J., and Ren, T. S. (2020). Root-induced changes in soil thermal and dielectric properties should not be ignored. Geoderma 370, 1–10. doi:10.1016/j.geoderma.2020.114352
He, R. X., Jin, H. J., Zhao, S. P., and Deng, Y. S. (2018). Review of status and progress of the study in thermal conductivity of frozen soil. J. Glaciol. Geocryol. 40, 116–126. doi:10.7522/j.issn.1000-0240.2017.0314
Jiang, X. Y., Yang, S. Q., Feng, F., Chen, Y. H., and Xu, M. R. (2022). Experimental study on the influence of vegetation roots on soil permeability. J. Hefei Univ. Technol. Nat. Sci. . 45, 370–375. doi:10.3969/j.issn.1003-5060.2022.03.014
Jotisankasa, A., and Sirirattanachat, T. (2017). Effects of grass roots on soil-water retention curve and permeability function 1. Can. Geotech. J. 54, 1612–1622. doi:10.1139/cgj-2016-0281
Li, S. Y., Liu, K. N., Yu, H., Lin, Y. M., and Wang, D. J. (2021). The influence of soil physical properties on the infiltration of soil covered by different vegetation types in the Jiangjia Gully, Yunnan province, China. Mt. Res. 39, 867–878. doi:10.16089/j.cnki.1008-2786.000645
Song, J., Wan, S. Q., Peng, S. S., Piao, S. L., Ciais, P., Han, X. G., et al. (2018). The carbon sequestration potential of China’s grasslands. Ecosphere 9, 1–17. doi:10.1002/ecs2.2452
Su, P. X., Zhou, Z. J., Shi, R., and Xie, T. T. (2018). Variation in basic properties and carbon sequestration capacity of an alpine sod layer along moisture and elevation gradients. Acta Ecol. Sin. 38, 1040–1052. doi:10.5846/stxb201611222384
Su, Y. Q., Zhang, Y., Shang, L. Y., Wang, S. Y., Hu, G. J., Song, M. H., et al. (2021). Root-induced alterations in soil hydrothermal properties in alpine meadows of the Qinghai-Tibet Plateau. Rhizosphere 20, 1–11. doi:10.1016/j.rhisph.2021.100451
Wang, G. X., Liu, G. S., Li, C. J., and Yang, Y. (2012). The variability of soil thermal and hydrological dynamics with vegetation cover in a permafrost region. Agric. For. Meteorol. 162-163. doi:10.1016/j.agrformet.2012.04.006
Wang, X. H., Wang, Y. Q., Ma, C., and Wang, Y. J. (2018). Effect of root architecture on soil permeability. Sci. Soil Water Conserv. 16, 73–82. doi:10.16843/j.sswc.2018.04.010
Wang, Y. B., Lv, M. X., Zhao, H. P., and Gao, Z. Y. (2021). Analysis on soil water infiltration characteristics and mechanism in active layer in permafrost area of the Qinghai-Tibet Plateau. J. Glaciol. Geocryol. 43, 1301–1311. doi:10.7522/j.issn.1000-0240.2021.0084
Wei, Y. L., Han, F. X., and Observatory, Y. M. (2015). Climate change characteristics of nangqian county during 1961∼2013. Sci. Technol. Qinghai Agric. For., 39–44.
Wu, Q. H., Mao, S. J., Liu, X. Q., Li, H. Q., Zhang, F. W., and Li, Y. N. (2014). Analysis of the soil water-holding capacity in alpine forb meadow under grazing gradient and relevant influence factors. J. Glaciol. Geocryol. 36, 590–598. doi:10.7522/j.issn.1000-0240.2014.0070
Yang, K., Chen, Y. Y., and Qin, J. (2009). Some practical notes on the land surface modeling in the Tibetan Plateau. Hydrol. Earth Syst. Sci. 13, 687–701. doi:10.5194/hess-13-687-2009
Yang, K., Koike, T., Ye, B. S., and Bastidas, L. (2015). Inverse analysis of the role of soil vertical heterogeneity in controlling surface soil state and energy partition. J. Geophys. Res. Atmos. 110, 1–15. doi:10.1029/2004JD005500
Yang, S. W., Zhang, D. G., Niu, Y. J., Wang, G. Z., Liu, L., Chu, B., et al. (2016). Effect of short-term grazing on surface soil infiltration and soil water retention in alpine meadow. J. Soil Water Conserv. 30, 96–101. doi:10.13870/j.cnki.stbcxb.2016.04.017
Keywords: alpine meadow, hydraulic conductivity, thermal conductivity, root biomass, Tibetan Plateau (TP)
Citation: Hou M, Zhang Z and Wang X (2023) Insight into influence of roots on hydrothermal properties of alpine meadow in Tibetan Plateau. Front. Environ. Sci. 11:1132366. doi: 10.3389/fenvs.2023.1132366
Received: 27 December 2022; Accepted: 16 January 2023;
Published: 01 February 2023.
Edited by:
Jing Luo, Northwest Institute of Eco-Environment and Resources (CAS), ChinaReviewed by:
Weihua Zhang, Beijing City University, ChinaMin Chen, Cardiff University, United Kingdom
Copyright © 2023 Hou, Zhang and Wang. This is an open-access article distributed under the terms of the Creative Commons Attribution License (CC BY). The use, distribution or reproduction in other forums is permitted, provided the original author(s) and the copyright owner(s) are credited and that the original publication in this journal is cited, in accordance with accepted academic practice. No use, distribution or reproduction is permitted which does not comply with these terms.
*Correspondence: Xia Wang, d2FuZ3hpYUBianV0LmVkdS5jbg==