- 1Department of Chemistry, Sardar Bahadur Khan Women’s University, Quetta, Pakistan
- 2Department of Environmental Sciences, Sardar Bahadur Khan Women’s University, Quetta, Pakistan
- 3Department of Botany, Sardar Bahadur Khan Women’s University, Quetta, Pakistan
- 4Department of Environmental Science, Faculty of Life Sciences and Informatics, Balochistan University of Information, Technology, Engineering, and Management Sciences, Quetta, Pakistan
- 5Department of Veterinary Sciences, National Institute of Health Islamabad, Quetta, Pakistan
- 6Department of Chemistry, Division of Science and Technology, University of Education, Lahore, Pakistan
- 7Department of Pharmacognosy, College of Pharmacy, King Saud University, Riyadh, Saudi Arabia
- 8Department of Chemistry, School of Computer, Mathematical, and Natural Sciences, Morgan State University, Baltimore, MD, United States
Heavy metals create serious health problems, so the practical implementation and development of low-cost sorbent materials to remove heavy metals from the ecosystem is a worldwide issue. The purpose of this study is to find a low-cost ligand that has the potential to adsorb heavy metals from aqueous and soil samples and also has biological potential. For this, a Schiff base, dimeric o-toluidine (SBL), has been synthesized through condensation, characterized by spectroscopic analysis, and had its biological activities measured. We also studied its adsorption efficiency through a batch technique to remove Zn(II), Co(II), and Cu(II) from aqueous and soil samples under different conditions such as metal ion concentration, pH, contact time, and SBL concentration. The adsorption potential of SBL was analyzed by the Langmuir and Freundlich adsorption isotherms. The values of correlation coefficients revealed that the Freundlich isotherm elucidated results that were more appropriable than the Langmuir model. Adsorption equilibrium was established in 90 min for aqueous samples and in 1,440 min for soil samples. For the maximum adsorption of all metals, the optimum pH was 8, and it showed a capacity to remove 77 to 95 percent of metals from the samples. The maximum adsorption capacity (qmax) of SBL were 75.75, 62.50, and 9.17 mg g-1 in the case of Cu(II), Zn(II), and Co(II) ions, respectively, from aqueous samples and 10.95, 64.10, and 88.49 mg g-1 in the case of Zn(II), Cu (II), and Co(II), respectively, from soil samples. The effectiveness of SBL in the sorption of the selected metals was found to be Cu+2 > Zn+2 > Co+2 for aqueous samples and Co+2 > Cu+2 > Zn+2 for soil samples. The antimicrobial activity of SBL was also investigated. The results revealed that SBL showed moderate inhibitory activity against Staphylococcus dysentria, C. albican, and Aspergillus niger, whereas it exhibited weak activity against S. aureus, P. aureginosa, K. pneumoniae, P. vulgaris, and E.coli when compared to Fluconazole and Ciprofloxacin as the standard. Acute toxicity of the synthesized compound was measured through its daily oral administration with various doses ranging from 0.1 to 1,000 mg/kg of the mice’s body weights. Even at the dose of 1,000 mg/kg, the SBL showed no mortality or any type of general behavioral change in the treated mice. Based on preparation cost, metal removal capacity, toxicity, and antimicrobial activities, SBL is an excellent sorbent and should be studied at pilot scale levels.
1 Introduction
Heavy metals cause toxic effects on human health and the environment. They are among the most common environmental pollutants and release in the environmental segments from both sources (natural and anthropogenic). Heavy metals are accumulating, depositing, and distributing in natural ecosystems, such as water and soil, at a very high rate and affecting organisms of all kinds (Gong et al., 2009; Kavamura and Esposito, 2010; Udeigwe et al., 2011; Xu et al., 2012; Hu et al., 2013; Zeng et al., 2013a; Zeng et al., 2013b; Tang et al., 2014; Chen et al., 2015; Islam et al., 2015; Das et al., 2022). Therefore, the extraction of heavy metals from water and soil is a matter of great importance (Bhargava and Uma, 2018; Zhang et al., 2020). There are a wide range of conventional and modern methods, such as adsorption (Sun et al., 2012; Sun et al. 2013; Sun et al. 2014a; Sun et al. 2015; Zou et al., 2015; Sun et al., 2016a; Sun et al., 2016b), chemical precipitation (Matlock et al., 2002; Meunier et al., 2006; Roman-Ross et al., 2006), membrane (Gao et al., 2014), solvent extraction (Li et al., 2012), electrochemical treatment (Meunier et al., 2006), electro dialysis (Meunier et al., 2006), ion-exchange (Oehmen et al., 2006; Azarudeen et al., 2013), reverse osmosis (Akin et al., 2011), ultra-filtration (Zondervan and Roffel, 2007), oxidation/reduction (Ramos et al., 2009; Sun et al., 2014b; Ding et al., 2015), and evaporative recovery (Bouhamed et al., 2012) that have been used to remove toxic heavy metals (Gusain et al., 2019; Naeimi et al., 2022). From all of the known treatment techniques, adsorption is a widely employed method because it offers flexibility in design and operation and usually produces high-quality effluent after treatment (Hu et al., 2015; Ihsanullah et al., 2016). The other reasons for using adsorption methods are: adsorption is usually a reversible process and adsorbent materials can be regenerated using a suitable desorption method. Therefore, it is a more economical process (Fu and Wang, 2011). Adsorption is basically a transfer process in which a material is moved from the liquid to the solid phase by physical and chemical interactions (Babel and Kurniawan, 2003). As low-cost adsorbents that develop a more economical and efficient treatment method (Gupta, Khan, and Singh, 2021), Schiff bases are great adsorbents for the extraction of heavy metal ions due to their excellent ligand nature and capacity of forming complexes (Tighadouini et al., 2021; Cyril et al., 2022). To remove the dissolved heavy metal ions, a batch technique was used under various conditions, such as contact time, pH, and temperature (Shahmohammadi-Kalalagh, 2011; Hassan et al., 2013; Alluhaybi et al., 2022).
Here, we report the synthesis of a Schiff base (SBL) using low-cost starting materials, characterization, and its potential to remove Co(II), Cu(II), and Zn(II) metals from aqueous and soil samples under the studied conditions. We also report on the antimicrobial activities of SBL against two fungi, namely, C. albican and A. niger, and six bacteria, namely, S. dysentria, P. aureginosa, S. aureus, K. pneumoniae, E.coli, and P. vulgaris, as well as on the in-vivo acute toxicity on mice.
2 Materials and methods
The dimeric o-toluidine and 3-nitrobenzaldehyde were purchased from Daejung, Republic of Korea, and acetic acid and ethanol were purchased from Merck. Thin layer chromatography (TLC) was performed on a silica gel-coated aluminum sheet and purchased from E. Merck, Germany. TLC was observed under a UV lamp at 254 and 366 nm. The FTIR spectrum was obtained using a Jasco-320-A spectrometer by KBr pellets. 1H NMR spectrum was recorded on a Bruker-AV-400 spectrometer and reported in ppm (δ). The FAB-MS spectrum was obtained using a JEOL-600H-2 spectrometer, in m/z (%).
2.1 Synthesis of Schiff base
3-Nitrobenzaldehyde (141.8 mg, 0.97 mmol) and o-toluidine (100 mg, 0.47 mmol) were dissolved in ethanol followed by the addition of a few drops of acetic acid as a catalyst. The mixture was stirred for an hour and then refluxed for 2 h. Subsequently, the reaction mixture was cooled to 4°C to obtain the precipitated product. The product was filtered off, washed with ethanol, dried in a vacuum oven at 40°C, and then recrystallized with ethanol. The purity was assessed by thin-layer chromatography Scheme 1.
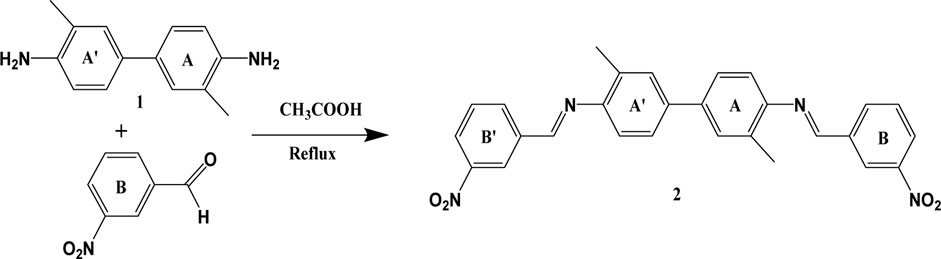
SCHEME 1. Synthesis of 1E,1′E)-N,N'-(3,3′-dimethyl-[1,1′-biphenyl]-4,4′-diyl) bis (1-(3-nitrophenyl) methanimine.
2.2 Adsorption behavior of metal ions on Schiff base
2.2.1 Aqueous medium
The adsorption potential of the Schiff base (SBL) for Cu, Co, and Zn metals in an aqueous medium was measured using various pH values at room temperature to check the effect of pH on adsorption. For this purpose, metal ion solutions of 100 mg/L (10 mL) were agitated with 0.1 g of dry Schiff base for 1 hour at 150 rpm. Solutions of different pH ranges of 2–12 were adjusted with the help of buffers. Similarly, the contact time required for metal uptake from the solution was measured by agitating 100 mg/L of metal ions solutions (10 mL) with 0.1 g of SBL in a series of stoppered flasks at pH 8, room temperature, and at 150 rpm via mechanical shaker. For this, various exposure times were studied, including 5, 15, 30, 60, 120, 300, and 1,440 min. The metal ions uptake capacity of SBL was assessed using 0.1 g of SBL in a series of flasks containing different concentrations of metal cations from 10 to 250 mg/L at pH 8. Under the same conditions (agitation speed and time) the effect of SBL dosage (0.1, 0.25, 0.5, 1, 1.25, 1.5, and 2 g) on metal ions removal from the solution was also studied. After a specific time of exposure and agitation, the solutions were filtered off and digests were prepared to calculate the metal ions concentration.
2.2.2 Soil
The soil used in this study was collected from 0–1.5 m beneath the ground surface of the Dasht area of the province of Balochistan, Pakistan. Three soil samples (each weighing 0.5 kg) were separately spiked with the salt solutions of CuCl2, ZnCl2, and CoCl2. To prepare synthetic contaminated soil in the laboratory with good homogeneity having a consistent concentration of heavy metals, the soil was equilibrated for 2 weeks followed by 2 months of drying in an oven. The homogenized soil samples were stored in a refrigerator for experimental studies. The synthetic polluted soil was digested with a 3:1 ratio of HCl and HNO3 before and after remediation experiments for the detection of the total concentration of metal ions using Atomic Absorption Spectrometry (AAA-1400 Thermo). Batch experimental procedures were adopted for the heavy metals removal from polluted soil by keeping at the required exposure time and metal ion concentration at constant pH and temperature. In the batch experiments, 25 mL of suspensions were prepared in water using 1 g of air-dried contaminated soil and 0.1 g of SBL and agitated at 25°C ± 2°C and 200 ± 5 rpm on a rotary shaker to prevent the occurrence of any sedimentation.
The % removal in both cases (aqueous and soil) was calculated as follows:
Where Co and Ce are the initial and final metal ion concentrations at equilibrium, respectively.
2.3 Antimicrobial activities
To assess the antimicrobial potential of SBL, the well diffusion method was adopted with some modifications (Egorov, 1965). The spore suspension of the test strains, Escherichia coli, Proteus vulgarus, Klebsiella pneumonia, Pseudomonas aeruginosa, Shigella dysenteriae five Gram-negative bacteria, S. aureus, a Gram-positive bacteria, and A. niger and Candida albicans, two yeasts, were prepared in 9.0 mL of 0.9% of a sterile saline solution. For bacterial growth, a solid nutrient agar (beef extract 3.0, peptone 10.0, and agar-agar 20.0) and, for yeast, Sabouraud dextrose agar (peptone 10.0, dextrose 40.0, agar-agar 15.0), were sterilized and divided while warm (45:40°C) in 15 mL portions among sterile Petri dishes with diameters of 9.0 cm. A spore suspension of 1 mL was placed on the surface of the solid media in the Petri dish and spread all over the surface. Then each dish was loaded with 20 µL from each concentration of SBL per 5 mm hole. The Petri dishes were incubated at 5°C for 2 h to permit good diffusion and then transferred to an incubator at 37°C for tested bacteria and at 30 °C for 72 h for tested yeast.
2.4 Toxicity test
The acute toxicity was performed by following the test guidelines of OECD 423, an international organization that develops better policies and protocols for animal studies (OECD, 2002). Toxicity studies were carried out at the National Institute of Health (NIH, Islamabad, Pakistan). The experimental protocol and procedures used in this study were approved by the IBC (Institutional Bioethical Committee), SBKWU, Quetta. There were 24 albino mice weighing 32–34 g that were divided into four groups, each consisting of six animals. Group 1 was the control and these mice were fed daily with a normal laboratory diet and water, while Group 2–4 were treated with SBL. The SBL was given orally using the various dosages of1, 500, and 1,000 mg/kg of body weight, respectively. Animals were observed for their morphological and physical behavior as well as for mortality. The sub-acute oral toxicity was investigated by measuring the body weight of each animal of Group 1–4 after intervals of every 5 days. All six animals from each group were euthanized using ketamine and xylazine (10 mg/kg) after 15 days for hematological and biochemical studies (Lupașcu et al., 2021; Maia et al., 2022; Aroua et al., 2022).
The relative weight of organs (kidneys and liver) was also calculated, by carefully dissecting the organs and weighing them separately, using the following equation:
3 Results and discussion
3.1 Characterization of the SBL
SBL was obtained as a yellow powder in 81% yield. The compound was characterized via UV, IR, 1H NMR, and FAB-MS spectral measurements. FAB-MS showed the molecular ion peak [M-H]+at m/z 477.4, confirming the molecular formula C28H22N4O4. The FTIR spectrum exhibited a new peak at 1,621 cm−1, suggesting the formation of imine moiety. The UV spectrum displayed the absorption maxima in nm for SBL at 440, 371, 294, and 245. The 1H NMR (AV-400 MHz; DMSO) spectrum showed the most downfield singlet at δ 8.78 for azomethine proton, further supporting the successful synthesis of SBL. The aromatic protons of SBL were displayed at δ 7.26–8.39. Whereas, the methyl protons of the dimeric o-toluidine appeared as a singlet at δ 2.66.
3.2 Adsorption potential of SBL towards metal cations
3.2.1 Metal ions removal from aqueous samples
3.2.1.1 Initial solution pH effect on removal efficiency
The initial pH value of the metal ion solution is an important controlling factor in the adsorption process because it has considerable influence on the solubility of metal cations, on the concentration of opposite ions, and the ionization of surface active sites of the adsorbent (Amin et al., 2020). To examine the initial pH effect on the adsorption efficiencies of Cu(II), Co(II), and Zn(II) by SBL, the pH of the test solutions was varied from 2.0 (extremely acidic) to 12.0 (extremely basic) using suitable buffers. As depicted in Figure 1 the removal of ionic Cu, Co, and Zn depended greatly on the pH of the test solutions; the adsorption potential was increased as the pH was increased from 2–8. However, little change was observed with increasing pH above 8. This trend was expected, as the acidic pH can affect the metal ions sorption on the adsorbent because the H+ ions could compete with the metallic cations for the binding sites present on the surface of the sorbent material. Also, under strong acidic environments, the active functional group of the sorbent becomes protonated; thus creating an electrostatic repulsive force between positively charged metallic ions and the sorbent’s active functionality, which may prevent the adsorption of metal ions. On the other hand, a higher basic pH of pH > 8 caused the sorption potential to decrease due to the formation of hydroxides of the small metallic cations (Hassan et al., 2013). Considering the deposition of metal hydroxides at high basic pH, pH 8 was found to be the optimum pH value for this metal uptake study.
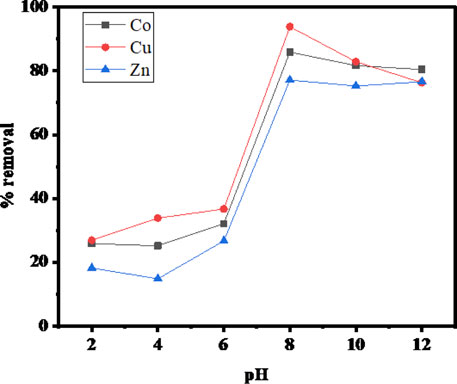
FIGURE 1. pH versus the rate of metal ion uptake (operating conditions: adsorbent dose 0.1 mg, contact time 1.5 h, metal ions concentration 100 ppm, and temperature 25°C).
3.2.1.2 Contact time effects on removal efficiency
The sorbent-sorbate interacting time required for the maximum adsorption potential is another key parameter to be optimized in order to understand the equilibrium condition. The effect of exposure time for the sorption of Cu(II), Co(II), and Zn(II) at pH 8, initial metal ions concentration 100 mg/L, and 0.1 g of SBL was studied as presented in Figure 2 The equilibrium sorption time was 1.5 h. The maximum uptake efficiency at the equilibrium time was 90% for Cu+2, 85% for Co+2, and 75% for Zn+2. Initially, adsorption was rapid due to the easy accessibility of vacant active sites of the adsorbent. Thereafter, it slowed down or stayed constant once the equilibrium was reached owing to the repulsive forces between the adsorbed metallic ions on the adsorbent surface and bulk phases (Sheikh et al., 2021). The removal efficiency of Cu (II) was found greater as compared to Co (II) and Zn (II) with respect to the exposure time. This was possibly due to the interaction of metallic ions to the surface functional group of the sorbent as the pollutant removal efficiency is solely associated with the nature of the functionalities of the adsorbent (Amin and Chetpattananondh, 2019). Hence, at 90 min, the sorption of the investigated metal ions was found to be at a maximum level. Therefore, further experiments were subsequently conducted at this optimized equilibrium time.
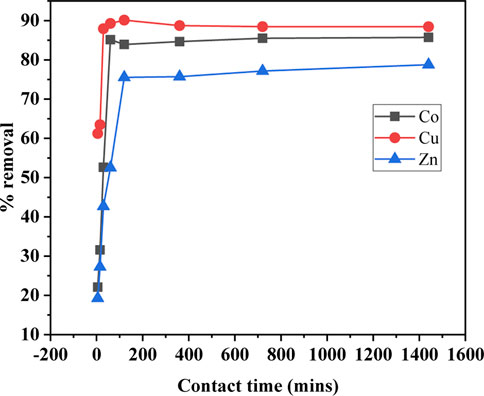
FIGURE 2. Contact time versus percentage of metal ion uptake (operating conditions: adsorbent dose 0.1 mg, pH 8, metal ions concentration 100 ppm, and temperature 25°C).
3.2.1.3 Adsorbent dosage effects on removal efficiency
The effect of SBL dosage on the selected metal ion sorption was studied. Different weight values of SBL (0.1–2 g) were used by adding a definite amount of solid test adsorbent to the 10 mL solution containing the definite initial concentration of the test metal ions (100 mg/L). The outcomes of the experiment are presented in Figure 3. As can be seen, the optimized SBL weight was 0.1 g and exhibited maximum sorption efficiency of 80% for Co (II) and 70% for Cu (II) and Zn (II) ions. It can also be seen that the increased quantity of SBL from 0.1 g did not significantly influence the sorption percentage. This could be associated with the excess amount of the sorbent preventing the metallic cations from approaching the active sites of the adsorbent (Sharef and Fakhre, 2022).
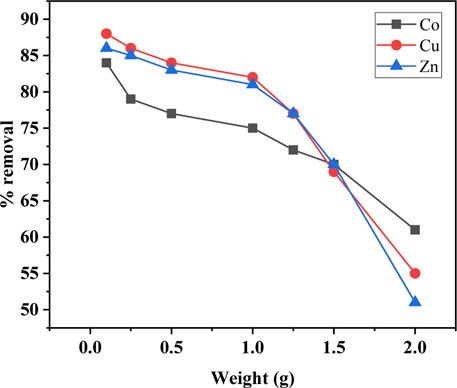
FIGURE 3. Schiff base weight versus the rate of metal ion uptake (operating conditions; metal ion concentration 100 ppm, pH 8, contact time 1.5 h, and temperature 25°C).
3.2.1.4 Initial solution concentration effects on removal efficiency
The effect of initial concentrations of metal ion solutions on the adsorption behavior of the synthesized SBL was investigated and the data obtained are depicted in Figure 4. The metal removal mechanism particularly depends on the initial concentration of the corresponding metal ions. At low concentrations, metal remedial efficiency is high because at lower concentrations the ratio of the surface functional group of the sorbent to the total ions present is high; therefore, the probability of interaction between sorbent and metal cations is high, hence leading to the higher sorption rate. Whereas, with increasing metal cation concentrations the active sites become rapidly saturated as the quantity of the sorbent remained constant (Ahmed et al., 2019). Similar outcomes were reported for the sorption of metal ions on a cross-linked Schiff base (Monier et al., 2010).
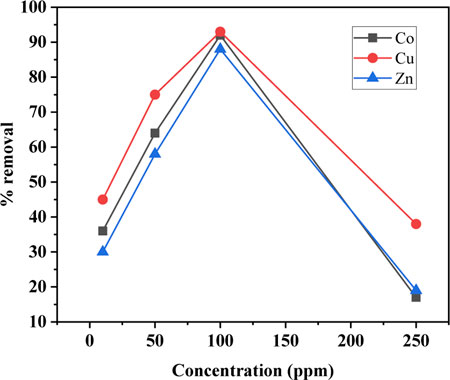
FIGURE 4. Initial metal ion concentrations versus the rate of metal ion uptake (Operating conditions; Adsorbent dose 0.1 mg, pH 8, contact time 1.5 h, and temperature 25°C).
3.2.2 Metal ions removal from soil samples
3.2.2.1 Exposure time and initial solution concentration effects on removal efficiency
Contact time is an important factor because this parameter determines the equilibrium condition of the adsorption process. The exposure time for the adsorption of metal ions by SBL was studied for 120 h. The effect of varying initial concentrations of adsorbate ions was also investigated using 1, 10, 100, and 500 mg/L of Zn(II), Cu(II), and Co(II) on SBL at pH 8. Figures 5A–C demonstrate the removal percentage of metal ions by SBL as a function of contact time and initial concentration of the abovementioned metal ions. As shown in the aforementioned figures, all metal ions follow almost similar adsorption trends. Also, it is observed that initially the removal percentage of each metal ion is zero and increases considerably in a short contact time then ends up with very minor or no change in adsorption. The quick initial change is because of the presence of several active sites, which consequently create a concentration gradient between metal ions in the solution and binding sites at the adsorbent. Over time, this concentration is decreased due to the saturation of active sites and hence the adsorption is decreased (Sheikh et al., 2021).
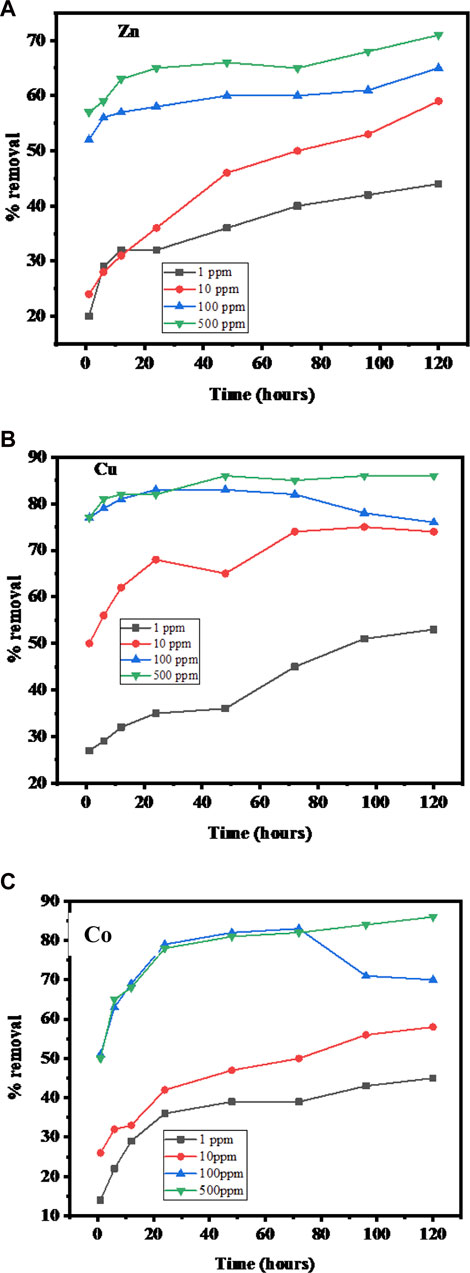
FIGURE 5. (A–C) Percentage removal of Zn(II), Cu(II), and Co(II) versus initial concentration and contact time from soil samples (Operating conditions: adsorbent dose 0.1 mg, pH 8, and temperature 25°C).
The time required to achieve equilibrium depends on the initial metallic ion concentration means; at a lower initial concentration, the system achieves equilibrium in a short period of time. At 1 mg/L initial concentration, the time to reach the equilibrium point is approximately 12 h for all three metal ions. In the cases of 10, 100, and 500 mg/L initial concentrations, the equilibrium time was 24 h for Cu(II), Co(II), and Zn(II). Therefore, the recommended experimental test time for the removal of all three metal ions was 24 h. The experimental results were in line with the results from a previous research (Shahmohammadi-Kalalagh et al., 2011).
3.2.3 Adsorption isotherms study for aqueous and soil samples
The equilibrium adsorption data of Zn(II), Cu(II), and Co(II) ions on to the test sorbent SBL was analyzed using the Langmuir and Freundlich models, as demonstrated in Figures 6A–F; Figures 7A–F for water and soil samples, respectively. As shown in Table 1 for aqueous samples; Table 2 for soil samples, the R2 of the Freundlich isotherm was greater than the Langmuir isotherms for the adsorption of the three investigated metal ions, indicating that the adsorption data were well fitted with the F. isotherm. According to the assumption of the isotherm model, the sorption of the selected metal ions onto the applied sorbent SBL occurred as a multilayer on a surface that was heterogeneous in adsorption affinity. Furthermore, the sorption isotherm of the three metal ions on the SBL was chemical in nature because the value of n for each metal ion was greater than 1 (Es-said et al., 2020; Balkaya and Cesur, 2008; Jiang et al., 2002), and 1/n less than one showed the SBL had a heterogeneous surface with equal energy in all sites (Mishra et al., 2008). These findings affirmed the assertions reported in related studies (Ogbozige et al., 2020; Es-said et al., 2020).
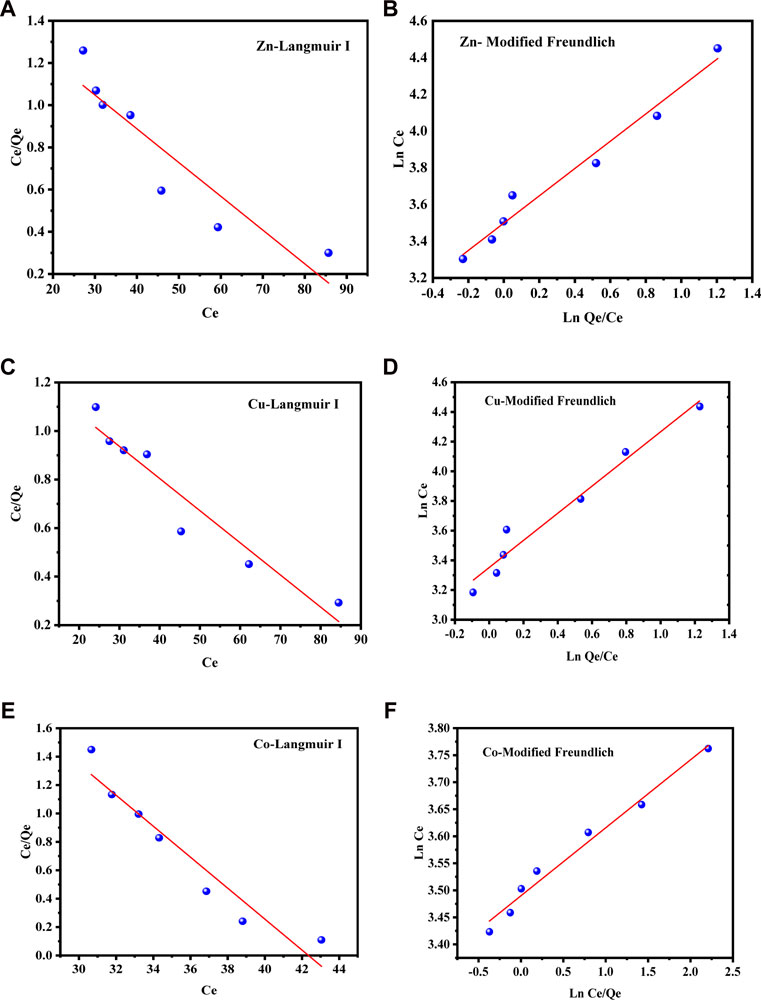
FIGURE 6. (A–F) Langmuir and Freundlich isotherm sorption models of Zn(II), Cu(II), and Co(II) on SBL from aqueous samples.
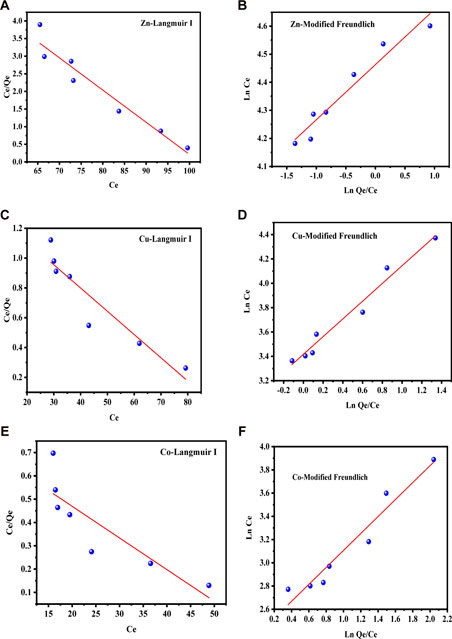
FIGURE 7. (A–F) Langmuir and Freundlich isotherm sorption models of Zn(II), Cu(II), and Co(II) on SBL from soil samples.
The most essential parameter of the Langmuir isotherm is the separation factor (SF), also called the dimensionless constant. The SF values for the three metal ions on SBL at metal ion concentrations of 1 mg/L and 2000 mg/L were studied and are presented in Tables 3; Table 4 for water and soil samples, respectively. The SF values were less than unity for all the metal ions from both samples, demonstrating the favorable potential of the sorbent SBL for the selected metal ions uptake; similar findings were observed in other studies (Sharef and Fakhre, 2022).
3.3 Antimicrobial activity
Antimicrobial efficacy of the prepared Schiff base was checked against P. aeruginosa, S. aureus, K pneumonia, E. coli, S. dysenteriae, and P. vulgarius bacteria, and against A. niger and C. albicans yeast, as presented in Table 5. The results showed that the Schiff base was moderately active against S. dysentriae, P. vulgaris, C. albican, and A. niger, and slightly active against P.aureginosa, S.aureus, K. pneumoniae, and E.coli as compared to the standard drugs Ciprofloxacin and Fluconazole.
3.4 Toxicity
The toxicity of the Schiff base was measured on mice. The 15-day toxicity study on mice showed that there were no signs of changes in behavior, skin color, eyelids, food and water intake, and sleep routine. Table 6
Furthermore, no symptoms of mortality or toxicity were observed. All experimented groups of mice survived till the completion of this study (Table 7), which indicates that no disorder was created in the levels of proteins, carbohydrates, or metabolism of fat (Jothy et al., 2011).
The gradual increase in body weight was registered in both the control and treated groups with no significant difference. Table 7 showed that SBL has negligible acute toxicity on the growth of the mice and similar findings were reported previously on Schiff bases (Saremi et al. (2020)). Concerning body weight, no significant variations were observed in organ and relative organ weight of the liver and kidney (Table 8). The organ weight increase is an indicator of nephrotoxicity (Kluwe, 1981) but the studied Schiff base did not exert any harmful and toxic effects on the kidney and liver, as shown in Tables 9, 10, respectively.
Likewise, no significant changes in the levels of creatinine and uric acid in the control and treated groups of mice were observed (Table 10). The results are in line with the studies published by Ping et al. (2013). Furthermore, total bilirubin, ALP (alkaline phosphatase), ALT (alanine aminotransferase), and AST (aspartate transaminase) levels also showed no significant variations, however, a slight difference in the latter two was detected at 1,000 mg/kg body weight in the control group (Table 10). Elevation of the ALT levels resulted in liver injury, which is characterized as hepatocellular disease, whereas increased activity of AST (a mitochondria enzyme) leads to the severe tissue injuries (Ndrepepa 2021). Hypoproteinemia, a condition observed in cases of liver damage (Larrey, 2002), was also not observed in the present study; indicating that the synthesized Schiff base did not cause any kind of liver damage at the tested dose levels. Similar observations were reported by Priyadarshini et al. (2014), Maia et al. (2022), and Aroua et al. (2022).
4 Conclusion
In this research, the Schiff Base (SBL) was synthesized, characterized, and applied as a sorbent for heavy metal uptake from the prepared contaminated water and soil samples. With a large surface area and small volume, SBL effectively removed Cu(II), Co(II), and Zn(II) from aqueous and soil samples. The experimental data were elucidated using Freundlich and Langmuir isotherms. In the Freundlich, the R2 values were 0.9711, 0.9436, and 0.9421 and 0.8829, 0.9368, and 0.8602 for sorption of Cu+2, Zn+2, and Co+2 from water and soil samples, respectively; and were higher than in the Langmuir model. As reflected by regression coefficients (R2), the sorption data were well-fitted with the Freunlich isotherms. The Freunlich model suggests that the sorption was chemical in nature and the mechanism was multilayer on a heterogeneous surface with equal energy in all sites. Furthermore, the separation factor SF values were less than unity, specifying favorable sorption of investigated metal ions by SBL. The antimicrobial study revealed that SBL exhibited moderate to weak inhibitory activity against the tested six bacterial and two fungal strains. In toxicity of SBL, no signs of acute toxicity were found in treated mice even at its highest dose of 1,000 mg/kg. Furthermore, kidney and liver biomarkers tests revealed that the compound exerted no hepatotoxicity and nephrotoxicity in the treated mice; however, SBL can be further tested for its chronic toxicity.
Data availability statement
The raw data supporting the conclusion of this article will be made available by the authors, without undue reservation.
Author contributions
All authors listed have made a substantial, direct, and intellectual contribution to the work and approved it for publication.
Funding
Researcher Supporting Project number (RSP2023R208) King Saud University Saud Arabia, for funding this research work.
Acknowledgments
The authors extend their thanks to the Research Supporting Project through Project number (RSP2023R208), King Saud University, Riyadh, Saudi Arabia, for funding this research.
Conflict of interest
The authors declare that the research was conducted in the absence of any commercial or financial relationships that could be construed as a potential conflict of interest.
Publisher’s note
All claims expressed in this article are solely those of the authors and do not necessarily represent those of their affiliated organizations, or those of the publisher, the editors and the reviewers. Any product that may be evaluated in this article, or claim that may be made by its manufacturer, is not guaranteed or endorsed by the publisher.
Supplementary material
The Supplementary Material for this article can be found online at: https://www.frontiersin.org/articles/10.3389/fenvs.2023.1128256/full#supplementary-material
References
Ahmed, A., Younesa, H., and El-Maghrabi, H. (2019). Removal of lead ions from wastewater using novel Schiff-base functionalized solid-phase adsorbent. Sep. Sci. Technol. 55, 1589–1602. doi:10.1080/01496395.2019.1604758
Akin, I., Arslan, G., Tor, A., Cengeloglu, Y., and Ersoz, M. (2011). Removal of arsenate [As (V)] and arsenite [As (III)] from water by SWHR and BW-30 reverse osmosis. Desalination 281, 88–92. doi:10.1016/j.desal.2011.07.062
Alluhaybi, A. A., Alharbi, A., Hameed, A. M., Gouda, A. A., Hassen, F. S., El-Gendy, H. S., et al. (2022). A novel triazole schiff base derivatives for remediation of chromium contamination from tannery waste water. Molecules 27, 5087. doi:10.3390/molecules27165087
Amin, M., and Chetpattananondh, P. (2019). Biochar from extracted marine Chlorella sp. residue for high efficiency adsorption with ultrasonication to remove Cr(VI), Zn(II) and Ni(II). Bioresour. Technol. 289. doi:10.1016/j.biortech.2019.121578
Amin, M., Chetpattananondh, P., and Khan, M. N. (2020). Ultrasound assisted adsorption of reactive dye-145 by biochars from marine Chlorella sp. extracted solid waste pyrolyzed at various temperatures. J. Environ. Chem. Eng. 8. doi:10.1016/j.jece.2020.104403
Aroua, L. M., Al-Hakimi, A. N., Abdulghani, M. A., and Alhag, S. K. (2022). Elaboration of novel urea bearing Schiff bases as potent in vitro anticancer candidates with low in vivo acute oral toxicity. Main. Group Chem. 21, 953–973. doi:10.3233/MGC-220019
Azarudeen, R. S., Subha, R., Jeyakumar, D., and Burkanudeen, A. R. (2013). Batch separation studies for the removal of heavy metal ions using a chelating terpolymer: Synthesis, characterization and isotherm models. Sep. Purif. Technol. 116, 366–377. doi:10.1016/j.seppur.2013.05.043
Babel, S., and Kurniawan, T. A. (2003). Low-cost adsorbents for heavy metals uptake from contaminated water: A review. J. Hazard. Mater. 97, 219–243. doi:10.1016/s0304-3894(02)00263-7
Balkaya, N., and Cesur, H. (2008). Adsorption of cadmium from aqueous solution by phosphogypsum. Chem. Eng. J. 140, 247–254. doi:10.1016/j.cej.2007.10.002
Bhargava, S., and Uma, V. (2018). Rapid extraction of Cu (II) heavy metal from industrial waste water by using silver nanoparticles anchored with novel Schiff base. Sep. Sci. Technol. 10, 1182–1193. doi:10.1080/01496395.2018.1527853
Bouhamed, F., Elouear, Z., and Bouzid, J. (2012). Adsorptive removal of copper (II) from aqueous solutions on activated carbon prepared from Tunisian date stones: Equilibrium, kinetics and thermodynamics. J. Taiwan Inst. Chem. Eng. 43, 741–749. doi:10.1016/j.jtice.2012.02.011
Chen, M., Xu, P., Zeng, G., Yang, C., Huang, D., and Zhang, J. (2015). Bioremediation of soils contaminated with polycyclic aromatic hydrocarbons, petroleum, pesticides, chlorophenols and heavy metals by composting: Applications, microbes and future research needs. Biotechnol. Adv. 33, 745–755. doi:10.1016/j.biotechadv.2015.05.003
Cyril, A., Senthamilselvan, G., Sithi, B., and Dhanalakshmi, A. (2022). Potentially active metal of cobalt, copper and zinc complexes derived from schiff base ligand of 3-ethoxy-2-hydroxy-benzaldehyde and aniline for their anticancer activity. J. Adv. Sci. Research 13, 76–84. doi:10.55218/jasr.202213211
Das, S., Das, S., and Ghangrekar, M. M. (2022). Efficacious bioremediation of heavy metals and radionuclides from wastewater employing aquatic macro-and microphytes. J. Basic Microbiology 62, 260–278. doi:10.1002/jobm.202100372
Ding, C., Cheng, W., Sun, Y., and Wang, X. (2015). Retracted: Effects of Bacillus subtilis on the reduction of U(VI) by nano-Fe0. Geochimica Cosmochimica Acta 165, 86–107. doi:10.1016/j.gca.2015.05.036
Egorov, N. S. (1965). Microbe antagonists and biological methods of determining antibiotic activity Microbe antagonists and biological methods of determining antibiotic activity. Moscow: Izdatel'stvo ' Vysshaya Shkola.
Es-Said, A., Nafai, H., Hamdaoui, L. E., Bouhaouss, A., and Bchitou, R. (2020). Adsorptivity and selectivity of heavy metals Cd(II), Cu(II), and Zn(II) toward phosphogypsum. Desalination and Water Treatment 197, 291–299. doi:10.5004/dwt.2020.25964
Fu, F., and Wang, Q. (2011). Removal of heavy metal ions from wastewaters: A review. J. Environ. Manage. 92, 407–418. doi:10.1016/j.jenvman.2010.11.011
Gao, J., Sun, S. P., Zhu, W. P., and Chung, T. S. (2014). Chelating polymer modified P84 nanofiltration (NF) hollow fiber membranes for high efficient heavy metal removal. Water Res. 63, 252–261. doi:10.1016/j.watres.2014.06.006
Gong, J. L., Wang, B., Zeng, G. M., Yang, C. P., Niu, C. G., Niu, Q. Y., et al. (2009). Removal of cationic dyes from aqueous solution using magnetic multi-wall carbon nanotube nanocomposite as adsorbent. J. Hazard. Mater. 164, 1517–1522. doi:10.1016/j.jhazmat.2008.09.072
Gusain, R., Gupta, K., Joshi, P., and Khatri, O. P. (2019). Adsorptive removal and photocatalytic degradation of organic pollutants using metal oxides and their composites: A comprehensive review. Adv. in Colloid and Interface Sci. 272, 102009. doi:10.1016/j.cis.2019.102009
Gupta, G., Khan, J., and Singh, N. K. (2021). Application and efficacy of low-cost adsorbents for metal removal from contaminated water: A review. Mater. Today Proc. 43, 2958–2964. doi:10.1016/j.matpr.2021.01.316
Hassan, R., Arida, H., Montasser, M., and AbdelLatif, N. (2013). Synthesis of new Schiff base from natural products for remediation of water pollution with heavy metals in industrial areas. J. Chem. 2013, 1–10. doi:10.1155/2013/240568
Hu, G., Li, J., and Zeng, G. (2013). Recent development in the treatment of oily sludge from petroleum industry: A review. J. Hazard. Mater. 261, 470–490. doi:10.1016/j.jhazmat.2013.07.069
Hu, R., Wang, X., Dai, S., Shao, D., Hayat, T., and Alsaedi, A. (2015). Application of graphitic carbon nitride for the removal of Pb(II) and aniline from aqueous solutions. Chem. Eng. J. 260, 469–477. doi:10.1016/j.cej.2014.09.013
Ihsanullah, A. A., Al-Amer, A. M., Laoui, T., Al-Marri, M. J., Nasser, M. S., Khraisheh, M., et al. (2016). Heavy metal removal from aqueous solution by advanced carbon nanotubes: Critical review of adsorption applications. Sep. Purif. Technol. 157, 141–161. doi:10.1016/j.seppur.2015.11.039
Islam, M. S., Ahmed, M. K., Raknuzzaman, M., Habibullah-Al-Mamun, M., and Islam, M. K. (2015). Heavy metal pollution in surface water and sediment: A preliminary assessment of an urban river in a developing country. Ecol. Indic. 48, 282–291. doi:10.1016/j.ecolind.2014.08.016
Jiang, J. Q., Cooper, C., and Ouiki, S. (2002). Comparison of modified montmorillonite adsorbents. Part I: Preparation, characterization and phenol adsorption. Chemosphere 47, 711–716. doi:10.1016/s0045-6535(02)00011-5
Jothy, S. L., Zakaria, Z., Chen, Y., Lau, Y. L., Latha, L. Y., and Sasidharan, S. (2011). Acute oral toxicity of methanolic seed extract of Cassia fistula in Mice. Molecules 16, 5268–5282. doi:10.3390/molecules16065268
Kavamura, V. N., and Esposito, E. (2010). Biotechnological strategies applied to the decontamination of soils polluted with heavy metals. Biotechnol. Adv. 28, 61–69. doi:10.1016/j.biotechadv.2009.09.002
Kluwe, W.M. (1981). Renal function tests as indicators of kidney injury in subacute toxicity studies. Toxicol. Appl. Pharmacol. 57, 414–424. doi:10.1016/0041-008x(81)90239-8
Larrey, D. (2002). Epidemiology and individual susceptibility to adverse drug reactions affecting the liver. Semin Liver Dis. 22, 145–156. doi:10.1055/s-2002-30105
Li, X., Du, Y., Wu, G., Li, Z., Li, H., and Sui, H. (2012). Solvent extraction for heavy crude oil removal from contaminated soils. Chemosphere 88, 245–249. doi:10.1016/j.chemosphere.2012.03.021
Lupașcu, G., Pahonțu, E., Shova, S., Bărbuceanu, Ș. F., Badea, M., Paraschivescu, C., et al. (2021). Co (II), Cu (II), Mn (II), Ni (II), Pd (II), and Pt (II) complexes of bidentate Schiff base ligand: Synthesis, crystal structure, and acute toxicity evaluation. Appl. Organomet. Chem. 35, e6149. doi:10.1002/aoc.6149
Maia, D. O., Santos, V. F., Barbosa, C. R., Fróes, Y. N., Muniz, D. F., Santos, A. L., et al. (2022). Nickel (II) chloride Schiff base complex: Synthesis, characterization, toxicity, antibacterial and leishmanicidal activity. Chemico-Biological Interact. 351, 109714. doi:10.1016/j.cbi.2021.109714
Matlock, M. M., Howerton, B. S., and Atwood, D. A. (2002). Chemical precipitation of heavy metals from acid mine drainage. Water Res. 36, 4757–4764. doi:10.1016/s0043-1354(02)00149-5
Meunier, N., Drogui, P., Montane, C., Hausler, R., Mercier, G., and Blais, J. F. (2006). Comparison between electrocoagulation and chemical precipitation for metals removal from acidic soil leachate. J. Hazard. Mater. 137, 581–590. doi:10.1016/j.jhazmat.2006.02.050
Mishra, V. K., and Tripathi, B. D. (2008). Concurrent removal and accumulation of heavy metals by the three aquatic macrophytes. Bioresour. Technol. 99, 7091–7097. doi:10.1016/j.biortech.2008.01.002
Monier, M., Ayad, D. M., Wei, Y., and Sarhan, A. A. (2010). Adsorption of Cu (II), Co (II), and Ni (II) ions by modified magnetic chitosan chelating resin. J. Hazard. Mater. 177, 962–970. doi:10.1016/j.jhazmat.2010.01.012
Naeimi, A., Amini, M., and Okati, N. (2022). Removal of heavy metals from wastewaters using an effective and natural bionanopolymer based on Schiff base chitosan/graphene oxide. Int. J. Environ. Sci. Technol. 19, 1301–1312. doi:10.1007/s13762-021-03247-9
Ndrepepa, G. (2021). Aspartate aminotransferase and cardiovascular disease—a narrative review. J Lab Precis Med. 6.
OECD (2002). Test No. 423: Acute oral toxicity—acute toxic class method; OECD guidelines for the testing of chemicals, section 4. Paris, France: OECD Publishing.
Oehmen, A., Viegas, R., Velizarov, S., Reis, M. A., and Crespo, J. G. (2006). Removal of heavy metals from drinking water supplies through the ion exchange membrane bioreactor. Desalination 199, 405–407. doi:10.1016/j.desal.2006.03.091
Ogbozige, F. J., and Toko, M. A. (2020). Adsorption isotherms and kinetics of Lead and Cadmium ions: comparative studies using modified melon (Citrullus colocynthis) Husk, Iranian (Iranica). Journal of Energy and Environment (IJEE) 11, 157–162. doi:10.5829/ijee.2020.11.02.10
Ping, K. Y., Darah, I., Chen, Y., Sreeramanan, S., and Sasidharan, S. (2013). Acute and subchronic toxicity study of Euphorbia hirta L. methanol extract in rats. BioMed Research International 14. doi:10.1155/2013/182064
Priyadarshini, L., Mazumder, P. B., and Choudhury, M. D. (2014). Acute toxicity and oral glucosetolerance test of ethanol and methanol extracts of antihyperglycaemic plant Cassia alata Linn. J Pharm Biol Sci. 9, 43–46. doi:10.9790/3008-09274346
Ramos, M. A., Yan, W., Li, X. Q., Koel, B. E., and Zhang, W. X. (2009). Simultaneous oxidation and reduction of arsenic by zero-valent iron nanoparticles: Understanding the significance of the core−shell structure. J. Phys. Chem. C 113, 14591–14594. doi:10.1021/jp9051837
Roman-Ross, G., Cuello, G. J., Turrillas, X., Fernandez-Martinez, A., and Charlet, L. (2006). Arsenite sorption and co-precipitation with calcite. Chem. Geol. 233, 328–336. doi:10.1016/j.chemgeo.2006.04.007
Saremi, K., Rad, S. K., Khalilzadeh, M., Hussaini, J., and Abdul Majid, N. (2020). In vivo acute toxicity and anti-gastric evaluation of a novel dichloro Schiff base: Bax and HSP70 alteration. Acta Biochimica et Biophysica Sinica 52, 26–37. doi:10.1093/abbs/gmz140
Shahmohammadi-Kalalagh, S. (2011). Isotherm and kinetic studies on adsorption of Pb, Zn and Cu by kaolinite. Casp. J. Environ. Sci. 9, 243–255.
Sharef, H. Y., and Fakhre, N. A. (2022). Rapid adsorption of some heavy metals using extracted chitosan anchored with new aldehyde to form a Schiff base. PloS one 17, e0274123. doi:10.1371/journal.pone.0274123
Sheikh, Z., Amin, M., Khan, N., Khan, M. N., Sami, S. K., Khan, S. B., et al. (2021). Potential application of Allium Cepa seeds as a novel biosorbent for efficient biosorption of heavy metals ions from aqueous solution. Chemosphere 279, 130545. doi:10.1016/j.chemosphere.2021.130545
Sun, Y., Li, J., and Wang, X. (2014b). Retracted: The retention of uranium and europium onto sepiolite investigated by macroscopic, spectroscopic and modeling techniques. Geochimica Cosmochimica Acta 140, 621–643. doi:10.1016/j.gca.2014.06.001
Sun, Y., Ding, C., Cheng, W., and Wang, X. (2014a). Simultaneous adsorption and reduction of U (VI) on reduced graphene oxide-supported nanoscale zerovalent iron. J. Hazard. Mater. 280, 399–408. doi:10.1016/j.jhazmat.2014.08.023
Sun, Y., Shao, D., Chen, C., Yang, S., and Wang, X. (2013). Highly efficient enrichment of radionuclides on graphene oxide-supported polyaniline. Environ. Sci. Technol. 47, 9904–9910. doi:10.1021/es401174n
Sun, Y., Wang, Q., Chen, C., Tan, X., and Wang, X. (2012). Interaction between Eu (III) and graphene oxide nanosheets investigated by batch and extended X-ray absorption fine structure spectroscopy and by modeling techniques. Environ. Sci. Technol. 46, 6020–6027. doi:10.1021/es300720f
Sun, Y., Wu, Z. Y., Wang, X., Ding, C., Cheng, W., Yu, S. H., et al. (2016a). Macroscopic and microscopic investigation of U (VI) and Eu (III) adsorption on carbonaceous nanofibers. Environ. Sci. Technol. 50, 4459–4467. doi:10.1021/acs.est.6b00058
Sun, Y., Yang, S., Chen, Y., Ding, C., Cheng, W., and Wang, X. (2015). Adsorption and desorption of U (VI) on functionalized graphene oxides: A combined experimental and theoretical study. Environ. Sci. Technol. 49, 4255–4262. doi:10.1021/es505590j
Sun, Y., Zhang, R., Ding, C., Wang, X., Cheng, W., Chen, C., et al. (2016b). Retracted: Adsorption of U(VI) on sericite in the presence of Bacillus subtilis: A combined batch, exafs and modeling techniques. Geochimica Cosmochimica Acta 180, 51–65. doi:10.1016/j.gca.2016.02.012
Tang, W. W., Zeng, G. M., Gong, J. L., Liang, J., Xu, P., Zhang, C., et al. (2014). Impact of humic/fulvic acid on the removal of heavy metals from aqueous solutions using nanomaterials: A review. Sci. total Environ. 468, 1014–1027. doi:10.1016/j.scitotenv.2013.09.044
Tighadouini, S., Roby, O., Radi, S., Lakbaibi, Z., Saddik, R., Mabkhot, Y. N., et al. (2021). A highly efficient environmental-friendly adsorbent based on Schiff base for removal of Cu(II) from aqueous solutions: a combined experimental and theoretical study. Molecules 26, 5164. doi:10.3390/molecules26175164
Udeigwe, T. K., Eze, P. N., Teboh, J. M., and Stietiya, M. H. (2011). Application, chemistry, and environmental implications of contaminant-immobilization amendments on agricultural soil and water quality. Environ. Int. 37, 258–267. doi:10.1016/j.envint.2010.08.008
Xu, P., Zeng, G. M., Huang, D. L., Feng, C. L., Hu, S., Zhao, M. H., et al. (2012). Use of iron oxide nanomaterials in wastewater treatment: A review. Sci. Total Environ. 424, 1–10. doi:10.1016/j.scitotenv.2012.02.023
Zeng, G., Chen, M., and Zeng, Z. (2013b). Risks of neonicotinoid pesticides. Science 340, 1403. doi:10.1126/science.340.6139.1403-a
Zeng, G., Chen, M., and Zeng, Z. (2013a). Shale gas: Surface water also at risk. Nature 499, 154. doi:10.1038/499154c
Zhang, Y., Cao, X., Sun, J., Wu, G., Wang, J., and Zhang, D. (2020). Synthesis of pyridyl Schiff base functionalized SBA-15 mesoporous silica for the removal of Cu (II) and Pb (II) from aqueous solution. J. Sol-Gel Sci. Technol. 94, 658–670. doi:10.1007/s10971-019-05205-x
Zondervan, E., and Roffel, B. (2007). Evaluation of different cleaning agents used for cleaning ultra filtration membranes fouled by surface water. J. Membr. Sci. 304, 40–49. doi:10.1016/j.memsci.2007.06.041
Keywords: Schiff base, low-cost sorbent, metal ion adsorption, antimicrobial activity, acute oral toxicity
Citation: Khan N, Jamil N, Jabeen R, Akhtar MM, Ali H, Mehmood R, Orfali R, Perveen S, Peng J and Al-Taweel AM (2023) A cost-effective o-toulidine-based Schiff base as an efficient sorbent for metal ion uptake from aqueous and soil samples: Synthesis, antimicrobial, and acute toxicity analyses. Front. Environ. Sci. 11:1128256. doi: 10.3389/fenvs.2023.1128256
Received: 20 December 2022; Accepted: 28 February 2023;
Published: 20 March 2023.
Edited by:
Shah Fahad, Leshan Normal University, ChinaReviewed by:
Abeer Moneer, National Institute of Oceanography and Fisheries (NIOF), EgyptYasir Niaz, Khwaja Fareed UEIT, Pakistan
Copyright © 2023 Khan, Jamil, Jabeen, Akhtar, Ali, Mehmood, Orfali, Perveen, Peng and Al-Taweel. This is an open-access article distributed under the terms of the Creative Commons Attribution License (CC BY). The use, distribution or reproduction in other forums is permitted, provided the original author(s) and the copyright owner(s) are credited and that the original publication in this journal is cited, in accordance with accepted academic practice. No use, distribution or reproduction is permitted which does not comply with these terms.
*Correspondence: Malik Muhammad Akhtar, drmalikma21@gmail.com; Nelofer Jamil, nelofer.sbkwu@gmail.com; Raha Orfali, rorfali@ksu.edu.sa