- Institut de Chimie Moléculaire de Reims (ICMR)-UMR CNRS 7312, Université de Reims Champagne Ardenne, Reims, France
The use of agro-industrial wastewater in the agricultural sector is an interesting practice to save resources but it can bring various contaminants to the receiving soils. In this study 19 organic pollutants [8 polycyclic aromatic hydrocarbons (PAH), 6 BTEX, 4-tert-octylphenol, 4-octylphenol, 4-n-nonylphenol, tributyltin and diethylphtalate] were analyzed by gas chromatography-mass spectrometry (GC-MS) in soils irrigated with agro-industrial wastewater from sugar refinery. The soils were sampled at five different locations to evaluate the contamination heterogeneity. Microwave-assisted extraction (MAE) was performed using methanol and hexane and extraction yields ranged from 44% to 96%. The detection limits of the method were between 1.6 and 64 μg·L−1 for polycyclic aromatic hydrocarbons, 0.42 μg·L−1 for diethylphthalate, 0.77 μg·L−1 for tributyltin and until 9.8 μg·L−1 for phenol compounds. The highest contaminant concentrations measured in the soil samples, between 0.4 and 1.2 ng·g−1, were monitored for polycyclic aromatic hydrocarbons molecules, including napthalene, anthracene, fluoranthene, indeno(1,2,3-c,d)pyrene, benzo[a]pyrene, benzo[b]fluoranthene, benzo[k]fluoranthene, and benzo[ghi]perylene. Toluene, ethylbenzene, xylene isomers accounted from 21 to 66 ng·g−1 (mostly toluene). Benzene, 4-tert-octylphenol, 4-octylphenol, 4-n-nonylphenol, tributyltin and diethylphtalate were not detected in soils. The proposed method enables analysis of a wide variety of regulated compounds in a unique preparation step and a unique analytical method. The mean amounts of pollutants were in agreement with measurements or estimates performed in similar contexts.
1 Introduction
The increasing world population and associated resource consumption make water reuse a significant concern. Human activity uses about 4,600 km3 of water yearly and a 20% increase is projected for 2050 (Burek et al., 2016). Irrigation, industrial and municipal uses account for 70%, 19%, and 11%, respectively. Hence water reuse, as a water source, represents a mean to reduce wastewater footprint as well as plant nutrients for agriculture. In addition, as wastewater contain nitrogen, phosphorus, potassium and organic carbon, it serves as plant nutrients for agriculture. As these nutrients usually need energy to be produced, recycling water is also a source of energy saving. That’s why wastewater from municipal treatment plant or agro-industrial plant as an agricultural resource is used in several countries (Brockmann et al., 2018).
However, due to its previous uses in various activities, wastewater can be loaded with undesired products such as hazardous chemical substances towards human or crop growth. The stability and chemical properties of these organic pollutants result in their accumulation in the topsoil due to its rich organic content (Holoubek et al., 2009). Consequently, many countries have put in place regulations to limit the emission of pollutants in wastewater in order to preserve its quality for reuse. In Table 1 is shown the list of dangerous substances in the environment and the corresponding quantification limits needed to monitor water quality in France in 2014 (Ministère de l'écologie du développement durable et de l'énergie, 2014). It includes five classes of molecules such as polycyclic aromatic hydrocarbons (PAH), alkylphenols, phthalates, organotin and benzene, toluene, ethylbenzene and xylenes (BTEX). Fees are applied on non-domestic wastewater based on pollutant concentrations. Hence, monitoring the output of these substances has become an economical concern for agro-industrial actors reusing wastewater.
In France, contaminants in soils are monitored by the French National Soil Monitoring Network (Réseau de Mesures de la Qualité des Soils—RMQS). Several studies focused on the persistence in soils of PAHs by assessing the impact of atmospheric flux and road transportation (Clément et al., 2015; Gaspéri et al., 2018). BTEX are emitted by vehicle combustion in the atmosphere and then carried by rain into soils by leaching the atmosphere (Popescu and Popescu, 2017). Alkylphenol ethoxylates are found in several goods such as detergents, pesticides, inks, and paper leading to octylphenol and nonylphenol by degradation. They have been detected at high concentrations in soils and few countries have legislation about their concentration in agricultural soils (Llorca-Pórcel et al., 2009; Pérez et al., 2012). Tributyltin is known to be toxic for numerous organisms including humans. Many of industrial or domestic products like fungicides, insecticides, bactericides, wood preservatives and polyvinyl chloride (PVC) stabilizers have been recognized as potential sources of contamination (Champ, 2000). Phthalates have numerous applications such as automotive manufacturing and packaging. Diethylhexyl phthalate may reach up to 50% in PVC composition, and as it is not chemically bound to the polymer, it can be easily released in the environment during PVC production as well as end-product use, storage, or degradation (Heudorf et al., 2007). For these compounds, sludge application is one of the most important sources of agricultural soil contamination (Marcic et al., 2006). Thereby, monitoring the concentration of these pollutants is important to maintain the quality of agricultural soils.
All these contaminants were previously analyzed in soils by mass spectrometry using several extraction methods. PAHs were classically analyzed by gas chromatography coupled with mass spectrometry (GC-MS) (Poster et al., 2006). Their soil extraction was performed using various techniques such as soxhlet extraction, microwave assisted extraction (Wang et al., 2007; Xu and Lee, 2008) and pressurized liquid extraction (Gibson et al., 2005; Martínez Vidal et al., 2009). Alkylphenols can also be analyzed using GC-MS (Gibsons et al., 2005) after soil extraction with pressurized liquid extraction (Andreu et al., 2007) or microwave assisted extraction (Pérez et al., 2012). Heroult et al. (2008) compared several extraction techniques for tributyltin analysis in soils including mechanical stirring, accelerated solvent, microwave and ultrasonic extraction also using GC-MS. Phthalates analysis in soils was performed by liquid or gas chromatography after ultrasonic extraction (Ma et al., 2005) or microwave extraction (Chee et al., 1996; Liang et al., 2010). From these different studies, microwave extraction followed by GC-MS analysis was found to be a reliable analytical method for the monitoring of these pollutants. As for the BTEX class compounds, they are usually extracted from soils via headspace chromatography (Ezquerro et al., 2004; Shin, 2012) or liquid-liquid extraction (Khajeh et al., 2014) and to our knowledge no extraction was performed using a microwave oven. Thus it is important to optimize the methods allowing to analyze the whole set of compounds of concern which have various properties in a simple and fast way while respecting the performances required by the legislator.
In this context, this study aims at standardizing the extraction and analysis (quantification) of these different compound families with low detection limits. For this purpose, five soils sampled in different sites irrigated with wastewater from agro-industrial plant were analyzed by GC-MS using microwave-assisted extraction (MAE) targeting the organic substances identified by the French regulation.
2 Materials and methods
2.1 Sample sites
Five sites were sampled to characterize their physico-chemical properties in the Grand Reims area (Figure 1), which is an intercommunal structure located in the Grand Est Region (France) with 300,699 inhabitants in 2019 and a population density of 211/km2. Agriculture in this area fall into four main activities which are vineyard, beets and alfalfa. Beet cultures are valorized locally in several products such as sugar, ethanol and alcohol. The area of the sampling site is a large beet agricultural zone crossed by active traffic roads, close to the Reims airfield, agricultural facilities and concrete industry. Several villages with a population between 500 and 1,000 inhabitants are surrounding the sites in a 2 km radius in addition to the city of Reims located 5 km west. All these activities may act as indirect sources of pollutants especially combustion residues. The cultures were spread with the effluents of a sugar factory at a 600 m3·ha−1 (60 mm) maximum rate with a minimum of 2 years between each spreading on the same parcel. Land application with such effluents started in 1978. Only the data focusing on the nutritious capacity of the effluents were available. The concentrations of targeted pollutants were not known. Soil samples were provided by the association of agronomic spreading monitoring (ASAE, France). The sampling was focused on the rich organic content layer of the soil where the pollutants can accumulate. The topsoil was collected with a shovel until 25 cm depth and stored in a closed polyethylene bag at 4°C before use. The soils were characterized for particle size distribution without decarbonation, pH, cation exchange capacity (CEC), organic carbon (OC), and CaCO3 contents according to French and International standard methods, following NF X31-107, ISO 10390, NF X 31-130, ISO 14235, and ISO 10693, respectively (AFNOR, 1995; AFNOR, 1998; AFNOR, 1999; AFNOR, 2003; AFNOR, 2005). Organic matter (OM) content was calculated as follows: % OM = 1.72% OC.
2.2 Chemicals and reagents
Methanol and hexane (HiPerSolv Chromanorm), as well as nylon filter (0.2 µm) syringes and filter supports were purchased from VWR. The standards benzo(k)fluoranthene, benzo(b)fluoranthene, benzo(a)pyrene, indéno(1,2,3-c,d)pyrene, benzo(g,h,i)perylene, anthracene, fluoranthene, naphtalene, benzene, toluene, ethylbenzene, xylene (o,m,p), diethylphtalate, tributyltin, 4-octylphenol, 4-tert-octylphenol, 4-n-nonylphenol were of the highest purity and purchased from Sigma Aldrich.
2.3 Microwave assisted extraction
Soil extraction was performed using a Thermo Scientific Ethos Easy microwave extractor with a Fast-24 rotor. The samples in glass tubes were subjected to a 1,800 W power to reach 110°C in 10 min, this temperature was then maintained during 10 min. PAH and BTEX compounds were extracted from 2 g of soils in 10 mL of hexane; for diethylphtalate, tributyltin and phenolic compounds, 5 g of soils in 10 mL of methanol were used. After microwave extraction the liquid phase was filtered through a nylon membrane and stored in a glass tube. The solvent was then evaporated to dryness in a centrifugal concentrator Genevac HT Series (SP Scientific, Warminster, PA, United States). The manufacturer programs “very low boiling point” and “medium boiling point” were used for hexane and methanol extraction respectively. The temperature was set at 40°C with a rotation speed of 1,400 rpm. The evaporation process started with a decrease in pressure from the atmospheric pressure to full vacuum in 1 h and was maintained for 6 h. The residue was dissolved in 500 µL of methanol and put inside a micro-vial for GC-MS analysis.
2.4 GC-MS analysis
The target compound concentrations were determined using a Thermo Scientific TRACE 1300 GC system coupled to a Thermo Scientific ISQ Series quadrupole mass spectrometer equipped with an electronic impact source (Low activity). The separation was achieved by injecting 0.5 µL of the sample in a Thermo Scientific TR5-5MS column (30 m, I.D. 0.25 mm, film 0.25 µm). Data were processed with the XCalibur™ 2.2 software. Injection was made in splitless mode. The transfer temperature was set at 250°C with a source at 230°C. The dwell time was set at 0.5 s for each ion. Single Ion Monitoring (SIM) mode was used with ion quantification and identification as listed in Table 2. The temperature program was from 90°C to 300°C with a ramp of 5°C/min and from 40°C during 8 min–300°C with a ramp of 40°C/min for PAH and BTEX, respectively. For the other compounds, it was from 90°C to 300°C with a ramp of 50°C/min. Each soil sample was analyzed three times and a blank extraction without soil was inserted in each extraction batch to check for potential contamination during extraction process.
2.5 Quantification and validation method
The concentrations of target compounds were calculated by an external standard calibration method. A calibration curve with five points was obtained using the pure standard in hexane for HAP and BTEX and methanol for alkylphenol, phtalate and TBT. The limits of quantification (LOQ) was calculated based on the signal to noise approach defined in the ICH Harmonized Tripartite Guideline (2005) by dividing the concentration found in extracted samples by a tenth of the signal-to-noise ratio measured in the chromatogram. Finally, the soil contaminant concentrations were calculated by applying a yield factor on each concentration measured after extraction. The extraction yield factors of adsorbed pollutant were calculated by spiking in triplicate a pristine soil in water with a known amount of each contaminant (100 ng per g of soil) and shaking for 24 h to ensure that equilibrium adsorption is reached. After filtration through a nylon membrane (0.2 µm), the liquid fraction was analyzed. The adsorbed amount (deduced by difference between the introduced concentration (100 ng·g−1) and the remaining concentration after shaking) was extracted from the solid fraction by MAE and analyzed to determine the extraction yield. The method was validated by following exactly the same protocol meaning by spiking in triplicated another pristine soil with a known amout of each contaminant. Then the sampled soil was analyzed using the validated method.
2.6 Statistical analysis
Standard deviation, linearity and coefficient of determination were calculated with Excel 2016 datasheet using build-in function (LINEST and STDEV.S) for linear regression. The LOD and LOQ was calculated with the signal to noise approach according to ICH Harmonized Tripartite Guideline (2005). The noise was determinated graphically on the chromatogram for each compounds then the LOQ was calculated as the equivalent concentration of ten times the noise and the LOD as the equivalent concentration of three times the noise.
3 Results and discussion
3.1 Soil characterisation
The soil physico-chemical properties are reported in Table 3. The five soils were very similar in texture: PYZC8, PYZB2, PYZC7, and NOZH22 were loam, and NOZD69 was silt loam. They displayed a basic pH (≈8.4) which is characteristic of calcareous soils of the Champagne-Ardenne region and in accordance with their high CaCO3 content (between 44% and 73%). Their OC content was relatively high, ranging between 2.9% and 3.3%, and their CEC was comprised between 8.1 and 13.4 cmol·kg−1. The lack of diversity in soil physico-chemical properties and composition makes extrapolation of data difficult, however a previous study of extractability of organic pollutants using MAE with a wide variety of matrix type has shown that the main soil constituant influencing the extractability of pollutants is total organic carbon (Báez et al., 2003).
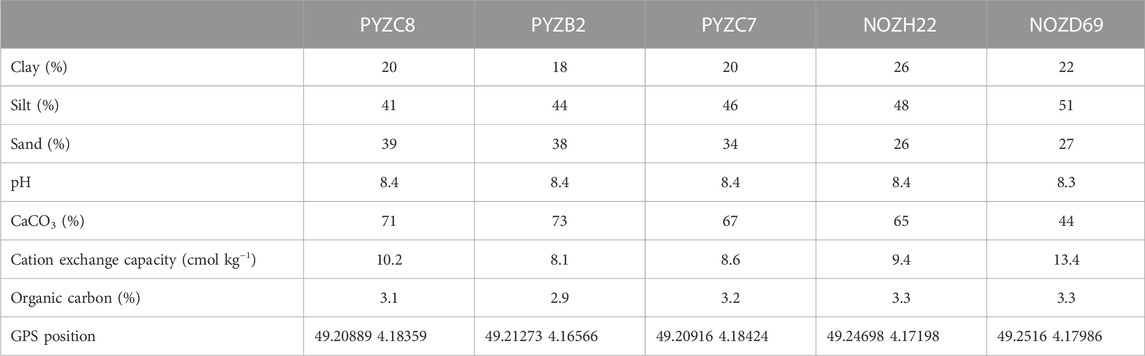
TABLE 3. Physico-chemical characteristics of the sampled soils and GPS localization. Percentages of organic matter and CaCO3 are expressed as a weight percentage of dry whole solid. Percentages of sand, silt and clay are expressed as weight percentages of dry mineral solid.
3.2 Analytical performance
The performance of the method is summarized in Table 1. Extraction yields ranged from 44% to 96%. The extraction of diethylphtalate, octylphenols, nonylphenols, and toluene was almost quantitative, the one of BTEX compounds ranged from 72% to 96%, while half of the PAH and tributhyltin cation remained adsorbed or lost by degradation during the extraction process. The extraction procedure was intended to be as simple as possible leading in some cases to relatively low extraction yield. However, in each case, the accuracy of the extraction yields was validated by the reproducibility of the extraction allowing an extrapolation of the exact content. In most cases, the LOQ were in the range of a tenth of a ng·g−1 (few hundreds of ppt). In the case of phenolic compounds, the LOQ were significantly higher, around 50 ng·g−1. The LOQ values obtained in this paper for the analysis of BTEX and PAH in soils are similar to those reported in previous studies also measured by GC-MS but after solid-phase microextraction (SPME) extraction for BTEX (Franco et al., 2015) or solvent extraction for PAH (Maliszewska-Kordybach et al., 2009). A significant decrease of the detection limits could be reached for alkylphenols by including a derivation step with N-methyltrifluoroacetamide (Zhang et al., 2009) and an alkylation step for tributyltin (Crnoja et al., 2001). Khosravi and Price, 2015 obtained a better quantification limit of less than 1 pg·g−1 for diethylphtalate using an ASE-SPE-GC-MS method with a higher volume of sample concentrated and transferred in the injector during the analysis. Although other methods have better quantification limits especially when the methods are specifically designed for a single class of compound adding derivation and purification steps (e.g., for alkylphenols) the advantage of our method is the ability to analyze all the target compounds with a small number of preparation steps thus reducing the global cost of analysis. Nevertheless, this strategy leds to a reduction of the performance for some compounds by balancing selectivity and extraction efficiency but allows to reach the quantification limit fixed by the regulation for the whole list of compounds.
3.3 Concentrations of organic contaminants in soil samples
The results of soil analyses are reported in Table 4. PAH and BTEX (except benzene) were identified in all samples at relatively high concentrations, reaching levels higher than 100 ng·g−1 in the case of benzo(b)fluoranthene, indeno(1,2,3-c,d)pyrene, benzo(g,h,i)perylene, and fluoranthene. The total concentration ranged from 406 to 1,237 ng·g−1 for ∑8PAH and from 21 to 65 ng·g−1 for ∑BTEX. In France there are no mandatory values defining concentration limits of these pollutants in soil. Concentration limits only exist for ground-water and solid waste. Usually, concentrations are discussed according to the local context or the mean background concentrations. Various field campaigns evidenced the presence of PAH compounds in European soils at amounts depending on the anthropic activities in the area. For example, in Switzerland, concentrations of 98–219 ng·g−1 were found in different forest sites (Bucheli et al., 2004), whereas these concentrations could reach 575 ng·g−1 in agricultural soils impacted by anthropic activities (Berset and Holzer, 1995). Sometimes, very high contents of PAH were found like in Poland with concentrations reaching 6,680 ng·g−1 in soil less than 5 km from the emission source typically industries and urban areas (Maliszewska-Kordybach et al., 2009). In France, a model of the PAH contamination distribution based on 549 uncultivated soil samples throughout the country showed that HAP remained below the detection limits (<5–50 ng·g−1) in most samples (Villanneau et al., 2013). However, in highly contaminated sites (mining, metallurgy and coal burning activities) individual HAP reached 100–1,000 ng·g−1. The authors evidenced that fluoranthene, pyrene and phenanthrene concentrations where geographically correlated with the contamination levels in the northeastern part of France due to historical industrial activities. The concentrations of each PAH in the contaminated soils were between 15 and 35 ng·g−1. In another study, higher values ranging from 190 to 760 ng·g−1 were detected in unamended cropland influenced by urban activity and possibly impacted by direct deposition from agricultural engine particles (Gateuille et al., 2014). In the present study, the individual PAH concentrations measured (n.d to 429 ng·g−1) were in the line with European agricultural soils considering its localization (located in the northeastern part of France and near urban and agricultural areas). In agricultural soil, PAH may impact crops growth or germination and invertebrates reproduction. Exposition studies on terrestrial plants (Sinapsis alba, Trifolium pratense, and Lolium perenne) with several PAHs found EC20 values from 30 to 650 μg·g−1 for the most sensitive values which is above the total of measured PAH (Sverdrup et al., 2003). Survival and reproduction studies of springtail Folsomia fimetaria L., in contaminated soil showed a typical LC50 and EC50 of 21 and 11 μg·g−1 respectively with no-observed-effect concentration of 8.6 μg·g−1 for the most sensitive PAH (Sverdrup et al., 2001) which is also above the total of measured PAH.
Concerning the BTEX, few data are available in the literature concerning their concentration into agricultural soils because they are considered to be mainly present in the atmospheric compartment due to their volatility. BTEX are emitted directly by leakage, stock manipulation or sludge application, but they can reach the soil compartment after their emission into the atmosphere by industry, wastewater treatment plant and vehicle exhausts. For benzene, a predicted environmental concentration of 0.02 ng·g−1 based on all the releases into the environment was determined in non-contaminated soils or without direct emission at vicinity (EU, 2008). Taking into account the deposition through the atmosphere, the predicted concentrations in agricultural soils increased in the range 1.21–129 ng·g−1 (EU, 2008). In the present study, the benzene concentration in the five studied soils was lower than the quantification limit (0.2 ng·g−1) which was consistent with field studies conducted in uncontaminated soils (Ellison et al., 1997). Concerning toluene, modelisation based on emission calculated from traffic and industry was used to estimate its concentration in air, water and soils at local and regional scale (Hansen et al., 2003). According to this model the expected background concentration of toluene in agricultural was 0.1 ng·g−1 and the mean concentration in agricultural soils located near different industrial sites was 7 ng·g−1. In addition, in the case of field studies without any direct pollution context, toluene soil concentrations of 1 ng·g−1 and 2.1–5.8 ng·g−1 were reported in the Netherland (Sloof and Blokzijl, 1987) and in Poland near petrol station or allotment garden (Zygmunt and Namiesnik, 2001), respectively. In this study, the toluene concentration ranged from 5 to 55 ng·g−1. As for ethylbenzene and xylene data in agricultural soils are very scarce and mostly related to direct spill incident via leakage with relative high concentrations in soils. However, using a simple box model, the concentration of ethylbenzene coming from atmospheric depositions was estimated at 0.07 ng·g−1 in agricultural soils and until 39 ng·g−1 in local areas (EU, 2007). In our studied soils, the ethylbenzene measured concentrations were in the lowest part of this range (around 2 ng·g−1); xylene concentrations were between 1 and 9 ng·g−1 which is relatively low compared to the concentrations detected in United States soils ranging from 1 to 190 ng·g−1 (Agency for Toxic Substances and Disease Registry (ATSDR), 2007). Thus, according to the cited examples, the measured concentrations of BTEX in the present study is in the same order of level as the measured value in the literature.
The other contaminants, alkylphenols, phthalates and tributyltin were not detected in the studied soil samples. They are surfactants and plasticizers introduced by contaminated wastewater applications or direct deposition from agricultural treatments. Their concentration are thus related to wastewater contamination and agricultural practices. Tributyltin can also be introduced by rainfall leading to very weak concentrations (<1 ng·g−1 Sn) (Huang et al., 2004). These compounds are known to persist several days before their degradation in well oxygenated soils (Topp and Starratt, 2000; Hoch, 2001) or deeper migration in soils (Sopheak et al., 2015). As the study focused on topsoil, deeper migration in soil cannot be ruled out. Moreover, this study only focused on regulated pollutants without taking into account their degradation products that may impact the ecosystems. Further experiments will be performed, including these degradation products, to assess their occurrence and impact. Alternatively, other data could indicate a historical contamination such as crop health, ecosystem description, soil fertility or soil microbial content. In any case our study shown that no gradient or trace is left on the topsoil.
4 Conclusion
This study aimed at quantifying multiple organic contaminants targeted by the regulation on industrial wastewater management. The main advantage of the proposed method is to minimize the preparation step of the samples and to allow the compound analysis in an only one analytical method, where other methods need several extraction and derivatization steps (with obvious higher performance) with higher costs. The performances (LOQ) reached by the optimized method meet the legislator requirements. Significant quantities of PAH and BTEX were found in sampled agricultural soils. The mean amounts of these compounds were in agreement with measurements or estimates performed in similar contexts. However, a more detailed work is required to improve the understanding of the contamination scope and location. Investigations are needed to quantify source apportionment of the hydrocarbons found in soils as well as their potential accumulation. In contrast, no plasticizer or surfactant was detected suggesting that degradation or migration efficiently removed the potential soil contamination. Also a more detailed study dedicated to the spreading activities would enable to get a better insight into these processes.
Data availability statement
The raw data supporting the conclusion of this article will be made available by the authors, without undue reservation.
Author contributions
Formal analysis and investigation by NM; Conceptualization, Methodology, Writing—original draft preparation review and editing: NM, SS, and EG; Funding acquisition, Resources, Supervision: EG; NM, SS, and EG read and approved the final manuscript.
Funding
This work was supported by the “Seine-Normandie” water agency (AESN).
Acknowledgments
The “Association de Suivi des Epandages Agronomiques” (ASAE, M. Robert) is gratefully acknowledged for providing the soil samples. The “Seine-Normandie” water agency (AESN) is gratefully thanked for its financial support.
Conflict of interest
The authors declare that the research was conducted in the absence of any commercial or financial relationships that could be construed as a potential conflict of interest.
Publisher’s note
All claims expressed in this article are solely those of the authors and do not necessarily represent those of their affiliated organizations, or those of the publisher, the editors and the reviewers. Any product that may be evaluated in this article, or claim that may be made by its manufacturer, is not guaranteed or endorsed by the publisher.
References
AFNOR (Association Française de Normalisation) (1999). Qualité des sols - Méthodes chimiques - Détermination de la capacité d'échange cationique (CEC) et des cations extractibles (NF X31-130). Paris: AFNOR. (in French).
AFNOR (Association Française de Normalisation) (2003). Qualité du sol Détermination de la Distribution Granulométrique des Particules du sol Méthode à la Pipette (AFNOR NF X31-107). Paris: AFNOR. (in French).
AFNOR (Association Française de Normalisation) (1995). Qualité du sol Détermination de la Teneur en Carbonate – Méthode Volumétrique (NF ISO 10693). Paris: AFNOR. (in French).
AFNOR (Association Française de Normalisation) (2005). Qualité du sol Détermination du pH (NF ISO 10390). Paris: AFNOR. (in French).
AFNOR (Association Française de Normalisation) (1998). Qualité du sol Dosage du Carbone Organique par Oxydation Sulfochromique (NF ISO 14235). Paris: AFNOR. (in French).
Agency for Toxic Substances and Disease Registry (ATSDR) (2007). Toxicological profile for Xylenes. Atlanta, GA: U.S. Environmental Protection Agency. Available at: https://wwwn.cdc.gov/TSP/ToxProfiles/ToxProfiles.aspx?id=296&tid=53.
Andreu, V., Ferrer, E., Rubio, J. L., Font, G., and Picó, Y. (2007). Quantitative determination of octylphenol, nonylphenol, alkylphenol ethoxylates and alcohol ethoxylates by pressurized liquid extraction and liquid chromatography–mass spectrometry in soils treated with sewage sludges. Sci. Total Environ. 378, 124–129. doi:10.1016/j.scitotenv.2007.01.024
Báez, M. E., Aponte, A., and Sánchez-Rasero, F. (2003). Microwave-assisted solvent extraction of the herbicide methabenzthiazuron from soils and some soil natural organic and inorganic constituents. Influence of environmental factors on its extractability. Analyst 128, 1478–1484. doi:10.1039/B312288C
Berset, J. D., and Holzer, R. (1995). Organic micropollutants in swiss agriculture: Distribution of polynuclear aromatic hydrocarbons (PAH) and polychlorinated biphenyls (PCB) in soil, liquid manure, sewage sludge and compost samples; a comparative study. J. Environ. Anal. Chem. 59, 145–165. doi:10.1080/03067319508041324
Brockmann, D., Pradel, M., and Hélias, A. (2018). Agricultural use of organic residues in life cycle assessment: Current practices and proposal for the computation of field emissions and of the nitrogen mineral fertilizer equivalent. Resour. Resour. Conserv. Recycl. 133, 50–62. doi:10.1016/j.resconrec.2018.01.034
Bucheli, T. D., Blum, F., Desaules, A., and Gustafsson, Ö. (2004). Polycyclic aromatic hydrocarbons, black carbon, and molecular markers in soils of Switzerland. Chemosphere 56, 1061–1076. doi:10.1016/j.chemosphere.2004.06.002
Burek, P., Satoh, Y., Fischer, G., Kahil, M. T., Scherzer, A., Tramberend, S., et al. (2016). “Water futures and solution - fast track initiative (final report),” in IIASA working paper WP 16-006. Available at: http://pure.iiasa.ac.at/13008.
Champ, M. A. (2000). A review of organotin regulatory strategies, pending actions, related costs and benefits. Sci. Total Environ. 258, 21–71. doi:10.1016/S0048-9697(00)00506-4
Chee, K. K., Wong, M. K., and Lee, H. K. (1996). Microwave extraction of phthalate esters from marine sediment and soil. Chromatographia 42, 378–384. doi:10.1007/BF02272126
Clément, N., Muresan, B., Hedde, M., and François, D. (2015). PAH dynamics in roadside environments: Influence on the consistency of diagnostic ratio values and ecosystem contamination assessments. Sci. Total Environ. 538, 997–1009. doi:10.1016/j.scitotenv.2015.08.072
Crnoja, M., Haberhauer-Troyer, C., Rosenberg, E., and Grasserbauera, M. (2001). Determination of Sn- and Pb-organic compounds by solid-phase microextraction-gas chromatography-atomic emission detection (SPME-GC-AED) after in situ propylation with sodium tetrapropylborate. J. Anal. At. Spectrom. 16, 1160–1166. doi:10.1039/B104694M
Ellison, R. J., Larnimaa, K., Margary, S. A., Mata, J. M., Muller, J-M., Peterson, D. R., et al. (1997). Exposure profile: Gasoline, 97/52. Brussels: Concawe Report No.
EU (2007). “European union risk assessment report,” in Ethylbenzene. Available at: https://echa.europa.eu/documents/10162/f9b4577b-c57f-439b-b15a-9e268b1d0a58.
EU (2008). “European union risk assessment report,” in Benzene. Available at: https://echa.europa.eu/documents/10162/be2a96a7-40f6-40d7-81e5-b8c3f948efc2.
Ezquerro, Ó., Ortiz, G., Pons, B., and Tena, M. (2004). Determination of benzene, toluene, ethylbenzene and xylenes in soils by multiple headspace solid-phase microextraction. J. Chromatogr. A 1035, 17–22. doi:10.1016/j.chroma.2004.02.030
Franco, M. S., da Silva, M. R., Santos-Neto, A. J., and Lanças, F. M. (2015). SPME determination of low concentration levels of monoaromatic chemical markers in soils after remediation by supercritical fluid extraction. Anal. Methods 7, 4901–4907. doi:10.1039/C5AY00467E
Gaspéri, J., Ayrault, S., Moreau-Guigon, E., Alliot, F., Labadie, P., Budzinski, H., et al. (2018). Contamination of soils by metals and organic micropollutants: Case study of the parisian conurbation. Environ. Sci. Pollut. Res. 25, 23559–23573. doi:10.1007/s11356-016-8005-2
Gateuille, D., Evrard, O., Lefevre, I., Moreau-Guigon, E., Alliot, F., Chevreuil, M., et al. (2014). Mass balance and decontamination times of Polycyclic Aromatic Hydrocarbons in rural nested catchments of an early industrialized region (Seine River basin, France). Sci. Total Environ. 470–471, 608–617. doi:10.1016/j.scitotenv.2013.10.009
Gibson, R., Wang, M.-J., Padgett, E., and Beck, A. J. (2005). Analysis of 4-nonylphenols, phthalates, and polychlorinated biphenyls in soils and biosolids. Chemosphere 61, 1336–1344. doi:10.1016/j.chemosphere.2005.03.072
Hansen, B., Munn, S., Pakalin, S., Luotamo, M., Allanou, R., Pellegrini, G., et al. (2003). European union risk assessment report toluene. Available at: https://publications.jrc.ec.europa.eu/repository/handle/JRC24380.
Heroult, J., Zuliani, T., Bueno, M., Denaix, L., and Lespes, G. (2008). Analytical advances in butyl-phenyl- and octyltin speciation analysis in soil by GC-PFPD. Talanta 75, 486–493. doi:10.1016/j.talanta.2007.11.053
Heudorf, U., Mersch-Sundermann, V., and Angerer, J. (2007). Phthalates: Toxicology and exposure. Int. J. Hyg. Environ. Health 210, 623–634. doi:10.1016/j.ijheh.2007.07.011
Hoch, M. (2001). Organotin compounds in the environment — An overview. Appl. Geochem. 16, 719–743. doi:10.1016/S0883-2927(00)00067-6
Holoubek, I., Dušek, L., Sáňka, M., Hofman, J., Čupr, P., Jarkovský, J., et al. (2009). Soil burdens of persistent organic pollutants – their levels, fate and risk. Part I. Variation of concentration ranges according to different soil uses and locations. Environ. Pollut. 157, 3207–3217. doi:10.1016/j.envpol.2009.05.031
Huang, J.-H., Schwesig, D., and Matzner, E. (2004). Organotin compounds in precipitation, fog and soils of a forested ecosystem in Germany. Environ. Pollut. 130, 177–186. doi:10.1016/j.envpol.2003.12.012
ICH Harmonized Tripartite Guideline (2005). Validation of analytical procedures: Text and methodology, Q2.R1
Khajeh, M., Moosavi-Movahedi, A. A., Shakeri, M., Zadeh, F. M., Khajeh, A., and Bohlooli, M. (2014). Dispersive solid phase extraction combined with dispersive liquid–liquid extraction for the determination of BTEX in soil samples: Ant colony optimization–artificial neural network. J. Chemom. 29, 245–252. doi:10.1002/cem.2706
Khosravi, K., and Price, G. W. (2015). Determination of phthalates in soils and biosolids using accelerated solvent extraction coupled with SPE cleanup and GC–MS quantification. Microchem. J. 121, 205–212. doi:10.1016/j.microc.2015.03.013
Llorca-Pórcel, J., Martínez-Parreño, M., Martínez-Soriano, E., and Valor, I. (2009). Analysis of chlorophenols, bisphenol-A, 4-tert-octylphenol and 4-nonylphenols in soil by means of ultrasonic solvent extraction and stir bar sorptive extraction with in situ derivatisation. J. Chromatogr. A 1216, 5955–5961. doi:10.1016/j.chroma.2009.06.043
Liang, P., Zhang, L., Peng, L., Li, Q., and Zhao, E. (2010). Determination of phthalate esters in soil samples by microwave assisted extraction and high performance liquid chromatography. Bull. Environ. Contam. Toxicol. 85, 147–151. doi:10.1007/s00128-010-0078-x
Ma, L. L., Muir, D., Wang, X. T., and Xu, X. B. (2005). Simultaneous analysis of organic pollutants in soils by gas chromatography and gas chromatography–mass spectrometry. Int. J. Environ. Anal. Chem. 85, 89–98. doi:10.1080/03067310512331324736
Maliszewska-Kordybach, B., Smreczak, B., and Klimkowicz-Pawlas, A. (2009). Concentrations, sources, and spatial distribution of individual polycyclic aromatic hydrocarbons (PAHs) in agricultural soils in the eastern part of the EU: Poland as a case study. Sci. Total Environ. 407, 3746–3753. doi:10.1016/j.scitotenv.2009.01.010
Marcic, C., Hecho, I. L., Denaix, L., and Lespes, G. (2006). TBT and TPhT persistence in a sludged soil. Chemosphere 65, 2322–2332. doi:10.1016/j.chemosphere.2006.05.007
Martínez Vidal, J. L., Garrido Frenich, A., de las Nieves Barco Bonilla, M., Romero-González, R., and Padilla Sánchez, J. A. (2009). Simultaneous extraction of polycyclic aromatic hydrocarbons and polychlorinated biphenyls in agricultural soils by pressurized liquid extraction and determination by gas chromatography coupled to tandem mass spectrometry. Anal. Bioanal. Chem. 395, 1551–1562. doi:10.1007/s00216-009-3111-3
Ministère de l’écologie du développement durable et de l’énergie (2014). Décret n° 2014-1578 du 23 décembre 2014 paru au JO du 26 décembre 2014. (in French). Available at: https://www.legifrance.gouv.fr/loda/id/JORFTEXT000029958114/.
Pérez, R. A., Albero, B., Miguel, E., and Sánchez-Brunete, C. (2012). Determination of parabens and endocrine-disrupting alkylphenols in soil by gas chromatography--mass spectrometry following matrix solid-phase dispersion or in-column microwave-assisted extraction: A comparative study. Anal. Bioanal. Chem. 402, 2347–2357. doi:10.1007/s00216-011-5248-0
Popescu, R. S., and Popescu, L. L. (2017). Assessment of air pollution, by the urban traffic, in university campus of bucharest. J. Environ. Prot. 8, 884–897. doi:10.4236/jep.2017.88055
Poster, D. L., Schantz, M. M., Sander, L. C., and Wise, S. A. (2006). Analysis of polycyclic aromatic hydrocarbons (PAHs) in environmental samples: A critical review of gas chromatographic (GC) methods. Anal. Bioanal. Chem. 386, 859–881. doi:10.1007/s00216-006-0771-0
Shin, H.-S. (2012). Determination of MTBE, TBA and BTEX in soil by headspace gas chromatography-mass spectrometry. Bull. Korean Chem. Soc. 33, 1693–1698. doi:10.5012/bkcs.2012.33.5.1693
Sopheak, N., Sempéré, R., Delmont, A., Paluselli, A., and Ouddane, B. (2015). Occurrence, fate, behavior and ecotoxicological state of phthalates in different environmental matrices. Environ. Sci. Technol. 49, 4019–4035. doi:10.1021/es505233b
Sverdrup, L. E., Kelley, A. E., Krogh, P. H., Nielsen, T., Jensen, J., Scott-Fordsmand, J., et al. (2001). Effects of eight polycyclic aromatic compounds on the survival and reproduction of thespringtail Folsomia fimetaria L. (collembolan, isotomidae). Environ. Toxicol. Chem. 20, 1332–1338.doi:10.1897/1551-5028(2001)020<1332:eoepac>2.0.co;2
Sverdrup, L. E., Krogh, P. H., Nielsen, T., Kjaer, C., and Stenersen, J. (2003). Toxicity of eight polycyclic aromatic compounds to red clover (Trifolium pratense), ryegrass (Lolium perenne) and mustard (Sinapsis alba). Chemosphere 53, 993–1003. doi:10.1016/S0045-6535(03)00584-8
Topp, E., and Starratt, A. (2000). Rapid mineralization of the endocrine-disrupting chemical 4-nonylphenol in soil. Environ. Toxicol. Chem. 19, 313–318. doi:10.1002/etc.5620190210
Villanneau, E. E., Saby, N. N., Orton, T. T., Jolivet, C. C., Boulonne, L. L., Caria, G. G., et al. (2013). First evidence of large-scale PAH trends in French soils. Environ. Chem. Lett. 11, 99–104. doi:10.1007/s10311-013-0401-y
Wang, W., Meng, B., Lu, X., Liu, Y., and Tao, S. (2007). Extraction of polycyclic aromatic hydrocarbons and organochlorine pesticides from soils: A comparison between soxhlet extraction, microwave-assisted extraction and accelerated solvent extraction techniques. Anal. Chim. Acta 602, 211–222. doi:10.1016/j.aca.2007.09.023
Xu, L., and Lee, H. K. (2008). Novel approach to microwave-assisted extraction and micro-solid-phase extraction from soil using graphite fibers as sorbent. J. Chromatogr. A 1192, 203–207. doi:10.1016/j.chroma.2008.03.060
Keywords: agro-industrial wastewater, soil, gas chromatography, mass spectrometry, organic contaminations, field measurement
Citation: Maurin N, Sayen S and Guillon E (2023) Gas chromatography–mass spectrometry analysis of organic pollutants in French soils irrigated with agro-industrial wastewater. Front. Environ. Sci. 11:1125487. doi: 10.3389/fenvs.2023.1125487
Received: 16 December 2022; Accepted: 20 March 2023;
Published: 03 April 2023.
Edited by:
Xiaokai Zhang, Jiangnan University, ChinaReviewed by:
Zhao-Feng Yuan, Zhejiang University, ChinaJie Wang, Nanjing Agricultural University, China
Copyright © 2023 Maurin, Sayen and Guillon. This is an open-access article distributed under the terms of the Creative Commons Attribution License (CC BY). The use, distribution or reproduction in other forums is permitted, provided the original author(s) and the copyright owner(s) are credited and that the original publication in this journal is cited, in accordance with accepted academic practice. No use, distribution or reproduction is permitted which does not comply with these terms.
*Correspondence: Nicolas Maurin, nicolas.maurin@univ-reims.fr