- 1Faculty of Environment and Resource Studies, Mahidol University, Nakhon Pathom, Thailand
- 2Graduate School of Fisheries and Environmental Sciences, Nagasaki University, Nagasaki, Japan
- 3Department of Soil Science and Environment, Faculty of Agriculture, Khon Kaen University, Khon Kaen, Thailand
- 4Integrated Land and Water Resource Management Research and Development Center in Northeast Thailand, Khon Kaen University, Khon Kaen, Thailand
- 5Laboratory of Soil Science, Research Faculty of Agriculture, Hokkaido University, Sapporo, Japan
The hill tribes in Thailand traditionally depend on rotational shifting cultivation (RSC). However, insufficient understanding remains on post-fire soil properties and soil erodibility (k-values) with fallow years. To address this gap, the levels of soil organic carbon (SOC), soil total nitrogen (STN), soil nutrients, and soil erodibility after fire in RSC were investigated. Topsoil (0–10 cm) samples from sites with 4 (RSC-4Y), 5 (RSC-5Y), and 7 (RSC-7Y) fallow years in Chiang Mai Province, northern Thailand, were taken at four time points: before burning, 5 min after burning, 9 months after burning, and 2 years after burning. Soil pH, electrical conductivity, and soil nutrient (available P, K, and Ca) levels were increased after burning and remained higher than the pre-burning levels for at least 2 years. The SOC stock decreased after burning in all fallow fields. At 2 years after burning, the SOC stock in RSC-4Y was higher than before burning, whereas in RSC-5Y and RSC-7Y, the levels had not reached the pre-fire levels. The STN stocks of all studied fields significantly decreased after burning and had not reached the pre-burning levels after 2 years. After burning, the topsoil of RSC-4Y was most susceptible to erosion. However, only in RSC-4Y, the k-value was unchanged at 2 years after burning. Three different approaches are recommended for post-fire land management: 1) farmers should not cut and remove the weeds and grasses at the soil surface, 2) burning should be performed around late winter or early summer (November–February) to inhibit complete combustion, and 3) contour-felled log erosion barriers should be made by using the trunks remaining after the fire to trap the sediment and slow down surface runoff.
1 Introduction
Fire is a fundamental factor in ecological systems (Francos and Úbeda, 2021). It alters several ecosystem components, such as soil, air, water, plants, and microorganisms (DeBano et al., 1998; Caton et al., 2019; Lucas-Borja et al., 2019), which affects the changes in soil nutrients and plant growth. Fire regimes (intensity and severity) are crucial for changing soil properties and the recovery after a fire (Nolan et al., 2021), as well as for frequency and seasonality (Jurvélius, 2004; Flannigan et al., 2009). Previous studies have investigated the direct and indirect effects of wildfire on soil and vegetation (Certini, 2005; Lucas-Borja et al., 2021), including nutrient losses after burning by runoff and erosion (Robichaud et al., 2000; Mataix-Solera et al., 2002). In South and Southeast Asia, fire is used as a tool for land preparation in shifting cultivation, supporting many ethnic minorities in terms of livelihood and food security (AIPP and IWGIA, 2014).
Shifting cultivation, also known as slash-and-burn or swidden agriculture, refers to a rotational farming technique in which the land is cleared by fire for cultivation and then abandoned to recover after harvest (Heinimann et al., 2017). In northern Thailand, shifting cultivation is a traditional farming practice in the mountainous area, and fire is normally used for burning vegetation residues after cutting (Arunrat et al., 2022a). Generally, there are two basic types of shifting cultivation, namely, pioneer and rotational shifting cultivation (Rerkasem and Rerkasem, 1994). In pioneer shifting cultivation, the primary forest is cleared, burnt, cropped, harvested, and abandoned, and subsequently, the village is moved to a new site, for which a part of the primary forest is being cleared. Rotational shifting cultivation (RSC) consists of a long fallow period, and the village is a permanent settlement and not moved to a new site after harvesting. The cultivated plots in RSC are managed as fallow cycles, while one plot is temporarily cultivated and then abandoned. This allows the recovery of the vegetation and soil fertility while the cultivator moves on to another plot. Currently, there are strict forest protection laws in Thailand, and the clearing of pioneer forests and the building of new settlements are prohibited. Thus, the existing shifting cultivation areas in Thailand are referred to as RSC.
Several studies have reported the variation in soil properties after a fire. Giovannini et al. (1988) reported that organic matter (OM) was completely oxidized at about 460°C during a fire. This can lead to changes in nutrient availability to plants (Phillips et al., 2000; Zhu et al., 2021), facilitating the recovery of ecosystem processes (Pellegrini et al., 2018). With specific regard to soil nutrient losses and recovery, fire consumes vegetation, increases the surface runoff and soil erosion rates, and transports nutrients downslope (Cawson et al., 2012; Vieira et al., 2015). The factors determining nutrient loss are erodible soil particles, rainfall, and topographic characteristics. Pierson et al. (2019) reported that fire caused the loss of soil carbon and nitrogen, which was related to erosion rates. To date, a study by Arunrat et al. (2022a) investigated the impacts of fire on soil properties in RSC in northern Thailand. The authors found that soil pH and electrical conductivity significantly increased after a fire, whereas soil organic carbon (SOC), soil total nitrogen (STN), and some soil properties [soil texture, available P, exchangeable K, exchangeable Ca, exchangeable Mg, bulk density, and organic matter] were not significantly changed due to the low fire intensity and short fire duration. The OM at the soil surface has been reported as being most susceptible to high fire intensity, resulting in changes in soil properties. However, the effects of fire on post-fire recovery in RSC in northern Thailand are still unknown.
Fire and erosion interact with the change of soil physical and chemical properties after the fire due to the losses of vegetation and litter at the surface layer (Larsen et al., 2009). In the most concerning case, these losses of vegetation and litter usually occurred during intense rainfall periods on the hillslopes (Carroll et al., 2007). The Universal Soil Loss Equation (USLE) is a widely used model for assessing soil loss, elaborated by Wischmeier and Smith (1978) and later modified in the Revised Universal Soil Loss Equation (RUSLE) (Renard et al., 1997; 2017). The five erosion factors in the RUSLE model are soil erodibility (k-factor), rainfall erosivity (R), topography (LS), land cover (C), and practice (P). The k-factor is the most suitable factor for assessing soil susceptibility to erosion (USDA, 1954; Wischmeier and Smith, 1965; Wischmeier and Smith, 1978; Kyveryga et al., 2004), as organic carbon (OC) content and different-sized soil particles are the main parameters affecting soil erodibility, especially in hillslopes (Emadi et al., 2009). Soil erodibility is related to the granulometry, structure, and stability of soil aggregates, indicating that the soils with higher silt contents are more erodible than clayey soils. Meanwhile, OM also plays an important role in soil erodibility by maintaining the stability of soil aggregates (Deng et al., 2018). However, fire may alter the changes of these properties, which may increase or decrease the level of soil erodibility. A study by Arunrat et al. (2022b), studying terraced paddy fields and upland rice fields in northern Thailand, found that the increase in soil erodibility was consistent with a decrease in SOC stock. Unfortunately, the effect of post-fire on soil erodibility in RSC is still not well understood.
To fill the research gaps and extend the previous investigation in RSC, this study focused on deepening our understanding of post-fire recovery in RSC, with the following two objectives: 1) to examine the recovery of soil organic carbon, soil total nitrogen, and soil nutrients after a fire and 2) to assess soil erodibility changes in RSC. The results of this study provide a scientific reference for the minimization of post-fire risks and can contribute to implementing suitable management and restoration, which can help reduce soil degradation in RSC.
2 Materials and methods
2.1 Study area and RSC field selection
The study was conducted at Ban Mae Pok, Ban Thab subdistrict, Mae Chaem district, Chiang Mai province, northern Thailand (Figure 1). The terrain is mountainous, with an elevation of 700–1,000 m above sea level. The average annual temperature is 25.4°C. The winter season is from October to February, with a minimum temperature of 15°C, and the maximum temperature in summer is 39°C, from February to May. The rainy season is from September to October, with an average annual precipitation of 1,140.2 mm (Department of Mineral Resources, 2015). In the highlands (slope >35%) of Thailand, soils are classified as slope complex series (LDD, 1992; Arunrat et al., 2022c). The soil is mostly reddish-brown lateritic, with a sandy clay loam and a sandy clay texture based on USDA classification. The soil pH is acidic (5.0–5.8), and the organic matter level ranges from 2.60% to 3.86% (Mae Chaem Watershed Research Station, 2010).
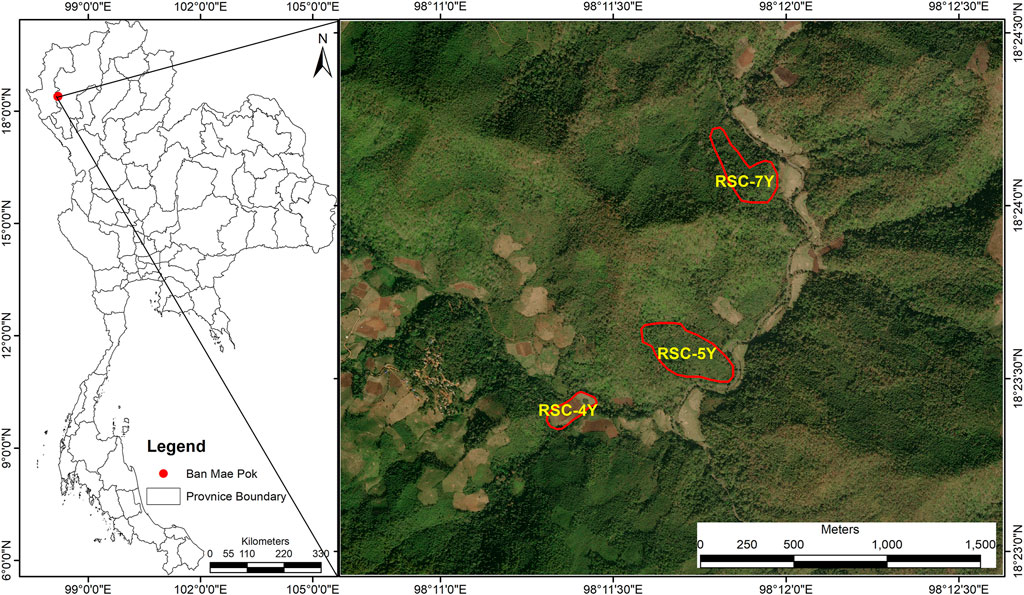
FIGURE 1. Study area; RSC-7Y = 7-year fallow (18° 24' 6.57"N, 98° 11' 53.68"E), RSC-5Y = 5-year fallow (18° 23' 33.38"N, 98° 11' 45.99"E), and RSC-4Y = 4-year fallow (18° 23' 24.99" N, 98° 11' 22.73" E); the aerial image was taken from Google Maps on 2 December 2022.
Three RSC fields were selected based on a similar previous land use type (maize cultivation) before fallow to allow secondary succession. The RSC-4Y field refers to the 4-year fallow field after maize harvesting; it was cleared, burnt, and cultivated with upland rice in March 2020, at an elevation of 614 m a.s.l and a slope gradient of 21%. The RSC-5Y field was a 5-year fallow field after maize harvesting; it was cleared, burned, and cultivated with upland rice in March 2020, at an elevation of 643 m a.s.l and with a slope gradient of 25%. The RSC-7Y field was a 7-year fallow field after maize harvesting; it was cleared, burnt, and cultivated with upland rice in March 2020, at an elevation of 632 m a.s.l and with a slope gradient of 22%. All RSC fields were identified with the boundary and background history by the head of the community and field owners. At each field, a transect was designed, starting from the uppermost toposequence point to the lowermost toposequence point, and then the five replication plots were marked along the transect for soil sampling. The dominant tree species are Pterocarpus macrocarpus Kurz, Xylia xylocarpa (Roxb.) Taub, and Lithocarpus ceriferus. Moreover, Tectona grandis Linn. f., Bombax ceiba Linn., Pinus kesiya, Mangifera caloneura Kurz, Oroxylum indicum (L.) Kurz, Diospyros glandulosa Lace, Spondias pinnata (L.f.) Kurz, and Syzygium cumini can also be found. The shrub and herbal vegetation are mostly Chromolaena odorata (L.) R.M.King & H. Rob, Brainea insignis (Hook.) J. Sm., and Betula alnoides Buch. Ham.
2.2 Fire measurements
In March 2020, all three experimental fields were cleared and burned for upland rice cultivation. During burning, the fire temperature at the surface layer at each plot of each field was measured using an infrared thermometer. Soil temperatures and soil moisture were measured at a soil depth of 1 cm before and 5 min after burning, using a thermocouple and moisture meter, respectively.
2.3 Soil and ash sampling and analysis
Topsoil samples were taken from all three fields at depths of 0–10 cm at four time points: before burning (March 2020), 5 min after burning (March 2020), 9 months after burning (harvest, December 2020), and 2 years after burning (March 2022). Topsoil was sampled because the effects of fire are best reflected at a depth of 0–10 cm, whereas soil erodibility is usually reflected at the soil surface.
Overall, 60 topsoil samples were obtained (three RSC fields × five plots × one depth × four time points). At each RSC field, topsoil samples were collected from the same five plots at each time point. At each plot (10 × 10 m), the topsoil samples were taken from five pits and thoroughly mixed to obtain one composite sample per plot. Grasses, stones, roots, and other residues were removed manually, and approximately 1 kg of soil was placed in a plastic bag. Ash colors were identified by the Munsell soil color charts 5 min after burning. Each color of ash was carefully determined for each sample plot. A steel soil corer (5.0 cm width × 5.5 cm length) was used to collect a soil sample for determining soil bulk density after oven-drying at 105°C for 24 h.
Soil texture was measured using the hydrometer method. Soil and ash pH values were determined using a pH meter with a 1:1 and 1:10 suspension of solid-to-water ratio, respectively (National Soil Survey Center, 1996). Electrical conductivity (ECe) was measured using an EC meter in saturation paste extracts (Sparks et al., 1996). Total nitrogen (TN) was determined using the micro-Kjeldahl method. The available calcium (Ca), magnesium (Mg), and potassium (K) concentrations were analyzed by atomic absorption spectrometry (NH4OAc pH 7.0 extraction) (Thomas, 1996). The available phosphorus (P) was measured using the molybdate blue method (Bray II extraction) (Bray and Kurtz, 1945), and the organic carbon (OC) content was analyzed following the method of Walkley and Black (1934).
2.4 Soil organic carbon and total nitrogen estimation
The SOC stock was estimated using the following equation:
where SOCstock is the soil organic carbon stock (Mg C ha−1), OC is the organic carbon content (%), BD is the soil bulk density (Mg m−3), and L is the soil thickness (m).
The STN stock was calculated using the following equation:
where STNstock is the soil total nitrogen (Mg N ha−1), TN is the total nitrogen content (%), BD is the soil bulk density (Mg m−3), and L is the soil thickness (m).
Since soil bulk density varies over time, the soil thickness (cm) was adjusted based on a soil mass basis for eliminating the error in the SOC stock calculations. The following equations were used (Ellert and Bettany, 1995):
where
The additional soil thickness (m) for each field was calculated using the following equation (Arunrat et al., 2021):
where
2.5 Soil erodibility estimation
There are three widely used approaches for assessing soil erodibility (k-factor), namely, the nomograph (Wischmeier et al., 1971), the K-factor in the Environmental Policy Integrated Climate (EPIC) model (Williams et al., 1983), and a formula proposed by Shirazi and Boersma (1984). Among them, the k-factor in the EPIC model is calculated using the OC content and the soil particle distribution (the contents of sand, silt, and clay); this approach is widely used to predict soil erosion and indicate soil degradation (Wu et al., 2012; Chen et al., 2013; Zhang et al., 2018; Liu et al., 2020; Arunrat et al., 2022b; Arunrat et al., 2022c). Therefore, the k-factor in the EPIC model was used in this study, with the following equation:
where SAN is the fraction of sand (%), SIL is the fraction of silt (%), CLA is the fraction of clay (%), OC refers to the organic carbon concentration (%), and 0.1317 is the conversion factor for United States business units (t acre h/100 acre/ft/tanf/in) to the international system of units (t h MJ-1 mm-1). A higher k-value indicates that the soil is easily eroded.
2.6 Statistical analysis
Significant differences in soil parameters along a fallow chronosequence were tested by analysis of variance (ANOVA). Tukey´s honestly significant difference (HSD) test was employed to perform the multiple post hoc mean comparisons when the ANOVA result was significant at p ≤ 0.05. Redundancy analysis (RDA) was used to investigate the multivariate response of soil properties to burning. The Hellinger transformation of values was applied to adjust the normality and homogeneity of the data. Variables with collinearity, variance inflation factor (VIF) > 10, and correlation coefficients |r | > 0.7 were eliminated. All statistical analyses and visualizations were performed using the R environment (v. 4.0.2).
3 Results
3.1 Fire temperature, soil temperature, and soil moisture after burning
The highest fire temperature of 579°C was detected for the RSC-5Y field, whereas the lowest temperature of 173°C was observed for the RSC-4Y field. At a soil depth of 1 cm, soil temperatures increased around 16.3°C–19.2°C at 5 min after burning in all RSC fields. Field RSC-7Y showed the highest soil temperature of 46.3°C, followed by the RSC-5Y (45.7°C) and RSC-4Y (43.5°C) fields, respectively. Soil moisture decreased by 0.4%–2.1% 5 min after burning due to the soil water content evaporating (Table 1). Fire temperatures in the surface layer and soil temperature mainly depended on the amount of dried fuel in each field.
3.2 Chemical properties of ash
The OM and TN contents were significantly different among dark reddish-brown, gray, and white ash colors (Table 2). The dark reddish-brown ash showed high values of pH, ECe, OM, and TN compared with gray and white ash (Table 2). White ash contained the lowest levels of OM (2.01%), TN (0.03%), and available K (1,567.33 mg kg−1), whereas available Ca was the highest (11,540.23 mg kg−1) (Table 2). The ashes were produced under different heating temperatures, which changed their color. Black ash is produced at low temperatures (<300°C) and is the product of incomplete combustion of the litter (Úbeda et al., 2009; Kuzyakov et al., 2018), which contains a high proportion of carbon (Khanna et al., 1994). The reddish color is due to the oxidation of iron minerals at low temperatures (Markl et al., 2006). At > 500°C, the ash became gray and white in color, which indicated that fire severity was high and litter underwent more complete combustion (Úbeda et al., 2009; Kuzyakov et al., 2018).
3.3 Soil organic carbon
The highest soil organic carbon (SOC) stock in the topsoil (0–10 cm) was found before burning in the RSC-7Y field, with 44.6 Mg C ha−1, whereas the SOC stock was decreased after burning in all fields. Before burning, the RSC-5Y and RSC-7Y fields showed similar SOC levels, significantly differing from that of the RSC-4Y field. No remarkable variation in the SOC stock was found when comparing the soils of the RSC-4Y and RSC-5Y fields at 5 min after burning. At 9 months after burning, the SOC stock had reached the highest level of 42.51 Mg C ha−1 in the RSC-5Y field. There was no significant difference in the SOC stock between the RSC-5Y and RSC-7Y fields at 2 years after burning, whereas the SOC stock was significantly lower in the RSC-4Y field (Figure 2).
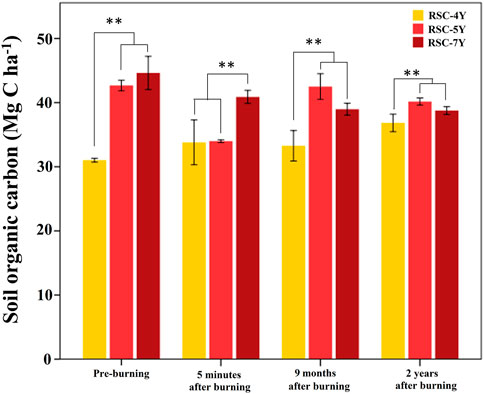
FIGURE 2. Soil organic carbon levels (Mg C ha−1) in the topsoil (0–10 cm) before burning and at 5 min, 9 months, and 2 years after burning in three fallow fields.
At 2 years after burning, the SOC stock was higher than before burning in the RSC-4Y field, with an increase from 31.03 to 36.83 Mg C ha−1 (18.7%). On the other hand, the SOC stocks in RSC-5Y (5.9% decrease) and RSC-7Y (13.1% decrease) could not reach pre-fire levels (Figure 2).
3.4 Soil total nitrogen
Before burning, the highest stock of soil total nitrogen (STN) in the topsoil (0–10 cm) was found in the RSC-7Y field, with 5.23 Mg N ha−1, whereas the STN level was significantly lower in RSC-4Y. A similar trend was observed at 5 min after burning in all fields. Notably, the STN stock was significantly higher in the RSC-5Y field, with 4.14 Mg N ha−1, at 9 months after burning compared to the other fields. All three fallow fields showed significant differences in STN stock levels at 2 years after burning (Figure 3).
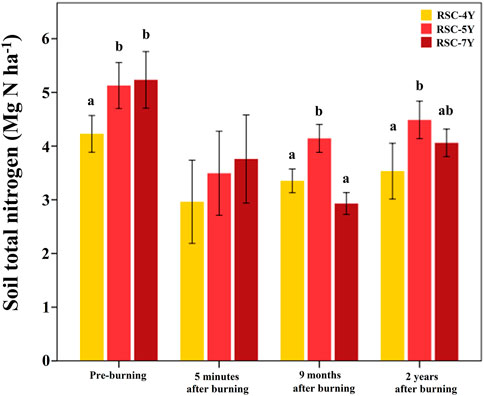
FIGURE 3. Soil total nitrogen (Mg N ha−1) in the topsoil (0–10 cm) before burning and at 5 min, 9 months, and 2 years after burning in three fallow fields.
In all fields, the STN stocks were significantly lower after burning (with an average decrease of 30.0%) and higher at 9 months after burning, although after 2 years, the initial levels were not reached (with an average decrease of 17.1%; Figure 3).
3.5 Soil chemical properties
The variations in soil chemical properties among the RSC-4Y, RSC-5Y, and RSC-7Y fields after maize harvesting under different conditions at the four time points are shown in Table 3.
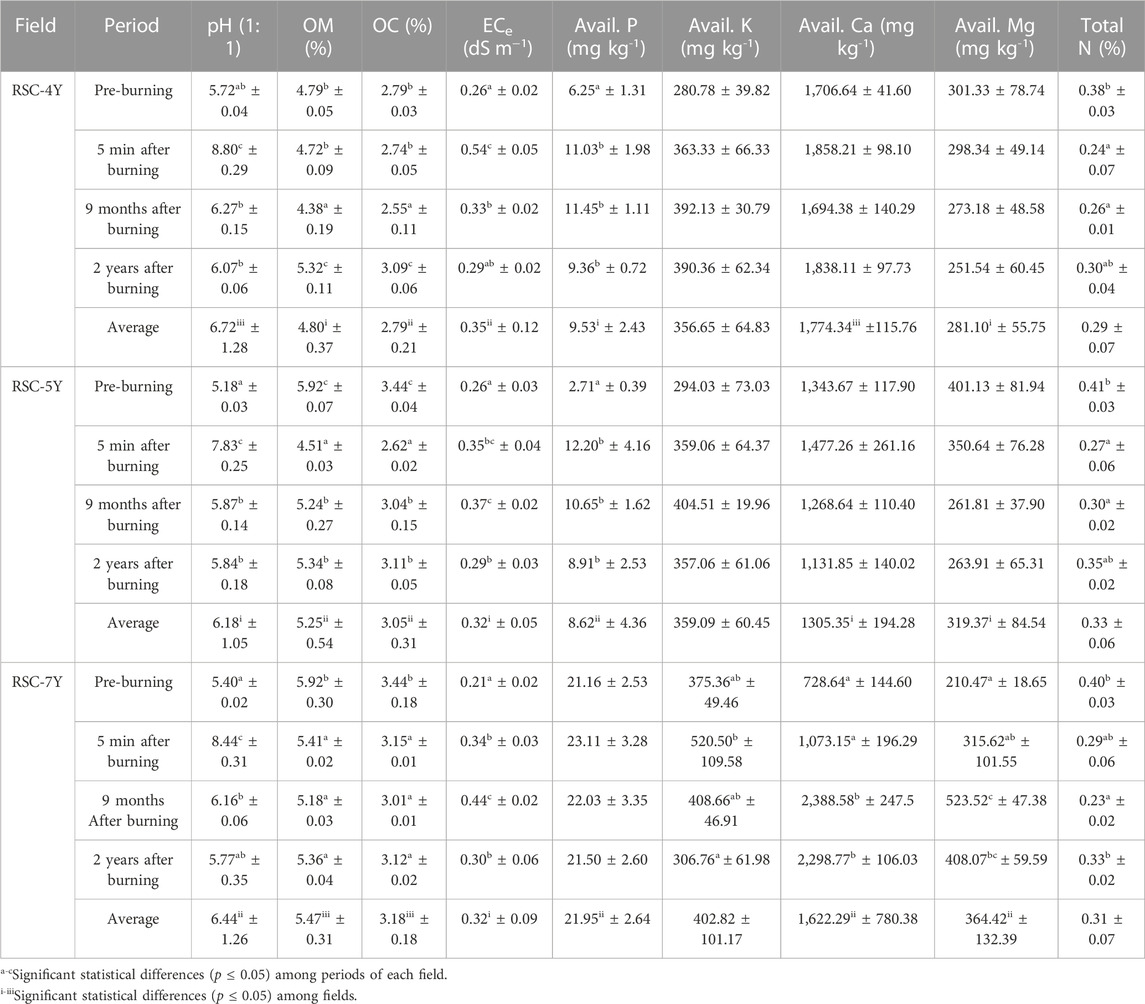
TABLE 3. Variations in soil chemical properties: pH; organic matter (%); organic carbon (%), ECe (dS m−1); available P, K, Ca, and Mg (mg kg−1); and total nitrogen (%) along the fallow chronosequence.
In the RSC-4Y field, the pH level was 5.72 before burning. However, it increased to 8.80 5 min after burning and decreased to 6.27 and 6.07 at 9 months and 2 years after burning, respectively. There was no significant difference in the pH value between 9 months and 2 years after burning in the RSC-5Y and RSC-7Y fields. When comparing the overall pH levels of the three fields, notable differences were observed between the RSC-4Y and RSC-5Y fields. The soil pH was significantly increased after burning at all fallow fields. At 2 years after burning, the soil pH levels were still high compared with pre-burning levels (Table 3).
In the RSC-4Y field, before burning, the percentage of OM was 4.79%, whereas the RSC-5Y and RSC-7Y fields showed OM percentages of 5.92% and 5.92%, respectively. Notably, in the RSC-7Y field, there were no significant differences in OM among the three time points after burning. However, the overall OM amount significantly differed among the three fields. A decrease in OM was detected at 5 min after burning in all fallow fields. At 2 years after burning, in the RSC-4Y field, the OM had recovered, with levels above those measured before burning, whereas in the RSC-5Y and RSC-7Y fields, the OM levels did not return to the initial levels (Table 3).
In the RSC-5Y and RSC-7Y fields, the amount of organic carbon (OC) was lower at all time points after burning, whereas in the RSC-4Y field, at 2 years after burning, the OC had recovered. Similar to OM, there was no significant variation in OC at all time points after burning in the RSC-7Y field. The overall OC percentage of the RSC-5Y field was not significantly different from that of the RSC-4Y field (Table 3).
In the RSC-4Y and RSC-5Y fields, before burning, the electrical conductivity (ECe) was around 0.26 dS m−1. Significantly different values of ECe were observed at all time points in the RSC-4Y and RSC-5Y fields, whereas the changes in ECe were not remarkable at 5 min and 2 years after burning in the RSC-7Y field. Regarding the overall ECe, the RSC-5Y and RSC-7Y fields did not differ considerably. The ECe values were significantly increased after burning and then slightly declined. After 2 years, the ECe levels were still above the pre-burning values (Table 3).
The differences in the content of available phosphorus (available P) at the time points after burning in the RSC-4Y and RSC-5Y fields were not significant, whereas the overall available P levels in the RSC-5Y and RSC-7Y fields were similar. The largest increase in available potassium (available K) was observed at 9 months after burning in the RSC-4Y and RSC-5Y fields, whereas the available K had reached the highest level of 520.50 mg kg−1 in the RSC-7Y field 5 min after burning. The highest overall available calcium (available Ca) and magnesium (available Mg) levels were found in the RSC-4Y and RSC-7Y fields, with 1,774.34 and 364.42 mg kg−1, respectively. The levels of soil nutrients (available P, available K, and available Ca) tended to be higher at 5 min after burning for three fallow fields, except for available Mg, which remained at similar levels in the RSC-7Y field. At 2 years after burning, the levels of available P, K, and Ca were higher than before burning (Table 3).
Before burning, the TN contents did not significantly differ among the three fields and ranged from 0.38% to 0.41%. However, a significant decrease in TN was detected 5 min after burning. Although the contents of TN were increased at 2 years after burning, they did not reach the pre-burning levels (Table 3).
3.6 Soil physical properties
Only in the RSC-4Y field was there a decrease in bulk density 5 min after burning. The highest bulk density levels were observed in the RSC-4Y and RSC-5Y fields at 9 months after burning, whereas in the RSC-7Y field, bulk density was not significantly different 5 min after burning. Overall, bulk density differed significantly among the three fields. The most significant difference in bulk density between pre-burning and 5 min after burning was observed in the RSC-5Y field (Table 4) due to the significant loss of OM.
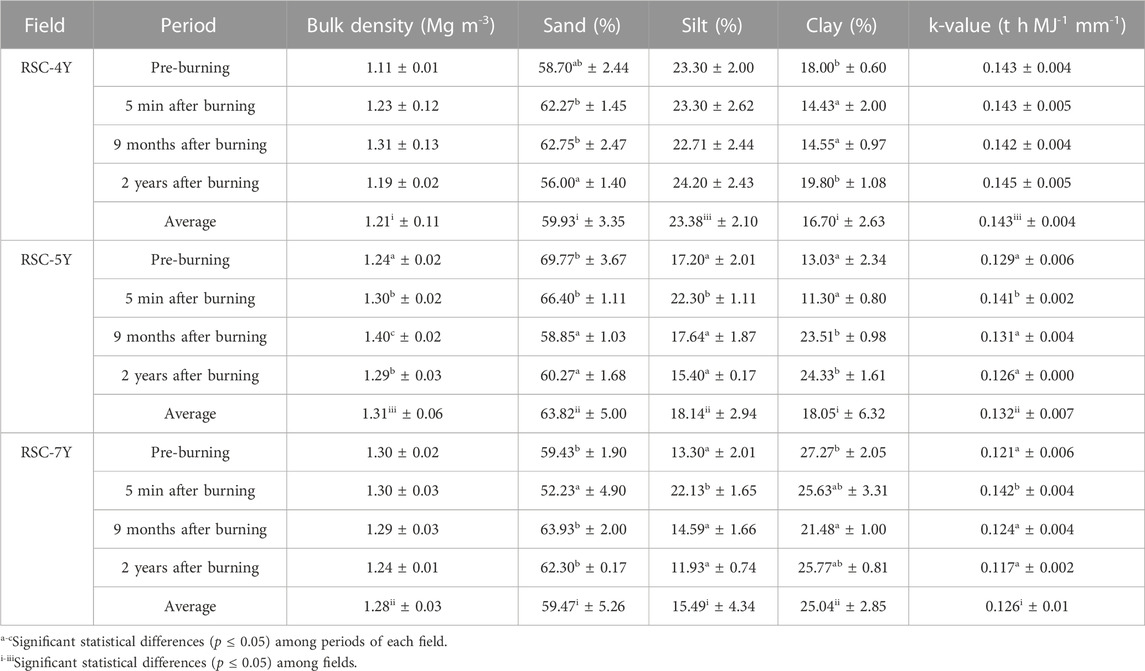
TABLE 4. Variations in soil physical properties: bulk density (Mg m−3), proportions of sand, silt, and clay (%), and k-value (t h MJ−1 mm−1) along the fallow chronosequence.
The proportion of sand in the soil of the RSC-4Y field was 62.27% and 62.75% higher at 5 min and 9 months after burning, respectively, whereas it was 56.00% lower at 2 years after burning. In the RSC-5Y field, the content of sand was lower at all time points after burning. On the other hand, sand was most prevalent in the RSC-7Y field, with 63.93% at 9 months after burning, although it had decreased by 52.23% 5 min after burning (Table 4). Although there was no significant change in the proportion of silt in the RSC-4Y field 5 min after burning, we observed remarkable changes in the RSC-5Y and RSC-7Y fields. The highest overall proportion of silt was observed in the RSC-4Y field, with 23.38%. Notably, the overall proportion of silt in the soil of all three fields varied significantly (Table 4). The contents of clay in the soil of the RSC-4Y and RSC-5Y fields were highest at 2 years after burning, whereas the amount of clay in the soil of the RSC-7Y field decreased at all time points after burning compared to pre-burning. There was no significant difference in the overall clay content between the RSC-4Y and RSC-5Y fields. The effect of fire on soil texture was detected 5 min after burning. The clay content significantly decreased from 18.0% to 14.43% in the RSC-4Y field, while the sand content significantly decreased from 59.43% to 52.23% in the RSC-7Y field. In the RSC-5Y and RSC-7Y fields, the proportion of silt was significantly increased (Table 4).
3.7 Soil erodibility
The overall soil erodibility (k-value) differed significantly among the three fields. The largest overall k-value was found in RSC-4Y, with 0.143 t h MJ−1 mm−1, whereas the k-value in the RSC-7Y field was lowest at 0.126 t h MJ−1 mm−1 (Table 4). This indicates that the soil in the RSC-4Y field was more susceptible to erosion than that in the other fields.
There were no significant differences in the k-value among the different time points within the same field. In the RSC-5Y field, the k-value was significantly higher at 5 min after burning, with 0.143 t h MJ−1 mm−1, followed by a gradual decrease. Likewise, a similar trend of the variation in the k-value was observed for the RSC-7Y field (Figure 4). This indicates that the soils were more susceptible to erosion after than before burning.
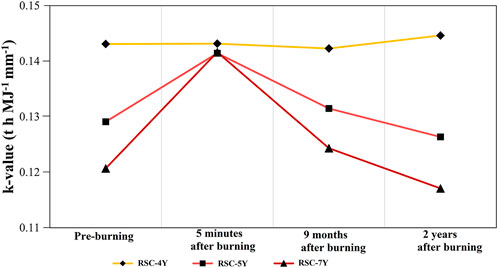
FIGURE 4. k-values (t h MJ−1 mm−1) before burning and at 5 min, 9 months, and 2 years after burning in three fallow fields.
3.8 Soil property response to fallow years and burning time
The RDA plot shows the relationships between soil variables and the different time points (Figures 5, 6). Since a significant correlation was found for ECe vs. N (r = −0.72, p < 0.05) and %silt vs. k-value (r = 1.00, p < 0.05), ECe and %silt were eliminated from the RDA. Soil variables with VIF >10, %clay, %sand, and bulk density were also eliminated.
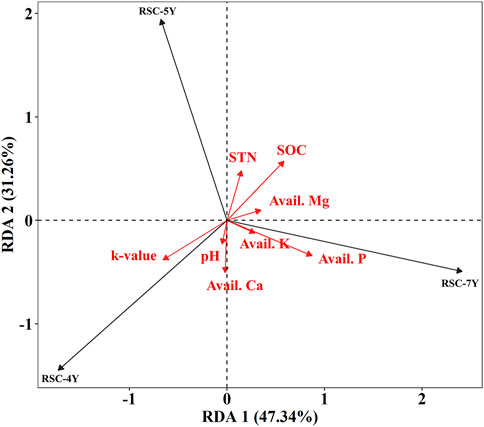
FIGURE 5. Redundancy analysis (RDA) of soil properties response variables and quantitative explanatory variables under rotational shifting cultivation for three fallow fields.
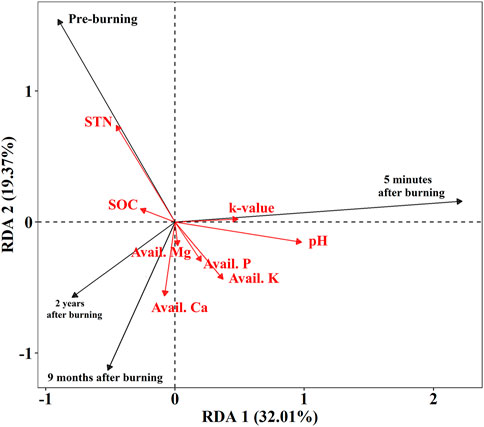
FIGURE 6. Redundancy analysis (RDA) of soil properties response variables and quantitative explanatory variables under rotational shifting cultivation before burning and at 5 min, 9 months, and 2 years after burning for three fallow fields.
As seen in Figure 5, two groups of samples are separated along the first axis (47.34%, p = 0.001), whereas the second axis (31.26%, p = 0.001) separates the samples according to the fallow chronosequence (adjusted R2 = 0.73). The RSC-4Y has a high positive correlation to k-value, pH, and available Ca; whereas STN and SOC show their higher effect in the RSC-5Y field. The available P, available K, and available Mg have a positive correlation to the RSC-7Y field.
In Figure 6, the RDA plot separates the first axis (32.01%, p = 0.001) and the second axis (19.37%, p = 0.012) into different time points (adjusted R2 = 0.44). STN and SOC show a significant relationship to pre-burning soils, while k-value and pH have a higher effect on the 5 min after burning soil. At 9 months after burning, the soils were more characterized by available C and available Mg and had a smaller relationship with available P and available K. At 2 years after burning, both available Ca and SOC have a higher positive relationship with the soils, among other properties.
4 Discussion
4.1 Recovery of SOC, STN, and soil nutrients after fire in rotational shifting cultivation
Fire alters soil properties due to heat and the formation of ashes. In the present study, the soil pH and ECe values were significantly increased after burning, followed by a slight decline until 2 years; the levels remained above the pre-burning values due to the incorporation of ashes and chars into the soil (Table 3). Previous studies (Ulery et al., 1993; Mataix-Solera et al., 2002; Muñoz-Rojas et al., 2016) also stated that the increases in soil pH and ECe after a fire are due to the burning of vegetation residues in the litter layer, generating ashes and resulting in the accumulation of ions, minerals, carbon, and other nutrients. However, the values of soil pH and ECe could be gradually decreased and returned to the pre-burning values due to soil leaching and runoff (Mataix- Solera et al., 2002; Lucas-Borja et al., 2020). Studies also detected high pH levels after burning for up to 6 months, followed by a return to the initial values after 36 months (Fonseca et al., 2017) or 25 months in a Mediterranean forest in southwestern Spain (Jiménez-González et al., 2016). In a study in the Sonoran Desert creosote bush scrub, at 21 years after burning, the pH was still considerably higher compared to pre-burning (Allen et al., 2011).
Soil texture is not easily affected by fire because sand, silt, and clay have high-temperature thresholds (Alcañiz et al., 2016). Sand and silt (1,414°C) have a higher temperature threshold than clay (400°C–800°C) (Neary et al., 2005). Thus, clay particles are more affected by fire when compared to sand and silt particles. As shown in Table 4, the increase in sand and silt particles after a fire is due to the collapse of clay particles, which is consistent with the study of Granged et al. (2011).
The OM levels in the RSC-5Y and RSC-7Y fields were higher than that in the RSC-4Y field before burning, which is due to the long fallow period. However, a decrease in OM was detected after burning at all fallow fields (Table 3). Neary et al. (1999) reported that the OM is mainly accumulated at the surface layer, where it is exposed to heat during burning, resulting in its combustion. Fire temperatures of 200°C–315°C can cause OM loss (Knicker, 2007). As shown in Table 3, OM recovery was observed in the RSC-4Y field, with higher levels after than before burning, whereas the OM in the RSC-5Y and RSC-7Y fields had not returned to the initial levels at 2 years after burning. This can be explained by the presence of grasses covering the surface soil in the RSC-4Y field, compared to the RSC-5Y and RSC-7Y fields, where trees were more common. After the fire, some shoots and roots of grasses in the RSC-4Y field showed rapid regrowth due to the available nutrients (available P, available K, available Ca, and available Mg) from ashes, which increased the OM inputs.
Before burning, the highest SOC and STN stocks were found in the RSC-7Y field (Figures 2, 3), indicating that the long fallow period can accumulate OM inputs from litter and roots, which is consistent with previous findings (Sarkar et al., 2015; Arunrat et al., 2021). Fire can alter soil carbon and nitrogen dynamics by changing carbon and nitrogen inputs and outputs, consequently altering SOC and STN stocks. During fires, some parts of aboveground biomass are incompletely combusted and transformed into pyrogenic carbon, which is resistant to degradation, whereas the aliphatic components are lost (Czimczik et al., 2002; Kasin et al., 2013). The SOC and STN stocks significantly declined at 5 min after burning in all fallow fields (Figures 2, 3) due to losses via volatilization by converting OM into mineral carbon and nitrogen (Nigussie and Kissi, 2011; Zavala et al., 2014). Unlike other soil nutrients (P, K, Ca, and Mg), carbon and nitrogen can be volatilized at lower temperatures (Knicker, 2007) and are thus dramatically lower after fire. For example, Neary et al. (1999) reported that nitrogen can be volatilized at 200°C, whereas Ca volatilization starts at 1,240°C. However, the accumulation of ashes in soil pore spaces can restrict water infiltration and increase surface runoff, leading to the leaching out of soil nutrients, which can explain the observed losses.
Changes in soil chemical and physical properties after fire alter the soil microbial community, thereby influencing the recovery of soil properties and the regrowth of vegetation. In the present study, the native successional and surviving plant species in the RSC fields were mostly grasses rather than woody shrubs, and their root systems may contribute to the recovery of ecosystem processes. Brockway and Lewis (1997) and Rasse et al. (2005) reported that early successional native grasses can facilitate the recovery of SOC after a fire and contribute to STN recovery by native nitrogen-fixing plants (Newland and DeLuca, 2000). Moreover, Johnson et al. (2004) reported that nitrogen can rapidly return to pre-fire levels when nitrogen-fixing plants appear. As presented in Figures 1, 2, 2 years of recovery in RSC fields were not long enough to restore the SOC and STN stocks. This is consistent with the study of Santorufo et al. (2021), who found a similar result in Mediterranean Andosol properties 2 years after fire. Moreover, the OM level was not recovered even 10 years after a wildfire in the semi-arid Zagros woodlands (Sadeghifar et al., 2020) and 6 years after a fire in the Mediterranean shrubland (Girona-García et al., 2019). In another study, the total carbon and nitrogen levels in a pine plantation (Pinus halepensis Miller) in Catalonia, Spain, could not be restored to the initial levels 9 years after the prescribed fire (Alcañiz et al., 2016), most likely because the recovery of SOC, STN, and soil nutrients (available P, K, Ca, and Mg) starts with vegetation restoration, especially grass species. The results of the redundancy analysis (Figure 6) also evidenced higher pH, available K, available P, and available Mg levels 5 min after burning. This was the case for the RSC-4Y field, where the SOC stock was slightly increased after the fire (Figure 2) due to the survival of weeds and grasses and because of the increased nutrient levels from ashes and charcoal. Quintero-Gradilla et al. (2015) reported the rapid recovery of carbon, nitrogen, and phosphorus in a Pinus douglasiana stand in central-western Mexico after severe fires. The authors explained this by the impact of climate and soil conditions on vegetation growth, resulting in considerable OM inputs and accumulation. Post and Kwon (2000) also reported that the recovery of SOC and STN stocks after a fire depends on the OM input, climate, soil type, plant species, nutrients available, and plant species.
4.2 Post-fire soil erodibility changes in rotational shifting cultivation
Since the vegetation is cut and burnt in the RSC field, soil degradation, erosion, and nutrient leaching frequently occur. Soil erodibility is widely used to describe the sensitivity of soil particles to detachment and transport by heavy rainfall and runoff (Wischmeier and Smith, 1978). According to Zhu et al. (2010), soil organic matter can influence the k-value due to its ability to absorb water and increase soil infiltration. As shown in Figure 4, the topsoil of the RSC-4Y field before burning was most susceptible to erosion because of the lower organic carbon (Table 3) and higher silt content (Table 4) compared with the RSC-5Y and RSC-7Y fields. At 5 min after burning, the k-values in the RSC-5Y and RSC-7Y fields were remarkably higher than before burning. This indicates an increase in erosive processes through the reduction of organic carbon (Table 3) and inputs from vegetation and an increase in silt contents after a fire (Table 4). According to Ostovari et al. (2016), fine soil particles lack adhesion properties and are easily broken and transported, whereas soil with coarse particles has a high permeability, impeding soil erosion. On the other hand, the k-value in the RSC-4Y field was unchanged, as the fire did not alter the organic carbon (Table 3) and silt contents (Table 3). We hypothesized that weeds and grasses may protect the OM in the surface layer from fire and that they regrow after a fire, in contrast to the trees. This was observed at 9 months and 2 years after burning; in the RSC-4Y field, the k-values were unchanged, most likely because of the survival and regrowth of weeds and grasses, slowing down runoff and leaching. In contrast, the RSC-5Y and RSC-7Y fields showed a slight increase in soil erosion resistance (reduced k-values) after burning compared to the pre-burning values, which can be explained by the increase in the clay content via the decomposition of new litter inputs and the reduction of the silt content through surface runoff. This is consistent with the study by Zhang et al. (2004), who also found a significant negative correlation between clay content and k-value when using the RUSLE model. Zhang et al. (2011) mentioned that the lack of grasses and litter under the canopy results in low water retention capacity, whereas the roots of grasses can potentially enhance the carbon input and reduce land-surface erodibility (Fu et al., 2011; Arunrat et al., 2022b). Garcia-Ruiz et al. (2015), in their meta-analysis, showed that agricultural lands have the highest erosion rates, whereas the lowest rates are found in forests and shrublands.
Ash and charcoal play important roles in post-fire soil. It is a consequence of the solubilization components that not only alter soil physio-chemical properties but also increase or decrease soil erosion and deposition. In the RSC fields, the ashes were reddish-brown, gray, and white (Table 2). Gray and white ashes are produced under complete combustion at temperatures of 500–1,400°C (Goforth et al., 2005) and are usually on top of black ash, whereas dark brown ash is produced through the oxidation and dehydration of Fe (Bodí et al., 2014). In contrast, combustion under low temperatures produces ash with a higher OC content and a dark color (Kuhlbusch and Crutzen, 1995). Before the first rain, the colors of ash can affect the soil’s thermal properties. Bodí et al. (2014) reported that darkened ash may increase soil temperatures by decreasing soil albedo. After the rain, the soil pores can be sealed by ash, charcoal, and clay minerals and become clogged (Mills and Fey, 2004), leading to changes in runoff and erosion (Onda et al., 2008; Woods and Balfour, 2010). Dark ash acts as a mulch cover by protecting the topsoil from raindrops, detachment, and increasing water infiltration, whereas white and gray ashes may increase overland flow due to the clogging of soil pores (Thomaz, 2018).
4.3 Implications for post-fire land management
Ash plays a crucial role in the altering and recovery of soil physio-chemical properties, whereas soil erodibility controls soil properties after a fire (Figure 6). Based on the present study, three possible effective practices for post-fire land management in the RSC fields are recommended. The first is allowing weed and grass growth before burning to reduce soil heat; the survival of shoots and roots of grasses and weeds can facilitate rapid regrowth, supporting soil recovery (Kirchmann et al., 2013; Arunrat et al., 2022c) (as seen in the RSC-4Y field; Table 3; Figures 1, 2). The second is burning while fuels contain high moisture levels, namely, in late winter or early summer (November–February), to impede the complete combustion of the topsoil layer; incomplete combustion produces dark ash and charcoal, which may reduce soil detachment and increase soil pyrogenic carbon. It should be noted that smoldering fires burn for longer periods, resulting in more smog compared with flaming fires (Rein et al., 2008; Arunrat et al., 2022a). Therefore, the burning schedule and cultivation calendar should be well planned based on weather conditions, especially precipitation. Finally, the trunks remaining after a fire can be used as contour-felled log erosion barriers to slow down surface runoff and trap the sediment, ash, charcoal, and nutrients (Robichaud et al., 2008) that can promote crop growth and soil recovery. However, it is necessary to develop appropriate post-fire land management practices and to study the effect of ash characteristics on soil erosion in RSC fields.
5 Conclusion
Soil pH, ECe, and soil nutrients (available P, available K, and available Ca) were increased after burning and were above the pre-burning levels at 2 years after burning, mostly because of the incorporation of ash and charcoal into the soil. The SOC stock decreased after burning in all fallow fields. At 2 years after burning, in the RSC-4Y field, the SOC stock was higher than before burning, whereas in the RSC-5Y and RSC-7Y fields, the levels were 5.9% and 13.1% lower than the pre-burning levels. Weeds and grasses were more prominent in the RSC-4Y field, and their regrowth after fire promoted OM inputs. The STN stocks of all studied fields were significantly decreased after burning (30.0%, on average), followed by an increase up to 9 months; however, at 2 years after burning, they were still lower than the pre-burning stocks (17.1% decrease, on average). These losses may be due to the sealing of soil pores by ash, charcoal, and clay minerals, impeding water infiltration and increasing surface runoff. Although before burning, the topsoil of the RSC-4Y field was most susceptible to erosion because of the lower organic carbon and higher silt contents compared with the RSC-5Y and RSC-7Y fields, the k-value in the RSC-4Y field was unchanged at 2 years after burning. This suggests that weeds and grasses protect the surface soil from fire and their regrowth after burning decreases runoff and leaching.
Data availability statement
The original contributions presented in the study are included in the article/Supplementary Material; further inquiries can be directed to the corresponding author.
Author contributions
NA: conceptualization, methodology, investigation, writing—original draft, and writing—review and editing. SS: conceptualization, methodology, investigation, and writing—original draft. PK: methodology, investigation, and writing—original draft. CI and MY: supervision. RH: conceptualization, methodology, and supervision.
Funding
This work was supported by the Office of the Permanent Secretary, Ministry of Higher Education, Science, Research, and Innovation (OPS MHESI), Thailand Science Research and Innovation (TSRI) and Mahidol University (Grant No. RGNS 64-153). The study was conducted according to the guidelines of the Declaration of Helsinki and approved by the Institutional Review Board of Institute for Population and Social Research, Mahidol University (IPSR-IRB) (COA. No. 2019/05-154) and (COA. No. 2022/03-059).
Conflict of interest
The authors declare that the research was conducted in the absence of any commercial or financial relationships that could be construed as a potential conflict of interest.
Publisher’s note
All claims expressed in this article are solely those of the authors and do not necessarily represent those of their affiliated organizations, or those of the publisher, the editors, and the reviewers. Any product that may be evaluated in this article, or claim that may be made by its manufacturer, is not guaranteed or endorsed by the publisher.
References
AIPP and IWGIA (2014). Shifting cultivation, livelihood and food security: New and old challenges for indigenous peoples in Asia in Food and agriculture organisation of the united nations (Chiang Mai, Thailand: AIPP Printing Press).
Alcañiz, M., Outeiro, L., Francos, M., Farguell, J., and Úbeda, X. (2016). Long-term dynamics of soil chemical properties after a prescribed fire in a Mediterranean forest (Montgrí massif, Catalonia, Spain). Sci. Total Environ. 572, 1329–1335. doi:10.1016/j.scitotenv.2016.01.115
Allen, E. B., Steers, R. J., and Dickens, S. J. (2011). Impacts of fire and invasive species on desert soil ecology. Rangel. Ecol. Manag. 64, 450–462. doi:10.2111/rem-d-09-00159.1
Arunrat, N., Sereenonchai, S., and Hatano, R. (2022a). Effects of fire on soil organic carbon, soil total nitrogen, and soil properties under rotational shifting cultivation in northern Thailand. J. Environ. Manag. 302, 113978. doi:10.1016/j.jenvman.2021.113978
Arunrat, N., Sereenonchai, S., and Hatano, R. (2021). Impact of burning on soil organic carbon ofmaize-upland rice system in Mae Chaem Basin of Northern Thailand. Geoderma 392, 115002. doi:10.1016/j.geoderma.2021.115002
Arunrat, N., Sereenonchai, S., Kongsurakan, P., and Hatano, R. (2022b). Assessing soil organic carbon, soil nutrients and soil erodibility under terraced paddy fields and upland rice in northern Thailand. Agronomy 12 (2), 537. doi:10.3390/agronomy12020537
Arunrat, N., Sereenonchai, S., Kongsurakan, P., and Hatano, R. (2022c). Soil organic carbon and soil erodibility response to various land-use changes in northern Thailand. Catena 219, 106595. doi:10.1016/j.catena.2022.106595
Bodí, M., Martin, D. A., Santín, C., Balfour, V., Doerr, S. H., Pereira, P., et al. (2014). Wildland fire ash: Production, composition and eco-hydro-geomorphic effects. Earth Sci. Rev., 130, 103–127. doi:10.1016/j.earscirev.2013.12.007
Bray, R. A., and Kurtz, L. T. (1945). Determination of total organic and available form of phosphorus in soil. Soil Sci. 59, 39–45. doi:10.1097/00010694-194501000-00006
Brockway, D. G., and Lewis, C. E. (1997). Long-term effects of dormant-season prescribed fire on plant community diversity, structure and productivity in a longleaf pine wiregrass ecosystem. For. Ecol. Manag. 96, 167–183. doi:10.1016/s0378-1127(96)03939-4
Carroll, E. M., Miller, W. W., Johnson, D. W., Saito, L., Qualls, R. G., and Walker, R. F. (2007).Spatial analysis of a large magnitude erosion event following a Sierran Wildfire. J. Environ. Qual. 36, 1105. doi:10.2134/jeq2006.0466
Catón, M., Morales, M. S., Whitworth-Hulse, J. I., Zeballos, S. R., Giorgis, M. A., Rodriguez Caton, M., et al. (2019). A 55-year-old natural experiment gives evidence of the effects of changes in fire frequency on ecosystem properties in a seasonal subtropical dry forest. Land Degrad. Dev. 30, 266–277. doi:10.1002/ldr.3219
Cawson, J. G., Sheridan, G. J., Smith, H. G., and Lane, P. N. J. (2012). Surface runoff and erosion after prescribed burning and the effect of different fire regimes in forests and shrublands: A review. Int. J. Wildland Fire. 21, 857–872. doi:10.1071/wf11160
Certini, G. (2005). Effects of fire on properties of forest soils: A review. Oecologia 143, 1–10. doi:10.1007/s00442-004-1788-8
Chen, J., He, B., Wang, X., Ma, Y., and Xi, W. (2013). The effects of Herba Andrographitis hedgerows on soil erodibility and fractal features on sloping cropland in the Three Gorges Reservoir Area. Environ. Sci. Pollut. Res. 20, 7063–7070. doi:10.1007/s11356-013-1704-z
Czimczik, C. I., Preston, C. M., Schmidt, M. W., Werner, R. A., and Schulze, E. D. (2002). Effects of charring on mass, organic carbon, and stable carbon isotope composition of wood. Org. Geochem. 33, 1207–1223. doi:10.1016/s0146-6380(02)00137-7
DeBano, L. F., Neary, D. G., and Ffolliott, P. F. (1998). Fire’s effects on ecosystems. New York: John Wiley & Sons, 71–80.
Deng, L., Kim, D.-G., Peng, C., and Shangguan, Z. (2018). Controls of soil and aggregate-associated organic carbon variations following natural vegetation restoration on the Loess Plateau in China. Land Degrad. Dev. 29, 3974–3984. doi:10.1002/ldr.3142
Department of Mineral Resource (2015). Landslide Harzard Map: Mae Thab Subdistrict, Mae Chaem District, Chiang Mai, Thailand (in Thai). Bangkok, Thailand
Ellert, B. H., and Bettany, J. R. (1995). Calculation of organic matter and nutrients stored in soils under contrasting management regimes. Can. J. Soil Sci. 75, 529–538. doi:10.4141/cjss95-075
Emadi, M., Baghernejad, M., and Memarian, H. R. (2009). Effect of land-use change on soil fertility characteristics within water-stable aggregates of two cultivated soils in northern Iran. Land Use Policy 26, 452–457. doi:10.1016/j.landusepol.2008.06.001
Flannigan, M. D., Krawchuk, M. A., De Groot, W. J., Wotton, B. M., and Gowman, L. M. (2009).Implications of changing climate for global wildland fire. Int. J. Wildland Fire, 18, 483–507. doi:10.1071/WF08187
Fonseca, F., de Figueiredo, T., Nogueira, C., and Queirós, A. (2017). Effect of prescribed fire on soil properties and soil erosion in a Mediterranean mountain area. Geoderma 307, 172–180. doi:10.1016/j.geoderma.2017.06.018
Francos, M., Úbeda, X, Francos, M., and Úbeda, X. (2021). Prescribed fire management. Curr. Opin. Environ. Sci. Health. 21, 100250. doi:10.1016/j.coesh.2021.100250
Fu, B. J., Liu, Y., Lü, Y. H., He, C. S., Zeng, Y., and Wu, B. F. (2011). Assessing the soil erosion control service of ecosystems change in the Loess Plateau of China. Ecol. Complex. 8, 284–293. doi:10.1016/j.ecocom.2011.07.003
Garcia-Ruiz, J. M., Begueria, S., Nadal-Romero, E., Gonzalez-Hidalgo, J. C., Lana-Renault, N., and Sanjuan, Y. (2015). A meta-analysis of soil erosion rates across the world. Geomorphology 239, 160–173. doi:10.1016/j.geomorph.2015.03.008
Giovannini, G., Lucchesi, S., and Giachetti, M. (1988). Effect of heating on some physical and chemical parameters related to soil aggregation and erodibility. Soil Sci. 146, 255–261. doi:10.1097/00010694-198810000-00006
Girona-García, A., Ortiz-Perpiñá, O., and Badía-Villas, D. (2019). Dynamics of topsoil carbon stocks after prescribed burning for pasture restoration in shrublands of the Central Pyrenees (NE-Spain). J. Environ. Manag. 233, 695–705. doi:10.1016/j.jenvman.2018.12.057
Goforth, B. R., Graham, R. C., Hubebrt, K. R., Zanner, C. W., and Minnich, R. A. (2005). Spatial distribution and properties of ash and thermally altered soils after high severity forest fire southern California. Int. J. Wildl. Fire. 14, 343–354. doi:10.1071/WF05038
Granged, A. J., Zavala, L. M., Jordán, A., and Bárcenas-Moreno, G. (2011). Post-fire evolution of soil properties and vegetation cover in a mediterranean heathland after experimental burning: A 3-year study. Geoderma 164 (1–2), 85–94. doi:10.1016/j.geoderma.2011.05.017
Heinimann, A., Mertz, O., Frolking, S., Christensen, A. E., Hurni, K., Sedano, F., et al. (2017). A global view of shifting cultivation: Recent, current, and future extent. PLoS One 12 (9), e0184479. doi:10.1371/journal.pone.0184479
Jiménez-González, M. A., De la Rosa, J. M., Jiménez-Morillo, N. T., Almendros-Martín, G., González-Pérez, J. A., and Knicker, H. (2016). Post-fire recovery of soil organic matter in a Cambisol from typical Mediterranean forest in Southwestern Spain. Sci. Total Environmen.t 572, 1414–1421. doi:10.1016/j.scitotenv.2016.02.134
Johnson, D., Susfalk, R., Caldwell, T., Murphy, J., Miller, W., and Walker, R. (2004). Fire effects on carbon and nitrogen budgets in forests. Water Air Soil Pollut. Focus 4, 263–275. doi:10.1023/b:wafo.0000028359.17442.d1
Jurvélius, M. (2004). “Forest fires (prediction, prevention, preparedness and suppression),” in Health and protection (Amsterdam, Netherlands: Elsevier). 334–339.
Kasin, I., Blanck, Y. L., Storaunet, K. O., Rolstad, J., and Ohlson, M. (2013). The charcoal record in peat and mineral soil across a boreal landscape and possible linkages to climate change and recent fire history. Holocene 23, 1052–1065. doi:10.1177/0959683613479678
Khanna, P. K., Raison, R. J., and Falkiner, R. A. (1994). Chemical properties of ash derived from Eucalyptus litter and its effects on Forest soils. For. Ecol. Manag. 66, 107–125. doi:10.1016/0378-1127(94)90151-1
Kirchmann, H., Börjesson, G., Schön, M., Hamnér, K., and Kätterer, T. (2013). Properties of soils in the Swedish long-term fertility experiments: VII. Changes in topsoil and upper subsoil at örja and fors after 50 years of nitrogen fertilization and manure application. Acta Agric. Scand. B 63, 25–36. doi:10.1080/09064710.2012.711352
Knicker, H. (2007). How does fire affect the nature and stability of soil organic nitrogen and carbon? A review. Biogeochemistry 85 (1), 91–118. doi:10.1007/s10533-007-9104-4
Kuhlbusch, T. A. J., and Crutzen, P. J. (1995). Toward a global estimate of black carbon in residues of vegetation fires representing a sink of atmospheric CO2and a source of O2fires representing a sink of atmospheric CO2 and source of O2. Glob.Biogeochem. Cycles. 9, 491–501. doi:10.1029/95gb02742
Kuzyakov, Y., Merino, A., and Pereira, P. (2018). Ash and fire, char, and biochar in the environment. Land Degrad. Dev. 29, 2040–2044. doi:10.1002/ldr.2979
Kyveryga, P. M., Blackmer, A. M., Ellsworth, J. W., and Isla, R. (2004). Soil pH effects on nitrification of fall-applied anhydrous ammonia. Soil Sci. Soc. Am. J. 68, 545–551. doi:10.2136/sssaj2004.0545
Larsen, I., Macdonald, L., Brown, E., Rough, D., Welsh, M., Pietraszek, J., et al. (2009). Causes of post-fire runoff and erosion: Water repellency, cover, or soil sealing? Soil Sci. Soc. Am. J. 73, 1393–1407. doi:10.2136/sssaj2007.0432
LDD (Land Development Department). (1992). Preliminary study on highland development project in northern Thailand. Bangkok: Soil Survey Division, Land Development Department, Bangkok, Thailand.
Liu, X., Zhang, Y., and Li, P. (2020). Spatial variation characteristics of soil erodibility in the yingwugou watershed of the middle dan river, China. Int. J. Environ. Res. Public Health. 17, 3568. doi:10.3390/ijerph17103568
Lucas-Borja, M. E., Delgado-Baquerizo, M., Muñoz-Rojas, M., Plaza-Álvarez, P. A., Gómez- Sanchez, M. E., González -Romero, J., et al. (2021). Changes in ecosystem properties after post-fire management strategies in wildfire-affected Mediterranean forests. J. Appl. Ecol. 58, 836–846. doi:10.1111/1365-2664.13819
Lucas-Borja, M. E., Ortega, R., Miralles, I., Plaza-Álvarez, P. A., González -Romero, J., Peña-Molina, E., et al. (2020). Effects of wildfire and logging on soil functionality in the short-term in Pinus halepensis M. forests. Eur. J. For. Res. 139, 935–945. doi:10.1007/s10342-020-01296-2
Lucas-Borja, M. E., Plaza-Álvarez, P. A., Gonzalez-Romero, J., Sagra, J., Alfaro-Sánchez, R., Zema, D. A., et al. (2019). Short-term effects of prescribed burning in Mediterranean pine plantations on surface runoff, soil erosion and water quality of runoff. Sci. Total Environ. 674, 615–622. doi:10.1016/j.scitotenv.2019.04.114
Mae Chaem Watershed Research Station (2010). Mae Chaem watershed. Available at: http://www.dnp.go.th/watershed/research/mj_station.htm.
Markl, G., Blackenburg, F. V., and Wagner, T. (2006). Iron isotope fractionation during hydrothermal ore deposition and alteration. Geochimica Cosmochimica Acta 70, 3011–3030. doi:10.1016/j.gca.2006.02.028
Mataix-Solera, J., Gómez, I., Navarro-Pedreño, J., Guerrero, C., and Moral, R. (2002). Soil organic matter and aggregates affected by wildfire in a Pinus halepensis forest in a Mediterranean environment. Int. J. Wildl. Fire. 11, 107–114.
Mills, A. J., and Fey, M. V. (2004). Frequent fires intensify soil crusting: Physicochemical feedback in the pedoderm of long-term burn experiments in south africafires intensify soil crusting: Physico-chemical feedback in the pedoderm of long-term burn experiments in south Africa. Geoderma 121, 45–64. doi:10.1016/j.geoderma.2003.10.004
Muñoz-Rojas, M., Erickson, T. E., Martini, D., Dixon, K. W., and Merritt, D. J. (2016). Soil physicochemical and microbiological indicators of short, medium and long term post-fire recovery in semi-arid ecosystems. Ecol. Indic. 63, 14–22. doi:10.1016/j.ecolind.2015.11.038
National Soil Survey Center. (1996). Soil Survey laboratory methods manual. Soil Survey investigations Report No. 42, Version 3.0, USDA. Washington, DC, USA.
Neary, D. G., Klopatek, C. C., DeBano, L. F., and Ffolliott, P. F. (1999). Fire effects on belowground sustainability: A review and synthesis. For. Ecol. Manage. 122, 51–71. doi:10.1016/s0378-1127(99)00032-8
Neary, D. G., Ryan, K. C., and DeBano, L. F. (2005). Wildland fire in ecosystems: Effects of fire on soils and water. General Tech. Rep. RMRSGTR-42- 4, 250. doi:10.2737/RMRS-GTR-42-V4
Newland, J. A., and DeLuca, T. H. (2000). Influence of fire on native nitrogen-fixing plants, and soil nitrogen status in ponderosa pine - douglas-fir forests in Western Montana. Can. J. For. Res. 30, 274–282. doi:10.1139/x99-206
Nigussie, A., and Kissi, E. (2011). Impact of biomass burning on physicochemical properties of nitisol in the southwestern Ethiopia. Asian J. Agric. Res. 5 (4), 223–233. doi:10.3923/ajar.2011.223.233
Nolan, R. H., Collins, L., Leigh, A., Ooi, M. K. J., Curran, T. J., Fairman, T. A., et al. (2021). Limits to post-fire vegetation recovery under climate change. Plant, Cell Environ. 44 (11), 3471–3489. doi:10.1111/pce.14176
Onda, Y., Dietrich, W. E., and Booker, F. (2008). Evolution of overland flow after a severe forest fire, Point Reyes, California. Catena 72, 13–20. doi:10.1016/j.catena.2007.02.003
Ostovari, Y., Ghorbani-Dashtaki, S., Bahrami, H.-A., Naderi, M., Dematte, J. A. M., and Kerry, R. (2016). Modification of the USLE K factor for soil erodibility assessment on calcareous soils in Iranfication of the USLE K factor for soil erodibility assessment on calcareous soils in Iran. Geomorphology 273, 385–395. doi:10.1016/j.geomorph.2016.08.003
Pellegrini, A. F. A., Ahlström, A., Hobbie, S. E., Reich, P. B., Nieradzik, L. P., Staver, A. C., et al. (2018). Fire frequency drives decadal changes in soil carbon and nitrogen and ecosystem productivity. Nature. 553, 194–198. doi:10.1038/nature24668
Phillips, D. H., Foss, J. E., Buckner, E. R., Evans, R. M., and FitzPatrick, E. A. (2000). Response of surface horizons in an oak forest to prescribed burning. Soil Sci. Soc. Am. J. 64, 754–760. doi:10.2136/sssaj2000.642754x
Pierson, D. N., Robichaud, P. R., Rhoades, C. C., and Brown, R. E. (2019). Soil carbon and nitrogen eroded after severe wildfire and erosion mitigation treatments. Int. J. Wildland Fire 28, 814–821. doi:10.1071/wf18193
Post, W. M., and Kwon, W. M. (2000). Soil carbon sequestration and land-use change: Processes and potential. Glob. Change Biol. 6, 317–327. doi:10.1046/j.1365-2486.2000.00308.x
Quintero-Gradilla, S. D., García-Oliva, F., Cuevas-Guzmán, R., Jardel-Peláez, E. J., and Martínez-Yrizar, A. (2015). Soil carbon and nutrient recovery after high-severity wildfire in Mexico. Fire Ecol. 11, 45–61. doi:10.4996/fireecology.1103045
Rasse, D. P., Rumpel, C., and Dignac, M-F. (2005). Is soil carbon mostly root carbon? Mechanisms for a specific stabilisation. Plant Soil. 269, 341–356. doi:10.1007/s11104-004-0907-y
Rein, G., Garcia, J., Simeoni, A., Tihay, V., and Ferrat, L. (2008). Smouldering natural fires: Comparison of burning dynamics in boreal peat and mediterranean humus. WIT Trans. Ecol. Environ. 119, 183–192.
Renard, K. G., Foster, G., Weesies, G., McCool, D., and Yoder, D. (1997). Predicting soil erosion by water: A guide to conservation planning with the revised universal soil loss equation (RUSLE) (agricultural handbook 703). US Department of Agriculture, Washington, DC.
Renard, K. G., Laflen, J. M., Foster, G. R., and McCool, D. K. (2017). The revised universal soil loss equation. Soil Eros. Res. Methods 55, 352.
Rerkasem, K., and Rerkasem, B. (1994). Shifting cultivation in Thailand: Its current situation and dynamics in the context of highland development. London, UK: International Institute for Environment and Development.
Robichaud, P. R., Beyers, J. L., and Neary, D. G. (2000). Evaluating the effectiveness of postfire rehabilitation treatments. Gen. Tech. Rep. RMRS-GTR-63. U.S.Department of Agriculture, Forest Service, Rocky Mountain Research Station, Fort Collins, 85
Robichaud, P. R., Wagenbrenner, J. W., Brown, R. E., Wohlgemuth, P. M., and Beyers, J. L. (2008). Evaluating the effectiveness of contour-felled log erosion barriers as a post-fire runoff and erosion mitigation treatment in the Western United States. Int. J. Wildland Fire. 17, 255–273. doi:10.1071/WF07032
Sadeghifar, M., Agha, A. B. A., and Pourreza, M. (2020). Comparing soil microbial eco-physiological and enzymatic response to fire in the semi-arid Zagros woodlands. Appl. Soil Ecol. 147, 103366. doi:10.1016/j.apsoil.2019.103366
Santorufo, L., Memoli, V., Panico, S. C., Santini, G., Barile, R., Di Natale, G., et al. (2021). Early post-fire changes in properties of Andosols within a Mediterranean area. Geoderma 394, 115016. doi:10.1016/j.geoderma.2021.115016
Sarkar, D., Meitei, Ch.B., Baishya, L. K., Das, A., Ghosh, S., Chongloi, K. L., et al. (2015). Potential of fallow chronosequence in shifting cultivation to conserve soil organic carbon in northeast India. Catena. 135, 321–327. doi:10.1016/j.catena.2015.08.012
Shirazi, M. A., and Boersma, L. (1984). A unifying quantitative analysis of soil texture. Soil Sci. Soc. Am. J. 48, 142–147. doi:10.2136/sssaj1984.03615995004800010026x
Sparks, D. L., Page, A. L., Helmke, P. A., Loeppert, R. H., Soltanpour, P. N., Tabatabai, M. A., et al. (1996). Methods of soil analysis—Part 3: Chemical methods. Madison: Soil Science Society of America.
Thomas, G. W. (1996). “Soil pH and soil acidity,” in Method of soil analysis, part 3: Chemical methods. Editors Sparks, D. L., Page, A. L., Helmke, P. A., Loeppert, R. H., Soltanpour, P. N., and Tabatabai, M. A. (Madison, WI: SSSA Inc.), 475–490.
Thomaz, E. L. (2018). Ash physical characteristics affects differently soil hydrology and erosion subprocesses. Land Degrad. Dev. 29, 690–700. doi:10.1002/ldr.2715
Úbeda, X., Pereira, P., Outeiro, L., and Martin, D. A. (2009). Effects of fire temperature on the physical and chemical characteristics of the ash from two plots of cork oak (Quercus suber). Land Degrad. Dev. 20, 589–608. doi:10.1002/ldr.930
Ulery, A., Graham, R., and Amrhein, C. (1993). Wood-ash composition and soil pH following intense burning. Soil Sci. 156 (5), 358–364. doi:10.1097/00010694-199311000-00008
USDA. (1954). Diagnosis and improvement of saline and alkali soils, agriculture. Handbook No. 60, U.S. Salinity Laboratory. Government Printing Office, Washington, DC.
Vieira, D. C. S., Fernández, C., Vega, J. A., and Keizer, J. J. (2015). Does soil burn severity affect the post-fire runoff and interrill erosion response? A review based on meta-analysis of field rainfall simulation data. J. Hydrology 523, 452–464. doi:10.1016/j.jhydrol.2015.01.071
Walkley, A., and Black, J. A. (1934). An examination of the dichormate method for determining soil organic matter and a proposed modification of the chromic acid titration method. Soil Sci. 37, 29–32. doi:10.1097/00010694-193401000-00003
Williams, J., Renard, K., and Dyke, P. (1983). Epic: A new method for assessing erosion’s effect on soil productivity. J. Soil Water Conserv. 38 (5), 381–383.
Wischmeier, W. H., Johnson, C. B., and Cross, B. V. (1971). A soil erodibility nomograph for farmland and construction sites. J. soil water conservation 26, 189–193.
Wischmeier, W. H., and Smith, D. D. (1965). Predicting rainfall erosion losses from cropland east of the rocky mountains: Guide for selection of practices for soil and water conservation planning. Washington, D.C: USDA Agriculture Handbook. US Government Printing Office.
Wischmeier, W. H., and Smith, D. D. (1978). “Predicting rainfall erosion losses: A guide to conservation planning,” in Agriculture handbook; No. 537 (Washington, DC, USA: US Department of Agriculture Science and Education Administration), 1–69.
Woods, S. W., and Balfour, V. N. (2010). The effects of soil texture and ash thickness on the post-fire hydrological response from ash-covered soils. J. Hydrology 393 (3-4), 274–286. doi:10.1016/j.jhydrol.2010.08.025
Wu, L., Long, T.-Y., Liu, X., and Mmereki, D. (2012). Simulation of soil loss processes based on rainfall runoff and the time factor of governance in the Jialing River Watershed, China. Environ. Monit. Assess. 184, 3731–3748. doi:10.1007/s10661-011-2220-6
Zavala, L. M., de Celis, R., and Jordán, A. (2014). How wildfires affect soil properties. A Brief Review. Cuad. Investig. Geográfica. 40, 311–331. doi:10.18172/cig.2522
Zhang, J., DeAngelis, D., and Zhuang, J. (2011). “Ecological and environmental characteristics in the hilly region of middle and lower yangtze river,” in Theory and practice of soil loss control in eastern China (New York, NY: Springer).
Zhang, K., Li, S., Peng, W., and Yu, B. (2004). Erodibility of agricultural soils on the loess plateau of China. Soil Tillage Res. 76(2), 157–165. doi:10.1016/j.still.2003.09.007
Zhang, X., Zhao, W., Wang, L., Liu, Y., Feng, Q., Fang, X., et al. (2018). Distribution of shrubland and grassland soil erodibility on the Loess Plateau. Int. J. Environ. Res. Public Health 15, 1193. doi:10.3390/ijerph15061193
Zhu, B., Li, Z., Li, P., Liu, G., and Xue, S. (2010). Soil erodibility, microbial biomass, and physical–chemical property changes during long-term natural vegetation restoration: A case study in the loess plateau, China. Ecol. Res. 25, 531–541. doi:10.1007/s11284-009-0683-5
Keywords: hill tribes, fire, soil recovery, soil properties, post-fire land management
Citation: Arunrat N, Sereenonchai S, Kongsurakan P, Iwai CB, Yuttitham M and Hatano R (2023) Post-fire recovery of soil organic carbon, soil total nitrogen, soil nutrients, and soil erodibility in rotational shifting cultivation in Northern Thailand. Front. Environ. Sci. 11:1117427. doi: 10.3389/fenvs.2023.1117427
Received: 06 December 2022; Accepted: 14 March 2023;
Published: 27 March 2023.
Edited by:
Wakene Negassa, University of Copenhagen, DenmarkReviewed by:
Yu Liu, Northwest A&F University, ChinaZhengan Su, Institute of Mountain Hazards and Environment (CAS), China
Copyright © 2023 Arunrat, Sereenonchai, Kongsurakan, Iwai, Yuttitham and Hatano. This is an open-access article distributed under the terms of the Creative Commons Attribution License (CC BY). The use, distribution or reproduction in other forums is permitted, provided the original author(s) and the copyright owner(s) are credited and that the original publication in this journal is cited, in accordance with accepted academic practice. No use, distribution or reproduction is permitted which does not comply with these terms.
*Correspondence: Noppol Arunrat, bm9wcG9sLmFydUBtYWhpZG9sLmFjLnRo