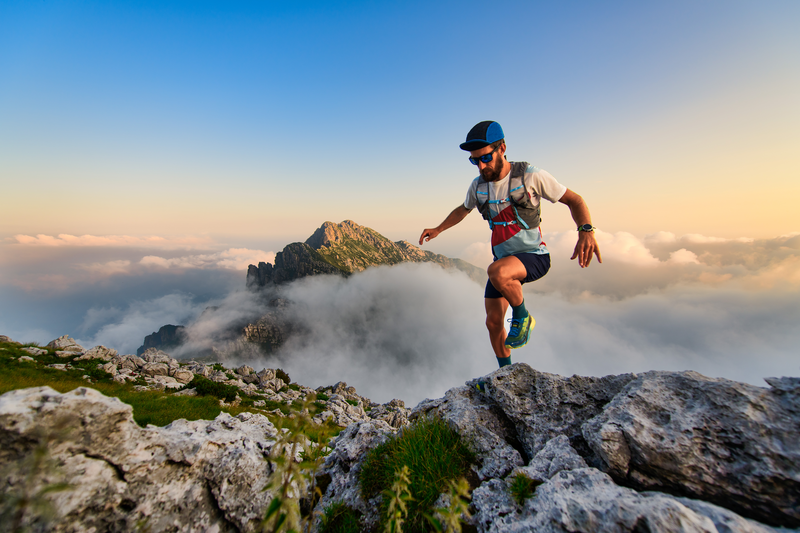
94% of researchers rate our articles as excellent or good
Learn more about the work of our research integrity team to safeguard the quality of each article we publish.
Find out more
ORIGINAL RESEARCH article
Front. Environ. Sci. , 09 May 2023
Sec. Conservation and Restoration Ecology
Volume 11 - 2023 | https://doi.org/10.3389/fenvs.2023.1115119
This article is part of the Research Topic Freshwater Biodiversity and Ecosystem Functioning: Novel Ideas and Approaches View all 9 articles
The effect of biodiversity on ecosystem productivity has been a controversial issue in ecological research. The species richness–productivity relationship is highly variable in natural ecosystems, with a positive relationship being one of the most commonly observed relationships. Previous regional studies from terrestrial ecosystems have demonstrated that environmental gradients can regulate the species richness–productivity relationship. However, how this relationship varies in freshwater ecosystems across spatial environment gradients remains unclear. In this study, we propose that the species richness–productivity relationship can be modulated by the water depth. Here, we surveyed the submerged macrophyte community structure by establishing 24 transects and 642 quadrats in Erhai Lake, Yunnan Plateau, China. Our findings highlight that the species richness–productivity relationship gradually changed from slightly positive to strongly positive as the environment became more light-limited with the increasing water depth, supporting the stress-gradient hypothesis. The results from this study provide new insights into the biodiversity–ecosystem functioning relationships and in managing lake macrophyte communities and productivity.
The relationship between biodiversity and community productivity has been one of the key issues and controversies in ecology in recent decades (Fraser et al., 2015; Dyola et al., 2022). This is mainly due to the important theoretical value and management implications of the biodiversity–productivity relationship (Loreau et al., 2001; Tilman et al., 2012; Wu et al., 2015; Grace et al., 2016). There have been substantial efforts to examine the effects of biodiversity on ecosystem functioning, and different relationships (e.g., positive, negative, and hump-shaped) or a lack of relationship has been found between biodiversity and ecosystem functioning (Hector et al., 1999; Huston, 2000; Duffy et al., 2017). The variations in their relationship can be attributed to the differences in the environmental gradient, ecosystem type, sampling method, and research method (e.g., biodiversity-manipulation experiments, field surveys, and meta-analyses) (Hector et al., 1999; Hooper et al., 2005; Roscher et al., 2005; Cardinale et al., 2012; Isbell et al., 2015).
Understanding how the species richness–productivity relationship changes across environmental gradients is crucial for improving ecosystem management (Schmid, 2002; Paquette and Messier, 2011; Liang et al., 2016; Liang et al., 2022). Although several contrasting species richness–productivity relationships have been observed in terrestrial ecosystems (Schmid, 2002; Guo et al., 2019; Su et al., 2019), a positive relationship is one of the most common patterns (Loreau et al., 2001; Hooper et al., 2005). Studying this question also helps us gain deeper insights into another important pattern in ecology (Loreau et al., 2001; Hooper et al., 2005), which is the hump-shaped relationship between productivity and species richness (Figure 1A). It has been suggested that the initial increase in species richness in an ecosystem is mainly due to the increased availability of resources, while species interactions (i.e., competition) drive a decline in species richness at latter stages (He et al., 2002; Fridley, 2003). Previous studies have proposed two important underlying mechanisms to describe biodiversity effects on productivity: 1) biodiversity effects on productivity can be enhanced with favorable environmental conditions (Loreau et al., 2001; Wang et al., 2019) (Figure 1B) because sufficient resources and space under a favorable environment reduce competition and provide opportunities for species to coexist, therefore increasing the positive effect of species diversity on productivity (Fridley, 2003; Paquette and Messier, 2011); 2) biodiversity effects can also be enhanced under unfavorable environmental conditions (Paquette and Messier, 2011; Steudel et al., 2012; Wang et al., 2019) (Figure 1C). For instance, Paquette and Messier (2011) showed that the relationship between species richness and productivity gradually changes from significantly positive to an insignificant relationship with the environment changing from harsh to favorable conditions. Particularly, the enhancement of the complementary effects and niche partitioning can increase the positive effects of species diversity on productivity in harsh environments (Fridley, 2003; Paquette and Messier, 2011).
FIGURE 1. Hypothesized relationships between species richness and community productivity in a natural lake ecosystem. (A) Overall unimodal relationship between productivity and species richness, which is shaped by the changing environmental conditions that have variable effects on species richness and productivity (Loreau et al., 2001). (B) and (C): local patterns of species richness in relation to productivity at specific environmental conditions (black lines) that modulate the overall species richness–productivity relationship the (dashed blue curve). (B) Effect of richness on community productivity is assumed to cause divergent species richness–productivity relationships, with increasing species richness along environmental gradients, modified from the studies by Loreau et al. (2001), Wu et al. (2015), and Wang et al. (2019). (C) Effect of richness on community productivity is expected to cause convergent species richness–productivity relationships, with increasing species richness along environmental gradients, modified from the studies by Paquette and Messier (2011), Wu et al. (2015), and Wang et al. (2019).
Previous biodiversity–productivity relationship studies were mainly concentrated on grassland and forest ecosystems, and these studies demonstrated that environmental gradients are important in modulating biodiversity–ecosystem functioning relationships (Mulder et al., 2001; Steudel et al., 2012; Guo et al., 2019). Nevertheless, lake ecosystems have received very little attention (Skacelova and Leps, 2014; Li et al., 2020; Danet et al., 2021), and the results from the limited existing studies are controversial. Some studies have demonstrated that species richness positively affects the community biomass of submerged macrophytes (Gustafsson and Bostrom, 2011; Wang et al., 2022b), while others found no relationships (Riis et al., 2018; Zhang et al., 2019). Water depth is widely acknowledged as the main factor determining the submerged macrophytes’ growth and distribution because water depth affects light intensity (Strand and Weisner, 2001; Bai et al., 2014; Dong et al., 2014). With the increasing water depth, the absorption and scattering of light in water increases (Middelboe and Markager, 1997), creating stronger light-limited conditions. Previous studies have revealed that increasing the water depth negatively affects species richness (Fu et al., 2014a; Ye et al., 2018; Lewerentz et al., 2021; Ma et al., 2021). Additionally, some studies have shown that the relationship between community biomass and water depth is unimodal or is either negative or positive (Fu et al., 2014a; Dong et al., 2014; Bolpagni et al., 2016; Ye et al., 2018; Wang et al., 2022a). Water depth can act as an environmental filter for selecting species with specific phenotypic characteristics, and it affects species interactions (Fu et al., 2014a; Fu et al., 2014b), potentially regulating the effects of species richness on the community biomass of submerged macrophytes. Nevertheless, limited information is available about how the macrophyte species richness–productivity relationship varies across water depth gradients.
In this study, we explored how the species richness–biomass relationship of submerged macrophytes varies with the water depth in a large subtropical lake ecosystem (here, biomass is used as a surrogate measure of productivity) (Peng and Huang, 2000). We conducted a field survey of the submerged macrophyte community structure in Erhai Lake, China. We hypothesized that 1) the relationship between the macrophyte community biomass and water depth would be hump-shaped, 2) the species richness of the macrophyte community decreases with the increasing water depth, and 3) the relationship between the macrophyte community biomass and species richness varies across water depth gradients.
Erhai Lake is a large subtropical lake located on the Yunnan–Guizhou Plateau in China (25◦52′N and 100◦06′E). It has a water surface area of 252 km2 situated 1,966 m above sea level (a.s.l.), with an average water depth of 10 m and an average water retention time of 4.5 years. During the study period, the average water level of the lake was 1,965.03 m a.s.l. The region has a subtropical monsoon climate, with an annual mean temperature of 15°C, wet seasonal precipitation of 870 mm (May–October), and dry seasonal precipitation of 170 mm (November–April) (Wen et al., 2021). Erhai Lake is in the early stages of eutrophication and is changing from mesotrophic to eutrophic, with total nitrogen (TN) and phosphorus (TP) concentrations ranging from 0.60 to 0.80 mg L-1 and 0.015 to 0.037 mg L-1, respectively (Yin et al., 2021). The dominant submerged macrophyte species in the lake include Potamogeton maackianus, Vallisneria natans, Ceratophyllum demersum, and Hydrilla verticillata (Wen et al., 2022). There are 6 common, 10 rare, and 2 endangered species in Erhai Lake, including Utricularia aurea Lour. and Ottelia acuminata (Ye et al., 2018; unpublished data). The maximum colonization depth of the submerged macrophytes is less than 5.5 m (Wen et al., 2022).
We conducted the study in Erhai Lake in June and July of 2017. Three evenly distributed transects were set perpendicular to the shoreline in each of the eight bays (i.e., Shapin, Xizhou, Majiuyi, Erhaiyue, Xiangyang, Wase, Changyu, and Hongshan) within the lake. With the local socioeconomic development in recent decades, increasing anthropogenic discharge and agricultural non-point pollution around these bays lead to the increased sediment nutrient content, i.e., TP and TN concentrations increased by ca. 0.10% and 0.46% within 2010, respectively (Li et al., 2016). There are 23 rivers flowing into the lake, with one river and one water diversion tunnel flowing out of the lake (Yang et al., 2021a; Yang et al., 2021b; Hu et al., 2021). Macrophyte sampling occurred at 0.5 m water depth intervals across each transect using a rotatable reaping hook to the maximum colonization depth (i.e., 5 m depth) of macrophytes (Figure 2). The sampling area per sample was 0.2 m2, and sampling was repeated three times at each depth. We sampled the submerged macrophyte communities from 642 quadrats (0.2 m2) (Figure 2). The macrophytes collected from each sample were washed, identified of their species level, drained, and weighed as fresh biomass. The total plant community biomass and the biomass of individual macrophytes at each sampling plot were expressed as the average value from three quadrats. The distribution depth of the submerged macrophytes was determined using a sonic depth finder. Water clarity at each quadrat across the water depth was measured in situ with the Secchi depth before sampling.
Based on our hypotheses, we applied the polynomial regression to explore the non-linear relationships of community biomass with the water depth and species richness. Since we assumed that species richness is negatively correlated with the water depth, unary linear regression analysis was used to explore their relationship. The macrophyte community biomass data were log(x + 1), transformed to improve the variance homogeneity and normality of data. In order to classify the distribution pattern of the submerged macrophyte community biomass along the water depth gradient, regression trees (RTs) were performed to divide the water depth into classes by applying the R package “Party.” RT models recursively partition data to find increasingly homogeneous subsets based on independent variable splitting criteria using variance-minimizing algorithms. The dependent data were partitioned into a series of descending left and right child nodes derived from parent nodes (Krzywinski and Altman, 2017). The RT divided the water depth into three classes, 0< water depth ≤1.5 m, 1.5< water depth ≤3.6 m, and 3.6< water depth ≤5 m (Supplementary Figures S1, S2), representing shallow, medium, and deep water areas, respectively. The species richness of the submerged macrophyte community was the average value at each sampling depth and was calculated using the R package “Vegan.” Simple linear regression was used to explore the relationship between the macrophyte community biomass and species richness of different water depth areas. Relative biomass is equal to the biomass of a species divided by the sum of the biomass of all species in each plot, and a species with a relative biomass of more than 5% was defined as a dominant species (Mouillot et al., 2013; Ma et al., 2017; Ratcliffe et al., 2017) (see Supplementary Figure S3 for biomass variation across the dominant species). The ratio of water clarity (i.e., Secchi depth) to water depth was calculated as a proxy for underwater light availability, and simple linear regression was applied to explore the relationship between the water clarity and the water depth ratio and water depth (Supplementary Figure S4). We used the ggplot2 package in R for graph plots. All statistical analyses and graph plots were performed using R 3.5.4 (R Core Team, 2019).
The macrophyte community biomass showed a unimodal relationship with the water depth gradient (R2 = 0.29; p < 0.001; Figure 3A). The macrophyte community biomass decreased as the water depth increased (R2 = 0.16; p < 0.001; Figure 3B). The water clarity to water depth ratio decreased with the increasing water depth (Supplementary Figure S4). Species richness also showed a unimodal relationship with the macrophyte community biomass (R2 = 0.06; p < 0.05; Figure 3C).
FIGURE 3. Results of polynomial regressions showing relationships between (A) water depth and the macrophyte community fresh biomass, (B) water depth and macrophyte species richness, and (C) macrophyte community biomass and species richness. The blue lines indicate the polynomial regression models, and the shades indicate the 95% confidence intervals. Data are the mean values of species richness and community biomass from three quadrats at each water depth.
Community biomass positively correlated with species richness both in shallow and deep water areas. However, the relationship was significant only in deep water (p < 0.05). The slope of biomass against species richness was small in shallow water but became steeper in deep water (Figure 4). The relationship between community biomass and species richness was insignificant at the medium water depth (p > 0.05; Figure 4).
FIGURE 4. Linear relationships between species richness and community biomass at individual depth classes (solid lines). The shades indicate the 95% confidence intervals of linear regressions. Red, blue, and green symbols represent shallow (0< water depth ≤1.5 m), medium (1.5< water depth ≤3.6 m), and deep (3.6< water depth ≤5 m) water depth categories, respectively. Data are averages of the three sampling points at individual water depth classes per transect.
In the shallow water area, dominant macrophytes species included P. maackianus (35.4%), followed by C. demersum (18.3%), Myriophyllum spicatum (13.7%), P. lucens (9.7%), H. verticillata (8.2%), Potamogeton wrightii (5.5%), and V. natans (5.2%). In the medium water area, the most dominant species were P. maackianus (59.5%), followed by C. demersum (11.7%), V. natans (8.4%), P. lucens (7.6%), and H. verticillata (5.9%). In the deep water area, the most dominant species comprised P. maackianus (35.7%), followed by V. natans (34.5%) and C. demersum (26.5%). The relative biomass of V. natans increased with the increasing water depth. P. maackianus had the highest relative biomass in the medium water depth, whereas C. demersum had the lowest relative biomass (Figure 5; Supplementary Figure S3).
FIGURE 5. Relative biomass of submerged macrophyte species in Erhai Lake. The scale is 0–1, i.e., 0%–100%.
Our results showed an overall humped-shaped relationship between community biomass and species richness of the submerged macrophytes, which is consistent with our first hypothesis. The species richness of the macrophyte community decreased with the increasing water depth, which is in line with our second hypothesis. The positive effect of species richness on macrophyte community biomass increased with the water depth, supporting the stress-gradient hypothesis (Figure 1C; hypothesis 3).
We found that species richness decreased with the increasing water depth. In addition, there was a significant decrease in the water clarity to water depth ratio (i.e., light availability) with the increasing water depth (Supplementary Figure S4). These findings imply that the increasing light limitation with the increasing water depth negatively affects macrophyte species richness (Chisholm et al., 2013). At the medium water depth, the macrophyte community composition changed dramatically compared to that in shallow water. As light availability became more limited in deeper water, the submerged macrophytes likely competed for light and space, and dense canopy-forming species, such as P. maackianus, displaced the neighboring species and eventually became dominant at the medium water depth area. Previous studies also showed that canopy-forming species could elongate their stems and form a dense canopy to gain a competitive advantage over non-canopy-forming species (Fu et al., 2014b; He et al., 2019). However, at the deep water area, the macrophyte community structure was dominated primarily by a few species tolerant to low-light conditions, including P. maackianus, C. demersum, and V. natans, thus reducing species richness. Consistent with our findings, previous studies also reported reduced species richness in deep water areas as only a few species can withstand low-light conditions and grow (He et al., 2019; Chou et al., 2022).
Our results showed an overall unimodal relationship between macrophyte biomass and species richness, likely due to the fact that the species richness effect on community biomass was enhanced at harsher low-light conditions. These findings support our prediction that environmental gradients can modulate species richness–biomass relationships at a finer spatial scale. For instance, in shallow water, macrophyte species richness did not significantly affect the community biomass, likely due to the weak effects of complementarity (e.g., species-complementary resource use) (Jiang et al., 2008; Jiang et al., 2009). At the medium water depth, the community biomass was, on average, the highest compared to that at other water depths, and the insignificant species richness–biomass relationship likely resulted from the competitive exclusion (i.e., two species competing for the same limited resource may not coexist) between dominant and subordinate species (Huston, 1997; Tilman et al., 1997). In deep water, the numbers of species reduced sharply due to a further decrease in the underwater light availability, and only a few low-light tolerant species, i.e., P. maackianus, C. demersum, and V. natans, were able to colonize (Wen et al., 2022). These species can adapt to low-light conditions through different adaptive strategies (e.g., P. maackianus allocates more biomass to stems and forms dense canopies, while V. natans increases both the leaf chlorophyll content and leaf biomass) and occupy different water-column spaces (Chen et al., 2016; He et al., 2019; Wen et al., 2022). In addition, P. maackianus and M. spicatum can provide feedback to improve the environmental conditions, such as water clarity by inhibiting the growth of phytoplankton and periphyton through allelopathy or nutrient competition (Liu et al., 2020) and facilitate the growth of other species that are less tolerant to low-light conditions. The stronger species richness–biomass relationship in the deep water area in our study indicates that interspecific interactions among species may be conducive to the community biomass at low-light environments. Our findings agree with previous studies, which demonstrated that positive species interactions among macrophytes could promote growth and reproduction under adverse environments (Le Bagousse-Pinguet et al., 2012; Hao et al., 2013; Liu et al., 2020). For instance, Hao et al. (2013) found that the positive interaction between P. maackianus and M. spicatum due to their morphological differences or niche complementarity has enhanced their survival in harsh environments (e.g., severe eutrophic conditions). Hence, our results support the stress-gradient hypothesis, which suggests that species that are dominant in tough conditions will reinforce each other. However, in favorable environmental conditions, they exhibit competitive interactions (Bertness and Callaway, 1994; Steudel et al., 2013).
Our research provides evidence that the effects of macrophyte species richness on community biomass vary with the water depth in a large subtropical lake, suggesting that water depth can modulate the species richness–biomass relationship. In addition, our results imply that maintaining multiple macrophyte species with adaptive strategies to low-light conditions is important for enhancing the overall macrophyte community biomass in light-limited environments. Consistent with our findings, previous studies also reported a hump-shaped relationship between community biomass and water depth (Lewerentz et al., 2021; Ma et al., 2021), suggesting that the submerged macrophyte biomass can be regulated by managing water levels in freshwater lakes. Our results have significant management implications for the lake macrophyte biodiversity conservation and productivity.
Understanding the species richness–biomass relationship is essential for biodiversity conservation and the sustainable management of natural ecosystems. Our results revealed a unimodal relationship between biomass and species richness of submerged macrophytes. However, the effects of species richness on community biomass strongly depended on the water depth, supporting the stress-gradient hypothesis. In the shallow water area, where light was not limited, macrophyte species richness showed a weak effect on community biomass. In contrast, species richness enhanced community biomass in deep water areas with relatively more light-limited conditions. This study provides support for biodiversity-ecosystem functioning relationships in freshwater ecosystems, but these relationships are affected by environmental gradients, i.e., the water depth in our case. Our findings imply that artificial water level management may be applied to modulate the relationship between macrophyte diversity and productivity in freshwater lakes.
The raw data supporting the conclusion of this article will be made available by the authors, without undue reservation.
TC and XZ contributed to the conception and design of the study. ZW, HW, and HS conducted the field study. ZW performed the statistical analysis and wrote the first draft of the manuscript. QCh, YC, LN, QCa, LT, and TZ reviewed and edited the manuscript. All authors contributed to the manuscript revision and editing, and approved the submitted version.
This study was supported by the National Key R&D Program of China (2018YFD0900806), the Normal Project (Grant Nos. 32201340, 31930074, and 32101319) of the National Science Foundation of China, and the State Key Laboratory of Freshwater Ecology and Biotechnology (Grant No. 2019FBZ01).
The authors declare that the research was conducted in the absence of any commercial or financial relationships that could be construed as a potential conflict of interest.
The reviewer HS declared a past co-authorship with the authors ZW, HW, YC and LN to the handling editor.
All claims expressed in this article are solely those of the authors and do not necessarily represent those of their affiliated organizations, or those of the publisher, the editors, and the reviewers. Any product that may be evaluated in this article, or claim that may be made by its manufacturer, is not guaranteed or endorsed by the publisher.
The Supplementary Material for this article can be found online at: https://www.frontiersin.org/articles/10.3389/fenvs.2023.1115119/full#supplementary-material
Bai, X., Chen, K., Zhao, H., and Chen, X. (2014). Impact of water depth and sediment type on root morphology of the submerged plant Vallisneria natans. J. Freshw. Ecol. 30 (1), 75–84. doi:10.1080/02705060.2014.970672
Bertness, M. D., and Callaway, R. (1994). Positive interactions in communities. Trends Ecol. Evol. 9 (5), 191–193. doi:10.1016/0169-5347(94)90088-4
Bolpagni, R., Laini, A., and Azzella, M. M. (2016). Short-term dynamics of submerged aquatic vegetation diversity and abundance in deep lakes. Appl. Veg. Sci. 19 (4), 711–723. doi:10.1111/avsc.12245
Cardinale, B. J., Duffy, J. E., Gonzalez, A., Hooper, D. U., Perrings, C., Venail, P., et al. (2012). Biodiversity loss and its impact on humanity. Nature 486 (7401), 59–67. doi:10.1038/nature11148
Chen, J., Cao, T., Zhang, X., Xi, Y., Ni, L., and Jeppesen, E. (2016). Differential photosynthetic and morphological adaptations to low light affect depth distribution of two submersed macrophytes in lakes. Sci. Rep. 6 (1), 34028. doi:10.1038/srep34028
Chisholm, R. A., Muller-Landau, H. C., Abdul Rahman, K., Bebber, D. P., Bin, Y., Bohlman, S. A., et al. (2013). Scale-dependent relationships between tree species richness and ecosystem function in forests. J. Ecol. 101 (5), 1214–1224. doi:10.1111/1365-2745.12132
Chou, Q., Zhang, W., Chen, J., Ren, W., Yuan, C., Wen, Z., et al. (2022). Phenotypic responses of a submerged macrophyte (Vallisneria natans) to low light combined with water depth. Aquat. Bot. 176, 103462. doi:10.1016/j.aquabot.2021.103462
Danet, A., Mouchet, M., Bonnaffe, W., Thebault, E., and Fontaine, C. (2021). Species richness and food-web structure jointly drive community biomass and its temporal stability in fish communities. Ecol. Lett. 24 (11), 2364–2377. doi:10.1111/ele.13857
Dong, B., Qin, B., Gao, G., and Cai, X. (2014). Submerged macrophyte communities and the controlling factors in large, shallow lake taihu (China): Sediment distribution and water depth. J. Gt. Lakes Res. 40 (3), 646–655. doi:10.1016/j.jglr.2014.04.007
Duffy, J. E., Godwin, C. M., and Cardinale, B. J. (2017). Biodiversity effects in the wild are common and as strong as key drivers of productivity. Nature 549 (7671), 261–264. doi:10.1038/nature23886
Dyola, N., Sigdel, S. R., Liang, E., Babst, F., Camarero, J. J., Aryal, S., et al. (2022). Species richness is a strong driver of forest biomass along broad bioclimatic gradients in the Himalayas. Ecosphere 13 (6). doi:10.1002/ecs2.4107
Fraser, L. H., Pither, J., Jentsch, A., Sternberg, M., Zobel, M., Askarizadeh, D., et al. (2015). Worldwide evidence of a unimodal relationship between productivity and plant species richness. Science 349 (6245), 302–305. doi:10.1126/science.aab3916
Fridley, J. D. (2003). Diversity effects on production in different light and fertility environments: An experiment with communities of annual plants. J. Ecol. 91 (3), 396–406. doi:10.1046/j.1365-2745.2003.00775.x
Fu, H., Zhong, J., Yuan, G., Ni, L., Xie, P., and Cao, T. (2014a). Functional traits composition predict macrophytes community productivity along a water depth gradient in a freshwater lake. Ecol. Evol. 4 (9), 1516–1523. doi:10.1002/ece3.1022
Fu, H., Zhong, J., Yuan, G., Xie, P., Guo, L., Zhang, X., et al. (2014b). Trait-based community assembly of aquatic macrophytes along a water depth gradient in a freshwater lake. Freshw. Biol. 59 (12), 2462–2471. doi:10.1111/fwb.12443
Grace, J. B., Anderson, T. M., Seabloom, E. W., Borer, E. T., Adler, P. B., Harpole, W. S., et al. (2016). Integrative modelling reveals mechanisms linking productivity and plant species richness. Nature 529 (7586), 390–393. doi:10.1038/nature16524
Guo, Y., Schob, C., Ma, W., Mohammat, A., Liu, H., Yu, S., et al. (2019). Increasing water availability and facilitation weaken biodiversity-biomass relationships in shrublands. Ecology 100 (3), e02624. doi:10.1002/ecy.2624
Gustafsson, C., and Bostrom, C. (2011). Biodiversity influences ecosystem functioning in aquatic angiosperm communities. Oikos 120 (7), 1037–1046. doi:10.1111/j.1600-0706.2010.19008.x
Hao, B. B., Wu, H., Shi, Q., Liu, G., and Xing, W. (2013). Facilitation and competition among foundation species of submerged macrophytes threatened by severe eutrophication and implications for restoration. Ecol. Eng. 60, 76–80. doi:10.1016/j.ecoleng.2013.07.067
He, J. S., Bazzaz, F. A., and Schmid, B. (2002). Interactive effects of diversity, nutrients and elevated CO2 on experimental plant communities. Oikos 97 (3), 337–348. doi:10.1034/j.1600-0706.2002.970304.x
He, L., Zhu, T., Wu, Y., Li, W., Zhang, H., Zhang, X., et al. (2019). Littoral slope, water depth and alternative response strategies to light attenuation shape the distribution of submerged macrophytes in a mesotrophic lake. Front. Plant Sci. 10, 169. doi:10.3389/fpls.2019.00169
Hector, A., Schmid, B., Beierkuhnlein, C., Caldeira, M. C., Diemer, M., Dimitrakopoulos, P. G., et al. (1999). Plant diversity and productivity experiments in European grasslands. Science 286 (5442), 1123–1127. doi:10.1126/science.286.5442.1123
Hooper, D. U., Chapin, F. S., Ewel, J. J., Hector, A., Inchausti, P., Lavorel, S., et al. (2005). Effects of biodiversity on ecosystem functioning: A consensus of current knowledge. Ecol. Monogr. 75 (1), 3–35. doi:10.1890/04-0922
Hu, L., Shan, K., Huang, L., Li, Y., Zhao, L., Zhou, Q., et al. (2021). Environmental factors associated with cyanobacterial assemblages in a mesotrophic subtropical plateau lake: A focus on bloom toxicity. Sci. Total Environ. 777, 146052. doi:10.1016/j.scitotenv.2021.146052
Huston, M., A., Aarssen, L. W., Austin, M. P., Cade, B. S., Fridley, J. D., Garnier, E., et al. (2000). No consistent effect of plant diversity on productivity. Science 289 (5483), 1255. doi:10.1126/science.289.5483.1255a
Huston, M. A. (1997). Hidden treatments in ecological experiments: Re-evaluating the ecosystem function of biodiversity. Oecologia 110 (4), 449–460. doi:10.1007/s004420050180
Isbell, F., Craven, D., Connolly, J., Loreau, M., Schmid, B., Beierkuhnlein, C., et al. (2015). Biodiversity increases the resistance of ecosystem productivity to climate extremes. Nature 526 (7574), 574–577. doi:10.1038/nature15374
Jiang, L., Pu, Z., and Nemergut, D. R. (2008). On the importance of the negative selection effect for the relationship between biodiversity and ecosystem functioning. Oikos 117 (4), 488–493. doi:10.1111/j.0030-1299.2008.16401.x
Jiang, L., Wan, S., and Li, L. (2009). Species diversity and productivity: Why do results of diversity-manipulation experiments differ from natural patterns? J. Ecol. 97 (4), 603–608. doi:10.1111/j.1365-2745.2009.01503.x
Krzywinski, M., and Altman, N. (2017). Classification and regression trees. Nat. Methods 14 (8), 757–758. doi:10.1038/nmeth.4370
Le Bagousse-Pinguet, Y., Liancourt, P., Gross, N., and Straile, D. (2012). Indirect facilitation promotes macrophyte survival and growth in freshwater ecosystems threatened by eutrophication. J. Ecol. 100 (2), 530–538. doi:10.1111/j.1365-2745.2011.01931.x
Lewerentz, A., Hoffmann, M., and Cabral, J. S. (2021). Depth diversity gradients of macrophytes: Shape, drivers, and recent shifts. Ecol. Evol. 11 (20), 13830–13845. doi:10.1002/ece3.8089
Li, Z., Shengrui, W., Haichao, Z., Yanping, L., Shouliang, H., Weibin, Q., et al. (2016). Using multiple combined analytical techniques to characterize water extractable organic nitrogen from Lake Erhai sediment. Sci. Total Environ. 542, 344–353. doi:10.1016/j.scitotenv.2015.10.039
Li, Z., Tian, Y., Zhou, Y., Gong, X., Peng, Q., Xu, Z., et al. (2020). Geometric constraints and area effects shape geographical patterns of aquatic plant diversity in a highly arid region. Ecol. Indic. 116, 106502. doi:10.1016/j.ecolind.2020.106502
Liang, J., Crowther, T. W., Picard, N., Wiser, S., Zhou, M., Alberti, G., et al. (2016). Positive biodiversity-productivity relationship predominant in global forests. Science 354 (6309), aaf8957. doi:10.1126/science.aaf8957
Liang, M., Baiser, B., Hallett, L. M., Hautier, Y., Jiang, L., Loreau, M., et al. (2022). Consistent stabilizing effects of plant diversity across spatial scales and climatic gradients. Nat. Ecol. Evol. 6 (11), 1669–1675. doi:10.1038/s41559-022-01868-y
Liu, H., Zhou, W., Li, X., Chu, Q., Tang, N., Shu, B., et al. (2020). How many submerged macrophyte species are needed to improve water clarity and quality in Yangtze floodplain lakes? Sci. Total Environ. 724, 138267. doi:10.1016/j.scitotenv.2020.138267
Loreau, M., Naeem, S., Inchausti, P., Bengtsson, J., Grime, J. P., Hector, A., et al. (2001). Biodiversity and ecosystem functioning: Current knowledge and future challenges. Science 294 (5543), 804–808. doi:10.1126/science.1064088
Ma, F., Yang, L., Lv, T., Zuo, Z., Zhao, H., Fan, S., et al. (2021). The biodiversity-biomass relationship of aquatic macrophytes is regulated by water depth: A case study of a shallow mesotrophic Lake in China. Front. Ecol. Evol. 9. doi:10.3389/fevo.2021.650001
Ma, Z., Liu, H., Mi, Z., Zhang, Z., Wang, Y., Xu, W., et al. (2017). Climate warming reduces the temporal stability of plant community biomass production. Nat. Commun. 8, 15378. doi:10.1038/ncomms15378
Middelboe, A. L., and Markager, S. (1997). Depth limits and minimum light requirements of freshwater macrophytes. Freshw. Biol. 37(3), 553–568. doi:10.1046/j.1365-2427.1997.00183.x
Mouillot, D., Bellwood, D. R., Baraloto, C., Chave, J., Galzin, R., Harmelin-Vivien, M., et al. (2013). Rare species support vulnerable functions in high-diversity ecosystems. PLoS. Biol. 11 (5), e1001569. doi:10.1371/journal.pbio.1001569
Mulder, C. P., Uliassi, D. D., and Doak, D. F. (2001). Physical stress and diversity-productivity relationships: The role of positive interactions. Proc. Natl. Acad. Sci. U. S. A. 98 (12), 6704–6708. doi:10.1073/pnas.111055298
Paquette, A., and Messier, C. (2011). The effect of biodiversity on tree productivity: From temperate to boreal forests. Glob. Ecol. Biogeogr. 20 (1), 170–180. doi:10.1111/j.1466-8238.2010.00592.x
Peng, S., and Huang, Z. (2000). Review on the relationship between productivity and biodiversity. Ecol. Sci. 19 (1), 1–9.
R Core Team (2019). R: A language and environment for statistical computing. United States: R Foundation for Statistical Computing.
Ratcliffe, S., Wirth, C., Jucker, T., van der Plas, F., Scherer-Lorenzen, M., Verheyen, K., et al. (2017). Biodiversity and ecosystem functioning relations in European forests depend on environmental context. Ecol. Lett. 20 (11), 1414–1426. doi:10.1111/ele.12849
Riis, T., Olesen, A., Jensen, S. M., Alnoee, A. B., Baattrup-Pedersen, A., Lauridsen, T. L., et al. (2018). Submerged freshwater plant communities do not show species complementarity effect in wetland mesocosms. Biol. Lett. 14 (12), 20180635. doi:10.1098/rsbl.2018.0635
Roscher, C., Temperton, V. M., Scherer-Lorenzen, M., Schmitz, M., Schumacher, J., Schmid, B., et al. (2005). Overyielding in experimental grassland communities - irrespective of species pool or spatial scale. Ecol. Lett. 8 (4), 419–429. doi:10.1111/j.1461-0248.2005.00736.x
Schmid, B. (2002). The species richness-productivity controversy. Trends Ecol. Evol. 17 (3), 113–114. doi:10.1016/S0169-5347(01)02422-3
Skacelova, O., and Leps, J. (2014). The relationship of diversity and biomass in phytoplankton communities weakens when accounting for species proportions. Hydrobiologia 724 (1), 67–77. doi:10.1007/s10750-013-1723-2
Steudel, B., Hector, A., Friedl, T., Loefke, C., Lorenz, M., Wesche, M., et al. (2012). Biodiversity effects on ecosystem functioning change along environmental stress gradients. Ecol. Lett. 15 (12), 1397–1405. doi:10.1111/j.1461-0248.2012.01863.x
Steudel, B., Hector, A., Friedl, T., Löfke, C., Lorenz, M., Wesche, M., et al. (2013). Biodiversity effects on ecosystem functioning change along environmental stress gradients. Ecol. Lett. 16 (4), 568–569. doi:10.1111/ele.12079
Strand, J. A., and Weisner, S. E. B. (2001). Morphological plastic responses to water depth and wave exposure in an aquatic plant (Myriophyllum spicatum). J. Ecol. 89 (2), 166–175. doi:10.1046/j.1365-2745.2001.00530.x
Su, H., Chen, J., Wu, Y., Chen, J., Guo, X., Yan, Z., et al. (2019). Morphological traits of submerged macrophytes reveal specific positive feedbacks to water clarity in freshwater ecosystems. Sci. Total Environ. 684, 578–586. doi:10.1016/j.scitotenv.2019.05.267
Tilman, D., Lehman, C. L., and Thomson, K. T. (1997). Plant diversity and ecosystem productivity: Theoretical considerations. Proc. Natl. Acad. Sci. U. S. A. 94 (5), 1857–1861. doi:10.1073/pnas.94.5.1857
Tilman, D., Reich, P. B., and Isbell, F. (2012). Biodiversity impacts ecosystem productivity as much as resources, disturbance, or herbivory. Proc. Natl. Acad. Sci. U. S. A. 109 (26), 10394–10397. doi:10.1073/pnas.1208240109
Wang, H., Wen, Z., Ren, W., Ni, L., Zhang, X., Li, K., et al. (2022a). What is the mechanism of submerged macrophyte biodiversity affecting biomass productivity along water depth: Niche complementarity or selection effect? Ecol. Indic. 138, 108858. doi:10.1016/j.ecolind.2022.108858
Wang, H., Zhang, X., Shan, H., Chaochao, L., Ren, W., Wen, Z., et al. (2022b). Biodiversity buffers the impact of eutrophication on ecosystem functioning of submerged macrophytes on the Yunnan-Guizhou Plateau, Southwest China. Environ. Pollut. 314, 120210. doi:10.1016/j.envpol.2022.120210
Wang, Y., Cadotte, M. W., Chen, Y., Fraser, L. H., Zhang, Y., Huang, F., et al. (2019). Global evidence of positive biodiversity effects on spatial ecosystem stability in natural grasslands. Nat. Commun. 10, 3207. doi:10.1038/s41467-019-11191-z
Wen, Z. H., Ma, Y. W., Wang, H., Cao, Y., Yuan, C. B., Ren, W. J., et al. (2021). Water level regulation for eco-social services under climate change in erhai lake over the past 68 years in China. Front. Environ. Sci. 9, 697694. doi:10.3389/fenvs.2021.697694
Wen, Z. H., Wang, H., Zhang, Z., Cao, Y., Yao, Y., Gao, X., et al. (2022). Depth distribution of three submerged macrophytes under water level fluctuations in a large plateau lake. Aquat. Bot. 176, 103451. doi:10.1016/j.aquabot.2021.103451
Wu, X., Wang, X., Tang, Z., Shen, Z., Zheng, C., Xia, X., et al. (2015). The relationship between species richness and biomass changes from boreal to subtropical forests in China. Ecography 38 (6), 602–613. doi:10.1111/ecog.00940
Yang, T., Yuang, C., Cao, T., Wen, Z., chuanChou, Q., Mao, Y., et al. (2021a). Preliminary study on recovery and optimization of submerged macrophyte community in Lake Erhai, China. J. Lake Sci. 33 (06), 1777–1787. doi:10.18307/2021.0614
Yang, T., Zhang, X., Li, J., Zhao, H., Jin, F., and Zhou, B. (2021b). Analysis and evaluation of the treatment and reuse of tailwater: A case study in Erhai Lake. J. Clean. Prod. 327, 129435. doi:10.1016/j.jclepro.2021.129435
Ye, B., Chu, Z., Wu, A., Hou, Z., and Wang, S. (2018). Optimum water depth ranges of dominant submersed macrophytes in a natural freshwater lake. PLoS One 13 (3), e0193176. doi:10.1371/journal.pone.0193176
Yin, C., Chen, Y., Guo, L., and Ni, L. (2021). Fish assemblage shift after Japanese Smelt (Hypomesus nipponensis McAllister, 1963) invasion in Lake Erhai, a subtropical plateau lake in China. Water 13 (13), 1800. doi:10.3390/w13131800
Keywords: water depth, submerged macrophyte, biodiversity–ecosystem functioning relationship, stress-gradient hypothesis, species richness–biomass relationship
Citation: Wen Z, Wang H, Shan H, Cao Y, Tan L, Zhu T, Cai Q, Ni L, Zhang X, Chou Q and Cao T (2023) Water depth modulates the species richness–biomass relationship in submerged macrophytes. Front. Environ. Sci. 11:1115119. doi: 10.3389/fenvs.2023.1115119
Received: 03 December 2022; Accepted: 21 April 2023;
Published: 09 May 2023.
Edited by:
Danny Chun Pong Lau, Swedish University of Agricultural Sciences, SwedenReviewed by:
Haojie Su, Yunnan University, ChinaCopyright © 2023 Wen, Wang, Shan, Cao, Tan, Zhu, Cai, Ni, Zhang, Chou and Cao. This is an open-access article distributed under the terms of the Creative Commons Attribution License (CC BY). The use, distribution or reproduction in other forums is permitted, provided the original author(s) and the copyright owner(s) are credited and that the original publication in this journal is cited, in accordance with accepted academic practice. No use, distribution or reproduction is permitted which does not comply with these terms.
*Correspondence: Qingchuan Chou, chouqc@163.com; Te Cao, caote@ihb.ac.cn
Disclaimer: All claims expressed in this article are solely those of the authors and do not necessarily represent those of their affiliated organizations, or those of the publisher, the editors and the reviewers. Any product that may be evaluated in this article or claim that may be made by its manufacturer is not guaranteed or endorsed by the publisher.
Research integrity at Frontiers
Learn more about the work of our research integrity team to safeguard the quality of each article we publish.