- 1The Water Institute of the Gulf, Baton Rouge, LA, United States
- 2EcoMetrics LLC, Lancaster, PA, United States
Port Fourchon is a vital staging area for Gulf of Mexico energy production and is strategically located in the Barataria-Terrebonne Estuary System, a biologically and economically productive ecosystem bounded by the Atchafalaya and Mississippi Rivers. This is also one of the most fragile and rapidly evolving landscapes in the United States, making the port and surrounding communities highly vulnerable to natural hazards and the impacts of climate change. Building resilience to climate-based disruptions is vital to Port Fourchon and other businesses operating in this dynamic landscape. The port plans to deepen its channel to 50 feet (15 m) to service larger vessels, generating millions of cubic yards of sediment and seeks to beneficially utilize this sediment to develop natural and nature-based solutions to help prepare for future challenges. To accomplish this goal, an Environmental Competency Group consisting of residents, coastal scientists, and key stakeholders was convened to co-develop and evaluate a series of marsh creation projects utilizing this sediment that will maximize social and ecological co-benefits and enhance the resilience of Port Fourchon and the surrounding communities. The group utilized participatory modeling and social return on investment methods to model long-term changes to the landscape and wetland vegetation communities resulting from the co-developed restoration strategies and assess the social value of these strategies. Residents who live and work around Port Fourchon were included in all stages of this research, including development and prioritization of potential restoration areas, identifying important physical and ecological parameters that should be modeled, evaluation of model results, and assessment of the social values expected to be generated by each restoration alternative under consideration. The transdisciplinary approach used in this research highlights the effectiveness of a community-informed, systematic approach to coastal restoration planning in building community resilience and ecosystem sustainability. This study provides approaches and tools that can be adapted for use elsewhere to develop holistic solutions that maximize the social, ecological, and economic co-benefits of coastal restoration.
1 Introduction
Nearly one-third of the United States population lives in coastal counties and this number has been rapidly increasing over the past several decades. Between 1960 and 2008, the population of coastal counties along the Gulf of Mexico alone increased by nearly 150 percent, more than twice the rate of increase of the nation’s population as a whole (Wilson and Fischetti, 2010). Much of this population is heavily dependent upon a range of natural resources found in the coastal zone for their physical and social wellbeing (Hemmerling et al., 2020c). The state’s coastal zone contains approximately 37% of all estuarine marshes in the contiguous United States which provide valuable ecosystem services including water regulation, recreation, fisheries production, carbon sequestration, wave attenuation, and surge reduction (Visser et al., 2012; Batker et al., 2014; Couvillion et al., 2017).
Louisiana is also largely a working coast, with many of its residents relying on the abundant renewable and non-renewable natural resources of the coast for their economic wellbeing. Of the five Gulf of Mexico states, Louisiana has the greatest proportion of coastal employment, with nearly 34 percent of its workforce residing in coastal counties (Adams et al., 2004).A large portion of this employment centers on specific coastal economy sectors, including oil and gas production, commercial and recreational fisheries, marine construction, ship and boatbuilding, tourism and recreation, and marine transportation (Kildow et al., 2014).
More than any other industry, the development of the offshore oil and gas sector has had a tremendous impact on the economic landscape of coastal Louisiana. According to the U.S. Energy Information Administration, Federal waters within the Gulf of Mexico accounts for 15% of total crude oil production and 5% of total consumer-grade natural gas production (U.S. Energy Information Administration, 2022). Coastal Louisiana processes a significant portion of the oil and gas extracted in the Gulf of Mexico and houses infrastructure necessary to support the drilling, shipping, refining and processing of the extracted product (Davis and Place, 1983; Boesch and Rabalais, 1987). The upstream and downstream oil and gas sector directly employs nearly 65,000 workers throughout Louisiana and for every one new job created in these fields, an additional 3.4 jobs are created in other sectors of the Louisiana economy (Scott, 2014).
While it does not employ as many workers as the oil and gas sector, Louisiana’s fisheries support both commercial and charter fishing industries and are a dominant economic driver in many coastal communities. Approximately 20% of all commercial fish and shellfish landings in the United States occur in Louisiana (Barataria-Terrebonne National Estuary Program, 2018). The ecosystems of the Barataria-Terrebonne Basin supports several species of commercial importance such as brown and white shrimp, oysters, and specked trout (Stanley and Sellers, 1986; Patillo et al., 1997; Pattillo et al., 1997; Hijuelos et al., 2017). In 2017, approximately 12.4 million pounds of seafood valued at $13.7 million was landed from Barataria Basin consisting of blue crab, shrimp, oysters, saltwater fish, and freshwater fish (Louisiana Department of Wildlife and Fisheries, 2020). Marine sport fishing also represents an important source of jobs and earnings for many coastal communities. The economic impacts of recreational fishing in Louisiana exceeds $757 million annually and creates nearly eight thousand jobs (McKinney, 2011).
Like other coastal areas around the globe, Louisiana’s coastal zone is becoming increasingly hazardous for coastal residents and workers. Levels of sea level rise, sea surface temperature rise, storm surges, saltwater intrusion, and coastal erosion are increasing and the impacts of tropical storm event coastal communities are becoming more severe. The impacts of coastal hazards events are often compounded by past and present patterns of unsustainable development that have degraded coastal ecosystems and increased social vulnerability (Tu et al., 2022). Louisiana’s coast lost more than 4,800 square kilometers of land from 1932 to 2016, due to a combination of factors, including the effects of climate change, sea level rise, subsidence, hurricanes, storm surges, disconnection of the Mississippi River from coastal marshes, and human impact. From 2004 through 2008 alone, more than 300 square miles of marshland were lost to Hurricanes Katrina, Rita, Gustav, and Ike (Couvillion et al., 2017). Without additional coastal protection and restoration actions, state agencies estimate that anywhere from 1,942 to 6,635 more square kilometers of land could be lost over the next half century (Louisiana Coastal Protection and Restoration Authority, 2017).
Living and working in such a dynamic coastal landscape requires resilience to disruptions based on adaptive, innovative approaches informed by reliable science. Resilience can be broadly conceptualized as a community’s ability to recover to a comparable functional state after an event that disrupts relationships among people and the environment that they inhabit (Colten et al., 2018). Key to this is the development of coastal protection and restoration strategies that enable a community to persist, even if modified, over time. In natural resource-dependent communities such as those found in coastal Louisiana, resilience is often tied to the ability of residents to pursue natural resources in alternate areas or to shift the object of natural resource collection (Colten et al., 2012).
Historically, there has been extensive human intervention to attempt to counter the impacts of climate change and protect Louisiana’s coastal communities and infrastructure (Boesch, 2020). Many of these interventions, such as the construction of the lower Mississippi River flood protection system after the Great Mississippi River Flood of 1927, have had the unintended consequence of altering natural coastal processes and hastening the loss of wetlands. As a result, there is an increasing recognition that future protection and restoration options need to include a number of natural and nature-based options. Due to the high rates of land loss, as well as the high rates of land subsidence, nature-based approaches are likely to be the most effective for increasing community resilience and protection in many resource-dependent communities in Louisiana’s coastal zone. Such approaches include building marshes, marsh terraces, and ridges as well as other methods that increase the intactness of the local ecosystem to maximize ecosystem benefits. These approaches are often necessary in coastal and deltaic environments where hard infrastructure solutions are likely to be impractical or cost prohibitive.
1.1 Transdisciplinary coastal protection and restoration planning
In this study, the Water Institute of the Gulf (the Institute) developed a transdisciplinary approach to coastal restoration planning designed to build community resilience for the communities around Port Fourchon, Louisiana. Port Fourchon, an oil and gas service facility located at the southernmost edge of Lafourche Parish, where Bayou Lafourche enters the Gulf of Mexico, is a critically important hub for many of Louisiana’s coastal economy sectors. The region around Port Fourchon is home to several coastal communities, high-value critical infrastructure supporting the oil and gas industry, and ecosystems that support fisheries and other ecosystem services. Given the port’s location, it is particularly vulnerable to tropical storm events, subsidence, sea level rise, and increasing temperatures (Day et al., 2000; Scavia et al., 2002). The 2020 and 2021 hurricane seasons brought multiple impacts to Port Fourchon and the surrounding areas, including hurricanes Zeta and Ida which both made landfall in the immediate vicinity of Port Fourchon.
Port Fourchon plans to deepen its channels and slips to service larger vessels and seeks to use the resultant dredge material to construct natural and nature-based solutions that will generate multiple co-benefits for the Port, nearby communities, and the regional ecology. This dredging project will initially generate between 13 and 20 million cubic yards of uncontaminated material as well as a smaller, more continuous supply from maintenance dredging (GIS Engineering, LLC, 2018; U.S. Army Corps of Engineers, New Orleans District, 2021). The amount of sediment produced by the dredging activities, and thus the number of different solutions that can be constructed, ranges widely. With the large quantity of sediment that will be available, the possibilities for what to build, how to build, and where to build are numerous. In addition to a full understanding of regional ecosystem dynamics, an understanding of the social and cultural landscape surrounding Port Fourchon is necessary to plan and select the most beneficial option for dredge material placement.
Successful implementation of coastal protection and restoration strategies that strive for resilience require that the accumulated local and traditional knowledge of residents and local stakeholders be taken into account at every stage of project planning (Martinez, 2021). Transdisciplinary research further requires the active participation of scientists, policymakers, residents, and local stakeholders working collaboratively throughout the entire research process, including the co-design of the research and the co-production of knowledge (Newton and Elliott, 2016). This collaborative approach contrasts with the more traditional approach to research, where knowledge is often fragmented by economic sector, region, administrative departments, and scientific expertise (Tu et al., 2022). In the traditional approach, technical science-based knowledge—including devices such as predictive models, risk indicators, monitoring instrumentation, ecosystem services calculations, and benefit cost analyses—is granted priority over local experience-based knowledge, often leading to a disconnect between the lived experience of residents and the outputs of the hydrological and hydraulic science that underpins much of coastal management (Barra et al., 2020).
The transdisciplinary approach utilized in this research, on the other hand, brings individuals from different scientific disciplines and from civil society together to actively collaborate in the co-development a series of natural and nature-based solutions (Krueger et al., 2016). This approach requires local stakeholders and scientists from diverse fields to work on the same problem, plan, and identify solutions that fully integrate local and traditional knowledge with physical and social scientific knowledge. This process adds significant value by leveraging the approaches, knowledge, and principles of the individual disciplines as well as the accumulated local knowledge of residents and local stakeholders. By integrating local knowledge experts into the scientific process, the results are much more likely to be actionable than those developed through traditional research (Bethel et al., 2014). This actionability highlights a key aspect of transdisciplinary research: socially relevant issues, rather than scientific disciplines, define the frame of inquiry (Krueger et al., 2016). The social relevance of transdisciplinary research requires a reconceptualization of public engagement and the traditional role that the public plays in scientific research.
To allow for sustained engagement between residents and local stakeholders from Port Fourchon and the surrounding communities and coastal scientists and engineers, the Institute utilized an environmental competency group (ECG) approach to participatory modeling (Hemmerling et al., 2020a; Baustian et al., 2020; Meselhe et al., 2020). While participatory modeling seeks to integrate scientific knowledge with local knowledge using through use of numerical models, the ECG approach seeks the co-production of knowledge through a redistribution of expertise through the collective investigation of problems (Landström et al., 2011). From the outset of this project, the Institute team has adapted this methodology, directly including industry and other coastal decision makers to develop a fully transdisciplinary process that will result in the construction of a co-developed coastal protection and restoration project. The ECG approach allowed for active dialogue between local knowledge experts and technical knowledge experts and ensured that both sources of knowledge were included and valued throughout the process modeling (Barra et al., 2020).
2 Materials and methods
Participatory modeling is a method of incorporating local stakeholders, including residents, resource users, and decision makers, into a technical science-based numerical modeling process to support formal decision-making involving complex environmental questions. In the participatory modeling process, stakeholders work directly with modelers and other technical knowledge experts to identify the local environmental problems to be addressed and then use modeling to develop and test solutions and to inform the decision-making and actions of the group (Voinov et al., 2018). The incorporation of local and traditional knowledge into numerical models often leads to improved understanding of a system’s interactions and behavior (Voinov and Gaddis, 2008) and planning outputs that are more socially just and responsive to community needs (Hemmerling et al., 2020b).
This research followed a structured, stepwise approach to participatory modeling piloted in an earlier project organized by the Institute (Hemmerling et al., 2020a; Barra et al., 2020; Baustian et al., 2020; Meselhe et al., 2020) to identify the optimal utilization and placement of dredge material. This approach involved convening a series of facilitated ECG meetings with the outputs of each meeting activity serving as inputs to subsequent meeting activities. Adapting Maskrey et al., 2016, the ECG approach to participatory modeling used for this research was structured into four key stages.
Stage 1. A coupled stakeholder-led determination of a set of objectives for enhancing the resilience of Port Fourchon and surrounding communities and analysis of the local and traditional knowledge of residents and local stakeholders necessary to inform the modelling process;
Stage 2. The co-development of natural and nature-based solutions representing the ECG’s shared understanding of the local social and ecological environment and the interactions between interventions and objectives within the study area;
Stage 3. The application of the Coastal Systems Modeling Framework to explore and assess the impact that applying different natural and nature-based solutions (and combinations thereof) had on the objectives;
State 4. A stakeholder-led social value assessment to assess the impacts that applying different natural and nature-based solutions (and combinations thereof) had on communities, regional ecology, and wildlife and fisheries.
2.1 Convening the environmental competency group and establishing objectives
Engagement activities followed a methodology that has been employed in Louisiana by the Institute before and adapted it for this specific location and community. This methodology involved forming a small group of community members and scientists into an ECG that would work together over the course of several meetings to review model inputs, develop model alternatives, and review model results (Hemmerling et al., 2020a; Baustian et al., 2020). A snowball sampling method was employed to select local knowledge experts for the ECG, in which recommendations are solicited from the community and the most frequently mentioned persons are asked to join the group (Bernard, 2017). Technical knowledge experts for the ECG were selected from the Institute based upon their scientific knowledge of the area in and around Port Fourchon. The final ECG membership included local landowners, recreational and commercial fishermen, representatives from the GLPC and its tenants, representatives from local government, and the local Sea Grant representative, as well as modelers, geologists, and ecologists from the Institute (Table 1). Recognizing that the technical knowledge experts in the ECG were being paid by their employers for their time taking part in the meetings, the local knowledge experts were financially reimbursed for each meeting they attended to appropriately acknowledge the value of the time they spent working on the study and providing key data to build and test the numerical models.
The first meeting took place on 18 February 2020, at the headquarters of the GLPC in the town of Cutoff, LA. This introductory meeting was focused on introducing the members of the ECG to one another and discussing the goals of the study with the group. This was also an opportunity for the technical knowledge experts to introduce the group to the types of models that would be developed through this process and what these models are intended to measure. The suite of models presented to the group included a landscape morphology model, a wetland carbon model, a hydrodynamics model, and a storm impacts model. Examples of model outputs for each of these models were presented to the group. In addition to discussing the models to be developed, this first meeting was also an opportunity for the local knowledge experts to discuss the environmental changes that they have seen take place in and around Port Fourchon. Several maps set up around the meeting room served as discussion prompts for the ECG group members.
In this first meeting, steps were taken towards developing a common language and establishing that the knowledge possessed by the technical knowledge experts was not going to be prioritized over the local and traditional knowledge possessed by residents and local stakeholders. It was also necessary to set specific expectations for what can and cannot be accomplished given environmental and economic constraints, including the potential type and magnitude of projects that can be constructed using the limited amount of sediment available. The technical knowledge experts communicated with the local knowledge experts about their prior modeling experience with similar project types across Louisiana. Similarly, the local knowledge experts discussed their experiences living and working and in the area around Port Fourchon. Building an understanding of each group member’s individual background and experience was important for the group to set reasonable expectations for this participatory modeling effort.
In addition to introducing the group members to one another and providing background on the project, the first ECG meeting provided an opportunity for the full group to discuss the expected composition of the dredged material, which is most likely to consist of cohesive fines and organics, with sparse amounts of very-fine sand. Based on their understanding of the expected properties of the dredge material, the ECG collectively decided to limit proposed restoration project types to wetland restoration and construction of intertidal habitat consisting of marsh grasses and mangroves. The group agreed that the composition of the dredge material would likely not be suitable for other project types such as beach, dune, and ridge restoration. At the conclusion of this first meeting, the ECG scheduled the second meeting for the following month, March of 2020.
2.2 Local knowledge mapping and participatory modeling
Qualitative and geospatial data collection efforts centered around a series of local knowledge mapping workshops and subsequent participatory modeling activities. The outputs of these engagement activities were analyzed both qualitatively and quantitatively to translate workshop outputs into geospatial data that could be incorporated into the numerical models that would be used to assess the impacts of the proposed project, with the results reviewed by the local and technical knowledge experts that comprise the ECG.
Local knowledge mapping is an approach that aims to encourage community member participation in sharing knowledge and perceptions of a given area and has been shown to provide an effective means of incorporating community and traditional ecological knowledge into a coastal protection and restoration framework (Curtis et al., 2018). This is traditionally done in a face-to-face setting, using paper maps, markers, and other physical materials. However, weeks after the initial ECG meeting, the first case of COVID-19 was diagnosed in Louisiana and by March of 2020, Louisiana had one of the world’s highest average COVID-19 daily growth rates (Madhav et al., 2020). The state’s governor ordered that all non-essential businesses be closed to the public and issued a stay-at-home order for all residents. As a result, the remaining ECG meetings were held virtually.
The second meeting, originally scheduled to take place in March 2020, was delayed until May of that year as the technical team worked to develop tools to conduct the local knowledge mapping workshops virtually. The team ultimately adapted an online survey software called Maptionnaire, an online public participation GIS portal which allows users to interactively mark-up maps in response to a number of survey questions (Maptionnaire, 2020). The remaining ECG meetings and participatory modeling activities were held virtually, utilizing a combination of Zoom and the online public participation GIS portal. The planned second and third meetings were combined into a single meeting comprised of two segments. During this meeting, the technical team provided an overview of the mapping portal and how to use it and well as the types of spatial data that can be collected. Participants were provided with a link to the mapping site to allow them to continue to add data to the maps on their own.
The first segment provided participants with an overview of progress to date as well as an update on modeling activities that took place between meetings. This included a discussion of many of the technical details related to the models, including mesh size, different sea level rise scenarios, and the period of analysis. A primary goal of this segment was to establish guidelines and set limits on what the technical team was able to model using the tools being developed.
The second segment of the second ECG meeting focused on the different inputs to the model, including bathymetry, topography, vegetation types, and storm impacts. The group was tasked with reviewing the model inputs and to identify inaccuracies in the datasets based upon the members’ local and traditional knowledge of the landscape. Each of the model input datasets were loaded into Maptionnaire, allowing ECG members to mark up and annotate the datasets as necessary. During this segment, using the Zoom breakout rooms function, the ECG divided into smaller virtual breakout groups to engage individual group more fully in deeper conversations and bring out greater details on the data developed through the public participation GIS activities. The breakout groups were organized such that each group had a representative grouping of technical and local knowledge experts. To optimize participant engagement. Breakout group roles were assigned among the technical team members prior to the meeting. A lead facilitator was tasked with guiding the discussion while a map manager navigated the onscreen map in real time, focusing on specific areas being discussed by the ECG. The map manager was also responsible for drawing and annotating the points, lines, and polygons for participants, if they were unable to do so themselves.
Once in the breakout groups, the Institute modeling team presented maps of the model inputs (e.g., elevation maps, vegetation maps) to the full ECG while facilitators guided the conversation between the technical experts and local knowledge experts in order to interrogate the accuracy of the model input data more fully. All conversations were recorded with the permission of the ECG members. This allowed the technical knowledge experts to analyze the qualitative data outputs and link these to the geospatial outputs. Following this meeting, the technical team reviewed the resultant data outputs and adjusted the model inputs as appropriate, providing detailed responses to the full ECG (Table 2). This served dual purposes. First, the process provided a valuable quality control check on the model inputs, utilizing the local knowledge of those who are on the ground every day. Secondly, this transparent process will enhance confidence in the models within the community and trust in the scientists that are developing them (Barra et al., 2020).
The third ECG meeting resulted in the co-development of a series of natural and nature-based solutions that group members felt would provide a balance of social, economic, and ecological benefit to the region around Port Fourchon. The meeting again had to take place virtually and began with a discussion of the types of coastal restoration projects in that have historically been constructed in the region. Following this, the group set about designing a number of wetland restoration projects throughout lower Lafourche Parish that the group felt would maximize social, environmental, and ecological co-benefits. Both the types of projects and locations of project that could be built were discussed. As with the second meeting, the ECG divided into smaller breakout groups with a representative sampling of local and technical knowledge experts in each. In each breakout group, community members and scientists were able to discuss their concerns about different areas of the landscape with the modelers so that, together, they could design potential solutions. Again, using the public participation GIS portal, group members collectively identified locations where sediment placement might generate the most benefit and mapped out a series of generalized project footprints that could be incorporated and tested in the numerical models being developed for this project. Group members all recognized that there are physical and financial limits to what can be constructed given the amount of sediment available.
2.3 Developing the coastal system modeling framework
Following this meeting, the technical team collaborated with other engineers and ecologists from the Institute to refine the generalized project footprints developed in the GIS portal and adapt them to fit the landscape. The Institute assigned geographically appropriate attributes to each project, which assured data comparability with the numerical models being developed by the team. As they were refining each project footprint and fitting to the currently landscape, the modeling team noted all changes made to the initial project footprints and provided explanations and rationale for why these decisions were made. The final footprints used in the modeling were provided to the ECG group member prior to the subsequent social value assessment phase of the research. This process again attempts to reinforce confidence in the modeling and trust in the technical team, as well as the outputs that the models are generating. As the modeling team worked to refine the project footprints, Institute social scientists transcribed and coded the audio from the third meeting, allowing the research team to link the desired outcomes of each proposed project established by the ECG to the geospatial data derived from the project footprints.
The final output of the participatory modeling phases of the project was a GIS database consisting of fully attributed project polygons developed and reviewed by the ECG. In total, 43 feasible sites were identified through this process, consisting of wetland creation and ridge restoration projects. Each project polygon was developed with specific goals in mind, ranging from protection of industrial infrastructure to protection of wildlife and fisheries habitat, to recreational utilization. The ECG meetings were recorded, transcribed, and coded to allow for additional qualitative data analysis. Through this process, the goals and intentions detailed by ECG members during project development were identified and tagged in the GIS.
Once the feasible projects were identified and mapped, the modeling team worked to develop the framework that would be used to efficiently make modeled projections of the ecosystem and landscape around Port Fourchon to assess the effectiveness and sustainability of each co-designed wetland restoration project over a 30-year analysis period. This “Coastal Systems Modeling Framework” was constructed such that important processes were grouped into sub-models that can be run independently while communicating through a reduced number of coupling points. The modeling framework consists of four component models: the Morphology Model, the Coastal Wetland Carbon Model, the Hydrodynamics Model, and the Storm Impacts Model (Figure 1). Each model is a combination of previously developed open-source codes and purpose-built software designed at the Institute. Several of the models employed in this research utilize the Delft3D FM modeling suite principally developed by Deltares. Delft3D FM consists of tools to model the flow of water in coastal settings (D-Flow FM), waves (D-Waves, which is based on the SWAN model), and sediment transport and morphology (D-Morphology).
For this analysis, two modeling grids were employed when using tools from Delft3D FM. The first, the “morph grid” is designed to run more quickly and is used for computationally intensive procedures within the Morphology Model. The second, the “hydro grid,” has more grid cells and provides more spatial detail but takes longer to run. Prior to running the models, the input files were adjusted based on the data collected during the second ECG meeting.
2.4 Calculating the social return on investment of co-designed projects
The co-design process allowed the ECG to identify and delineate the final set of project polygons and numerical modeling determined the physical outputs of these proposed wetland restoration features in terms of acres of land built and maintained, the tonnage of carbon captured, and storm surge reduction. These project outputs do not account for the types of social outcomes that may ultimately determine the success or failure of the projects and their ability to enhance community resilience. These methods do not allow for an accounting of the social value that might accrue to local stakeholder if the projects are implemented. To accomplish this, the technical team used a modified Social Return on Investment (SROI) framework to integrate community-based qualitative research, ecological site assessments, and economic proxies to calculate the social value of candidate projects. SROI is a performance measurement framework that directly accounts for the broad concept of social value, a measure of change that is relevant to people and organizations that experience it. Built upon a combination of traditional cost-benefit analysis and social accounting principles, the SROI process involves a systematic analysis of the effects of projects or programs on communities of interest and key stakeholders, with stakeholder input as part of the data that are analyzed (Nielsen et al., 2021). SROI encompasses a much broader concept of how change is created and valued, moving beyond what can be captured in pure, market-based financial terms. When used to assess environmental change, the process can be employed to help reduce inequality and environmental degradation and improve human health and wellbeing by incorporating social, environmental, and economic costs and benefits into project valuation (Teo et al., 2021).
SROI is classified into two types: evaluative analysis and forecast analysis. In the former, the goal is to evaluate the social value that has already been created by a project while the latter estimates how much social value a project could generate in the future (Teo et al., 2021). Forecast SROIs are especially useful in the planning stages of an activity. They can help show how investment can maximize social impact and are also useful for identifying what should be monitored and measured once the project is implemented (SROI Network, 2012).
For this study, the forecast SROI process was adapted to allow the technical team to focus on potential impacts to key stakeholders who have a direct physical connection to the project. SROI analyses are often used by corporate funders and governmental agencies that have fiduciary responsibility to the public and may include social benefits such as improved company reputation and social license to operate to these funders as key outcomes. While these social benefits would certainly accrue to Port Fourchon and the POWC partners following project construction and implementation, this was not a key focus of this research. Building on the traditional SROI methodology, this process was undertaken in three primary stages.
1. Identify and engage key stakeholders affected significantly by the proposed projects–Understand what each stakeholder wants changed (objectives), what they contribute (inputs), what activities they do (outputs) and what changes for them (outcomes, intended or unintended);
2. Measure and value the social impacts of the proposed projects–Understand the value created because of the changes experienced by each stakeholder group by using indicators to measure the outcomes and financial proxies to value the outcomes; and
3. Create a forecast analysis to measure and evaluate the impacts of the proposed projects–Articulate the key drivers of social value and identify what data are needed to best measure and evaluate the impacts of activities.
2.4.1 Identifying social impacts of co-designed projects though qualitative analysis
Potential social costs and benefits of each proposed wetland restoration project on nearby communities were assessed through qualitative research and stakeholder engagement including one-on-one interviews and questionnaire research. The technical team conducted a series of guided interviews using an option questionnaire to assess the social value that that local stakeholder felt would be generated by each project. To simplify the process and reduce the amount of time that would be required for respondents to assess the projects, the initial projects identified by the ECG were first grouped into four project clusters based upon geographical proximity and project type. Respondents were asked to review the project clusters and also to note if any of the constituent polygons differed significantly from the others in terms of outcomes generated. Initial review of the project clusters found that one proposed liner project feature identified by the ECG located along Louisiana Highway 1, was significantly different from the other in the project clusters, given its location along the primary roadway in and out of Port Fourchon. This single feature was therefore assessed separately from the other project clusters, resulting in five areas of analysis (Figure 2).
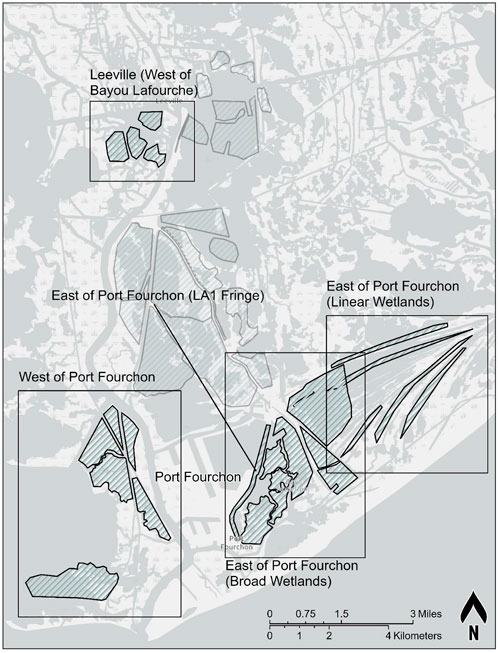
FIGURE 2. Groupings of potential natural and nature-based solutions identified by the ECG and assessed during stakeholder interviews.
The option questionnaire developed to assess each project cluster was constructed around the three broad categories identified through qualitative analysis of the workshop results: impacts on ecology, impacts on wildlife and fisheries, and impacts on human communities (Table 3). Potential project outcomes within each of these categories were identified by the technical knowledge experts in the ECG. Through a guided interview process, respondents were instructed to consider each project grouping separately and determine if that project grouping (or any of the individual constituent projects) will result in an impact. If the respondent determines that there will be an impact, they were instructed to consider each anticipated project outcome and determine the directionality of that outcome (e.g., will the project result in more or less habitats for crabs, shrimp, oysters and fish), its severity, how likely it is to occur, and over what timeframe and spatial scale it would take place. The respondents were also asked if the anticipated project outcome would be beneficial or harmful. Respondents were also given an opportunity to identify any other potential outcomes that were not included in the questionnaire.
In total, 13 interviews were conducted1. Each interview lasted approximately 85 min and ranged from 65 min to 110 min. In addition, one respondent was unable to find time for the interview and opted to fill out the questionnaire on their own. In this case, the respondent was provided with background information on how the project polygons were derived and detailed instructions on how to fill out the questionnaire.
Upon completion of the interviews, the Institute developed a scripted workflow to analyze the option questionnaire spreadsheets. To convert the categorical data into quantitative scores, each attribute rank was translated into a corresponding numeric value, so that low, medium, or high were respectively translated into 1, 2, or 3, respectively. In converting the categorical data for directionality, the scores for each category were then adjusted based upon whether an outcome was perceived to be positive or negative. By converting the responses to numbers, the ranks could then be averaged across all surveys.
The script then computed the average scores for the four assessed attributes (likelihood of occurrence, consequence of impact, how widespread, and over what period). To further refine risk averages, the scores were divided into three groups based on the average score. For outcomes that respondents believed would be negative, averages ranging from 1.0 to 1.67 received an A grade; 1.68–2.33 received a B, and 2.34–3.0 were given a C grade (Table 4). For positive outcomes, the grading scale was reversed, with averages from 2.34 to 3.0 were given aa A grade.
2.4.2 Quantifying the social value of co-designed projects
After the survey results were compiled and each of the project groupings received an initial grade, the technical team worked with partners from EcoMetrics LLC to incorporate these data into a social valuation model that incorporates both the survey outputs and the outcomes of the Coastal Systems Modeling Framework. Using a modified version of the EcoMetrics methodology developed by the Restore the Earth Foundation (REF) and previously piloted by the Institute and REF in coastal Louisiana (Hemmerling et al., 2017a, 2017b), a relative social value score was calculated for each of the project groupings assessed. EcoMetrics, built on the guiding principles of Social Value International’s SROI methodology, provides evidence-based understanding of environmental change for a full range of community stakeholders, recognizing both positive and negative changes as well as intended and unintended outcomes (SROI Network, 2012). The process utilizes data derived from stakeholder engagement to identify environmental, economic, and social costs and benefits resulting from nature-based solutions and quantifies the cumulative costs and benefits of these over time. The SROI calculated by EcoMetrics measures the value of social benefits created by an environmental action in relation to the estimated financial cost of achieving those benefits (Rotheroe and Richards, 2007). This quantification results in the calculation of a final SROI ratio for each of the project groupings. For this research, this SROI ratio is used to rank the project groupings on the basis of anticipated social value.
Value in this context refers to the relative importance placed by a stakeholder group on one potential outcome over another and uses financial proxies as key performance indicators for each of the identified outcomes (Nielsen et al., 2021). In SROI analyses, financial proxies estimate the value of non-monetary social outcomes by identifying the economic cost or benefit of activities that may accrue to different stakeholders as the result of a given social outcome (Kim and Belzer, 2021). The financial proxies were drawn from the literature and used in this analysis to create consistent “importance weights” for each of the potential outcomes of project groupings, based upon the social value that stakeholders placed on each of the analyzed outcomes. To link the outcomes of the social value assessment to the modeling outputs, each of the proxies needed to be geographically based and focused on the amount of land built or lost, including acres of both saltmarsh and black mangrove (Table 5). Where possible, the proxy values were drawn from economic studies of coastal Louisiana (Barnes et al., 2015). In all cases, the values were converted to 2022 US$/ha.
Several caveats should be noted regarding the financial proxies used for this analysis. The selection of financial proxies used in an SROI is an inherently subjective process (Kim and Belzer, 2021). In some cases, there was not a geographically based proxy that perfectly matched an anticipated outcome. In these cases, similar proxies were selected and reviewed by the technical members of the ECG. In other cases, the lack of granularity in some of the proxies necessitated that some be used for more than one outcome. For example, de Groot et al., 2012 provides a single value for the ability of coastal wetlands to moderate the impact of storms and other disturbances. For this research, this value is compounded based upon the anticipated impacts on human communities. For example, a proposed project that will moderate storm impacts on critical facilities, essential facilities, and homes is counted higher than one that only protects homes. While some of the same proxies were used for multiple outcomes, efforts were made to assure that no outcomes were double counted. For example, the question of impacts of storms on port facilities did arise during the ECG meetings. However, since port facilities were already included as part of industrial infrastructure, they were not included separately in the social valuation process, precisely to avoid double counting the same outcome on the same facility. As better or more applicable economic data becomes available, the EcoMetrics model will be updated.
The social value assessment also included an analysis of the social cost of carbon, defined by the USEPA as an “estimate of climate change damages and includes, among other things, changes in net agricultural productivity, human health, property damages from increased flood risk and changes in energy system costs, such as reduced costs for heating and increased costs for air conditioning” (USEPA, 2016). Utilizing the estimated tonnage of carbon calculated during the modeling phase of this research and the social cost values established by the USEPA for each specific year, the social value of carbon sequestered in dollars was calculated for each project grouping4F2. The financial proxies used to assess both the land built and carbon sequestered provided standard metrics by which each of the projects are ranked. To estimate the social value of each of the project groupings, EcoMetrics multiplies the quantity of the outcomes (in either hectares of marsh/mangrove or tonnes of carbon) derived from the Coastal Systems Modeling Framework by the value of the financial proxy and the percentage of deadweight for each outcome is deducted (Teo et al., 2021). These values were estimated and summed across the 30 year period of analysis used in the numerical modeling.
For this analysis, one additional step was taken to account for the expected likelihood and consequence of each outcome among local stakeholders, as determines through the social value interviews and questionnaires. The estimated social value generated by each outcome was weighted based upon the results of the option survey. The percentage of survey respondents identifying that specific outcome, the perceived likelihood of that outcome occurring, and the expected consequences of the outcome were all equally weighted in the final value calculation. If the perceived outcome is a benefit, then that outcome was assigned a positive value. Conversely, if the perceived outcome is a harm, then that outcome was assigned a negative value. The maximum weighted social value would therefore be an outcome that all respondents identified as a benefit with a high likelihood of occurring and a high level of impact. The same scoring rubric was applied to establish the minimum social value, which would be an outcome that all respondents identified as harmful. If no survey respondents identified a specific outcome as a possibility or if all respondents deemed the anticipated impacts of an outcome to be inconsequential, the weighted social value was estimated to be zero. Once the estimated impacts of all outcomes are estimated, the total impact of each project grouping is calculated by summing the results together (Teo et al., 2021). This resultant value represents the net present value of the project grouping, defined in the SROI as the summed costs and benefits paid or received over different time periods (SROI Network, 2012).
2.4.3 Estimated project construction costs
To estimate project construction costs, a series of assumptions was required. These assumptions characterize the shape and elevation of the features, the methods for estimating dredge fill volumes, and the geotechnical properties of the sediment and underlying soils in borrow and placement areas, which impact settlement, subsidence, and cut/fill dredging ratios. The cost of wetland restoration projects in Louisiana is influenced by the type of material to be dredged, the distance from the dredge location to the fill location, fuel costs, and mobilization/demobilization cost. Mobilization and demobilization cost are influenced by project size, borrow source, dredging distance, pipeline corridor, dredging equipment, and dredging volume. Where necessary, the USACE’s Civil Works Construction Cost Index System was used to inflate costs from prior years to present day dollars (USACE, 2021). All costs utilized in the SROI analysis are intended to provide planning level insights under significant uncertainty and are not intended to represent design or bid levels of detail or accuracy.
The final estimated construction costs and the associated maximum weighted social value for each project grouping were used as inputs for a modified version of part of the calculations component of the EcoMetrics model and calculated out to 30 years in the future to coincide with the timeframe of the ecosystem models. This process resulted in a final Adjusted SROI score for each project grouping, a ratio of the expected construction costs to the perceived social benefits and costs estimated using the final weighted social value scores.
3 Results
3.1 Coastal systems modeling framework results
Each proposed wetland restoration project was compared to future without action (FWOA) alternative to assess project performance, the persistence of the project after 30 years, project impact to storm surge and waves, and how it influences habitat changes for the estimation of the net GHG flux (Figure 3). An important note about the project alternative evaluations discussed here is that because multiple wetland restoration projects were modeled together, it is not possible to disentangle the performance or effects of any single project polygon from the others in the same area. Any single location where wetlands are restored is likely receiving some benefit from other restoration areas in its vicinity.
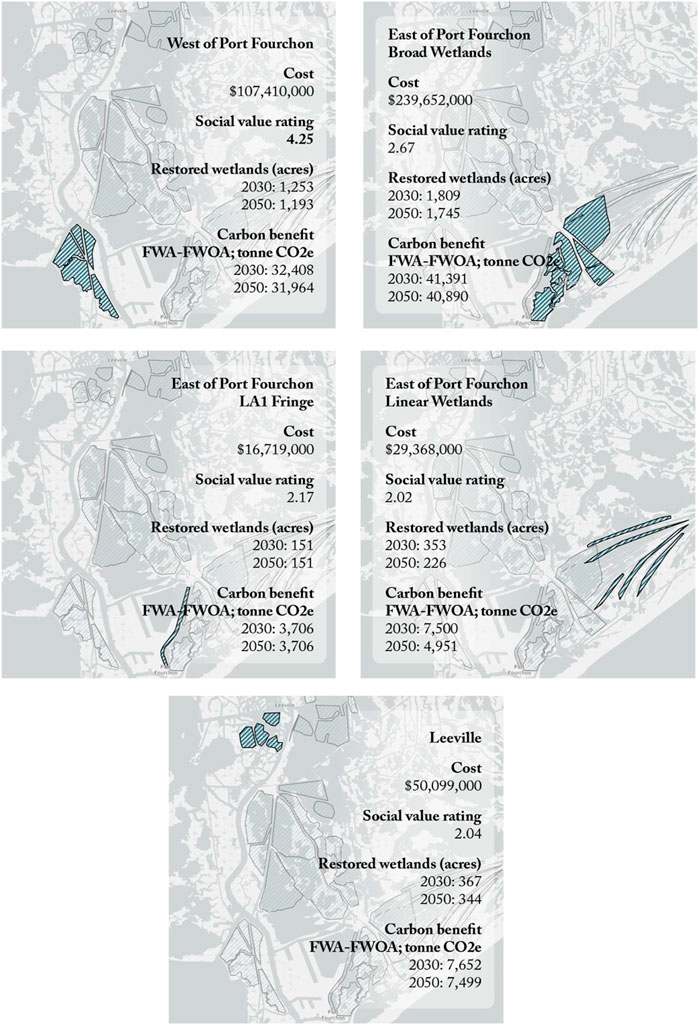
FIGURE 3. Model result for each wetlands restoration project grouping based on model projections from 2020 to 2050.
3.1.1 West of Port Fourchon
The wetlands to the west of Port Fourchon lose land area and transition to a more open water environment between 2020 and 2050 in the FWOA alternative. In the West of Port Fourchon project alternative, the models show that approximately 980 acres of land is created, while only 160 acres are lost by 2050. All wetland restorations remain largely intact in 2050. The wetlands constructed in this area are projected to be nearly 70% mangrove forests which was parameterized to capture more carbon than saline marshes due to the greater aboveground biomass in woody trunks and stems compared to marsh grasses. The wetlands in this area therefore represent a net sink of GHG emissions from years 2020–2050 with a range of −0.03 to −0.04 MMT CO2e. As soon as these projects are constructed (model year 2020) their influence on storm surge and wave heights are observed at the Port. The reductions in peak storm surge heights are modest in 2020 (<5 cm) while the reductions in peak and average wave height are greater (5–25 cm, in the project area). The greatest reductions are seen in the southernmost area where a large open water area was filled.
3.1.2 East of Port Fourchon
Two different sets of wetland restoration projects were modeled for East of Port Fourchon: broad wetland polygons, which are located closer to the Port, and narrow linear wetland polygons near Louisiana Highway 1, the primary road leading to the Port, and along existing ridges. In the FWOA alternative, about 250 acres of land is lost in the area in which the broad wetland polygons would be constructed. The broad wetland restoration project will build nearly 1,800 acres of wetlands, although more than 250 acres of this will be lost by 2050 around the edges of project areas and close to open water areas. These wetlands gained in this area are expected to be a mixture of mangrove forest and both brackish and saline marshes. Model results estimate that these wetlands will be a net GHG sink of −0.02 MMT CO2e. Wave heights are reduced by up to 0.25 m within the project footprint, even when surge heights are increased. However, where increased water levels are observed near the project footprint, wave heights increase. Wave height reductions are minimal, at best, however, for all modeled storms in 2050.
Model results found that the linear wetland features created about 320 acres of land and lost about the same amount of land area over the 30-year period of analysis. These linear features have a larger proportion of edges to interior wetland and thus were more susceptible to edge erosion compared to most other restoration projects modeled. The linear wetlands in this area include a mixture of mangrove forests and brackish and saline marshes that largely remained intact over time. At year 2050, it is estimated that these linear wetland feature will be a net GHG sink of −0.02 MMT CO2e. Wave height reductions from these linear wetland features are expected to be minimal for all storms in 2050. In some cases, wave heights are expected to increase due to increased water depths associated with higher surge allowing larger waves to propagate through the area.
3.1.3 Leeville
Restoration projects north of Port Fourchon near Leeville both nourish existing wetlands and create new wetlands in open water. These projects create over 1,200 acres of land compared to the FWOA alternative and result in about 1,140 additional acres of land within the project footprints. However, these wetlands do not persist on the landscape to 2050 as well as other alternatives modeled. Results show that more land will be lost with action than in the FWOA, likely because construction in open water with only fragments of existing wetlands will increase the area of wetlands able to be lost at this location. The creation of additional wetlands in this area does not result in long-term land gain in the model. Some smaller areas of created wetlands are almost entirely lost over 30 years. Over time, as this area converts back to open water year, it is projected to be a net GHG source of +0.05 MMT CO2e. Similarly, this area is not expected to experience a consistent reduction in storm surge heights and wave heights resulting from construction these wetlands. The magnitudes of the effects are generally small and localized to the project areas.
3.2 Social return on investment (SROI) results
Stakeholders interviewed believe that each of the project groupings will generate positive social outcomes overall, although the project alternatives featuring broad wetlands to the east and west of the port are expected to generate the greatest social value (Figure 4). The West of Port Fourchon project grouping is expected to generate the greatest social return, with almost all respondents believing that utilizing the dredge material for marsh creation in this area will result in an increase in saline marsh and mangrove forest habitat areas. As a result, survey respondents anticipated that bird and mammal habitat will increase. Many respondents also anticipate a concurrent improvement in crab, shrimp, oyster, and fish habitat if this area were restored, suggesting that they view these wetland areas as ecological systems supporting both aquatic and terrestrial species. It should be noted that there was not a consensus on this last point, however, with several respondents noting that they believe this project will harm aquatic habitats. Finally in terms of co-benefits, a majority of respondents believe that this project will reduce wave impacts on both fishing camps and oil and gas infrastructure while providing enhanced opportunities for recreation in the area.
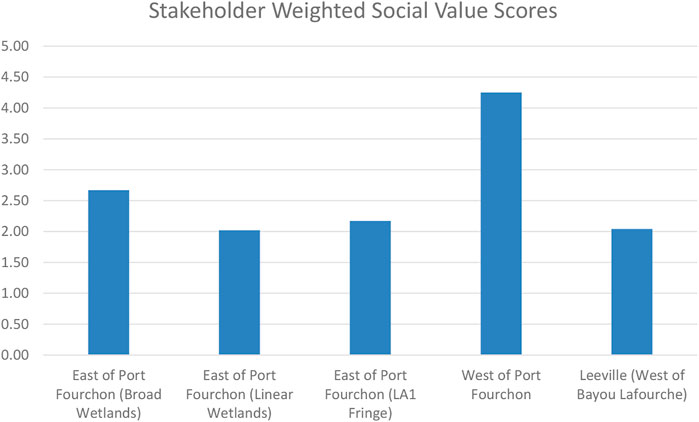
FIGURE 4. Final stakeholder weighted SROI scores for each project grouping analyzed during stakeholder interviews.
The East of Port Fourchon (Broad Marsh) project grouping is expected to have many of the same beneficial outcomes as the West of Port Fourchon project groups with some notable exceptions. Many more stakeholders expect that this project will ultimately harm crab, shrimp, oyster, and fish habitat with a trickledown effect on subsistence, recreational, and commercial fisheries than seen in the West of Port Fourchon results. Conversely, more respondents believe that building marsh in this location will protect more homes, fishing camps, and oil and gas infrastructure than any other project grouping examined here. The additional negative outcomes related to fishers, however, coupled with higher planned construction costs for this area, reduce the overall return on investment for this location.
The third broad marsh features project grouping examined in this study was in Leeville (West of Bayou Lafourche). Like the other broad marsh project groupings, survey respondents expect to see an increase in saltmarsh with fewer anticipating an increase in mangrove habitat (Figure 92). Given that this location is the farthest north of the areas surveyed, it is not surprising that fewer stakeholders anticipate improved mangrove forest habitat here. Beyond this, the results for this area are similar to those seen in the East of Port Fourchon (Broad Marsh) project grouping, with many respondents believing that harm to crab, shrimp, oyster, and fish habitat will result from this project, directly impacting subsistence, recreational, and commercial fisheries. Though not a majority, other respondents believe work in this area will directly impact navigability and boating access and will also result in more invasive species in the area. These negative impacts are balanced out however, by a belief that March creation projects constructed in this area will reduce erosion and also protect homes and camps from storm surge. This project grouping is expected to have the greatest cost per acre to build however, resulting in the second lowest social returns on investment among the project groups analyzed.
The linear marsh and ridge features, including those immediately adjacent to LA one and those further to the east of the proposed broad wetland features, are expected to generate less social value than broader wetland features. In general, the survey results show that the primary anticipated impacts of these projects are on reducing erosion. The East of Port Fourchon (LA one Fringe) feature, a proposed linear feature located directly adjacent to LA one and Port Fourchon itself, is expected to have the added benefit of reducing wave impacts on oil and gas infrastructure. These protection benefits are seen as coming at the expense of access to the area for recreational and subsistence fishing. However, even as stakeholders see minimal benefits of these project for the area’s coastal fisheries, they recognize that they do have the potential to generate saline marsh and mangrove forest habitats, which are valuable for birds and mammals.
Despite having the lowest planned construction cost per acre, the East of Port Fourchon (Linear Wetlands) project grouping is expected to generate the lowest social return on investment of all the project groupings examined. Most stakeholders see this project grouping as having a positive impact on the ecosystem and the wildlife that depend on that ecosystem. Additionally, they recognize that these ridges will result in more saline marsh and mangrove forests and improve bird habitat. They also expect that this project grouping will reduce both daily and storm induced erosion of wetlands, bays, bayous, and canals in the region. However, most respondents do not see this project grouping generating benefits for local residents and communities, nor do they see additional co-benefits to the area’s oil and gas infrastructure.
4 Discussion
For Port Fourchon and the communities in and around Lafourche Parish to remain viable into the future, it is necessary to support both the region’s valuable fisheries and the critical industrial infrastructure that supports offshore oil and gas production. As the Port moves toward improvements that could generate an initial 13 to 20 million cubic yards of sediment, the work set out in this report was initiated to inform the design of potential nature-based wetlands restoration projects using this future sediment resource.
The transdisciplinary approach developed and operationalized in this study resulted in a suite of wetland restoration project alternatives that are all expected to generate a range of ecological and societal co-benefits. The ECG approach to participatory modeling actively encouraged residents and local stakeholders to work with scientists and other technical knowledge experts to co-design a suite of projects that, by their very nature, support local values and concerns (Hemmerling et al., 2022). This work progressed in an iterative fashion, with the full ECG developing and reviewing each of the final project polygons. A key finding of this research is that the collaborative management approach resulted in a suite of project alternatives that are all expected to generate positive social value for stakeholders.
Each project grouping has a unique cost required to transfer the dredge material and construct and maintain the wetlands. The social value rating accounts for these costs as well as the anticipated value of a range of ecosystem, wildlife and fisheries, and human outcomes. These outcome values utilize financial proxies that are based upon model outputs, namely, acres of wetlands restored, and the net carbon flux generated by these wetlands. The social value generated is weighted based on the perceived likelihood and severity of the anticipated changes generated by each project. While still accounting for cost differentials, this stakeholder weighting effectively shifts the valuation process from a more output-based to an outcome-based assessment. As such, while the acres of wetland restored and the net carbon flux are important and easily measurable outputs of these projects, the final valuation looks at the outcomes generated and ways these outcomes will affect residents and the natural resources they rely upon for their sustenance and wellbeing.
For example, the proposed linear wetlands project east of Port Fourchon has the lowest cost per acre to construct and maintain among the project groupings analyzed. A pure cost-based model might be expected to prioritize these linear wetlands over more costly project alternatives. However, qualitative research found that the wetlands restored by this project grouping, while expected to generate a number of ecosystem and wildlife benefits, would not provide as much direct social value for human communities as some of the other groupings. The participation of local knowledge experts in this planning process provided insight into social and cultural values that could not be gained through technical approaches alone, allowing the research team to generate more alternatives, resulting in flexible actions and mutual co-benefits (Stringer et al., 2006; Zedler, 2017).
Finally, there is general agreement between the results of the social valuation model and those of the ecological and hydrodynamic models, highlighting the scientific value of utilizing local knowledge in the coastal planning process. The outputs of the Coastal Systems Modeling Framework identify the West of Port Fourchon project grouping as generating the greatest amount of sustained land building and carbon sequestration relative to project costs. Similarly, the social value assessment, which examines the anticipated positive and negative outcomes resulting from land building and carbon sequestration in each project location, identifies the West of Port Fourchon project grouping as having the greatest social value for residents and local stakeholders.
The final results of this project were presented to the ECG and the leadership of the Partnership for our Working Coast, a public-private partnership comprised of the Greater Lafourche Port Commission and several tenants of Port Fourchon, including Shell, Chevron, and Danos, during a public meeting held at Port Fourchon on 28 July 2022. Following this meeting, the port director announced that the West of Port Fourchon project would be constructed by the port with the generated dredge material. This outcome highlights that coastal planning that is supported by the generation and incorporation of reliable knowledge drawn from both the scientific community and from the local knowledge of stakeholders who live and work in the project area improves the likelihood of effective and sustainable project outcomes.
It is important to note that both the modeling and the social valuation assessment represent future forecasts of change. Forecast assessments and models such as the ones generated for this research are especially useful in the planning stages of an activity. In addition to identifying how planned projects can maximize social impact, forecast assessments based around local engagement are useful for identifying what should be monitored and measured once as project is implemented (SROI Network, 2012). Following the construction of the identified restoration project, project managers should make every effort to conduct periodic evaluative assessments of outcomes, following many of the same stakeholder engagement steps utilized in the forecast assessment. Residents and local stakeholders who live and work around the project site are unique sensors of their environment and often make observations for longer time periods and at finer spatial scales than any scientific instruments (Goodchild, 2007; Curtis et al., 2018). As such, they represent an important source of data for project evaluation and adaptive management of project outcomes.
5 Conclusion and future prospects
Community resilience is closely related to the concept of adaptive capacity, defined as the ability of a system to adjust to change, moderate the effects, and cope with a disturbance (Cutter et al., 2008). In natural resource-dependent communities like those around Port Fourchon, resilience is often tied to the ability of residents to pursue natural resources in alternate areas or to shift the object of natural resource collection (Colten et al., 2012). This ability to shift to alternate areas is particularly important when working with renewable natural resources, such as fisheries. For communities that are reliant upon mineral resources such as oil and gas, where the location of the resource is often fixed, community resilience is more closely tied to the ability to protect those resources, and the infrastructure necessary for their extraction and transport, in place.
The projects co-developed by the ECG through this research were specifically designed to maximize co-benefits, including the protection of the primary natural resources that residents and local stakeholders rely upon, fisheries and oil and gas. Several of these co-benefits were assessed directly through numerical modeling. The ability of the projects to build wetlands, including saltmarsh and mangroves, and to reduce wave impacts on infrastructure was examined for each project grouping. Recognizing that there is a social cost of carbon associated with the release of greenhouse gasses that will impact communities in the future, each project was assessed to determine its ability to serve as a sink or a source. One key resource that the coast requires is a workforce and communities to sustain that workforce. The ECG recognized this, and each of the project alternatives was examined and assessed for its ability to protect surrounding homes and camps from storm surge and flooding.
Beyond providing protection for communities, wetlands are tied to the region’s history and cultural heritage. Restored wetlands can provide enhanced opportunities for recreation and education. These more intangible aspects of coastal protection and restoration are not as readily modeled but no less important when it comes to building community resilience. All of these co-benefits were considered during each step of this research, from the initial conceptualization of the project footprints to the final social valuation and ranking of project alternatives. The framework developed and operationalized through this research represents a key advancement in the collaborative management of coastal protection and restoration planning and provides a framework and tools that can be leveraged to enhance resilience within the study area and adapted for other locations globally.
Data availability statement
The raw data supporting the conclusions of this article will be made available by the authors, without undue reservation. However, full interview recordings and transcripts are considered anonymous and confidential, and therefore cannot be released.
Ethics Statement
Ethical review and approval was not required for the study on human participants in accordance with the local legislation and institutional requirements. Written informed consent from the participants was not required to participate in this study in accordance with the national legislation and the institutional requirements.
Author contributions
SH: Conceptualization, Methodology, Formal Analysis, Writing–Original Draft Preparation; CD, JP: Methodology, Investigation–Qualitative Data Collection; AG, EP: Formal Analysis; MB, CE, IG, and HJ: Methodology, Formal Analysis–Modeling; MMB: Investigation, Methodology, Formal Analysis–Modeling; DL, BM: Formal Analysis, Methodology, Project Administration; MM: Funding Acquisition, Methodology, Project Administration.
Funding
The research was funded by the Partnership for our Working Coast is under Agreement Number POWC-2019-PF2-MM and the National Fish and Wildlife Foundation under grant number 0318.19.065625.
Acknowledgments
Many partners contributed to this study, including Madeline Foster-Martinez and the late Scott Duke-Sylvester for vegetation modeling assistance, and EP for SROI assistance. The Institute would additionally like to thank our partners at Deltares for technical support related to the Delft modeling suite. Through the course of the study, a group of representatives from Port Fourchon and its tenants formed a group termed the Kitchen Cabinet. This group, along with representatives from the National Fish and Wildlife Foundation, was instrumental in providing encouragement, guidance, and feedback for the effort. Last but certainly not least, the Institute would like to thank the residents, local stakeholders, and the Port Fourchon community who participated in the Environmental Competency Group meetings and the social return on investment interviews: Their input was invaluable to the success of this endeavor.
Conflict of interest
Author EP was employed by EcoMetrics, LLC.
The remaining authors declare that the research was conducted in the absence of any commercial or financial relationships that could be construed as a potential conflict of interest.
Publisher’s note
All claims expressed in this article are solely those of the authors and do not necessarily represent those of their affiliated organizations, or those of the publisher, the editors and the reviewers. Any product that may be evaluated in this article, or claim that may be made by its manufacturer, is not guaranteed or endorsed by the publisher.
Footnotes
1Interviews took place during the spring and summer of 2021 and concluded after Hurricane Ida made landfall at Port Fourchon on 29 August 2021, as a category four storm.
2For the SROI valuation, tonnes of carbon was calculated based on GHG flux (sinks) for vegetated habitats only (brackish and saline marsh + mangrove forest) of future with action (placement of dredge material) and future without action.
References
Adams, C. M., Hernandez, E., and Cato, J. C. (2004). The economic significance of the Gulf of Mexico related to population, income, employment, minerals, fisheries and shipping. Ocean Coast. Manag. 47, 565–580. doi:10.1016/j.ocecoaman.2004.12.002
Barataria-Terrebonne National Estuary Program (BTNEP) (2018). Btnep: Comprehensive conservation and management plan. Thibodaux, LA, USA: Barataria-Terrebonne National Estuary Program (BTNEP).
Barnes, S. R., Bond, C., Burger, N., Anania, K., Strong, A., Weilant, S., et al. (2015). Economic evaluation of coastal land loss in Louisiana. Baton Rouge, LA: Louisiana State University, Economics and Policy Research Group.
Barra, M. P., Hemmerling, S. A., and Baustian, M. M. (2020). A model controversy: Using environmental competency groups to inform coastal restoration planning in Louisiana. Prof. Geogr. 72, 511–520. doi:10.1080/00330124.2020.1777574
Batker, D., de la Torre, I., Costanza, R., Day, J. W., Swedeen, P., Boumans, R., et al. (2014). “The threats to the value of ecosystem goods and services of the Mississippi delta,” in Perspectives on the restoration of the Mississippi delta (Dordrecht, Netherlands: Springer), 155–173. Available at: http://link.springer.com/chapter/10.1007/978-94-017-8733-8_11 (Accessed October 6, 2016).
Baustian, M. M., Jung, H., Bienn, H. C., Barra, M., Hemmerling, S. A., Wang, Y., et al. (2020). Engaging coastal community members about natural and nature-based solutions and assessing their ecosystem functions. Ecol. Eng. 143, 100015. doi:10.1016/j.ecoena.2019.100015
Bernard, H. R. (2017). Research methods in anthropology: Qualitative and quantitative approaches. Lanham, Maryland, USA: Rowman and Littlefield.
Bethel, M. B., Brien, L. F., Esposito, M. M., Miller, C. T., Buras, H. S., Laska, S. B., et al. (2014). Sci-TEK: A GIS-based multidisciplinary method for incorporating traditional ecological knowledge into Louisiana’s coastal restoration decision-making processes. J. Coast. Res. 297, 1081–1099. doi:10.2112/JCOASTRES-D-13-00214.1
Boesch, D. F. (2020). “Managing risks in Louisiana’s rapidly changing coastal zone,” in Louisiana’s response to extreme weather: A coastal state’s adaptation challenges and successes. Editor S. Laska (Cham, Switzerland: Springer), 35–62.
Boesch, D. F., and Rabalais, N. N. (1987). Long-term environmental effects of offshore oil and gas development. Boca Raton, FL, USA: CRC Press.
Colten, C. E., Hay, J., and Giancarlo, A. (2012). Community resilience and oil spills in coastal Louisiana. Ecol. Soc. 17, art5. doi:10.5751/ES-05047-170305
Colten, C. E., Simms, J. R. Z., Grismore, A. A., and Hemmerling, S. A. (2018). Social justice and mobility in coastal Louisiana, USA. Reg. Environ. Change 18, 371–383. doi:10.1007/s10113-017-1115-7
Couvillion, B. R., Beck, H., Schoolmaster, D., and Fischer, M. (2017). Land area change in coastal Louisiana (1932 to 2016). Reston, VA: US Department of the Interior, US Geological Survey.
Curtis, J. W., Curtis, A., and Hemmerling, S. A. (2018). “Revealing the invisible environments of risk and resiliency in vulnerable communities through geospatial techniques,” in Tsunamis: Detection, risk assessment and crisis management. Editor A. Barberopoulou (Hauppauge, NY: Nova Science Publishers), 245–273.
Cutter, S. L., Barnes, L., Berry, M., Burton, C., Evans, E., Tate, E., et al. (2008). A place-based model for understanding community resilience to natural disasters. Glob. Environ. Change 18, 598–606. doi:10.1016/j.gloenvcha.2008.07.013
Davis, D. W., and Place, J. L. (1983). The oil and gas industry of coastal Louisiana and its effects on land use and other socioeconomic patterns. Geological Survey (U.S.). Available at: http://pubs.er.usgs.gov/publication/ofr83118 (Accessed March 20, 2015).
Day, J. W., Britsch, L. D., Hawes, S. R., Shaffer, G. P., Reed, D. J., and Cahoon, D. (2000). Pattern and process of land loss in the Mississippi delta: A spatial and temporal analysis of wetland habitat change. Estuaries 23, 425–438. doi:10.2307/1353136
de Groot, R., Brander, L., van der Ploeg, S., Costanza, R., Bernard, F., Braat, L., et al. (2012). Global estimates of the value of ecosystems and their services in monetary units. Ecosyst. Serv. 1, 50–61. doi:10.1016/j.ecoser.2012.07.005
GIS Engineering, LLC (2018). Port Fourchon belle pass channel deepening project, Fourchon, Louisiana, Draft environmental impact statement. Baton Rouge, LA, USA: GIS Engineering, LLC. Available at: http://portfourchon.com/news-events/publications/203docs/.
Goodchild, M. F. (2007). Citizens as sensors: The world of volunteered geography. GeoJournal 69, 211–221. doi:10.1007/s10708-007-9111-y
Hemmerling, S. A., Barra, M., Bienn, H. C., Baustian, M. M., Jung, H., Meselhe, E. A., et al. (2020a). Elevating local knowledge through participatory modeling: Active community engagement in restoration planning in coastal Louisiana. J. Geogr. Syst. 22, 241–266. doi:10.1007/s10109-019-00313-2
Hemmerling, S. A., Barra, M., and Bienn, H. C. (2017a). Restore the Earth foundation reforestation social return on investment report: Pointe-aux-Chenes Wildlife Management Area. Baton Rouge, LA: The Water Institute of the Gulf.
Hemmerling, S. A., Barra, M., and Bienn, H. C. (2017b). Restore the Earth foundation reforestation social return on investment report: Tensas river National Wildlife Refuge. Baton Rouge, LA: The Water Institute of the Gulf.
Hemmerling, S. A., Barra, M., and Bond, R. H. (2020b). “Adapting to a smaller coast: Restoration, protection, and social justice in coastal Louisiana,” in Louisiana’s response to extreme weather: A coastal state’s adaptation challenges and successes. Extreme weather and society. Editor S. Laska (Cham, Switzerland: Springer International Publishing), 113–144.
Hemmerling, S. A., Carruthers, C., Tim, J. B., Hijuelos, A. C., and Bienn, H. (2020c). Double exposure and dynamic vulnerability: Assessing economic well-being, ecological change and the development of the oil and gas industry in coastal Louisiana. Shore Beach 88, 72–82. doi:10.34237/1008819
Hemmerling, S. A., DeMyers, C. A., and Carruthers, T. J. (2022). Building resilience through collaborative management of coastal protection and restoration planning in Plaquemines Parish, Louisiana, USA. Sustainability 14, 2974. doi:10.3390/su14052974
Hijuelos, A. C., Sable, S. E., O’Connell, A. M., and Geaghan, J. P. (2017). Coastal Master Plan: Attachment C3-12: Eastern oyster, Crassostrea virginica, habitat suitability index model. Baton Rouge, LA: Coastal Protection and Restoration Authority.
Kildow, J. T., Colgan, C. S., Scorse, J. D., Johnston, P., and Nichols, M. (2014). State of the US ocean and coastal economies 2014. Available at: http://cbe.miis.edu/noep_publications/1/(Accessed July 26, 2016).
Kim, J., and Belzer, A. (2021). Return on investment for adult basic education: Existing evidence and future directions. Adult Educ. Q. 71, 356–372. doi:10.1177/07417136211002155
Krueger, T., Maynard, C., Carr, G., Bruns, A., Mueller, E. N., and Lane, S. (2016). A transdisciplinary account of water research: Transdisciplinary account of water research. Wiley Interdiscip. Rev. Water 3, 369–389. doi:10.1002/wat2.1132
Landström, C., Whatmore, S. J., Lane, S. N., Odoni, N. A., Ward, N., and Bradley, S. (2011). Coproducing flood risk knowledge: Redistributing expertise in critical ‘participatory modelling. Environ. Plan. A 43, 1617–1633. doi:10.1068/a43482
Louisiana Coastal Protection and Restoration Authority (2017). Louisiana’s comprehensive master plan for a sustainable coast. Baton Rouge, LA: Louisiana Coastal Protection and Restoration Authority. Available at: http://coastal.la.gov/wp-content/uploads/2016/08/2017-MP-Book_2-page-spread_Combined_01.05.2017.pdf.
Louisiana Department of Wildlife and Fisheries (2020). An assessment of the principal commerical fisheries in Barataria Bay and its environs. Baton Rouge, LA, USA: Louisiana Department of Wildlife and Fisheries, Office of Fisheries. Available at: https://www.wlf.louisiana.gov/assets/Resources/Publications/Economics/An_Assessment_of_the_Principal_Commercial_Fisheries_in_Barataria_Bay_and_Its_Environs_2020.pdf (Accessed March 31, 2022).
Madhav, K. C., Oral, E., Straif-Bourgeois, S., Rung, A. L., and Peters, E. S. (2020). The effect of area deprivation on COVID-19 risk in Louisiana. PLoS ONE 15, e0243028. doi:10.1371/journal.pone.0243028
Maptionnaire (2020). Maptionnaire community engagement platform. Available at: https://maptionnaire.com/product (Accessed September 30, 2020).
Martinez, G. (2021). Coastal risk cultures: Local and regional formation of knowledge and action. Front. Environ. Sci. 9, 578238. doi:10.3389/fenvs.2021.578238
Maskrey, S. A., Mount, N. J., Thorne, C. R., and Dryden, I. (2016). Participatory modelling for stakeholder involvement in the development of flood risk management intervention options. Environ. Model. Softw. 82, 275–294. doi:10.1016/j.envsoft.2016.04.027
McKinney, L. (2011). The Deepwater Horizon Oil Spill – Putting a Price on the Priceless. Corpus Christi, TX: Harte Research Institute for Gulf of Mexico Studies.
Meselhe, E., Wang, Y., White, E., Jung, H., Baustian, M. M., Hemmerling, S. A., et al. (2020). Knowledge-based predictive tools to assess effectiveness of natural and nature-based solutions for coastal restoration and protection planning. J. Hydraul. Eng. 146, 05019007. doi:10.1061/(ASCE)HY.1943-7900.0001659
Newton, A., and Elliott, M. (2016). A typology of stakeholders and guidelines for engagement in transdisciplinary, participatory processes. Front. Mar. Sci. 3. doi:10.3389/fmars.2016.00230
Nielsen, J. G., Lueg, R., and Van Liempd, D. (2021). Challenges and boundaries in implementing social return on investment: An inquiry into its situational appropriateness. Nonprofit Manag. Leadersh. 31, 413–435. doi:10.1002/nml.21439
Patillo, M. E., Czapla, T. E., Nelson, D. M., and Monaco, M. E. (1997). Distribution and abundance of fishes and invertebrates in Gulf of Mexico estuaries volume II: Species life history summaries. Washington, DC, USA: U.S. Department of Commerce, Nation Oceanic and Atmospheric Administration, National Ocean Service.
Pattillo, M. E., Czapla, T. E., Nelson, D. M., and Monaco, M. E. (1997). Distribution and abundance of fishes and invertebrates in Gulf of Mexico estuaries, Vol. II: Species life history summaries. Silver Spring, Md: U.S. Dept. of Commerce, National Oceanic and Atmospheric Administration, National Ocean Service. Available at: https://archive.org/details/distributionabun02nels (Accessed January 31, 2014).
Rotheroe, N., and Richards, A. (2007). Social return on investment and social enterprise: Transparent accountability for sustainable development. Soc. Enterp. J. 3, 31–48. doi:10.1108/17508610780000720
Salem, M. E., and Mercer, D. E. (2012). The economic value of mangroves: A meta-analysis. Sustainability 4, 359–383. doi:10.3390/su4030359
Scavia, D., Field, J. C., Boesch, D. F., Buddemeier, R. W., Burkett, V., Cayan, D. R., et al. (2002). Climate change impacts on U. S. coastal and marine ecosystems. Estuaries 25, 149–164. doi:10.1007/bf02691304
Scott, L. C. (2014). The energy sector: Still a giant economic engine for the Louisiana economy - an update. Baton Rouge, LA: Mid-Continent Oil and Gas Association.
Stanley, J. G., and Sellers, M. A. (1986). Species profiles: Life histories and environmental requirements of coastal fishes and invertebrates (Gulf of Mexico)--American oyster. Washington, DC, USA: U.S. Army Corps of Engineers.
Stringer, L. C., Dougill, A. J., Fraser, E., Hubacek, K., Prell, C., and Reed, M. S. (2006). Unpacking “participation” in the adaptive management of social: Ecological systems: A critical review. Ecol. Soc. 11, art39. doi:10.5751/es-01896-110239
Teo, W. S., Seow, T. W., Radzuan, I. S. M., Mohamed, S., and Abas, M. A. (2021). Social return on investment (SROI) for government flood recovery project in kuala krai, kelantan. IOP Conf. Ser. Earth Environ. Sci. 842, 012055. doi:10.1088/1755-1315/842/1/012055
Tu, C., Ma, H., Li, Y., Fu, C., You, Z.-J., Newton, A., et al. (2022). Transdisciplinary, Co-designed and adaptive management for the sustainable development of Rongcheng, a coastal city in China in the context of human activities and climate change. Front. Environ. Sci. 10, 670397. doi:10.3389/fenvs.2022.670397
USACE (2021). Engineering manual number 1110-2-1304: Civil works construction cost index system. Washington, DC, USA: USACE. Available at: https://www.publications.usace.army.mil/Portals/76/Users/182/86/2486/EM%201110-2-1304_2021.pdf?ver=GZ2azRTz_phBs2yb8gDCQQ%3d%3d (Accessed April 3, 2022).
U.S. Army Corps of Engineers, New Orleans District (2021). Dredging/BU spreadsheets. Washington, DC, USA: U.S. Army Corps of Engineers. Available at: https://www.mvn.usace.army.mil/About/Offices/Dredging-BU-Spreadsheets/.
U.S. Energy Information Administration (2022). Gulf of Mexico fact sheet. Washington, DC, USA: U.S. Energy Information Administration. Available at: https://www.eia.gov/special/gulf_of_mexico/(Accessed January 12, 2023).
USEPA (2016). EPA fact sheet: Social cost of carbon. Washington, DC, USA: U.S. Environmental Protection Agency.
Visser, J. M., Day, J. W., Battaglia, L. L., Shaffer, G. P., Hester, M. W., Batzer, D., et al. (2012). “Mississippi River delta wetlands,” in Wetland habitats of north America: Ecology and conservation concerns (Berkeley, CA: University of California Press), 63–74.
Voinov, A., and Gaddis, E. J. B. (2008). Lessons for successful participatory watershed modeling: A perspective from modeling practitioners. Ecol. Model. 216, 197–207. doi:10.1016/j.ecolmodel.2008.03.010
Voinov, A., Jenni, K., Gray, S., Kolagani, N., Glynn, P. D., Bommel, P., et al. (2018). Tools and methods in participatory modeling: Selecting the right tool for the job. Environ. Model. Softw. 109, 232–255. doi:10.1016/j.envsoft.2018.08.028
Wilson, S. G., and Fischetti, T. R. (2010). Coastline population trends in the United States: 1960 to 2008: Population estimates and projectsions. Washington, D.C.: U.S. Census Bureau.
Keywords: participatory modeling, social return of investment, beneficial use of dredged material, natural and nature-based features, coastal restoration planning
Citation: Hemmerling SA, DeMyers C, Parfait J, Piñero E, Baustian MM, Bregman M, Di Leonardo D, Esposito C, Georgiou IY, Grismore A, Jung H, McMann B and Miner MD (2023) A community-informed transdisciplinary approach to coastal restoration planning: Maximizing the social and ecological co-benefits of wetland creation in Port Fourchon, Louisiana, USA. Front. Environ. Sci. 11:1105671. doi: 10.3389/fenvs.2023.1105671
Received: 22 November 2022; Accepted: 10 February 2023;
Published: 23 February 2023.
Edited by:
Lynn Donelson Wright, William and Mary’s Virginia Institute of Marine Science, United StatesReviewed by:
Susan Harrell Yee, United States Environmental Protection Agency, United StatesDouglas Spieles, Denison University, United States
Copyright © 2023 Hemmerling, DeMyers, Parfait, Piñero, Baustian, Bregman, Di Leonardo, Esposito, Georgiou, Grismore, Jung, McMann and Miner. This is an open-access article distributed under the terms of the Creative Commons Attribution License (CC BY). The use, distribution or reproduction in other forums is permitted, provided the original author(s) and the copyright owner(s) are credited and that the original publication in this journal is cited, in accordance with accepted academic practice. No use, distribution or reproduction is permitted which does not comply with these terms.
*Correspondence: Scott A. Hemmerling, c2hlbW1lcmxpbmdAdGhld2F0ZXJpbnN0aXR1dGUub3Jn