- 1College of Food science and Engineering, Hainan University, Haikou, China
- 2Key Laboratory of Tropical Fruits and Vegetables Quality and Safety for State Market Regulation, Hainan University, Haikou, China
- 3Sanya Nanfan Research Institute of Hainan University, Hainan Yazhou Bay Seed Laboratory, Sanya, China
- 4College of plant protection, Hainan University, Haikou, China
- 5Rubber Research Institute, Chinese Academy of Tropical Agricultural Sciences, Haikou, Hainan, China
Pesticide residues have been one of the food safety problems that plague consumers. It is necessary to develop validated detection methods to monitor pesticide residues in food. In this study, fluopyram was analyzed in fruits (banana, grape, and citrus) and vegetables (tomato, cucumber, cowpea, pepper, eggplant, and potato) by optimizing the QuEChERS in combination with GC-MS/MS. The recoveries of fluopyram in all food matrices ranged from 87.02% to 101.42% with RSD below 9.25%. The matrix effect of fluopyram ranging from −1.41% to 17.67%. Finally, this market investigation resulted in a total of 19 positive samples out of 128 market samples, all of which fell below the MRL with the exception of one tomato sample, which was above the EU MRL. Field trial of fluopyram on cowpea was conducted, the half-lives of fluopyram was 3.03–3.95 days, terminal residues ranged from .031–.596 mg/kg. Dietary risk assessment was performed on cowpea. The result indicates that the dietary risk of fluopyram in cowpeas is acceptable. The method of detection developed in this study could enable better monitoring of fluopyram residues in foodstuffs.
1 Introduction
Pesticides are frequently used in modern agriculture to protect crops from pests, weeds and pathogenic bacteria. However, the dietary risks and ecological pollution caused by pesticides have been extensively documented (Duan et al., 2016; Deng et al., 2020; Duan et al., 2021). Consuming pesticide-contaminated food is a major food safety hazard, and some pesticides even have carcinogenic, teratogenic and mutagenic risks (Hotchkiss, 1992). Therefore, the analysis and detection techniques of pesticides have gradually become an important research field, especially of the new pesticides developed in recent years, whose hazards are still unclear during long-term exposure and application.
Fluopyram (N-[2-[3-chloro-5-(trifluoromethyl) pyridin-2-yl] ethyl]-2-(trifluoromethyl) benzamide) is a new succinate dehydrogenase-inhibiting fungicide. It is an effective fungicide applied to cucumbers, tomatoes, cowpeas and other crops by spray and drip irrigation. In animal thyroid tumor studies, fluopyram has been reported to produce toxicity, which induces carcinogenesis in the thyroid gland, and leads to an increased risk of developing tumors (Rouquié et al., 2014). In addition, fluopyram has a significant negative impact on soil microorganisms, especially respiration and biomass, leading to serious changes in the microbial community that are difficult to recover in the short term (Zhang et al., 2014). Maximum residue limits (MRL) for fluopyram have been established in many countries and regions to ensure food safety. For example, China has set MRL for fluopyram in commonly used crops (.01–2.00 mg/kg) (GB 2763–2021). The European Union has set MRL of .01–1.00 mg/kg for most fruits and vegetables (European Commission Pesticides database, 2020). To ensure that agricultural production complies to the MRL, the establishment and development of analytical methods for pesticides is particularly critical. Related studies have already reported the detection of fluopyram. Yogendraiah Matadha et al. (2021) developed the determination of fluopyram in pomegranate for the study of the dissipation process. The analytical method for fluopyram in tomatoes and cucumbers was established by Vargas Pérez et al. (2020) in their study of the residual behavior of fluopyram in greenhouses. Previous analytical method was applicable to fewer foodstuffs and could not complete the monitoring of different fruits and vegetables. According to the properties of fluopyram, it is essential to establish an analytical method that is applicable to a wide range of foodstuff matrix to enable accurate detection and monitoring.
Fluopyram has been widely used for disease and nematode control on tomatoes, grapes and other fruits and vegetables. Proffer et al. (2013) mention fluopyram as one of the most effective methods for controlling leaf spot and powdery mildew on cherries. Fluopyram plays a huge role in the treatment of grapes grey mould and alternating with other pesticides can reduce the development of resistance (Vitale et al., 2016). In addition, Ji et al. (2019) reported that fluopyram was significantly more effective than abamectin in controlling the southern root-knot nematode in tomatoes and could be used for root-knot nematode control in tomatoes. He et al. (2022) found that fluopyram slowed peel browning by inhibiting respiration and increasing glutamate dehydrogenase activity in litchi peel. The current study also indicates that fluopyram is of great potential for application on fruits and vegetables.
In recent years, common methods for the detection and quantification of pesticide residues in fruits and vegetables are indicated GC and LC, and the selection of a corresponding detector for the detection and analysis according to the physicochemical properties of pesticides (Narenderan et al., 2020). Traditional gas and liquid chromatographic methods are inadequate to provide reliable analytical results due to insufficient sensitivity for trace analysis, and sometimes even for MRL of pesticides. Therefore, there is a need to develop new alternative methods in combination with mass spectrometers to improve the sensitivity and accuracy of pesticide residue detection in fruits and vegetables. For example, applying mass detection on the foundation of traditional detection techniques, the method can become more sensitive and accurate. GC-MS/MS with selected ion monitoring mode (SIM) and selected reaction monitoring mode (SRM) greatly reduce matrix interference and occurrence of false positives by detecting ion pairs of target compounds (Ly et al., 2020). A well-defined pre-treatment method is essential when faced with complex foodstuff matrices. Recently, QuEChERS methods have come into play in the field of environmental monitoring, pesticide residue analysis and food analysis due to its simple operation steps, short extraction time and less solvent use (Narenderan et al., 2020; Gabardo et al., 2021; Zhou et al., 2021). For complex matrix, such as those containing large amounts of chlorophyll or sugars, QuEChERS should be adjusted and modified to varying degrees (adsorbent type, adsorbent dosage, etc.).
Natural degradation is the main degradation pathway of pesticides in crops, and environmental factors such as light, temperature and moisture are among the most important factors in the dissipation of pesticides in agricultural systems. Mukherjee et al. (2018) studied the ablation of pretilachlor and butachlor at three CO2 levels and temperatures and found that the half-lives of both herbicides shortened to varying degrees as the CO2 concentration increased. The half-lives of the two pesticides at 40°C were 9.7 and 19.4 days, respectively, which were nearly half as short as those at 25°C (16.2 and 26.7 days). Kwon and Armbrust (2006) found that chlorothalonil was nearly 90% degraded in water/sediment after 24 h under simulated sunlight conditions. In addition, pesticides are broken down into other substances by plants, microorganisms and animals. Microbial degradation is the more common mode of abatement (de Albuquerque et al., 2018). Microbial degradation is considered to be a more promising method for degrading pesticides (Huang et al., 2018). Yuan et al. (2021) found that Lactobacillus plantarum was able to rapidly degrade 81.28% of dimethoate in milk through phosphatase during milk fermentation. In agricultural production, differences in application methods can also slightly influence the process of pesticide dissipation in agricultural systems. Fu et al. (2020) applied acetamiprid and cyromazine at different doses and frequencies to cowpea fields and showed that dissipation of both pesticides slowed down with increasing dose and frequency of application. Similar findings were made by Sakthiselvi et al. (2020) where three concentrations of indoxacarb (60, 90 and 120 g a.i./ha) were applied to tomatoes and the half-life gradually increased with increasing concentration. Plants also play a vital role in the dissipation of pesticides. An example of this is the growth dilution effect caused by plant growth. Not only does plant growth reduce the proportion of pesticide residues in plant material, but vigorous plant growth is accompanied by a faster metabolism, which accelerates the metabolic transformation of pesticides within the plant. Wang et al. (2022) reported that the dissipation rate of emamectin benzoate in tender cowpeas was faster than that in old.
The QuEChERS pretreatment method combined with GC-MS/MS is a convenient and safe detection method. There is still a lack of GC-MS/MS assays for the detection of fluopyram in a variety of fruit and vegetable matrices. In this study, we screened suitable adsorbents by comparing the adsorption effectiveness of seven adsorbents (amino multi-walled carbon nanotubes (MWCNTs-NH2), multi-walled carbon nanotubes (MWCNTS), hydroxylated multi-walled carbon nanotubes (MWCNTs-OH), GCB, C18, PSA and acidic alumina (ALO). The selected adsorbents were used in a modified QuEChERS method combined with GC-MS/MS to detect fluopyram residues in nine foodstuffs (tomato, cucumber, cowpea, pepper, eggplant, potato, banana, grape, and citrus). Among the foodstuffs, field trials were conducted on cowpeas to investigate the dissipation and residue behavior of Fluopyram, which was used to assess the dietary risk of Fluopyram. The aim of this study was to improve the efficiency of monitoring fluopyram in various vegetables and fruits by shortening the analysis time of pesticide residues, which provides theoretical basis and technical support for scientific use of pesticides.
2 Materials and methods
2.1 Reagents and Apparatus
Fluopyram standard was purchased from Shanghai Macklin Biochemical Technology Co. (Shanghai, China). Acetonitrile, ethyl acetate and ethyl acetate were purchased from Xilong Chemical Co. (Shenzhen, China). Acetone was purchased from Guangzhou Chemical Reagent Factory (Guangzhou, China). Sodium chloride was purchased from Beijing Chemical Reagent Company (Beijing, China). PSA and GCB were purchased from Shanghai Ampoule Experimental Technology Co. (Shanghai, China). MWCNTs, MWCNTs-NH2, C18, MWCNTs-OH and ALO was purchased from Shanghai Macklin Biochemical Technology Co. (Shanghai, China). The rotary evaporator was purchased from Shanghai Shensheng Technology Co. (Shanghai, China). Vacuum pump purchased from Wenshi Vacuum Equipment Co. (Linhai, China). The H1850R centrifuge was purchased from Xiang Yi Centrifuge Co. (Hunan, China).
2.2 Analysis method
2.2.1 Solution preparation
Fluopyram standard stock solution (1000 mg/L, ethyl acetate) was configured using a solid standard of fluopyram. The fluopyram standard was weighed 10.0 mg (accurate to .0001 g) and added to 10.0 ml volumetric bottles, fixed with ethyl acetate and stored at −20°C. The standard working solutions (10.0 mg/L and 1.0 mg/L) diluted with ethyl acetate were placed in the refrigerator at 4.0°C until use.
2.2.2 Sample preparation
The sample was weighed 5.00 g and deposited in centrifuge tube (50.0 ml). Added 10.0 ml of acetonitrile and mix the sample for 5 min using a high-speed homogenizer. Afterwards, sodium chloride was accurately weighed 5 g and added to the centrifuge tube and vortexed for 3 min, followed centrifuge the processed tubes for 5 min (5000 r/min). Then supernatant (2 ml) was added to 5.0 ml centrifuge tube containing purifying agent and anhydrous MgSO4 and vortexed for 5 min and centrifuged at 5,000 r/min for 10 min. Removed 1 ml of supernatant from centrifuge tube and rotary evaporated at 40°C, then was re-dissolved with ethyl acetate and subsequently injected into the GC-MS/MS system. Sample preparation flow chart in Supplementary Figure S1.
2.2.3 GC-MS/MS
The analysis of fluopyram was carried out on a Thermo Fisher gas chromatograph equipped with a TRACE 1300 gas chromatograph and a TSQ 9000 triple quadrupole mass spectrometer. The column was a Thermo Fisher TG-5SilMS weakly polarised capillary column (30 m × .25 mm×.25 μm). The programmed ramp-up was started at 120°C and held for 2 min, then increased to 300°C at a rate of 30°C min−1 and held for 2 min, for a total run time of 10 min. The transmission line temperature and ion source temperature were both 280°C. The mass spectrometer sampled electron ionization (EI) for the quantitative analysis of fluopyram in SRM. Two transitions were used for quantification and qualification, with 144.9 > 95 (14 eV) and 144.9 > 75 (20 eV) for fluopyram.
2.3 Method validation
2.3.1 Linearity and recovery of the method
The established method for the determination of fluopyram in fruits and vegetables was evaluated in terms of linearity, accuracy and precision according to the document SANTE/11312/2021 (European Commission, 2021). Linearity was evaluated by linear fitting of fluopyram measurements for all foodstuffs matrix at seven concentration levels. The analysis was considered accurate when R2 > .99 (Li et al., 2021).
Accuracy was verified by spiking experiments at three concentration levels. Fluopyram was added to blank matrix of nine fruits and vegetables for spiked recovery at levels of .01, .2, and 2 mg/kg in grapes, tomatoes, and peppers; and .01, .1, and 1 mg/kg in the remaining fruits and vegetables. The treatments were performed according to the extraction method in Section 2.2.2. The spiking experiment was repeated three times for each level and the relative standard deviation (RSD) was calculated to verify the precision.
2.3.5 Matrix effect
Matrix effects (ME) can affect the reliability and sensitivity of analytical methods. The ME of fluopyram in each matrix was calculated using the following formula (Zaidon et al., 2019):
A and B represent the slope of the standard curve under ethyl acetate solution and blank matrix solution, respectively.
2.4 Actual sample analysis
2.4.1 Market sample collection
Market samples are collected and analysed in order to verify the reliability of the detection method in the application process. The samples are randomly selected and are representative. 128 samples were collected from the market in Sanya, Hainan Province, during June and July 2022. The market samples were analysed for residues according to the established detection method to count the residues of fluopyram in fruits and vegetables.
2.4.7 Field trial
In addition to market samples, residue analysis was performed on field samples as an example of cowpea samples. The field trial was conducted in Sanya, Hainan Province, China (18°23′N, 109°9′E). Cowpeas were selected for the dissipation and terminal residue tests, with an area of 100 m2 (20 m × 5 m). A buffer zone of 2 m width was divided between adjacent test areas. The field trials referred to NY/T 788-2018 (Guidelines for pesticide residue trials in crops) issued by China.
Dissipation: 41.7% fluopyram suspension was applied at 62.55 g a.i./ha (the maximum recommended dose). The dissipation experiment was applied when the cowpea fruits were halfway through growth to mature fruit. Cowpeas were collected in the upper, middle and lower layers of the cowpea plants, respectively. Cowpea samples were collected randomly for analysis at 0 (2 h), 1, 3, 5, 7, 10, and 14 days after application. All samples were labelled and stored at −20°C for further analysis. The dissipation of fluopyram in cowpea samples from field was delineated by a first-order kinetics model.
Terminal residues: The applied doses included a low dose of 62.55 g a.i./ha and a high dose of 93.825 g a.i./ha. Fluopyram was applied three or four times. The spraying interval of fluopyram was 7 days. Cowpea samples were collected at 5, 7, and 10 days after the last application. The picked cowpea were briefly processed and stored in a −20°C refrigerator.
2.5 Risk assessment
The long-term dietary risk and short-term dietary risk of fluopyram were assessed using national estimated daily intakes (NEDI) and risk quotients (RQ) based on data published by WHO GEMS/FOOD (Global Environment Monitoring System/Food Contamination Monitoring and Assessment Programme) in combination with the median residue values for fluopyram, the MRLs and the acute reference dose (ARfD). The calculation formula is as follows (Institute of Quality Standards and Testing Technology for Agro-products and Chinese Academy of Agricultural, 2007):
The above formula is used for long-term risk assessment, where STMR represents the median pesticide residue; Fi represents the dietary reference intake of a food for Chinese residents; bw1 represents the average body weight of Chinese residents (63 kg) (Wang et al., 2022); and ADI is the allowable daily intake of fluopyram, ADI = .012 mg/(kgbw).
The above formula is used for short-term dietary risk assessment. HR represents the maximum residue level; LP represents the large meal consumption of food (.3882 kg); U represents the quality of individual products in terms of edible portions (.0194 kg); v is the coefficient of variation, usually taken as three; bw2 represents the average body weight of our population of different sexes at all ages (Wu and Li, 2015). ARfD stands for acute reference dose, and fluopyram has an ARfD of .5 mg/(kgbw). Typically, %ARfD >100 indicates an unacceptable short-term exposure risk; conversely, it indicates an acceptable short-term dietary risk.
2.6 Statistical analysis
All the analysis was performed in triplicate. The results are expressed as mean and RSD. All analyses were performed using Microsoft Excel 2016 and IBM SPSS Statistics 23. Significant difference procedures were reported at p < .05 level. The graphics were drawn using Origin 2018.
3 Results and discussion
3.1 Optimization of QuEChERS method
3.1.1 Extraction solvent selection
In the analysis of pesticide residues, the selection of a suitable extraction solvent can be effective extraction of pesticides from the foodstuff matrix, the commonly used organic solvent extractants include acetonitrile, acetone and ethyl acetate (Dušek et al., 2020; Maragou et al., 2020; Tong et al., 2021). Three different extraction solvents were added to the nine foodstuff samples, and spiked recovery tests (.1 mg/kg) were conducted to screen the suitable extraction solvents. The results in Figure 1 showed that the highest average recovery among the nine fruits and vegetables was obtained from acetonitrile (93.91%), followed by acetone (91.12%), while ethyl acetate was the least effective extraction (85.39%). However, the more polar acetone may extract more impurities that would interfere with the analysis of the target compound (Zhou et al., 2021), and that ethyl acetate did not perform satisfactorily in grape (77.56%) and eggplant (76.66%), acetone and ethyl acetate were not suitable as extraction solvents for fluopyram. The extraction of acetonitrile was satisfactory. Therefore, the extraction study of acidic acetonitrile was not performed in this study. In several previous studies, acetonitrile was also used for the extraction of fipronil from meat products and acetamiprid from cowpea (Fu et al., 2020; Han et al., 2021). Therefore, acetonitrile was chosen as the extraction solvent for fluopyram for the further study.
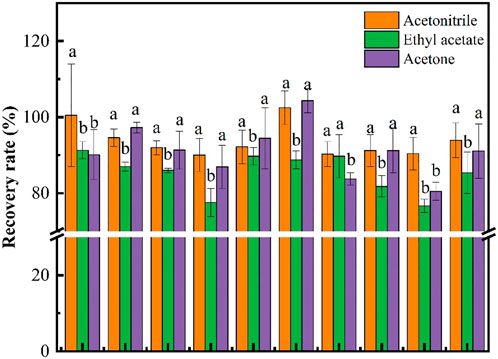
FIGURE 1. Extraction effect of three extraction solvents in matrices. Different letters indicate significant differences (p < .05) among the treatments.
3.1.2 Screening the adsorbent
The choice of sorbent for QuEChERS could vary depending on the analyte being used. Fruit and vegetable matrices are rich in pigments, water, polysaccharides and acids, a comparison of seven purification agents, including MWCNTs, MWCNTs-NH2, MWCNTs-OH, PSA, GCB, C18, and ALO, was used to select suitable purification agents for the next step in the study. These adsorbents have been used extensively for the pretreatment of pesticide residues in foodstuffs (Gonzalez-Curbelo et al., 2013; Chen et al., 2014; Chen et al., 2019; Chen H. et al., 2021).
The sorbents were compared at the .1 mg/kg level of fluopyram additive, the results are shown in the Supplementary Table S1, where the extraction of the seven sorbents in different fruit and vegetable matrices varied considerably. The recovery was unsatisfactory in different food matrices when any adsorbent was used alone. For example, the highest recovery in banana was only 71.35%, and in grapes the highest recovery was only 80.90% except for C18 and MWCNTs-NH2. However, in cucumber, which was a relatively simple matrix, the lowest recovery of all adsorbents was 85.20%, and all were in the range of 80%–110%.
As can be seen from Figure 2, the adsorption of GCB, MWCNTs and ALO was not satisfactory and the average recovery was below 80%, especially for banana, which were only 57.60%, 55.86% and 61.10% (Supplementary Table S1). The average recovery of PSA, C18, MWCNTs-NH2 and MWCNTs-OH were all in the range of 80%–110%. PSA contains amino functional groups and is effective in adsorbing acids from fruit and vegetable matrices. C18 can produce relatively high adsorption of non-polar compounds through van der Waals forces (Wang et al., 2019; Liu et al., 2021). MWCNTs-NH2 and MWCNTs-OH has a strong adsorption effect on interfering substances due to their large specific surface area (Pallavi et al., 2021). After a comprehensive evaluation, PSA, C18, MWCNTs-NH2 and MWCNTs-OH were selected for combined adsorption in this study to determine the optimum adsorbent formulation.
3.1.3 Optimizing the adsorbent
PSA, C18, MWCNTs-OH, and MWCNTs-NH2 were identified as adsorbents for optimization. Considering the similar functions of MWCNTs-NH2 and MWCNTs-OH, both of which have good pigment removal, two combination formulations were designed, as shown in the Table 1. In previous studies, QuEChERS has mostly used 50 mg of sorbent (Fu et al., 2020; Zhou et al., 2021; Wang et al., 2022), so this study has added 50 mg of each sorbent.
To verify the reliability of two methods, three levels of spiked recovery tests were designed in nine food matrices, with the spiked levels containing MRL. The results of the comparison of the recoveries at the three fluopyram levels are shown in Table 2. Method 1 showed a range of 70.38%–108.31% with RSD of 1.17%–8.25% at the three spiked levels; Method 1 showed a range of 87.02%–101.42% with RSD of .81%–8.81%. The recoveries and RSD of both methods were in accordance with the SANTE guidelines to analyse the pesticides residues. However, Method 1 is not as satisfactory as Method 2 in grapes and bananas. The recoveries of Method 1 were 70.38% (.01 mg/kg) and 78.19% (.1 mg/kg) in grapes and 78.46% (.1 mg/kg) in bananas. Method 2 had the lowest recovery of 87.02% across all foodstuffs. What is clear is that Method 2 is a better method. This may be because MWCNTs-NH2 contains more amino groups than MWCNTs-OH (Chen S. et al., 2021), which can additionally adsorb more acidic substances and ensure a more stable pretreatment method. It has been reported that the higher number of nitrogen atoms in MWCNT-NH2 can form hydrogen bonds with the hydrogen atoms in other interfering substances and thus adsorb more interfering substances (Yu et al., 2020). Therefore, Method 2 was chosen as the final pre-treatment method in this study.
3.2 Method validation
Each blank matrix was treated by selected pre-treatment methods and the resulting blank matrix solutions were diluted into a series of matrix standard solutions at concentrations of .01, .02, .05, .1, .2, .5, and 1.0/2.0 mg/L. Linear regression of the measured data for fluopyram concentrations was performed using Excel, fluopyram has a satisfactory linearity in every matrix and the R2 was >.999 (Table 3).
Three parallel recovery experiments were carried out on blank matrix samples at three spiked levels to validate the reliability of the method, and the recovery of fluopyram ranged from 87.02% to 101.42% and RSD between .81% and 9.25% (Table 3), in accordance with the requirement of SANTE guideline, indicating that the method can meet the requirements for routine analysis of the target compounds. The lowest concentration of the recovery experiment was taken as LOQ (for all matrices this is .01 mg/kg). As shown in Figure 3, fluopyram was detected in each matrix without interference from other compounds.
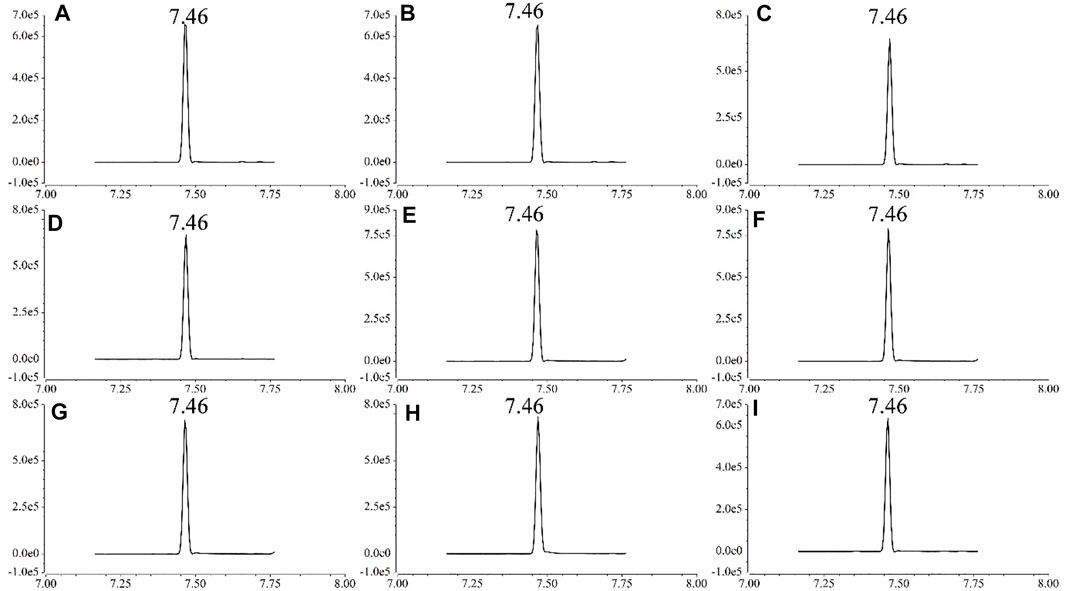
FIGURE 3. GC-MS/MS chromatograms of foodstuff samples spiked with 2 mg/kg fluopyram [(A) eggplant; (B) citrus; (C) banana; (D) cowpea; (E) tomato; (F) cucumber; (G) chili; (H) grape; (I) potato].
The standard curves were established using pure solvents cannot be used for residue analysis and detection in actual samples, because the matrix effects could enhance or diminish the response of the instrument to the target (Han et al., 2021). The matrix standard curve and matrix effect were listed in Table 3. It was found that the matrix effect of fluopyram was acceptable in all matrices, with all resulted ranging from −20% to +20% (−1.41% to −17.67%), which could be considered as a better QuEChERS method (Walorczyk, 2014). Yogendraiah Matadha et al. (2021) and Vargas Pérez et al. (2020) have both developed methods for the detection of fluopyram, but only for one or two food matrices. There are limitations in the practical application. In contrast, the method developed in this study is applicable to nine food matrices and can be better applied to the monitoring of pesticide residues. In summary, the method is reliable and can fulfill the demands of pesticide monitoring and analysis. The MRL of fluopyram in foodstuffs registered by countries and organizations are shown in Supplementary Table S2.
3.3 Actual sample analysis
3.3.1 Residue of fluopyram in the market sample
Based on the analytical method developed in this study, a total of 128 fruit and vegetable samples were tested for fluopyram residues in June and July 2022 in Sanya City, Hainan Province (Table 4), with a total of 19 positive samples. There were 10 cases of cucumber, 6 cases of cowpea and 3 cases of tomato. All samples did not exceed the limit according to GB 2763-2021, but one sample of tomato exceeded the EU MRL (.5 mg/kg).
3.3.2 Residue behavior of fluopyram in field cowpea
The samples from the field trials were analysed using the established method and the dissipation of fluopyram on cowpea was in accordance with the first order kinetic model. As shown in Figure 4, the half-life of fluopyram in cowpea plants ranged from 3.07 to 3.95 days. The half-life values reported in this study were similar to those of mango under similar treatments. On mango fruit, the half-life of fluopyram was 4.3–5.4 days (Mohapatra et al., 2018). This indicated that the persistence of fluopyram was weak in cowpea. The half-life of fluopyram in watermelon was 6.48–6.60 days (Dong and Hu, 2014). The dissipation in cowpea is faster than that in watermelon. The main reasons for this may be caused by differences in climatic conditions and crop types.
Apart from this, the half-life of fluopyram in cowpea was 3.07 days in the upper layer and 3.03 and 3.95 days in the middle and lower layers, respectively (Figure 4). The dissipation rate of cowpea in the upper and middle layers was higher than that in the lower. Cowpeas in the upper and middle layers have a stronger tendency to grow and a faster metabolism. Thus, there was a higher growth dilution effect than the lower cowpeas. Pesticides are generally degraded gradually as plants grow, which is one of the significant pathways for pesticide dissipation. Similar results have been reported in previous studies that significant differences in pesticide dissipation rate between tender and old cowpeas (Wang et al., 2022). In addition, environmental factors such as sunlight, temperature and humidity also play a fundamental role in the dissipation (Farha et al., 2016). For example, pesticides on greenhouse crops commonly dissipate more slowly than in the open due to lack of light and confined space (Chen H. et al., 2021). Upper and middle cowpeas have more exposure to sunlight, which promoted faster dissipation of fluopyram.
As shown in Table 5, the terminal residues of fluopyram in cowpea ranged from .031–.596 mg/kg. The terminal residues of fluopyram in cowpea increased with the frequency of spraying and application rate. In terms of spatial distribution, fluopyram residues were higher in the lower cowpea than in the middle and upper layers, with the least residues in the upper layer. This was similar to the findings following the application of boscalid and pyraclostrobine in grape fields (Chen et al., 2019). In China and EU, the MRL for fluopyram in cowpea (with pods) are 1 mg/kg and 3 mg/kg. Residue levels at 5, 7, and 10 days after fluopyram application were below the MRLs (China and EU). This indicates that cowpea is relatively safe to consume for 5 days after the application of fluopyram at the recommended dose.
3.4 Dietary risk assessment
Humans can gradually enrich pesticides through the living environment and food chain, leading to different hazards and diseases (Yang et al., 2020). Although most of the pesticides can be removed during food processing and cooking, the safety risk of pesticides is still not negligible (Liu et al., 2016). In this study, a risk assessment of cowpea fruit was carried out based on residue data from field trials.
The terminal residues of fluopyram in cowpea ranged from .031 to .596 mg/kg, with a STMP of .463 mg/kg and HR of .596 mg/kg. Based on the information reviewed, the ADI and ARfD were set at .012 and .5 mg/kg bw (PPDB: Pesticide Properties DataBase, 2022), respectively. The calculated NEDI, RQ and NESTI are recorded in Tables 6, 7.
In the long-term dietary risk assessment, the RQ value for fluopyram was 55.33%, which is less than 100%, indicating that the long-term dietary risk of this pesticide is acceptable (Fan et al., 2019). As shown in Table 7. The short-term dietary risk of fluopyram in cowpea was calculated using NESTI and ranged from .78% to 2.84%, with %ARfD less than 100% for all age groups, with the highest risk for children aged 2–7 years (2.84%), but well below 100%, indicating that the short-term dietary risk of fluopyram in cowpea is extremely low.
4 Conclusion
In this study, an analytical method was developed for the analysis of fluopyram in nine different plant matrices. The method was further optimised based on the QuEChERS method, and the clean-up effects of seven purification agents were compared. Four purification agents were selected, among which the combination of PSA, C18 and MWCNTs-NH2 could meet the purification requirements well. The recoveries of the nine foodstuffs from 87.02% to 101.42% with relative standard deviations below 9.25%. Finally, a total of 19 positive samples out of 128 market samples were analyzed based on the method used in this study, all samples were below the MRL but one case of tomato was above the EU MRL. Field trial of fluopyram on cowpea was conducted, and the half-lives of fluopyram was 3.03–3.95 days and terminal residues ranged from .031–.596 mg/kg. Dietary risk assessment was performed on cowpea. This result indicated that the dietary risk of fluopyram in cowpeas was acceptable. The establishment of a method for the determination of fluopyram in different crops is beneficial to better monitor the residue levels of fluopyram, to protect the dietary safety of consumers and to provide technical means for subsequent study.
Data availability statement
The original contributions presented in the study are included in the article/Supplementary Material, further inquiries can be directed to the corresponding authors.
Author contributions
SR: Data curation and Writing—Original draft preparation. YZ: Data Curation. SZ: Validation and Writing-review and editing. HL: Validation and Investigation. XL: Methodology. LW: Writing-review and editing. MW: Supervision. CZ: Supervision and Funding acquisition.
Funding
This research was supported by National Natural Science Foundation of China Regional Joint Priority Project (U22A20484), the Key R&D projects in Hainan Province (ZDYF2022XDNY198), Key Laboratory of Tropical Fruits and Vegetables Quality and Safety for State Market Regulation (ZX-2022001), and the 2019 Hainan Basic and Applied Basic Research Plan (Natural Science) High-level Talents Project (2019RC058), and Innovative Research Projects for Postgraduates in Hainan Province in 2021 (Qhys 2021-27).
Conflict of interest
The authors declare that the research was conducted in the absence of any commercial or financial relationships that could be construed as a potential conflict of interest.
Publisher’s note
All claims expressed in this article are solely those of the authors and do not necessarily represent those of their affiliated organizations, or those of the publisher, the editors and the reviewers. Any product that may be evaluated in this article, or claim that may be made by its manufacturer, is not guaranteed or endorsed by the publisher.
Supplementary material
The Supplementary Material for this article can be found online at: https://www.frontiersin.org/articles/10.3389/fenvs.2023.1105524/full#supplementary-material
References
Chen, H., Wang, X., Liu, P., Jia, Q., Han, H., Jiang, C., et al. (2021). Determination of three typical metabolites of pyrethroid pesticides in tea using a modified QuEChERS sample preparation by ultra-high performance liquid chromatography tandem mass spectrometry. Foods 10, 189. doi:10.3390/foods10010189
Chen, H., Yin, P., Wang, Q., Jiang, Y., and Liu, X. (2014). A modified QuEChERS sample preparation method for the analysis of 70 pesticide residues in tea using gas chromatography-tandem mass spectrometry. Food Anal. Method. 7, 1577–1587. doi:10.1007/s12161-014-9791-0
Chen, S., Cai, L., Zhang, H., Zhang, Q., Song, J., Zhang, Z., et al. (2021). Deposition distribution, metabolism characteristics, and reduced application dose of difenoconazole in the open field and greenhouse pepper ecosystem. Agric. Ecosyst. Environ. 313, 107370. doi:10.1016/j.agee.2021.107370
Chen, X., He, S., Gao, Y., Ma, Y., Hu, J., and Liu, X. (2019). Dissipation behavior, residue distribution and dietary risk assessment of field-incurred boscalid and pyraclostrobin in grape and grape field soil via MWCNTs-based QuEChERS using an RRLC-QqQ-MS/MS technique. Food Chem. 274, 291–297. doi:10.1016/j.foodchem.2018.08.136
de Albuquerque, N. C. P., Carrão, D. B., Habenschus, M. D., and de Oliveira, A. R. M. (2018). Metabolism studies of chiral pesticides: A critical review. J. Pharm. Biomed. Anal. 147, 89–109. doi:10.1016/j.jpba.2017.08.011
Deng, F., Sun, J., Dou, R., Yu, X., Wei, Z., Yang, C., et al. (2020). Contamination of pyrethroids in agricultural soils from the yangtze river delta, China. Sci. Total Environ. 731, 139181. doi:10.1016/j.scitotenv.2020.139181
Dong, B., and Hu, J. (2014). Dissipation and residue determination of fluopyram and tebuconazole residues in watermelon and soil by GC-MS. Int. j. Environ. An. Ch. 94 (5), 493–505. doi:10.1080/03067319.2013.841152
Duan, Y., Guan, N., Li, P., Li, J., and Luo, J. (2016). Monitoring and dietary exposure assessment of pesticide residues in cowpea (Vigna unguiculata L. Walp) in Hainan, China. Food control. 59, 250–255. doi:10.1016/j.foodcont.2015.05.036
Duan, Y., Ramilan, T., Luo, J., French, N., and Guan, N. (2021). Risk assessment approaches for evaluating cumulative exposures to multiple pesticide residues in agro-products using seasonal vegetable monitoring data from hainan, China: A case study. Environ. Monit. Assess. 193, 578. doi:10.1007/s10661-021-09328-z
Dušek, M., Jandovská, V., Kalachová, K., and Olšovská, J. (2020). Comparative study of three sample preparation methods for multi-residue extraction of pesticide residues in hop samples. Food Anal. Method. 13, 503–515. doi:10.1007/s12161-019-01658-6
European Commission (2021). Analytical quality control and method validation procedures for pesticide residues analysis in food and feed. SANTE/11312/2021.
European Commission Pesticides database (2020). European commission pesticides database. Available at: https://ec.europa.eu/food/plant/pesticides/eu-pesticides-database/mrls/?event= download.MRL (Accessed on August 25, 2022).
Fan, X., Zhao, S., and Hu, J. (2019). Dissipation behavior and dietary risk assessment of lambda-cyhalothrin, thiamethoxam and its metabolite clothianidin in apple after open field application. Regul. Toxicol. Pharm. 101, 135–141. doi:10.1016/j.yrtph.2018.11.003
Farha, W., Abd El-Aty, A. M., Rahman, M. M., Shin, H., and Shim, J. (2016). An overview on common aspects influencing the dissipation pattern of pesticides: A review. Environ. Monit. Assess. 188, 693. doi:10.1007/s10661-016-5709-1
Fu, D., Zhang, S., Wang, M., Liang, X., Xie, Y., Zhang, Y., et al. (2020). Dissipation behavior, residue distribution and dietary risk assessment of cyromazine, acetamiprid and their mixture in cowpea and cowpea field soil. J. Sci. Food Agr. 100, 4540–4548. doi:10.1002/jsfa.10495
Gabardo, R. P., Toyama, N. P., Amaral, B. D., Boroski, M., Toci, A. T., Benassi, S. F., et al. (2021). Determination of atrazine and main metabolites in natural waters based on a simple method of QuEChERS and liquid chromatography coupled to a diode-array detector. Microchem. J. 168, 106392. doi:10.1016/j.microc.2021.106392
Gonzalez-Curbelo, M. A., Herrera-Herrera, A. V., Hernandez-Borges, J., and Rodriguez-Delgado, M. A. (2013). Analysis of pesticides residues in environmental water samples using multiwalled carbon nanotubes dispersive solid-phase extraction. J. Sep. Sci. 36, 556–563. doi:10.1002/jssc.201200782
Han, C., Hu, B., Li, Z., Liu, C., Wang, N., Fu, C., et al. (2021). Determination of fipronil and four metabolites in foodstuffs of animal Origin using a modified QuEChERS method and GC–NCI–MS/MS. Food Anal. Method. 14, 237–249. doi:10.1007/s12161-020-01872-7
He, M., Xie, L., Wu, Y., Hong, M., Jiang, Y., and Li, T. (2022). Fluopyram inhibits tricarboxylic acid cycle but induces γ-aminobutyric acid-shunt pathway to prolong postharvest shelf life of litchi fruit. Postharvest Biol. tec. 192, 112036. doi:10.1016/j.postharvbio.2022.112036
Hotchkiss, J. H. C. U. (1992). Pesticide residue controls to ensure food safety. Crit. Rev. Food Sci.Crit. Rev. Food Sci. 31 (3), 191–203. doi:10.1080/10408399209527568
Huang, Y., Xiao, L., Li, F., Xiao, M., Lin, D., Long, X., et al. (2018). Microbial degradation of pesticide residues and an emphasis on the degradation of cypermethrin and 3-phenoxy benzoic acid: A review. Molecules 23 (9), 2313. doi:10.3390/molecules23092313
Institute of Quality Standards and Testing Technology for Agro-productsChinese Academy of Agricultural (2007). Risk assessment for quality and safety of agro-foods: Principles, methodologies and applications. Beijing: Standards Press of China.
Ji, X., Li, J., Dong, B., Zhang, H., Zhang, S., and Qiao, K. (2019). Evaluation of fluopyram for southern root-knot nematode management in tomato production in China. Crop Prot. 122, 84–89. doi:10.1016/j.cropro.2019.04.028
Kwon, J., and Armbrust, K. L. (2006). Degradation of chlorothalonil in irradiated water/sediment systems. J. Agric. Food Chem. 54 (10), 3651–3657. doi:10.1021/jf052847q
Li, L., Yin, Y., Zheng, G., Liu, S., Zhao, C., Xie, W., et al. (2021). Determination of multiclass herbicides in sediments and aquatic products using QuECHERS combined with ultra-high performance liquid chromatography-tandem mass spectrometry (UHPLC-MS/MS) and its application to risk assessment of rice-fish co-culture system in China. Microchem. J. 170, 106628. doi:10.1016/j.microc.2021.106628
Liu, T., Zhang, C., Peng, J., Zhang, Z., Sun, X., Xiao, H., et al. (2016). Residual behaviors of six pesticides in shiitake from cultivation to postharvest drying process and risk assessment. J. Agr. Food Chem. 64, 8977–8985. doi:10.1021/acs.jafc.6b04027
Liu, W., Su, Y., Liu, J., Zhang, K., Wang, X., Chen, Y., et al. (2021). Determination of cyflufenamid residues in 12 foodstuffs by QuEChERS-HPLC-MS/MS. Food Chem. 362, 130148. doi:10.1016/j.foodchem.2021.130148
Ly, T., Ho, T., Behra, P., and Nhu-Trang, T. (2020). Determination of 400 pesticide residues in green tea leaves by UPLC-MS/MS and GC-MS/MS combined with QuEChERS extraction and mixed-mode SPE clean-up method. Food Chem. 326, 126928. doi:10.1016/j.foodchem.2020.126928
Maragou, N. C., Thomaidis, N. S., Theodoridis, G. A., Lampi, E. N., and Koupparis, M. A. (2020). Determination of bisphenol A in canned food by microwave assisted extraction, molecularly imprinted polymer-solid phase extraction and liquid chromatography-mass spectrometry. J. Chromatogr. B 1137, 121938. doi:10.1016/j.jchromb.2019.121938
Mohapatra, S., Siddamallaiah, L., Buddidathi, R., and Yogendraiah Matadha, N. (2018). Dissipation kinetics and risk assessment of fluopyram and tebuconazole in mango (Mangifera indica). Int. J. Environ. An. Ch. 98, 229–246. doi:10.1080/03067319.2018.1445244
Mukherjee, I., Das, S. K., and Kumar, A. (2018). Atmospheric CO2 level and temperature affect degradation of pretilachlor and butachlor in Indian soil. Bull. Environ. Contam. Toxicol. 100 (6), 856–861. doi:10.1007/s00128-018-2340-6
Narenderan, S. T., Meyyanathan, S. N., and Babu, B. (2020). Review of pesticide residue analysis in fruits and vegetables. Pre-treatment, extraction and detection techniques. Food Res. Int. 133, 109141. doi:10.1016/j.foodres.2020.109141
National Health and Family Planning Commission (2021). National Health and Family Planning Commission and Ministry of Agriculture of the People's Republic of China, China national food safety standard-maximum residue limits for pesticides in food (GB 2763-2021).
Pallavi, M. S., Harischandra, N. R., RatnammaNidoni, U., Bheemanna, M., and Pramesh, D. (2021). Simultaneous determination, dissipation and decontamination of fungicides applied on cabbage using LC-MS/MS. Food Chem. 355, 129523. doi:10.1016/j.foodchem.2021.129523
PPDB: Pesticide Properties DataBase (2022). Fluopyram. Available at: https://sitem.herts.ac.uk/aeru/ppdb/en/Reports/1362.htm (Accessed on August 25, 2022).
Proffer, T. J., Lizotte, E., Rothwell, N. L., and Sundin, G. W. (2013). Evaluation of dodine, fluopyram and penthiopyrad for the management of leaf spot and powdery mildew of tart cherry, and fungicide sensitivity screening of Michigan populations of Blumeriella jaapii. Pest. Manag. Sci. 69 (6), 747–754. doi:10.1002/ps.3434
Rouquié, D., Tinwell, H., Blanck, O., Schorsch, F., Geter, D., Wason, S., et al. (2014). Thyroid tumor formation in the male mouse induced by fluopyram is mediated by activation of hepatic CAR/PXR nuclear receptors. Regul. Toxicol. Pharm. 70, 673–680. doi:10.1016/j.yrtph.2014.10.003
Sakthiselvi, T., Paramasivam, M., Vasanthi, D., and Bhuvaneswari, K. (2020). Persistence, dietary and ecological risk assessment of indoxacarb residue in/on tomato and soil using GC–MS. Food Chem. 328, 127134. doi:10.1016/j.foodchem.2020.127134
Tong, Z., Sun, M., Zhou, Z., Dong, X., Hu, B., and Duan, J. (2021). The fate and effect of chlorpyrifos and lambda-cyhalothrin in soybean (Glycine max L. Merril) field. Ecotox. Environ. Safe. 209, 111861. doi:10.1016/j.ecoenv.2020.111861
Vargas Pérez, M., Egea González, F. J., and Garrido Frenich, A. (2020). Dissipation and residue determination of fluopyram and its metabolites in greenhouse crops. J. Sci. Food Agr. 100, 4826–4833. doi:10.1002/jsfa.10542
Vitale, A., Panebianco, A., and Polizzi, G. (2016). Baseline sensitivity and efficacy of fluopyram againstBotrytis cinerea from table grape in Italy. Ann. Appl. Biol. 169 (1), 36–45. doi:10.1111/aab.12277
Walorczyk, S. (2014). Validation and use of a QuEChERS-based gas chromatographic–tandem mass spectrometric method for multiresidue pesticide analysis in blackcurrants including studies of matrix effects and estimation of measurement uncertainty. Talanta 120, 106–113. doi:10.1016/j.talanta.2013.11.087
Wang, F., Li, S., Feng, H., Yang, Y., Xiao, B., and Chen, D. (2019). An enhanced sensitivity and cleanup strategy for the nontargeted screening and targeted determination of pesticides in tea using modified dispersive solid-phase extraction and cold-induced acetonitrile aqueous two-phase systems coupled with liquid chromatography-high resolution mass spectrometry. Food Chem. 275, 530–538. doi:10.1016/j.foodchem.2018.09.142
Wang, S., Sun, H., Liu, Y., Wang, X., and Chang, H. (2022). The disappearance behavior, residue distribution, and risk assessment of kresoxim-methyl in banana (musanana lour.) based on a modified QuEChERS procedure using HPLC-MS/MS. Front. Environ. Sci. 10. doi:10.3389/fenvs.2022.853033
Yang, J., Luo, F., Zhou, L., Sun, H., Yu, H., Wang, X., et al. (2020). Residue reduction and risk evaluation of chlorfenapyr residue in tea planting, tea processing, and tea brewing. Sci. Total Environ. 738, 139613. doi:10.1016/j.scitotenv.2020.139613
Yogendraiah Matadha, N., Mohapatra, S., and Siddamallaiah, L. (2021). Distribution of fluopyram and tebuconazole in pomegranate tissues and their risk assessment. Food Chem. 358, 129909. doi:10.1016/j.foodchem.2021.129909
Yu, C., Hao, D., Chu, Q., Wang, T., Liu, S., Lan, T., et al. (2020). A one adsorbent QuEChERS method coupled with LC-MS/MS for simultaneous determination of 10 organophosphorus pesticide residues in tea. Food Chem. 321, 126657. doi:10.1016/j.foodchem.2020.126657
Yuan, S., Yang, F., Yu, H., Xie, Y., Guo, Y., and Yao, W. (2021). Biodegradation of the organophosphate dimethoate by Lactobacillus plantarum during milk fermentation. Food Chem. 360, 130042. doi:10.1016/j.foodchem.2021.130042
Zaidon, S. Z., Ho, Y. B., Hamsan, H., Hashim, Z., Saari, N., and Praveena, S. M. (2019). Improved QuEChERS and solid phase extraction for multi-residue analysis of pesticides in paddy soil and water using ultra-high performance liquid chromatography tandem mass spectrometry. Microchem. J. 145, 614–621. doi:10.1016/j.microc.2018.11.025
Zhang, Y., Xu, J., Dong, F., Liu, X., Wu, X., and Zheng, Y. (2014). Response of microbial community to a new fungicide fluopyram in the silty-loam agricultural soil. Environ. Safe. 108, 273–280. doi:10.1016/j.ecoenv.2014.07.018
Keywords: GC-MS/MS, fluopyram, adsorbent, QuEChERS, risk assessment
Citation: Ren S, Zhang Y, Zhang S, Lu H, Liang X, Wang L, Wang M and Zhang C (2023) Residue behavior and dietary risk assessment of fluopyram in cowpea and determination in nine foodstuffs. Front. Environ. Sci. 11:1105524. doi: 10.3389/fenvs.2023.1105524
Received: 22 November 2022; Accepted: 04 January 2023;
Published: 16 January 2023.
Edited by:
Sudhakar Srivastava, Banaras Hindu University, IndiaReviewed by:
Canping Pan, China Agricultural University, ChinaShuying Li, Zhejiang University, China
Copyright © 2023 Ren, Zhang, Zhang, Lu, Liang, Wang, Wang and Zhang. This is an open-access article distributed under the terms of the Creative Commons Attribution License (CC BY). The use, distribution or reproduction in other forums is permitted, provided the original author(s) and the copyright owner(s) are credited and that the original publication in this journal is cited, in accordance with accepted academic practice. No use, distribution or reproduction is permitted which does not comply with these terms.
*Correspondence: Meng Wang, wangmeng@hainanu.edu.cn; Chenghui Zhang, zchlm@hainanu.edu.cn
†These authors have contributed equally to this work